- 1Psychiatry Department, Massachusetts General Hospital, Harvard Medical School, Charlestown, MA, United States
- 2Guang’anmen Hospital, China Academy of Chinese Medical Sciences, Beijing, China
- 3Institute of Acupuncture and Moxibustion, China Academy of Chinese Medical Sciences, Beijing, China
Depression is a highly prevalent disorder, and its treatment is far from satisfactory. There is an urgent need to develop a new treatment for depression. Although still at its early stage, transcutaneous auricular vagus nerve stimulation (taVNS) has shown promising potential for treating depression. In this article, we first summarize the results of clinical studies on the treatment effect of taVNS on depression. Then, we re-analyze a previous study to identify the specific symptoms taVNS can relieve as indicated by subscores of the 24-item Hamilton Depression Scale in patients with depression. We found that taVNS can significantly reduce multiple symptoms of depression patients, including anxiety, psychomotor retardation, sleep disturbance, and hopelessness. Next, we pose several hypotheses on the mechanism of taVNS treatment of depression, including directly and indirectly modulating the activity and connectivity of key brain regions involved in depression and mood regulation; inhibiting neuro-inflammatory sensitization; modulating hippocampal neurogenesis; and regulating the microbiome–brain–gut axis. Finally, we outline current challenges and lay out the future directions of taVNS treatment of depression, which include (1) intensively comparing stimulation parameters and “dose effect” (treatment frequency and duration) to maximize the treatment effect of taVNS; (2) exploring the effect of taVNS on disorders comorbid with depression (such as chronic pain disorders, cardiovascular disorder, and autism) to provide new “two-for-one” treatment approaches for patients with these disorders; and (3) applying multiple scale methods to explore the underlying mechanism of taVNS.
Introduction
The vagus nerve (VN) is the longest cranial nerve in the human body and is involved in the regulation of multiple systems (1). Due to this wide influence on multiple systems and its important role in maintaining homeostasis, stimulating the VN to modulate the function of related organs has long drawn the attention of investigators (2). As a slow-acting therapy, cervical vagus nerve stimulation (VNS) has been approved by the US Food and Drug Administration for managing treatment-refractory epilepsy in 1997 and for chronic treatment-resistant depression in 2005 (1). However, surgical risks, technical challenges, and potential side effects have limited the application of VNS (3, 4).
To overcome such barriers of applying invasive VNS (iVNS), non-invasive transcutaneous vagus nerve stimulation (tVNS) methods have been developed. Currently, there are two main ways to apply tVNS. One is to superficially apply stimulation on the cervical nerve using a specially designed device, such as GammaCore, and the other is to apply stimulation on the ear. In this paper, we will focus on the latter. The rationale of tVNS on the ear (transcutaneous auricular VNS, taVNS) is based on anatomical studies demonstrating that certain parts of the ear area (concha and lower half of the back ear over the mastoid process) have afferent VN distribution (5–7). According to the “bottom-up” mechanism of the central nervous system (CNS), electrical stimulation of these areas may produce activity changes in the VN pathway in the brain stem and central structures (8), producing a modulation effect similar to iVNS (9–11). taVNS has been used to treat disorders, such as epilepsy (12, 13), pre-diabetes (14), depression, and chronic tinnitus (15), as well as to boost associative memory (16).
In this manuscript, we summarize the findings of clinical studies on taVNS treatment of depression, re-analyze a previous data set to explore the specific symptoms tVNS can relieve in patients with depression [as indicated by Hamilton Depression Rating Scale (HAMD) subscores] (17), and discuss the potential underlying mechanism, limitations, and future direction of taVNS. Please also see recent review articles on iVNS treatment of depression (2), iVNS/taVNS treatment of chronic pain (18), clinical application (19), and efficacy and tolerability (20).
Potentials of taVNS Treatment of Depression and its Side Effects
Major depressive disorder (MDD) is a highly prevalent disorder that can significantly reduce quality of life (21). Current treatments for MDD are far from satisfactory (22–24), thereby calling for new treatments for MDD. As a non-invasive peripheral neuromodulation method, taVNS may be a promising treatment option for patients with MDD.
The first taVNS clinical trial on individuals with MDD was performed by Hein and colleagues (9). They investigated the treatment effect of bilateral taVNS on MDD patients using an add-on design (antidepressant therapy with real or sham taVNS). They found that compared to the sham group, the real taVNS group showed significant improvement on the Beck Depression Inventory after a 2-week treatment (five times per week). However, there was no significant difference on the HAMD between the two groups.
In a subsequent non-randomized clinical study with 160 MDD patients (17), we investigated the taVNS treatment effect by training the patients to apply bilateral taVNS at home. The first cohort of patients (n = 91) received taVNS for 12 weeks; the second cohort (n = 69) first received 4 weeks of sham taVNS followed by 8 weeks of real taVNS. After the fourth week, patients in the taVNS group had greater decreases in the 24-item HAMD score and higher rates of good responders than those of the sham taVNS group. The clinical improvements continued until week 12.
In a recent single-arm study (25), Trevizol and colleagues recruited 12 patients with MDD and tested the effect of taVNS on the bilateral mastoid process (10-session taVNS over 2 weeks). The results showed that 17-item HAMD scores were reduced significantly after the 2-week treatment. All patients exhibited a clinical response, defined as a reduction of HAMD scores of at least 50%. The effect remained 1 month after treatment.
Although the above studies suggest that taVNS can reduce the symptoms of MDD, no study has reported how it can modulate the specific symptoms of MDD patients. To address the question, we re-analyzed the data of our previous study (17) and explored how taVNS can modulate HAMD subscores of patients with MDD (Table 1) by performing a repeated measurement analysis with Bonferroni correction to adjust the p-value (0.05/7 = 0.007 significance level). We found that compared with sham taVNS, 1-month taVNS can significantly reduce multiple symptoms of MDD patients, including anxiety, psychomotor retardation, sleep disturbance, and hopelessness. We also observed a downward trend in cognitive disturbance and diurnal variation (Table 1).
