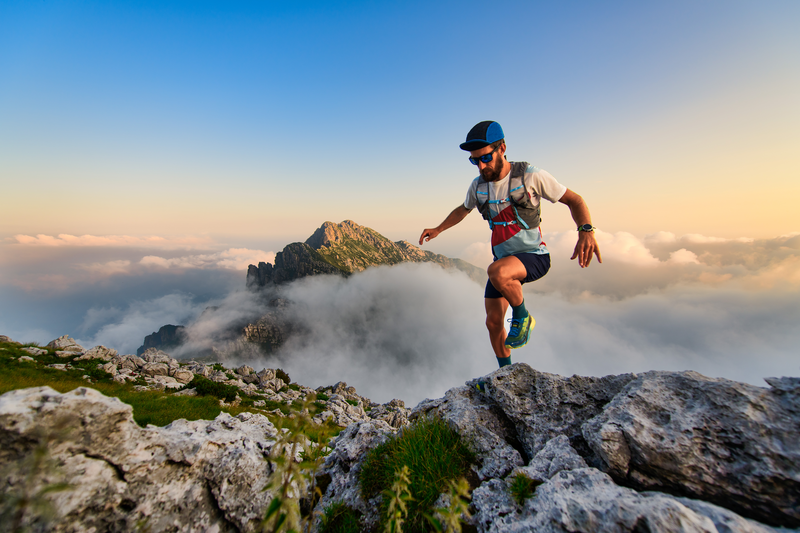
95% of researchers rate our articles as excellent or good
Learn more about the work of our research integrity team to safeguard the quality of each article we publish.
Find out more
ORIGINAL RESEARCH article
Front. Plant Sci. , 17 February 2025
Sec. Functional and Applied Plant Genomics
Volume 16 - 2025 | https://doi.org/10.3389/fpls.2025.1541944
This article is part of the Research Topic Pioneering Climate-Resilient Agriculture: Integrative Genomics and Phenomics for Crop Enhancement View all articles
Introduction: Abiotic stress significantly reduces the wheat yield by hindering several physiological processes in plant. Stay-green (SG) and stem reserve mobilization (SRM) are the two key physiological traits, which can contribute significantly to grain filling during stress period. Validation of genomic regions linked to SG and SRM is needed for its subsequent use in marker-assisted selection in breeding program.
Methods: Using a physiological and gene expression approach, quantitative trait loci (QTLs) for stay-green (SG) and stem reserve mobilization (SRM) were validated in a pot experiment study using contrasting recombinant inbred lines including its parental lines (HD3086/HI1500) in wheat. The experiment was laid down in a completely randomized design under normal (control, drought) and late sown (heat and combined stress) conditions during the 2022-2023 rabi season. Drought stress was imposed by withholding irrigation at the anthesis stage, whereas heat stress was imposed by 1-month late sowing compared to the normal sowing condition. Combined stress was imposed by 1-month late sowing along with restricted irrigation at the flowering stage. Superior lines (HDHI113 and HDHI87) had both SG and SRM traits, whereas inferior lines (HDHI185 and HDHI80) had contrasting traits, i.e., lower SG and SRM traits. HD3086 and HI1500 had SG and SRM traits respectively. Potential candidate genes were identified based on the flanking markers of the mapped QTLs using the BioMart tool in the Ensembl Plants database to validate the identified QTLs. Real-time gene expression was conducted with SG-linked genes in the flag leaf and SRM-linked genes in the peduncle.
Results and Discussion: In this study, HDHI113 and HDHI87 showed higher expression of SG-related genes in the flag leaf under stress conditions. Furthermore, HDHI113 and HDHI87 maintained higher chlorophyll a content of 7.08 and 6.62 mg/gDW, respectively, and higher net photosynthetic rates (PN) of 17.18 and 16.48 µmol CO2/m2/s, respectively, under the combined stress condition. However, these lines showed higher expression of SRM-linked genes in the peduncle under drought stress, indicating that drought stress aggravates SRM in wheat. HDHI113 and HDHI87 recorded higher 1,000-grain weights and spike weight differences under combined stress, further validating the identified QTLs being linked to SG and SRM traits. Henceforth, the identified QTLs can be transferred to developed wheat varieties through efficient breeding strategies for yield improvement in harsh climate conditions.
Wheat is an important staple food crop of humankind; however, its production potential is hindered due to drought, heat, and combined stress (Kumar et al., 2021; Meena et al., 2023). Due to a decline in precipitation and an increase in air temperature, the effects of global warming have become much more noticeable. In addition, crop yield is significantly affected by the frequent combined action of heat and drought stress (Sato et al., 2024). To ensure global food and nutritional security by 2050, there must be a major improvement in the rate of genetic gain in crop yield, quality, input use efficiency, and adaptation to biotic and abiotic challenges (Kumar et al., 2016). Delving into this, it is imperative to develop wheat varieties with increased resilience to abiotic stress. Stay-green (SG) and stem reserve mobilization (SRM) are two important physiological traits that can be targeted for yield improvement in wheat in the current era of global climate change. Thus, the identification of genomic regions linked to SG and SRM and the mining of candidate genes within the quantitative trait locus (QTL) region are of utmost importance for future breeding approaches for yield enhancement in wheat.
SG is a trait in which leaves retain green tissue from flowering to physiological maturity instead of senescing and it is considered an important trait for grain filling (Zhang et al., 2019). SG traits improve the grain yield by improving photosynthetic capacity, retaining higher chlorophyll content, and extending the grain filling period (Reynolds et al., 2000; Kumari et al., 2013; Pinto et al., 2016). It is well known that rubisco is the most abundant protein in the earth. Although both large (rbcL) and small subunits of proteins (rbcS) are required for the functionality of a protein, the amount of rubisco in plant has been thought to be influenced by the transcript levels of rbcS (Kumar et al., 2022). In addition, chlorophyll degradation is a marker of leaf senescence and is catalyzed by six chloroplast-localized chlorophyll catabolic enzymes namely, non-yellow coloring 1(NYC1)/NYC1-like (NOL), 7-hydroxymethyl chlorophyll a reductase (7-HCAR), magnesium dechelatase, pheophytin pheophorbide reductase (PPH), pheophorbide a oxygenase (PAO), and red chlorophyll catabolite reductase (RCCR) (Hörtensteiner and Kräutler, 2011). Furthermore, leaf senescence is also regulated by proteases such as aspartic protease (Kato et al., 2004), leaf nutritional status such as potassium (K+) in the flag leaf (Hosseini et al., 2016), and glutamate decarboxylase, a rate-limiting enzyme of GABA (gamma-aminobutyric acid) cycle (Khan et al., 2021). SG is also controlled by the photosystem-II activity of mesophyll cells and antioxidant status (Luo et al., 2013; De Simone et al., 2014). It was demonstrated that Δ-1-pyrroline-5-carboxylate synthase 2 (P5CS2), a proline biosynthetic enzyme, was highly expressed in SG sorghum line than in senescent lines (Johnson et al., 2015). Plant hormones such as cytokinin delay leaf senescence in plants (Wu et al., 2021; Glanz-Idan et al., 2022). Although isopentenyl transferase is the rate-limiting step in cytokinin biosynthesis, lonely guy (LOG) also catalyzes the direct activation of cytokinin biosynthesis (Kuroha et al., 2009), thereby contributing to SG traits.
During stress, stem reserves, particularly fructans, serve as a potential buffer for grain filling, when current leaf photosynthesis is inhibited by various stress (Blum, 1998). Stem reserves can contribute 20% to 40% of the final grain weight in non-stressed environments (Vignjevic et al., 2015), which can rise up to 70% under stressed conditions during grain filling (Rebetzke et al., 2008). The plant hormone ABA enhances the fructans metabolism in wheat (Valluru, 2015; Zhang et al., 2020) and a correlation between the expression of NCED1 and ABA content and the remobilization of stem water-soluble carbohydrates (WSCs) has been well demonstrated (Xu et al., 2016). Furthermore, sucrose non-fermenting-1 related protein kinase1 (SnRK1) regulates the transcriptional network through the phosphorylation of transcription factors in response to sugar starvation and energy stress (Baena-González et al., 2007; Mair et al., 2015). In addition, pentatricopeptide-repeat (PPR) containing protein genes were found to be associated with stem WSCs in bread wheat (Dong et al., 2016), indicating the possible involvement of PPR genes in SRM. The involvement of endoglucanase in stem WSC accumulation and remobilization in wheat was reported by Guerra et al. (2021) using the genome-wide association study (GWAS) approach. However, SG and SRM are two mutually exclusive traits (Blum, 1998) that can be combined to improve the grain filling in wheat under stress conditions. In our previous study, we mapped 11, 2, and 1 QTLs for soil plant analysis development (SPAD) value, leaf senescence rate, and SRM efficiency, respectively, in wheat under stress conditions (Taria et al., 2023).
To our knowledge, functional molecular markers for SG and SRM in wheat are not available. Furthermore, there is a lack of research on breaking the negative linkage between SG and SRM to pyramid these traits to enhance resource availability for grain filling under stress conditions. Moreover, the QTLs for SRM are unmapped for use in marker-assisted selection (MAS). Thus, it is necessary to unveil the genomic regions linked to these traits for further use in MAS. Given the fact mentioned above, four selected lines from our previous study (Taria et al., 2023) including two parental lines (HD3086/HI1500) were used to validate the identified QTLs by employing a physiological approach and in-vivo gene expression of QTL-linked genes. Leaf gas exchange studies were conducted using a portable infrared gas analyzer (IRGA) and photosynthetic pigment studies were accomplished using a spectrophotometer. Principal component analysis and clustering analysis were conducted to find out the latent variables and to check the variability in these lines. In this study, it was confirmed that the identified QTLs governed the SG and SRM traits by modulating the expression of the putative candidate genes. The identified genes can be further manipulated by gene editing technologies for further improvement in these traits. Furthermore, the superior lines, HDHI113 and HDHI87, can be used as donor parents for SG and SRM traits in elite wheat cultivars.
