- 1Faculty of Medicine of Lisbon, Instituto de Medicina Molecular, University of Lisbon, Lisbon, Portugal
- 2Faculty of Medicine of Lisbon, Institute of Pharmacology and Neurosciences, University of Lisbon, Lisbon, Portugal
- 3CNC-Center for Neurosciences and Cell Biology, University of Coimbra, Coimbra, Portugal
- 4Faculty of Medicine, University of Coimbra, Coimbra, Portugal
- 5Max-Delbrück-Center for Molecular Medicine (MDC), Berlin, Germany
Adenosine A2A receptors (A2AR) are a sub-type of receptors enriched in basal ganglia, activated by the neuromodulator adenosine, which interact with dopamine D2 receptors. Although this reciprocal antagonistic interaction is well-established in motor function, the outcome in dopamine-related behaviors remains uncertain, in particular in depression and anxiety. We have demonstrated an upsurge of A2AR associated to aging and chronic stress. Furthermore, Alzheimer’s disease patients present A2AR accumulation in cortical areas together with depressive signs. We now tested the impact of overexpressing A2AR in forebrain neurons on dopamine-related behavior, namely depression. Adult male rats overexpressing human A2AR under the control of CaMKII promoter [Tg(CaMKII-hA2AR)] and aged-matched wild-types (WT) of the same strain (Sprague-Dawley) were studied. The forced swimming test (FST), sucrose preference test (SPT), and the open-field test (OFT) were performed to evaluate behavioral despair, anhedonia, locomotion, and anxiety. Tg(CaMKII-hA2AR) animals spent more time floating and less time swimming in the FST and presented a decreased sucrose preference at 48 h in the SPT. They also covered higher distances in the OFT and spent more time in the central zone than the WT. The results indicate that Tg(CaMKII-hA2AR) rats exhibit depressive-like behavior, hyperlocomotion, and altered exploratory behavior. This A2AR overexpression may explain the depressive signs found in aging, chronic stress, and Alzheimer’s disease.
Introduction
Adenosine is a purine nucleoside, which acts as neuromodulator in several brain areas, playing important fine tuning influences on other neurotransmitters (1). It has an important role in central nervous system and its involvement in a wide range of brain processes and diseases has been researched, namely sleep (2, 3), epilepsy (4, 5), panic disorder (6), anxiety (7), Alzheimer’s disease (8), Parkinson’s disease (9), and schizophrenia (10).
So far, four adenosine receptors have been cloned and characterized: A1R, adenosine A2A receptor (A2AR), A2BR, and A3R. A1R and A3R are coupled to Gi proteins, inhibiting cAMP production; A2AR is coupled to Gs proteins, stimulating cAMP production; A2BR is coupled to Gs and to Gq proteins, stimulating cAMP production, and phosphatidylinositol signal pathway activation, respectively (11). These receptors are not uniformly distributed in the central nervous system. A1R is highly expressed in brain cortex, cerebellum, hippocampus, and dorsal horn of spinal cord (1, 11, 12). A2AR is highly expressed in the olfactory bulb and in the GABAergic neurons of caudate–putamen, nucleus accumbens, and tuberculum olfactorium (1, 11). A2BR and A3R are also present in the brain, however, in low levels (11).
Adenosine A2A receptor activation influences the function of several receptors, but the interaction with dopamine D2 receptor (D2R) is one the most intensively studied (1). Dopamine is a catecholamine neurotransmitter. It activates five known types of receptors, D1R–D5R, which may be grouped in D1-like receptors – D1R and D5R – and D2-like receptors – D2R, D3R, and D4R (13). Dopaminergic neurons are mostly localized in the arcuate nucleus of hypothalamus, substantia nigra pars compacta, and ventral tegmental area. From the substantia nigra–ventral tegmental area complex, three distinct dopamine projection pathways are formed (14). In one of them, axons project to cortical areas, particularly to the frontal cortex, forming the classically described mesocortical pathway. In another pathway, classically known as mesolimbic pathway, axons project to the nucleus accumbens, amygdala, and hippocampus. Due to their functional interrelationships, these two pathways are commonly referred as a single system – the mesocorticolimbic system (15). This system is involved in emotional response, motivation, reward, addiction, and learning. Its role has been emphasized in the pathophysiology of schizophrenia, depression, and drug addiction, and it appears also to be implicated in anxiety disorders (16–20). In the third pathway, axons project to the striatum, forming the classically described nigrostriatal pathway. This pathway integrates the neural circuits of the basal ganglia responsible for motor control and its malfunctioning is classically involved in the pathophysiology of Parkinson’s disease (21), although recent evidence also points to a very important role in the motor changes associated with severe depression (22).
Adenosine A2A receptor and D2R are co-localized in the dorsal and ventral striatum and are reciprocal inhibitors: on one hand, A2AR–D2R heteromers are formed and, when the A2AR is activated, conformational changes are transferred to the D2R – this leads to a reduction in D2R recognition and signaling (23, 24); on the other hand, D2R activation inhibits cAMP mediated-effects of A2AR by inhibiting adenylyl-cyclase (23, 24).
Knowing these possible interactions between A2AR and dopamine, we investigated the impact of A2AR overexpression in cortical areas onto dopamine-related behavior. Thus, in the present work a group of behavioral tests was performed in order to analyze the effect of A2AR overexpression: (1) sucrose preference test (SPT), considered for a behavioral evaluation of anhedonia (25); (2) forced swim test to evaluate motivation and behavioral despair (26, 27); (3) open-field test (OFT) to study locomotor activity and anxiety-like behavior (28, 29).
Materials and Methods
Animals
Animal procedures were performed in accordance with the guidelines of the European Community guidelines (Directive 2010/63/EU), Portuguese law on animal care (1005/92), and approved by the Instituto de Medicina Molecular Internal Committee and the Portuguese Animal Ethics Committee (Direcção Geral de Veterinária). Transgenic rats with an overexpression of the human A2AR under the control of the CaMKII promotor, tg(CaMKII-hA2AR), were generated by microinjection of a linearized DNA construct into the male pronucleus of Sprague-Dawley rat zygotes with established methods (30). The construct contained a full-length human A2A cDNA cloned into an expression vector with the 8.5 kb mouse CaMKIIα promoter (31) and a polyadenylation cassette of bovine growth hormone (see Figure 1, top panel). Sprague-Dawley wild-type (WT) rats were used as controls. Genotyping: transgenic rats were identified by PCR (30 cycles, 58°C annealing temperature) of their genomic DNA isolated from ear biopsies by the use of the transgene-specific primers CaMKII-hA2A and rat β-actin primers as an internal control (Invitrogen). According to the performed RNase protection assay, these animals expressed A2AR pre-dominantly in the brain. qPCR and Western blotting showed that the overexpression was mostly in hippocampus and cortex; there was also overexpression in striatum, however at a lesser extent (Figure 1). Nine week-old WT and transgenic Sprague-Dawley (CaMKII-hA2AR) male rats were used. They were maintained in groups of three in appropriate cages with food and water ad libitum, temperature of 21 ± 0.5°C, humidity of 60 ± 10%, and 12 h light/dark cycles beginning at 8 a.m.