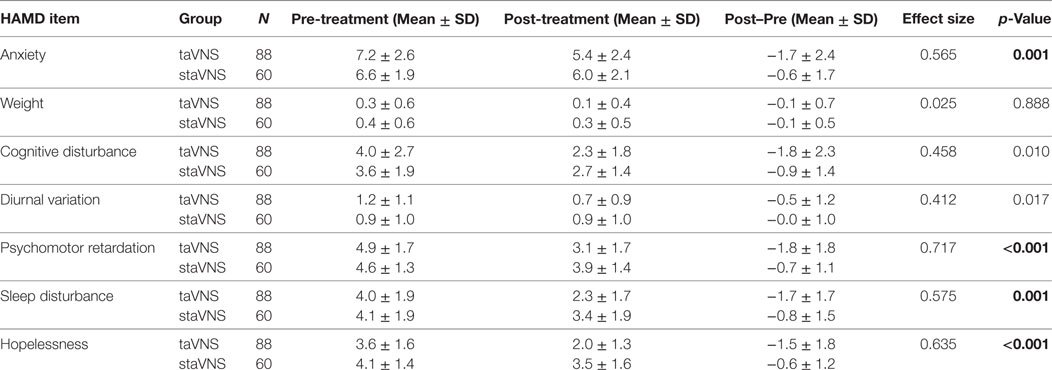
Table 1. Pre- and post-treatment differences in HAMD subscores between real and sham transcutaneous auricular vagus nerve stimulation (taVNS) cohorts; p values indicating significant difference after Bonferroni correction (p = 0.05/7 = 0.007) are marked in bold.
Transcutaneous auricular vagus nerve stimulation is a quite safe and well-tolerable treatment method (20). Reported mild/moderate side effects include tinnitus or acceleration of original tinnitus and local problems at stimulation sites, such as pain, paresthesia, or pruritus during or after stimulation (17, 26). Since there are no direct fibers connecting the ear VN to the heart (27, 28), both left and right ears should be safe for applying taVNS. In a recent study (28), Kreuzer et al. measured EKG changes after 24 months of taVNS and found that taVNS has no arrhythmic effects on cardiac function in tinnitus patients with no known pre-existing cardiac pathology. In another study on taVNS treatment of MDD (9), investigators also found that heart rate, blood pressure, and blood test values did not change over the 2-week treatment period.
Interestingly, applying taVNS on the bilateral mastoid process (25) seems to be associated with more severe side effects as compared to taVNS applied on the concha (9, 17). In the Trevizol study (25), of the total 12 patients, 10 patients reported mild to moderate diurnal sleepiness after stimulation, six reported mild to moderate tension headaches with no need for medication, and four reported mild to moderate nausea. We speculate this may be due to the electrical current flowing across the whole brain during bilateral stimulation. Further study is needed to explore the side effects of taVNS on the bilateral mastoid process.
Mechanisms/Hypothesis on taVNS Treatment of Depression
taVNS Can Modulate the Brain Network Associated with the Neuropathology of Depression
A growing body of evidence has shown that depression is associated with structural and functional abnormalities in multiple brain regions involved in emotional processing, self-representation, reward, and external stimulus (stress, distress) interactions (29–37). Based on the limbic-cortical dysregulation hypothesis (38–40), the brain regions involved in MDD are associated with two components: the vegetative-somatic component, including the subgenual cingulate cortex, anterior insula, hippocampus, hypothalamus, and amygdala, and the attention-cognition component, including the dorsal frontal area, dorsal cingulate cortex, inferior parietal cortex, and posterior cingulate cortex. Located between the two components are the basal ganglia and thalamus, which closely communicate with the two components (Figure 1A).
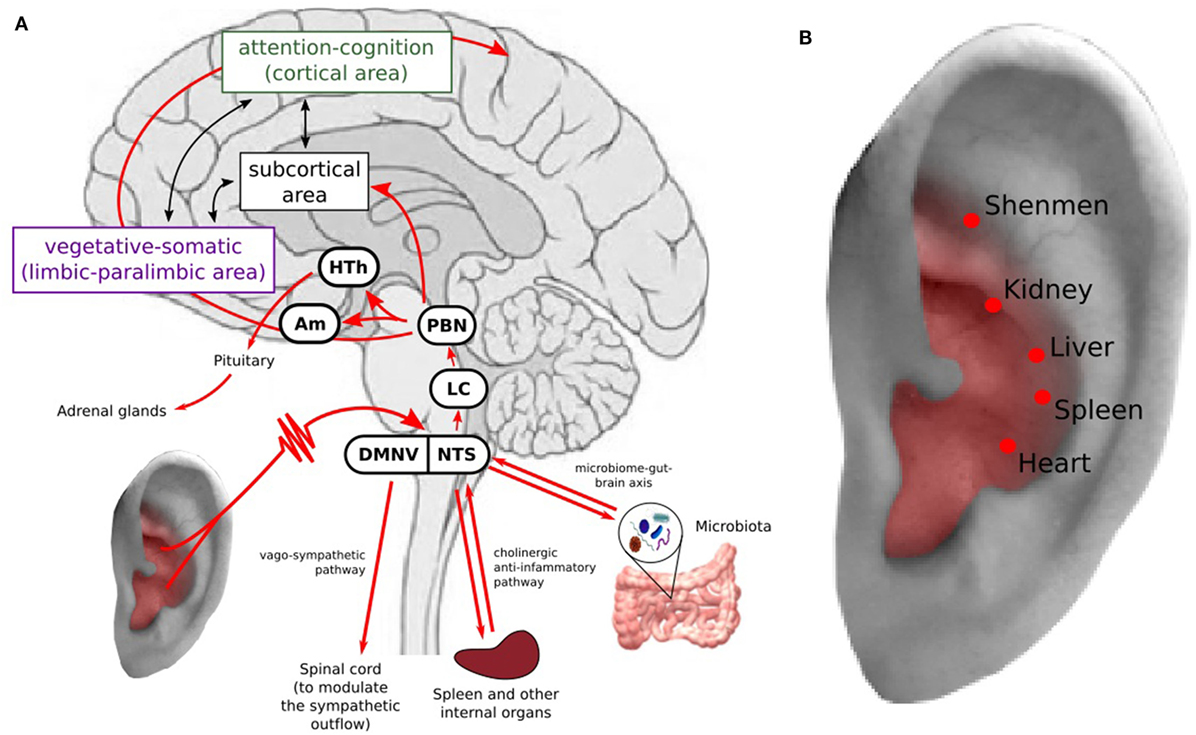
Figure 1. (A) Transcutaneous auricular vagus nerve stimulation (taVNS) can modulate the brain network associated with the neuropathology of depression and inhibit inflammation response. Stimulation of the auricular branch of the vagus nerve (VN, indicated in red), which projects to the nucleus tractus solitari (NTS), continuing to the locus coeruleus and parabrachial nucleus. From the parabrachial nucleus, it propagates to various brain regions involved in depression (39, 40). taVNS may inhibit inflammation response to relieve stress and depressive symptoms. HTh, hypothalamus; PBN, parabrachial nucleus; LC, locus coeruleus; NTS, nucleus tractus solitary; DMNV, dorsal motor nucleus of the vagus nerve. (B) Auricular acupuncture points used for treating depression and other mental disorders at area with VN distribution.