A total of six lines (HDHI113, HDHI87, HDHI185, HDHI80, HD3086, and HI1500) were grown at a pot culture experimental site at the Division of Plant Physiology, IARI, New Delhi. Four lines (HDHI113, HDHI87, HDHI185, and HDHI80) were selected from our earlier experiment during the 2021-2022 rabi season (Taria et al., 2023). The set of contrasting lines was selected using the multi-trait genotype-ideotype index (MGIDI) across multi-environment stress conditions (Olivoto and Nardino, 2021). HDHI113 and HDHI87 have combined SG and high SRM potential, whereas HDHI185 and HDHI80 have non-SG and lower SRM potential traits. The selected lines were evaluated under normal and late-sown conditions during the 2022-2023 rabi season. Drought stress was imposed at the anthesis stage by withholding irrigation after the booting stage. Heat stress was imposed by 1-month delayed sowing compared to the normal sown condition. In contrast, combined heat and drought (HD) stress was imposed by delayed 1-month sowing with restricted irrigation after the booting stage to impose drought stress at the anthesis stage. The temperature (minimum and maximum temperatures in °C) and rainfall (mm) during the wheat cropping season (2022-2023) are depicted in Supplementary Figure S1. The maximum and minimum temperatures at the time of anthesis under the control, drought, heat, and combined stress conditions are given in Supplementary Table S1. The average temperature at anthesis in the normal sown condition was 15.5°C, whereas an average temperature of 24.3°C was recorded in the late-sown condition.
The soil moisture content (SMC %) was estimated periodically from the anthesis stage up to physiological maturity under the control, drought, heat, and combined stress conditions. Fresh soil samples of 25g were taken from the rooting zone of potted plants and kept in a hot oven at 105°C. The SMC was calculated according to Faulkner et al. (1989) and calculated as
where FM represents soil fresh mass (g) and DM represents soil dry mass (g).
The SMC at different developmental stages under the control, drought, heat, and combined stress is depicted in Supplementary Figure S2.
The potential candidate genes were selected from identified QTLs for SG (SPAD and LSR) and SRM traits (Taria et al., 2023). To identify potential candidate genes, the flanking marker was used to search in the Chinese Spring (CS) wheat genome using the BioMart tool, available in the Ensembl Plants database (https://plants.ensembl.org/biomart/martview). The list of selected candidate genes and respective primers are given in Supplementary Table S2.
Primers were designed using the PrimerQuest™ tools available at Integrated DNA Technology (IDT) software (https://www.idtdna.com/PrimerQuest/Home/Index). The primers were designed by selecting quantitative PCR (qPCR) with two primer (intercalating dyes) icons available in IDT software. The selection of the primers was done by checking the hairpin (secondary) structures of the primer list and cross-checking their melting temperature and ΔG value (Supplementary Table S2). The amplicon size of the genes was also confirmed using the sequence manipulation suite (SMS) tool (https://www.bioinformatics.org/sms).
Plant flag leaf and peduncle RNA were isolated using a Sigma™ Plant Total RNA kit. For the removal of the trace amount of genomic DNA from the RNA sample, column DNase I digestion was used. The quality and quantification of isolated RNA were checked using 1.2% agarose gel electrophoresis (for integrity) and a spectrophotometer (Nano Drop™ 1000, Thermo Fisher Scientific) respectively. The gel was run for 5V/cm for 60 min.
A Thermo Scientific Verso cDNA synthesis kit was used for the synthesis of complementary DNA (cDNA) from the RNA sample. Anchored Oligo dT primers were used to provide flexible RNA priming for cDNA synthesis. In total, 20µl of the reaction mixture (5X cDNA synthesis buffer, dNTP mix, RNA primer, Verso Enzymes Mix, RNA template, and nuclease-free water) was provided with a thermal cycle of 30 min at 42°C for 1 cycle followed by inactivation for 2 min at 95°C for 1 cycle. To check the amplification of cDNA, a normal PCR reaction was set up with housekeeping gene primers (actin genes).
The real-time quantitative PCR reaction was performed using a Step One Plus TM real-time detection system to determine gene expression under the control, drought, heat, and combined stress conditions and 10µl of reaction mixture (SYBR, Forward primer, Reverse primer, cDNA, High ROX and Nuclease free water) was used in the reaction. The program was set at 95°C for denaturation followed by an annealing temperature of 58°C for primer binding. Finally, 72°C was set for the final extension. Two melt peaks were recorded for two genes put in one plate (24 samples for each gene) for confirmation of the specific gene amplification. Fold changes in gene expression (as 2ΔCt) were calculated by the comparative Ct method (Schmittgen and Livak, 2008), where ΔCt = [Ct target gene - Ct reference gene]. Actin was set as the reference gene (housekeeping gene) for normalization of the gene expression data.
At the anthesis stage, the concentration of chlorophyll and carotenoids in the flag leaves was determined according to the method outlined by Hiscox and Israelstam (1979). 6 mg of fresh leaf sample was added to the test tube containing 6 ml of dimethyl sulfoxide (DMSO). Tubes were kept in the dark for 4 h at 65 °C. The sample was then removed and cooled at room temperature. The absorbance of a known volume of leachates was measured at 663 and 645 nm for the leaf chlorophyll concentration and 480, 649, and 665 nm for the total leaf carotenoid concentration. Chlorophyll a (Chl a), chlorophyll b (Chl b), and total chlorophyll content (Total Chl) were estimated using the formula given by Arnon (1949), while total carotenoid content (Total Car) was determined by following the formula provided by Wellburn (1994) as follows:
For the calculation of the total carotenoids, the following formulae were used
Whereas,
For dry weight conversion, 1 g of fresh leaf tissue was kept in a hot oven (70 °C) to obtain the dry weight of the respective samples and the chlorophyll and carotenoid concentrations were expressed on a dry weight basis (Dwivedi et al., 2018). The percentage decrease of photosynthetic pigments under stress was calculated with reference to the control condition.
Gas exchange traits were measured in fully expanded (sunlit exposed) flag leaves using a portable IRGA, model LI6400XT (Li-COR Ltd., Lincoln, Nebraska, USA), at the anthesis stage. All the parameters were measured between 10:00 am and 11:00 am by providing an artificial light source of 1,000 µmol (photon) m-2 s-1 (Dwivedi et al., 2018). The recorded parameters were net photosynthetic rate (PN), stomatal conductance to water vapor (gsw), transpiration rate (E), and instantaneous water use efficiency (WUEi). WUEi was calculated by dividing the net photosynthetic rate (PN) by the transpiration rate (E) (Yan et al., 2015; Redhu et al., 2024). The percentage decrease in PN under stress was calculated in reference to the control condition.
For SG traits, we recorded the 1000-grain weight (TGW) of each line at physiological maturity. For SRM traits, all the leaves of the five main culms were defoliated at 12 days after anthesis and the stem weight was recorded. The remaining five defoliated culms were left in the field and were sampled at physiological maturity by allowing the mobilization of stem reserve from stems to grains. Spike weight difference (SWD) was calculated by adopting the methods described by Ehdaie et al. (2006) as follows:
The least significant difference (LSD) test was conducted to check significant differences among the lines at α = 0.05 (n=5). Principal components were extracted using the FactomineR package and enhanced visualization was carried out using the factoextra package. Agglomerative clustering was performed using Euclidean distance measures. A hierarchical rectangular dendrogram was created using the hclust function by following the agglomeration methods of Ward.D2. In addition, the cluster score was calculated as a weighted linear combination of physiological traits (i.e., summation of weightage multiplied by their respective physiological trait), where Xi referred to the mean value of the ith physiological trait of a given cluster and Wi referred to the weightage associated with the ith physiological trait of a given cluster. The weightage was obtained from the commonalities in the PCA analysis. The cluster score was calculated by the methods described by Nagar et al. (2015). Figures were created using the inbuilt ggplot2 package in R. Values are presented in the graphs as the means of traits, whereas vertical bars represent standard error.
The gene expression of candidate genes was visualized through a heat map using the “pheatmap” package in R. A spider network chart was prepared using the “fmsb” package in R.
The RT-PCR analysis revealed that the superior lines, HDHI113 and HDHI87, showed a higher expression of photosynthesis-related genes such as a LHCII-type chlorophyll a-b binding protein (TraesCS5B02G353200), PSB28 protein (TraesCS5B02G516600), chlorophyll synthase (TraesCS1D02G226100), and rubisco small subunit (TraesCS2B02G079100). These lines also recorded a higher expression of L-ascorbate peroxidase (TraesCS2B02G096200), pyrroline carboxylate synthase (TraesCS3B02G395900), and cytokinin riboside 5’-monophosphate phosphoribohydrolase LOGL10 (TraesCS1A02G156100) under the combined stress condition. Furthermore, the superior RILs exhibited a lower expression of the TraesCS1D02G241000 gene, encoding 7-HCAR, compared to the inferior RILs under the combined stress condition (Figure 1A). Expression analysis of senescence-associated genes were also studied. The superior RILs, HDHI113 and HDHI87, showed a lower expression of aspartyl protease family protein 2-like (TraesCS2D02G112800) compared to the inferior RILs and parents under combined stress conditions. Furthermore, expression of potassium transporter-9 (TraesCS2D02G106600) and glutamate decarboxylase1-like (TraesCS4B02G052300) was found to be higher in the superior RILs under the drought, heat, and combined stress conditions (Figure 1B).
