- 1Department of Child and Adolescent Psychiatry, Psychotherapy, and Psychosomatics, Albert-Ludwigs-University Freiburg, Freiburg, Germany
- 2Department of Psychiatry and Psychotherapy, Philipps-University, Marburg, Germany
Objectives: Psychiatric disorders, such as schizophrenia and other neuroinflammatory diseases are accompanied by an increase in the oxidative stress and changes in the immune system and in the metabolic, hormonal and neurological components of the central nervous system (CNS). Hydrogen sulfide (H2S) is a gaseous molecule that is endogenously produced in the peripheral and central nervous system through cysteine by the following major H2S producing enzymes in the brain: cystathionine-γlyase (CSE), cystathionine ß-synthase (CBS) and 3-mercaptopyruvate sulfurtransferase (MPST). The physiological effects of H2S are broad, with antioxidative properties being a major role in the body. The aims of our investigation were to analyze the central nervous antioxidant, metabolic and neuronal effects in the hippocampus of the rat after inflammatory peripheral lipopolysaccharide (LPS) treatment; and to examine the effects of antipsychotics on the expression of these enzymes in human cell lines.
Material and Methods: Male Lewis rats (250 g) received an i.p. LPS injection (1 mg/kg) 24 h before microdialysis experiments. Conscious rats were infused via these probes (1.5 μl/min) with a radical scavenger 1-hydroxy-3-methoxycarbonyl-2,2,5,5-tetramethylpyrrolidine (CMH) in Krebs-Ringer solution. Sodiumhydrogensulfide (NaHS, 10 μg/min) was infused after a 2- h baseline for 1 h. Corticosterone, glutamate, glucose and lactate were measured by Elisa. Reactive oxygen species (ROS) were detected by electron spin resonance spectroscopy (ESR). The impact of the antipsychotics haloperidol, clozapine, olanzapine and risperidone on the expression of genes encoding the key enzymes of H2S synthesis was studied at the human neuroblastoma SH-SY5Y and monocytic U-937 cell lines. The cells were incubated for 24 h with 30 μM antipsychotic following which mRNA levels were measured by polymerase chain reaction.
Results: Microdialysate glucose and lactate levels dramatically increased in the hippocampus of LPS untreated rats by local application of NaHS. By contrast, in the LPS pretreated rats, there was no effect of NaHS infusion on glucose but a further significant increase in microdialysate lactate was found. It was LPS pretreatment alone that particularly enhanced lactate levels. There was a marked increase in hippocampal microdialysate glutamate levels after local NaHS infusion in LPS untreated animals. In LPS treated rats, no change was observed by NaHS, but LPS itself had the strongest effect on microdialysate glutamate levels. Microdialysate corticosterone levels were reduced by NaHS in both LPS pretreated and untreated rats. The formation of free radicals in the hippocampus significantly reduced in LPS pretreated rats, while in LPS untreated rats a significant increase was observed after NaHS infusion. In human SH-SY5Y and U-937 cells, all three major enzymes of H2S-Synthesis, namely cystathionine-γ-lyase, cystathione ß-synthase and 3-mercaptopyruvate sulfurtransferase, could be detected by PCR. The antipsychotics haloperidol, clozapine, olanzapine and risperidone affected all three enzymes in different ways; with haloperidol and risperidone showing major effects that led to reductions in CBS or CSE expression.
Discussion: The local application of NaHS in the hippocampus of the rat strongly affected glucose, lactate and glutamate release. Contrastingly, in LPS pretreated rats, a decreased radical formation was the only effect found. H2S synthetizing enzymes may be involved in antipsychotic mechanisms, although no clear common mechanism could be found.
Introduction
Psychiatric disorders, such as schizophrenia and other neuroinflammatory diseases are accompanied by an increased oxidative stress, changes in the immune system and in metabolic, hormonal and neurological components of the central nervous system (1–22). More and more evidence suggests various dysregulations of the hypothalamus-pituitary-adrenal (HPA)-Axis in the course of numerous mental disorders, such as affective disorders (23–25), and schizophrenia (18–21).
The response to antipsychotics in schizophrenia shows a high variability. There are several patient cases that are resistant to clozapine, which is called “ultra-resistance to treatments in schizophrenia” (UTRS). Peripheral inflammations are associated with UTRS (26).
Hydrogen sulfide (H2S) is a gaseous molecule that is endogenously and enzymatically produced in the peripheral and central nervous system by three major H2S producing enzymes: cystathionine-γ-lyase (CSE) (27), cystathionine ß-synthase (CBS) (28) and 3-mercaptopyruvate sulfurtransferase (MPST) (29, 30); besides other mechanisms (31). High endogenous concentrations of H2S were found in the hippocampus and cerebellum which are parts of the human brain where CBS seems to be the most important enzyme for the synthesis (32, 33).
H2S is known to regulate a multitude of physiological and pathophysiological functions in the vascular, immune and nervous system [for review see (34, 35)]. There is evidence for a role of H2S in neurodegeneration (36), but also radical scavenging effects regarding nitric oxide (37) or glutamate mediated oxidative stress (38). Other authors report a role for H2S in Alzheimer's disease (39) or Parkinson's disease (40).
Promising studies have demonstrated the therapeutic effect of H2S donation in various disease models of cancer, inflammation or neuroinflammation (41, 42). Additionally, the pharmacological inhibition of H2S production (43–47) or the genetic deficiency of H2S producing enzymes (48–50) results in beneficial effects.
Xiong et al. could show that the H2S levels of patients with schizophrenia are significantly low. H2S is a regulator for the N-methyl-D-aspartate receptor (NMDAR) function and low H2S levels can cause a hypofunction of NMDAR. Given that a hypofunction of the NMDAR receptor is related to the pathogenesis of this disorder, H2S levels in the liquor are also likely to have an effect on the pathophysiology of this disorder (51).
The aims of our investigation were to analyze antioxidant, metabolic, hormonal and neuronal changes of NaHS application in the hippocampus in naive and LPS pretreated rats. Furthermore, we aim to show the effects of different antipsychotics on the expression of several enzymes related with the H2S synthesis in a neuronal and an immunological human cell lines. Especially the role of exogenous H2S as a possible therapeutic mediator in inflammatory and neurodegenerative diseases will be analyzed.
Materials and Methods
Animals
Animal experiments were carried out at the Department of Surgical Research, University Hospital, Freiburg, Germany. All procedures were performed in accordance with the German animal protection law, FELASA, the national animal welfare body, GV-SOLAS and the NIH guide for the care and use of laboratory animals; and were approved by the animal welfare committee of the University of Freiburg (AZ: G-10/44). Male Lewis rats (250–350 g; Charles River, Germany) were housed at a temperature of 21° C in plastic cages with lights turned on from 06:00 to 19:00 h and with free access to food and water. Experiments were conducted during the light phase after at least 1 week of adaptation.