Neural anatomy has shown that the auricular branch of the vagus nerve (ABVN) projects to the nucleus tractus solitari (NTS), which is further connected with other brain regions, such as the locus coeruleus, parabrachial nucleus, hypothalamus, thalamus, amygdala, hippocampus, anterior cingulate cortex (ACC), anterior insula, and lateral prefrontal cortex (19, 41). Thus, the VN has direct and indirect connections to the depression-related cortical–limbic-thalamic–striatal neural circuits, influencing the activity of these regions (42–46) (Figure 1A).
Recent neuroimaging studies (47–53) found that compared with a control condition, taVNS stimulation can produce activation of the “classical” central vagal projections, e.g., widespread activity in the NTS, dorsal raphe, locus coeruleus, parabrachial area, hypothalamus, amygdala, ACC, anterior insula, and nucleus accumbens. For instance, in a recent study (53), we found that taVNS produced fMRI signal increases in the anterior insula compared to sham stimulation in patients with MDD. The insula activation level during the first stimulation session in the taVNS group was significantly associated with clinical improvement after 4 weeks, as shown by the reduction of HAMD scores. In addition, we found that after 1 month of taVNS treatment, resting-state functional connectivity (rsFC) between the default mode network (DMN), a key network involved in depression (54–60), and the anterior insula and parahippocampus decreased, while the FC between the DMN and the orbital prefrontal cortex and precuneus increased compared with sham taVNS (61). In another study using the same dataset, we found that taVNS can significantly increase rsFC between the right amygdala and left dorsolateral prefrontal cortex compared with sham taVNS (62). These results further endorse the extensive modulation effect of taVNS on brain regions involved in depression.
taVNS May Relieve Symptoms of Depression by Modulating the Inflammation System
Literature suggests that stress initiates cognitive, affective, and possibly biological processes that increase risk for depression (63, 64). Inflammation may play an important role in this process. Specifically, neuro-inflammatory sensitization provoked by stress elicits profound changes in behavior, including common symptoms of depression such as sad mood, anhedonia, fatigue, psychomotor retardation, and social–behavioral withdrawal (63–66). In this process, the hypothalamus, anterior insula, and ACC play an important role (63).
Studies have suggested that the VN plays a crucial role in bidirectionally connecting the brain and immune system, reducing exacerbated inflammation processes outside the CNS (67). Specifically, the VN may participate in the modulation of the inflammation system through two pathways: (1) activating the hypothalamic–pituitary–adrenal axis and suppressing peripheral inflammation via glucocorticoids (68) and (2) through the mechanism of the “inflammatory reflex” (67, 69–72) (Figure 1A). In the inflammatory reflex, accumulation of inflammatory cytokines activates VN fibers from which afferent signals ascend to the NTS (69). The NTS projects to efferent vagal neurons in the dorsal motor nucleus of the VN, which projects to intrinsic ganglia in the viscera such as in the spleen and liver. Then, acetylcholine is released in the parenchyma of target organs, activating local nAChRa7 macrophages. Production of inflammatory cytokines is inhibited, attenuating the activity of the immune system (73). In addition, VNS may also trigger the vago-sympathetic pathway, i.e., vagal afferents in the NTS trigger the dorsal motor nucleus of the vagus nerve to modulate the sympathetic outflow by innervating preganglionic sympathetic neurons in the spinal cord (74, 75) (Figure 1A).
Other Potential Mechanisms
Recently, accumulating evidence has demonstrated that microbe interactions are crucial in maintaining homeostasis in humans. Studies (76–79) have suggested that gut microbiota can influence brain function, mood, and behavior by interacting with the central nervous system through neural, endocrine, and immune pathways. Particularly, studies have shown that the microbiota is crucial in modulating the stress response and stress-related behaviors, such as depression and anxiety (76, 78–80). It is well-known that the VN can significantly modulate the gastrointestinal, immune, and endocrine systems (1). Thus, taVNS may also regulate the functions of the above systems and achieve a treatment effect in depression by adjusting the microbiome–brain–gut axis (80) (Figure 1A).
Also, based on the neurogenic theory of depression (81), depression results from impaired adult hippocampal neurogenesis, and restoration of adult hippocampal neurogenesis leads to recovery. Studies have shown that VNS may stimulate hippocampal neurogenesis, providing another possible mechanism for depression treatment. For instance, studies have shown that VNS can alter the transmission of neurotransmitters, such as serotonin and norepinephrine, which can modulate hippocampal cell proliferation (2). Thus, taVNS may also relieve depression symptoms by modulating hippocampal neurogenesis (2).
taVNS and Auricular Acupuncture—Old Wine in a New Bottle
Stimulating certain areas on the ear to treat disorders is not something new. Acupuncture, an ancient therapeutic method, has a long history of applying stimulation on different parts of the body, including the ear, to treat disorders. Nowadays, auricular acupuncture has become a crucial school of acupuncture and is widely used in acupuncture practice (82). Nevertheless, the underlying mechanism of auricular acupuncture remains unclear.
Transcutaneous auricular vagus nerve stimulation provides a new angle to understand auricular acupuncture (83). For instance, the auricular acupoints used for depression are also located at the area with VN distribution (Figure 1B), Thus, auricular acupuncture and taVNS perform the same or similar treatment procedure guided by different theories. Usichenko et al. found that the analgesic effects of auricular acupuncture may be explained by stimulation of ABVN (83). Further study to verify the specificity of auricular acupuncture will not only deepen our understanding of auricular acupuncture, but also facilitate the development of taVNS and peripheral neuromodulation.
