- College of Biological and Food Engineering, Qujing Normal University, Qujing, Yunnan, China
Plants live under various biotic and abiotic stress conditions, and to cope with the adversity and severity of these conditions, they have developed well-established resistance mechanisms. These mechanisms begin with the perception of stimuli, followed by molecular, biochemical, and physiological adaptive measures. Tomato (Solanum lycopersicum) is a globally significant vegetable crop that experiences several biotic and abiotic stress events that can adversely impact its quality and production. Mitogen-activated protein kinases (MAPKs) in tomato plants have crucial functions of mediating responses to environmental cues, internal signals, defense mechanisms, cellular processes, and plant development and growth. MAPK cascades respond to various environmental stress factors by modulating associated gene expression, influencing plant hormone synthesis, and facilitating interactions with other environmental stressors. Here, we review the evolutionary relationships of 16 tomato SlMAPK family members and emphasize on recent studies describing the regulatory functions of tomato SlMAPKs in both abiotic and biotic stress conditions. This review could enhance our comprehension of the MAPK regulatory network in biotic and abiotic stress conditions and provide theoretical support for breeding tomatoes with agronomic traits of excellent stress resistance.
1 Introduction
Tomato (Solanum lycopersicum) is a crucial vegetable crop globally. Tomato cultivation is a major industry, and global production was estimated at 182 million tons in 2018, rising to 186 million tons in 2020 (Collins et al., 2022). Nonetheless, several abiotic and biotic factors affect tomato cultivation. Drought, salinity, extreme temperatures, and nutrient deficiencies are abiotic stress factors, while biotic stress originates from insects, fungi, bacteria, nematodes, and viruses (Kissoudis et al., 2015; Collins et al., 2022). Global climate change has led to harsh field environments, resulting in consistently increasing agricultural losses and diminishing production of gains. Numerous climate models have predicted high frequency of extreme temperatures, floods, and droughts in the future (Boyer et al., 2013; Chen et al., 2021). Various stimulus response mechanisms and activation strategies have emerged in plants to meet these challenges. Plant receptors are a key element in plant-environment interaction, as they transmit information and enable the plant to recognize its surroundings (Osakabe et al., 2013; Dievart et al., 2020). Mitogen-activated protein kinase (MAPK) cascades are critical elements of all eukaryotic signaling networks. The MAPKs function downstream of sensors and receptors and facilitate cellular responses, thereby achieving integrated plant immunity, plant development and growth, and adaptation to an ever-changing environment (Zhang and Zhang, 2022). The MAPK cascade pathway includes at least one MAPK, mitogen-activated protein kinase kinases (MAP2Ks, MKKs, and MAPKKs), and mitogen-activated protein kinase kinase kinases (MKKKs, MAPKKKs, MAP3Ks, and MEKKs) (Tena et al., 2001). The abovementioned three kinase tiers in a cell comprise numerous members that function to ensure that the transmitted signal has the required specificity (Zhang and Klessig, 2001). Plant MAPK cascades are crucial in governing plant growth and facilitating plants’ response to an array of stress stimuli, such as pathogen invasion, injury, temperature fluctuations, salinity, ultraviolet (UV) exposure, osmotic changes, reactive oxygen species (ROS), drought, and ozone (Meng and Zhang, 2013; Lin et al., 2021; Majeed et al., 2023). Here, we present a review of recent advances in research on MAPK cascades implicated in signaling networks in tomato for coping with abiotic and biotic stress conditions.
2 Overview of MAPKs
Plant MAPKs, as a crucial component of intricate signaling networks, play vital roles in enabling plants to recognize and interact with environmental cues and internal signals; they also mediate defense mechanisms, regulate growth and development, and fine-tune various cellular processes (Colcombet and Hirt, 2008; Lin et al., 2021). MAPKs are activated by the dual phosphorylation of Thr and Tyr residues in a TXY motif located in the activation loop between subdomains VII and VIII by their upstream MAPK kinases. Subsequently, MAPKs phosphorylate their substrates, which are mainly transcription factors. These transcription factors then trigger downstream reactions (Hazzalin and Mahadevan, 2002; Wu et al., 2007).
