- 1Center for Addiction Research, University of Texas Medical Branch, Galveston, TX, USA
- 2Department of Pharmacology and Toxicology, University of Texas Medical Branch, Galveston, TX, USA
- 3Department of Neuroscience and Cell Biology, University of Texas Medical Branch, Galveston, TX, USA
The dopamine mesocorticoaccumbens pathway which originates in the ventral tegmental area (VTA) and projects to the nucleus accumbens and prefrontal cortex is a circuit important in mediating the actions of psychostimulants. The function of this circuit is modulated by the actions of serotonin (5-HT) at 5-HT2A receptors (5-HT2AR) localized to the VTA. In the present study, we tested the hypothesis that virally mediated overexpression of 5-HT2AR in the VTA would increase cocaine-evoked locomotor activity in the absence of alterations in basal locomotor activity. A plasmid containing the gene for the 5-HT2AR linked to a synthetic marker peptide (Flag) was created and the construct was packaged in an adeno-associated virus vector (rAAV-5-HT2AR-Flag). This viral vector (2 μl; 109–10 transducing units/ml) was unilaterally infused into the VTA of male rats, while control animals received an intra-VTA infusion of Ringer’s solution. Virus-pretreated rats exhibited normal spontaneous locomotor activity measured in a modified open-field apparatus at 7, 14, and 21 days following infusion. After an injection of cocaine (15 mg/kg, ip), both horizontal hyperactivity and rearing were significantly enhanced in virus-treated rats (p < 0.05). Immunohistochemical analysis confirmed expression of Flag and overexpression of the 5-HT2AR protein. These data indicate that the vulnerability of adult male rats to hyperactivity induced by cocaine is enhanced following increased levels of expression of the 5-HT2AR in the VTA and suggest that the 5-HT2AR receptor in the VTA plays a role in regulation of responsiveness to cocaine.
Introduction
Cocaine addiction is marked by significant morbidity and loss of human potential, yet consistently effective and accessible recovery options remain limited. This fact underscores the continuing need to uncover the neural factors that drive vulnerability to cocaine addiction and relapse and to establish new pharmacological strategies to halt or reverse the progression of the disorder. Cocaine inhibits reuptake of monoamines, including dopamine (DA) and serotonin (5-hydroxytryptamine; 5-HT; Koe, 1976) and the enhanced efflux of DA within the mesocorticoaccumbens circuit is critical in the generation of cocaine-evoked behaviors (Kelly and Iversen, 1976; Delfs et al., 1990; Callahan et al., 1994). The mesocorticoaccumbens DA neurons, which originate in the ventral tegmental area (VTA) and project prominently to subcortical [e.g., nucleus accumbens (NAc)] and cortical structures [e.g., prefrontal cortex (PFC)], are under the modulatory control of the 5-HT system (Alex and Pehek, 2007), with 5-HT neurons in the dorsal raphe nucleus innervating both cell body and terminal regions of the mesocorticoaccumbens circuit (Halliday and Tork, 1989). As such, the 5-HT system is also an important mediator of cocaine-evoked behaviors (for reviews, see Walsh and Cunningham, 1997; Muller and Huston, 2006; Bubar and Cunningham, 2008; Filip et al., 2010).
The 5-HT2A receptor (5-HT2AR), one of 14 subtypes of 5-HT receptors (Hoyer et al., 2002), plays an integral role in mediating the serotonergic influence upon cocaine-evoked behaviors (Muller and Huston, 2006; Bubar and Cunningham, 2008). Blockade of the 5-HT2AR with a selective antagonist (e.g., M100907; SR46349B) significantly reduces cocaine-evoked hypermotility (O’Neill et al., 1999; McMahon and Cunningham, 2001; Fletcher et al., 2002; Filip et al., 2004; Szucs et al., 2005), behavioral sensitization (Filip et al., 2004; Zayara et al., 2011), and behavioral disinhibition (Anastasio et al., 2011; Fletcher et al., 2011; Cunningham et al., 2013), as well as the discriminative stimulus effects of cocaine (Filip et al., 2006) and cocaine- (Fletcher et al., 2002) and cue-primed (Nic Dhonnchadha et al., 2009; Pockros et al., 2011) reinstatement of cocaine-seeking in rats. Systemic administration of the non-selective 5-HT2AR antagonist ketanserin inhibited cocaine-evoked hypermotility simultaneous with inhibition of cocaine-evoked DA release in the NAc (Broderick et al., 2004), suggesting an important role for 5-HT2AR-modulation of DA mesocorticoaccumbens neurotransmission in cocaine-evoked behaviors mediated by this circuit.
The 5-HT2AR is a G protein-coupled receptor (Berg et al., 1994) expressed throughout the nodes of the mesocorticoaccumbens circuit (Cornea-Hebert et al., 1999; Doherty and Pickel, 2000; Xu and Pandey, 2000; Nocjar et al., 2002; Miner et al., 2003). The 5-HT2AR resident in the VTA is localized to both DA and non-DAergic [presumably γ-aminobutyric (GABA) or glutamate] neurons within the VTA (Doherty and Pickel, 2000; Nocjar et al., 2002), and appear to be integral in modulating psychostimulant-induced behaviors mediated by the mesocorticoaccumbens circuit. Microinfusion of the selective 5-HT2AR antagonist M100907 into the VTA, but not the NAc, attenuated hyperactivity evoked by systemic administration of cocaine at doses that did not alter basal motor activation (McMahon et al., 2001). Likewise, intra-VTA 5-HT2AR antagonist administration significantly blocked amphetamine-evoked hyperactivity and associated DA release in the NAc, with no effect upon basal motor activity or DA efflux in NAc (Auclair et al., 2004). We have observed that microinfusion of the preferential 5-HT2AR agonist 1-(2,5-dimethoxy-4-iodo)-2-aminopropane (DOI) alone into the VTA is sufficient to evoke hyperactivity in rats (Herin et al., unpublished observations). Thus, activation of 5-HT2AR resident in the VTA results in behaviorally significant outcomes, and likewise appears to play a critical role in cocaine-evoked behaviors mediated by the DA mesocorticoaccumbens circuit.
The virally mediated gene transfer technique represents a targeted means to manipulate the expression of important proteins in the brains of adult animals (Carlezon et al., 1997; Bolanos et al., 2003; Edry et al., 2011). A recombinant adeno-associated virus (rAAV) can be used to selectively transduce neurons for a long duration (weeks to months) with a minimum of toxicity and inflammation (McCown et al., 1996; Lo et al., 1999). In the present study, we have exploited rAAV-mediated gene transfer to investigate whether overexpression of the 5-HT2AR in the VTA alters the vulnerability of adult male rats to the hypermotive effects of cocaine. We developed an rAAV containing the coding region for the 5-HT2AR linked to a synthetic marker peptide (Flag; rAAV-5-HT2AR-Flag), and infused vehicle or rAAV-5-HT2AR-Flag unilaterally into the VTA of experimental animals, followed by measurement of basal and cocaine-evoked hyperactivity. Immunohistochemical analyses were used to confirm 5-HT2AR overexpression as well as expression of the Flag peptide.
Materials and Methods
Animals
Male Sprague-Dawley rats (Harlan Sprague-Dawley, Inc., Indianapolis, IN, USA) weighed 250–275 g at the beginning of the study. The rats were housed (initially four/cage) in standard plastic rodent cages in a temperature (21–23°C) and humidity (55–65%) controlled environment under a 12-h light/dark cycle (lights on 07:00 h). Animals were acclimated to the colony for 3–5 days prior to surgery, after which they were single-housed and allowed to recover for at least one week prior to the start of experimental sessions. All animals were provided with food and water ad libitum. Experiments were conducted during the light phase of the light-dark cycle (1200–1800 h) and were in accordance with the National Institutes of Health Guide for the Care and Use of Laboratory Animals and with approval from the UTMB Institutional Animal Care and Use Committee.
Viral Vectors
The cDNA containing the coding region for the rat 5-HT2AR was obtained (Dr. J. Liu, University of Cincinnati). Primers were designed to amplify only the coding region of the 5-HT2AR and to add a BamHI site (to the 5′ end), SpeI site (to the 3′ end), 24 bases coding for a synthetic marker (Flag) protein and a stop codon. PCR amplification was performed using an rTtH-XL polymerase (Perkin Elmer, Boston, MA, USA) and the product (5-HT2AR-Flag) was purified by preparative agarose gel electrophoresis followed by dialysis, phenol/chloroform/isoamyl alcohol (25:24:1) extraction and ethanol precipitation.
The 5-HT2AR-Flag DNA was then ligated into a pCW plasmid, which is appropriate for viral packaging. The pCW plasmid (provided by Dr. D. J. Poulsen; University of Montana) contains the inverted terminal repeats (ITR) of AAV, a chick β-actin (CAG) promoter, multiple cloning sites, and a woodchuck hepatitis virus posttranscriptional regulatory element (WPRE; Stone et al., 2005). 5-HT2AR-Flag (300 fmol) and pCW (30 fmol) were digested with BamHI (2 units) and SpeI (5 units) for 2 h at 37°C. Ligation was performed with a kit (TaKaRa Biochemical, Inc., Berkeley, CA, USA). Ten microliters of the resultant cDNA was used to transform DH5-α ultracompetent E. coli containing 50 μg/ml ampicillin. Plasmid DNA was isolated from 20 colonies and tested for inclusion of the plasmid by digestion with BamHI and SpeI. One positive colony was CsCl-purified and sequenced at the UTMB Molecular Biology Core Facility. Functionality of the transgene was determined by transfection in raphe RN46A cells (provided by Dr. Scott Whittemore, University of Miami; White et al., 1994) followed by immunocytochemical detection with 5-HT2AR and Flag antibodies.