Challenges and Future Directions
Where to Stimulate and How to Stimulate
A neural anatomy study (6) showed that the auricular branch of the VN is mainly distributed on the concha (including the outer auditory canal) and lower half of the back ear. Thus, these areas should be the target of taVNS. Nevertheless, given that the branching of the nerve in the concha is variable across individuals and there are other nerve branches in the area, it remains a challenge to stimulate VN consistently across different individuals.
In a recent study, Kraus et al. (49) compared taVNS-evoked fMRI signal changes at the anterior and posterior sides of the left outer auditory canal. Many brain regions excluding the insular cortex showed fMRI signal changes. The fMRI signals were notably decreased in the parahippocampal gyrus, posterior cingulate cortex, and right thalamus (pulvinar) following anterior auditory canal wall stimulation (49). In another brain imaging study (84), the authors compared the fMRI signal changes evoked by 25 Hz stimulation at the inner tragus, inferoposterior wall of the ear canal, cymba concha, and earlobe (control location without VN distribution). The results showed that stimulation at the inner tragus and cymba concha produced significantly greater activation in the NTS and LC compared with the control location (earlobe). Further ROI analysis showed that only stimulating the cymba concha produced a significantly stronger activation in both the NTS and LC than stimulating the control location.
These results suggest that taVNS at different locations of the ear with VN innervation may modulate different brain pathways, which may be associated with different modulation effects. More studies are needed to systemically investigate the linkage between the brain regions and different ear areas.
Stimulation frequency and intensity are both crucial parameters in taVNS. One may imagine that low-frequency stimulation (2–10 Hz) is not as efficient as higher frequency stimulation (20–30 Hz) which is currently used in iVNS for epilepsy and depression. In reality, investigators have used different frequencies in previous studies with wide ranges [1.5 Hz (9), 20 Hz (17), and 120 Hz (25)].
Studies suggest that different stimulation frequencies could produce different brain changes and neurotransmitter releases (85, 86). In an animal study (87), investigators found that the anti-epileptic effect of 20 Hz taVNS was significantly longer than those of 2 and 100 Hz as measured by the duration of seizure suppression. A recent study (88) on taVNS treatment of drug-resistant epilepsy showed a significant reduction in seizure frequency in patients of the 25 Hz group as compared to the 1 Hz group. However, in another study (26) on migraine patients, investigators found that 1 Hz taVNS produced greater improvement than 25 Hz taVNS. Taken together, these studies imply that the optimal stimulation frequency may vary depending on the disorder.
Likewise, there are few systematic studies on the optimal intensity of taVNS. Previous studies have suggested that stimulation intensity could be set to a level that could arouse a tingling but tolerable sensation (17, 61, 62). In addition, the intensity may interact with frequency [individuals with low frequency stimulation tend to be able to tolerate higher stimulus intensities than those who receive high frequency stimulation (88)]. However, investigators (9) have applied subthreshold taVNS (the patients could not feel the sensation) and relieved symptoms in patients with MDD, which calls for further research on this topic.
Finally, very few studies have been carried out to explore the “dose effect” of taVNS, i.e., how long and how frequently we should apply taVNS. iVNS stimulation usually lasts for many hours per day. Such durations are unrealistic for taVNS. Current studies range from 30-min stimulation durations two times per day (17) to 15-min stimulation durations five times per week (9). Also, if the patients were trained to apply the taVNS by themselves, the problem of compliance is difficult to evaluate and may somehow counterbalance the interest for such a technique.
In summary, investigators have used a wide range of stimulation parameters in taVNS treatment of depression. Identifying the optimal stimulation parameters and “dose” may represent the crucial next step for taVNS research.
Future Directions
(1) Although previous studies have suggested that taVNS holds potential for patients with MDD, the key parameters and “dose” that can maximize the treatment effect remain unknown. Studies to directly compare different stimulation parameters (frequency and intensity), duration, and frequency of treatment are needed. In addition, large randomized clinical trials are also needed to test the treatment effect of taVNS on patients with different age ranges (from children and teenagers to older adults), as well as different depression severities, so that we can have a better idea of the target population for taVNS.
(2) Depression can be comorbid with many other disorders, such as chronic pain (89, 90), cardiovascular disorder (91, 92), inflammatory bowel disease (93), irritable bowel syndrome (94), and autism (95). Thus, it may also provide a new treatment option for “two-for-one” treatment approaches for patients with disorders comorbid with depression.
(3) Multiple scale mechanism studies incorporating brain imaging tools, inflammation markers, vagal tone measurements, and neural transmitters are needed to deepen our understanding of taVNS and facilitate development of new treatment methods for depression and disorders comorbid with depression.
In summary, taVNS can significantly reduce anxiety, retardation, sleep disturbance, and hopelessness symptoms in patients with depression. Current literature suggests that it may relieve the symptoms of MDD through multiple mechanisms. Further research is needed to identify the optimal stimulation parameters and “dose” of taVNS, testing its effect on MDD patients of different ages and severities, as well as on disorders with comorbid depression.
Author Contributions
JK, PR, and JF conceived the idea; JK, PR, JF, JP, and SL did the literature search and prepared figures and manuscript.
Conflict of Interest Statement
JK holds equity in a startup company (MNT) and pending patents to develop new neuromodulation devices. All other authors declare no competing financial interests.
Funding
JK is supported by TR01 AT006367 and K24AT007323 to Dr. Chenchen Wang. JK is supported by R01AT006364, R01AT008563, R21AT008707, R61AT009310, and P01 AT006663 from NIH/NCCIH. PR is supported by Fundamental Research Funds for Beijing Municipal Science & Technology Commission Z161100002616003 and International project (GH2017-07). JF is supported by National Natural Science Foundation 81774433 and 81273674. SL is supported by an Innovation Talent Fund (CX201708).