Figure 1. Relative gene expression of stay-green-related genes (A), leaf senescence-related genes (B), and stem reserve mobilization-related genes (C) under drought, heat, and combined stress conditions.
Gene expression analysis of putative candidate genes for SRM was also conducted in the stem peduncle at 12 DAA under the multi-environment stress condition. In this study, we found a higher expression of PPR5 (TraesCS6B02G332800), endoglucanse-8-like (TraesCS6B02G368000), serine-threonine protein kinase-OSR1-like (TraesCS6B02G386100), and NCED1 (TraesCS6B02G298800) in the superior lines (HDHI113 and HDHI87) under the drought stress condition followed by the combined stress, control, and heat stress conditions, respectively (Figure 1C).
From the gene expression study, it was observed that the superior lines had a higher expression of SG-related genes and a lower expression of 7-HCAR under the drought, heat, and combined stress conditions. However, the superior lines had higher transcript levels of SRM-related candidate genes under the drought stress condition, indicating that drought stress aggravated the mobilization of stored carbon to grains.
Lower concentrations of Chla, Chlb, total Chl, and total Car were observed under drought, heat, and combined stress, respectively, compared to the control condition at the anthesis stage in wheat for all the lines tested, including the parental lines (Supplementary Table S3). However, a lower level of leaf photosynthetic pigment was observed under combined stress compared to individual stress. A significant difference in photosynthetic pigment was observed between the superior and inferior lines across all the environmental conditions. Under combined stress, HDHI113 recorded the highest chlorophyll a concentration of 7.08 mg/gDW (14.91% decrease), whereas HDHI185 recorded the lowest chlorophyll a concentration of 4.55 mg/gDW (30.09% decrease) (Figure 2A). For chlorophyll b, HDHI113 recorded the highest chlorophyll b concentration of 2.78 mg/gDW (8.24% decrease), whereas HDHI185 and HDHI80 recorded the lowest chlorophyll b concentration of 0.49 mg/gDW (79.53% and 79.58% decreases respectively) under the combined stress condition (Figure 2B). For total chlorophyll, HDHI113 recorded the highest total chlorophyll concentration of 9.86 mg/gDW (13.14% decrease), whereas HDHI185 recorded the lowest total chlorophyll concentration of 5.03 mg/gDW (43.31% decrease) under the combined stress condition (Figure 2C). For total carotenoid, HDHI113 recorded the highest total carotenoid concentration of 1.36 mg/gDW (29.14% decrease), whereas HDHI80 recorded the lowest total carotenoid concentration of 0.40 mg/gDW (71.86% decrease) under combined heat and drought stress (Figure 2D). Furthermore, one of the parents, HD3086, recorded higher photosynthetic pigments at the anthesis stage across multi-environment stress conditions, indicating potential SG traits.
Figure 2. Effect of drought, heat, and combined stress on chlorophyll a (A), chlorophyll b (B), total chlorophyll (C), and total carotenoid (D) at the anthesis stage. Different letters indicate significant genotype × treatment interactions among the lines using the LSD test at P<0.05.
From the photosynthetic pigment study, it was found that HDHI113 and HDHI87 maintained higher chlorophyll and carotenoid pigment levels under combined stress conditions.
In our study, significant differences in gas exchange traits were noted between the superior and inferior lines across all the environmental conditions (Supplementary Table S4). We observed a significant decrease in the PN under combined stress conditions compared to the control, drought, and heat stress conditions (Figure 3A, Supplementary Table S4). HDHI113 maintained a PN of 17.18 µmol CO2 m-2 s-1 (35.66% decrease) and HDHI185 recorded the lowest PN of 10.87 µmol CO2 m-2 s-1 (46.41% decrease) at the anthesis stage under combined stress conditions. For gsw, we observed a significant decrease in gsw in all the lines under stress conditions compared to the control plants (Figure 3B, Supplementary Table S4). Under combined stress conditions, the superior lines (HDHI113 and HDHI87) maintained a higher gsw (0.25 mol H2O m-2 s-1) and HDHI185 (inferior line) maintained the lowest gsw (0.16 mol H2O m-2 s-1).
Figure 3. Effect of drought, heat, and combined stress on net photosynthetic rate (PN) (A), stomatal conductance (gsw) (B), transpiration rate (E) (C), and instantaneous water use efficiency (WUEi) (D) at the anthesis stage. Different letters indicate significant genotype × treatment interactions among the lines using the LSD test at P<0.05.
E declined under drought stress in all the RILs including the parental lines (Figure 3C, Supplementary Table S4). Under heat stress conditions, the transpiration rate was enhanced in all the RILs compared to the control condition. The highest E of 7.84 mmol H2O m-2 s-1 was recorded by HDHI113 and the lowest E of 5.38 mmol H2O m-2 s-1 was recorded by HDHI185 under heat stress conditions. Under combined stress conditions, the highest E of 5.39 mmol H2O m-2 s-1 was recorded by HDHI87 and the lowest E of 4.32 mmol H2O m-2 s-1 was recorded by HDHI80. WUEi was enhanced for the superior lines, HDHI113 and HDHI87 under drought stress. However, it declined for the inferior lines, HDHI185 and HDHI80 (Figure 3D, Supplementary Table S4). Under heat stress conditions, WUEi was found to be decreased in all the tested lines compared to the control and drought stress conditions. However, HDHI87 recorded a higher WUEi (3.04 µmol CO2/mmol H2O) and HDHI80 recorded the lowest E of 2.49 µmol CO2/mmol H2O under heat stress conditions. Moreover, a higher WUEi was recorded by HDHI113 (3.21 µmol CO2/mmol H2O) and the lowest E of 2.38 µmol CO2/mmol H2O was recorded by HDHI185 under combined stress conditions.
From the gas exchange traits study, it was observed that HDHI113 and HDHI87 maintained higher PN under combined stress conditions.
Combined stress significantly reduced the TGW in all the RILs including the parents (Figure 4A). Moreover, HDHI113 recorded the highest TGW of 31.13 g and HDHI80 recorded the lowest TGW of 17.12 g under combined stress conditions (Supplementary Table S4). Furthermore, drought stress accelerated SRM to grains in all the RILs and HDHI113 recorded the highest SWD of 1.73 g under drought stress (Figure 4B, Supplementary Table S4) due to the higher SRM. However, heat stress resulted in a lower SWD in all the RILs.
Figure 4. Effect of drought, heat, and combined stress on 1000-grain weight (TGW) (A) and spike weight difference (SWD) (B) in wheat RILs including the parental lines. Different letters indicate significant genotype × treatment interactions among the lines using the LSD test at P<0.05.
Based on the SG traits, it was found that HDHI113 and HDHI87 recorded higher TGW under all environmental stress conditions. However, these lines recorded higher SWD under drought stress conditions based on SRM traits.
A comparison of the performance of the RILs including the parents revealed that there were differences in the trait values under the control, drought, heat, and combined stress conditions. With improved gas exchange and photosynthetic pigment characteristics, including grain yield, as shown in the spider network chart, HDHI113 and HDHI87 performed better in multi-environment conditions (Figures 5A-D).
Figure 5. Relative performance of RILs and parents under control (A), drought (B), heat (C), and combined stress conditions (D). Pn, net photosynthetic rate; Gsw, stomatal conductance; (E), transpiration rate; WUE, water use efficiency; TGW, 1000-grain weight; SWD, spike weight difference; Chl a, chlorophyll a; Chl b, chlorophyll b; Total Chl, total chlorophyll; Total Car, total carotenoid.
Using PCA, it was inferred that most of the traits were captured by Dimension 1 (Dim1) under control (Figure 6A), drought (Figure 6B), heat (Figure 6C), and combined stress conditions (Figure 6D). Under control conditions, traits including PN, TGW, E, Chl b, Total Car, Total Chl, and Chl a were captured by Dim1 (Supplementary Figure S3A). Under drought stress conditions, the maximum numbers of traits were captured by Dim1 except for TGW, SWD, Gsw, and E (Supplementary Figure S3B). Similarly, all the important traits were captured by Dim1 except for E, Gsw, and WUE under heat stress (Supplementary Figure S3C) and total Car, Chlb, SWD, and WUE under combined stress (Supplementary Figure S3D).
Figure 6. PCA biplot depicting the contribution of traits to Dim1 and Dim2 in the control (A), drought (B), heat (C) and combined stress conditions (D). Pn, net photosynthetic rate; Gsw, stomatal conductance; E, transpiration rate; WUE, water use efficiency; TGW, 1000-grain weight; SWD, spike weight difference; Chl a, chlorophyll a; Chl b, chlorophyll b; Total Chl, total chlorophyll; Total Car, total carotenoid.
As most of the traits were captured by Dim1, it can be inferred that these traits significantly contributed towards the grain filling in wheat.
Under each environmental condition, three clusters were formed. Under control conditions, each group contained two lines (Figure 7A). Group 1 retained HDHI113 and HDHI87 with the highest cluster score of 106.389 (Supplementary Table S5A). Under drought stress conditions, cluster sizes of 1, 3, and 2 were recorded in clusters I, II, and III respectively (Figure 7B). Group 1 retained only HDHI113 with the highest cluster score of 95.974 (Supplementary Table S5B). Under heat stress conditions, cluster sizes of 3, 1, and 2 in clusters I, II, and III respectively were recorded (Figure 7C). Group 1 retained HDHI113, HDHI87, and HD3086 with the highest cluster score of 77.258 (Supplementary Table S5C). Under combined stress conditions, each group contained two lines (Figure 7D). Group 1 retained HDHI113 and HDHI87 with the highest cluster score of 76.872 (Supplementary Table S5D).