2.1 MAPK cascades and their influence on signal transduction
The MAPK signaling pathway functions as a universally preserved mechanism in eukaryotic organisms for transmitting signals from the cell exterior (Huang et al., 2022). The MAPK cascade participates in plants’ response to manage abiotic stress (de Zelicourt et al., 2016). MAPKs are structurally highly conserved serine/threonine protein kinases; they phosphorylate diverse substrates, for example, cytoskeleton-related proteins, protein kinases, and transcription factors; moreover, they have key functions in controlling how plants respond to stress, such as drought, heavy metals, extreme temperatures, and salinity (Moustafa et al., 2014; Gao et al., 2019). MAPK, MAPKK, and MAPKKK are the three distinct sets of protein kinases in the MAPK cascade. These kinases activate one another sequentially through phosphorylation (Danquah et al., 2014). Typically, MAP3Ks are activated through extracellular signals. The activated MAP3Ks then induce phosphorylation and activation of the S/T-X3-5-S/T motif present in downstream MAPKKs. Next, the activated MAPKKs stimulate the phosphorylation and activation of MAPKs at the TXY activation loop, thereby facilitating signal transmission to the nucleus (Figure 1) (Tena et al., 2001; Meng and Zhang, 2013; Jiang et al., 2022). MAPKKKs, may serve as adaptors to establish a link between upstream signaling events and the main MAPK cascades.
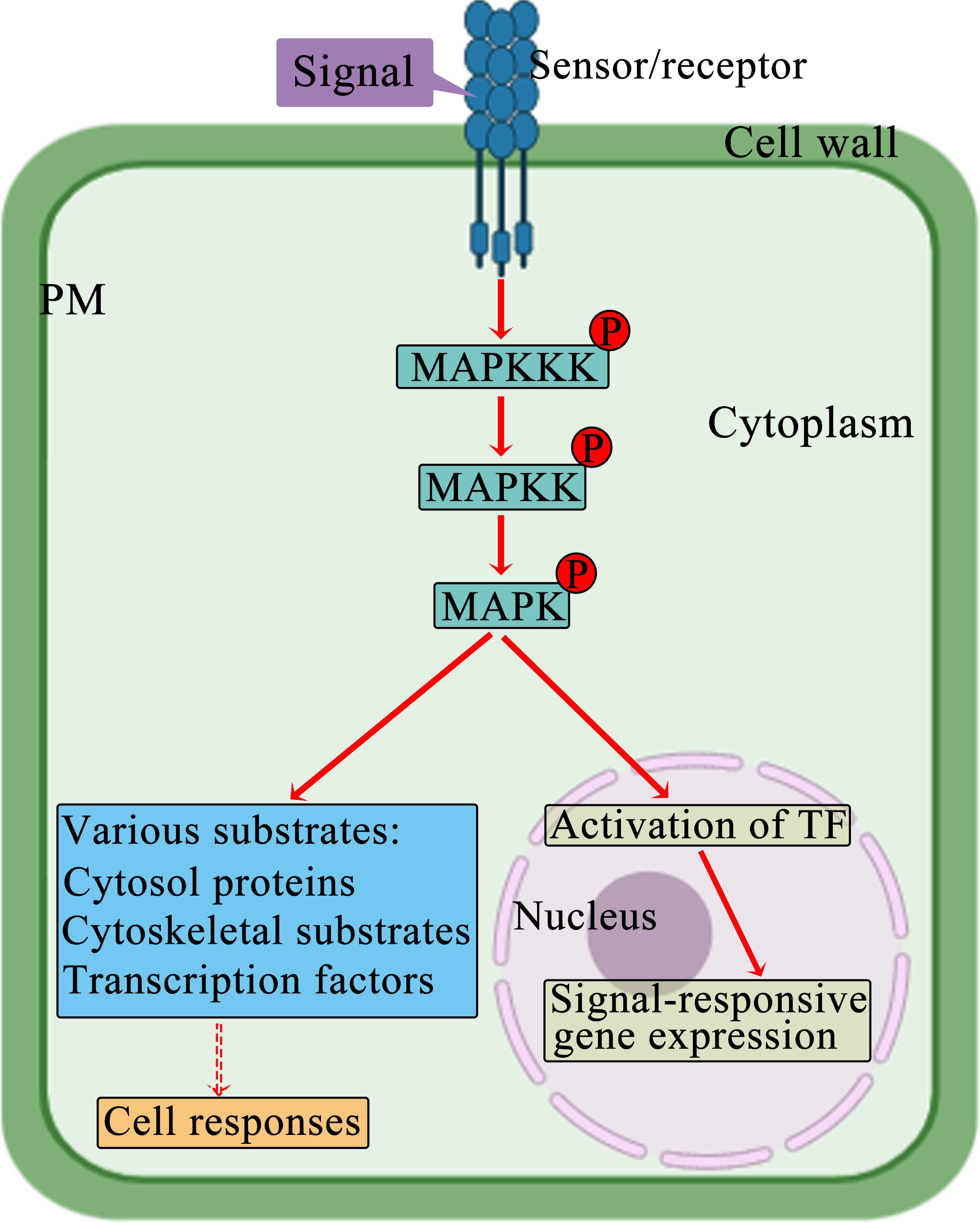