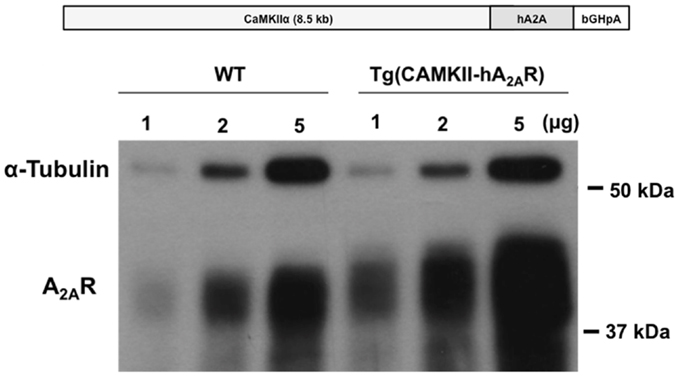
Figure 1. Neuronal overexpression of adenosine A2A receptor (A2AR) in Tg(CaMII-hA2AR) rats. A2AR overexpression in the striatum of Tg(CaMKII-hA2AR) rats compared to WT animals was confirmed by western blotting.
Behavioral Testing
The behavioral testing was performed as before (32), during the light period of the cycle, in a silent room, under dim light. From the first to the third day of experiments, the animals were handled for approximately 1 min each. On the fourth day, at 2.30 p.m. the OFT was done. On the fifth day, at 1 p.m. the SPT was initiated. This test was concluded on the seventh day at 9 a.m. On the seventh and eighth day, both at 2 p.m., the forced swim test was executed.
Open-field Test
The rats were placed in a designated corner of a square apparatus, surrounded by vertical walls (66 cm × 66 cm × 66 cm) – open-field arena. They freely explored the maze for 5 min. Their movements were recorded and analyzed using the video-tracking software – SMART®. The reference point used by the software to determine the position of the animal was the center of the rat’s dorsum (also true for the other experiments). Three different zones were defined for analysis (29): (1) the area adjacent to the wall (1896 cm2); (2) the central area of the arena (552 cm2); (3) the intermediary area between the two previous ones (1908 cm2). The percentage of time spent in each zone, the total distance traveled, the average speed (calculated after the elimination of the resting time), and the number of rearings and defecations were determined. At the end of the 5 min test, the rat was removed from the open-field arena and placed into its home cage.
Elevated plus maze
The maze is shaped like a plus sign and consists of two “open” (no walls, 5 cm × 29 cm) and two “closed” 122 (5 cm × 29 cm × 15 cm) arms, arranged perpendicularly, and elevated 50 cm above the floor. Each animal was placed on the center of the equipment, facing an open arm. Each test lasted 5 min and all testing sessions were performed between 10:00 a.m. and 17:00 p.m. in a sound attenuated room. The maze was cleaned with a 70% ethanol solution between each animal. The total time spent in the open arms and the total arms entries (number of entries in open + closed arms) were used as anxiety and locomotor parameters as before (32).
Sucrose preference test
Rats were given two previously weighed bottles: with 1% (w/v) sucrose solution (33). The bottles had the same characteristics and approximately the same volume of liquid and were positioned side-by-side at the rear of the cage. The rats had free access to both bottles. The position of the bottles in the cage is switched halfway through this period. There was no food or water deprivation before the test. The bottles were weighed again at 48 h and the consumed weight of each liquid was determined. The sucrose preference was calculated according to Bekris et al. (34):
Forced Swim Test
On the first of the two test days, all animals were gently placed individually in a vertical Plexiglas cylinder (height: 45 cm, diameter: 19 cm) filled with 23°C tap water at a depth that made it impossible for rats to reach the bottom with hind paws (28–30 cm). The animals were removed from the water after 10 min, and dried before being returned to their home cages. The water was changed after each session. On the next day, the procedure was repeated with two differences: the animals were removed from the water after 5 min, instead of 10 min; the session was video-recorded. An observer blinded to the animal group analyzed the videos. Three different behaviors were considered: (1) immobility – according to the criteria of Porsolt et al. (35), a rat is judged to be immobile when it floated passively, making only small movements to keep its nose above the surface; (2) climbing (or thrashing) – upward-directed movements with its forepaws, in and out of the water, along the side of the swim chamber; (3) swimming – active movements (usually horizontal) more than necessary to merely maintain its head above the water (36, 37). Diving and face shaking behaviors were not considered.
The time (t) spent in immobility and climbing was measured; the time spent swimming was calculated: t swimming = 5 − (t climbing + t immobility). Additionally, the latency to the first bout of immobility was determined (38): period of time since the beginning of the rat mobilization in the water until the first episode (at least 1 s) of immobility.
Western blotting
The animals were killed by decapitation after anesthesia under halothane atmosphere. After decapitation the brain was rapidly removed and the striata were dissected rapidly frozen in liquid nitrogen for further analysis. Samples were denatured by heating at 70°C for 30 min for A2AR. Samples and molecular weight marker were resolved by SDS-PAGE (8 or 10% for resolving and a 5% for stacking gels) in denaturing conditions and electro-transferred to PVDF membranes (Millipore). Membranes were blocked with 5% non-fat dry milk in TBS-T (Tris buffer saline with 0.1% Tween-20, 200 nM Tris, 1.5 M NaCl). After washing with TBS-T, membranes were incubated with primary antibody in TBS-T with 3% BSA. Secondary antibody incubation was in 5% non-fat dry milk in TBS-T. Primary antibody was mouse anti-A2AR (1:2000, Upstate/Millipore – 05-717, Darmstadt, Germany), secondary antibodies conjugated with horseradish peroxidase were goat anti-mouse (Santa Cruz Biotechnology, Heidelberg, Germany). Chemoluminescent detection was performed with ECL-PLUS western blotting detection reagent (GE Healthcare) using X-Ray films (Fujifilm).
Statistical Analysis
The software used to perform the statistical analysis was Prism 5 – GraphPad software®. Unpaired t test with Welch’s correction was applied to compare the differences between groups. p < 0.05 was considered as statistical significant. Data are expressed as means ± SEM.
Results
Weight
The weight of transgenic CaMKII-hA2AR rats was significantly lower than WT rats (283 ± 11 vs. 400 ± 5 g; p < 0.001) (Figure 2).
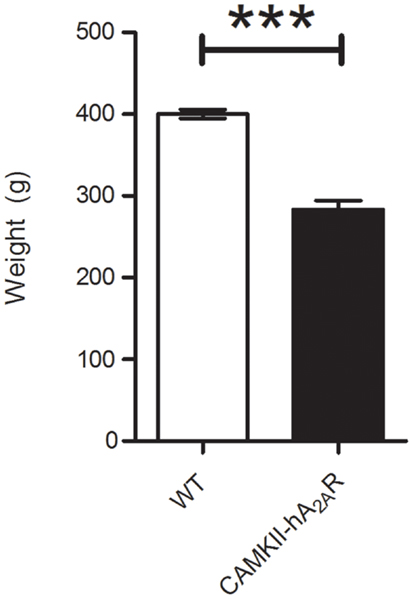
Figure 2. Weight control of Tg(CaMKII-hA2AR) animals. Results are expressed as mean ± SEM. ***p < 0.001 WT: n = 12; Tg(CaMKII-hA2AR): n = 4.