In vivo Microdialysis Experimental Design
CMA/12 microdialysis probes were implanted under isoflurane anesthesia (3%) using stereotaxic coordinates according to (52) A: +5.2 mm; L: +2.0 mm; V: −4.4 mm from Cortex top (53). The microdialysis experiments started after awakening using the CMA freely moving system in groups of 8 animals. Microdialysis samples were collected every 30 min for a period of 4 h at a constant flow rate of 1.5 μL/min (Krebs-Ringer). Microdialysis samples were collected and stored at −20°C until analysis. Electron spin resonance (ESR) measurements were performed immediately. The same effluents were used to measure Corticosterone (Enzyme immunoassay, IBL, Hamburg, Germany), Glucose, Lactate and Glutamate (Colorimetric Assays, BioCat, Heidelberg, Germany).
To detect central nervous protective effects of hydrogen sulfide, a series of rats received an intraperitoneal injection of 1 mg/kg body weight Lipopolysaccharide from E. coli (LPS; Sigma Aldrich Chemicals, Steinheim, Germany). The injections were made 24 h before microdialysis probes were implanted. NaHS was infused intrahippocampal via the microdialysis cannula at a flowrate and dose of 10 μg/min 2 h after beginning of sampling. ROS, Glutamate, Corticosterone, Lactate and Glucose-Uptake (difference between glucose inflow concentration and outflow concentration) were measured.
Histology
At the end of the experiment, one group of animals was euthanized with CO2. To verify probe placement, the brain was removed and stored at −20°C. Subsequently, serial coronal brain sections (thickness: 20 μm) were cut on a freezing microtome at −16°C and sections were stained with a 0.5% cresyl violet solution.
Chemicals
Krebs-Ringer's solution was obtained from Delta-Select, Pfullingen, Germany; LPS (Lipopolysaccharides from Escherichia coli, Serotype 0127:B8 purified by trichloroacetic acid extraction) from Sigma, Steinheim, Germany; 1-hydroxy-3-methoxycarbonyl-2,2,5,5-tetramethylpyrrolidine (CMH) from Noxygen, Elzach, Germany; Isofluorane from Abbott, Wiesbaden, Germany; Primers were obtained from MWG Biotech, Ebersberg, Germany.
ROS Measurements
Hippocampal detection of reactive oxygen species is based on the reaction of CMH and ROS as reactant in dialysates. CMH solution (1 mg/ml Krebs-Ringer) was prepared fresh every day. The spin probe CMH was applied by infusion via a microdialysis cannula at a flow rate of 1.5 μl/min. The oxidation of spin probe CMH by reactive oxygen species generates stable 3-methoxycarbonyl-proxyl radicals (CM) as shown by Dikalov et al. (54, 55). Autoxidation was found to be in the range of 1–2%. The amount of CM. radical is equivalent to the formation of reactive oxygen species in vivo. The amount of reacted ROS was determined from the ESR amplitude according to a calibration curve using standard CM. solutions. ESR measurements were performed at room temperature using an EMX ESR spectrometer (MiniScope MS 200, Magnettech, Berlin, Germany). The ESR had the following settings: center field g = 2.001, sweep wide 60 G, sweep time 5 ms over 10 scans, modulation amplitude 2.4 G, microwave power 20 mW. The total spin probe concentration was measured to determine the concentration of free radicals (56).
Cells and Culture Conditions
U-937 cells were maintained from the Roswell Park Memorial Institute (RPMI) 1640 medium supplemented with 10% heat-inactivated fetal calf serum (FCS) (Gibco/BRL and Seromed, Berlin, Germany), 0.5% glutamine, and 1% gentamycine at 37°C in a 5% CO2 atmosphere. For the studies, cells were plated at a concentration of 225,000 cells in 3 ml medium per well into six-well culture plates (Greiner, Frickenhausen, Germany).
Cells were preincubated for 24 h and then treated with the antipsychotics, dissolved in ethanol at a concentration of 30 μM. These were added to the plates at a quantity of 300 μl to each well. The same procedure was performed with the ethanol controls without antipsychotics. Antipsychotics were obtained from Sigma, Deisenhofen, Germany.
Neuroblastoma SH-SY5Y cells were cultured in heat-inactivated Roswell Park Memorial Institute medium (RPMI) (Gibco/BRL, Eggenstein, Germany) supplemented with 15% fetal calf serum (FCS) (Biochrom, Berlin, Germany), 1% penicillin-streptomycin and 1% glutamine in a 5% CO2 atmosphere. For further studies, cells were plated at a number of 225.000 cells/dish in 10 mm culture dishes. Antipsychotics and ethanol-control treatments were performed at a density of 450.000 cells/dish. The antipsychotics were dissolved in ethanol and were further diluted in culture medium. The cells were exposed to antipsychotics at 30 μM for 24 h at 37°C.
RNA Extraction
After incubation, the cells were collected from the culture dishes and total RNA was extracted by the use of Trizol® reagent in accordance with the manufacturer's instructions. The amount of extracted total RNA was quantified by established optical methods at A260/A280 (Genequant II, Pharmacia Biotech, Freiburg, Germany) and structural integrity checked by agarose-gel electrophoresis [1.5% agarose (Gibco/BRL)].
Reverse Transcriptase-Polymerase Chain Reaction (RT-PCR)
RT-PCR was used to analyse the transcription of the GRs and the house-keeping genes glyceraldehyde-3-phosphate dehydrogenase (GAPDH). 1 mg of cellular total RNA was reverse-transcribed with 40 U of Superscript II (Gibco/BRL) and 1 mg oligo-(dT) in a volume of 20 ml following the manufacturer's protocol.
The following primer pairs were used to amplify the cDNA's:
CBS: Sense 5′-CGATGGGTACCATATGCAGAAAAGACGCCTCCTCACAAGG-3′, Antisense 5′-CGGTACCTCGAGTTACTACTGTGATTCCACTTGGAGGGTGTGCTGCC-3′. CSE: Sense 5′-GGCCTGAAGTGTGAGCTCTT-3′, Antisense 5′-TTGGGGATTTCGTTCTTCAG-3′. MPST: Sense 5′-GACCCCGCCTTCATCAAG-3′, Antisense 5′-CATGTACCACTCCACCCA-3′. GAPDH: Sense 5′-CGTCTTCACCACCATGGAGA-3′, Antisense 5′-CGGCCATCACGCCACAGTTT-3′.
Aliquots of 1 ml cDNA were amplified with a PCR cycler (Biometra Trio, Göttingen, Germany) for the enzymes and GAPDH using the primers described above with the following cycling program: denaturation for 45 s at 95°C, annealing for 60 s at 59°C, and extension for 60 s at 72°C. PCR products were analyzed for all enzymes after amplification with 28 cycles and gel electrophoresis in 1.5% agarose gels. Semi-quantitative determination was achieved by digitization of gels with a Polaroid video system (Rothaar & Schroeder, Heidelberg, Germany) and further densitometric evaluation achieved with the Gelscan 4.0 Professional Program (LTF/BioSciTec, Landau/Frankfurt, Germany).
