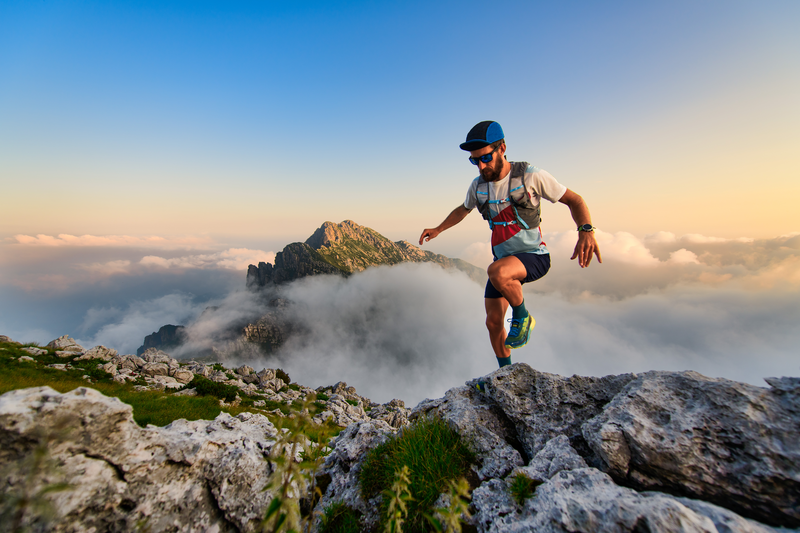
95% of researchers rate our articles as excellent or good
Learn more about the work of our research integrity team to safeguard the quality of each article we publish.
Find out more
PERSPECTIVE article
Front. Psychiatry , 13 May 2016
Sec. Neuroimaging
Volume 7 - 2016 | https://doi.org/10.3389/fpsyt.2016.00085
This article is part of the Research Topic Neural mechanisms of posttraumatic stress disorder View all 7 articles
Mild traumatic brain injury (mTBI), or concussion, is among the most common injuries affecting Veterans of recent combat deployments. Military mTBI differs from civilian mTBI in fundamental ways that make assessment and diagnosis difficult, including a reliance on retrospective self-report and the potential influence of comorbid psychopathology. These unique features and their implications for research and clinical practice are summarized, and neuroimaging studies are discussed in the context of these complicating factors.
Mild traumatic brain injury (mTBI), or concussion, has been deemed the “signature injury” of Operation Enduring Freedom and Operation Iraqi Freedom (OEF/OIF) affecting 15–25% of American military service members deployed to these recent conflicts (1–3). Approximately 75% of these mTBI events have involved exposure to explosive blast (4, 5), which may have unique injury mechanisms (6, 7). Furthermore, even outside of deployments, mTBI is more common among military service members than civilians (3). Consequently, there has been substantial interest recently, both from clinical and scientific perspectives, in the identification of Service Members who have sustained mTBI to determine whether there are long-term effects. However, these efforts have been complicated by several unique features of military mTBI, such as reliance on retrospective self-report, overlap with symptoms of posttraumatic stress disorder (PTSD) and depression, and variability in assessment approaches. This manuscript presents an overview of some of these considerations, with brief discussions of their impact on clinical care and neuroimaging research.
According to the definition established by the American Congress of Rehabilitation Medicine (8), mTBI is characterized by a blow to the head (e.g., striking an object, being struck) accompanied by evidence of physiological disruption of brain function [i.e., loss of consciousness (LOC) less than 30 min, altered mental state (AMS), posttraumatic amnesia (PTA) less than 24 h, and neurological deficits]. While the major diagnostic symptoms of LOC, AMS, and PTA are generally limited in duration to the mTBI event itself, some physical (e.g., headaches and sleep disturbance), behavioral (e.g., irritability and disinhibition), and cognitive (e.g., difficulty in concentrating and memory problems) symptoms can persist for several days or weeks. These postconcussive symptoms (PCS) generally resolve gradually over time and, for the majority of individuals, are completely remitted within 3 months (9). However, a substantial subset of individuals report persistent PCS lasting several years or longer (10). Persistent PCS tend to be non-specific, correlate with anxiety and depression symptoms (11), and do not correspond well to objective neurological measures (12), leading to the hypothesis of a psychological rather than neurological basis. However, persistent PCS, PTSD, and depression are all risk factors for degenerative conditions, such as cerebral atrophy and chronic traumatic encephalopathy, raising the possibility that long-term effects of mTBI, including the relationship between PCS and psychopathology, are more complex (13).