The rAAV-5-HT2AR-Flag was prepared by cotransfecting three plasmids into human embryonic kidney cells (HEK 293 cell line) based on previous protocols (Xiao et al., 1998; Wu et al., 2002) utilizing an AAV helper plasmid (pXX2) and an adenovirus helper plasmid (pXX6). The HEK cells were cultured in 150 mm dishes containing DMEM/10% FBS at 37°C, 5% CO2. When cells reached 80% confluence, calcium phosphate precipitation was used for co-transfection with pCW-5-HT2AR-Flag, pXX2, and pXX6. Following a brief rinse with DMEM, OptiMEM (Life Technologies)/10% FBS/120 μM chloroquine was added to the cells. Then 2.5 ml of DNA–calcium phosphate solution was added per plate. This solution contained three plasmids at the molar ratio of 7:2:4, 125 mM CaCl2 and 1× HBS (2.5 M NaCl, 0.25 M HEPES, 75 mM Na2HPO4, pH 7.1). Cells were cultured with 5% CO2 at 37°C for 18 h, and changed with OptiMEM/10% FBS. Two days after co-transfection, cells and medium were collected, centrifuged at 1140 g for 15 min, and then resuspended in 150 mM NaCl/20 mM Tris pH 8.0 at 5 × 106 cells/ml. The cell suspension was further treated with 0.54% deoxycholate (Sigma, St. Louis, MO, USA) and 50 U/ml Benxonase (Sigma) at 37°C for 1 h. Following centrifugation at 3000 g at room temperature for 20 min, supernatants were subjected to a cycle of freeze − thaw, and then centrifuged again at 10,000 g at 4°C for 30 min. The supernatant was collected, filtered through a 1-μm disk filter (Fisher, Pittsburgh, PA, USA), and then run by gravity through a heparin agarose type I column (Sigma) pre-equilibrated with phosphate buffer saline/1 mM MgCl2/2.5 mM KCl phosphate-buffered saline (PBS-MK). After four washes with 5 ml PBS-MK each, rAAV viruses were eluted by 9 ml of 1 M NaCl/PBS-MK. The first 2 ml was discarded. The next 7 ml was collected, desalted by running through a Centricon Plus-20/Biomax-100 (Fisher) with four changes of lactated Ringer’s solution, then concentrated by centrifugation at 3000 g at room temperature, and the elution was collected. Dot blot indicated that the titer of the packaged virus was in the range of 109–1010 transducing units/ml.
Animal Surgery
Rats (n = 10/group) were anesthetized intramuscularly (IM) with 43 mg/kg of ketamine, 8.6 mg/kg of xylazine, and 1.5 mg/kg of acepromazine in physiological saline (0.9% NaCl) and placed in a Kopf rat stereotaxic apparatus (David Kopf Instruments, Tujunga, CA, USA) with the upper incisor bar at −3.8 mm below the interaural line. A Hamilton microsyringe (Hamilton, Reno, NV, USA) was then lowered unilaterally into the VTA at a 9° from the midsaggital plane in relation to bregma: [anteroposterior (AP) −5.3 mm, mediolateral (ML) + 1.3 mm, and dorsoventral (DV) −8.1 and 8.5 mm from skull (Paxinos and Watson, 1998; McMahon et al., 2001; Shank et al., 2007)]. The rAAV-5-HT2AR-Flag (2 μl, 109–1010 transducing units/ml) or lactated ringer’s solution vehicle control (2 μl) was infused into the VTA (n = 10 per group) using the UMP II infusion pump (WPI, Sarasota, FL, USA) at a rate of 18 nl/min; the infusion lasted 2 h. Following infusion, the needle was left in place for 10 min followed by withdrawal from the brain and wound closure. Rats received a single injection (IM) of 300,000 U of sodium ampicillin after surgery and were allowed 1 week to recover, during which time they were handled and weighed daily.
Behavioral Procedures
Apparatus
Locomotor activity was quantified using a modified open-field activity system under low-light conditions (San Diego Instruments, San Diego, CA, USA). Each enclosure consisted of a clear Plexiglas open-field (40 cm × 40 cm × 40 cm) and a 4 × 4 photobeam matrix located 4 cm above the cage floor for the measurement of horizontal activity; each monitor was housed within sound-attenuating chambers. A second horizontal row of 16 photobeams located 16 cm from the floor allowed the measurement of rearing. Activity counts were made by the control software (Photobeam Activity Software, San Diego Instruments, San Diego, CA, USA) and stored for statistical evaluation. Video cameras located above the enclosures were used to monitor activity continuously without disruption of behavior.
Effects of 5-HT2AR overexpression on basal and cocaine-evoked locomotor activity
On Day 7 following surgery, animals were placed in activity monitors and horizontal activity and rearing were recorded for 60 min, followed by return to the animal colony. Additionally, activity was again measured in these same animals on Days 14 and 21 following surgery for 60 min on each day. On Day 21, following the measurement of basal locomotor activity, all animals were challenged with 15 mg/kg of cocaine [(-)-cocaine HCl salt; National Institute on Drug Abuse, Research Triangle, NC, USA dissolved in 0.9% NaCl], a dose that consistently produces hyperactivity in our laboratory (McCreary and Cunningham, 1999; De La Garza and Cunningham, 2000; Liu and Cunningham, 2006; Cunningham et al., 2013). Immediately following injection, horizontal activity and rearing were measured for 60 min.
Both horizontal activity and rearing counts were totaled for each animal in 10-min time bins and across the 60-min test sessions. All data are presented as mean horizontal activity counts or rearing counts (±SEM). For basal locomotor activity, a two-way ANOVA was used to analyze the effects of intra-VTA pretreatment (control or rAAV-5-HT2AR-Flag; factor 1) and day (Days 7, 14, 21; factor 2) with pretreatment as a between-subjects factor and day as a within-subjects factor. Planned comparisons for each test day were made with a Student’s t-test with a Bonferroni correction. To analyze the time course of cocaine-evoked activity on Day 21, a two-way ANOVA with factors of intra-VTA pretreatment (between-subjects) and time (within-subjects) was utilized followed by planned comparisons at each time point using a Student’s t-test with a Bonferroni correction. Differences in the mean total hyperactivity observed for the 60-min period following cocaine injection on Day 21 were analyzed with a Student’s t-test. All statistical tests were determined using SAS for Windows (Version 8.1) with an experiment wise α = 0.05.
Histology and transgene detection
At the end of behavioral testing on Day 21, animals were deeply anesthetized with an intraperitoneal (IP) injection of pentobarbital (Sigma, 100 mg/kg) and transcardially perfused with PBS followed by 3% buffered paraformaldehyde. Brains were then removed, blocked at the mid-pons, and post-fixed in paraformaldehyde at room temperature for 2 h. Tissue was then cryoprotected in 30% sucrose solution at 4°C for 48 h. Brains were frozen with crushed dry ice and stored at −80°C. Coronal sections (50 μm) were prepared with a Leica cryostat (CM 1850) at −20°C and processed to verify microinjection placement and transgene expression using immunohistochemistry (see below). Data obtained from rats with infusion sites outside of the VTA were excluded from analysis.
To validate the ability of the rAAV construct to establish expression of 5-HT2AR and Flag within the VTA, we employed immunohistochemical techniques using diaminobenzidine detection and light microscopy as described previously (Allen and MacPhail, 1991; Ross et al., 2006; Shank et al., 2007). Briefly, sections were blocked with a solution containing 1.5% normal goat serum (Vector Laboratories, Burlingame, CA, USA) in PBS with 0.4% Triton-X (PBS-T; Sigma), followed by incubation in PBS-T containing a polyclonal antibody for either the 5-HT2AR (1:1000; courtesy of Dr. Bryan Roth, Case Western University Cleveland, OH, USA; Garlow et al., 1993; Roth et al., 1995; Cornea-Hebert et al., 2002; Nocjar et al., 2002; Bubar et al., 2005; Ross et al., 2006) or Flag peptide (1:1000; Sigma). Sections were washed in PBS, incubated in PBS-T containing biotinylated goat-anti-rabbit IGg (1:400; Vector), incubated in an avidin-biotin-horseradish peroxidase complex (Vector), washed in TRIS buffer and developed in 3,3′-diaminobenzidine (0.5 mg/ml; Sigma) with 0.005% H2O2. Sections were mounted onto slides and coverslipped, followed by visualization with an Olympus Vanox-T AH2 microscope and image capture using a Pixera Professional camera (VCS10132; Sherwood Dallas, Co., Dallas, TX, USA) that was connected to a personal computer. Images of the VTA ipsilateral and contralateral to the injection site were captured and each image was subsequently cropped to a fixed-size rectangle of 420 × 1238 pixels located within the parabrachial-paranigral subnuclei of the VTA (Phillipson, 1979; Swanson, 1982) for comparative analyses. In accordance with recent image guidelines (Couzin, 2006), Adobe Photoshop (Adobe Systems, San Jose, CA, USA) was employed to mask dark shadows arising as injection artifacts on several sections.
The fixed-size images of the VTA were analyzed using a program written in Matlab (MathWorks, Inc., Natick, MA, USA) and the red channel of the image data was used for analysis (Hillman, 1984; Pollandt et al., 2005; Liu et al., 2007). The intensity histogram was computed, producing a bell-shaped curve, skewed toward the dense side by the presence of darker (stained) pixels, which occupy a small fraction of the image area. The non-stained tissue density was modeled by fitting a normal curve to the upper portion of the histogram, using the Marquard non-linear least-squares method. The fitted curve was subtracted from the observed histogram on the dense side of the peak, providing an estimate of the intensity distribution of staining. A threshold was selected that was 2.6 standard deviations below the mean of the fitted background curve. Pixels darker than the threshold were considered to be stained and were displayed as a map for visual confirmation. The number of such pixels was counted to quantify immunolabeling (Hillman, 1984; Pollandt et al., 2005; Liu et al., 2007). Total immunolabeling was determined as the sum of stained pixels weighted by their density below the staining threshold and was calculated from VTA images ipsilateral and contralateral to the infusion site. The difference in total immunolabeling from the ipsilateral minus contralateral VTA from each animal was compared between infusion groups with an unpaired Wilcoxon test.