Quantification of mRNA by RT-PCR and Densitometry
Different approaches were used to minimize variations and to ensure the reliability of the quantification procedure: first, the purity of mRNA probes was determined by measuring the optical density at A260/A280. This revealed a ratio of 1.56 ± 0.05 for all extractable probes. Second, integrity and amounts of mRNA measured were checked by gel electrophoresis. Third, relevant impurities of DNA were routinely excluded by PCR-amplification of extracted mRNA probes after omission of the RT reaction. The reliability of the further steps of quantification (PCR reaction, gel densitometry and evaluation of results) including non-saturating conditions of the PCR was determined using different amounts of RT products. This yielded a near-linear dose-product relationship in the gel electrophoresis.
Statistical Analyses
The statistical analysis of the microdialysis data was performed using Microsoft Excel 8.0 and SPSS version 9.0 (SPSS Inc., Chicago, USA). The given data comprises mean ± standard deviation (SD). For microdialysis experiments significance was assessed by unpaired Student t-test, one-tailed (since we had a clear hypothesis from the previous in-vitro studies). An α-level of p < 0.05 was considered significant (57). Data are presented in percent of the mean of the respective control samples and are shown as mean ± SD values. The results from at least three independent different experiments were pooled and analyzed by Mann-Whitney Rank Sum tests. A p < 0.05 (*) was accepted as a statistically significant difference. For statistical evaluations, Sigmastat (Jandel Scientific, Kerpenich, Germany) was applied (58).
Results
The local intrahippocampal application of NaHS via microdialysis cannula (10 μg/min. at 1.5 ml/min. for 1 h) affected different neuronal parameters. In LPS untreated rats, NaHS infusion exerted massive increases in both microdialysis glucose and lactate release in the hippocampus. These effects were not seen in LPS treated rats (1 mg/kg i.p. 24 h before microdialysis experiments), Figure 1.
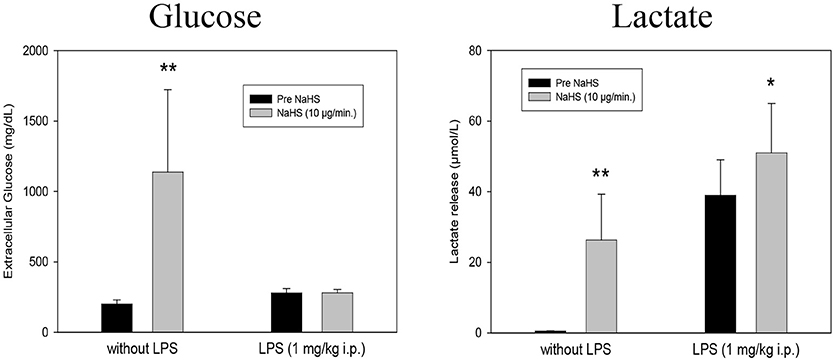
Figure 1. Effect of intrahippocampal NaHS infusion (10 μg/min) via CMA/12 micordialysis cannula on Glucose uptake (Left) and Lactate release (Right) with and without LPS treatment (1 mg/kg i.p., 24 h before microdialysis, LPS) as compared to control. Means ± SD, n = 8, *p < 0.05; **p < 0.01, Student t-test.
Microdialysis monitoring of Glutamate and Corticosterone showed a highly significant increase in Glutamate and a diminished formation of Corticosterone after 10 μg/min NaHS compared to the previous control periods. In the LPS treated rats no further increase in Glutamate could be observed. The decreasing effect of NaHS on Corticosterone was also observed in LPS treated animals (Figure 2).
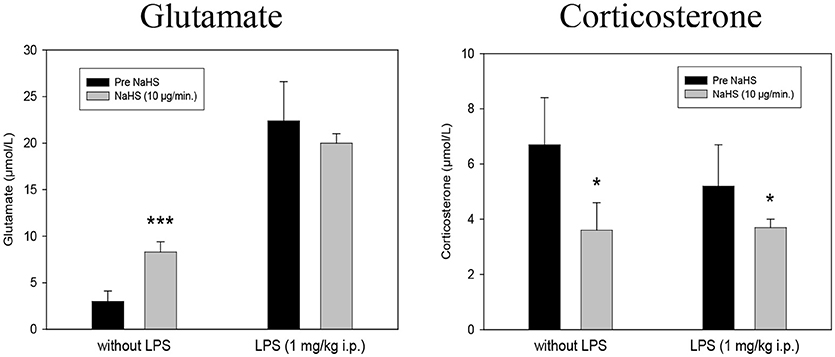
Figure 2. Effect of intrahippocampal NaHS infusion (10 μg/min) via CMA/12 micordialysis cannula on Glutamate release (Left) and Corticosterone release (Right) with and without LPS treatment (1 mg/kg i.p., 24 h before microdialysis, LPS) as compared to control. Means ± SD, n = 8, *p < 0.05; ***p < 0.001, Student t-test.
ROS are also affected significantly by NaHS (Figure 3). The infusion of NaHS, 10 μg/min, led to a significant increase in free radical formation in LPS-untreated animals. In contrary, in LPS treated animals we saw a free radical decrease of NaHS.
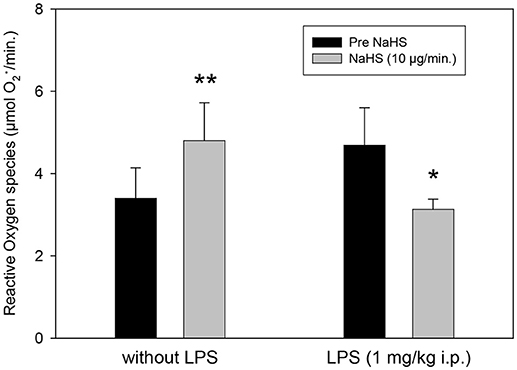
Figure 3. Effect of intrahippocampal NaHS infusion (10 μg/min) via CMA/12 micordialysis cannula on ROS production (as measured by CM.) with and without LPS treatment (1 mg/kg i.p., 24 h before microdialysis, LPS) as compared to control. Means ± SD, n = 8, *p < 0.05; **p < 0.01, Student t-test.
Our present study demonstrated effects of typical (haloperidol) as well as atypical antipsychotics (clozapine, olanzapine, risperidone) on the main H2S synthesizing enzymes CBS, CBE, and MPST in SH-SY5Y cells, and U-937 cells (Figure 4). In essence, all measured significant effects of the used antipsychotics in the employed concentration were reductions in the expression of both enzymes CBS and CSE in the neuronal cell line. Haloperidol significantly reduced the expression of CBS and CSE in SH-SY5Y cells, but the effect of haloperidol was not significant in U-937 cells. In U-937 cells the antipsychotics olanzapine and risperidone reduced the m-RNA of CSE significantly. The expression of MPST was only affected in U-937 cells and only by olanzapine. Clozapine, the most important antipsychotic did not show any effect in both cell lines.