Perhaps, the most striking differences between civilian and military mTBI are those inherent to the diagnostic process itself. Three elements in particular make the diagnosis of military mTBI unique: temporal proximity to the event, goals of establishing a diagnosis, and influence of trauma during and after the event (see Table 1). Approximately 60–75% of civilian mTBI events are reported to medical professionals shortly afterward (14, 15), creating a record proximal to the event. Diagnosis of mTBI, even in the hours and days following an event, involves clinical judgment and can be complicated by symptoms of acute stress disorder (16), delayed emergence of PCS, and individual differences in reporting; however, these factors are balanced by recency of memory and potential availability of witnesses. In contrast, documentation of military mTBI in theater has historically been rare and has only improved slightly since implementation of electronic medical records in 2010 (17). Therefore, diagnosis of military mTBI relies heavily on retrospective self-report of events that were traumatic in nature and involved states of altered consciousness and/or amnesia. When corroborating accounts are incorporated from independent witnesses, information is often still recounted through the individual being assessed (e.g., “My friend says I was unconscious for about 3 minutes”), limiting the independence of the source (18, 19). Lack of documentation at time of injury is largely due to reduced seeking of medical care for several reasons, including partial resolution of symptoms by the time access is possible (e.g., upon returning from patrol), fear of unnecessary reduction in duty, and reduced awareness of acute symptoms in the context of stressful events. In addition to changing the nature of the diagnostic process, it is possible that differences in acute care (e.g., rest) have effects on recovery, neurobiological processes, and long-term outcomes.
While there are obvious limitations to relying on retrospective accounts of events that occurred months or years previously, there are also more subtle consequences. The primarily retrospective approach to diagnosis will naturally overestimate the prevalence of persistent PCS relative to a prospective approach due to selection bias. To improve identification of veterans who experienced deployment-related mTBI, the Veterans Health Administration (VHA) developed a TBI clinical reminder screening questionnaire to be administered to all OEF/OIF Veterans seeking care at a VHA medical facility (20), with positive screens referred to specialists for formal mTBI assessment. While the merits and effectiveness of this strategy have been debated in depth elsewhere [e.g., Ref. (21)], it has highlighted the question of what the goal of retrospective diagnosis should be. In the acute phase of mTBI, diagnostic assessment of symptoms can be used to determine severity and make judgments about reductions in activities, return to work or play, and monitoring of course. In this sense, early diagnosis has the goal of limiting underlying neurodegenerative processes and speeding recovery, and following full recovery the diagnosis is typically considered historical rather than ongoing. Retrospective diagnoses conducted months or years later cannot achieve the goal of limiting damage, and the value of an historical mTBI diagnosis has not been established. While it may be beneficial to identify veterans who are experiencing persistent PCS or who may be at risk for progressive neurodegeneration (13), the discrepancy between the two diagnostic approaches limits the generalization of scientific knowledge and clinical practice between civilian and military contexts.
Finally, military mTBI is distinct from civilian mTBI in terms of context, specifically, the prominence of psychological trauma. While civilian mTBI often involves highly stressful or potentially life-threatening contexts (e.g., motor vehicle accidents and assaults), the need for immediate action (i.e., during acute symptoms) to remove oneself from further harm is rare. In contrast, military mTBI events, 75% of which involve explosive blasts (e.g., mortars and roadside bombs), often require the person to respond quickly by removing themselves or others from harm, assess damage or casualties, secure equipment, identify additional threats, and respond according to their military operational specialty (22). Consequently, it can be difficult for a clinician to differentiate confusion due to altered consciousness from confusion inherent to an ambiguous threatening situation, especially when based on retrospective report (17, 19). Furthermore, due to immediate need for action, often relying on reflexive heavily trained behaviors, the individual may not be aware of acute symptoms until much later or may attribute these symptoms to the stressful situation rather than biological insults, further contributing to low rates of mTBI reporting proximal to the event.