Figure 7. Agglomerative hierarchical clustering depicting various clusters under control (A), drought (B), heat (C), and combined stress (D) conditions.
One of the main aims of wheat breeding globally is the development of stress-tolerant cultivars; this is particularly important for rain-fed conditions in India. The broadening of genetic diversity by employing diverse alleles for SG and SRM traits is needed for further improvements in wheat yield. Previous studies have reported numerous QTLs for SG traits, however, genomic regions linked to SRM and its potential candidate genes have not been explored so far. In this study, we validated the mapped QTLs by employing a gene expression and physiological approach.
In our study, we found a higher expression of SG-related genes such as chlorophyll synthase, chlorophyll a/b binding protein, Psb28, L-ascorbate peroxidase, pyrroline carboxylate synthase, and LOG10 in the superior lines under stress conditions. This indicated the influence of true positive SG-QTLs in maintaining the leaf greenness in the superior lines. It is well-known that chlorophyll a/b binding proteins are part of light-harvesting complexes (LHCs) and genes encoding for LHCs (Lhca and Lhcb) are of great importance for maintaining photosynthetic activity (Standfuss et al., 2005; Sato et al., 2009). In addition, Psb28 has been shown to protect the RC47 assembly intermediates of PSII and its absence impaired PSII recovery after photodamage in high-temperature and high-light conditions (Weisz et al., 2017). Through transcriptomic analysis, it was also found that the pyrolline carboxylate synthase 2 (P5CS2) gene was highly expressed in SG lines compared to senescent lines in sorghum (Johnson et al., 2015). It has been established that the application of 6-benzylaminopurine improved the grain yield and quality of wheat under water deficit conditions (Zarea and Karimi, 2023). The role of APX in inducing cold tolerance (Xu et al., 2014), heat tolerance (Zhang et al., 2023), and ROS detoxification (Guan et al., 2015; Yan et al., 2016; Shafi et al., 2017) is well reported.
Again, the higher expression of chlorophyll synthase in the superior lines (HDHI113 and HDHI87) was accompanied by higher levels of photosynthetic pigments under stress conditions. However, we found a lower level of photosynthetic pigments under combined stress. Our finding was in line with other studies in wheat (Zaefyzadeh et al., 2009; Sharifi and Mohammadkhani, 2016; Dwivedi et al., 2018; Yousaf et al., 2022), chickpea (Mafakheri et al., 2010), and tomato (Raja et al., 2020). Furthermore, we observed a lower level of photosynthetic pigments in wheat at the anthesis stage under heat stress compared to drought stress (Lamaoui et al., 2018; Yousaf et al., 2022). This greater decrease in leaf chlorophyll under heat stress in the sensitive lines was also probably because of an increase in proteolytic enzyme activity (Al-Khatib and Paulsen, 1984; Harding et al., 1990) and due to the damage to the thylakoid membrane and PSII complex (Ristic et al., 2007). Moreover, retention of chlorophyll content during post-flowering heat stress was reported to minimize yield losses in winter wheat (Fu et al., 2023).
In our study, we also studied the expression patterns of senescence-associated genes. We found a higher expression of 7-HCAR and aspartyl protease in the inferior lines (HDHI185 and HDHI80) under combined stress conditions. This higher expression was also substantiated by lower photosynthetic pigments in these inferior lines. The overexpression of 7-HCAR from cucumber in tobacco has been shown to hasten the dark-induced degradation of chlorophyll (Liu et al., 2021). However, we found a lower expression of K+-transporter-9 and glutamate decarboxylase genes in the inferior lines. The role of potassium in inhibiting drought-induced leaf senescence by promoting ABA degradation in barley has been reported (Hosseini et al., 2016). Furthermore, Arabidopsis thaliana, which produces less GABA, exhibited a greater vulnerability to drought stress (Mekonnen et al., 2016). Furthermore, it was also reported that the application of GABA to Oryza sativa seedlings, creeping bent grass, and Piper nigrum improves the performance of the individuals under heat and drought stress conditions respectively (Nayyar et al., 2014; Li et al., 2016; Vijayakumari and Puthur, 2016).
We also found greater downregulation of rubisco small subunit expression under stress conditions in the inferior lines. Rubisco is an important protein for photosynthetic carbon fixation (Ogren and Bowes, 1971; Le Roux et al., 2020). Amongst the senescence downregulated genes (SDGs), the rubisco small subunit (rbcs) and chlorophyll a/b binding (cab) proteins are mainly downregulated during the senescence process (Rampino et al., 2006). This lower expression of rubisco small subunit in the inferior lines was correlated with a lower PN value under stress conditions. In the present study, a decrease in PN under drought stress was due to stomatal limitation, i.e., we observed a lower gsw in our study (Centritto et al., 2003; Flexas et al., 2004; Grassi and Magnani, 2005; Erismann et al., 2008; Chaves et al., 2009; Peeva and Cornic, 2009), and non-stomatal limitation, i.e., lower chlorophyll content and lower rubisco activity (Xu et al., 2010; Simova-Stoilova et al., 2020). This decrease in gsw was probably due to lower soil moisture content (18.20%) at the anthesis stage (Supplementary Figure S2), which induced stomatal closing as a drought avoidance response (Chaves et al., 2009; Ghotbi-Ravandi et al., 2014). The decrease in gsw was substantiated by a decrease in E. The lowering of E under drought stress was reported by various researchers in wheat (Li et al., 2017; Itam et al., 2021; Guizani et al., 2023), naked oat (Zhang et al., 2022), and cowpea (Singh and Reddy, 2011). An increase in WUEi in the superior lines under drought stress was reported by previous researchers in wheat (Van den Boogaard et al., 1997; Sikder et al., 2016) and cowpea (Anyia and Herzog, 2004) as well. However, we observed a greater decrease in PN under combined stress than heat stress at the anthesis stage. In C3 plants such as wheat, an increase in temperature above the optimal growth temperature generally decreases PN (Berry and Bjorkman, 1980). In our study, a decrease in PN under heat stress was due to lower gsw, except for HI1500 and HDHI80 (as compared to drought stress), lower chlorophyll content, and lower rubisco activity. However, a decrease in gsw under heat stress was decoupled from increased E and lower WUEi. Moreover, higher E was observed in HDHI113 under heat stress, probably to make the canopy cool (Ayeneh et al., 2002; Gommers, 2020), and it has been reported that heat-tolerant lines with higher yields exhibited better cooling capacity (Reynolds et al., 1997). Moreover, greater E under heat stress was also because a higher VPD is a natural consequence of higher temperatures (Sharma et al., 2015) and higher stomatal conductance, as transpiration rate can be approximated as VPD×stomatal conductance (Cowan, 1977; Will et al., 2013). Increased E under heat stress was also reported in wheat (Sharma et al., 2015; Nagar et al., 2021). Moreover, decreases in WUEi under heat stress were probably because of higher E compared to the control condition (Sharma et al., 2015) and a decrease in PN (Agarwal et al., 2021), which was the case in our findings. The decrease in PN under combined stress was due to a lowering of gsw, lower chlorophyll content, and lower rubisco activity (Kumar et al., 2021; Meena et al., 2023). A decrease in gsw was reported by various researchers in plants under combined stress (El Habti et al., 2020; MaChado and Paulsen, 2001; Shah and Paulsen, 2003). It was reported that the maintenance of E during combined stress is indispensable for sustaining wheat productivity (El Habti et al., 2020). According to Zandalinas et al. (2016), the ability of citrus trees to withstand the combined effects of heat and drought was facilitated by an increase in E. The higher WUEi in the superior lines under combined stress was probably due to the better trade-off mechanisms of the superior RILs leading to the maximizing of carbon assimilation and reducing water loss through transpiration (El Habti et al., 2020).
From this study, it was inferred that HDHI113 and HDHI87 maintained their SG traits under stress conditions by upregulating the SG-linked gene expression to maintain leaf greenness and by activating ROS scavenging systems such as ascorbate peroxidase.
During the post-anthesis periods, the photosynthetic capacity of leaves declines due to natural senescence and various biotic and abiotic stresses. There is a rapid decline in the current leaf photosynthesis rate under terminal drought conditions (Johnson et al., 1981). Grain development in wheat depends upon carbohydrates from three sources: (i) carbohydrates produced before anthesis stored in the stem and remobilized to grains during grain filling, (ii) carbohydrates produced after anthesis and translocated directly to the grains, and (iii) carbohydrates produced after anthesis but stored temporarily in the stem before being remobilized to the grains (Gallagher et al., 1975; Daniels et al., 1982; Kobata et al., 1992). Thus, as the highest accumulation of water-soluble carbohydrates (WSCs) occurred at 12 DAA (Gurumurthy et al., 2023) and all the leaves including the flag leaves were defoliated at 12 DAA, spike weight difference between 12 DAA and at physiological maturity was most likely due to the mobilization of stored reserves from the stems to the grains.
The resulting higher expression of PPR5 and endoglucanse-8 like in the superior lines under drought stress in the peduncle at 12 DAA was probably to mobilize the stored carbon pool to the grain for sink requirements. This was further substantiated by the observation that the highest starch metabolism occurs at 14 days after anthesis in wheat (Cimini et al., 2015). The role of PPR proteins in inducing drought (Luo et al., 2022) and salt tolerance (Lu et al., 2022) in rice has been reported. The role of β-glucanase in plant development and adaptive response has also been stated (Perrot et al., 2022). In addition, the enhanced activity of ST protein kinase OSR1-like under drought stress in the superior lines was probably due to the fact that the ABA level enhanced the activity of the ST protein kinase for carbon mobilization. CaDIK1, an ST kinase, has been reported to regulate drought tolerance by modulating ABA sensitivity in pepper (Lim et al., 2020). Hu et al. (2022) reported that SnRK1 regulates the transport of non-structural carbohydrates from the sheath to the grain during grain filling in rice. Exposure to drought stress was shown to induce NCED gene expression in maize (Tan et al., 1997), tomato (Burbidge et al., 1999), Arabidopsis (Iuchi et al., 2001), and cowpea (Iuchi et al., 2000). The increase in the activity of NCED1 under drought stress might be due to the fact that the stress level enhanced the ABA level to provide stress tolerance to plants through fructans breakdown (Xiong and Zhu, 2003). Moreover, the role of ABA in SRM has also been demonstrated (Xu et al., 2016), indicating the role of ABA in the mobilization of temporarily stored carbon to grain under abiotic stress conditions, particularly under drought stress.