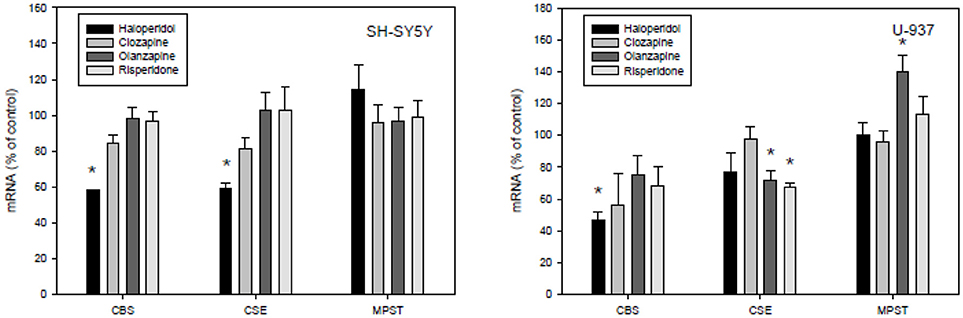
Figure 4. Effects of antipsychotic treatment on cystathione-y-lyase (CSE), cystathione ß-synthase (CBS), and 3-mercaptopyruvate sulfurtransferase (3MST) in human SH-SY5Y and U-937 cell lines. Means ± SD, n = 8, *p < 0.05; Mann-Whitney Rank Sum test.
Discussion
Our data indicate that H2S affects parameters involved in the pathophysiology of inflammatory psychiatric illnesses, such as depression and schizophrenia. Especially in normal Lewis rats, extracellular glucose, lactate release and glutamate release are highly increased in the hippocampus after local application of NaHS via microdialysis cannula. Hippocampal corticosterone levels are found to be significantly reduced under these conditions. At this concentration, an increase in free radical formation could also be observed, whereas after LPS pretreatment of animals a significant reduction could be observed. Under the conditions of LPS pretreatment the effects of NaHS on glutamate, corticosterone, glucose, and lactate release were missing. Antipsychotics seem to have individual effects on the expression of the major H2S-synthetizing enzymes with haloperidol exerting mostly reducing effects, while clozapine was ineffective in both SH-SY5Y and U-937 cell lines.
Hayden et al. (59) have also shown elevated blood glucose levels after exposure with H2S. Pichette and Gagnon summarize the literature on the effects of H2S and glucose regulation, indicating that mechanisms besides insulin regulation, such as glucagon-like-peptide (GLP)-1 or peptide YY are involved (60). Our data confirm findings of Lin et al. (61) that exogenous H2S protects cells by its glucose reducing activity.
The increase in lactate by NaHS-Application may be supported by the observation that in fish exposed to H2S, an increase in whole blood lactate could be observed (62). Without LPS pretreatment, our results suggest that the local application of NaHS leads to a general metabolic activation of neurons and glial cells in the hippocampus.
Our findings of the significant release of glutamate in the hippocampus of the rat induced by local NaHS application might be due to postsynaptic effects of H2S on the membrane potential, and thus excitability of CNS neurons, as it has been reported in dorsal raphe (63), paraventricular nucleus (64), subfornical organs (65) and dorsal root ganglion neurons (66). The effects in the dorsal raphe have been suggested to be the result of direct modulatory actions of H2S on calcium dependent potassium channels (63).
Significant alterations in the HPA axis activity occur in course of the schizophrenia, regarding basal cortisol secretion, probably in response to a decrease in the amount of glucocorticoid receptors (25, 67, 68). Walder et al. (69) revealed a significant positive correlation between salivary cortisol concentrations and symptoms severity. A study of Walker et al. (70) revealed that increased cortisol levels in patients who developed psychosis. Therefore, the hypothesis that HPA axis distortion is closely bound with symptoms and pathophysiology of the schizophrenia is becoming increasingly recognized. Antipsychotic medication leads to the reduction of cortisol concentrations in patients as well as in healthy controls (71, 72). Flores et al. (73) suggested that this might be responsible for their effectivity (17). Wang et al. ( 74) showed that inhibitors of the H2S producing enzymes, such as CBS or CSE, or the application of small interfering RNAs lead to mitochondrial oxidative stress and dysfunction, resulting in an even blunted corticosterone response to ACTH in adrenal glands. These effects were significantly attenuated by the treatment of H2S donor GYY4137. As shown by Navarra et al. (75), NaHS application is associated with the inhibition of the stimulated release of corticotropin-releasing hormone from rat hypothalamic explants.
In our experiments we found a significant reduction on free radical production in the hippocampus induced by the local NaHS application in the LPS pretreated rats. These findings support the role of H2S as an important antioxidative anti-inflammatory mediator. H2S was found to increase the antioxidative properties of cells in different ways (76). It decreases lipid peroxidation induced by homocysteine (77). H2S is able to directly react with reactive oxygen but its endogenous concentrations are too low to act as an important endogenous antioxidant. On the other hand H2S has a cytoprotective effect in brain cells by elevating the reduced glutathione (GSH) production via activation of cystine/cysteine transporters and redistribution of GSH to mitochondria (38, 78, 79). On the molecular level, several mechanisms seem to be involved in the antioxidant and neuroprotective role of H2S, such as the nuclear factor (NF)-κB pathway (80). H2S significantly reduced levels of malondialdehyde and 4-hydroxynonenal and elevated levels of superoxide dismutase and reduced glutathione in the hippocampus of streptozotocin (STZ)-induced diabetic rats (81). They also found that H2S alleviated depressive-like behaviors of STZ-induced diabetic rats in the forced swimming and tail suspension tests and reduced their anxiety-like behaviors in the elevated plus maze test. The results provide evidence for antidepressant-like and anxiolytic-like effects of H2S in STZ-induced diabetic rats and suggest that the therapeutic effects may result from inhibition of hippocampal oxidative stress (81). In HT22 neuronal cells, Kimura et al. (82) observed a cytoprotective role of H2S by activating ATP-dependent K+ (KATP) and Cl- channels, in addition to increasing the levels of glutathione. In LPS-treated mice the findings of (48) indicated that a deficiency in MPST does not significantly affect endotoxemia but a deficiency in CBS or CSE slightly ameliorates the outcome of LPS-induced endotoxemia in vivo.
In summary our data support Huang and Moore's (83) notion, that H2S is not only a toxic agent but also a gasotransmitter with growing therapeutical potential. Here we could demonstrate that it might be a double sided sword and that the body condition is important for the effects of H2S.
We here observed that the neuroleptics haloperidol, clozapine, olanzapine and risperidone have different effects on human SH-SY5Y and U-937 cell lines. Several drugs or even vitamins, such as vitamin D3 (84) are able to change the concentrations of H2S and these effects seem to be tissue dependent (35). The mechanisms through which these effects occur are still unclear. The regulation of gene expression seems to be different in respect to tissue and cell type since antipsychotics, such as haloperidol and quetiapine seem to reduce genes encoding antioxidant enzyme expression (58), the reduction of H2S-forming enzymes, CBS and CSE, especially by haloperidol in neuronal cell line. It cannot be excluded that the inhibition of CSE in U-937 cells by olanzapine and risperidone is combined not only with beneficial effects of these drugs but also with shared side effects. Furthermore, Fond et al. (26) recently found that ultra-resistance to treatment in schizophrenia (URTS) is independently associated with peripheral low-grade inflammation in schizophrenia patients (26).