Conversely, a high rate of comorbidity between mTBI and PTSD has been consistently reported among recent veterans. In an early and prominent example of this relationship, Hoge et al. (1) demonstrated that 43.9% of the OIF Soldiers who reported mTBI with LOC met criteria for PTSD, compared to 27.3% of those reporting mTBI without LOC, 16.2% of those reporting non-mTBI injuries, and 9.1% of uninjured Soldiers. It has also been consistently reported that the association between mTBI and higher levels of PCS is no longer significant after accounting for PTSD and/or depression symptoms (1, 11, 23, 24), suggesting a psychological rather than physiological basis for persistent PCS. In addition to making the distinction of PTSD from chronic effects of mTBI even more difficult, this relationship raises the possibility that the experience of PTSD affects diagnosis of mTBI either directly or indirectly, such as by misattribution of symptoms by the clinician or the patient. Two recent independent reports investigating inconsistency of reported mTBI experiences between the end of a deployment and 6–9 months later both determined that higher PTSD symptom levels correspond to increased likelihood of inconsistent mTBI reporting, specifically changing from no initial report of mTBI to later endorsement of such experiences (25, 26). Taken together with the typical retrospective nature of diagnosis and high rate of comorbidity with PTSD, the demonstration that mTBI reporting changes over time raises concerns about reliability of military mTBI diagnosis.
In response to the challenges posed by military mTBI diagnosis, particularly the reliability of retrospective self-report and influence of psychopathology, there has been interest in determining whether specific elements of the mTBI event uniquely relate to outcomes and underlying neuropathology. Exposure to explosive blast has received substantial attention in this regard due to its specificity to military combat and evidence from animal models demonstrating unique biophysical damage mechanisms relative to traditional acceleration–deceleration forces involved in civilian injuries (4, 6, 7). Blast exposure is particularly common among OEF/OIF veterans due to the prominence of improvised explosive devices, combined with advanced body protection improving survival rates (3, 22). While blast exposure is associated with increased rates of PTSD (3), especially the re-experiencing symptom domain (27), it is also associated with higher rates of combat exposure (28, 29), but not with more persistent PCS (5), suggesting that blast exposure may be psychologically rather than physiologically traumatic.
Loss of consciousness is another logical candidate as a unique element of mTBI, because it is more objective than AMS, often observed by others, and may be indicative of somewhat greater magnitude of injury. In a pair of studies comparing independent effects of mTBI and LOC on psychological measures, Verfaellie et al. (24, 30) demonstrated a specific association between LOC and emotional PCS, but no relationship of mTBI or LOC with neuropsychological performance; however, neuropsychological performance was associated with anxiety and depression symptoms. Notably, corroborative report was used to make 25 out of the 28 determinations of LOC but was absent in all cases of AMS (18). While this serves to highlight the value of witness report to the assessment of LOC, it also highlights the possibility that the inclusion of third-party report in mTBI assessment may confound the determination of loss vs. alteration of consciousness with overall diagnostic confidence.