References
1. Yuan H, Silberstein SD. Vagus nerve and vagus nerve stimulation, a comprehensive review: part I. Headache (2016) 56(1):71–8. doi:10.1111/head.12647
2. Yuan TF, Li A, Sun X, Arias-Carrion O, Machado S. Vagus nerve stimulation in treating depression: a tale of two stories. Curr Mol Med (2016) 16(1):33–9. doi:10.2174/1566524016666151222143609
3. Ventureyra EC. Transcutaneous vagus nerve stimulation for partial onset seizure therapy. a new concept. Childs Nerv Syst (2000) 16(2):101–2. doi:10.1007/s003810050021
4. Fitzgerald PB. Non-pharmacological biological treatment approaches to difficult-to-treat depression. Med J Aust (2013) 199(6 Suppl):S48–51.
5. Henry TR. Therapeutic mechanisms of vagus nerve stimulation. Neurology (2002) 59(6 Suppl 4):S3–14. doi:10.1212/WNL.59.6_suppl_4.S3
6. Peuker ET, Filler TJ. The nerve supply of the human auricle. Clin Anat (2002) 15(1):35–7. doi:10.1002/ca.1089
7. Trevizol A, Barros MD, Liquidato B, Cordeiro Q, Shiozawa P. Vagus nerve stimulation in neuropsychiatry: targeting anatomy-based stimulation sites. Epilepsy Behav (2015) 51:18. doi:10.1016/j.yebeh.2015.07.009
8. Shiozawa P, Silva ME, Carvalho TC, Cordeiro Q, Brunoni AR, Fregni F. Transcutaneous vagus and trigeminal nerve stimulation for neuropsychiatric disorders: a systematic review. Arq Neuropsiquiatr (2014) 72(7):542–7. doi:10.1590/0004-282X20140061
9. Hein E, Nowak M, Kiess O, Biermann T, Bayerlein K, Kornhuber J, et al. Auricular transcutaneous electrical nerve stimulation in depressed patients: a randomized controlled pilot study. J Neural Transm (2013) 120(5):821–7. doi:10.1007/s00702-012-0908-6
10. Rong PJ, Fang JL, Wang LP, Meng H, Liu J, Ma YG, et al. Transcutaneous vagus nerve stimulation for the treatment of depression: a study protocol for a double blinded randomized clinical trial. BMC Complement Altern Med (2012) 12:255. doi:10.1186/1472-6882-12-255
11. Carreno FR, Frazer A. The allure of transcutaneous vagus nerve stimulation as a novel therapeutic modality. Biol Psychiatry (2016) 79(4):260–1. doi:10.1016/j.biopsych.2015.11.016
12. Rong P, Liu A, Zhang J, Wang Y, Yang A, Li L, et al. An alternative therapy for drug-resistant epilepsy: transcutaneous auricular vagus nerve stimulation. Chin Med J (Engl) (2014) 127(2):300–4.
13. Stefan H, Kreiselmeyer G, Kerling F, Kurzbuch K, Rauch C, Heers M, et al. Transcutaneous vagus nerve stimulation (t-VNS) in pharmacoresistant epilepsies: a proof of concept trial. Epilepsia (2012) 53(7):e115–8. doi:10.1111/j.1528-1167.2012.03492.x
14. Huang F, Dong J, Kong J, Wang H, Meng H, Spaeth RB, et al. Effect of transcutaneous auricular vagus nerve stimulation on impaired glucose tolerance: a pilot randomized study. BMC Complement Altern Med (2014) 14(1):203. doi:10.1186/1472-6882-14-203
15. Shim HJ, Kwak MY, An YH, Kim DH, Kim YJ, Kim HJ. Feasibility and safety of transcutaneous vagus nerve stimulation paired with notched music therapy for the treatment of chronic tinnitus. J Audiol Otol (2015) 19(3):159–67. doi:10.7874/jao.2015.19.3.159
16. Jacobs HI, Riphagen JM, Razat CM, Wiese S, Sack AT. Transcutaneous vagus nerve stimulation boosts associative memory in older individuals. Neurobiol Aging (2015) 36(5):1860–7. doi:10.1016/j.neurobiolaging.2015.02.023
17. Rong P, Liu J, Wang L, Liu R, Fang J, Zhao J, et al. Effect of transcutaneous auricular vagus nerve stimulation on major depressive disorder: a nonrandomized controlled pilot study. J Affect Disord (2016) 195:172–9. doi:10.1016/j.jad.2016.02.031
18. Chakravarthy K, Chaudhry H, Williams K, Christo PJ. Review of the uses of vagal nerve stimulation in chronic pain management. Curr Pain Headache Rep (2015) 19(12):54. doi:10.1007/s11916-015-0528-6
19. Beekwilder JP, Beems T. Overview of the clinical applications of vagus nerve stimulation. J Clin Neurophysiol (2010) 27(2):130–8. doi:10.1097/WNP.0b013e3181d64d8a
20. Ben-Menachem E, Revesz D, Simon BJ, Silberstein S. Surgically implanted and non-invasive vagus nerve stimulation: a review of efficacy, safety and tolerability. Eur J Neurol (2015) 22(9):1260–8. doi:10.1111/ene.12629
21. Daly EJ, Trivedi MH, Wisniewski SR, Nierenberg AA, Gaynes BN, Warden D, et al. Health-related quality of life in depression: a STAR*D report. Ann Clin Psychiatry (2010) 22(1):43–55.
22. Sackeim HA, Lisanby SH. Physical treatments in psychiatry. In: Weissman MM, editor. Treatment of Depression: Bridging the 21st Century. Washington, DC: American psychiatric press (2001). p. 151–72.
23. Rush AJ. Vagus nerve stimulation: clinical results in depression. In: Schachter SC, Schmidt D, editors. Vagus Nerve Stimulation. London: Martin Dunitz (2003). p. 85–112.