H2S does not seem to be a classical neurotransmitter as specific receptors are not reported until now. Changes in cytokine production, such as IL-1 or TNF-a in the LPS model are reported (85). Although several mechanisms report that H2S can exert its physiological effects, such as sulfhydration or hemeprotein interactions [for review see (31)], other mechanisms, such as the influence on receptor heterocomplexes that are important in the pathophysiology of schizophrenia cannot be excluded from consideration (86, 87).
Schizophrenic Patients have olfactory impairments as was shown by Turetsky et al. (88) and show higher depolarization responses after stimulation with H2S indicating a special role for H2S in schizophrenia. Nevertheless, it is still unclear whether there is avoidance or an even beneficial effect (88).
Taken together our data confirm that hydrogen sulfide affects mechanisms involved in the pathophysiology of neuroinflammatory diseases, such as schizophrenia and depression and antipsychotic treatment might alter H2S-related mechanisms. We could show that H2S has effects on several brain metabolites and hormones, and our data confirm the idea that H2S can have toxic and protective properties depending on the state the body is in, here demonstrated for the case of inflammation. Nevertheless, the collected results of the study have not adequately clarified the therapeutic benefits H2S has for neurodegenerative diseases in brain, and the conditions and concentrations under which the beneficial properties H2S exceed the toxicity of this gasotransmitter. Further beneficial effects of H2S and related compounds might be studied in animal models of schizophrenia.
Author Contributions
All the authors meet the following criteria: made substantial contributions to the conception or design of the work; or the acquisition, analysis, or interpretation of data for the work; and drafted the work or revised it critically for important intellectual content; and approved the final the version to be published; and agreed to be accountable for all aspects of the work in ensuring that questions related to the accuracy or integrity of any part of the work are appropriately investigated and resolved. In addition OS performed the animal experiments and AS performed the cell culture experiments.
Conflict of Interest Statement
The authors declare that the research was conducted in the absence of any commercial or financial relationships that could be construed as a potential conflict of interest.
Acknowledgments
We thank S. Fischer for her technical assistance.
References
1. Müller N, Schwarz MJ. A psychoneuroimmunological perspective to Emil Kraepelins dichotomy: schizophrenia and major depression as inflammatory CNS disorders. Eur Arch Psychiatry Clin Neurosci. (2008) 258(Suppl. 2):97–106. doi: 10.1007/s00406-008-2012-3
2. Bechter K. Schizophrenia–a mild encephalitis? Fortschr Neurol Psychiatry (2013) 81:250–9. doi: 10.1055/s-0033-1335253
3. Busse M, Busse S, Myint AM, Gos T, Dobrowolny H, Müller UJ, et al. Decreased quinolinic acid in the hippocampus of depressive patients: evidence for local anti-inflammatory and neuroprotective responses? Eur Arch Psychiatry Clin Neurosci. (2015) 265:321–9. doi: 10.1007/s00406-014-0562-0
4. Perry BI, McIntosh G, Weich S, Singh S, Rees K. The association between first-episode psychosis and abnormal glycaemic control: systematic review and meta-analysis. Lancet Psychiatry (2016) 3:1049–58. doi: 10.1016/S2215-0366(16)30262-0
5. Nasrallah HA, Newcomer JW, Risinger R, Du Y, Zummo J, Bose A, et al. Effect of aripiprazole lauroxil on metabolic and endocrine profiles and related safety considerations among patients with acute schizophrenia. J Clin Psychiatry (2016) 77:1519–25. doi: 10.4088/JCP.15m10467
6. Huang YC, Lin PY, Lee Y, Wu CC, Hsu ST, Hung CF, et al. β-hydroxybutyrate, pyruvate and metabolic profiles in patients with schizophrenia: a case control study. Psychoneuroendocrinology (2016) 73:1–8. doi: 10.1016/j.psyneuen.2016.07.209
7. Reale M, Patruno A, De Lutiis MA, Pesce M, Felaco M, Di Giannantonio M, et al. Dysregulation of chemo-cytokine production in schizophrenic patients versus healthy controls. BMC Neurosci. (2011) 12:13. doi: 10.1186/1471-2202-12-13
8. Berk M, Copolov D, Dean O, Lu K, Jeavons S, Schapkaitz I, et al. N-acetyl cysteine as a glutathione precursor for schizophrenia-a double-blind, randomized, placebo-controlled trial. Biol Psychiatry (2008) 64:361–8. doi: 10.1016/j.biopsych.2008.03.004
9. Berk M, Copolov DL, Dean O, Lu K, Jeavons S, Schapkaitz I, et al. N-acetyl cysteine for depressive symptoms in bipolar disorder-a double-blind randomized placebo-controlled trial. Biol Psychiatry (2008) 64:468–75. doi: 10.1016/j.biopsych.2008.04.022
10. Brown GC. Mechanisms of inflammatory neurodegeneration: iNOS and NADPH oxidase. Biochem Soc Trans. (2007) 35:1119–921. doi: 10.1042/BST0351119
11. Chiang SS, Riedel M, Schwarz M, Mueller N. Is T-helper type 2 shift schizophrenia-specific? Primary results from a comparison of related psychiatric disorders and healthy controls. Psychiatry Clin Neurosci. (2013) 67:228–36. doi: 10.1111/pcn.12040
12. Brambilla D, Mancuso C, Scuderi MR, Bosco P, Cantarella G, Lempereur L, et al. The role of antioxidant supplement in immune system, neoplastic, and neurodegenerative disorders: a point of view for an assessment of the risk/benefit profile. Nutr J. (2008) 7:29. doi: 10.1186/1475-2891-7-29
13. da Silva Araújo T, Maia Chaves Filho AJ, Monte AS, Isabelle de Góis Queiroz A, Cordeiro RC, de Jesus Souza Machado M, et al. Reversal of schizophrenia-like symptoms and immune alterations in mice by immunomodulatory drugs. J Psychiatr Res. (2016) 84:49–58. doi: 10.1016/j.jpsychires.2016.09.017
14. Kim SY, Cohen BM, Chen X, Lukas SE, Shinn AK, Yuksel AC, et al. Redox dysregulation in schizophrenia revealed by in vivo NAD+/NADH measurement. Schizophr Bull. (2016) 43:197–204. doi: 10.1093/schbul/sbw129
15. Miller BJ, Goldsmith DR. Towards an immunophenotype of schizophrenia: progress, potential mechanisms, and future directions. Neuropsychopharmacology (2016) 42:299–317. doi: 10.1038/npp.2016.211
16. Galvez-Contreras AY, Campos-Ordonez T, Lopez-Virgen V, Gomez-Plascencia J, Ramos-Zuniga R, Gonzalez-Perez O. Growth factors as clinical biomarkers of prognosis and diagnosis in psychiatric disorders. Cytokine Growth Factor Rev. (2016) 32:85–96. doi: 10.1016/j.cytogfr.2016.08.004
17. Tobolska D, Wilczynski KM, Lorek M, Mazgaj E, Krysta K, Gawlik A, et al. Evaluation of the cortisol concentrations in patients with schizophrenia. Psychiatr Danub. (2016) 28(Suppl. 1):162–4.