To explore localization of 5-HT2AR within VTA cells and co-localization of 5-HT2AR in DA neurons, confocal microscopy (Bubar and Cunningham, 2007; Bubar et al., 2011; Anastasio et al., 2013) was utilized to study double-label immunofluorescence with previously validated antibodies for the 5-HT2AR (Garlow et al., 1993; Roth et al., 1995; Cornea-Hebert et al., 2002; Nocjar et al., 2002; Bubar et al., 2005) and tyrosine hydroxylase (TH; Browning et al., 2005). Methods for immunofluorescence were similar to those described above with a few minor modifications. A separate cohort of rats (n = 7) was unilaterally infused with lactated ringer’s solution control or rAAV-5-HT2AR-Flag (as described above) and sacrificed 4 weeks following infusion. Sections (25 μm) were prepared using the Leica cryostat, followed by several washes and incubation in blocking serum (PBS-T plus 1.5% goat serum) as described above. Single sections were then incubated in PBS-T containing both the 5-HT2AR antibody (1:1000) and a monoclonal antibody for TH (1:2500; Immunostar). Sections were then washed in PBS and incubated in PBS-T containing secondary fluorescent goat-anti-rabbit (1:2000; Alexa fluor 555; Invitrogen, Carlsbad, CA, USA) and goat-anti-mouse (1:2000; Alexa fluor 488; Invitrogen) antibodies at room temperature. Last, sections were mounted as described above and labeling visualized at the UTMB Infectious Disease Optical Imaging Core using a Zeiss LSM 510 Meta confocal microscope and image capture with LSM 5 imaging software (Carl Zeiss Microimaging, Thornwood, NY, USA) that was connected to a personal computer.
Results
Effects of 5-HT2AR Overexpression on Basal and Cocaine-Evoked Activity
To test the hypothesis that overexpression of the 5-HT2AR in the VTA enhances basal or cocaine-evoked hyperactivity, male rats (n = 10/group) were pretreated with intra-VTA infusion of either lactated Ringer’s solution (control) or rAAV-5-HT2AR-Flag (virus). Of these, nine control rats exhibited needle placements positioned in the VTA (see below); one animal contained a needle placement outside of the VTA, and was thus excluded. Of virus-pretreated animals, five exhibited proper VTA placement as well as virally mediated overexpression of the 5-HT2AR (see below). One virus-pretreated animal exhibited overexpression in the hypothalamus as a result of incorrect placement, and four animals did not exhibit 5-HT2AR overexpression; these animals were excluded from analysis.
Basal locomotor activity measured on Days 7, 14, and 21 was analyzed for control animals with proper VTA placements and virus-pretreated animals with overexpression confined to the VTA (Figure 1). The levels of basal locomotor activity observed, regardless of pretreatment, were similar to levels of locomotor activity evoked upon saline injection in previous studies (McMahon and Cunningham, 2001; McMahon et al., 2001; Filip and Cunningham, 2002; Bubar et al., 2003). There was no main effect of pretreatment (F1, 41 = 0.11, p = 0.747), day (F2, 41 = 3.17, p = 0.06), or a pretreatment × day interaction (F2, 41 = 3.18, p = 0.06) observed for basal horizontal activity on Days 7, 14, and 21 after viral injections. A priori comparisons indicated that the basal horizontal activity did not differ between pretreatment groups on any test day (Figure 1A). For basal rearing activity, a main effect of day (F2, 41 = 10.97, p = 0.0004) in the absence of a main effect of pretreatment (F1, 41 = 0.65, p = 0.435) or a pretreatment × day interaction (F2, 41 = 0.46, p = 0.638) was observed (Figure 1B); a priori comparisons between treatment groups failed to indicate significant differences in basal rearing activity between control and virus treatment groups on any given test day. Levels of basal activity in animals with misplaced rAAV-5-HT2AR-Flag infusions outside of the VTA did not differ from control animals on days 7, 14, or 21 (data not shown; p > 0.05).
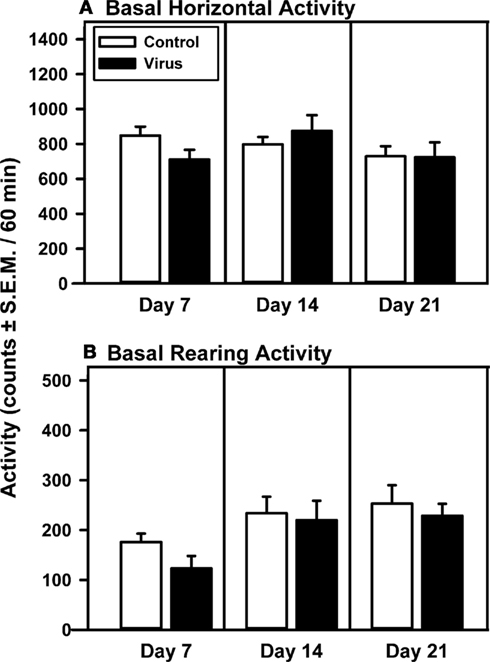
Figure 1. Basal levels of motility are unchanged following intra-VTA infusion of rAAV-5-HT2AR-Flag. Mean total horizontal (A) or rearing activity (B) [counts/60 min (±SEM)] were recorded 7, 14, and 21 days following intracerebral pretreatment with 2 μl of vehicle (Control; open bar; n = 9) or rAAV-5-HT2AR-Flag (Virus, filled bar; n = 5). Both pretreatment groups exhibited similar levels of horizontal and rearing activity on each day analyzed.
On Day 21 following the measurement of basal locomotor activity, all animals were challenged with 15 mg/kg of cocaine and activity was recorded for 60 min (Figure 2). A main effect of pretreatment (F1, 83 = 7.84, p = 0.016), time (F5, 83 = 28.37, p < 0.0001), and a pretreatment × time interaction (F5, 83 = 28.37, p < 0.0001) were observed for cocaine-evoked horizontal activity measured in 10-min time bins during the 60-min test (Figure 2A, left panel). A priori comparisons indicated that viral pretreatment was associated with significantly greater cocaine-evoked horizontal activity during each of the first two time bins (10 and 20 min) of the test period as compared to control animals (p < 0.008/comparison). A trend for increased cocaine-evoked horizontal activity was observed but not statistically significant at the 30 min (p = 0.04) and 40 min time bins (p = 0.04). The a priori analysis indicated that virus pretreatment was associated with significantly greater levels of cocaine-evoked horizontal activity totaled for the entire 60-min test session (Figure 2A, right panel; p < 0.05). Levels of cocaine-evoked horizontal activity in animals with misplaced rAAV-5-HT2AR-Flag infusions outside of the VTA did not differ from control animals (data not shown; p > 0.05).
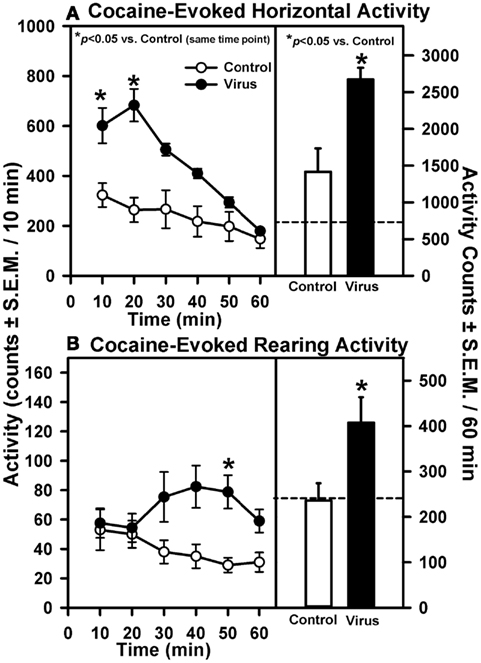
Figure 2. Intra-VTA infusion of rAAV-5-HT2AR-Flag is associated with elevated cocaine-induced motility. Data represent the mean total horizontal (A) or rearing activity (B) in rats induced upon injection with cocaine (15 mg/kg, ip) 21 days after a 2 μl infusion of vehicle (Control; open symbols; n = 9) or rAAV-5-HT2AR-Flag (Virus; filled symbols; n = 5). Left panels represent the timecourse across the 60 min session divided into 10 min bins [mean activity counts/10 min (±SEM)]; *p < 0.008 vs. control at same time point. Right panels illustrate mean total horizontal (A) or rearing activity (B) for the entire 60 min session [counts/60 min (±SEM); *p < 0.05 vs. control. Dashed line represents average level of basal horizontal or rearing activity determined in the 60 min period prior to cocaine injection (see Figure 1).
Rearing activity was also measured following cocaine injection on Day 21. A main effect of pretreatment (F1, 83 = 6.61, p = 0.025) and a pretreatment × time interaction (F5, 83 = 3.13, p = 0.014), but not a main effect of time (F5, 83 = 0.82, p = 0.538), were observed for cocaine-evoked rearing activity measured in 10-min time bins during the 60-min test (Figure 2B). A priori planned comparisons indicated that the viral pretreatment was associated with greater cocaine-evoked rearing activity at the 50 min time bin (p < 0.008/comparison), with the comparisons made at the 30 min (p = 0.047), 40 min (p = 0.01), and 60 min time bins (p = 0.023) of the test period approaching statistical significance (Figure 2B, left panel). The a priori analysis indicated that virus pretreatment was associated with significantly greater levels of cocaine-evoked rearing activity totaled across the entire 60-min test session compared to control animals (Figure 2B, left panel; p < 0.05). Levels of cocaine-evoked rearing activity in animals with misplaced rAAV-5-HT2AR-Flag infusions outside of the VTA did not differ from control animals (data not shown; p > 0.05).
