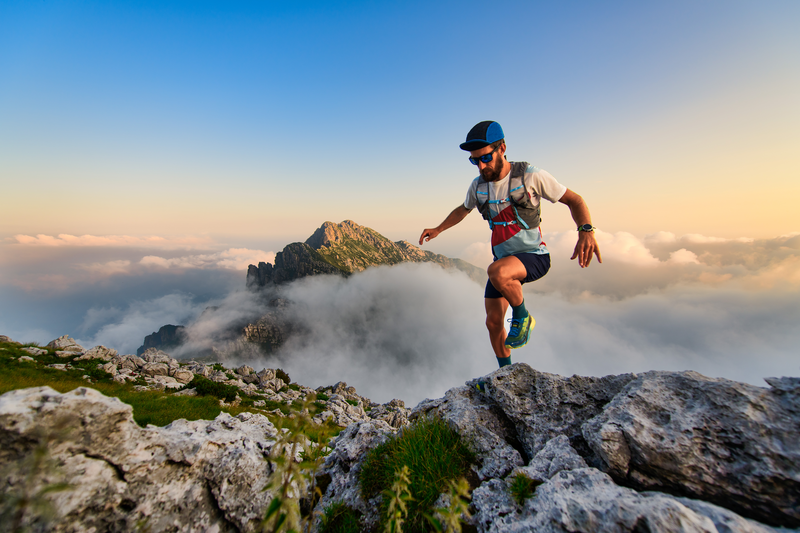
94% of researchers rate our articles as excellent or good
Learn more about the work of our research integrity team to safeguard the quality of each article we publish.
Find out more
ORIGINAL RESEARCH article
Front. Plant Sci. , 19 February 2025
Sec. Functional Plant Ecology
Volume 16 - 2025 | https://doi.org/10.3389/fpls.2025.1550692
This article is part of the Research Topic Fire Effects on Native Plant Species Diversity: Patterns and Mechanisms View all articles
Plant fire ephemerals are well-adapted to fire-prone environments, with germination strategies linked to fire-related cues like smoke. Germination requirements linked to fire cues in Patagonian fire ephemerals are poorly studied, with no research on the effects of smoke water (SW) and smoke isolated karrikinolide (KAR) on their germination. We assessed the germination responses of two native ephemeral herbs, Boopis gracilis, and Nicotiana linearis, to SW and KAR1 at three concentrations (1/100, 1/1000, and 1/10000) and a Control (no SW or KAR1). Seeds collected on different harvest dates were incubated in a germination chamber, and germination responses were analyzed using Generalized Linear Models and Kaplan-Meier survival curves. KAR1 significantly enhanced and accelerated germination in both species, with the strongest effect at the highest concentration (1/100). In contrast, SW did not stimulate germination and inhibited germination in N. linearis at the highest concentration. This inhibitory effect decreased with storage time, suggesting that toxic compounds in SW degrade over time. Additionally, N. linearis seeds exhibited variable germination across harvest years, likely due to after-ripening requirements. Our findings highlight the contrasting effects of SW and KAR1 on seed germination; KAR1 being a reliable germination stimulant. The chemical complexity of smoke water (heterogeneous composition and uncertain concentration) and the time elapsed since its production likely limit its efficacy in promoting germination. These results provide valuable insights into post-fire seedling dynamics in Patagonian grasslands for the conservation in fire-affected ecosystems.
Non-sprouting plant species mainly persist in post-fire communities through seedling recruitment from soil seed banks (Keeley and Fotheringham, 2000; Keeley et al., 2011a). Fire provides a greater opportunity for seedling establishment by releasing resources (e.g. space, light, and nutrients), eliminating pathogens and allelopathic compounds, and reducing competition (Van Staden et al., 2000; Dixon et al., 2009). Additionally, fire can break seed dormancy through fire-related cues, primarily heat shock and smoke, thereby stimulating germination (Keeley and Fotheringham, 2000). The positive response of species to fire cues may confer a significant advantage to seedlings, allowing them to benefit from the post-fire flush of resources, thereby ensuring their establishment (Crosti et al., 2006; Rice and Ross, 2013).
Plant-derived smoke plays an important role in enhancing seed germination of species in fire-prone communities in Mediterranean-type climates (Keeley et al., 2011b). For example, smoke-stimulated seed germination has been observed in five Asteraceae species in Australia (Merritt et al., 2006) and 25 Californian chaparral species (Keeley and Fotheringham, 1998). In South Africa, a significantly higher number of seedlings of Audounia capitata (Bruniaceae) were found in smoke-treated areas (de Lange and Boucher, 1990). Smoke water also played a clear role in seedling recruitment of annual species, increasing seedling emergence and establishment in treated soils within the Mediterranean Basin (Tormo et al., 2014).
Smoke can be applied to seeds as an aerosol or as a liquid generated by bubbling smoke through water (Flematti et al., 2004). Germination response to smoke varies greatly among species (Light et al., 2002; Baker et al., 2005a; Buhk and Hensen, 2006), with some species experiencing inhibited germination (Pennacchio et al., 2007; Gómez-González, 2008). Germination inhibition generally occurs with high concentrations or prolonged exposure to smoke (Pennacchio et al., 2007). Because of this species-specific response, it is necessary to conduct experiments over a wide concentration gradient of smoke (Dixon et al., 1995; Lloyd et al., 2000).