Open-Field Test
The total distance covered in the open-field arena was significantly higher in Tg(CaMKII-hA2AR) rats [WT: 2956 ± 160 cm; Tg(CaMKII-hA2AR): 3644 ± 64 cm; p = 0.0013], and was accompanied by a significant increase in the number of rearings [WT: 5.9 ± 0.7; Tg(CaMKII-hA2AR): 10.8 ± 1.4; p = 0.0390], suggesting that Tg(CaMKII-hA2AR) rats display hyperlocomotion, Figure 3.
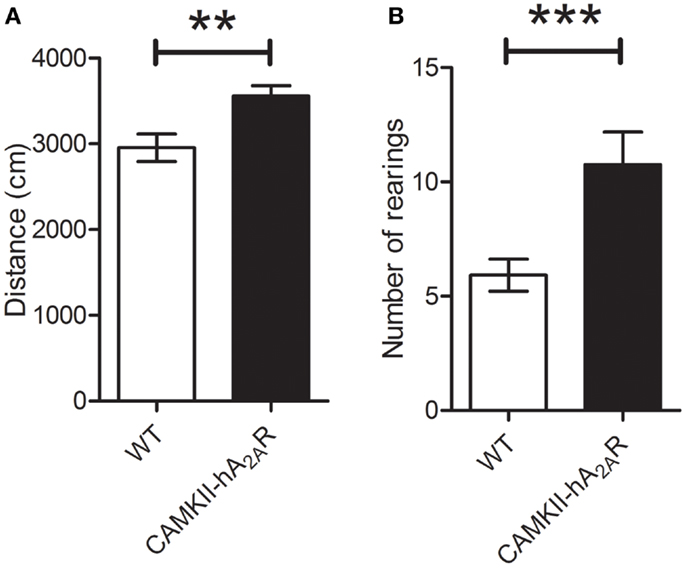
Figure 3. Open-field test. (A) Distance covered in centimeter. (B) Number of rearings – results are expressed as mean ± SEM. **p < 0.01; ***p < 0.001. WT: n = 12; Tg(CAMKII-hA2AR):n = 4.
Transgenic (CaMKII-hA2AR) rats spent less time at the wall zone [WT: 73.97 ± 1.6%; Tg(CaMKII-hA2AR): 61.59 ± 1.94%; p < 0.0001] and more time in the central zone of the open-field box [WT: 3.36 ± 0.48%; Tg(CaMKII-hA2AR): 5.21 ± 0.40%; p = 0.0083, Figure 4] suggesting that Tg(CaMKII-hA2AR) rats have increased exploratory behavior. We could not detect significant changes in the anxious behavior evaluated by EPM (Figure 4C). There were no statistical significant differences regarding the number of defecations and the average speed between the two groups (data not shown).
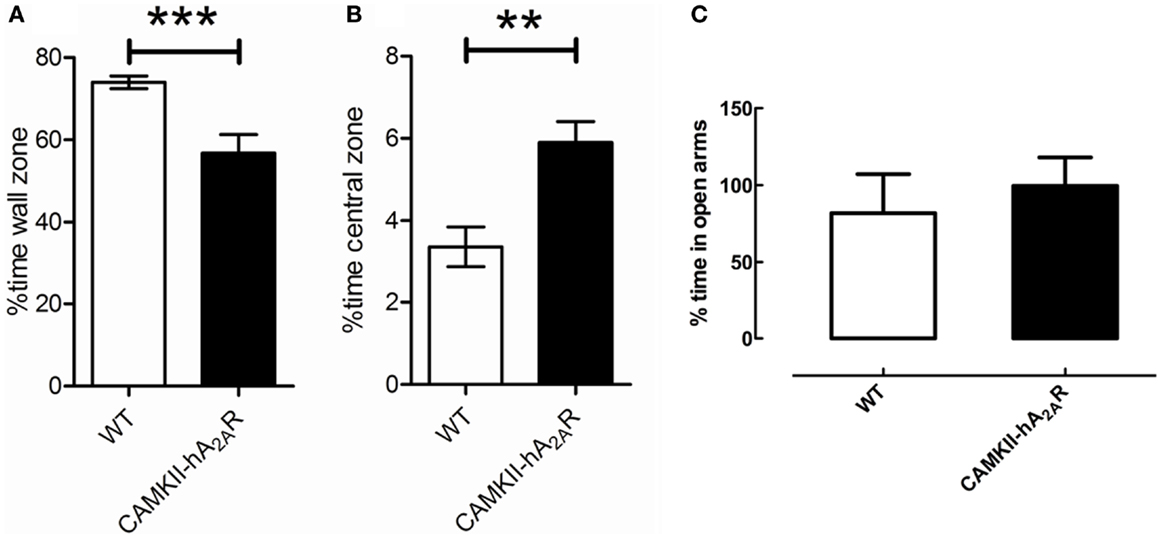
Figure 4. Open-field test. Percentage of time spent (A) at the wall zone and (B) in the central zone. (C) Percentage time in the open arms of the elevated plus maze (EPM). Results are expressed as mean ± SEM. **p < 0.01; ***p < 0.001. WT: n = 12; Tg(CaMKII-hA2AR): n = 4.
Sucrose Preference at 48 h
At 48 h, the preference index for sucrose was significantly higher in WT than in Tg(CaMKII-hA2AR) rats [WT: 91.88 ± 1.4%; Tg(CaMKII-hA2AR): 44.85 ± 23.78%, p = 0.0081, Figure 5], suggesting that Tg(CaMKII-hA2AR) rats have an anhedonic-like phenotype.
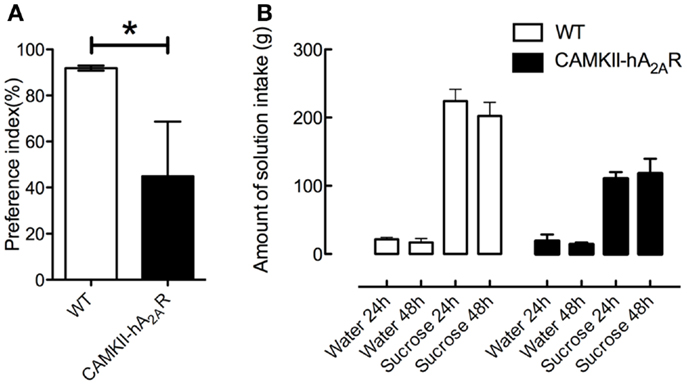
Figure 5. Sucrose preference test. (A) Preference index at 48 h and (B) control of amount of solution intake. Results are expressed as mean ± SEM. p < 0.05 WT: n = 8; Tg(CaMKII-hA2AR):n = 4.
Forced Swim Test
Transgenic (CaMKII-hA2AR) rats spent significantly more time floating than WT rats (2.47 ± 0.18 vs. 3.04 ± 0.16 min, p = 0.0452, Figure 6A) indicating that transgenic animals have increased behavioral despair. No significant changes were apparent in both swimming and climbing times, despite a tendency to lower performance in Tg(CaMKII-hA2AR) animals (Climbing: 0.63 ± 0.08 vs. 0.50 ± 0.02 min, p = 0.1486; swimming: 1.88 ± 0.16 vs. 1.46 ± 0.17 min, p = 0.1060).