5-HT2AR and Flag Immunohistochemistry
Following the completion of behavioral testing on Day 21, animals were sacrificed and immunohistochemistry performed to confirm overexpression of 5-HT2AR and expression of Flag in the VTA (Figure 3). A representative photomicrograph depicting 5-HT2AR immunolabeling in the VTA ipsilateral to the infusion site from a control animal illustrates that the majority of the 5-HT2AR immunoreactivity seems to be confined to cell bodies, with little fiber labeling (Figure 3A). Control animals exhibited little Flag background labeling (Figure 3B). In contrast, 5-HT2AR immunolabeling in the ipsilateral VTA from a virus animal infused with rAAV-5-HT2AR-Flag (Figure 3C) illustrates a distinct pattern of 5-HT2AR immunolabeling characterized by robust 5-HT2AR immunoreactivity in both cell bodies and fibers. A brain section labeled with the anti-Flag antibody and adjacent to that shown in Figure 3C shows a similar expression of immunoreactivity, with labeled cell bodies as well as fibers (Figure 3D). Additionally, the arrows indicate labeled cells in Figures 3C,D seem to be identical, providing further evidence of successful overexpression.
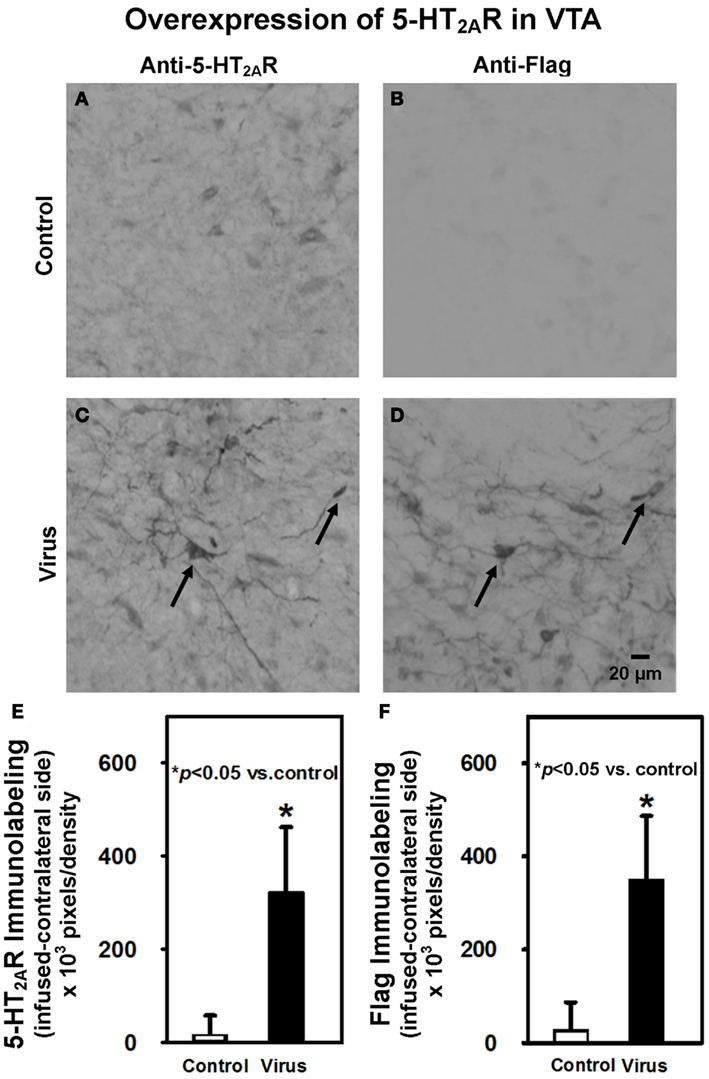
Figure 3. Intra-VTA infusion of rAAV-5-HT2AR-Flag is associated with overexpression of 5-HT2AR and Flag peptide immunolabeling. Shown are representative photomicrographs of (A) anti-5-HT2AR antibody labeling of VTA neurons in control animals; (B) anti-Flag antibody labeling of VTA neurons in control animals; (C) anti-5-HT2AR antibody labeling of VTA neurons in virus animals infused with rAAV-5-HT2AR-Flag; (D) labeling with anti-Flag antibody in an immediately adjacent tissue section of virus-pretreated animal indicates co-localization of 5-HT2AR and Flag. (E) Quantification of net 5-HT2AR immunoreactivity (±SEM) in VTA of control animals (open bar; n = 9) vs. virus animals infused with rAAV-5-HT2AR-Flag-(filled bar; n = 5). Animals that previously received intra-VTA viral infusion exhibited greater 5-HT2AR immunoreactivity than control animals, confirming viral overexpression of 5-HT2AR. (F) Quantification of net Flag immunoreactivity (±SEM) in VTA of control animals (open bar; n = 9) vs. virus animals infused with rAAV-5-HT2AR-Flag (filled bar; n = 5). Animals that previously received intra-VTA viral infusion exhibited greater Flag immunoreactivity than those control animals, further confirming viral overexpression of 5-HT2AR. Scale bar = 20 μm. *p < 0.05 vs. control.
A comparison of the 5-HT2AR immunoreactivity quantified in the VTA (Figure 3E) was made using an unpaired Wilcoxon test. Control animals exhibited similar, moderate levels of 5-HT2AR immunoreactivity in the VTA ipsilateral (Figure 3A) and contralateral (data not shown) to the infusion site. Infusion of rAAV-5-HT2AR-Flag resulted in overexpression of 5-HT2AR in the ipsilateral (Figure 3C), but not contralateral, VTA (data not shown). The total net immunolabeling (ipsilateral immunolabeling – contralateral immunolabeling) was then calculated in individual animals in order to normalize 5-HT2AR overexpression to basal 5-HT2AR levels in the brain hemisphere contralateral to viral infusion. This total net immunolabeling was then compared between pretreatment groups and the results indicate that greater levels of 5-HT2AR expression were exhibited in virus-pretreated animals (p < 0.05; Figure 3E). Quantification of Flag immunoreactivity with this same procedure also revealed robust levels of Flag labeling in virus-pretreated animals, as compared to control animals (p < 0.05; Figure 3F), further confirming successful expression of the transgene. Levels of 5-HT2AR and Flag immunoreactivity in animals with misplaced rAAV-5-HT2AR-Flag infusions outside of the VTA did not differ from control animals (data not shown; p > 0.05).
Confocal microscopy was utilized to analyze tissue sections processed for double-labeled 5-HT2AR and TH immunofluorescence in the VTA in order to assess localization of 5-HT2AR to DA neurons (Figure 4) from animals pretreated with vehicle (Figure 4A) or AAV-5-HT2AR-Flag (Figures 4B–F). Figure 4A demonstrates a composite confocal image (24 sections, 0.68 μm/slice) of 5-HT2AR immunoreactivity from the VTA of a control animal. Immunolabeling is predominantly confined to cell body regions (Figure 4A) in keeping with our observation of 5-HT2AR staining using DAB (above; Figure 3) and previous studies using the same anti-5-HT2AR antibody (Nocjar et al., 2002; Bubar et al., 2005). Figure 4B demonstrates a composite confocal image (24 sections, 0.70 μm/slice) of 5-HT2AR immunoreactivity in the VTA of an animal infused with rAAV-5-HT2AR-Flag. Overexpression is indicated by the robust immunoreactivity in both cell bodies and fibers (Figure 4B). Figures 4C–F represents composite confocal images of double-label immunofluorescence from an animal infused with rAAV-5-HT2AR-Flag. Figure 4C demonstrates a composite confocal image (24 sections, 0.71 μm/slice) of 5-HT2AR immunoreactivity in the VTA of an animal infused with AAV-5-HT2AR-Flag, and Figure 4D demonstrates immunoreactivity for TH in the same tissue section as that shown in Figure 4C. The composite image represents localization of 5-HT2AR immunoreactivity in a TH-positive cell (Figure 4E). Additionally, 20 of the serial Z-sections that comprise the composite image in Figure 4E are shown in Figure 4F (Bubar and Cunningham, 2007; Bubar et al., 2011; Anastasio et al., 2013).