Smoke contains thousands of different compounds, and many attempts have been made to identify the active compound responsible for stimulating germination (Baldwin et al., 1994; Van Staden et al., 1995; Dixon et al., 1995). In 2004, two independent studies isolated an organic molecule from the smoke of burning plant material, known as 3-methyl-2H-furo[2,3-c] pyran-2-one (1) (karrikinolide or KAR1) (Flematti et al., 2004; van Staden et al., 2004). KAR1 is highly active, heat-stable, long-lasting, water-soluble, and very effective in triggering seed germination and seedling growth at extremely low concentrations (< 1 nM) (Flematti et al., 2004; van Staden et al., 2004; Stevens et al., 2007; Light et al., 2009). Unlike smoke, KAR1 solutions are not inhibitory at high concentrations (Flematti et al., 2004). KAR1 functions similarly to gibberellic acid by altering the sensitivity of seeds to light, thereby enhancing seed germination (eg. Angianthus tomentosus, Myriocephalus guerinae, and Podolepis canescens) (Merritt et al., 2006). Several subsequent studies demonstrated the presence of other compounds in smoke that promote germination, including five related karrikins (KAR2-KAR6) (Flematti et al., 2009) as well as cyanohydrins (Flematti et al., 2011). Consequently, karrikins are considered an important family of plant growth regulators (Chiwocha et al., 2009; Nelson et al., 2012).
In the semi-arid steppe of Northwestern Patagonia, dominated by grass-shrub vegetation, fire plays an important role in the structure and function of the ecosystem (Ghermandi et al., 2004). Paleo-climatic studies indicate that fire has been present in Patagonia for at least 10,000 years (Bianchi, 2000). These grasslands exhibit resilience due to the rapid regrowth of grasses and shrubs, as well as the early post-fire mass seedling recruitment of annual native ephemeral herbs from the soil seed bank (Gonzalez and Ghermandi, 2008; Ghermandi et al., 2013). Fire ephemerals are a functional group of plants found in many fire-prone regions of the world such as California (Keeley et al., 2005), South Africa (Keeley and Bond, 1997) and Australia (Baker et al., 2005a, 2005b). Referred to as “the phantom community” by Ghermandi et al. (2004), these species can recruit in great abundance after fires, grow rapidly, produce seeds, and disappear from above-ground vegetation, with their seeds remaining in the soil bank until another fire occurs. They are often confined to heavily disturbed areas such as roadsides, where the dark soil, with its low albedo, absorbs ultraviolet rays, heating the soil and raising its temperature above that of the air at ground level (Matthias et al., 2000). Moreover, in these habitats, summer surface temperatures are like those found at 1 cm in depth during fires (Franzese and Ghermandi, 2012).
Unlike other fire-prone communities worldwide, the germination requirements associated with fire-cues for Patagonian fire ephemeral species have been scarcely studied (Gonzalez and Ghermandi, 2012). These authors reported that the germination of the herb Boopis gracilis (Calyceraceae) was stimulated by aerosol smoke combined with heat-shock, while seeds of Nicotiana linearis (Solanaceae) were not stimulated by either smoke or heat-shock. These species contribute to the post-fire increase in native species richness and diversity in grasslands (Ghermandi et al., 2004; Gonzalez et al., 2010). Understanding the germination behavior of fire ephemerals could be helpful in developing conservation and management strategies in post-fire grasslands and may provide insights into how these species can be used for restoration purposes. Our objective was to assess the effects of different smoke water and karrikinolide (KAR1) concentrations on the seed germination of two fire ephemeral species in NW Patagonian grasslands. We hypothesized that smoke water and karrikinolide solutions enhance the germination, but that the response is species-specific and dependent on the concentration of the solutions. Our study will contribute with valuable knowledge to understanding post-fire dynamics of Mediterranean environments, where fire ephemerals contribute.
We collected seeds of the studied species in sectors of northwestern Patagonia semi-arid grasslands, Argentina (San Ramón Ranch; 41°03´19´´S and 18°01´50´´W). These grasslands were affected by a large-scale fire originated by lightning, caused by a severe drought in 1999 (Ghermandi et al., 2004). Immediately after the fire, we observed a great abundance of seven native fire ephemerals: Boopis gracilis Phil, Nicotiana linearis Phil, Camissonia dentata (Cav.) Reiche, Montiopsis polycarpioides (Phil.) Peralta, Heliotropium paronychioides A.DC., Chenopodium scabricaule Speg., and Descurrainia pimpinellifolia (Barnéoud) O.E. Schulz (Ghermandi et al., 2004; Gonzalez et al., 2010) (Figure 1), which demonstrated their importance in the postfire succession and motivated the study of the effect of fire cues on their germination.
Figure 1. Massive post-fire establishment of the native fire ephemeral Boopis gracilis in northwestern Patagonian grasslands.
The climate is temperate with a Mediterranean precipitation regime (60% of the precipitation falls in autumn and winter). Mean annual precipitation is 569 mm, and mean annual temperature is 8.6°C (Meteorological station of San Ramón ranch, 1928-2023, unpublished data).
The vegetation is dominated by the perennial tussock grasses Pappostipa speciosa Trin. et Rupr var. major (Speg) and Festuca pallescens (St. Yves) Parodi, and the shrubs Mulinum spinosum (Cav.) Pers. and Senecio bracteolatus Hook et Arnott (Ghermandi et al., 2004). The vegetation cover is approximately 60%, and the gaps (inter-tussock areas) are colonized by native and exotic herbaceous species (Ghermandi and Gonzalez, 2009).
Fires occur during the austral spring-summer period (October-March), when low precipitation and high temperatures promote highly flammable fuel (de Torres Curth et al., 2008). In this region, the relationship between the ENSO phenomenon and fires, is demonstrated by the coupling between El Niño (rainy, biomass accumulation), La Niña (dry, biomass drying). This coupling resulted in the occurrence of major wildfires at the San Ramón ranch in 1964, 1976, 1989, and 1999 (Ghermandi et al., 2019). Additionally, the trend of longer droughts in northwestern Patagonia has been present since 1991 and climate models for the Andean-Patagonian region predict not only an increase in temperature but also a decrease in precipitation (Ghermandi et al., 2019; Pessacg et al., 2020), which translates to extensive and more severe fires.