Neuroimaging has been used to further characterize the neural underpinnings of mTBI and potential effects of PTSD, LOC, and blast exposure. Based on evidence of diffuse axonal injury associated with moderate and severe TBI (31, 32), diffusion tensor imaging (DTI) and resting state functional magnetic resonance imaging (rsfMRI) have been used extensively to assess structural and functional connectivity, respectively. Briefly, DTI models water diffusivity within each voxel as a tensor of three orthogonal vectors with varying magnitude (33). Because diffusion in white matter is directionally restricted by axon walls, myelin, and other cytoskeletal elements, the two primary DTI measures used to describe white matter integrity are the degree to which diffusion has a single primary orientation [fractional anisotropy (FA)] and the total magnitude of diffusion across the three vectors [mean diffusivity (MD)] (34). In general, healthy white matter is characterized by high FA and low MD (35), and studies of civilian mTBI have typically reported lower FA and higher MD, relative to uninjured controls, in the chronic phase (36–39). Studies of veterans have been more variable, with early studies reporting no differences in FA or MD at the level of individual regions or voxels within deployed veterans (40, 41), although one group reported reduced anisotropy among Service Members medically evacuated for combat injuries including mTBI compared to those whose injuries did not include mTBI (42). Notably, diagnoses of mTBI in the two former studies were made several years after the injury, whereas the latter positive result was based on diagnoses made within 90 days of the mTBI event, though this may reflect recency of injury rather than proximity of the report to the injury. While PTSD symptoms or diagnoses were not explicitly considered in these early reports, subsequent studies that included PTSD symptom severity as a regressor (43) or excluded participants meeting diagnostic criteria for PTSD at the time of study (44) found evidence of lower FA associated with mTBI in widespread white matter. When looking specifically at effects of PTSD within a military mTBI sample, one study reported a negative correlation between FA and PTSD symptom severity (45), whereas another reported higher anisotropy and lower MD associated with PTSD diagnosis (46). A follow-up to the latter demonstrated that both mTBI and PTSD were associated with persistent PCS, but mTBI was associated with fewer regions of abnormal MD while PTSD was associated with more such regions (23). Overall, this pattern of decreased FA across studies suggests that white matter integrity is indeed lower among veterans reporting a history of mTBI, though the association appears to be weaker than in civilian studies, possibly due to the influence of PTSD. While lower FA may represent white matter damage as a consequence of mTBI, additional longitudinal studies are required to establish this interpretation.
Because DTI uses a simple tensor model, it can only account for one fiber orientation per voxel and underestimates anisotropy in voxels containing crossing or diverging fibers. Using a measure of generalized FA (GFA) that accounts for multiple fiber orientations, we previously reported that GFA was more sensitive than FA to effects of PTSD, though neither measure demonstrated an effect of mTBI (46). This improved sensitivity of GFA suggests that crossing and diverging fibers, rather than well-organized tracts, may be particularly involved in PTSD pathology. As advanced measures (e.g., GFA) and techniques (e.g., diffusional kurtosis imaging) become more common, the underlying pathology will likely be clarified further.
Several studies have reported an association between LOC and lower FA, relative to AMS, in widespread white matter regions (18, 47, 48). Similarly, Sorg et al. (49) found that while there was no overall effect of mTBI on FA, a subset of the mTBI group who also demonstrated executive function deficits had lower FA in widespread frontal and subcortical white matter. Furthermore, participants with LOC were more likely to demonstrate executive function deficits and had reduced FA in ventromedial prefrontal white matter, demonstrating a relationship among LOC, executive function, and white matter integrity. This is consistent with reports that LOC is associated with PCS and verbal memory performance through its relationship with white matter integrity even after accounting for PTSD symptoms (18, 48). Finally, one study reported a negative relationship, observed only among veterans with LOC, between a measure of blast load and FA in two subcortical white matter regions, demonstrating an additional dose–response relationship within the more affected group (18). Overall, these results indicate that mTBI involving LOC demonstrates unique relationships with white matter integrity, cognitive function, and symptom expression. Future work to characterize these relationships further, especially their stability over time, has substantial potential to advance understanding of effects of mTBI.
While there have been relatively fewer studies of functional connectivity in military mTBI, two, in particular, have revealed novel features. First, Costanzo et al. (50) reported that left anterior default mode network connectivity (i.e., correlated activity between medial frontal and posterior cingulate cortex) was positively associated with FA of the left cingulum (i.e., the structural pathway between the two functional seeds) and negatively associated with PTSD re-experiencing symptoms, indicating PTSD-related functional disconnection. These associations were observed among veterans with mTBI, but not among veterans without mTBI, suggesting that they represent an emergent property of mTBI neuropathology rather than naturally occurring intersubject variability. Second, Robinson et al. (29) tested a wide range of contrasts involving blast exposure, mTBI symptoms, and injury mechanism (i.e., blast vs. impact) to determine which grouping variable best discriminated cingulate connectivity. Based on comparisons of these tests, the most critical factor was determined to be exposure to a blast at a distance of less than 10 m, regardless of acute mTBI symptoms. Specifically, these results indicate that the presence of the injury mechanism (i.e., close-range blast) may be more critical to understanding the associated neuropathology than the presence of symptoms that are, at least in part, subjective and variable across individuals. These studies demonstrate that functional connectivity may provide valuable information, complementary to that from structural connectivity studies, for the characterization of neuropathology underlying military mTBI.