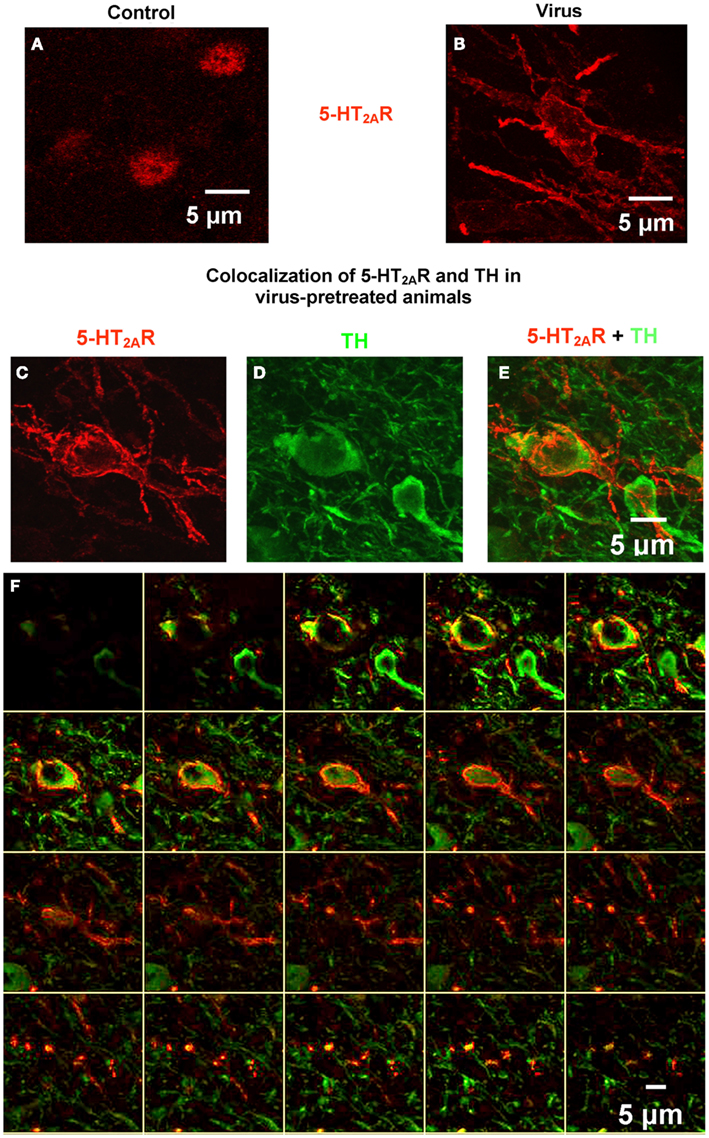
Figure 4. Confocal microscopy demonstrates robust 5-HT2AR labeling in the VTA and co-localization in cells immunopositive for tyrosine hydroxylase (TH). (A) Representative composite confocal image (24 slices, 0.68 μm/slice) demonstrates 5-HT2AR immunoreactivity (red) in VTA neurons from control animals. (B) Representative composite confocal image (24 slices, 0.7 μm/slice) demonstrates 5-HT2AR immunoreactivity (red) in VTA neurons from virus animals pretreated with rAAV-5-HT2AR-Flag. (C–F) Representative composite confocal image (24 slices, 0.71 μm/slice) demonstrating co-localization of 5-HT2AR and TH in VTA neurons from virus animals pretreated with rAAV-5-HT2AR-Flag. In the same tissue section, there is robust 5-HT2AR immunoreactivity (C) and TH immunoreactivity (D) that is colocalized to the same cells (E). (F) Contains 20 of the Z-sections that make up the composite image. Scale bar = 5 μm.
Discussion
The present study is the first to demonstrate that overexpression of 5-HT2AR protein in the VTA enhances the behavioral effects of cocaine following successful virally mediated overexpression of the 5-HT2AR in the adult rat. Intra-VTA transduction with the rAAV-5-HT2AR-Flag vector, which produced quantifiable overexpression of 5-HT2AR and appearance of the Flag protein in VTA neurons, had little effect on basal levels of motor activity, but significantly enhanced cocaine-evoked motility relative to controls. These results are in line with an overall facilitatory role for the 5-HT2AR in mediating cocaine-evoked behaviors (see, Bubar and Cunningham, 2008; Nic Dhonnchadha and Cunningham, 2008) and support the hypothesis that the VTA is a key site of action for the 5-HT2AR to control the behavioral effects of cocaine.
The current results revealing that overexpression of 5-HT2AR in the VTA enhances cocaine-evoked hyperactivity are in accordance with a previous study from our laboratory demonstrating that intra-VTA microinjection of the selective 5-HT2AR antagonist M100907 blocked cocaine-evoked hyperactivity (McMahon et al., 2001). These effects parallel those of systemic injection of 5-HT2AR ligands, as 5-HT2AR antagonists block and 5-HT2AR agonists enhance the hypermotive effects of cocaine (McMahon and Cunningham, 2001; Fletcher et al., 2002; Filip et al., 2004) and other stimulants (Auclair et al., 2004; Herin et al., 2005). These data implicate the VTA as a critical site of action for the positive modulatory control of 5-HT2AR over psychostimulant-evoked motor activity. This stimulatory role for VTA 5-HT2AR upon cocaine-evoked hypermotility appears to occur in the absence of an apparent tonic regulatory influence of this receptor on motor activity. Overexpression of the 5-HT2AR in the VTA has no effect upon basal levels of motility evoked upon exposure to the activity monitors. These data are consistent with studies in the literature demonstrating that selective blockade of 5-HT2AR in the VTA does not alter spontaneous locomotor behavior (McMahon et al., 2001; Auclair et al., 2004). However, we have observed the enhancement of motor activity upon intra-VTA infusion of the non-selective 5-HT2AR agonist DOI (Herin et al., unpublished observations). Together, these data indicate that, despite robust enhancement of 5-HT2AR immunoreactivity in both cell bodies and fibers relative to controls, elevated expression of the 5-HT2AR in the VTA alone is not sufficient to induce overt alterations in basal motor activation. However, the elevated VTA 5-HT2AR expression generates an augmented and positive modulatory effect over cocaine-evoked hyperactivity.
Several characteristics of the 5-HT2AR may account for the low levels of basal 5-HT2AR function. For example, the 5-HT2AR exhibits moderate affinity for 5-HT (Peroutka, 1986; Rothman et al., 2000; Leysen, 2004) and possesses modest constitutive activity in the absence of ligand binding (Berg et al., 2005). In addition, although the 5-HT2AR is thought to primarily localize to somata or dendrites postsynaptic to 5-HT terminals, ultrastructural localization studies indicate that the receptor prominently localizes to the cytoplasm, rather than the plasma membrane, and is primarily found in extrasynaptic regions (Cornea-Hebert et al., 1999; Doherty and Pickel, 2000). Such localization patterns suggest that a component of 5-HT actions at the 5-HT2AR may occur via paracrine or volume transmission which may be minimal at baseline (Miner et al., 2000; Jansson et al., 2001). Thus, activation of the 5-HT2AR receptors in VTA may only occur during periods of stimulated 5-HT release like that evoked following systemic cocaine administration (Chen and Reith, 1994).
The differential effects of 5-HT2AR antagonists delivered into the VTA upon basal vs. cocaine-stimulated motor activity have been attributed to their efficacy to alter the activation status of the DA mesoaccumbens pathway to control locomotor activity (Kelly and Iversen, 1976; Broderick et al., 2004), and are in accordance with a prevailing hypothesis that the 5-HT2AR modulates DA mesocorticoaccumbens neurotransmission only under “stimulated” conditions (Schmidt et al., 1992; De Deurwaerdere and Spampinato, 1999; Di Giovanni et al., 1999; Bonaccorso et al., 2002; Kuroki et al., 2003; Auclair et al., 2004). In accordance with the lack of effects of intra-VTA administration of 5-HT2AR antagonists on basal levels of motor activation (McMahon et al., 2001; Auclair et al., 2004), local infusion of 5-HT2AR antagonists into the VTA failed to alter basal DA release in the NAc (Auclair et al., 2004), nor did perfusion of 5-HT2AR antagonists alter firing rates of VTA DA neurons in a midbrain slice preparation (Olijslagers et al., 2004). Conversely, intra-VTA 5-HT2AR antagonist administration significantly blocked systemic cocaine- (McMahon et al., 2001) and amphetamine-evoked hypermotility (Auclair et al., 2004) and associated amphetamine-evoked NAc DA release (Auclair et al., 2004). Thus the selective effects of VTA 5-HT2AR overexpression upon cocaine-evoked as opposed to basal motor activity are likely due to 5-HT2AR-mediated facilitation of DA mesoaccumbens neurotransmission under stimulated vs. tonic conditions, respectively.
The 5-HT2AR is natively resident within DA and non-DAergic (GABA- or possibly glutamate-containing) neurons in the VTA (Doherty and Pickel, 2000; Ikemoto et al., 2000; Nocjar et al., 2002; Yamaguchi et al., 2007) although DA neurons comprise the majority of VTA neuronal cells (Swanson, 1982; Johnson and North, 1992; Ikemoto, 2007). Indeed, our confocal immunofluorescence studies (see Figure 4) provide evidence of 5-HT2AR overexpression in VTA DA neurons. Activation of elevated levels of 5-HT2AR resident in DA neurons consequent to cocaine-evoked elevations in 5-HT efflux (Chen and Reith, 1994) would be expected to increase activity of DA neurons (Pessia et al., 1994) and release of DA in terminal regions (De Deurwaerdere and Spampinato, 1999). As noted above, enhanced DA release in the NAc correlates positively with generation of hypermotility (Kelly and Iversen, 1976). Thus, overexpression of 5-HT2AR within DA neurons that project to the NAc would serve to enhance cocaine-evoked hyperactivity, as was observed in the present study. Although the overexpression of 5-HT2AR in the DA neurons aligns with the observed behavioral profile, 5-HT2AR overexpression also likely occurred in non-DAergic VTA neurons, presumably GABA interneurons and/or projection neurons, or possibly glutamate neurons (Yamaguchi et al., 2007; see Figure 4), since the constitutively active promoter utilized evokes gene expression in all neuronal cell types (Kaplitt et al., 2007; St Martin et al., 2007). Future studies employing a promotor that would direct viral expression to either DA, GABA, or glutamate neurons would help to discern the contribution of 5-HT2AR overexpression within the particular neuronal cell type to basal vs. cocaine-evoked locomotor activity.
The behavioral phenotype observed following rAAV-5-HT2AR-Flag infusion is most likely mediated by neurons intrinsic to the VTA. Measurable 5-HT2AR overexpression was confined to the site of infusion, and adjacent brain regions (especially, substantia nigra; data not shown) did not demonstrate patterns of 5-HT2AR overexpression. Second, in keeping with this observation, cells projecting to the VTA were not likely to be transduced, as the virus utilized in these studies (AAV-2) is not readily transported retrogradely following infusion into the brain (Chamberlin et al., 1998). Third, the cells transduced in the VTA fit the morphological profile suggestive of neurons, consistent with previous observations that AAV-2 does not readily transduce glial cells (Chamberlin et al., 1998). Fourth, while theoretically possible that viral vector transduction per se could evoke behaviorally relevant cellular changes, this possibility is highly unlikely. Previous studies have shown that transduction of neural tissue with AAV vectors does not alter the electrophysiological properties of neurons (Ehrengruber et al., 2001) or result in neurotoxicity (Lo et al., 1999), while animals infused intracranially with either vehicle or control viral vectors (Carlezon Jr. et al., 1997; Pliakas et al., 2001), including AAV (Landgraf et al., 2003), exhibit equally normal patterns of behavior. Basal locomotor activity did not differ between the animals infused with control vs. AAV in the present study, and the levels of activity observed were similar to that reported in previous studies following saline injection studies (McMahon and Cunningham, 2001; McMahon et al., 2001; Filip and Cunningham, 2002; Bubar et al., 2003). Finally, animals with rAAV-5-HT2AR-Flag infusions located outside the VTA exhibited levels of cocaine-evoked hyperactivity similar to that of control animals. Altogether, these data point to the overexpression of 5-HT2AR in the VTA as the most likely contributor to the observed enhancement of cocaine-evoked hyperactivity following rAAV-5-HT2AR-Flag infusion.