Figure 1. Schematic representation of the MAPK cascade. A signal transduction cascade navigates the signal from MAPKKK to MAPK by triggering a series of Thr/Tyrosine (Tyr) and Ser/Thr phosphorylation events. Eventually, the activated MAPKs are transported to the nucleus, where they phosphorylate transcription factors, altering their binding affinity to the promoter regions of target genes and thereby suppressing or promoting gene expression. The MAPK pathways function as kinases and convert external signals from the environment for modifying specific target proteins, for example, transcription factors, through post-translational processes. This induces reconfiguration of gene expression patterns and an adjustment in the stress response mechanism of the plant.
2.2 Phylogeny and classification of MAPKs
The complete Arabidopsis thaliana genome sequence revealed 20 genes potentially encoding MAPKs. In tomato, 16 SlMAPKs have been identified. These MAPKs are divided into four groups (Figure 2) (MAPK Group, 2002; Kong et al., 2012). SlMAPK1-3, SlMAPK4-7, SlMAPK8 and 9, and SlMAPK10-16 were included in Groups A, B, C, and D, respectively. Conserved domain analysis shows that members of the A, B, and C subfamilies have a Thr-Glu-Tyr (TEY) phosphorylation motif in their active sites, while members of the D subfamily have a Thr-Asp-Tyr (TDY) motif in their active sites (Kong et al., 2012). MAPKs of Group A, specifically Arabidopsis thaliana MAPK3 and MAPK6, were initially associated with plant defense mechanisms and responses to abiotic stresses. Subsequently, their significant contributions to plant development and growth were also demonstrated (Wang et al., 2023; Wu et al., 2024; Wu and Wang, 2024).
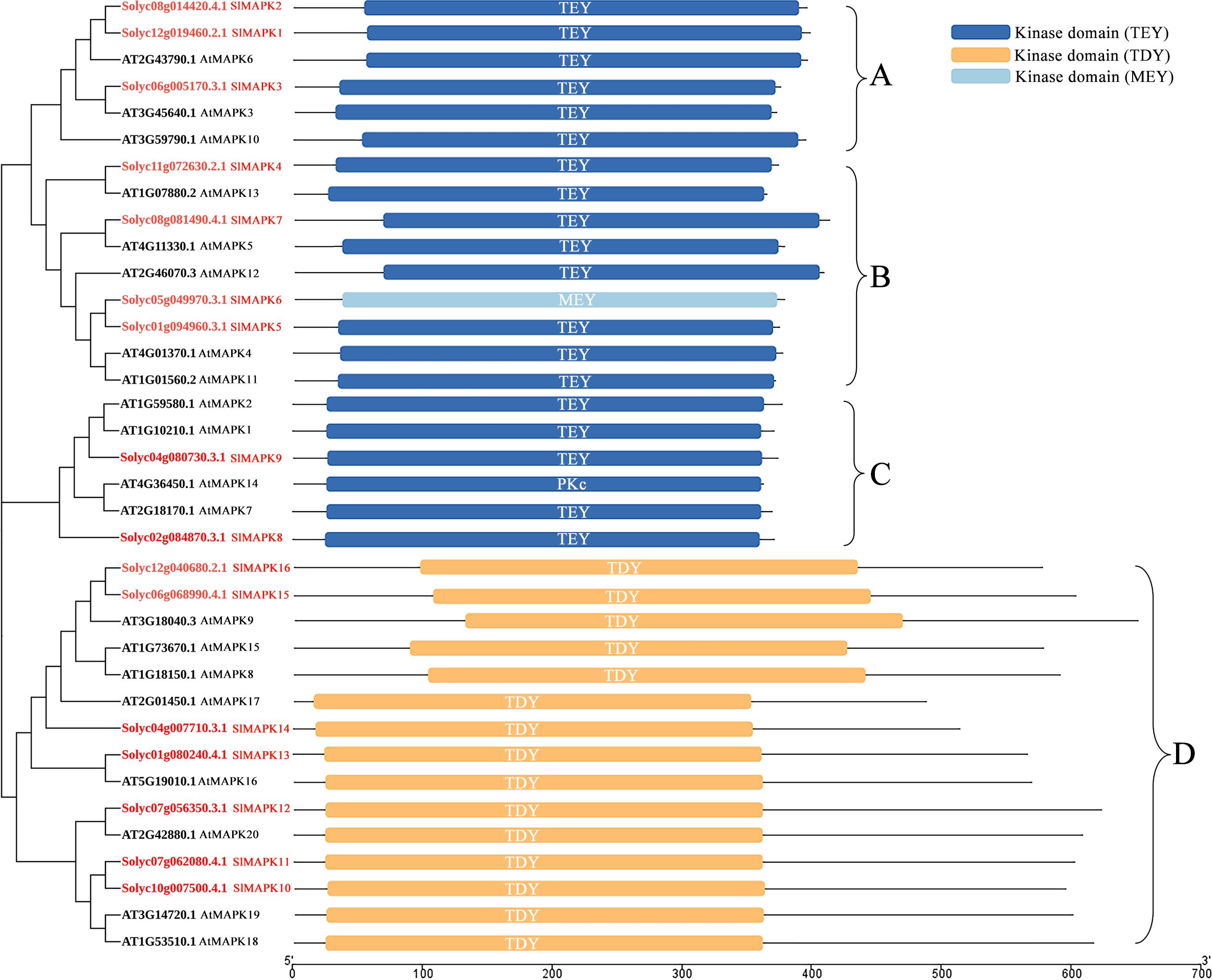