24. Holtzheimer PE, Mayberg HS. Stuck in a rut: rethinking depression and its treatment. Trends Neurosci (2011) 34(1):1–9. doi:10.1016/j.tins.2010.10.004
25. Trevizol AP, Shiozawa P, Taiar I, Soares A, Gomes JS, Barros MD, et al. Transcutaneous vagus nerve stimulation (taVNS) for major depressive disorder: an open label proof-of-concept trial. Brain Stimul (2016) 9(3):453–4. doi:10.1016/j.brs.2016.02.001
26. Straube A, Ellrich J, Eren O, Blum B, Ruscheweyh R. Treatment of chronic migraine with transcutaneous stimulation of the auricular branch of the vagal nerve (auricular t-VNS): a randomized, monocentric clinical trial. J Headache Pain (2015) 16:543. doi:10.1186/s10194-015-0543-3
27. Sperling W, Reulbach U, Bleich S, Padberg F, Kornhuber J, Mueck-Weymann M. Cardiac effects of vagus nerve stimulation in patients with major depression. Pharmacopsychiatry (2010) 43(1):7–11. doi:10.1055/s-0029-1237374
28. Kreuzer PM, Landgrebe M, Husser O, Resch M, Schecklmann M, Geisreiter F, et al. Transcutaneous vagus nerve stimulation: retrospective assessment of cardiac safety in a pilot study. Front Psychiatry (2012) 3:70. doi:10.3389/fpsyt.2012.00070
29. Davidson RJ, Pizzagalli D, Nitschke JB, Putnam K. Depression: perspectives from affective neuroscience. Annu Rev Psychol (2002) 53:545–74. doi:10.1146/annurev.psych.53.100901.135148
30. Greicius M. Resting-state functional connectivity in neuropsychiatric disorders. Curr Opin Neurol (2008) 21(4):424–30. doi:10.1097/WCO.0b013e328306f2c5
31. Northoff G, Wiebking C, Feinberg T, Panksepp J. The ‘resting-state hypothesis’ of major depressive disorder-a translational subcortical-cortical framework for a system disorder. Neurosci Biobehav Rev (2011) 35(9):1929–45. doi:10.1016/j.neubiorev.2010.12.007
32. Pizzagalli DA. Frontocingulate dysfunction in depression: toward biomarkers of treatment response. Neuropsychopharmacology (2011) 36(1):183–206. doi:10.1038/npp.2010.166
33. Mwangi B, Ebmeier KP, Matthews K, Steele JD. Multi-centre diagnostic classification of individual structural neuroimaging scans from patients with major depressive disorder. Brain (2012) 135(Pt 5):1508–21. doi:10.1093/brain/aws084
34. Silbersweig D. Default mode subnetworks, connectivity, depression and its treatment: toward brain-based biomarker development. Biol Psychiatry (2013) 74(1):5–6. doi:10.1016/j.biopsych.2013.05.011
35. Hasler G, Northoff G. Discovering imaging endophenotypes for major depression. Mol Psychiatry (2011) 16(6):604–19. doi:10.1038/mp.2011.23
36. Wang Z, Liu J, Zhong N, Qin Y, Zhou H, Li K. Changes in the brain intrinsic organization in both on-task state and post-task resting state. Neuroimage (2012) 62(1):394–407. doi:10.1016/j.neuroimage.2012.04.051
37. Hwang J, Egorova N, Yang XQ, Zhang WY, Chen J, Yang XY, et al. Subthreshold depression is associated with impaired resting state functional connectivity of the cognitive control network. Transl Psychiatry (2015) 5:e683. doi:10.1038/tp.2015.174
38. Mayberg HS. Limbic-cortical dysregulation: a proposed model of depression. J Neuropsychiatry Clin Neurosci (1997) 9(3):471–81. doi:10.1176/jnp.9.3.471
39. Mayberg HS, Brannan SK, Tekell JL, Silva JA, Mahurin RK, McGinnis S, et al. Regional metabolic effects of fluoxetine in major depression: serial changes and relationship to clinical response. Biol Psychiatry (2000) 48(8):830–43. doi:10.1016/S0006-3223(00)01036-2
40. Mayberg HS, Liotti M, Brannan SK, McGinnis S, Mahurin RK, Jerabek PA, et al. Reciprocal limbic-cortical function and negative mood: converging PET findings in depression and normal sadness. Am J Psychiatry (1999) 156(5):675–82.
42. Conway CR, Chibnall JT, Gebara MA, Price JL, Snyder AZ, Mintun MA, et al. Association of cerebral metabolic activity changes with vagus nerve stimulation antidepressant response in treatment-resistant depression. Brain Stimul (2013) 6(5):788–97. doi:10.1016/j.brs.2012.11.006
43. Conway CR, Sheline YI, Chibnall JT, Bucholz RD, Price JL, Gangwani S, et al. Brain blood-flow change with acute vagus nerve stimulation in treatment-refractory major depressive disorder. Brain Stimul (2012) 5(2):163–71. doi:10.1016/j.brs.2011.03.001
44. Conway CR, Sheline YI, Chibnall JT, George MS, Fletcher JW, Mintun MA. Cerebral blood flow changes during vagus nerve stimulation for depression. Psychiatry Res (2006) 146(2):179–84. doi:10.1016/j.pscychresns.2005.12.007
45. Kosel M, Brockmann H, Frick C, Zobel A, Schlaepfer TE. Chronic vagus nerve stimulation for treatment-resistant depression increases regional cerebral blood flow in the dorsolateral prefrontal cortex. Psychiatry Res (2011) 191(3):153–9. doi:10.1016/j.pscychresns.2010.11.004
46. Ruffoli R, Giorgi FS, Pizzanelli C, Murri L, Paparelli A, Fornai F. The chemical neuroanatomy of vagus nerve stimulation. J Chem Neuroanat (2011) 42(4):288–96. doi:10.1016/j.jchemneu.2010.12.002
47. Kraus T, Hosl K, Kiess O, Schanze A, Kornhuber J, Forster C. BOLD fMRI deactivation of limbic and temporal brain structures and mood enhancing effect by transcutaneous vagus nerve stimulation. J Neural Transm (2007) 114(11):1485–93. doi:10.1007/s00702-007-0755-z
48. Dietrich S, Smith J, Scherzinger C, Hofmann-Preiss K, Freitag T, Eisenkolb A, et al. [A novel transcutaneous vagus nerve stimulation leads to brainstem and cerebral activations measured by functional MRI]. Biomed Tech (Berl) (2008) 53(3):104–11. doi:10.1515/BMT.2008.022
49. Kraus T, Kiess O, Hosl K, Terekhin P, Kornhuber J, Forster C. CNS BOLD fMRI effects of sham-controlled transcutaneous electrical nerve stimulation in the left outer auditory canal – a pilot study. Brain Stimul (2013) 6(5):798–804. doi:10.1016/j.brs.2013.01.011
50. Krause B, Marquez-Ruiz J, Kadosh RC. The effect of transcranial direct current stimulation: a role for cortical excitation/inhibition balance? Front Hum Neurosci (2013) 7:602. doi:10.3389/fnhum.2013.00602
51. Frangos E, Ellrich J, Komisaruk BR. Non-invasive access to the vagus nerve central projections via electrical stimulation of the external ear: fMRI evidence in humans. Brain Stimul (2015) 8(3):624–36. doi:10.1016/j.brs.2014.11.018
52. Fang JL, Hong Y, Fan YY, Liu J, Ma YY, Xiu CH, et al. Brain response to transcutaneous electronical stimulation on auricular concha of the healthy subjects using fMRI (Chinese). Chin J Magn Reson Imaging (2014) 5(6):416–22.