18. Pruessner M, Vracotas N, Joober R, Pruessner JC, Malla AK. Blunted cortisol awakening response in men with first episode psychosis: relationship to parental bonding. Psychoneuroendocrinology (2013) 38:229–40. doi: 10.1016/j.psyneuen.2012.06.002
19. Holtzman CW, Trotman HD, Goulding SM, Ryan AT, Macdonald AN, Shapiro DI, et al. Stress and neurodevelopmental processes in the emergence of psychosis. Neuroscience (2013) 249:172–91. doi: 10.1016/j.neuroscience.2012.12.017
20. Jansen L, Wied C, Kahn R. Selective impairments in the stress response in schizophrenic patients. Psychopharmacology (2000) 149:319–25. doi: 10.1007/s002130000381
21. Borges S, Gayer-Anderson C, Mondelli V. A systematic review of the activity of the hypothalamic-pituitary-adrenal axis in first episode psychosis. Psychoneuroendocrinology (2013) 38:603–11. doi: 10.1016/j.psyneuen.2012.12.025
22. Nash AI. Crosstalk between insulin and dopamine signaling: a basis for the metabolic effects of antipsychotic drugs. J Chem Neuroanat. (2016) 83–4:59–68. doi: 10.1016/j.jchemneu.2016.07.010
23. Mazurka R, Wynne-Edwards KE, Harkness KL. Stressful life events prior to depression onset and the cortisol response to stress in youth with first onset versus recurrent depression. J Abnorm Child Psychol. (2016) 44:1173–84. doi: 10.1007/s10802-015-0103-y
24. Anderson G, Maes M. How immune-inflammatory processes link CNS and psychiatric disorders: classification and treatment implications. CNS Neurol Disord Drug Targets (2017) 16:266–78. doi: 10.2174/1871527315666161122144659
25. Daban C, Vieta E, Mackin P, Young AH. Hypothalamic-pituitary-adrenal axis and bipolar disorder. Psychiatr Clin North Am. (2005) 28:469–80. doi: 10.1016/j.psc.2005.01.005
26. Fond G, Godin O, Boyer L, Berna F, Andrianarisoa M, Coulon N, et al. Chronic low-grade peripheral inflammation is associated with ultra resistant schizophrenia. Results from the FACE-SZ cohort. Eur Arch Psychiatry Clin Neurosci. (2018). doi: 10.1007/s00406-018-0908-0. [Epub ahead of print].
27. Yamanishi T, Tuboi S. The mechanism of the L-cystine cleavage reaction cat- alyzed by rat liver gamma-cystathionase. J Biochem. (1981) 89:1913–21. doi: 10.1093/oxfordjournals.jbchem.a133393
28. Ida T, Sawa T, Ihara H, Tsuchiya Y, Watanabe Y, Kumagai Y, et al. Reactive cysteine persulfides and S-polythiolation regulate oxidative stress and redox signaling. Proc Natl Acad Sci USA. (2014) 111:7606–11. doi: 10.1073/pnas.1321232111
29. Kabil O, Banerjee R. Enzymology of H2S biogenesis, decay and signaling. Anti-oxid Redox Signal. (2014) 20:770–82. doi: 10.1089/ars.2013.5339
30. Nagahara N. Regulation of mercaptopyruvate sulfurtransferase activity via intrasubunit and intersubunit redox-sensing switches. Antioxid Redox Signal. (2013) 19:1792–802. doi: 10.1089/ars.2012.5031
31. Nagy P. Mechanistic chemical perspective of hydrogen sulfide signaling. Methods Enzymol. (2015) 554:3–29. doi: 10.1016/bs.mie.2014.11.036
32. Abe K, Kimura H. The possible role of hydrogen sulfide as an endogenous neuromodulator. J Neurosci. (1996) 16:1066–71. doi: 10.1523/JNEUROSCI.16-03-01066.1996
33. Kimura H. Physiological roles of hydrogen sulfide and polysulfides. Handb Exp Pharmacol. (2015) 230:61–81. doi: 10.1007/978-3-319-18144-8_3
34. Cuevasanta E, Möller MN, Alvarez B. Biological chemistry of hydrogen sulfide and persulfides. Arch Biochem Biophys. (2016) 617:9–25. doi: 10.1016/j.abb.2016.09.018
35. Bełtowski J. Hydrogen sulfide in pharmacology and medicine–an update. Pharmacol Rep. (2015) 67:647–58. doi: 10.1016/j.pharep.2015.01.005
36. Chen WL, Niu YY, Jiang WZ, Tang HL, Zhang C, Xia QM, et al. Neuroprotective effects of hydrogen sulfide and the underlying signaling pathways. Rev Neurosci. (2015) 26:129–142. doi: 10.1515/revneuro-2014-0051
37. Lo Faro ML, Fox B, Whatmore JL, Winyard PG, Whiteman M. Hydrogen sulfide and nitric oxide interactions in inflammation. Nitric Oxide (2014) 41:38–47. doi: 10.1016/j.niox.2014.05.014
38. Kimura Y, Kimura H. Hydrogen sulfide protects neurons from oxidative stress. FASEB J. (2004) 18:1165–7. doi: 10.1096/fj.04-1815fje
39. Zhao FL, Fang F, Qiao PF, Yan N, Gao D, Yan Y. AP39, a mitochondria-targeted hydrogen sulfide donor, supports cellular bioenergetics and protects against Alzheimer's disease by preserving mitochondrial function in APP/PS1 mice and neurons. Oxid Med Cell Longev. (2016) 2016:8360738. doi: 10.1155/2016/8360738
40. Vandiver MS, Paul BD, Xu R, Karuppagounder S, Rao F, Snowman AM, et al. Sulfhydration mediates neuroprotective actions of parkin. Nat Commun. (2013) 4:1626. doi: 10.1038/ncomms2623
41. Sulaieva O, Wallace JL. Gaseous mediator-based anti-inflammatory drugs. Curr Opin Pharmacol. (2015) 25:1–6. doi: 10.1016/j.coph.2015.08.005
42. De Cicco P, Panza E, Ercolano G, Armogida C, Sessa G, Pirozzi G, et al. ATB-346, a novel hydrogen sulfide-releasing anti-inflammatory drug, induces apoptosis of human melanoma cells and inhibits melanoma development in vivo. Pharmacol Res. (2016) 114:67–73. doi: 10.1016/j.phrs.2016.10.019