Figure 2. The phylogenetic tree and domain structure of plant MAPKs. On the left, the phylogenetic relationships of MAPKs from tomato (Solanum lycopersicum, Sl) and Arabidopsis thaliana (At). On the right, the domain structure of the MAPKs. TEY, ZThr-Glu-Tyr; TDY, Thr-Asp-Tyr; MEY, Met-Glu-Tyr.
Group B MAPKs, such as Arabidopsis thaliana MAPK4, MAPK5, MAPK11, MAPK12, and MAPK13, play roles in plant immune responses, adaptation to environmental conditions, and plant development and growth (de Zelicourt et al., 2016; Thulasi Devendrakumar et al., 2018). Furthermore, Group C MAPKs, including Arabidopsis thaliana MAPK1, MAPK2, MAPK7, and MAPK14, along with MAP3K17/18, constitute the MAP3K17/18-MKK3-MAPK1/2/7/14 cascade; these MAPKs have important functions in abscisic acid (ABA) signaling, senescence, and tolerance to drought (Matsuoka et al., 2015; Li et al., 2017; Tajdel-Zielińska et al., 2024). Groups A-C included the TEY subtype MAPKs, while Group D MAPK is a TDY subtype with eight members in Arabidopsis thaliana: MAPK8, MAPK9, MAPK15, MAPK16, MAPK17, MAPK18, MAPK19, and MAPK20. Compared to the members of Group A-C, Group D members possess a longer C-terminal region and show absence of the common MAPKK-binding docking motif (Dóczi et al., 2012; Zhang and Zhang, 2022). MAPK9, a Group D member, is activated independent of upstream MAPKKs; it is activated by autophosphorylation. Furthermore, BRASSINOSTEROID-SIGNALING KINASE1 modulates the phosphorylation of MAPK15 to induce resistance in Arabidopsis thaliana to powdery mildew (Nagy et al., 2015; Shi et al., 2022). Thus, MAPKs are pivotal in enabling overall development and growth of plants and in interacting with biotic and abiotic stresses.