In this study, it was observed that drought stress enhanced the expression of key genes linked to SRM, thereby causing the mobilization of stored carbons in the stems to the grains.
The combined effect of heat and drought stress significantly decreased the grain yield in all the RILs (Kumar et al., 2021; Meena et al., 2023). Drought and heat stress decrease the grain filling duration and grain filling rate, thereby reducing the grain yield (Prasad et al., 2008). However, drought stress enhanced the SWD due to a higher SRM (Gurumurthy et al., 2023; Ntawuguranayo et al., 2024). In the cluster analysis, it was shown that HDHI1113 and HDHI87 were grouped together with the highest cluster score. The PCA also revealed that most of the traits contributed towards the total variance, indicating a significant contribution of SG and SRM traits towards grain filling in wheat under stress conditions. The spider chart also clearly displays the significant contribution of SG and SRM traits to grain yield under stress conditions, which can be further exploited for yield improvements.
In this era of global climate change, there is an urgent need to develop new crop varieties to meet the ever-increasing demand for food. The identification of genomic regions regulating physiological traits will pave the way to breeding new and improved crop varieties. SG and SRM are crucial for grain filling in wheat during abiotic stress. In this study, we validated our mapped QTLs for SG and SRM by employing a gene expression and physiological approach, which can be used for MAS to improve the yield potential under stress conditions. By employing selection indexes, we selected two superior lines (HDHI113 and HDHI87) that can be used as suitable donor parents for SG and SRM traits in elite wheat cultivars. Moreover, the identified QTL-linked markers will help wheat breeders to accumulate desirable allelic combinations in future breeding programs. Thus, understanding the genetic basis of SG and SRM will improve crop productivity under abiotic stress conditions not only in wheat but in other cereal crops as well.
The datasets presented in this study can be found in online repositories. The names of the repository/repositories and accession number(s) can be found in the article/Supplementary Material.
ST: Investigation, Methodology, Visualization, Writing – original draft. AjA: Conceptualization, Supervision, Writing – review & editing. SudK: Data curation, Formal analysis, Methodology, Writing – review & editing. HK: Supervision, Writing- review & editing. BS: Formal analysis, Writing – review & editing. SM: Writing – review & editing. AC: Writing – review & editing. KS: Writing – review & editing. JP: Resources, Supervision, Writing – review & editing. PS: Resources, Supervision, Writing – review & editing. BA: Writing – review & editing. SusK: Writing – review & editing. AyA: Writing – review & editing.
The author(s) declare that financial support was received for the research, authorship, and/or publication of this article. This work was funded by the Science and Engineering Research Board (SERB) research grant (CRG-2020-00339-SERB). Part of this research was supported by a grant from the Bill & Melinda Gates Foundation (Grant number: # OPP1215722).
We would like to acknowledge the Indian Agricultural Research Institute (ICAR), New Delhi for providing research facilities to conduct this work. ST acknowledges ICAR-Central Agroforestry Research Institute, Jhansi, Uttar Pradesh for granting study leave to complete this work as part of their Ph.D. thesis.
The authors declare that the research was conducted in the absence of any commercial or financial relationships that could be construed as a potential conflict of interest.
The author(s) declare that no Generative AI was used in the creation of this manuscript.
All claims expressed in this article are solely those of the authors and do not necessarily represent those of their affiliated organizations, or those of the publisher, the editors and the reviewers. Any product that may be evaluated in this article, or claim that may be made by its manufacturer, is not guaranteed or endorsed by the publisher.
The Supplementary Material for this article can be found online at: https://www.frontiersin.org/articles/10.3389/fpls.2025.1541944/full#supplementary-material
Agarwal, V. P., Gupta, N. K., Gupta, S., Singh, G. (2021). Screening of wheat germplasm for terminal heat tolerance under hyper-arid conditions. Cereal Res. Commun. 49, 375–383. doi: 10.1007/s42976-020-00116-y
Al-Khatib, K., Paulsen, G. M. (1984). Mode of high temperature injury to wheat during grain development. Physiol. Plant. 61, 363–368. doi: 10.1111/j.1399-3054.1984.tb06341.x
Anyia, A. O., Herzog, H. (2004). Water-use efficiency, leaf area and leaf gas exchange of cowpeas under mid-season drought. Eur. J. Agron. 20, 327–339. doi: 10.1016/S1161-0301(03)00038-8
Arnon, D. I. (1949). Copper enzymes in isolated chloroplasts. Polyphenoloxidase in Beta vulgaris. Plant Physiol. 24, 1. doi: 10.1104/pp.24.1.1
Ayeneh, A., Van Ginkel, M., Reynolds, M. P., Ammar, K. (2002). Comparison of leaf, spike, peduncle and canopy temperature depression in wheat under heat stress. Field Crops Res. 79, 173–184. doi: 10.1016/S0378-4290(02)00138-7
Baena-González, E., Rolland, F., Thevelein, J. M., Sheen, J. (2007). A central integrator of transcription networks in plant stress and energy signalling. Nature 448, 938–942. doi: 10.1038/nature06069
Berry, J., Bjorkman, O. (1980). Photosynthetic response and adaptation to temperature in higher plants. Annu. Rev. Plant Physiol. 31, 491–543. doi: 10.1146/annurev.pp.31.060180.002423
Blum, A. (1998). Improving wheat grain filling under stress by stem reserve mobilisation. Euphytica 100, 77–83. doi: 10.1023/A:1018303922482
Burbidge, A., Grieve, T. M., Jackson, A., Thompson, A., McCarty, D. R., Taylor, I. B. (1999). Characterization of the ABA-deficient tomato mutant notabilis and its relationship with maize Vp14. Plant J. 17, 427–431. doi: 10.1046/j.1365-313X.1999.00386.x
Centritto, M., Loreto, F., Chartzoulakis, K. (2003). The use of low [CO2] to estimate diffusional and non-diffusional limitations of photosynthetic capacity of salt-stressed olive saplings. Plant Cell Environ. 26, 585–594. doi: 10.1046/j.1365-3040.2003.00993.x
Chaves, M. M., Flexas, J., Pinheiro, C. (2009). Photosynthesis under drought and salt stress: regulation mechanisms from whole plant to cell. Ann. Bot. 103, 551–560. doi: 10.1093/aob/mcn125
Cimini, S., Locato, V., Vergauwen, R., Paradiso, A., Cecchini, C., Vandenpoel, L., et al. (2015). Fructan biosynthesis and degradation as part of plant metabolism controlling sugar fluxes during durum wheat kernel maturation. Front. Plant Sci. 6, 89. doi: 10.3389/fpls.2015.00089
Cowan, I. R. (1977). Stomatal behaviour and environment. Adv. Bot. Res. 4, 117–128. doi: 10.1016/S00652296(08)60370-5
Daniels, R. W., Alcock, M. B., Scarisbrick, D. H. (1982). A reappraisal of stem reserve contribution to grain yield in spring barley (Hordeum vulgare L.). J. Agric. Sci. 98, 347–355. doi: 10.1017/S0021859600041897
De Simone, V., Soccio, M., Borrelli, G. M., Pastore, D., Trono, D. (2014). Stay-green trait-antioxidant status interrelationship in durum wheat (Triticum durum) flag leaf during post-flowering. J. Plant Res. 127, 159–171. doi: 10.1007/s10265-013-0584-0
Dong, Y., Liu, J., Zhang, Y., Geng, H., Rasheed, A., Xiao, Y., et al. (2016). Genome-wide association of stem water soluble carbohydrates in bread wheat. PloS One 11, e0164293. doi: 10.1371/journal.pone.0164293