While there are clear challenges inherent to establishing diagnoses based primarily on retrospective self-report of events that, by definition, involve states of altered consciousness, there are also substantial opportunities to better understand the dynamic relationships among psychological and physiological trauma. Innovative study designs that parse individual elements of the mTBI event, subsequent experiences and symptoms, and underlying traits (e.g., personality, genetic predisposition, and premorbid psychopathology) will be valuable and likely necessary.
The author confirms being the sole contributor of this work and approved it for publication.
The author declares that the research was conducted in the absence of any commercial or financial relationships that could be construed as a potential conflict of interest.
Preparation of this manuscript was supported by a VA Rehabilitation Research and Development Career Development Award (IK2RX000709) to the author. The views expressed are solely those of the author and do not represent the views of the U.S. Department of Veterans Affairs or the United States Government.
1. Hoge CW, McGurk D, Thomas JL, Cox AL, Engel CC, Castro CA, et al. Mild traumatic brain injury in US soldiers returning from Iraq. N Engl J Med (2008) 358(5):453–63. doi:10.1056/NEJMoa072972
2. Terrio H, Brenner LA, Ivins BJ, Cho JM, Helmick K, Schwab K, et al. Traumatic brain injury screening: preliminary findings in a US army Brigade Combat Team. J Head Trauma Rehabil (2009) 24(1):14–23. doi:10.1097/HTR.0b013e31819581d8
3. Warden D. Military TBI during the Iraq and Afghanistan wars. J Head Trauma Rehabil (2006) 21(5):398–402. doi:10.1097/00001199-200609000-00004
4. Taber KH, Warden DL, Hurley RA. Blast-related traumatic brain injury: what is known? J Neuropsychiatry Clin Neurosci (2006) 18(2):141–5. doi:10.1176/jnp.2006.18.2.141
5. Wilk JE, Thomas JL, McGurk DM, Riviere LA, Castro CA, Hoge CW. Mild traumatic brain injury (concussion) during combat: lack of association of blast mechanism with persistent postconcussive symptoms. J Head Trauma Rehabil (2010) 25(1):9–14. doi:10.1097/HTR.0b013e3181bd090f
6. Cernak I, Merkle AC, Koliatsos VE, Bilik JM, Luong QT, Mahota TM, et al. The pathobiology of blast injuries and blast-induced neurotrauma as identified using a new experimental model of injury in mice. Neurobiol Dis (2011) 41(2):538–51. doi:10.1016/j.nbd.2010.10.025
7. Magnuson J, Leonessa F, Ling GSF. Neuropathology of explosive blast traumatic brain injury. Curr Neurol Neurosci Rep (2012) 12(5):570–9. doi:10.1007/s11910-012-0303-6
8. Kay T, Harrington DE, Adams RE, Anderson TW, Berrol S, Cicerone K. Definition of mild traumatic brain injury: report from the Mild Traumatic Brain Injury Committee of the Head Injury Interdisciplinary Special Interest Group of the American Congress of Rehabilitation Medicine. J Head Trauma Rehabil (1993) 8(3):86–7. doi:10.1097/00001199-199309000-00010
9. Karr JE, Areshenkoff CN, Garcia-Barrera MA. The neuropsychological outcomes of concussion: a systematic review of meta-analyses on the cognitive sequelae of mild traumatic brain injury. Neuropsychology (2014) 28(3):321–36. doi:10.1037/neu0000037
10. Ruff RM, Camenzuli L, Mueller J. Miserable minority: emotional risk factors that influence the outcome of a mild traumatic brain injury. Brain Inj (1996) 10(8):551–65. doi:10.1080/026990596124124