3 Functions of MAPKs in abiotic stresses
Plants can detect unfavorable environmental changes, including elevated salinity, high temperatures, and drought. They translate these stress indicators into cellular responses, which enable them to modify their development, metabolism, and growth effectively to ensure survival and reproduction (Fujita et al., 2006; Zhu, 2016; Chen et al., 2021). Numerous signaling reactions and events at the physiological, biochemical, and molecular levels are reported in plants experiencing abiotic stresses (Zhang et al., 2022a). Moreover, the MAPK cascade pathway has an essential function in adaptation to various stress events in different plants and at varying development and growth stages (Danquah et al., 2014). MAPK activation serves as a secondary response to physiological alterations that plant cells undergo during stress. Additionally, the absence of sensor mutants complicates the direct association of MAPK activation with specific abiotic stresses (Zhang and Zhang, 2022; Wei et al., 2022). Several plant hormones, such as ABA, jasmonic acid (JA), and ethylene (ET), have crucial functions in the response of plants to abiotic stress (Halim et al., 2006; Lee and Luan, 2012; Gontia-Mishra et al., 2014). Additionally, MAPKs are implicated in the signaling and biosynthesis pathways of these hormones (Figure 3).
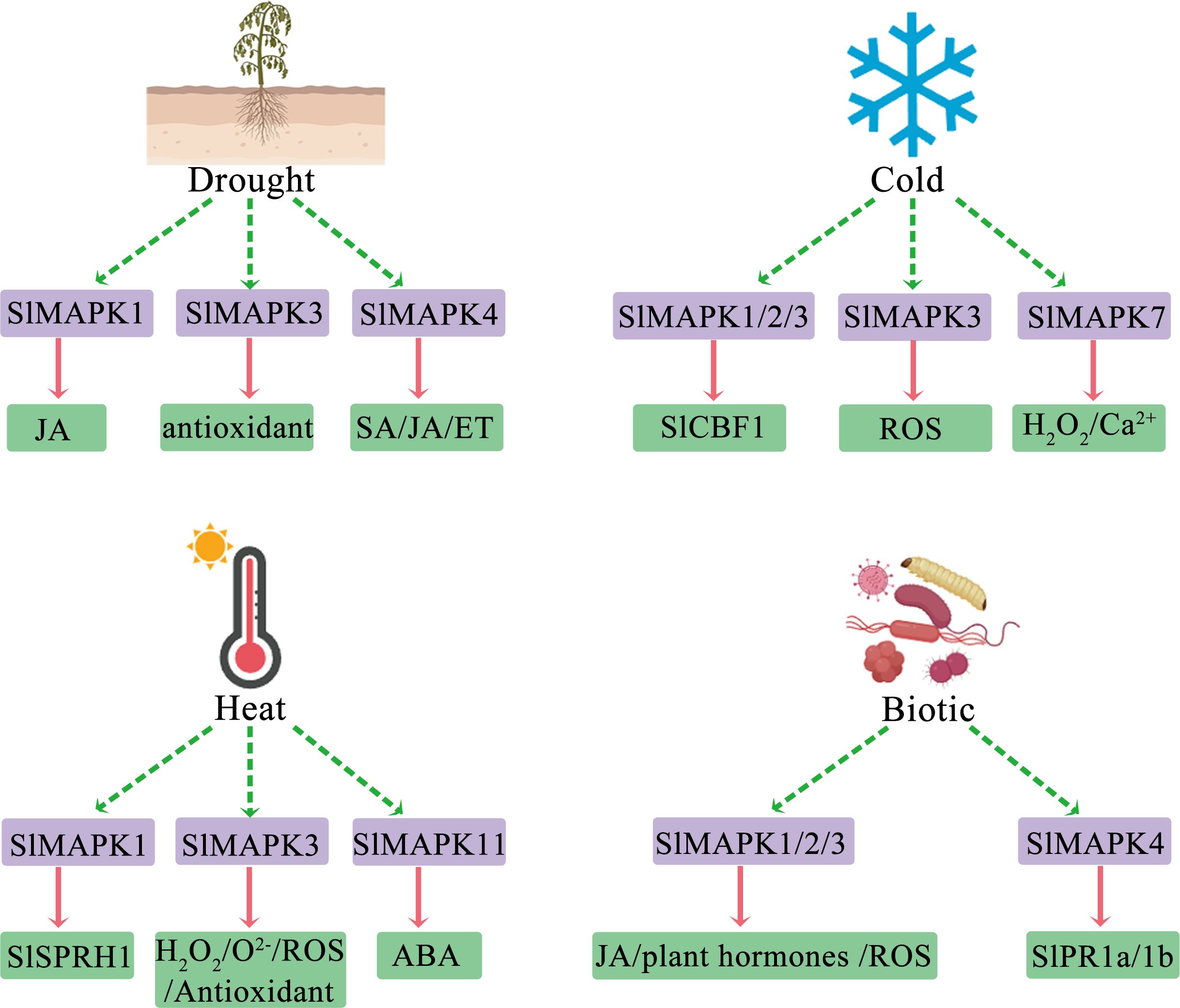
Figure 3. Overview of roles of various SlMAPKs in stress responses and their regulation and potential downstream effects. ET, ethylene; JA, jasmonic acid; SA, salicylic acid; SlCBF1, C-repeat binding factor 1; ROS, reactive oxygen; H2O2, hydrogen peroxide; SlSPRH1, serine-proline-rich protein homology; ABA, abscisic acid; SlPR1a/b; Pathogenesis-Related 1a/b.