We tested the smoke effect on the germination of two fire ephemeral species; Boopis gracilis (Calyceraceae) and Nicotiana linearis (Solanaceae). Both species are native annual herbs that recruit massively in the post-fire grasslands and disappear two to three years after the fire but persist in the soil seed bank as they have very small seeds (0.10-0.20 mg) (Ghermandi et al., 2004; Gonzalez and Ghermandi, 2012; Ghermandi et al., 2013).
We collected seeds from 10 randomly selected plants of each species in January 2016, 2018, and 2023 for N. linearis and in February 2017 and 2018 for B. gracilis. The seeds were dry-stored in paper bags, in darkness, at room temperature (20-25°C), and relativity humidity of 30-40% until the assays. To discard empty seeds, we applied the pressure test using tweezers under a stereoscopic microscope (Borza et al., 2007).
We used smoke water and karrikinolide solutions to evaluate the effect of different concentrations on the seed germination of ephemeral species.
We followed the protocol of Flematti et al. (2004) to produce smoke water (SW) from the combustion of green (1 kg) and dry plant biomass (2 kg) of the dominant vegetation of the grassland, inside a 200 L metal drum. The smoke was drawn by suction through 5 L of deionized water for 40 minutes. The resultant dark brown solution (plant-derived smoke water) was stored at 5°C. We produced the smoke water in November 2016 and April 2022, after which we prepared three smoke water concentrations (v/v): 1:100, 1:1000, and 1:10000.
To prepare the karrikinolide solutions, we dissolved 1.5 mg of KAR1 (3 methyl-2H-furo[2,3-cpyran-2-one) in 100 mL of deionized water (15 mg/L) (Dr. Gavin Flematti personal communication). We handled KAR1 (hereafter KAR) with gloves, safety glasses, and a lab coat. We heated the solution for a better dissolution. The final solution was protected from light with aluminum foil, and we prepared the solution concentrations (v/v): 1:100, 1:1000, and 1:10000.
We applied a strict sterilization protocol for the seeds and the materials used in the germination trials to prevent mold contamination and avoid the use of fungicides. Tap water and material (forceps, Petri dishes, pipettes, Whatman No.1 filter paper) were sterilized in an autoclave. The assay was set up under a laminar flow hood to maintain sterility. To ensure surface sterility, seeds were immersed in 0.5% v/v sodium hypochlorite solution for 5 minutes prior to treatments and rinsed three times with the sterilized water through a strainer (Gonzalez and Ghermandi, 2012).
Twenty seeds of each species were placed in 45-mm Petri dishes on two layers of filter paper moistened with 1.5 ml of distiller water (control), smoke water (SW), or KAR solution in different concentrations. The treatments were SW1/100, SW1/1000, SW1/10000, and its Control (no SW), and KAR 1/100, KAR 1/1000, KAR 1/10000, and its Control (no KAR) (10 replicates per treatment for each species). We performed four germination trials. In January 2017, we applied SW produced in November 2016 to seeds of N. linearis harvested in January 2016. In February 2018, we applied SW produced in November 2106 to seeds of B. gracilis harvested in February 2017. In April 2018, we applied KAR to seeds of B. gracilis and N. linearis harvested in February and January 2018, respectively. Finally, in April 2023, we applied SW produced in April 2022 to seeds of N. linearis harvested in January 2022 (Table 1). Petri dishes were sealed with a layer of plastic parafilm to reduce desiccation. Finally, seeds were incubated in a germination chamber under controlled temperature and photoperiod 12 h light (30°C)/12 h dark (5°C). Monitoring lasted one month, and the germinated seeds were counted under a stereoscopic microscope without opening the Petri dish every two or three days. The protrusion of the radicle was the criterion for germination. The filter paper was moistened with sterile tap water when necessary.
Table 1. Seed harvesting dates, experiment dates, the type of solution applied (SW, smoke water; KAR, karrikinolide) to Nicotiana linearis and Boopis gracilis, and the production date of smoke water were included.
We analyzed the effects of the main factors (smoke water and KAR) and levels (1/100, 1/1000, 1/10000, and Control) on total seed germination by using a two-way ANOVA for Boopis gracilis, and by fitting generalized linear models with a Poisson distribution and log link function for Nicotiana linearis.
We used Kaplan–Meier curves to describe the probability of seed germination as a function of time (days) of B. gracilis and N. linearis under smoke water and KAR treatments. These curves also serve as indicators of seed germination speed and rate. The log rank test was used to compare the probability of germination between concentrations for both species. All analyses were performed using R software (R Core Team, 2019). The “glm” function from the stats package was used to fit the GLM for total seed germination of N. linearis. Pairwise comparisons were performed using the multcomp package. Kaplan-Meier curves were constructed using the “survfit” function of the survival package, and the “survdiff” function from survminer package was employed to assess differences in germination probabilities among treatments.
The interaction between the main factors (Smoke water: SW and Karrikinolide: KAR) and levels (1/100, 1/1000, 1/10000, and Control) was significant in both species (Table 2). This indicates that the effects of SW and KAR solutions on seed germination depends on the level of concentration (Table 2).
Table 2. Effects of smoke water and karrikinolide (main factors), their different concentrations 1/100, 1/1000, 1/10000, and Control (levels), and their interactions on seed germination of Boopis gracilis and Nicotiana linearis.
In Nicotiana linearis, germination was negatively affected by the highest SW concentration (1/100) in both years, with a more significant impact on seeds harvested in 2016 (Figure 2). Germination was significantly lower in the Control for seeds harvested in 2018 compared to those from 2016 and 2022 (Figure 2). In contrast, the highest concentration of KAR (1/100) increased germination 4-fold compared to the Control (Figure 2).