The results of present study suggest that expression levels of the 5-HT2AR in the VTA regulate vulnerability to the hypermotive effects of cocaine and support a possible role for VTA 5-HT2AR in modulating other behavioral effects of cocaine mediated by DA mesocorticoaccumbens circuitry. In addition to altering cocaine-evoked hyperactivity, systemic administration of 5-HT2AR antagonists has been shown to reduce the discriminative stimulus effects of cocaine (McMahon and Cunningham, 2001; Filip et al., 2006) and cocaine-evoked behavioral disinhibition (i.e., impulsivity; Anastasio et al., 2011; Fletcher et al., 2011), as well as to block expression of cocaine sensitization (Filip et al., 2004; Zayara et al., 2011). Furthermore, although the 5-HT2AR does not appear to modulate cocaine intake in the self-administration assay (Fletcher et al., 2002; Nic Dhonnchadha et al., 2009), 5-HT2AR antagonists have been shown to attenuate both cocaine- and cue-evoked reinstatement of cocaine-seeking (Fletcher et al., 2002; Nic Dhonnchadha et al., 2009). However, no studies have evaluated specifically the role of VTA 5-HT2AR receptors in cocaine-evoked behaviors other than locomotor hyperactivity (McMahon et al., 2001), though 5-HT2AR in the NAc (Zayara et al., 2011) and PFC (Pockros et al., 2011) have been implicated in sensitization and cue-evoked reinstatement of cocaine-seeking, respectively. Here we employed a single, low dose of cocaine (15 mg/kg) that consistently induces hypermotility in the absence of overt stereotypic behaviors (Herges and Taylor, 1998) to further establish a critical role for VTA 5-HT2AR in the hypermotive effects of cocaine. Even with the small sample size employed in the current study, the behavioral response to the single dose of cocaine produced a robust behavioral response with little variability. Our results combined with the knowledge regarding 5-HT2AR regulation of DA mesocorticoaccumbens activation provide the impetus to conduct more thorough investigations into the role of 5-HT2AR regulation in the VTA not only in the hypermotive effects of cocaine, but also more complex cocaine-associated behaviors. Furthermore, the methods established here utilizing rAAV-5-HT2AR-Flag to overexpress the 5-HT2AR can be employed to evaluate the role of elevated 5-HT2AR expression throughout the brain.
Conflict of Interest Statement
The authors declare that the research was conducted in the absence of any commercial or financial relationships that could be construed as a potential conflict of interest.
Acknowledgments
This research was supported by National Institute on Drug Abuse grants DA 000260, DA 006511, DA 015272, DA 020087, DA 024157. The 5-HT2AR cDNA and RN46A cells were kindly provided by Dr. J. Liu (University of Cincinnati) and Dr. Scott Whittemore (University of Miami), respectively. The authors would also like to thank Dr. D. J. Poulsen (University of Montana) for a gift of the pCW plasmid, Dr. Bryan Roth (University of North Carolina) for the polyclonal 5-HT2AR antibody as well as the National Institute on Drug Abuse for providing (-)-cocaine. Assistance with confocal microscopy was kindly provided by the Dr. Thomas Albrecht and Eugene Knutson at the UTMB Infectious Disease Optical Imaging Core Facility. We also thank Dr. William Carlezon (Harvard University) for his assistance with the establishment of these techniques. This manuscript was presented by David V. Herin in partial fulfillment of the requirements for the Ph.D. degree for the Graduate School of Biomedical Sciences at the University of Texas Medical Branch.
References
Alex, K. D., and Pehek, E. A. (2007). Pharmacologic mechanisms of serotonergic regulation of dopamine neurotransmission. Pharmacol. Ther. 113, 296–320.
Allen, A. R., and MacPhail, R. C. (1991). Effects of triadimefon on a multiple schedule of fixed-interval performance: comparison with methylphenidate, d-amphetamine and chlorpromazine. Pharmacol. Biochem. Behav. 40, 775–780.
Anastasio, N. C., Gilbertson, S. R., Bubar, M. J., Agarkov, A., Stutz, S. J., Jeng, Y. J., et al. (2013). Peptide inhibitors disrupt the serotonin 5-HT2C receptor interaction with phosphatase and tensin homologue (PTEN) to allosterically modulate cellular signaling and behavior. J. Neurosci. 33, 1615–1630.
Anastasio, N. C., Stoffel, E. C., Fox, R. G., Bubar, M. J., Rice, K. C., Moeller, F. G., et al. (2011). Serotonin (5-hydroxytryptamine) 5-HT2A receptor: Association with inherent and cocaine-evoked behavioral disinhibition in rats. Behav. Pharmacol. 22, 248–261.
Auclair, A., Blanc, G., Glowinski, J., and Tassin, J. P. (2004). Role of serotonin 2A receptors in the D-amphetamine-induced release of dopamine: comparison with previous data on alpha1b-adrenergic receptors. J. Neurochem. 91, 318–326.
Berg, K. A., Clarke, W. P., Chen, Y., Ebersole, B. J., McKay, R. D. G., and Maayani, S. (1994). 5-hydroxytryptamine type 2A receptors regulate cyclic AMP accumulation in a neuronal cell line by protein kinase C-dependent and calcium/calmodulin-dependent mechanisms. Mol. Pharmacol. 45, 826–836.
Berg, K. A., Harvey, J. A., Spampinato, U., and Clarke, W. P. (2005). Physiological relevance of constitutive activity of 5-HT2A and 5-HT2C receptors. Trends Pharmacol. Sci. 26, 625–630.
Bolanos, C. A., Perrotti, L. I., Edwards, S., Eisch, A. J., Barrot, M., Olson, V. G., et al. (2003). Phospholipase Cgamma in distinct regions of the ventral tegmental area differentially modulates mood-related behaviors. J. Neurosci. 23, 7569–7576.
Bonaccorso, S., Meltzer, H. Y., Li, Z., Dai, J., Alboszta, A. R., and Ichikawa, J. (2002). SR46349-B, a 5-HT(2A/2C) receptor antagonist, potentiates haloperidol-induced dopamine release in rat medial prefrontal cortex and nucleus accumbens. Neuropsychopharmacology 27, 430–441.
Broderick, P. A., Olabisi, O. A., Rahni, D. N., and Zhou, Y. (2004). Cocaine acts on accumbens monoamines and locomotor behavior via a 5-HT2A/2C receptor mechanism as shown by ketanserin: 24-h follow-up studies. Prog. Neuropsychopharmacol. Biol. Psychiatry 28, 547–557.
Browning, J. L., Patel, T., Brandt, P. C., Young, K. A., Holcomb, L. A., and Hicks, P. B. (2005). Clozapine and the mitogen-activated protein kinase signal transduction pathway: implications for antipsychotic actions. Biol. Psychiatry 57, 617–623.
Bubar, M. J., and Cunningham, K. A. (2007). Distribution of serotonin 5-HT2C receptors in the ventral tegmental area. Neuroscience 146, 286–277.
Bubar, M. J., and Cunningham, K. A. (2008). Prospects for serotonin 5-HT2R pharmacotherapy in psychostimulant abuse. Prog. Brain Res. 172, 319–346.
Bubar, M. J., McMahon, L. R., De Deurwaerdere, P., Spampinato, U., and Cunningham, K. A. (2003). Selective serotonin reuptake inhibitors enhance cocaine-induced locomotor activity and dopamine release in the nucleus accumbens. Neuropharmacology 44, 342–353.
Bubar, M. J., Seitz, P. K., Thomas, M. L., and Cunningham, K. A. (2005). Validation of a selective serotonin 5-HT(2C) receptor antibody for utilization in fluorescence immunohistochemistry studies. Brain Res. 1063, 105–113.
Bubar, M. J., Stutz, S. J., and Cunningham, K. A. (2011). 5-HT(2C) receptors localize to dopamine and GABA neurons in the rat mesoaccumbens pathway. PLoS ONE 6:e20508. doi:10.1371/journal.pone.0020508
Callahan, P. M., De La Garza, R., and Cunningham, K. A. (1994). Discriminative stimulus properties of cocaine: Modification by dopamine D1 receptors in nucleus accumbens. Psychopharmacology (Berl.) 115, 110–114.
Carlezon, W. A. Jr., Boundy, V. A., Haile, C. N., Lane, S. B., Kalb, R. G., Neve, R. L., et al. (1997). Sensitization to morphine induced by viral-mediated gene transfer. Science 277, 812–814.
Chamberlin, N. L., Du, B., de, L. S., and Saper, C. B. (1998). Recombinant adeno-associated virus vector: use for transgene expression and anterograde tract tracing in the CNS. Brain Res. 793, 169–175.
Chen, N.-H., and Reith, M. E. A. (1994). Effects of locally applied cocaine, lidocaine, and various uptake blockers on monoamine transmission in the ventral tegmental area of freely moving rats: a microdialysis study on monoamine interrelationships. J. Neurochem. 63, 1701–1713.
Cornea-Hebert, V., Riad, M., Wu, C., Singh, S. K., and Descarries, L. (1999). Cellular and subcellular distribution of the serotonin 5-HT2A receptor in the central nervous system of adult rat. J. Comp. Neurol. 409, 187–209.