11. Polusny MA, Kehle SM, Nelson NW, Erbes CR, Arbisi PA, Thuras P. Longitudinal effects of mild traumatic brain injury and posttraumatic stress disorder comorbidity on postdeployment outcomes in National Guard soldiers deployed to Iraq. Arch Gen Psychiatry (2011) 68(1):79–89. doi:10.1001/archgenpsychiatry.2010.172
12. Spencer RJ, Drag LL, Walker SJ, Bieliauskas LA. Self-reported cognitive symptoms following mild traumatic brain injury are poorly associated with neuropsychological performance in OIF/OEF veterans. J Rehabil Res Dev (2010) 47(6):521–30. doi:10.1682/JRRD.2009.11.0181
13. McKee AC, Stern RA, Nowinski CJ, Stein TD, Alvarez VE, Daneshvar DH, et al. The spectrum of disease in chronic traumatic encephalopathy. Brain (2013) 136(Pt 1):43–64. doi:10.1093/brain/aws307
14. Setnik L, Bazarian JJ. The characteristics of patients who do not seek medical treatment for traumatic brain injury. Brain Inj (2007) 21(1):1–9. doi:10.1080/02699050601111419
15. Sosin DM, Sniezek JE, Thurman DJ. Incidence of mild and moderate brain injury in the United States, 1991. Brain Inj (1996) 10(1):47–54. doi:10.1080/026990596124719
16. Harvey AG, Bryant RA. Acute stress disorder: a synthesis and critique. Psychol Bull (2002) 128(6):886–902. doi:10.1037/0033-2909.128.6.886
17. Fortier CB, Amick MM, Grande L, McGlynn S, Kenna A, Morra L, et al. The Boston assessment of traumatic brain injury-lifetime (BAT-L) semistructured interview. J Head Trauma Rehabil (2014) 29(1):89–98. doi:10.1097/HTR.0b013e3182865859
18. Hayes JP, Miller DR, Lafleche G, Salat DH, Verfaellie M. The nature of white matter abnormalities in blast-related mild traumatic brain injury. Neuroimage Clin (2015) 8:148–56. doi:10.1016/j.nicl.2015.04.001
19. Walker WC, Cifu DX, Hudak AM, Goldberg G, Kunz RD, Sima AP. Structured interview for mild traumatic brain injury after military blast: inter-rater agreement and development of diagnostic algorithm. J Neurotrauma (2015) 32(7):464–73. doi:10.1089/neu.2014.3433
20. Belanger HG, Vanderploeg RD, Sayer N. Screening for remote history of mild traumatic brain injury in VHA: a critical literature review. J Head Trauma Rehabil (2015):1–10. doi:10.1097/HTR.0000000000000168
21. Vanderploeg RD, Belanger HG. Screening for a remote history of mild traumatic brain injury: when a good idea is bad. J Head Trauma Rehabil (2013) 28(3):211–8. doi:10.1097/HTR.0b013e31828b50db
22. Moore DF, Jaffee MS. Military traumatic brain injury and blast. NeuroRehabilitation (2010) 26(3):179–81. doi:10.3233/NRE-2010-0553
23. Davenport ND, Lim KO, Sponheim SR. Personality and neuroimaging measures differentiate PTSD from mTBI in veterans. Brain Imaging Behav (2015) 9(3):472–83. doi:10.1007/s11682-015-9371-y
24. Verfaellie M, Lafleche G, Spiro A, Tun C, Bousquet K. Chronic postconcussion symptoms and functional outcomes in OEF/OIF veterans with self-report of blast exposure. J Int Neuropsychol Soc (2013) 19(1):1–10. doi:10.1017/S1355617712000902
25. Alosco ML, Aslan M, Du M, Ko J, Grande L, Proctor SP, et al. Consistency of recall for deployment-related traumatic brain injury. J Head Trauma Rehabil (2015):1–9. doi:10.1097/HTR.0000000000000201
26. Nelson NW, Anderson CR, Thuras P, Kehle-Forbes SM, Arbisi PA, Erbes CR, et al. Factors associated with inconsistency in self-reported mild traumatic brain injury over time among military personnel in Iraq. Br J Psychiatry (2015) 206(3):1–8. doi:10.1192/bjp.bp.114.149096