Dwivedi, S. K., Arora, A., Singh, V. P., Singh, G. P. (2018). Induction of water deficit tolerance in wheat due to exogenous application of plant growth regulators: membrane stability, water relations and photosynthesis. Photosynthetica 56, 478–486. doi: 10.1007/s11099-017-0695-2
Ehdaie, B., Alloush, G. A., Madore, M. A., Waines, J. G. (2006). Genotypic variation for stem reserves and mobilization in wheat: I. Post-anthesis changes in internode dry matter. Crop Sci. 46, 735–746. doi: 10.2135/cropsci2005.04-0033
El Habti, A., Fleury, D., Jewell, N., Garnett, T., Tricker, P. J. (2020). Tolerance of combined drought and heat stress is associated with transpiration maintenance and water-soluble carbohydrates in wheat grains. Front. Plant Sci. 11, 568693. doi: 10.3389/fpls.2020.568693
Erismann, N. D. M., Caruso MaChado, E., Sant’Anna Tucci, M. L. (2008). Photosynthetic limitation by CO2 diffusion in drought stressed orange leaves on three rootstocks. Photosynth. Res. 96, 163–172. doi: 10.1007/s11120-008-9297-y
Faulkner, S. P., Patrick, W. H., Jr., Gambrell, R. P. (1989). Field techniques for measuring wetland soil parameters. Soil Sci. Soc. America J. 53, 883–890. doi: 10.2136/sssaj1989.03615995005300030042x
Flexas, J., Bota, J., Loreto, F., Cornic, G., Sharkey, T. D. (2004). Diffusive and metabolic limitations to photosynthesis under drought and salinity in C3 plants. Plant Biol. 6, 269–279. doi: 10.1055/s-2004-820867
Fu, J., Bowden, R. L., Jagadish, S. K., Prasad, P. V. (2023). Genetic variation for terminal heat stress tolerance in winter wheat. Front. Plant Sci. 14. doi: 10.3389/fpls.2023.1132108
Gallagher, J. N., Biscoe, P. V., Scott, R. K. (1975). Barley and its environment. V. Stability of grain weight. J. Appl. Ecol. 12, 319–336. doi: 10.2307/2401735
Ghotbi-Ravandi, A. A., Shahbazi, M., Shariati, M., Mulo, P. (2014). Effects of mild and severe drought stress on photosynthetic efficiency in tolerant and susceptible barley (Hordeum vulgare L.) genotypes. J. Agron. Crop Sci. 200, 403–415. doi: 10.1111/jac.12062
Glanz-Idan, N., Lach, M., Tarkowski, P., Vrobel, O., Wolf, S. (2022). Delayed leaf senescence by upregulation of cytokinin biosynthesis specifically in tomato roots. Front. Plant Sci. 13, 922106. doi: 10.3389/fpls.2022.922106
Gommers, C. (2020). Keep cool and Open Up: Temperature induced stomatal opening. Plant Physiol. 182, 1188–1189. doi: 10.1104/pp.20.00158
Grassi, G., Magnani, F. (2005). Stomatal, mesophyll conductance and biochemical limitations to photosynthesis as affected by drought and leaf ontogeny in ash and oak trees. Plant Cell Environ. 28, 834–849. doi: 10.1111/j.1365-3040.2005.01333.x
Guan, Q., Wang, Z., Wang, X., Takano, T., Liu, S. (2015). A peroxisomal APX from Puccinellia tenuiflora improves the abiotic stress tolerance of transgenic Arabidopsis thaliana through decreasing of H2O2 accumulation. J. Plant Physiol. 175, 183–191. doi: 10.1016/j.jplph.2014.10.020
Guerra, F. P., Yáñez, A., Matus, I., Del Pozo, A. (2021). Genome-wide association of stem carbohydrate accumulation and remobilization during grain growth in bread wheat (Triticum aestivum L.) in mediterranean environments. Plants 10, 539. doi: 10.3390/plants10030539
Guizani, A., Askri, H., Amenta, M. L., Defez, R., Babay, E., Bianco, C., et al. (2023). Drought responsiveness in six wheat genotypes: identification of stress resistance indicators. Front. Plant Sci. 14, 1232583. doi: 10.3389/fpls.2023.1232583
Gurumurthy, S., Arora, A., Krishna, H., Chinnusamy, V., Hazra, K. K. (2023). Genotypic capacity of post-anthesis stem reserve mobilization in wheat for yield sustainability under drought and heat stress in the subtropical region. Front. Genet. 14. doi: 10.3389/fgene.2023.1180941
Harding, S. A., Guikema, J. A., Paulsen, G. M. (1990). Photosynthetic decline from high temperature stress during maturation of wheat: I. Interaction with senescence processes. Plant Physiol. 92, 648–653. doi: 10.1104/pp.92.3.648
Hiscox, J. D., Israelstam, G. F. (1979). A method for the extraction of chlorophyll from leaf tissue without maceration. Can. J. Bot. 57, 1332–1334. doi: 10.1139/b79-163
Hörtensteiner, S., Kräutler, B. (2011). Chlorophyll breakdown in higher plants. Biochim. Biophys. Acta (BBA)-Bioenerg. 1807, 977–988. doi: 10.1016/j.bbabio.2010.12.007
Hosseini, S. A., Hajirezaei, M. R., Seiler, C., Sreenivasulu, N., von Wirén, N. (2016). A potential role of flag leaf potassium in conferring tolerance to drought-induced leaf senescence in barley. Front. Plant Sci. 7. doi: 10.3389/fpls.2016.00206
Hu, Y., Liu, J., Lin, Y., Xu, X., Xia, Y., Bai, J., et al. (2022). Sucrose nonfermenting-1-related protein kinase 1 regulates sheath-to-panicle transport of nonstructural carbohydrates during rice grain filling. Plant Physiol. 189, 1694–1714. doi: 10.1093/plphys/kiac124
Itam, M. O., Wahbi, A., Fujimaki, H., Tsujimoto, H. (2021). Transpiration response of two bread wheat lines differing in drought resilience and their backcross parent under dry-down conditions. Breed. Sci. 71, 575–583. doi: 10.1270/jsbbs.20154
Iuchi, S., Kobayashi, M., Taji, T., Naramoto, M., Seki, M., Kato, T., et al. (2001). Regulation of drought tolerance by gene manipulation of 9-cis-epoxycarotenoid dioxygenase, a key enzyme in abscisic acid biosynthesis in Arabidopsis. Plant J. 27, 325–333. doi: 10.1046/j.1365-313x.2001.01096.x
Iuchi, S., Kobayashi, M., Yamaguchi-Shinozaki, K., Shinozaki, K. (2000). A stress-inducible gene for 9-cis-epoxycarotenoid dioxygenase involved in abscisic acid biosynthesis under water stress in drought-tolerant cowpea. Plant Physiol. 123, 553–562. doi: 10.1104/pp.123.2.553
Johnson, S. M., Cummins, I., Lim, F. L., Slabas, A. R., Knight, M. R. (2015). Transcriptomic analysis comparing stay-green and senescent Sorghum bicolor lines identifies a role for proline biosynthesis in the stay-green trait. J. Exp. Bot. 66, 7061–7073. doi: 10.1093/jxb/erv405
Johnson, R. C., Witters, R. E., Ciha, A. J. (1981). Daily patterns of apparent photosynthesis and evapotranspiration in a developing winter wheat crop 1. Agron. J. 73, 414–418. doi: 10.2134/agronj1981.00021962007300030008x
Kato, Y., Murakami, S., Yamamoto, Y., Chatani, H., Kondo, Y., Nakano, T., et al. (2004). The DNA-binding protease, CND41, and the degradation of ribulose-1, 5-bisphosphate carboxylase/oxygenase in senescent leaves of tobacco. Planta 220, 97–104. doi: 10.1007/s00425-004-1328-0
Khan, M. I. R., Jalil, S. U., Chopra, P., Chhillar, H., Ferrante, A., Khan, N. A., et al. (2021). Role of GABA in plant growth, development and senescence. Plant Gene 26, 100283. doi: 10.1016/j.plgene.2021.100283
Kobata, T., Palta, J. A., Turner, N. C. (1992). Rate of development of postanthesis water deficits and grain filling of spring wheat. Crop Sci. 32, 1238–1242. doi: 10.2135/cropsci1992.0011183X003200050035x
Kumar, R., Harikrishna, Barman, D., Ghimire, O. P., Gurumurthy, S., Singh, P. K., et al. (2022). Stay-green trait serves as yield stability attribute under combined heat and drought stress in wheat (Triticum aestivum L.). Plant Growth Regul. 96, 67–78. doi: 10.1007/s10725-021-00758-w
Kumar, R., Harikrishna, Ghimire, O., Barman, D., Chinnusamy, V., Singh, P. K., et al. (2021). Stay-green trait ameliorates combined heat and drought stress in wheat. Indian J. Agric. Sci. 91, 100–104. doi: 10.56093/ijas.v91i4.112682
Kumar, S., Raju, D., Sahoo, R. N., Chinnusamy, V. (2016). Phenomics: unlocking the hidden genetic variation for breaking the barriers in yield and stress tolerance. Indian J. Plant Physiol. 21, 409–419. doi: 10.1007/s40502-016-0261-0