3.1 MAPKs regulate response in tomato plants to drought stress
Drought, one of the major abiotic stress, severely impacts crop production. It not only has drastic effects on plant productivity and metabolism but also leads to widespread socioeconomic burden and damage (Lesk et al., 2016). Several biochemical and transcriptional studies have shown that MAPKs are involved in tomato plant’s response to drought. SlMAPK1 has a pivotal function in how plants respond to abiotic stressors such as drought. As reported previously, SlMAPK1 contributes to JA biosynthesis, a hormone essential for plant defense and stress response (Alfagham et al., 2024). During drought, SlMAPK1 activation increases JA levels, thereby improving the plant’s capacity to withstand dehydration and recuperate from water deficiency. JA is crucial for stomatal closure, which reduces transpiration-mediated loss of water, as well as for root growth that enhances water absorption from soil (Alfagham et al., 2024; Wang et al., 2018). Furthermore, SlMAPK1 interacts with various proteins and transcription factors within the MAPK pathway, thereby activating genes that facilitate plant acclimation to drought stress. Previous research has also indicated that the metabolite albaflavenone, produced by microbial endophytes, improves drought resistance in tomato plants by activating the SlMAPK1 protein in the MAPK signaling pathway (Alfagham et al., 2024). SlMAPK3 has a remarkable function in responding to abiotic stress events, for example, stress due to heavy metals (cadmium) and drought (Muhammad et al., 2019). SlMAPK3 gene knockdown by CRISPR/Cas9 gene editing technology increased tomato plants’ sensitivity to drought stress, led to greater membrane damage, and reduced antioxidant enzyme activity (Wang et al., 2017a). In contrast, transgenic plants overexpressing SlMAPK3 showed enhanced antioxidant enzyme activity and improved tolerance to stress due to drought and cadmium exposure. These findings revealed the function of SlMAPK3 in regulating antioxidant responses and protecting cellular membrane in tomato plants, thus suggesting potential molecular mechanisms for developing tomato varieties with elevated abiotic stress tolerance (Wang et al., 2017a; Muhammad et al., 2019; Huang et al., 2023). Compared to control plants, SlMAPK4 gene silencing diminished drought stress tolerance, resulting in earlier wilting in drought (Virk et al., 2013). Additionally, silencing of SlMAPK4 in tomato plants upregulated the expression of Pathogenesis-Related 1a (SlPR1a) and SlPR1b, which are defense-related genes. Thus, in tomato, SlMAPK4 may participate in salicylic acid (SA) - and JA/ET-mediated signaling pathways (Nambeesan et al., 2012; Virk et al., 2013).