27. Kennedy JE, Leal FO, Lewis JD, Cullen MA, Amador RR. Posttraumatic stress symptoms in OIF/OEF service members with blast-related and non-blast-related mild TBI. NeuroRehabilitation (2010) 26(3):223–31. doi:10.3233/NRE-2010-0558
28. Bazarian JJ, Donnelly K, Peterson DR, Warner GC, Zhu T, Zhong J. The relation between posttraumatic stress disorder and mild traumatic brain injury acquired during operations enduring freedom and Iraqi freedom. J Head Trauma Rehabil (2013) 28(1):1–12. doi:10.1097/HTR.0b013e318256d3d3
29. Robinson ME, Lindemer ER, Fonda JR, Milberg WP, McGlinchey RE, Salat DH. Close-range blast exposure is associated with altered functional connectivity in Veterans independent of concussion symptoms at time of exposure. Hum Brain Mapp (2014) 36(3):911–22. doi:10.1002/hbm.22675
30. Verfaellie M, Lafleche G, Spiro A, Bousquet K. Neuropsychological outcomes in OEF/OIF veterans with self-report of blast exposure: associations with mental health, but not mTBI. Neuropsychology (2014) 28(3):337–46. doi:10.1037/neu0000027
31. Hammoud DA, Wasserman BA. Diffuse axonal injuries: pathophysiology and imaging. Neuroimaging Clin N Am (2002) 12(2):205–16. doi:10.1016/S1052-5149(02)00011-4
32. Mittl RL, Grossman RI, Hiehle JF, Hurst RW, Kauder DR, Gennarelli TA, et al. Prevalence of MR evidence of diffuse axonal injury in patients with mild head injury and normal head CT findings. AJNR Am J Neuroradiol (1994) 15(8):1583–9.
33. Basser PJ, Mattiello J, LeBihan D. Estimation of the effective self-diffusion tensor from the NMR spin echo. J Magn Reson B (1994) 103(3):247–54. doi:10.1006/jmrb.1994.1037
34. Basser PJ, Pierpaoli C. Microstructural and physiological features of tissues elucidated by quantitative-diffusion-tensor MRI. J Magn Reson B (1996) 111(3):209–19. doi:10.1006/jmrb.1996.0086
35. Beaulieu C. The basis of anisotropic water diffusion in the nervous system – a technical review. NMR Biomed (2002) 15:435–55. doi:10.1002/nbm.782
36. Aoki Y, Inokuchi R, Gunshin M, Yahagi N, Suwa H. Diffusion tensor imaging studies of mild traumatic brain injury: a meta-analysis. J Neurol Neurosurg Psychiatry (2012) 83(9):870–6. doi:10.1136/jnnp-2012-302742
37. Hulkower MB, Poliak DB, Rosenbaum SB, Zimmerman ME, Lipton ML. A decade of DTI in traumatic brain injury: 10 years and 100 articles later. AJNR Am J Neuroradiol (2013) 34:2064–74. doi:10.3174/ajnr.A3395
38. Niogi SN, Mukherjee P. Diffusion tensor imaging of mild traumatic brain injury. J Head Trauma Rehabil (2010) 25(4):241–55. doi:10.1097/HTR.0b013e3181e52c2a
39. Shenton ME, Hamoda HM, Schneiderman JS, Bouix S, Pasternak O, Rathi Y, et al. A review of magnetic resonance imaging and diffusion tensor imaging findings in mild traumatic brain injury. Brain Imaging Behav (2012) 6(2):137–92. doi:10.1007/s11682-012-9156-5
40. Levin HS, Wilde E, Troyanskaya M, Petersen NJ, Scheibel R, Newsome M, et al. Diffusion tensor imaging of mild to moderate blast-related traumatic brain injury and its sequelae. J Neurotrauma (2010) 27(4):683–94. doi:10.1089/neu.2009.1073
41. Sponheim SR, Mcguire KA, Kang SS, Davenport ND, Aviyente S, Bernat EM, et al. Evidence of disrupted functional connectivity in the brain after combat-related blast injury. Neuroimage (2011) 54:S21–9. doi:10.1016/j.neuroimage.2010.09.007