Kumari, M., Pudake, R. N., Singh, V. P., Joshi, A. K. (2013). Association of staygreen trait with canopy temperature depression and yield traits under terminal heat stress in wheat (Triticum aestivum L.). Euphytica 190, 87–97. doi: 10.1007/s10681-012-0780-3
Kuroha, T., Tokunaga, H., Kojima, M., Ueda, N., Ishida, T., Nagawa, S., et al. (2009). Functional analyses of LONELY GUY cytokinin-activating enzymes reveal the importance of the direct activation pathway in Arabidopsis. Plant Cell 21, 3152–3169. doi: 10.1105/tpc.109.068676
Lamaoui, M., Jemo, M., Datla, R., Bekkaoui, F. (2018). Heat and drought stresses in crops and approaches for their mitigation. Front. Chem. 6. doi: 10.3389/fchem.2018.00026
Le Roux, M. S. L., Burger, N. F. V., Vlok, M., Kunert, K. J., Cullis, C. A., Botha, A. M. (2020). Wheat line “RYNO3936” is associated with delayed water stress-induced leaf senescence and rapid water-deficit stress recovery. Front. Plant Sci. 11, 523678. doi: 10.3389/fpls.2020.01053
Li, Y., Li, H., Li, Y., Zhang, S. (2017). Improving water-use efficiency by decreasing stomatal conductance and transpiration rate to maintain higher ear photosynthetic rate in drought-resistant wheat. Crop J. 5, 231–239. doi: 10.1016/j.cj.2017.01.001
Li, Z., Yu, J., Peng, Y., Huang, B. (2016). Metabolic pathways regulated by γ-aminobutyric acid (GABA) contributing to heat tolerance in creeping bentgrass (Agrostis stolonifera). Sci. Rep. 6, 30338. doi: 10.1038/srep30338
Lim, J., Lim, C. W., Lee, S. C. (2020). Pepper novel serine-threonine kinase CaDIK1 regulates drought tolerance via modulating ABA sensitivity. Front. Plant Sci. 11, 1133. doi: 10.3389/fpls.2020.01133
Liu, W., Chen, G., Chen, J., Jahan, M. S., Guo, S., Wang, Y., et al. (2021). Overexpression of 7-hydroxymethyl chlorophyll a reductase from cucumber in tobacco accelerates dark-induced chlorophyll degradation. Plants 10, 1820. doi: 10.3390/plants10091820
Lu, K., Li, C., Guan, J., Liang, W. H., Chen, T., Zhao, Q. Y., et al. (2022). The PPR-domain protein SOAR1 regulates salt tolerance in rice. Rice 15, 62. doi: 10.1186/s12284-022-00608-x
Luo, P. G., Deng, K. J., Hu, X. Y., Li, L. Q., Li, X., Chen, J. B., et al. (2013). Chloroplast ultrastructure regeneration with protection of photosystem II is responsible for the functional ‘stay-green’trait in wheat. Plant Cell Environ. 36, 683–696. doi: 10.1111/pce.2013.36.issue-3
Luo, Z., Xiong, J., Xia, H., Wang, L., Hou, G., Li, Z., et al. (2022). Pentatricopeptide repeat gene-mediated mitochondrial RNA editing impacts on rice drought tolerance. Front. Plant Sci. 13. doi: 10.3389/fpls.2022.926285
MaChado, S., Paulsen, G. M. (2001). Combined effects of drought and high temperature on water relations of wheat and sorghum. Plant Soil 233, 179–187. doi: 10.1023/A:1010346601643
Mafakheri, A., Siosemardeh, A. F., Bahramnejad, B., Struik, P. C., Sohrabi, Y. (2010). Effect of drought stress on yield, proline and chlorophyll contents in three chickpea cultivars. Aust. J. Crop Sci. 4, 580–585. doi: 10.3316/informit.857341254680658
Mair, A., Pedrotti, L., Wurzinger, B., Anrather, D., Simeunovic, A., Weiste, C., et al. (2015). SnRK1-triggered switch of bZIP63 dimerization mediates the low-energy response in plants. elife 4, e05828. doi: 10.7554/eLife.05828.043
Meena, S., Arora, A., Taria, S., Yadav, S., Kumar, S., Pushkar, S., et al. (2023). Unraveling the physiological and molecular mechanisms regulating grain yield under combined drought and heat stress in wheat (Triticum aestivum). Indian J. Agric. Sci. 93, 821–826. doi: 10.56093/ijas.v93i8.138471
Mekonnen, D. W., Flügge, U. I., Ludewig, F. (2016). Gamma-aminobutyric acid depletion affects stomata closure and drought tolerance of Arabidopsis thaliana. Plant Sci. 245, 25–34. doi: 10.1016/j.plantsci.2016.01.005
Nagar, S., Singh, V. P., Arora, A., Dhakar, R., Ramakrishnan, S. (2015). Assessment of terminal heat tolerance ability of wheat genotypes based on physiological traits using multivariate analysis. Acta Physiol. Plant. 37, 1–9. doi: 10.1007/s11738-015-2017-2
Nagar, S., Singh, V. P., Arora, A., Dhakar, R., Singh, N., Singh, G. P., et al. (2021). Understanding the role of gibberellic acid and paclobutrazol in terminal heat stress tolerance in wheat. Front. Plant Sci. 12, 692252. doi: 10.3389/fpls.2021.692252
Nayyar, H., Kaur, R., Kaur, S., Singh, R. (2014). [amp]]gamma;-Aminobutyric acid (GABA) imparts partial protection from heat stress injury to rice seedlings by improving leaf turgor and upregulating osmoprotectants and antioxidants. J. Plant Growth Regul. 33, 408–419. doi: 10.1007/s00344-013-9389-6
Ntawuguranayo, S., Zilberberg, M., Nashef, K., Bonfil, D. J., Bainsla, N. K., Piñera-Chavez, F. J., et al. (2024). Stem traits promote wheat climate-resilience. Front. Plant Sci. 15. doi: 10.3389/fpls.2024.1388881
Ogren, W. L., Bowes, G. (1971). Ribulose diphosphate carboxylase regulates soybean photorespiration. Nat. New Biol. 230, 159–160. doi: 10.1038/newbio230159a0
Olivoto, T., Nardino, M. (2021). MGIDI: Toward an effective multivariate selection in biological experiments. Bioinformatics 37, 1383–1389. doi: 10.1093/bioinformatics/btaa981
Peeva, V., Cornic, G. (2009). Leaf photosynthesis of Haberlea rhodopensis before and during drought. Environ. Exp. Bot. 65, 310–318. doi: 10.1016/j.envexpbot.2008.09.009
Perrot, T., Pauly, M., Ramírez, V. (2022). Emerging roles of β-glucanases in plant development and adaptative responses. Plants 11, 1119. doi: 10.3390/plants11091119
Pinto, R. S., Lopes, M. S., Collins, N. C., Reynolds, M. P. (2016). Modelling and genetic dissection of staygreen under heat stress. Theor. Appl. Genet. 129, 2055–2074. doi: 10.1007/s00122-016-2757-4
Prasad, P. V., Pisipati, S. R., Mutava, R. N., Tuinstra, M. R. (2008). Sensitivity of grain sorghum to high temperature stress during reproductive development. Crop Sci. 48, 1911–1917. doi: 10.2135/cropsci2008.01.0036
Raja, V., Qadir, S. U., AlYemeni, M. N., Ahmad, P. (2020). Impact of drought and heat stress individually and in combination on physio-biochemical parameters, antioxidant responses, and gene expression in Solanum lycopersicum. 3 Biotech. 10, 1–18. doi: 10.1007/s13205-020-02206-4
Rampino, P., Spano, G., Pataleo, S., Mita, G., Napier, J. A., Di Fonzo, N., et al. (2006). Molecular analysis of a durum wheat ‘stay green’mutant: expression pattern of photosynthesis-related genes. J. Cereal Sci. 43, 160–168. doi: 10.1016/j.jcs.2005.07.004
Rebetzke, G. J., Van Herwaarden, A. F., Jenkins, C., Weiss, M., Lewis, D., Ruuska, S., et al. (2008). Quantitative trait loci for water-soluble carbohydrates and associations with agronomic traits in wheat. Aust. J. Agric. Res. 59, 891–905. doi: 10.1071/AR08067
Redhu, M., Singh, V., Kumari, A., Munjal, R., Yashveer, S., Nimbal, S., et al. (2024). Unlocking genetic insights: Evaluating wheat RILs for physiobiochemical traits under terminal heat stress conditions. BMC Plant Biol. 24, 429. doi: 10.1186/s12870-024-05062-z
Reynolds, M. P., Gutiérrez-Rodríguez, M., Larqué-Saavedra, A. (2000). Photosynthesis of wheat in a warm, irrigated environment: I: genetic diversity and crop productivity. Field Crops Res. 66, 37–50. doi: 10.1016/S0378-4290(99)00077-5
Reynolds, M. P., Nagarajan, S., Razzaque, M. A., Ageeb, O. A. A. (1997). Using canopy temperature depression to select for yield potential of wheat in heat-stressed environments. Wheat Program Special Report (WPSR), WPSR No. 42., Mexico, DF. CIMMYT, 1–46.