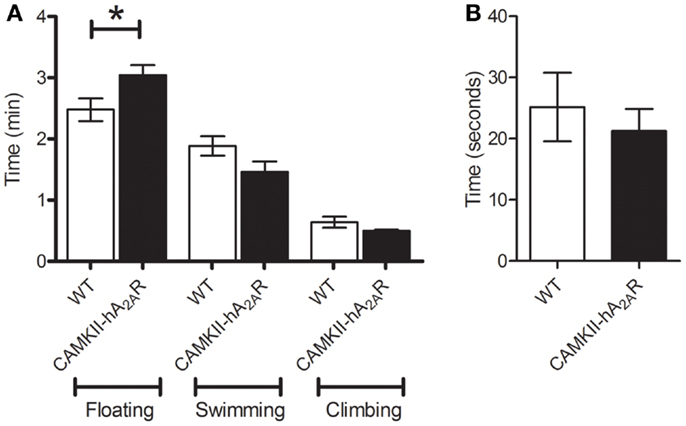
Figure 6. Forced swim test. (A) Time spent floating, swimming, and climbing; (B) latency to the first period of immobility – results are expressed as means ± SEM. p < 0.05 WT: n = 12; Tg(CaMKII-hA2AR):n = 4.
The latency to the first period of immobility was not different between WT and transgenic animals [WT: 25.2 ± 5.6 s; Tg(CaMKII-hA2AR): 26.6 ± 3.8 s; p = 0.8304, Figure 6B].
Discussion
We now report that rats overexpressing A2AR in the hippocampus, cortex and striatum, display depressive-like behavior, increased locomotor activity, and altered exploratory behavior.
Depressive-Like Behavior
The SPT is considered a behavioral test for anhedonia, defined as the inability to feel pleasure from usually enjoyable activities, a core symptom of depression. Decreased sucrose solution intake, resulting from chronic mild stress, is used as a model of depression in rats, and can reversed by the administration of antidepressants (25, 39, 40). Thus, a decreased sucrose preference is associated to depressive-like behavior, specifically anhedonia. In our study, rats overexpressing A2AR, had a decrease in the preference index for sucrose solution at 48 h.
Additionally, when rats are placed in an inescapable cylinder of water – forced swim test – following initial escape-directed movements, they develop an immobile posture (27, 36). Immobility indicates either a failure in the persistence to escape (behavioral despair) or the act of giving up an active form of coping with the stressful stimuli (36). The immobility period and the latency to the first bout of immobility both decrease with the administration of antidepressants (38, 41, 42). Thus, an increased period of immobility and reduced latency to immobility represent depressive-like behavior. A2AR overexpressing animals spent more time floating, while no significant differences were observed in the time spent swimming, climbing, or latency to immobility. These behaviors, again, suggest a depressive-like phenotype.
Genetic inactivation and pharmacological blockade of A2AR have antidepressant-like effects (43). However, selective A2AR agonists are also able to decrease the immobility time in the forced swim test (44, 45). Our observations indicate that A2AR overexpression in neurons results in a depressive-like phenotype. Interestingly, depressive symptoms are found in Alzheimer’s disease patients, which have an abnormal accumulation of A2AR in cortical areas (8).
Locomotor Activity
The increase in locomotor activity displayed by the Tg(CaMKII-hA2AR) rats is in accordance with the hypolocomotor phenotype of mice with genetic deletion of A2AR (46, 47). However, this genetic manipulation does not reflect the effect of acutely administered A2AR agonists that reduce locomotor activity (48, 49). The A2AR–D2R interaction hypothesis of reciprocal inhibition is not suitable to explain the obtained result: with increased amounts of A2AR, we would expect a decreased activation of D2R, which results in hypo-locomotion; and it is known that D2R antagonists suppress locomotion (50, 51).
Anxious-Like Behavior
Since rat is a gregarious animal, which usually lives in small spaces, its separation from its social group and placing in a large arena trigger an anxious behavior. In these situations, they naturally display a propensity to walk close to the walls and to avoid open spaces, a behavior called thigmotaxis. Based on this, it is considered that increased time spent on the central zone of the OFT represents a less anxious behavior (29, 52). Similarly, rats display a pre-disposition toward protected, enclosed areas, which is in conflict with their innate motivation to explore new environments. The Tg(CaMKII-hA2AR)rats spent more time on the central zone and less time on the wall zone of the OFT. This increase in exploratory behavior is not a consequence of an anxious-like phenotype of rats overexpressing A2AR and is in line is in line with previous studies of the other known model of rats overexpressing A2AR receptors in which no difference was found concerning anxiety-like behavior (53). This could also be due to the postulated differences in the cellular origin of A2AR, which has been highlighted recently in a study showing that inactivation of striatal A2AR s facilitates Pavlovian fear conditioning, whereas inactivation of extrastriatal A2ARs in the forebrain inhibits fear conditioning and also affects anxiety-related behavior (54). Also, there is no evidence for anxiogenic or anxiolytic effects of A2AR agonists or antagonists (49, 55).
The A2AR–D2R interaction hypothesis of reciprocal inhibition does not again provide an explanation for our findings: D2R agonists have anxiolytic properties, which are blocked by D2R antagonists (56, 57); consequently, we would expect an increased anxious state in animals overexpressing A2AR. Similar results obtained with animals overexpressing A2AR but controlled by the widespread neuronal promoter enolase (NSE) (53), seem to indicate that the striatal overexpression is not as disturbing as one cortical and hippocampal, dominant in these CaMKII A2A rats.
There could be a mutual influence between anxiety and locomotor activity behaviors. On one hand, the anxiety state can change locomotor activity (58). On the other hand, the anxiety-related tests depend on motor activity (59). However, there is evidence that locomotion and anxiety are differentially regulated by adenosine A2AR: studies using A2AR knockout mice showed that the hypo-locomotion pattern was equal in homozygous and heterozygous mice, as well as in forebrain selective vs. striatal KO, irrespectively of the effects on anxiety (54, 60).
Additional and Integrative Explanations for the Observed Phenotype
As previously mentioned, the A2AR–D2R interaction hypothesis of reciprocal inhibition is not enough to explain the hyperlocomotor and the less anxious-like phenotype of Tg(CaMKII-hA2AR) rats. These findings can be explained by two possibilities: (a) these behaviors are regulated by other neurotransmitters influenced by A2AR, whose actions are pre-dominant over dopamine action; (b) there are alternative interactions between A2AR and dopamine receptors, as some studies about rewarding and habit formation have suggested (46, 61–63). A2AR interacts with several G protein-coupled (besides D2R), ionotropic, and receptors for neurotrophic factors (1). In this group of interactions, there are some, which can explain the hyperlocomotor and/or the decreased anxious-like behaviors: CB1 (56); delta-opioid (56, 64, 65); NMDA (66, 67); nAch (56, 68); GDNF (69), BDNF (56), or GABAA (70, 71) receptors. Additionally, A2AR receptors interact either synergistically or antagonistically with A1R receptors (72–74), which, per se, influence several other receptors, increasing the complexity of the above mentioned neuromodulation. Furthermore, A2AR are also located at glutamatergic synapses (75) and in astrocytes (76), allowing A2AR to directly control synaptic transmission and plasticity (77), which we have shown before to be directly involved in adaptive brain behaviors, namely to early-life stress (32). One hypothesis than cannot be ruled out is the possibility that these animals may have adaptive dopamine receptor alterations, which still needs to be determined.