Carbon monoxide (CO), nitric oxide (NO), and hydrogen sulfide (H2S) are the three endogenously produced gaseous signaling molecules (Yamasaki and Cohen, 2016). Exogenous H2S and NO treatment enhances plant stress tolerance against harsh environments, including extreme temperatures, salinity, and drought, by enhancing antioxidant systems and cellular resistance (Tian et al., 2016; Ni et al., 2016; Kolbert, 2019). The MAPK signaling pathway facilitates the promotion of tomato seedling growth by H2S, and some MAPK family genes, particularly SlMAPK3 and SlMAPK13, exhibit remarkably altered expression pattern following exogenous addition of NaHS The MAPK inhibitor PD98059 reduces H2S levels and L-cysteine demercase (LCD) activity in tomato seedlings (Ba et al., 2021). SNP, an NO donor, at 100 μM concentration effectively promotes tomato seedling growth, enhances nitrogen metabolism, and minimizes oxidative damage in low nitrogen stress condition. The molecular response to low nitrogen concentration stress and NO treatment in tomato seedlings involves the MAPK pathway as a key factor (Zhang et al., 2022b; Lu et al., 2024). PD98059, the MAPK inhibitor, weakens NO effects, thus suggesting that, in low nitrogen concentration stress condition, the MAPK signaling pathway mediates NO-based nitrogen metabolism and abolition of oxidative damage and growth inhibition in tomato seedlings (Ba et al., 2021; Zhang et al., 2022b; Lu et al., 2024).
3.2 Response of MAPKs in tomato to temperature stress
Temperature significantly impacts plant productivity and growth. Plants can thrive within a specific temperature range; however, extreme temperatures can cause damage to plant cells. Cold stress inhibits germination, growth, and metabolism and disrupts membranes by forming ice crystals. High temperatures induce heat stress, increase respiration, and cause ROS overproduction, thereby impacting plant productivity and growth (Moustafa et al., 2014; Wu et al., 2022; Manasa et al., 2022; Kan et al., 2023). MAPKs are critically involved in temperature signal perception and transduction (Sangwan and Dhindsa, 2002). MAPKs and C-repeat binding factors (CBF) have essential functions in monitoring cold response. Exogenous hydrogen peroxide treatment improves tomato plants tolerance to cold stress by stimulating SlCBF1 and SlMAPK1/2/3 expression and modulating antioxidant enzyme activity and phytohormone levels (Wang et al., 2017b). SlMAPK3 knockout resulted in reduced content of ferulic acid (FA) and inhibition of FA synthesis-associated gene expression (SlC3H, SlC4H, SlCOMT, and SlPAL5) (Kumar and Pruthi, 2014; Song et al., 2023). Specifically, FA showed reduced effects on osmotic regulatory substances and antioxidant enzymes together with a decline in CBF pathway-associated gene expression. These results revealed that FA positively contributes to the resistance of tomato fruits to chilling stress through MAPK3-dependent upregulation of genes linked with the CBF transcriptional pathway (Miura et al., 2012; Shu et al., 2022). Remarkably, SlMAPK1 and SlMAPK2 are crucial for mediating B-box (SlBBX17) proteins phosphorylation. This phosphorylation event enhances the formation of a complex between SlHY5 and SlBBX17, which then modulates the activity of SlCBFs, ultimately conferring low-temperature tolerance to the plants (Heng et al., 2019; Song et al., 2023).
In tomato plants, the SlMAPK3 gene participates in environmental stress response, particularly to temperature stress (Yu et al., 2019). SlMAPK3 gene knockout enhances heat stress tolerance through the prevention of ROS accumulation as well as elevation of the expression levels of heat stress transcription factors (HSFs), antioxidant enzymes, and heat shock proteins (Iba, 2002; Yu et al., 2019). Conversely, SlMAPK3 overexpression in tobacco enhances low-temperature-stress tolerance by activating cellular antioxidant systems, altering stress-responsive gene transcription, and improving ROS scavenging- and stress tolerance-associated gene expression. Collectively, these findings highlight the critical regulatory function of SlMAPK3 in plants’ response to temperature stress (Yu et al., 2015a, 2019). SlMAPK7 is a Group B MAPK gene. As shown previously, SlMAPK7 responds to various stresses and signaling molecules, and its mRNA expression level is regulated by H2O2 and calcium ions (Ca2+). SlMAPK7 is localized primarily in the cell nucleus, and its overexpression enhances cold stress tolerance in transgenic tomato plants (Yu et al., 2015b). This enhancement is linked to cellular antioxidant system activation, modulation of stress-related gene expression, and more efficient scavenging of ROS. Thus, SlMAPK7 positively regulates plant cell response to cold stress by altering ROS homeostasis and affecting stress-responsive gene expression (Xing et al., 2008; Pitzschke et al., 2009; Yu et al., 2015b). Thus, MAPK signaling is a critical input for plants to recognize and act to tolerate temperature stress and to regulate cold tolerance by activating key pathways and transcription factors, which ultimately enhance growth and productivity under extreme conditions.