Ristic, Z., Bukovnik, U., Prasad, P. V. (2007). Correlation between heat stability of thylakoid membranes and loss of chlorophyll in winter wheat under heat stress. Crop Sci. 47, 2067–2073. doi: 10.2135/cropsci2006.10.0674
Sato, H., Mizoi, J., Shinozaki, K., Yamaguchi-Shinozaki, K. (2024). Complex plant responses to drought and heat stress under climate change. Plant J. 117, 1873–1892. doi: 10.1111/tpj.v117.6
Sato, Y., Morita, R., Katsuma, S., Nishimura, M., Tanaka, A., Kusaba, M. (2009). Two short-chain dehydrogenase/reductases, NON-YELLOW COLORING 1 and NYC1-LIKE, are required for chlorophyll b and light-harvesting complex II degradation during senescence in rice. Plant J. 57, 120–131. doi: 10.1111/j.1365-313X.2008.03670.x
Schmittgen, T. D., Livak, K. J. (2008). Analyzing real-time PCR data by the comparative CT method. Nat. Protoc. 3, 1101–1108. doi: 10.1038/nprot.2008.73
Shafi, A., Pal, A. K., Sharma, V., Kalia, S., Kumar, S., Ahuja, P. S., et al. (2017). Transgenic potato plants overexpressing SOD and APX exhibit enhanced lignification and starch biosynthesis with improved salt stress tolerance. Plant Mol. Biol. Rep. 35, 504–518. doi: 10.1007/s11105-017-1041-3
Shah, N. H., Paulsen, G. M. (2003). Interaction of drought and high temperature on photosynthesis and grain-filling of wheat. Plant Soil 257, 219–226. doi: 10.1023/A:1026237816578
Sharifi, P., Mohammadkhani, N. (2016). Effects of drought stress on photosynthesis factors in wheat genotypes during anthesis. Cereal Res. Commun. 44, 229–239. doi: 10.1556/0806.43.2015.054
Sharma, D. K., Andersen, S. B., Ottosen, C. O., Rosenqvist, E. (2015). Wheat cultivars selected for high Fv/Fm under heat stress maintain high photosynthesis, total chlorophyll, stomatal conductance, transpiration and dry matter. Physiol. Plant. 153, 284–298. doi: 10.1111/ppl.2015.153.issue-2
Sikder, S., Qiao, Y., Baodi, D., Shi, C., Liu, M. (2016). Effect of water stress on leaf level gas exchange capacity and water-use efficiency of wheat cultivars. Indian J. Plant Physiol. 21, 300–305. doi: 10.1007/s40502-016-0238-z
Simova-Stoilova, L., Pecheva, D., Kirova, E. (2020). Drought stress response in winter wheat varieties–changes in leaf proteins and proteolytic activities. Acta Botanica Croatica 79, 121–130. doi: 10.37427/botcro-2020-018
Singh, S. K., Reddy, K. R. (2011). Regulation of photosynthesis, fluorescence, stomatal conductance and water-use efficiency of cowpea (Vigna unguiculata [L.] Walp.) under drought. J. Photochem. Photobiol. B: Biol. 105, 40–50. doi: 10.1016/j.jphotobiol.2011.07.001
Standfuss, J., Terwisscha van Scheltinga, A. C., Lamborghini, M., Kühlbrandt, W. (2005). Mechanisms of photoprotection and nonphotochemical quenching in pea light-harvesting complex at 2.5 Å resolution. EMBO J. 24, 919–928. doi: 10.1038/sj.emboj.7600585
Tan, B. C., Schwartz, S. H., Zeevaart, J. A., McCarty, D. R. (1997). Genetic control of abscisic acid biosynthesis in maize. Proc. Natl. Acad. Sci. 94, 12235–12240. doi: 10.1073/pnas.94.22.12235
Taria, S., Arora, A., Krishna, H., Manjunath, K. K., Meena, S., Kumar, S., et al. (2023). Multivariate analysis and genetic dissection of staygreen and stem reserve mobilisation under combined drought and heat stress in wheat (Triticum aestivum L.). Front. Genet. 14. doi: 10.3389/fgene.2023.1242048
Valluru, R. (2015). Fructan and hormone connections. Front. Plant Sci. 6. doi: 10.3389/fpls.2015.00180
Van den Boogaard, R., Alewijnse, D., Veneklaas, E. J., Lambers, H. (1997). Growth and water-use efficiency of 10 Triticum aestivum cultivars at different water availability in relation to allocation of biomass. Plant Cell Environ. 20, 200–210. doi: 10.1046/j.1365-3040.1997.d01-60.x
Vignjevic, M., Wang, X., Olesen, J. E., Wollenweber, B. (2015). Traits in spring wheat cultivars associated with yield loss caused by a heat stress episode after anthesis. J. Agron. Crop Sci. 201, 32–48. doi: 10.1111/jac.2014.201.issue-1
Vijayakumari, K., Puthur, J. T. (2016). [amp]]gamma;-Aminobutyric acid (GABA) priming enhances the osmotic stress tolerance in Piper nigrum Linn. plants subjected to PEG-induced stress. Plant Growth Regul. 78, 57–67. doi: 10.1007/s10725-015-0074-6
Weisz, D. A., Liu, H., Zhang, H., Thangapandian, S., Tajkhorshid, E., Gross, M. L., et al. (2017). Mass spectrometry-based cross-linking study shows that the Psb28 protein binds to cytochrome b 559 in Photosystem II. Proc. Natl. Acad. Sci. 114, 2224–2229. doi: 10.1073/pnas.1620360114
Wellburn, A. R. (1994). The spectral determination of chlorophylls a and b, as well as total carotenoids, using various solvents with spectrophotometers of different resolution. J. Plant Physiol. 144, 307–313. doi: 10.1016/S0176-1617(11)81192-2
Will, R. E., Wilson, S. M., Zou, C. B., Hennessey, T. C. (2013). Increased vapor pressure deficit due to higher temperature leads to greater transpiration and faster mortality during drought for tree seedlings common to the forest–grassland ecotone. New Phytol. 200, 366–374. doi: 10.1111/nph.2013.200.issue-2
Wu, W., Du, K., Kang, X., Wei, H. (2021). The diverse roles of cytokinins in regulating leaf development. Horticult. Res. 8, 118. doi: 10.1038/s41438-021-00558-3
Xiong, L., Zhu, J. K. (2003). Regulation of abscisic acid biosynthesis. Plant Physiol. 133, 29–36. doi: 10.1104/pp.103.025395
Xu, J., Yang, J., Duan, X., Jiang, Y., Zhang, P. (2014). Increased expression of native cytosolic Cu/Zn superoxide dismutase and ascorbate peroxidase improves tolerance to oxidative and chilling stresses in cassava (Manihot esculenta Crantz). BMC Plant Biol. 14, 1–14. doi: 10.1186/s12870-014-0208-4
Xu, Y., Zhang, W., Ju, C., Li, Y., Yang, J., Zhang, J. (2016). Involvement of abscisic acid in fructan hydrolysis and starch biosynthesis in wheat under soil drying. Plant Growth Regul. 80, 265–279. doi: 10.1007/s10725-016-0164-0
Xu, Z., Zhou, G., Shimizu, H. (2010). Plant responses to drought and rewatering. Plant Signaling Behav. 5, 649–654. doi: 10.4161/psb.5.6.11398
Yan, H., Li, Q., Park, S. C., Wang, X., Liu, Y. J., Zhang, Y. G., et al. (2016). Overexpression of CuZnSOD and APX enhance salt stress tolerance in sweet potato. Plant Physiol. Biochem. 109, 20–27. doi: 10.1016/j.plaphy.2016.09.003
Yan, J., Zhu, C., Liu, W., Luo, F., Mi, J., Ren, Y., et al. (2015). High photosynthetic rate and water use efficiency of Miscanthus lutarioriparius characterize an energy crop in the semiarid temperate region. Gcb Bioenergy 7, 207–218. doi: 10.1111/gcbb.2015.7.issue-2
Yousaf, M. I., Riaz, M. W., Jiang, Y., Yasir, M., Aslam, M. Z., Hussain, S., et al. (2022). Concurrent effects of drought and heat stresses on physio-chemical attributes, antioxidant status and kernel quality traits in maize (Zea mays L.) hybrids. Front. Plant Sci. 13. doi: 10.3389/fpls.2022.898823
Zaefyzadeh, M., Quliyev, R. A., Babayeva, S. M., Abbasov, M. A. (2009). The effect of the interaction between genotypes and drought stress on the superoxide dismutase and chlorophyll content in durum wheat landraces. Turkish J. Biol. 33, 1–7. doi: 10.3906/biy-0801-12
Zandalinas, S. I., Rivero, R. M., Martínez, V., Gómez-Cadenas, A., Arbona, V. (2016). Tolerance of citrus plants to the combination of high temperatures and drought is associated to the increase in transpiration modulated by a reduction in abscisic acid levels. BMC Plant Biol. 16, 1–16. doi: 10.1186/s12870-016-0791-7
Zarea, M. J., Karimi, N. (2023). Grain yield and quality of wheat are improved through post-flowering foliar application of zinc and 6-benzylaminopurine under water deficit condition. Front. Plant Sci. 13, 1068649. doi: 10.3389/fpls.2022.1068649
Zhang, J., Fengler, K. A., Van Hemert, J. L., Gupta, R., Mongar, N., Sun, J., et al. (2019). Identification and characterization of a novel stay-green QTL that increases yield in maize. Plant Biotechnol. J. 17, 2272–2285. doi: 10.1111/pbi.v17.12
Zhang, P., Liu, Y., Li, M., Ma, J., Wang, C., Su, J., et al. (2020). Abscisic acid associated with key enzymes and genes involving in dynamic flux of water-soluble carbohydrates in wheat peduncle under terminal drought stress. Plant Physiol. Biochem. 151, 719–728. doi: 10.1016/j.plaphy.2020.04.018
Zhang, X., Liu, W., Lv, Y., Li, T., Tang, J., Yang, X., et al. (2022). Effects of drought stress during critical periods on the photosynthetic characteristics and production performance of Naked oat (Avena nuda L.). Sci. Rep. 12, 11199. doi: 10.1038/s41598-022-15322-3
Keywords: combined stress, QTLs, stay-green, stem reserve mobilization, wheat, yield stability
Citation: Taria S, Arora A, Kumar S, Krishna H, Meena S, Singh B, China Malakondaiah A, S. K, Padaria JC, Singh PK, Alam B, Kumar S and Arunachalam A (2025) Validation of stay-green and stem reserve mobilization QTLs: physiological and gene expression approach. Front. Plant Sci. 16:1541944. doi: 10.3389/fpls.2025.1541944
Received: 09 December 2024; Accepted: 20 January 2025;
Published: 17 February 2025.
Edited by:
Sunita Munda, Assam Agricultural University, IndiaReviewed by:
Chenchen Zhao, University of Tasmania, AustraliaCopyright © 2025 Taria, Arora, Kumar, Krishna, Meena, Singh, China Malakondaiah, S., Padaria, Singh, Alam, Kumar and Arunachalam. This is an open-access article distributed under the terms of the Creative Commons Attribution License (CC BY). The use, distribution or reproduction in other forums is permitted, provided the original author(s) and the copyright owner(s) are credited and that the original publication in this journal is cited, in accordance with accepted academic practice. No use, distribution or reproduction is permitted which does not comply with these terms.
*Correspondence: Ajay Arora, cm9taWFyb3JhQHlhaG9vLmNvbQ==; Hari Krishna, aGFyaWtyaXNobmEuYWdyaUBnbWFpbC5jb20=
Disclaimer: All claims expressed in this article are solely those of the authors and do not necessarily represent those of their affiliated organizations, or those of the publisher, the editors and the reviewers. Any product that may be evaluated in this article or claim that may be made by its manufacturer is not guaranteed or endorsed by the publisher.
Research integrity at Frontiers
Learn more about the work of our research integrity team to safeguard the quality of each article we publish.