43. Collin M, Anuar FB, Murch O, Bhatia M, Moore PK, Thiemermann C. Inhibition of endogenous hydrogen sulfide formation reduces the organ injury caused by endotoxemia. Br J Pharmacol. (2005) 146:498–505. doi: 10.1038/sj.bjp.0706367
44. Li L, Bhatia M, Zhu YZ, Zhu YC, Ramnath RD, Wang ZJ, et al. Hydrogen sulfide is a novel mediator of lipopolysaccharide induced inflammation in the mouse. FASEB J. (2005) 19:1196–8. doi: 10.1096/fj.04-3583fje
45. Zhang H, Zhi L, Moochhala S, Moore PK, Bhatia M. Hydrogen sulfide acts as an inflammatory mediator in cecal ligation and puncture induced sepsis in mice by upregulating the production of cytokines and chemokines via NF-kappaB. Am J Physiol Lung Cell Mol Physiol. (2007) 292:L960–71. doi: 10.1152/ajplung.00388.2006
46. Zhang H, Moochhala SM, Bhatia M. Endogenous hydrogen sulfide regulates inflammatory response by activating the ERK pathway in polymicrobial sepsis. J Immunol. (2008) 181:4320–31. doi: 10.4049/jimmunol.181.6.4320
47. Yan Y, Chen C, Zhou H, Gao H, Chen L, Chen L, et al. Endogenous hydrogen sulfide formation mediates the liver damage in endotoxemic rats. Res Vet Sci. (2013) 94:590–5. doi: 10.1016/j.rvsc.2012.10.009
48. Ahmad A, Gerö D, Olah G, Szabo C. Effect of endotoxemia in mice genetically deficient in cystathionine-γ-lyase, cystathionine-β-synthase or 3-mercaptopyruvate sulfurtransferase. Int J Mol Med. (2016) 38:1683–92. doi: 10.3892/ijmm.2016.2771
49. Shirozu K, Tokuda K, Marutani E, Lefer D, Wang R, Ichinose F. Cystathionine γ-lyase deficiency protects mice from galactosamine/lipopolysaccharide-induced acute liver failure. Antioxid Redox Signal. (2014) 20:204–16. doi: 10.1089/ars.2013.5354
50. Badiei A, Chambers ST, Gaddam RR, Bhatia M. Cystathionine-γ-lyase gene silencing with siRNA in monocytes/ macrophages attenuates inflammation in cecal ligation and puncture-induced sepsis in the mouse. J Biosci. (2016) 41:87–95. doi: 10.1007/s12038-016-9598-9
51. Xiong J, Wie B, Li Y, Zhan J, Jiang S, Chen HB, et al. Decreased plasma levels of gasotransmitter hydrogen sulfide in patients with schizophrenia: correlation with psychopathology and cognition. Psychopharmacology (2018) 235:2267–74. doi: 10.1007/s00213-018-4923-7
52. Paxinos G, Watson C. The Rat Brain in Stereotaxic Coordinates. New York, NY: Academic Press (1982).
53. Clement HW, Pschibul A, Schulz E. Effects of secretin on extracellular GABA and other amino acid concentrations in the rat hippocampus. Int Rev Neurobiol. (2005) 71:239–71. doi: 10.1016/S0074-7742(05)71011-4
54. Dikalov SI, Grigor'ev I, Voinov M, Bassenge E. Detection of superoxide radicals and peroxynitrite by 1-hydroxy-4-phosphonooxy-2,2,6,6-tetramethylpiperidine: quantification of extracellular superoxide radicals formation. Biochem Biophys Res Commun. (1998) 248:211–5.
55. Dikalov SI, Li W, Mehranpour P, Wang SS, Zafari AM. Production of extracellular superoxide by human lymphoblast cell lines: comparison of electron spin resonance techniques and cytochrome C reduction assay. Biochem Pharmacol. (2007) 73:972–80. doi: 10.1016/j.bcp.2006.12.012
56. Clement HW, Vazquez JF, Sommer O, Heiser P, Morawietz H, Hopt U, et al. Lipopolysaccharide-induced radical formation in the striatum is abolished in Nox2 gp91phox-deficient mice. J Neural Transm. (2010) 117:13–22. doi: 10.1007/s00702-009-0327-5
57. Vazquez JF, Clement HW, Sommer O, Schulz E, van Calker D. Local stimulation of the adenosine A2B receptors induces an increased release of IL-6 in mouse striatum: and in vivo microdialysis study. J Neurochem. (2008) 105:904–9. doi: 10.1111/j.1471-4159.2007.05191.x
58. Schmidt AJ, Hemmeter UM, Krieg JC, Vedder H, Heiser P. Impact of haloperidol and quetiapine on the expression of genes encoding antioxidant enzymes in human neuroblastoma SH-SY5Y cells. J Psychiatr Res. (2009) 43:818–23. doi: 10.1016/j.jpsychires.2008.11.005
59. Hayden LJ, Goeden H, Roth SH. Exposure to low levels of hydrogen sulfide elevates circulating glucose in maternal rats. J Toxicol Environ Health (1990) 31:45–52. doi: 10.1080/15287399009531436
60. Pichette J, Gagnon J. Implications of hydrogen sulfide in glucose regulation: how H2S can alter glucose homeostasis through metabolic hormones. Oxid Med Cell Longev. (2016) 2016:3285074. doi: 10.1155/2016/3285074
61. Lin J, Chen M, Liu D, Guo R, Lin K, Deng H, et al. Exogenous hydrogen sulfide protects human umbilical vein endothelial cells against high glucose-induced injury by inhibiting the necroptosis pathway. Int J Mol Med. (2017) 41:1477–86. doi: 10.3892/ijmm.2017.3330
62. Torrans EL, Clemens HP. Physiological and biochemical effects of acute exposure of fish to hydrogen sulfide. Comp Biochem Physiol C. (1982) 71:183–90. doi: 10.1016/0306-4492(82)90034-X
63. Kombian SB, Reiffenstein RJ, Colmers WF. The actions of hydrogen sulfide on dorsal raphe serotonergic neurons in vitro. J Neurophysiol. (1993) 70:81–96.