53. Fang J, Egorova N, Rong P, Liu J, Hong Y, Fan Y, et al. Early cortical biomarkers of longitudinal transcutaneous vagus nerve stimulation treatment success in depression. Neuroimage Clin (2017) 14:105–11. doi:10.1016/j.nicl.2016.12.016
54. Greicius MD, Flores BH, Menon V, Glover GH, Solvason HB, Kenna H, et al. Resting-state functional connectivity in major depression: abnormally increased contributions from subgenual cingulate cortex and thalamus. Biol Psychiatry (2007) 62(5):429–37. doi:10.1016/j.biopsych.2006.09.020
55. Bluhm R, Williamson P, Lanius R, Theberge J, Densmore M, Bartha R, et al. Resting state default-mode network connectivity in early depression using a seed region-of-interest analysis: decreased connectivity with caudate nucleus. Psychiatry Clin Neurosci (2009) 63(6):754–61. doi:10.1111/j.1440-1819.2009.02030.x
56. Wang L, Hermens DF, Hickies IB, Lagopoulos J. A systematic review of resting-state functional-MRI studies in major depression. J Affect Disord (2012) 142(1–3):6–12. doi:10.1016/j.jad.2012.04.013
57. Posner J, Hellerstein DJ, Gat I, Mechling A, Klahr K, Wang Z, et al. Antidepressants normalize the default mode network in patients with dysthymia. JAMA Psychiatry (2013) 70(4):373–82. doi:10.1001/jamapsychiatry.2013.455
58. Liston C, Chen AC, Zebley BD, Drysdale AT, Gordon R, Leuchter B, et al. Default mode network mechanisms of transcranial magnetic stimulation in depression. Biol Psychiatry (2014) 76(7):517–26. doi:10.1016/j.biopsych.2014.01.023
59. Zhu X, Wang X, Xiao J, Liao J, Zhong M, Wang W, et al. Evidence of a dissociation pattern in resting-state default mode network connectivity in first-episode, treatment-naive major depression patients. Biol Psychiatry (2012) 71(7):611–7. doi:10.1016/j.biopsych.2011.10.035
60. Wu D, Yuan Y, Bai F, You J, Li L, Zhang Z. Abnormal functional connectivity of the default mode network in remitted late-onset depression. J Affect Disord (2013) 147(1–3):277–87. doi:10.1016/j.jad.2012.11.019
61. Fang J, Rong P, Hong Y, Fan Y, Liu J, Wang H, et al. Transcutaneous vagus nerve stimulation modulates default mode network in major depressive disorder. Biol Psychiatry (2016) 79(4):266–73. doi:10.1016/j.biopsych.2015.03.025
62. Liu J, Fang J, Wang Z, Rong P, Hong Y, Fan Y, et al. Transcutaneous vagus nerve stimulation modulates amygdala functional connectivity in patients with depression. J Affect Disord (2016) 205:319–26. doi:10.1016/j.jad.2016.08.003
63. Slavich GM, Irwin MR. From stress to inflammation and major depressive disorder: a social signal transduction theory of depression. Psychol Bull (2014) 140(3):774–815. doi:10.1037/a0035302
64. Gold PW. The organization of the stress system and its dysregulation in depressive illness. Mol Psychiatry (2015) 20(1):32–47. doi:10.1038/mp.2014.163
65. Rieder R, Wisniewski PJ, Alderman BL, Campbell SC. Microbes and mental health: a review. Brain Behav Immun (2017) 66:9–17. doi:10.1016/j.bbi.2017.01.016
66. Crupi R, Cuzzocrea S. Neuroinflammation and immunity: a new pharmacological target in depression. CNS Neurol Disord Drug Targets (2016) 15(4):464–76. doi:10.2174/1871527315666160321105339
67. Pereira MR, Leite PE. The involvement of parasympathetic and sympathetic nerve in the inflammatory reflex. J Cell Physiol (2016) 231(9):1862–9. doi:10.1002/jcp.25307
68. Bellavance MA, Rivest S. The HPA – immune axis and the immunomodulatory actions of glucocorticoids in the brain. Front Immunol (2014) 5:136. doi:10.3389/fimmu.2014.00136
70. Willemze RA, Luyer MD, Buurman WA, de Jonge WJ. Neural reflex pathways in intestinal inflammation: hypotheses to viable therapy. Nat Rev Gastroenterol Hepatol (2015) 12(6):353–62. doi:10.1038/nrgastro.2015.56
71. Borovikova LV, Ivanova S, Zhang M, Yang H, Botchkina GI, Watkins LR, et al. Vagus nerve stimulation attenuates the systemic inflammatory response to endotoxin. Nature (2000) 405(6785):458–62. doi:10.1038/35013070
72. Browning KN, Verheijden S, Boeckxstaens GE. The vagus nerve in appetite regulation, mood, and intestinal inflammation. Gastroenterology (2016) 152(4):730–44. doi:10.1053/j.gastro.2016.10.046
73. Pavlov VA, Tracey KJ. The cholinergic anti-inflammatory pathway. Brain Behav Immun (2005) 19(6):493–9. doi:10.1016/j.bbi.2005.03.015
74. Bonaz B, Sinniger V, Pellissier S. The vagus nerve in the neuro-immune axis: implications in the pathology of the gastrointestinal tract. Front Immunol (2017) 8:1452. doi:10.3389/fimmu.2017.01452
75. Bonaz B, Sinniger V, Pellissier S. Vagus nerve stimulation: a new promising therapeutic tool in inflammatory bowel disease. J Intern Med (2017) 282(1):46–63. doi:10.1111/joim.12611
76. Cryan JF, Dinan TG. Mind-altering microorganisms: the impact of the gut microbiota on brain and behaviour. Nat Rev Neurosci (2012) 13(10):701–12. doi:10.1038/nrn3346
77. Galland L. The gut microbiome and the brain. J Med Food (2014) 17(12):1261–72. doi:10.1089/jmf.2014.7000
78. Dinan TG, Cryan JF. The microbiome-gut-brain axis in health and disease. Gastroenterol Clin North Am (2017) 46(1):77–89. doi:10.1016/j.gtc.2016.09.007
79. Fung TC, Olson CA, Hsiao EY. Interactions between the microbiota, immune and nervous systems in health and disease. Nat Neurosci (2017) 20(2):145–55. doi:10.1038/nn.4476
80. Forsythe P, Bienenstock J, Kunze WA. Vagal pathways for microbiome-brain-gut axis communication. Adv Exp Med Biol (2014) 817:115–33. doi:10.1007/978-1-4939-0897-4_5
81. Miller BR, Hen R. The current state of the neurogenic theory of depression and anxiety. Curr Opin Neurobiol (2015) 30:51–8. doi:10.1016/j.conb.2014.08.012