Cornea-Hebert, V., Watkins, K. C., Roth, B. L., Kroeze, W. K., Gaudreau, P., Leclerc, N., et al. (2002). Similar ultrastructural distribution of the 5-HT(2A) serotonin receptor and microtubule-associated protein MAP1A in cortical dendrites of adult rat. Neuroscience 113, 23–35.
Couzin, J. (2006). Scientific publishing. Don’t pretty up that picture just yet. Science 314, 1866–1868.
Cunningham, K. A., Anastasio, N. C., Fox, R. G., Stutz, S. J., Bubar, M. J., Swinford, S. E., et al. (2013). Synergism between a serotonin 5-HT2A receptor (5-HT2AR) antagonist and 5-HT2CR agonist suggests new pharmacotherapeutics for cocaine addiction. ACS Chem. Neurosci. 4, 110–121.
De Deurwaerdere, P., and Spampinato, U. (1999). Role of serotonin(2A) and serotonin(2B/2C) receptor subtypes in the control of accumbal and striatal dopamine release elicited in vivo by dorsal raphe nucleus electrical stimulation. J. Neurochem. 73, 1033–1042.
De La Garza, R., and Cunningham, K. A. (2000). The effects of the 5-HT1A agonist 8-hydroxy-2(di-n-propylamino)tetralin on spontaneous activity and cocaine-induced hyperactivity and behavioral sensitization. J. Pharmacol. Exp. Ther. 292, 610–617.
Delfs, J. M., Schreiber, L., and Kelley, A. E. (1990). Microinjection of cocaine into the nucleus accumbens elicits locomotor activation in the rat. J. Neurosci. 10, 303–310.
Di Giovanni, G., De Deurwaerdere, P., Di Mascio, M., Di Matteo, V., Esposito, E., and Spampinato, U. (1999). Selective blockade of serotonin-2C/2B receptors enhances mesolimbic and mesostriatal dopaminergic function: a combined in vivo electrophysiological and microdialysis study. Neuroscience 91, 587–597.
Doherty, M. D., and Pickel, V. M. (2000). Ultrastructural localization of the 5-HT2A receptor in dopaminergic neurons in the ventral tegmental area. Brain Res. 864, 176–185.
Edry, E., Lamprecht, R., Wagner, S., and Rosenblum, K. (2011). Virally mediated gene manipulation in the adult CNS. Front. Mol. Neurosci. 4:57. doi:10.3389/fnmol.2011.00057
Ehrengruber, M. U., Hennou, S., Bueler, H., Naim, H. Y., Deglon, N., and Lundstrom, K. (2001). Gene transfer into neurons from hippocampal slices: comparison of recombinant Semliki forest virus, adenovirus, adeno-associated virus, lentivirus, and measles virus. Mol. Cell Neurosci. 17, 855–871.
Filip, M., Alenina, N., Bader, M., and Przegalinski, E. (2010). Behavioral evidence for the significance of serotoninergic (5-HT) receptors in cocaine addiction. Addict. Biol. 15, 227–249.
Filip, M., Bubar, M. J., and Cunningham, K. A. (2004). Contribution of serotonin (5-hydroxytryptamine; 5-HT) 5-HT2 receptor subtypes to the hyperlocomotor effects of cocaine: acute and chronic pharmacological analyses. J. Pharmacol. Exp. Ther. 310, 1246–1254.
Filip, M., Bubar, M. J., and Cunningham, K. A. (2006). Contribution of serotonin (5-HT) 5-HT2 receptor subtypes to the discriminative stimulus effects of cocaine in rats. Psychopharmacology (Berl.) 183, 482–489.
Filip, M., and Cunningham, K. A. (2002). Serotonin 5-HT(2C) receptors in nucleus accumbens regulate expression of the hyperlocomotive and discriminative stimulus effects of cocaine. Pharmacol. Biochem. Behav. 71, 745–756.
Fletcher, P. J., Grottick, A. J., and Higgins, G. A. (2002). Differential effects of the 5-HT2A receptor antagonist M100,907 and the 5-HT2C receptor antagonist SB242,084 on cocaine-induced locomotor activity, cocaine self-administration and cocaine-induced reinstatement of responding. Neuropsychopharmacology 27, 576–586.
Fletcher, P. J., Rizos, Z., Noble, K., and Higgins, G. A. (2011). Impulsive action induced by amphetamine, cocaine and MK801 is reduced by 5-HT(2C) receptor stimulation and 5-HT(2A) receptor blockade. Neuropharmacology 61, 468–477.
Garlow, S. J., Morilak, D. A., Dean, R. R., Roth, B. L., and Ciaranello, R. D. (1993). Production and characterization of a specific 5-HT2 receptor antibody. Brain Res. 615, 113–120.
Halliday, G. M., and Tork, I. (1989). Serotonin-Like immunoreactive cells and fibres in the rat ventromedial mesencephalic tegmentum. Brain Res. Bull. 22, 725–735.
Herges, S., and Taylor, D. A. (1998). Involvement of serotonin in the modulation of cocaine-induced locomotor activity in the rat. Pharmacol. Biochem. Behav. 59, 595–611.
Herin, D. V., Liu, S., Ullrich, T., Rice, K. C., and Cunningham, K. A. (2005). Role of the serotonin 5-HT2A receptor in the hyperlocomotive and hyperthermic effects of (+)-3,4-methylenedioxymethamphetamine. Psychopharmacology (Berl.) 178, 505–513.
Hillman, G. (1984). Quantitation of acetylcholinesterase in frozen tissue sections by computer image analysis. Pharmacologist 26, 225.
Hoyer, D., Hannon, J. P., and Martin, G. R. (2002). Molecular, pharmacological and functional diversity of 5-HT receptors. Pharmacol. Biochem. Behav. 71, 533–554.
Ikemoto, K., Nishimura, A., Okado, N., Mikuni, M., Nishi, K., and Nagatsu, I. (2000). Human midbrain dopamine neurons express serotonin 2A receptor: an immunohistochemical demonstration. Brain Res. 853, 377–380.
Ikemoto, S. (2007). Dopamine reward circuitry: two projection systems from the ventral midbrain to the nucleus accumbens-olfactory tubercle complex. Brain Res. Rev. 56, 27–78.
Jansson, A., Tinner, B., Bancila, M., Verge, D., Steinbusch, H. W., Agnati, L. F., et al. (2001). Relationships of 5-hydroxytryptamine immunoreactive terminal-like varicosities to 5-hydroxytryptamine-2A receptor-immunoreactive neuronal processes in the rat forebrain. J. Chem. Neuroanat. 22, 185–203.
Johnson, S. W., and North, R. A. (1992). Two types of neurone in the rat ventral tegmental area and their synaptic inputs. J. Physiol. (Lond.) 450, 455–468.
Kaplitt, M. G., Feigin, A., Tang, C., Fitzsimons, H. L., Mattis, P., Lawlor, P. A., et al. (2007). Safety and tolerability of gene therapy with an adeno-associated virus (AAV) borne GAD gene for Parkinson’s disease: an open label, phase I trial. Lancet 369, 2097–2105.
Kelly, P. H., and Iversen, S. D. (1976). Selective 6-OHDA-induced destruction of mesolimbic dopamine neurons: abolition of psychostimulant-induced locomotor activity in rats. Eur. J. Pharmacol. 40, 45–56.
Koe, B. K. (1976). Molecular geometry of inhibitors of the uptake of catecholamines and serotonin in synaptosomal preparations of rat brain. J. Pharmacol. Exp. Ther. 199, 649–661.
Kuroki, T., Meltzer, H. Y., and Ichikawa, J. (2003). 5-HT 2A receptor stimulation by DOI, a 5-HT 2A/2C receptor agonist, potentiates amphetamine-induced dopamine release in rat medial prefrontal cortex and nucleus accumbens. Brain Res. 972, 216–221.
Landgraf, R., Frank, E., Aldag, J. M., Neumann, I. D., Sharer, C. A., Ren, X., et al. (2003). Viral vector-mediated gene transfer of the vole V1a vasopressin receptor in the rat septum: improved social discrimination and active social behaviour. Eur. J. Neurosci. 18, 403–411.
Liu, S., Bubar, M. J., Lanfranco, M. F., Hillman, G. R., and Cunningham, K. A. (2007). Serotonin(2C) receptor localization in GABA neurons of the rat medial prefrontal cortex: implications for understanding the neurobiology of addiction. Neuroscience 146, 1667–1688.
Liu, S., and Cunningham, K. A. (2006). Serotonin(2C) receptors (5-HT(2C)R) control expression of cocaine-induced conditioned hyperactivity. Drug Alcohol Depend. 81, 275–282.
Lo, W. D., Qu, G., Sferra, T. J., Clark, R., Chen, R., and Johnson, P. R. (1999). Adeno-associated virus-mediated gene transfer to the brain: duration and modulation of expression. Hum. Gene Ther. 10, 201–213.
McCown, T. J., Xiao, X., Li, J., Breese, G. R., and Samulski, R. J. (1996). Differential and persistent expression patterns of CNS gene transfer by an adeno-associated virus (AAV) vector. Brain Res. 713, 99–107.
McCreary, A. C., and Cunningham, K. A. (1999). The effects of the 5-HT2C/2B antagonist SB 206553 on cocaine-induced locomotor activity. Neuropsychopharmacology 20, 556–564.
McMahon, L. R., and Cunningham, K. A. (2001). Antagonism of 5-hydroxytryptamine(2a) receptors attenuates the behavioral effects of cocaine in rats. J. Pharmacol. Exp. Ther. 297, 357–363.
McMahon, L. R., Filip, M., and Cunningham, K. A. (2001). Differential regulation of the mesoaccumbens circuit by serotonin 5-hydroxytryptamine (5-HT)2A and 5-HT2C receptors. J. Neurosci. 21, 7781–7787.