64. Khademullah CS, Ferguson AV. Depolarizing actions of hydrogen sulfide on hypothalamic paraventricular nucleus neurons. PLoS ONE (2013) 8:e64495. doi: 10.1371/journal.pone.0064495
65. Kuksis M, Smith PM, Ferguson AV. Hydrogen Sulfide regulates cardiovascular function by influencing the excitability of subfornical organ neurons. PLoS ONE (2014) 9:e105772. doi: 10.1371/journal.pone.0105772
66. Andersson DA, Gentry C, Bevan S. TRPA1 has a key role in the somatic pro-nociceptive actions of hydrogen sulfide. PLoS ONE (2012) 7:e46917. doi: 10.1371/journal.pone.0046917
67. Steen NE, Methlie P, Lorentzen S, Dieset I, Aas M, Nerhus M, et al. Altered systemic cortisol metabolism in bipolar disorder and schizophrenia spectrum disorders. J Psychiatr Res. (2014) 52:57–62. doi: 10.1016/j.jpsychires.2014.01.017
68. Streit F, Memic A, Hasandedić L, Rietschel L, Frank J, Lang M, et al. Perceived stress and hair cortisol: differences in bipolar disorder and schizophrenia. Psychoneuroendocrinology. (2016) 69:26–34. doi: 10.1016/j.psyneuen.2016.03.010
69. Walder DJ, Walker EF, Lewine RJ. Cognitive functioning, cortisol release, and symptom severity in patients with schizophrenia. Biol Psychiatry (2000) 48:1121–32. doi: 10.1016/S0006-3223(00)01052-0
70. Walker EF, Trotman HD, Pearce BD, Addington J, Cadenhead KS, Cornblatt BA, et al. Cortisol levels and risk for psychosis: initial findings from the North American prodrome longitudinal study. Biol Psychiatry (2013) 74:410–7. doi: 10.1016/j.biopsych.2013.02.016
71. Cohrs S, Röher C, Jordan W, Meier A, Huether G, Wuttke W, et al. The atypical antipsychotics olanzapine and quetiapine, but not haloperidol, reduce ACTH and cortisol secretion in healthy subjects. Psychopharmacology (Berl). (2006) 185:11–8. doi: 10.1007/s00213-005-0279-x
72. Zhang XY, Zhou DF, Cao LY, Wu GY, Shen YC. Cortisol and cytokines in chronic and treatment-resistant patients with schizophrenia: association with psychopathology and response to antipsychotics. Neuropsychopharmacology (2005) 30:1532–8. doi: 10.1038/sj.npp.1300756
73. Flores BH, Kenna H, Keller J, Solvason HB, Schatzberg AF. Clinical and biological effects of mifepristone treatment for psychotic depression. Neuropsychopharmacology (2006) 31:628–36. doi: 10.1038/sj.npp.1300884
74. Wang CN, Liu YJ, Duan GL, Zhao W, Li XH, Zhu XY, et al. CBS and CSE are critical for maintenance of mitochondrial function and glucocorticoid production in adrenal cortex. Antioxid Redox Signal (2014) 21:2192–207. doi: 10.1089/ars.2013.5682
75. Navarra P, Dello Russo C, Mancuso C, Preziosi P, Grossman A. Gaseous neuromodulators in the control of neuroendocrine stress axis. Ann NY Acad Sci USA. (2000) 917:638–46. doi: 10.1111/j.1749-6632.2000.tb05429.x
76. Longen S, Beck KF, Pfeilschifter J. H2S-induced thiol-based redox switches: biochemistry and functional relevance for inflammatory diseases. Pharmacol Res. (2016) 111:642–51. doi: 10.1016/j.phrs.2016.07.026
77. Olas B, Kontek B. Hydrogen sulfide decreases the plasma lipid peroxidation induced by homocysteine and its thiolactone. Mol Cell Biochem. (2015) 404:39–43. doi: 10.1007/s11010-015-2364-8
78. Kimura Y, Goto Y, Kimura H. Hydrogen sulfide increases glutathione production and suppresses oxidative stress in mitochondria. Antioxid Redox Signal. (2010) 12:1–13. doi: 10.1089/ars.2008.2282
79. Lee ZW, Low YL, Huang S, Wang T, Deng LW. The cystathionine Y-lyase/hydrogen sulfide system maintains cellular glutathione status. Biochem J. (2014) 3:425–35. doi: 10.1042/BJ20131434
80. Sen N, Paul BD, Gadalla MM, Mustafa AK, Sen T, Xu R, et al. Hydrogen sulfide-linked sulfhydration of NF-κB mediates its antiapoptotic actions. Mol Cell. (2012) 45:13–24. doi: 10.1016/j.molcel.2011.10.021
81. Tang ZJ, Zou W, Yuan J, Zhang P, Tian Y, Xiao ZF, et al. Antidepressant-like and anxiolytic-like effects of hydrogen sulfide in streptozotocin-induced diabetic rats through inhibition of hippocampal oxidative stress. Behav Pharmacol. (2015) 26:427–35. doi: 10.1097/FBP.0000000000000143
82. Kimura Y, Dargusch R, Schubert D, Kimura H. Hydrogen sulfide protects HT22 neuronal cells from oxidative stress. Antioxid Redox Signal. (2006) 8:661–70. doi: 10.1089/ars.2006.8.661
83. Huang CW, Moore PK. H2S synthesizing enzymes: biochemistry and molecular aspects. In: Moore PK, Whiteman M, editors. Chemistry, Biochemistry and Pharmacology of Hydrogen Sulfide. Basel: Springer (2015). p. 3–25.
84. Wilinski B, Wilinski J, Somogyi E, Piotrowska J, Opoka W. Vitamin D3 (cholecalciferol) boosts hydrogen sulfide tissue concentrations in heart and other mouse organs. Folia Biol (Krakow) (2012) 60:243–7. doi: 10.3409/fb60_3-4.243-247
85. Burguera EF, Vela-Anero A, Magalhaes J, Meijide-Failde R, Blanco FJ. Effect of hydrogen sulfide sources on inflammation and catabolic markers on interleukin 1ß-stimulated human articular chondrocytes. Osteoarthritis Cartilage (2014) 22:1026–35. doi: 10.1016/j.joca.2014.04.031
86. Di Liberto V, Borroto-Escuela DO, Frinchi M, Verdi V, Fuxe K, Belluardo N, et al. Existence of muscarinic acetylcholine receptor (mAChR) and fibroblast growth factor receptor (FGFR) heteroreceptor complexes and their enhancement of neurite outgrowth in neural hippocampal cultures. Biochim Biophys Acta. (2016) 1861:235–45. doi: 10.1016/j.bbagen.2016.10.026
87. Borroto-Escuela DO, Tarakanov AO, Bechter K, Fuxe K. IL1R2, CCR2, and CYCR4 may form heteroreceptor comlexes with NMDAR and D2R: relevance for schizophrenia. Front Psychiatry (2017) 8:24. doi: 10.3389/fpsyt.2017.00024
Keywords: schizophrenia, electron spin resonance spectroscopy (ESR), reactive oxygen species (ROS), hydrogensulfide (H2S), lipopolysaccharide (LPS)
Citation: Sommer O, Aug RL, Schmidt AJ, Heiser P, Schulz E, Vedder H and Clement H-W (2018) Hydrogen Sulfide Affects Radical Formation in the Hippocampus of LPS Treated Rats and the Effect of Antipsychotics on Hydrogen Sulfide Forming Enzymes in Human Cell Lines. Front. Psychiatry 9:501. doi: 10.3389/fpsyt.2018.00501
Received: 14 December 2016; Accepted: 24 September 2018;
Published: 16 October 2018.
Edited by:
Karl Bechter, Universität Ulm, GermanyReviewed by:
Dasiel Oscar Borroto-Escuela, Karolinska Institute (KI), SwedenXu Cao, Memorial Sloan Kettering Cancer Center, United States
Copyright © 2018 Sommer, Aug, Schmidt, Heiser, Schulz, Vedder and Clement. This is an open-access article distributed under the terms of the Creative Commons Attribution License (CC BY). The use, distribution or reproduction in other forums is permitted, provided the original author(s) and the copyright owner(s) are credited and that the original publication in this journal is cited, in accordance with accepted academic practice. No use, distribution or reproduction is permitted which does not comply with these terms.
*Correspondence: Hans-Willi Clement, aGFucy13aWxsaS5jbGVtZW50QHVuaWtsaW5pay1mcmVpYnVyZy5kZQ==
†These authors have contributed equally to this work