In what concerns to the second hypothesis, two possibilities can be taken into consideration: (1) a single alternative interaction between A2AR and dopamine receptors is suitable to explain all behavioral results; (2) different interactions occur in distinct neuronal populations, which control different behavioral processes. In what regards the first possibility, just one of alternative interactions is able to explain all behavioral results – antagonistic interaction between A2AR and D1R occurring at a network level, considering their scarce co-expression, (23, 61, 78). D1R antagonists increase locomotion when administered chronically (51), whereas D1R agonists have anxiogenic and antidepressive-like effects (56, 79–81). Therefore, with the A2AR overexpression and the associated decrease in D1R action, there would be a hyperlocomotor, less anxious, and more depressive-like behavior – which perfectly matches the results we obtained.
If we consider that different interactions control different processes, then, three alternative interactions can explain the increased locomotion and the decreased anxious-like behaviors – particular synergism between A2AR and D2R mediated by G protein beta/gamma dimers (61, 82), a supposed functional hyperdopaminergic state in Tg(CaMKII-hA2AR) rats (61, 83); and a presumed increased level of DARPP-32 (a downstream effector molecule of D2-like receptors) in CaMKII-hA2AR rats (62, 84) – whereas one alternative interaction is able to explain the increased depressive-like behavior – synergism between A2AR and mGluR5 (46, 85, 86), which were found to be decreased in the NSE A2A overexpressing rats (53).
Conclusion
We conclude that Tg(CaMKII-hA2AR) rats overexpressing A2AR in hippocampus, cortex, and striatum, have depressive-like behavior and increased locomotor activity. Additionally, we found that the A2AR–D2R interaction hypothesis of reciprocal inhibition is not sufficient to explain all the observed behavioral outcomes. Finally, we conclude that and A2AR overexpression in forebrain is associated with depression, which may explain the depressive signs seen in aging, chronic stress, and Alzheimer’s disease.
Conflict of Interest Statement
The authors declare that the research was conducted in the absence of any commercial or financial relationships that could be construed as a potential conflict of interest.
Acknowledgments
The authors acknowledge J. Baião for technical assistance in the animal house. Joana E. Coelho, Vânia L. Batalha and Diana G. Ferreira were supported by a grant from Fundação para a Ciência e Tecnologia (FCT); Paula M. Canas and Rodrigo A. Cunha were supported by FCT (PTDC/SAU-NSC/122254/2010) and Defense Advanced Research Projects Agency (DARPA, grant 09-68-ESR- FP-010). Luísa V. Lopes is an Investigator FCT, funded by Fundação para a Ciência e Tecnologia (PTDC-099853/2009) and Bial.
References
1. Sebastião AM, Ribeiro JA. Adenosine receptors and the central nervous system. Handb Exp Pharmacol (2009) (193):471–534.
2. Rétey JV, Adam M, Gottselig JM, Khatami R, Dürr R, Achermann P, et al. Adenosinergic mechanisms contribute to individual differences in sleep deprivation-induced changes in neurobehavioral function and brain rhythmic activity. J Neurosci (2006) 26(41):10472–9. doi: 10.1523/JNEUROSCI.1538-06.2006
3. Elmenhorst D, Meyer PT, Winz OH, Matusch A, Ermert J, Coenen HH, et al. Sleep deprivation increases A1 adenosine receptor binding in the human brain: a positron emission tomography study. J Neurosci (2007) 27(9):2410–5. doi:10.1523/JNEUROSCI.5066-06.2007
4. Boison D. Adenosine dysfunction and adenosine kinase in epileptogenesis. Open Neurosci J (2010) 4:93–101. doi:10.2174/1874082001004020093
5. Van Dycke A, Raedt R, Dauwe I, Sante T, Wyckhuys T, Meurs A, et al. Continuous local intrahippocampal delivery of adenosine reduces seizure frequency in rats with spontaneous seizures. Epilepsia (2010) 51(9):1721–8. doi:10.1111/j.1528-1167.2010.02700.x
6. Lam P, Hong CJ, Tsai SJ. Association study of A2a adenosine receptor genetic polymorphism in panic disorder. Neurosci Lett (2005) 378(2):98–101. doi:10.1016/j.neulet.2004.12.012
7. Alsene K, Deckert J, Sand P, de Wit H. Association between A2a receptor gene polymorphisms and caffeine-induced anxiety. Neuropsychopharmacology (2003) 28(9):1694–702. doi:10.1038/sj.npp.1300232
8. Albasanz JL, Perez S, Barrachina M, Ferrer I, Martín M. Up-regulation of adenosine receptors in the frontal cortex in Alzheimer’s disease. Brain Pathol (2008) 18(2):211–9. doi:10.1111/j.1750-3639.2007.00112.x
9. Hurley MJ, Mash DC, Jenner P. Adenosine A(2A) receptor mRNA expression in Parkinson’s disease. Neurosci Lett (2000) 291(1):54–8. doi:10.1016/S0304-3940(00)01371-9
10. Urigüen L, García-Fuster MJ, Callado LF, Morentin B, La Harpe R, Casadó V, et al. Immunodensity and mRNA expression of A2A adenosine, D2 dopamine, and CB1 cannabinoid receptors in postmortem frontal cortex of subjects with schizophrenia: effect of antipsychotic treatment. Psychopharmacology (2009) 206(2):313–24. doi:10.1007/s00213-009-1608-2
11. Fredholm BB, IJzerman AP, Jacobson KA, Klotz KN, Linden J. International union of pharmacology. XXV. Nomenclature and classification of adenosine receptors. Pharmacol Rev (2001) 53(4):527–52.