82. Oleson T. Auriculotherapy Manual. 3rd ed. Los Angeles: Churchill Livingstone: Elsevier Limited (2003).
83. Usichenko T, Hacker H, Lotze M. Transcutaneous auricular vagal nerve stimulation (taVNS) might be a mechanism behind the analgesic effects of auricular acupuncture. Brain Stimul (2017) 10(6):1042–4. doi:10.1016/j.brs.2017.07.013
84. Yakunina N, Kim SS, Nam EC. Optimization of transcutaneous vagus nerve stimulation using functional MRI. Neuromodulation (2016) 20(3):290–300. doi:10.1111/ner.12541
85. Han JS. Acupuncture: neuropeptide release produced by electrical stimulation of different frequencies. Trends Neurosci (2003) 26:17–22. doi:10.1016/S0166-2236(02)00006-1
86. Zhang WT, Jin Z, Cui GH, Zhang KL, Zhang L, Zeng YW, et al. Relations between brain network activation and analgesic effect induced by low vs. high frequency electrical acupoint stimulation in different subjects: a functional magnetic resonance imaging study. Brain Res (2003) 982:168–78. doi:10.1016/S0006-8993(03)02983-4
87. Wang XY, Shang HY, He W, Shi H, Jing XH, Zhu B. [Effects of transcutaneous electrostimulation of auricular concha at different stimulating frequencies and duration on acute seizures in epilepsy rats]. Zhen Ci Yan Jiu (2012) 37(6):447–52.
88. Bauer S, Baier H, Baumgartner C, Bohlmann K, Fauser S, Graf W, et al. Transcutaneous vagus nerve stimulation (tVNS) for treatment of drug-resistant epilepsy: a randomized, double-blind clinical trial (cMPsE02). Brain Stimul (2016) 9(3):356–63. doi:10.1016/j.brs.2015.11.003
89. Dersh J, Gatchel RJ, Mayer T, Polatin P, Temple OR. Prevalence of psychiatric disorders in patients with chronic disabling occupational spinal disorders. Spine (Phila Pa 1976) (2006) 31(10):1156–62. doi:10.1097/01.brs.0000216441.83135.6f
90. Von Korff M, Crane P, Lane M, Miglioretti DL, Simon G, Saunders K, et al. Chronic spinal pain and physical-mental comorbidity in the United States: results from the national comorbidity survey replication. Pain (2005) 113(3):331–9. doi:10.1016/j.pain.2004.11.010
91. Carney RM, Freedland KE. Depression and coronary heart disease. Nat Rev Cardiol (2017) 14(3):145–55. doi:10.1038/nrcardio.2016.181
92. Aydin Sunbul E, Sunbul M, Gulec H. The impact of major depression on heart rate variability and endothelial dysfunction in patients with stable coronary artery disease. Gen Hosp Psychiatry (2017) 44:4–9. doi:10.1016/j.genhosppsych.2016.10.006
93. Abautret-Daly A, Dempsey E, Parra-Blanco A, Medina C, Harkin A. Gut-brain actions underlying comorbid anxiety and depression associated with inflammatory bowel disease. Acta Neuropsychiatr (2017) 8:1–22. doi:10.1017/neu.2017.3
94. Lee C, Doo E, Choi JM, Jang SH, Ryu HS, Lee JY, et al. The increased level of depression and anxiety in irritable bowel syndrome patients compared with healthy controls: systematic review and meta-analysis. J Neurogastroenterol Motil (2017) 23(3):349–62. doi:10.5056/jnm16220
Keywords: vagus nerve, transcutaneous vagus nerve stimulation, transcutaneous auricular vagus nerve stimulation, depression, brain network, anti-inflammation
Citation: Kong J, Fang J, Park J, Li S and Rong P (2018) Treating Depression with Transcutaneous Auricular Vagus Nerve Stimulation: State of the Art and Future Perspectives. Front. Psychiatry 9:20. doi: 10.3389/fpsyt.2018.00020
Received: 03 October 2017; Accepted: 18 January 2018;
Published: 05 February 2018
Edited by:
Renerio Fraguas, University of São Paulo, BrazilReviewed by:
Bruno Bonaz, Grenoble Faculty of Medicine and Hospital, FranceChrista McIntyre, The University of Texas at Dallas, United States
Copyright: © 2018 Kong, Fang, Park, Li and Rong. This is an open-access article distributed under the terms of the Creative Commons Attribution License (CC BY). The use, distribution or reproduction in other forums is permitted, provided the original author(s) and the copyright owner are credited and that the original publication in this journal is cited, in accordance with accepted academic practice. No use, distribution or reproduction is permitted which does not comply with these terms.
*Correspondence: Peijing Rong, cm9uZ3BqJiN4MDAwNDA7bWFpbC5jaW50Y20uYWMuY24=, ZHJyb25ncGomI3gwMDA0MDsxNjMuY29t