Figure 2. Mean seed germination (%) in response to high (1/100), medium (1/1000), and low (1/10000) concentrations of smoke water and karrikinolide (KAR) solutions for Nicotiana linearis and Boopis gracilis. For N. linearis, experiments were conducted in January 2017 and April 2023 with seeds harvested in 2016 and 2022 and treated with smoke water produced in November 2016 and April 2022. For B. gracilis, the experiment was conducted in April 2018 using seeds harvested in 2017 and treated with smoke water produced in November 2016. Seeds treated with KAR were harvested in 2018 for both species. Different lowercase letters indicate statistically significant differences.
In Boopis gracilis, germination increased significantly in KAR compared to SW solutions. All KAR concentrations significantly increased germination compared to both the Control and equivalent SW concentrations (Figure 2).
Kaplan–Meier survival analysis of Nicotiana linearis showed that the probability of germination was significantly different among KAR concentrations (χ2= 113, df= 7, p < 0.05) and SW concentrations for the 2016 and 2022 harvests (χ2= 113, df= 7, p < 0.05) (Figure 3). The highest KAR concentration (1/100) resulted in higher germination probability than the other concentrations and Control (p < 0.001). This concentration also accelerated germination, from day 24, with cumulative germination of 22%, compared to 6% in 1/1000, 8% in 1/10000, and 3% in Control (Figure 3; Supplementary Table S1). The highest SW concentration (1/100) reduced germination probability in seeds from both 2016 and 2022 harvests (p < 0.001), delaying germination onset and reducing cumulative germination (Figure 3).
Figure 3. Kaplan–Meier of germination curves for seeds of Nicotiana linearis and Boopis gracilis at smoke water and karrikinolide (KAR) solutions and concentrations: high (1/100), medium (1/1000), and low (1/10000). Different lowercase letters indicate significant differences among concentrations.
Seed germination of seeds harvested in 2016 was accelerated at the medium (1/1000) and lowest (1/10000) SW concentrations. Also, the germination probability at both concentrations was higher than that of the Control (p < 0.001) (Figure 3). The difference became more pronounced from day 11 onwards, with cumulative germination of 58% in 1/1000 and 60% in 1/10000, compared to 47% in the Control (Figure 3; Supplementary Table S1). In seeds harvested in 2022, the lowest SW concentration (1/10000) resulted in higher germination than 1/1000 and the Control (p < 0.001) (Figure 3), accelerating germination by day 8 day, with cumulative germination of 55%, compared to 26% in 1/1000 and 36% in the Control with similar cumulative germination thereafter (Figure 3; Supplementary Table S1).
Kaplan–Meier survival analysis of Boopis gracilis showed that the probability of germination was significatively different among concentrations in both the KAR (χ2= 113, df= 7, p < 0.05) and SW solutions (χ2= 113, df= 7, p < 0.05) (Figure 3). In KAR solutions, the probability of germination in 1/100 concentration was significantly higher than those at 1/1000, 1/10000 (p < 0.01), and Control (p < 0.001). The probability of germination at 1/1000 and 10000 was also higher than Control (p < 0.001). All KAR concentrations accelerated germination by day 17,particularly 1/100, which showed 43% cumulative germination compared to 12% in the Control (Figure 3; Supplementary Table S2). In SW treatment solutions, the germination probability was higher in 1/100, 1/1000, and Control compared to 1/10000 (p < 0.05). This difference started to become noticeable on day 19 when the cumulative germination was 36% in 1/100, 40% in 1/1000, 32% in the Control, and 24% in 1/10000 (Figure 3; Supplementary Table S2).
Our study shows how the application of smoke water and karrikinolide (KAR) solutions affects the seed germination of Boopis gracilis and Nicotiana linearis, two abundant post-fire ephemeral native species in semiarid Patagonian grasslands (Ghermandi et al., 2004). KAR enhanced and accelerated the germination in both species, with responses varying by concentration. Both species responded positively to the highest concentration (1/100), while smoke water did not stimulate germination in either species. Additionally, the highest concentration of smoke water (1/100) inhibited germination in N. linearis, likely due to the time elapsed between smoke water production and application. Nicotiana linearis seeds require after-ripening to release dormancy, as observed in other congeners (Leubner-Metzger, 2005), and KAR application promote and accelerated this process. Our findings are significant for understanding the ecological dynamics of fire ephemerals, which contribute to post-fire increases in species richness and diversity in grasslands (Ghermandi et al., 2004; Gonzalez et al., 2010).
All KAR solutions enhanced seed germination in B. gracilis, while only the highest KAR concentration significantly increased germination in N. linearis, achieving a fourfold increase compared to untreated seeds. This concentration also accelerated germination, with seeds initiating at 17 days in B. gracilis and 24 days in N. linearis. Similar processes have been observed in Nicotiana attenuata, where germination is regulated by interactions between KARs and phytohormones such as gibberellins (GA) and abscisic acid (ABA). KARs and GA synergistically promote germination, while ABA inhibits it (Schwachtje and Baldwin, 2004; Elsadek and Yousef, 2019); Furthermore, KARs are involved in the early induction of cell-cycle activity, facilitating radicle emergence (Nelson et al., 2012; Naghipour et al., 2016). Rapid germination and establishment facilitated by KARs may confer an ecological advantage in post-fire environments, enabling early colonization and resource capture (Bond and van Wilgen, 1996; Dayamba et al., 2008). Although physiological studies were not assessed in this work, GA and ABA may have influenced the observed germination responses to KAR solutions. Future research should investigate these interactions to clarify their role in promoting rapid germination.
Smoke water failed to stimulate seed germination in either species. Similarly, a previous study found no germination stimulation after exposing these species to aerosol smoke for 60 minutes (Gonzalez and Ghermandi, 2012). However, B. gracilis seeds responded positively to a combination of heat shock at 80°C for 5 minutes and smoke (Gonzalez and Ghermandi, 2012). The lack of smoke effect on germination may stem from the complex composition of smoke and the variability in its chemical concentrations (Mojzes et al., 2015). Smoke contains both stimulatory compounds, mixture of chemical compounds such as cyanohydrins, nitrogen oxides, catechol, and the terpenoid 1,8-cineole (Keeley and Fotheringham, 1997; Adriansz et al., 2010; Flematti et al., 2011; Wang et al., 2017; Khatoon et al., 2020), and inhibitory compounds, such as trimethylbutenolide and certain furanones (Light et al., 2010; Gupta et al., 2020).