42. MacDonald CL, Johnson AM, Cooper D, Nelson EC, Werner NJ, Shimony JS, et al. Detection of blast-related traumatic brain injury in U.S. military personnel. N Engl J Med (2011) 364(22):2091–100. doi:10.1056/NEJMoa1008069
43. Morey RA, Haswell CC, Selgrade ES, Massoglia D, Liu C, Weiner J, et al. Effects of chronic mild traumatic brain injury on white matter integrity in Iraq and Afghanistan war veterans. Hum Brain Mapp (2013) 34(11):2986–99. doi:10.1002/hbm.22117
44. Davenport ND, Lim KO, Armstrong MT, Sponheim SR. Diffuse and spatially variable white matter disruptions are associated with blast-related mild traumatic brain injury. Neuroimage (2012) 59(3):2017–24. doi:10.1016/j.neuroimage.2011.10.050
45. Yeh P-H, Wang B, Oakes TR, French LM, Pan H, Graner J, et al. Postconcussional disorder and PTSD symptoms of military-related traumatic brain injury associated with compromised neurocircuitry. Hum Brain Mapp (2014) 35(6):2652–73. doi:10.1002/hbm.22358
46. Davenport ND, Lim KO, Sponheim SR. White matter abnormalities associated with military PTSD in the context of blast TBI. Hum Brain Mapp (2015) 36:1053–64. doi:10.1002/hbm.22685
47. Matthews SC, Spadoni AD, Lohr JB, Strigo IA, Simmons AN. Diffusion tensor imaging evidence of white matter disruption associated with loss versus alteration of consciousness in warfighters exposed to combat in Operations Enduring and Iraqi Freedom. Psychiatry Res (2012) 204(2–3):149–54. doi:10.1016/j.pscychresns.2012.04.018
48. Miller DR, Hayes JP, Lafleche G, Salat DH, Verfaellie M. White matter abnormalities are associated with chronic postconcussion symptoms in blast-related mild traumatic brain injury. Hum Brain Mapp (2015) 37(1):220–9. doi:10.1002/hbm.23022
49. Sorg SF, Delano-Wood L, Luc N, Schiehser DM, Hanson KL, Nation DA, et al. White matter integrity in veterans with mild traumatic brain injury: associations with executive function and loss of consciousness. J Head Trauma Rehabil (2013) 29(1):21–32. doi:10.1097/HTR.0b013e31828a1aa4
Keywords: mTBI, concussion, military, neuroimaging
Citation: Davenport ND (2016) The Chaos of Combat: An Overview of Challenges in Military Mild Traumatic Brain Injury Research. Front. Psychiatry 7:85. doi: 10.3389/fpsyt.2016.00085
Received: 24 February 2016; Accepted: 02 May 2016;
Published: 13 May 2016
Edited by:
Paul Croarkin, Mayo Clinic, USAReviewed by:
Niels Bergsland, Buffalo Neuroimaging Analysis Center, USACopyright: © 2016 Davenport. This is an open-access article distributed under the terms of the Creative Commons Attribution License (CC BY). The use, distribution or reproduction in other forums is permitted, provided the original author(s) or licensor are credited and that the original publication in this journal is cited, in accordance with accepted academic practice. No use, distribution or reproduction is permitted which does not comply with these terms.
*Correspondence: Nicholas D. Davenport, bmljaG9sYXMuZGF2ZW5wb3J0QHZhLmdvdg==
Disclaimer: All claims expressed in this article are solely those of the authors and do not necessarily represent those of their affiliated organizations, or those of the publisher, the editors and the reviewers. Any product that may be evaluated in this article or claim that may be made by its manufacturer is not guaranteed or endorsed by the publisher.
Research integrity at Frontiers
Learn more about the work of our research integrity team to safeguard the quality of each article we publish.