Miner, L. A., Backstrom, J. R., Sanders-Bush, E., and Sesack, S. R. (2003). Ultrastructural localization of serotonin2A receptors in the middle layers of the rat prelimbic prefrontal cortex. Neuroscience 116, 107–117.
Miner, L. H., Schroeter, S., Blakely, R. D., and Sesack, S. R. (2000). Ultrastructural localization of the serotonin transporter in superficial and deep layers of the rat prelimbic prefrontal cortex and its spatial relationship to dopamine terminals. J. Comp. Neurol. 427, 220–234.
Muller, C. P., and Huston, J. P. (2006). Determining the region-specific contributions of 5-HT receptors to the psychostimulant effects of cocaine. Trends Pharmacol. Sci. 27, 105–112.
Nic Dhonnchadha, B. A., and Cunningham, K. A. (2008). Serotonergic mechanisms in addiction-related memories. Behav. Brain Res. 195, 39–53.
Nic Dhonnchadha, B. A., Fox, R. G., Stutz, S. J., Rice, K. C., and Cunningham, K. A. (2009). Blockade of the serotonin 5-HT2A receptor suppresses cue-evoked reinstatement of cocaine-seeking behavior in a rat self-administration model. Behav. Neurosci. 123, 382–396.
Nocjar, C., Roth, B. L., and Pehek, E. A. (2002). Localization of 5-HT(2A) receptors on dopamine cells in subnuclei of the midbrain A10 cell group. Neuroscience 111, 163–176.
Olijslagers, J. E., Werkman, T. R., McCreary, A. C., Siarey, R., Kruse, C. G., and Wadman, W. J. (2004). 5-HT2 receptors differentially modulate dopamine-mediated auto-inhibition in A9 and A10 midbrain areas of the rat. Neuropharmacology 46, 504–510.
O’Neill, M. F., Heron-Maxwell, C. L., and Shaw, G. (1999). 5-HT2 receptor antagonism reduces hyperactivity induced by amphetamine, cocaine, and MK-801 but not D1 agonist C-APB. Pharmacol. Biochem. Behav. 63, 237–243.
Paxinos, W., and Watson, C. (1998). The Rat Brain in Stereotaxic Coordinates, 4th Edn. San Diego, CA: Academic Press.
Peroutka, S. J. (1986). Pharmacological differentiation and characterization of 5-HT1A, 5-HT1B, and 5-HT1C binding sites in rat frontal cortex. J. Neurochem. 47, 529–540.
Pessia, M., Jiang, Z.-G., North, R. A., and Johnson, S. W. (1994). Actions of 5-hydroxytryptamine on ventral tegmental area neurons of the rat in vitro. Brain Res. 654, 324–330.
Phillipson, O. T. (1979). The cytoarchitecture of the interfascicular nucleus and ventral tegmental area of Tsai in the rat. J. Comp. Neurol. 187, 85–98.
Pliakas, A. M., Carlson, R. R., Neve, R. L., Konradi, C., Nestler, E. J., and Carlezon, W. A. Jr. (2001). Altered responsiveness to cocaine and increased immobility in the forced swim test associated with elevated cAMP response element-binding protein expression in nucleus accumbens. J. Neurosci. 21, 7397–7403.
Pockros, L. A., Pentkowski, N. S., Swinford, S. E., and Neisewander, J. L. (2011). Blockade of 5-HT2A receptors in the medial prefrontal cortex attenuates reinstatement of cue-elicited cocaine-seeking behavior in rats. Psychopharmacology (Berl.) 213, 307–320.
Pollandt, S.W., Orozco-Cabal, L., Scott, M. T., Liu, J., Krishnan, B., Hillman, G. R., et al. (2005). CRF1/2-Immunoreactivity in the Frontolimbic Circuitry is Increased after Acute and Decreased after Protracted Withdrawal from Repeated Cocaine Administration in Rats. Program No. 682.2, Neuroscience 2005 Abstracts, Washington: Society for Neuroscience, Online.
Ross, J. D., Herin, D. V., Frankel, P. S., Thomas, M. L., and Cunningham, K. A. (2006). Chronic treatment with a serotonin(2) receptor (5-HT(2)R) agonist modulates the behavioral and cellular response to (+)-3,4-methylenedioxymethamphetamine [(+)-MDMA]. Drug Alcohol Depend. 81, 117–127.
Roth, B. L., Palvimaki, E.-P., Berry, S., Khan, N., Sachs, N., Uluer, A., et al. (1995). 5-Hydroxytryptamine2A (5-HT2A) receptor desensitization can occur without down-regulation. J. Pharmacol. Exp. Ther. 275, 1638–1646.
Rothman, R. B., Baumann, M. H., Savage, J. E., Rauser, L., McBride, A., Hufeisen, S. J., et al. (2000). Evidence for possible involvement of 5-HT2B receptors in the cardiac valvulopathy associated with fenfluramine and other serotonergic medications. Circulation 102, 2836–2841.
Schmidt, C. J., Fadayel, G. M., Sullivan, C. K., and Taylor, V. L. (1992). 5-HT2 Receptors exert a state-dependent regulation of dopaminergic function: Studies with MDL 100,907 and the amphetamine analogue, 3,4-methylenedioxymethamphetamine. Eur. J. Pharmacol. 223, 65–74.
Shank, E. J., Seitz, P. K., Bubar, M. J., Stutz, S. J., and Cunningham, K. A. (2007). Selective ablation of GABA neurons in the ventral tegmental area increases spontaneous locomotor activity. Behav. Neurosci. 121, 1224–1233.
St Martin, J. L., Klucken, J., Outeiro, T. F., Nguyen, P., Keller-McGandy, C., Cantuti-Castelvetri, I., et al. (2007). Dopaminergic neuron loss and up-regulation of chaperone protein mRNA induced by targeted over-expression of alpha-synuclein in mouse substantia nigra. J. Neurochem. 100, 1449–1457.
Stone, I. M., Lurie, D. I., Kelley, M. W., and Poulsen, D. J. (2005). Adeno-associated virus-mediated gene transfer to hair cells and support cells of the murine cochlea. Mol. Ther. 11, 843–848.
Swanson, L. W. (1982). The projections of the ventral tegmental area and adjacent regions: a combined fluorescent retrograde tracer and immunofluorescence study in the rat. Brain Res. Bull. 9, 321–353.
Szucs, R. P., Frankel, P. S., McMahon, L. R., and Cunningham, K. A. (2005). Relationship of cocaine-induced c-Fos expression to behaviors and the role of serotonin 5-HT2A receptors in cocaine-induced c-Fos expression. Behav. Neurosci. 119, 1173–1183.
Walsh, S. L., and Cunningham, K. A. (1997). Serotonergic mechanisms involved in the discriminative stimulus, reinforcing and subjective effects of cocaine. Psychopharmacology (Berl.) 130, 41–58.
White, L. A., Eaton, M. J., Castro, M. C., Klose, K. J., Globus, M. Y. T., Shaw, G., et al. (1994). Distinct regulatory pathways control neurofilament expression and neurotransmitter synthesis in immortalized serotonergic neurons. J. Neurosci. 14, 6744–6753.
Wu, P., Ye, Y., and Svendsen, C. N. (2002). Transduction of human neural progenitor cells using recombinant adeno-associated viral vectors. Gene Ther. 9, 245–255.
Xiao, X., Li, J., and Samulski, R. J. (1998). Production of high-titer recombinant adeno-associated virus vectors in the absence of helper adenovirus. J. Virol. 72, 2224–2232.
Xu, T., and Pandey, S. C. (2000). Cellular localization of serotonin(2A) (5HT(2A)) receptors in the rat brain. Brain Res. Bull. 51, 499–505.
Yamaguchi, T., Sheen, W., and Morales, M. (2007). Glutamatergic neurons are present in the rat ventral tegmental area. Eur. J. Neurosci. 25, 106–118.
Zayara, A. E., McIver, G., Valdivia, P. N., Lominac, K. D., McCreary, A. C., and Szumlinski, K. K. (2011). Blockade of nucleus accumbens 5-HT2A and 5-HT2C receptors prevents the expression of cocaine-induced behavioral and neurochemical sensitization in rats. Psychopharmacology (Berl.) 213, 321–335.
Keywords: 5-HT2A receptor, cocaine, serotonin, virally mediated gene transfer, AAV, ventral tegmental area, locomotor activity, psychostimulants
Citation: Herin DV, Bubar MJ, Seitz PK, Thomas ML, Hillman GR, Tarasenko YI, Wu P and Cunningham KA (2013) Elevated expression of serotonin 5-HT2A receptors in the rat ventral tegmental area enhances vulnerability to the behavioral effects of cocaine. Front. Psychiatry 4:2. doi: 10.3389/fpsyt.2013.00002
Received: 10 November 2012; Paper pending published: 30 November 2012;
Accepted: 07 January 2013; Published online: 06 February 2013.
Edited by:
F. G. Moeller, University of Texas Health Science Center at Houston, USAReviewed by:
Therese A. Kosten, Baylor College of Medicine, USAMehmet Sofuoglu, VA Connecticut Healthcare System, USA
Copyright: © 2013 Herin, Bubar, Seitz, Thomas, Hillman, Tarasenko, Wu and Cunningham. This is an open-access article distributed under the terms of the Creative Commons Attribution License, which permits use, distribution and reproduction in other forums, provided the original authors and source are credited and subject to any copyright notices concerning any third-party graphics etc.
*Correspondence: Kathryn A. Cunningham, Department of Pharmacology and Toxicology, Center for Addiction Research, University of Texas Medical Branch, 301 University Boulevard, Galveston, TX 77555-0615, USA. e-mail: kcunning@utmb.edu
†Current address: Mary L. Thomas, Department of Pharmacology, Ross University, North Brunswick, NJ, USA.
‡David V. Herin author deceased