In evaluating the smoke effect on germination, both commercial liquid smoke (without specification of its components or their proportions) or laboratory-produced smoke water are commonly used, although the methodologies lack standardization. Variations include differences in biomass type and amount, smoke water volume, and the duration of smoke bubbling. For instance, Chumpookam et al. (2012) burned 5 kg of Oryza sativa for 45 minutes, to produce 500 ml of smoke water, whereas Gupta et al. (2020) burned 26 kg of grass species to produce 26 l, though combustion time was not reported. Determining the specific compounds in smoke water and their proportions is challenging, and the lack of standardized experimental conditions limits the comparability of results. Our findings can be compared with those of Flematti et al. (2004), as we followed their methodology, although the type of biomass (i.e. different species) we used was different. In contrast, the results obtained with KAR solutions are more directly comparable since they consist of a single substance. We emphasize the need to standardize experimental methods to improve the comparability of findings across studies.
The highest smoke water concentration (1/100) significantly slowed and reduced N. linearis germination in both 2017 and 2023. Inhibitory compounds can be toxic at high concentrations or with prolonged exposure (Dixon et al., 1995; Light et al., 2010; Chiwocha et al., 2009; Gupta et al., 2020). Gupta et al. (2020) demonstrated that dilutions of smoke water mitigate the inhibitory effects of these compounds. Our findings support the conclusion that the highest concentration (1/100) exhibits greater inhibition compared to more diluted treatments and that the concentrations of stimulatory compounds are likely low.
The inhibitory effects of the highest concentration (1/100) decreased over time (in 2017, germination of N. linearis was 44% lower than in 2023), suggesting that inhibitory compounds may degrade during storage. The smoke water applied in 2017 was produced four months prior to the experiment (November 2016), while the smoke water used in 2023 was produced a year earlier (April 2022). A similar result was observed in B. gracilis when smoke water applied in February 2018, produced in November 2016 (with a storage period of one year and three months), did not exhibit inhibitory effects on germination. However, more studies are needed to confirm the degradation of inhibitory compounds in smoke water over time.
The germination percentage of N. linearis seeds varied significantly depending on the harvest year. Seeds harvested in 2016 (germinated in 2017), and 2022 (germinated in 2023) showed higher germination compared to seeds harvested and germinated in 2018, suggesting that after-ripening is required for optimal germination. After-ripening involves physiological changes in dry, mature seeds stored at room temperature, promoting dormancy release and enhancing germination (Fenner and Thompson, 2005). In Nicotiana species, germination occurs in two steps: testa rupture followed by endosperm rupture, regulated by hormonal dynamics, including the antagonistic interaction between GA and ABA (Leubner-Metzger, 2005; Finch-Savage and Leubner-Metzger, 2006). After-ripening reduces ABA levels, increasing sensitivity to GA and facilitating the sequential rupture of the testa and endosperm (Grappin et al., 2000). Gonzalez et al. (2022) reported higher germination in N. linearis of the same seed lot, after two months of incubation compared to this study, while Leubner-Metzger (2005) linked rapid testa rupture in N. tabacum to β-1,3-glucanase activation after 60 days of dry storage. Our findings suggest that seeds harvested in 2018 required longer after-ripening to overcome dormancy, leading to lower germination due to insufficient storage time. However, it is noteworthy that the highest KAR concentration (1/100) accelerated this process and increased seed germination.
Our findings enhance the understanding of fire-related cues in post-fire environments, where rapid germination is advantageous. We highlight the contrasting effects of smoke water and KAR on the seed germination of the two fire ephemerals. The chemical complexity of smoke water, its concentrations, and storage time all influence its efficacy in promoting germination, underlining the need for standardized methodologies. In contrast, KAR is a reliable germination stimulant, accelerating dormancy release of N. linearis seeds, even with shorter after-ripening periods. Understanding the responses of fire ephemerals to fire-related cues is relevant in fire-affected grasslands, where these species play a significant role in diversity and ecosystem recovery.
The original contributions presented in the study are included in the article/Supplementary Material. Further inquiries can be directed to the corresponding author.
SG: Conceptualization, Formal analysis, Investigation, Methodology, Supervision, Validation, Writing – original draft, Writing – review & editing. JF: Data curation, Formal analysis, Investigation, Methodology, Writing – review & editing. LG: Conceptualization, Funding acquisition, Investigation, Resources, Supervision, Validation, Writing – review & editing.
The author(s) declare financial support was received for the research, authorship, and/or publication of this article. This work (field and laboratory equipment) was supported by Agencia Nacional de Promoción Científica y Tecnológica, Grant Award Number: 0375-2015.
We thank Dr. Gavin Flematti, who kindly provided us the karrikinolide used in this study. We thank Leandro Ballerini San Ramón ranch manager for allowing us access to the study area. This work was supported by Agencia Nacional de Promoción Científica y Tecnológica, Grant Award Number: 0375-2015.
The authors declare that the research was conducted in the absence of any commercial or financial relationships that could be construed as a potential conflict of interest.
The author(s) declare that no Generative AI was used in the creation of this manuscript.
All claims expressed in this article are solely those of the authors and do not necessarily represent those of their affiliated organizations, or those of the publisher, the editors and the reviewers. Any product that may be evaluated in this article, or claim that may be made by its manufacturer, is not guaranteed or endorsed by the publisher.