12. Ribeiro JA, Sebastião AM, de Mendonca A. Adenosine receptors in the nervous system: pathophysiological implications. Prog Neurobiol (2002) 68(6):377–92. doi:10.1016/S0301-0082(02)00155-7
13. Girault JA, Greengard P. The neurobiology of dopamine signaling. Arch Neurol (2004) 61(5):641–4. doi:10.1001/archneur.61.5.641
14. Bjorklund A, Dunnett SB. Dopamine neuron systems in the brain: an update. Trends Neurosci (2007) 30(5):194–202. doi:10.1016/j.tins.2007.03.006
15. Pierce RC, Kumaresan V. The mesolimbic dopamine system: the final common pathway for the reinforcing effect of drugs of abuse? Neurosci Biobehav Rev (2006) 30(2):215–38. doi:10.1016/j.neubiorev.2005.04.016
16. Howes OD, Kapur S. The dopamine hypothesis of schizophrenia: version III – the final common pathway. Schizophr Bull (2009) 35(3):549–62. doi:10.1093/schbul/sbp006
17. Pizzagalli DA, Holmes AJ, Dillon DG, Goetz EL, Birk JL, Bogdan R, et al. Reduced caudate and nucleus accumbens response to rewards in unmedicated individuals with major depressive disorder. Am J Psychiatry (2009) 166(6):702–10. doi:10.1176/appi.ajp.2008.08081201
18. Keedwell PA, Andrew C, Williams SC, Brammer MJ, Phillips ML. The neural correlates of anhedonia in major depressive disorder. Biol Psychiatry (2005) 58(11):843–53. doi:10.1016/j.biopsych.2005.05.019
19. Wacker J, Dillon DG, Pizzagalli DA. The role of the nucleus accumbens and rostral anterior cingulate cortex in anhedonia: integration of resting EEG, fMRI, and volumetric techniques. Neuroimage (2009) 46(1):327–37. doi:10.1016/j.neuroimage.2009.01.058
20. Sadock J, Sadock A, Ruiz P. Kaplan and Sadock’s Comprehensive Textbook of Psychiatry. 9th ed. (Vol. 2). Philadelphia: Lippincott Williams & Wilkins (2009). p. 1875–6.
21. Korchounov A, Meyer MF, Krasnianski M. Postsynaptic nigrostriatal dopamine receptors and their role in movement regulation. J Neural Transm (2010) 117(12):1359–69. doi:10.1007/s00702-010-0454-z
22. Meyer JH, McNeely HE, Sagrati S, Boovariwala A, Martin K, Verhoeff NP, et al. Elevated putamen D(2) receptor binding potential in major depression with motor retardation: an [11C]raclopride positron emission tomography study. Am J Psychiatry (2006) 163(9):1594–602. doi:10.1176/appi.ajp.163.9.1594
23. Ferré S, Fredholm BB, Morelli M, Popoli P, Fuxe K. Adenosine-dopamine receptor-receptor interactions as an integrative mechanism in the basal ganglia. Trends Neurosci (1997) 20(10):482–7. doi:10.1016/S0166-2236(97)01096-5
24. Fuxe K, Ferré S, Genedani S, Franco R, Agnati LF. Adenosine receptor-dopamine receptor interactions in the basal ganglia and their relevance for brain function. Physiol Behav (2007) 92(1–2):210–7. doi:10.1016/j.physbeh.2007.05.034
25. Muscat R, Willner P. Suppression of sucrose drinking by chronic mild unpredictable stress: a methodological analysis. Neurosci Biobehav Rev (1992) 16(4):507–17. doi:10.1016/S0149-7634(05)80192-7
26. Rygula R, Abumaria N, Flügge G, Fuchs E, Rüther E, Havemann-Reinecke U. Anhedonia and motivational deficits in rats: impact of chronic social stress. Behav Brain Res (2005) 162(1):127–34. doi:10.1016/j.bbr.2005.03.009
27. Yan HC, Cao X, Das M, Zhu XH, Gao TM. Behavioral animal models of depression. Neurosci Bull (2010) 26(4):327–37. doi:10.1007/s12264-010-0323-7
28. Brooks SP, Dunnett SB. Tests to assess motor phenotype in mice: a user’s guide. Nat Rev Neurosci (2009) 10(7):519–29. doi:10.1038/nrn2652
29. Choleris E, Thomas AW, Kavaliers M, Prato FS. A detailed ethological analysis of the mouse open field test: effects of diazepam, chlordiazepoxide and an extremely low frequency pulsed magnetic field. Neurosci Biobehav Rev (2001) 25(3):235–60. doi:10.1016/S0149-7634(01)00011-2
30. Popova EA, Krivokharchenko AS, Vil’ianovich LI. In vitro development of murine embryos using different types of microinjections. Ontogenez (2002) 33:107–10. doi:10.1023/A:1014912128311
31. Mayford M, Bach ME, Kandel E. CaMKII function in the nervous system explored from a genetic perspective. Cold Spring Harb Symp Quant Biol (1996) 61:219–24. doi:10.1101/SQB.1996.061.01.024
32. Batalha VL, Pego JM, Fontinha BM, Costenla AR, Valadas JS, Baqi Y, et al. Adenosine A2A receptor blockade reverts hippocampal stress-induced deficits and restores corticosterone circadian oscillation. Mol Psychiatry (2013) 18:320–31. doi:10.1038/mp.2012.8
33. Strekalova T, Spanagel R, Bartsch D, Henn FA, Gass P. Stress-induced anhedonia in mice is associated with deficits in forced swimming and exploration. Neuropsychopharmacology (2004) 29(11):2007–17. doi:10.1038/sj.npp.1300532
34. Bekris S, Antoniou K, Daskas S, Papadopoulou-Daifoti Z. Behavioural and neurochemical effects induced by chronic mild stress applied to two different rat strains. Behav Brain Res (2005) 161(1):45–59. doi:10.1016/j.bbr.2005.01.005
35. Porsolt RD, Le Pichon M, Jalfre M. Depression: a new animal model sensitive to antidepressant treatments. Nature (1977) 266(5604):730–2. doi:10.1038/266730a0
36. Cryan JF, Markou A, Lucki I. Assessing antidepressant activity in rodents: recent developments and future needs. Trends Pharmacol Sci (2002) 23(5):238–45. doi:10.1016/S0165-6147(02)02017-5
37. Detke MJ, Rickels M, Lucki I. Active behaviors in the rat forced swimming test differentially produced by serotonergic and noradrenergic antidepressants. Psychopharmacology (1995) 121(1):66–72. doi:10.1007/BF02245592
38. Castagne V, Porsolt RD, Moser P. Use of latency to immobility improves detection of antidepressant-like activity in the behavioral despair test in the mouse. Eur J Pharmacol (2009) 616(1–3):128–33. doi:10.1016/j.ejphar.2009.06.018
39. Papp M, Willner P, Muscat R. An animal model of anhedonia: attenuation of sucrose consumption and place preference conditioning by chronic unpredictable mild stress. Psychopharmacology (1991) 104(2):255–9. doi:10.1007/BF02244188
40. Bessa JM, Ferreira D, Melo I, Marques F, Cerqueira JJ, Palha JA, et al. The mood-improving actions of antidepressants do not depend on neurogenesis but are associated with neuronal remodeling. Mol Psychiatry (2009) 14(8):764–73, 739. doi:10.1038/mp.2008.119
41. Simões do Couto F, Batalha VL, Valadas JS, Franco J, Ribeiro JA, Lopes LV. Escitalopram improves memory deficits induced by maternal separation in the rat. Eur J Pharmacol (2012) 695(1–3):71. doi:10.1016/j.ejphar.2012.08.020
42. Cryan JF, Valentino RJ, Lucki I. Assessing substrates underlying the behavioral effects of antidepressants using the modified rat forced swimming test. Neurosci Biobehav Rev (2005) 29(4–5):547–69. doi:10.1016/j.neubiorev.2005.03.008
43. El Yacoubi M, Costentin J, Vaugeois JM. Adenosine A2A receptors and depression. Neurology (2003) 61(11 Suppl 6):S82–7. doi:10.1212/01.WNL.0000095220.87550.F6
44. Kaster MP, Rosa AO, Rosso MM, Goulart EC, Santos AR, Rodrigues AL. Adenosine administration produces an antidepressant-like effect in mice: evidence for the involvement of A1 and A2A receptors. Neurosci Lett (2004) 355(1–2):21–4. doi:10.1016/j.neulet.2003.10.040
45. Lobato KR, Binfaré RW, Budni J, Rosa AO, Santos AR, Rodrigues AL. Involvement of the adenosine A1 and A2A receptors in the antidepressant-like effect of zinc in the forced swimming test. Prog Neuropsychopharmacol Biol Psychiatry (2008) 32(4):994–9. doi:10.1016/j.pnpbp.2008.01.012
46. Castañé A, Soria G, Ledent C, Maldonado R, Valverde O. Attenuation of nicotine-induced rewarding effects in A2A knockout mice. Neuropharmacology (2006) 51(3):631–40. doi:10.1016/j.neuropharm.2006.05.005
47. Ledent C, Vaugeois JM, Schiffmann SN, Pedrazzini T, El Yacoubi M, Vanderhaeghen JJ, et al. Aggressiveness, hypoalgesia and high blood pressure in mice lacking the adenosine A2a receptor. Nature (1997) 388(6643):674–8. doi:10.1038/41771
48. Karcz-Kubicha M, Antoniou K, Terasmaa A, Quarta D, Solinas M, Justinova Z, et al. Involvement of adenosine A1 and A2A receptors in the motor effects of caffeine after its acute and chronic administration. Neuropsychopharmacology (2003) 28(7):1281–91. doi:10.1038/sj.npp.1300167
49. Jain N, Kemp N, Adeyemo O, Buchanan P, Stone TW. Anxiolytic activity of adenosine receptor activation in mice. Br J Pharmacol (1995) 116(3):2127–33. doi:10.1111/j.1476-5381.1995.tb16421.x
50. Collins LE, Galtieri DJ, Collins P, Jones SK, Port RG, Paul NE, et al. Interactions between adenosine and dopamine receptor antagonists with different selectivity profiles: effects on locomotor activity. Behav Brain Res (2010) 211(2):148–55. doi:10.1016/j.bbr.2010.03.003
51. Braun AR, Laruelle M, Mouradian MM. Interactions between D1 and D2 dopamine receptor family agonists and antagonists: the effects of chronic exposure on behavior and receptor binding in rats and their clinical implications. J Neural Transm (1997) 104(4–5):341–62. doi:10.1007/BF01277656
52. Prut L, Belzung C. The open field as a paradigm to measure the effects of drugs on anxiety-like behaviors: a review. Eur J Pharmacol (2003) 463(1–3):3–33. doi:10.1016/S0014-2999(03)01272-X
53. Giménez-Llort L, Schiffmann SN, Shmidt T, Canela L, Camón L, Wassholm M, et al. Working memory deficits in transgenic rats overexpressing human adenosine A2A receptors in the brain. Neurobiol Learn Mem (2007) 87(1):42–56. doi:10.1016/j.nlm.2006.05.004
54. Wei CJ, Augusto E, Gomes CA, Singer P, Wang Y, Boison D, et al. Regulation of fear responses by striatal and extrastriatal adenosine A2A receptors in forebrain. Biol Psychiatry (2013) 75(11):855–63. doi:10.1016/j.biopsych.2013.05.003
55. El Yacoubi M, Ledent C, Parmentier M, Costentin J, Vaugeois JM. The anxiogenic-like effect of caffeine in two experimental procedures measuring anxiety in the mouse is not shared by selective A(2A) adenosine receptor antagonists. Psychopharmacology (2000) 148(2):153–63. doi:10.1007/s002130050037
56. Schiffmann SN, Fisone G, Moresco R, Cunha RA, Ferré S. Adenosine A2A receptors and basal ganglia physiology. Prog Neurobiol (2007) 83(5):277–92. doi:10.1016/j.pneurobio.2007.05.001
57. Millan MJ, Brocco M, Papp M, Serres F, La Rochelle CD, Sharp T, et al. S32504, a novel naphtoxazine agonist at dopamine D3/D2 receptors: III. Actions in models of potential antidepressive and anxiolytic activity in comparison with ropinirole. J Pharmacol Exp Ther (2004) 309(3):936–50.
58. McAuley JD, Stewart AL, Webber ES, Cromwell HC, Servatius RJ, Pang KC. Wistar-Kyoto rats as an animal model of anxiety vulnerability: support for a hypervigilance hypothesis. Behav Brain Res (2009) 204(1):162–8. doi:10.1016/j.bbr.2009.05.036
59. Ramos A. Animal models of anxiety: do I need multiple tests? Trends Pharmacol Sci (2008) 29(10):493–8. doi:10.1016/j.tips.2008.07.005
60. Bilbao A, Cippitelli A, Martín AB, Granado N, Ortiz O, Bezard E, et al. Absence of quasi-morphine withdrawal syndrome in adenosine A2A receptor knockout mice. Psychopharmacology (2006) 185(2):160–8. doi:10.1007/s00213-005-0284-0
61. Brown RM, Short JL, Cowen MS, Ledent C, Lawrence AJ. A differential role for the adenosine A2A receptor in opiate reinforcement vs opiate-seeking behavior. Neuropsychopharmacology (2009) 34(4):844–56. doi:10.1038/npp.2008.72
62. Soria G, Castañé A, Ledent C, Parmentier M, Maldonado R, Valverde O. The lack of A2A adenosine receptors diminishes the reinforcing efficacy of cocaine. Neuropsychopharmacology (2006) 31(5):978–87. doi:10.1038/sj.npp.1300876
63. Yu C, Gupta J, Chen JF, Yin HH. Genetic deletion of A2A adenosine receptors in the striatum selectively impairs habit formation. J Neurosci (2009) 29(48):15100–3. doi:10.1523/JNEUROSCI.4215-09.2009
64. Jutkiewicz EM, Baladi MG, Folk JE, Rice KC, Woods JH. The delta-opioid receptor agonist SNC80 [(+)-4-[alpha(R)-alpha-[(2S,5R)-4-allyl-2,5-dimethyl-1-piperazinyl]-(3-met hoxybenzyl)-N,N-diethylbenzamide] synergistically enhances the locomotor-activating effects of some psychomotor stimulants, but not direct dopamine agonists, in rats. J Pharmacol Exp Ther (2008) 324(2):714–24. doi:10.1124/jpet.107.123844
65. Katsuura Y, Taha SA. Modulation of feeding and locomotion through mu and delta opioid receptor signaling in the nucleus accumbens. Neuropeptides (2010) 44(3):225–32. doi:10.1016/j.npep.2009.12.002
66. Kuzmin A, Madjid N, Terenius L, Ogren SO, Bakalkin G. Big dynorphin, a prodynorphin-derived peptide produces NMDA receptor-mediated effects on memory, anxiolytic-like and locomotor behavior in mice. Neuropsychopharmacology (2006) 31(9):1928–37. doi:10.1038/sj.npp.1300959
67. Li FW, Deurveilher S, Semba K. Behavioural and neuronal activation after microinjections of AMPA and NMDA into the perifornical lateral hypothalamus in rats. Behav Brain Res (2011) 224(2):376–86. doi:10.1016/j.bbr.2011.06.021
68. Garção P, Szabó EC, Wopereis S, Castro AA, Tomé ÂR, Prediger RD, et al. Functional interaction between pre-synaptic α6β2-containing nicotinic and adenosine A2A receptors in the control of dopamine release in the rat striatum. Br J Pharmacol (2013) 169(7):1600–11. doi:10.1111/bph.12234
69. Gomes CA, Simões PF, Canas PM, Quiroz C, Sebastião AM, Ferré S, et al. GDNF control of the glutamatergic cortico-striatal pathway requires tonic activation of adenosine A receptors. J Neurochem (2009) 108(5):1208–19. doi:10.1111/j.1471-4159.2009.05876.x
70. Zhao H, Ohinata K, Yoshikawa M. Central prostaglandin D(2) exhibits anxiolytic-like activity via the DP(1) receptor in mice. Prostaglandins Other Lipid Mediat (2009) 88(3–4):68–72. doi:10.1016/j.prostaglandins.2008.10.001
71. Listos J, Malec D, Fidecka S. Influence of adenosine receptor agonists on benzodiazepine withdrawal signs in mice. Eur J Pharmacol (2005) 523(1–3):71–8. doi:10.1016/j.ejphar.2005.07.025
72. Lopes LV, Cunha RA, Ribeiro JA. Cross talk between A(1) and A(2A) adenosine receptors in the hippocampus and cortex of young adult and old rats. J Neurophysiol (1999) 82(3):196–203.