The Supplementary Material for this article can be found online at: https://www.frontiersin.org/articles/10.3389/fpls.2025.1550692/full#supplementary-material
Adriansz, T. D., Rummey, J. M., Bennett, I. J. (2000). Solid phase extraction and subsequent identification by gas chromatography, mass-spectrometry of a germination cue present in smoky water. Anal. Lett. 33, 2793–2804. doi: 10.1080/00032710008543223
Baker, K. S., Steadman, K. J., Plummer, J. A., Merritt, D. J., Dixon, K. W. (2005a). Dormancy release in Australian fire ephemeral seeds during burial increases germination response to smoke water or heat. Seed Sci. Res. 15, 339–348. doi: 10.1079/SSR2005222
Baker, K. S., Steadman, K. J., Plummer, J. A., Merritt, D. J., Dixon, K. W. (2005b). The changing window of conditions that promotes germination of two fire ephemerals, Actinotus leucocephalus (Apiaceae) and Tersonia cyathiflora (Gyrostemonaceae). Ann. Bot. 96, 1225–1236. doi: 10.1093/aob/mci274
Baldwin, I. T., Staszak-Kozinski, L., Davidson, R. (1994). Up in smoke. I. Smoke-derived germination cues for post-fire annual, Nicotiana attenuata Torr ex Watson. J Chem Ecol. 20, 2345–237. doi: 10.1007/BF02033207
Bianchi, M. M. (2000). “Historia de fuego en Patagonia: Registro de carbón vegetal sedimentario durante el Post-glacial y el Holoceno en Lago Escondido (41° S; 72° W),” in Cuaternario y Ciencias del Ambiente, vol. 1. (Argentina: Asociación Geológica Argentina), 23–29.
Borza, J. K., Westerman, P. R., Liebman, M. (2007). Comparing estimates of seed viability in three foxtail (Setaria) species using the imbibed seed crush test with and without additional tetrazolium testing. Weed Technol. 21, 518–522. doi: 10.1614/WT-06-110
Buhk, C., Hensen, I. (2006). Fire seeders” during early post-fire succession and their quantitative importance in south-eastern Spain. J. Arid Environ. 66, 193–209. doi: 10.1016/j.jaridenv.2005.11.007
Chiwocha, S. D. S., Dixon, K. W., Flematti, G. R., Ghisalberti, E. L., Merritt, D. J., Nelson, D. C., et al. (2009). Karrikins: A new family of plant growth regulators in smoke. Plant Sci. 177, 252–256. doi: 10.1016/j.plantsci.2009.06.007
Chumpookam, J., Lin, H., Shiesh, C. C. (2012). Effect of smoke-water on seed germination and seedling growth of papaya (Carica papaya cv. Tainung No. 2). HortScience 47, 741–744. doi: 10.21273/HORTSCI.47.6.741
Crosti, R., Ladd, P. G., Dixon, K. W., Piotto, B. (2006). Post-fire germination: The effect of smoke on seeds of selected species from the central Mediterranean basin. For. Ecol. Manage 221, 306–312. doi: 10.1016/j.foreco.2005.10.005
Dayamba, S., Tigabu, M., Sawadogo, L., Oden, P. (2008). Seed germination of herbaceous and woody species of the Sudanian savanna-woodland in response to heat shock and smoke. For. Ecol. Manage 256, 462–470. doi: 10.1016/j.foreco.2008.04.051
de Lange, J. H., Boucher, C. (1990). Autecological studies on Audouinia capitata (Bruniaceae). I. Plant-derived smoke as a seed germination cue. S. Afr. J. Bot. 56, 700–703. doi: 10.1016/S0254-6299(16)31009-2
de Torres Curth, M. I., Ghermandi, L., Pfister, G. (2008). Los incendios en el noroeste de la Patagonia: su relación con las condiciones meteorológicas y la presión antrópica a lo largo de 20 años. Ecol. Austral 18, 153–167.
Dixon, K. W., Merritt, D. J., Flematti, G. R., Ghisalberti, E. L. (2009). Karrikinolide: A phytoreactive compound derived from smoke with applications in horticulture, ecological restoration, and agriculture. Acta Hortic. 813, 155–170. doi: 10.17660/ActaHortic.2009.813.20
Dixon, K. W., Roche, S., Pate, J. S. (1995). The promotive effect of smoke derived from burnt native vegetation on seed germination of Western Australian plants. Oecologia. 101, 185–192. doi: 10.1007/BF00317282
Elsadek, M., Yousef, E. (2019). Smoke-water enhances germination and seedling growth of four horticultural crops. Plants 8, 104. doi: 10.3390/plants8040104
Finch-Savage, W. E., Leubner-Metzger, G. (2006). Seed dormancy and the control of germination. New Phytol. 171, 501–523. doi: 10.1111/j.1469-8137.2006.01787.x
Flematti, G. R., Ghisalberti, E. L., Dixon, K. W., Trengove, R. D. (2004). A compound from smoke that promotes seed germination. Science 305, 977. doi: 10.1126/science.1099944
Flematti, G. R., Ghisalberti, E. L., Dixon, K. W., Trengove, R. D. (2009). Identification of alkyl substituted 2H-furo[2,3-c]pyran-2-ones as germination stimulants present in smoke. J. Agric. Food Chem. 57, 9475–9480. doi: 10.1021/jf9028128
Flematti, G. R., Scaffidi, A., Dixon, K. W., Smith, S. M., Ghisalberti, E. L. (2011). Production of the seed germination stimulant karrikinolide from combustion of simple carbohydrates. J. Agric. Food Chem. 59, 1195–1198. doi: 10.1021/jf1041728
Franzese, J., Ghermandi, L. (2012). Effect of fire on recruitment of two dominant perennial grasses with different palatability from semi-arid grasslands of NW Patagonia (Argentina). Plant Ecol. 213, 471–481. doi: 10.1007/s11258-011-9995-y
Ghermandi, L., Gonzalez, S. (2009). Diversity and functional groups dynamics affected by drought and fire in Patagonian grasslands. Écoscience 16, 408–417. doi: 10.2980/16-3-3264
Ghermandi, L., Gonzalez, S. L., Lescano, N. E., Oddi, F. J. (2013). Effects of fire severity on early recovery of Patagonian steppes. Int. J. Wildland Fire 22, 1055–1062. doi: 10.1071/WF12198
Ghermandi, L., Guthmann, N., Bran, D. (2004). Early post-fire succession in northwestern Patagonia grasslands. J. Veg. Sci. 15, 67–76. doi: 10.1111/j.1654-1103.2004.tb02238.x
Ghermandi, L., Lanorte, A., Oddi, F. J., Lasaponara, R. (2019). Assessing fire severity in semiarid environments with the DNBR and RDNBR indices. Glob. J. Sci. Front. Res. 19, 27–44.
Gómez-González, S., Sierra-Almeida, A., Cavieres, L. A. (2008). Does plant-derived smoke affect seed germination in dominant woody species of the Mediterranean matorral of central Chile? Forest Ecol. Manag. 255, 5–6. doi: 10.1016/j.foreco.2007.11.006
Gonzalez, S., Franzese, J., Ghermandi, L. (2010). Role of fire on Patagonian grasslands: changes in aboveground vegetation and soil seed bank. Glob. J. Sci. Front. Res. 2, 243–264.
Gonzalez, S. L., Ghermandi, L. (2008). Postfire seed bank dynamics in semiarid grasslands. Plant Ecol. 199, 175–185. doi: 10.1007/s11258-008-9422-1
Gonzalez, S. L., Ghermandi, L. (2012). Fire cue effects on seed germination of six species of northwestern Patagonian grasslands. Nat. Hazards Earth Syst. Sci. 12, 2753–2758. doi: 10.5194/nhess-12-2753-2012
Gonzalez, S., Salazar, C. V., Ghermandi, L. (2022). Burning the seed bank: Seed response of eight species to different fire intensities in Patagonian steppe. Appl. Vegetation Sci. 25, e12684. doi: 10.1111/avsc.12684
Grappin, P., Bouinot, D., Sotta, B., Miginiac, E., Jullien, M. (2000). Control of seed dormancy in Nicotiana plumbaginifolia: Post-imbibition abscisic acid synthesis imposes dormancy maintenance. Planta 210, 279–285. doi: 10.1007/PL00008135
Gupta, S., Hrdlička, J., Ngoroyemoto, N., Nemahunguni, N., Gucký, T., Novák, O., et al. (2020). Preparation and standardisation of smoke-water for seed germination and plant growth stimulation. J. Plant Growth Regul. 39, 338–345. doi: 10.1007/s00344-019-09985-y
Keeley, J. E., Bond, W. J. (1997). Convergent seed germination in South African fynbos and Californian chaparral. Plant Ecol. 133, 153–167. doi: 10.1023/A:1009748603202
Keeley, J. E., Bond, W. J., Bradstock, R. A., Pausas, J. G., Rundel, P. W. (2011a). Fire in Mediterranean Ecosystems: Ecology, Evolution and Management (Cambridge: Cambridge University Press).
Keeley, J. E., Fotheringham, C. J. (1997). Trace gas emissions and smoke-induced seed germination. Science 276, 1248–1250. doi: 10.1126/science.276.5316.1248
Keeley, J. E., Fotheringham, C. J. (1998). Mechanism of smoke-induced seed germination in a post-fire chaparral annual. J. Ecol. 86, 27–36. doi: 10.1046/j.1365-2745.1998.00230.x
Keeley, J. E., Fotheringham, C. J. (2000). “Role of fire in regeneration from seed,” in Seeds: The Ecology of Regeneration in Plant Communities, ed. Fenner, M. (Cambridge: Cambridge University Press), 311–330.
Keeley, J. E., McGinnis, T. W., Bollens, K. A. (2005). Seed germination of Sierra Nevada postfire chaparral species. Madroño 52, 175–181. doi: 10.3120/0024-9637
Keeley, J. E., Pausas, J. G., Rundel, P. W., Bond, W. J., Bradstock, R. A. (2011b). Fire as an evolutionary pressure shaping plant traits. Trends Plant Sci. 16, 406–411. doi: 10.1016/j.tplants.2011.04.002
Khatoon, A., Rehman, S., Aslam, M., Jamil, M., Komatsu, S. (2020). Plant-derived smoke affects biochemical mechanism on plant growth and seed germination. Int. J. Mol. Sci. 21, 7760. doi: 10.3390/ijms21207760
Leubner-Metzger, G. (2005). [amp]]beta;-1,3-Glucanase gene expression in low-hydrated seeds as a mechanism for dormancy release during tobacco after-ripening. Plant J. 41, 133–145. doi: 10.1111/j.1365-313X.2004.02284.x
Light, M. E., Burger, B. V., Staerk, D., Kohout, L., Van Staden, J. (2010). Butenolides from plant-derived smoke: Natural plant-growth regulators with antagonistic actions on seed germination. J. Natural Products 73, 267–269. doi: 10.1021/np900630w
Light, M. E., Daws, M. I., van Staden, J. (2009). Smoke-derived butenolide: towards understanding its biological effects. S. Afr. J. Bot. 75, 1–7. doi: 10.1016/j.sajb.2008.10.004
Light, M. E., Gardner, M. J., Jager, A. K., van Staden, J. (2002). Dual regulation of seed germination by smoke solutions. Plant Growth Regul. 37, 135–141. doi: 10.1023/A:1020536711989
Lloyd, M. V., Dixon, K. W., Sivasithamparam, K. (2000). Comparative effects of different smoke treatments on germination of Australian native plants. Austral Ecol. 25, 610–615. doi: 10.1111/j.1442-9993.2000.tb00066.x
Matthias, A. D., Fimbres, A., Sano, E. E., Post, D. F., Accioly, L., Batchily, A. K., et al. (2000). Surface roughness effects on soil albedo. Soil Sci. Soc Am. J. 64, 1035–1041. doi: 10.2136/sssaj2000.6431035x
Merritt, D. J., Kristiansen, M., Flematti, G. R., Turner, S. R., Ghisalberti, E. L., Trengove, R. D., et al. (2006). Effects of a butenolide present in smoke on light-mediated germination of Australian Asteraceae. Seed Sci. Res. 16, 29–35. doi: 10.1079/SSR2005232
Mojzes, A., Csontos, P., Kalapos, T. (2015). Is the positive response of seed germination to plant-derived smoke associated with plant traits? Acta Oecol 65, 24–31. doi: 10.1016/j.actao.2015.05.001