73. Lopes LV, Cunha RA, Kull B, Fredholm BB, Ribeiro JA. Adenosine A(2A) receptor facilitation of hippocampal synaptic transmission is dependent on tonic A(1) receptor inhibition. Neuroscience (2002) 112:319–29. doi:10.1016/S0306-4522(02)00080-5
74. Ciruela F, Casadó V, Rodrigues RJ, Luján R, Burgueño J, Canals M, et al. Presynaptic control of striatal glutamatergic neurotransmission by adenosine A1-A2A receptor heteromers. J Neurosci (2006) 26:2080–7. doi:10.1523/JNEUROSCI.3574-05.2006
75. Rebola N, Rodrigues RJ, Lopes LV, Richardson PJ, Oliveira CR, Cunha RA. Adenosine A1 and A2A receptors are co-expressed in pyramidal neurons and co-localized in glutamatergic nerve terminals of the rat hippocampus. Neuroscience (2005) 133:79–83. doi:10.1016/j.neuroscience.2005.01.054
76. Matos M, Augusto E, Santos-Rodrigues AD, Schwarzschild MA, Chen JF, Cunha RA, et al. Adenosine A2A receptors modulate glutamate uptake in cultured astrocytes and gliosomes. Glia (2012) 60(5):702–16. doi:10.1002/glia.22290
77. Costenla AR, Diógenes MJ, Canas PM, Rodrigues RJ, Nogueira C, Maroco J, et al. Enhanced role of adenosine A(2A) receptors in the modulation of LTP in the rat hippocampus upon ageing. Eur J Neurosci (2011) 34:12–21. doi:10.1111/j.1460-9568.2011.07719.x
78. Short JL, Ledent C, Drago J, Lawrence AJ. Receptor crosstalk: characterization of mice deficient in dopamine D1 and adenosine A2A receptors. Neuropsychopharmacology (2006) 31(3):525–34. doi:10.1038/sj.npp.1300852
79. Simon P, Panissaud C, Costentin J. Anxiogenic-like effects induced by stimulation of dopamine receptors. Pharmacol Biochem Behav (1993) 45(3):685–90. doi:10.1016/0091-3057(93)90525-X
80. D’Aquila PS, Collu M, Pani L, Gessa GL, Serra G. Antidepressant-like effect of selective dopamine D1 receptor agonists in the behavioural despair animal model of depression. Eur J Pharmacol (1994) 262(1–2):107–11.
81. Hirano S, Miyata S, Onodera K, Kamei J. Involvement of dopamine D1 receptors and alpha1-adrenoceptors in the antidepressant-like effect of chlorpheniramine in the mouse tail suspension test. Eur J Pharmacol (2007) 562(1–2):72–6. doi:10.1016/j.ejphar.2007.01.063
82. Yao L, Arolfo MP, Dohrman DP, Jiang Z, Fan P, Fuchs S, et al. beta gamma Dimers mediate synergy of dopamine D2 and adenosine A2 receptor-stimulated PKA signaling and regulate ethanol consumption. Cell (2002) 109(6):733–43. doi:10.1016/S0092-8674(02)00763-8
83. Dassesse D, Massie A, Ferrari R, Ledent C, Parmentier M, Arckens L, et al. Functional striatal hypodopaminergic activity in mice lacking adenosine A(2A) receptors. J Neurochem (2001) 78(1):183–98. doi:10.1046/j.1471-4159.2001.00389.x
84. Svenningsson P, Lindskog M, Ledent C, Parmentier M, Greengard P, Fredholm BB, et al. Regulation of the phosphorylation of the dopamine- and cAMP-regulated phosphoprotein of 32 kDa in vivo by dopamine D1, dopamine D2, and adenosine A2A receptors. Proc Natl Acad Sci U S A (2000) 97(4):1856–60. doi:10.1073/pnas.97.4.1856
85. Rodrigues RJ, Alfaro TM, Rebola N, Oliveira CR, Cunha RA. Co-localization and functional interaction between adenosine A(2A) and metabotropic group 5 receptors in glutamatergic nerve terminals of the rat striatum. J Neurochem (2005) 92(3):433–41. doi:10.1111/j.1471-4159.2004.02887.x
Keywords: adenosine A2A receptors, memory, anxiety, depression, stress, locomotion, dopamine
Citation: Coelho JE, Alves P, Canas PM, Valadas JS, Shmidt T, Batalha VL, Ferreira DG, Ribeiro JA, Bader M, Cunha RA, do Couto FS and Lopes LV (2014) Overexpression of adenosine A2A receptors in rats: effects on depression, locomotion, and anxiety. Front. Psychiatry 5:67. doi: 10.3389/fpsyt.2014.00067
Received: 03 April 2014; Accepted: 24 May 2014;
Published online: 13 June 2014.
Edited by:
Silvia Raquel Soares Ouakinin, University of Lisbon, PortugalCopyright: © 2014 Coelho, Alves, Canas, Valadas, Shmidt, Batalha, Ferreira, Ribeiro, Bader, Cunha, do Couto and Lopes. This is an open-access article distributed under the terms of the Creative Commons Attribution License (CC BY). The use, distribution or reproduction in other forums is permitted, provided the original author(s) or licensor are credited and that the original publication in this journal is cited, in accordance with accepted academic practice. No use, distribution or reproduction is permitted which does not comply with these terms.
*Correspondence: Luísa V. Lopes, Neurosciences Unit, Instituto de Medicina Molecular, Av. Professor Egas Moniz 1, Lisbon 1649-028, Portugal e-mail: lvlopes@medicina.ulisboa.pt
†Joana E. Coelho and Pedro Alves have contributed equally to this work.