Naghipour, A. A., Bashari, H., Khajeddin, S. J., Tahmasebi, P., Iravani, M. (2016). Effects of smoke, ash and heat shock on seed germination of seven species from Central Zagros rangelands in the semi-arid region of Iran. Afr. J. Range Forage Sci. 33, 67–71. doi: 10.2989/10220119.2015.1119194
Nelson, D. C., Flematti, G. R., Ghisalberti, E. L., Dixon, K. W., Smith, S. M. (2012). Regulation of seed germination and seedling growth by chemical signals from burning vegetation. Annu. Rev. Plant Biol. 63, 107–130. doi: 10.1146/annurev-arplant-042811-105545
Pennacchio, M., Jefferson, L. V., Havens, K. (2007). Allelopathic effects of plant-derived aerosol smoke on seed germination of Arabidopsis thaliana (L.) Heynh. Int. J. Ecol. 065083, 4. doi: 10.1155/2007/65083
Pessacg, N. L., Flaherty, S., Solman, S. A., Pascual, M. A. (2020). Climate change in northern Patagonia: critical decrease in water resources. Theory Appl. Climatology 140, 807–822. doi: 10.1007/s00704-020-03104-8
R Core Team. (2019). R: A language and environment for statistical computing (R Foundation for Statistical Computing). Available at: https://www.R-project.org (Accessed February 13, 2025).
Rice, S., Ross, S. (2013). Smoke-induced germination in Phacelia strictaflora. Okla. Native Plant Rec 13, 48–54. doi: 10.22488/okstate.17.100098
Schwachtje, J., Baldwin, I. T. (2004). Smoke exposure alters endogenous gibberellin and abscisic acid pools and gibberellin sensitivity while eliciting germination in the post-fire annual, Nicotiana attenuata. Seed Sci. Res. 14, 51–60. doi: 10.1079/SSR2003154
Stevens, J. C., Merritt, D. J., Flematti, G. R., Ghisalberti, E. L., Dixon, K. W. (2007). Seed germination of agricultural weeds is promoted by the butenolide 3-methyl-2H-furo[2,3-c]pyran-2-one under laboratory and field conditions. Plant Soil 298, 113–124. doi: 10.1007/s11104-007-9344-z
Tormo, J., Moreira, B., Pausas, J. G. (2014). Field evidence of smoke-stimulated seedling emergence and establishment in Mediterranean Basin flora. J. Veg. Sci. 25, 771–777. doi: 10.1111/jvs.12120
Van Staden, J., Brown, N. A. C., Jäger, A. K., Johnson, T. A. (2000). Smoke as a germination cue. Plant Species Biol. 15, 167–178. doi: 10.1046/j.1442-1984.2000.00037.x
Van Staden, J., Drewes, F. E., Jäger, A. K. (1995). The search for germination stimulants in plant-derived smoke extracts. South African J Botany. 61, 260–263. doi: 10.1016/S0254-6299(15)30533-0
Van Staden, J., Jäger, A. K., Light, M. E., Burger, B. V. (2004). Isolation of the major germination cue from plant-derived smoke. S Afr J. Bot. 70, 654–659. doi: 10.1016/S0254-6299(15)30206-4
Wang, M., Schoettner, M., Xu, S., Paetz, C., Wilde, J., Baldwin, I. T., et al. (2017). Catechol, a major component of smoke, influences primary root growth and root hair elongation through reactive oxygen species-mediated redox signaling. New Phytol. 213, 1755–1770. doi: 10.1111/nph.2017.213.issue-4
Keywords: fire-related cues, KAR 1, native herbs, seed germination, semi-arid steppe, smoke water
Citation: Gonzalez S, Franzese J and Ghermandi L (2025) Germination responses of native fire ephemerals of Patagonian grasslands to smoke water and karrikinolide. Front. Plant Sci. 16:1550692. doi: 10.3389/fpls.2025.1550692
Received: 23 December 2024; Accepted: 03 February 2025;
Published: 19 February 2025.
Edited by:
Sergio Rossi, Université du Québec à Chicoutimi, CanadaReviewed by:
Manoj Gajanan Kulkarni, University of KwaZulu-Natal, South AfricaCopyright © 2025 Gonzalez, Franzese and Ghermandi. This is an open-access article distributed under the terms of the Creative Commons Attribution License (CC BY). The use, distribution or reproduction in other forums is permitted, provided the original author(s) and the copyright owner(s) are credited and that the original publication in this journal is cited, in accordance with accepted academic practice. No use, distribution or reproduction is permitted which does not comply with these terms.
*Correspondence: Sofía Gonzalez, c2dvbnphbGV6QGNvbWFodWUtY29uaWNldC5nb2IuYXI=
Disclaimer: All claims expressed in this article are solely those of the authors and do not necessarily represent those of their affiliated organizations, or those of the publisher, the editors and the reviewers. Any product that may be evaluated in this article or claim that may be made by its manufacturer is not guaranteed or endorsed by the publisher.
Research integrity at Frontiers
Learn more about the work of our research integrity team to safeguard the quality of each article we publish.