The occurrence of extreme weather events has increased recently, with high temperature (HT) being a notable example. Such elevated temperatures have become a primary environmental condition affecting the overall development and growth of crops (Teshome et al., 2020). In tomato plants, heat stress activates MAPKs. Heat-activated MAPK phosphorylates and promotes HSF clade A3 (HsfA3) expression (Link et al., 2002). The increased focus on MAPK-mediated responses to HT is noteworthy. SlMAPK1 silencing improves the heat tolerance of tomato plants, whereas SlMAPK1 overexpression leads to a decrease in heat tolerance in transgenic tomatoes. Additionally, following exposure to heat stress, the levels of antioxidant defense proteins in plants with SlMAPK1 interference are markedly increased, while plants with SlMAPK1 overexpression show a reduction in antioxidant defense capacity (Ding et al., 2018). SlMAPK1 interacts with the serine-proline-rich protein homology (SlSPRH1) and phosphorylates its Ser-44 site. This interaction regulates the antioxidant defense protein, which participates in response to HT, thus negatively regulating tomatoes’ tolerance to HT (Ding et al., 2018; Mo et al., 2021). CRISPR/Cas9-mediated slmapk3 mutants exhibited more tolerance to heat stress than WT plants, suggesting that SlMAPK3 was a negative regulator of thermotolerance (Yu et al., 2019). Additionally, the expression of antioxidant enzymes and heat shock proteins/heat shock factors (HSPs/HSFs) genes played a role in the heat stress response mediated by SlMAPK3 in tomato plants (Yu et al., 2019; Mo et al., 2021). SlMAPK11 is a novel gene that influences tomato seed germination, with higher expression in seeds adapted to lower germination temperatures (Song et al., 2021). Overexpression of SlMAPK11 reduces tomato seed germination and increases ABA sensitivity by upregulating 9-cis-epoxycarotenoid dioxygenase (NCED1) and affecting ABA signaling through SNF1-related kinase 2.2 (SnRK2.2) phosphorylation (Lefebvre et al., 2006; Song et al., 2021). Additionally, MAPK11 interacts with SnRK1, potentially inhibiting its activation and influencing ABA-insensitive 5 (ABI5) transcription (Song et al., 2021). Thus, MAPKs have relevant functions in tomato plants in responding to drought and temperature stress, mainly by regulating signaling pathways and antioxidant systems to enhance tomato tolerance.
4 Functions of MAPKs in biotic stresses
Stresses induced by attack of pathogens, including bacteria, fungi, viruses, and other microorganisms or macroorganisms, are referred to as biotic stress. These biotic stresses can harm crops and hinder their development and growth at various stages of their lifecycle. Disease is a leading cause of postharvest decay of tomato fruits (Chisholm et al., 2006; Luo et al., 2024). In recent years, various bacterial and fungal phytopathogens have posed a substantial threat to tomato cultivation (Noman et al., 2021). To counter attacks from pathogens, intricate signaling networks have emerged in plants for recognizing these threats and initiating a defensive response (Saijo and Loo, 2020). The MAPK gene family is crucial in responding to pathogen attack and modulates development and growth during different biotic stresses and microbial invasions (Figure 3) (Jiang et al., 2022; Majeed et al., 2023).
SlMAPK1, 2, and 3 are activated in terms of MAPK activity in cells cultured in suspension by various stimuli. These stimuli include oligosaccharide elicitors, systemin, Cf-4/Avr4-mediated hypersensitive response (HR), and ultraviolet B (UVB) radiation (Holley et al., 2003; Stulemeijer et al., 2007). Additionally, in tomatoes, MAPK1 and MAPK2 compromise prosystemin-mediated resistance to herbivory by Manduca sexta (Lepidoptera), demonstrating that they are also required for successful defense against herbivorous insects (Kandoth et al., 2007). SlMAPK2 and SlMAPK3 play a crucial role in the Pto-mediated defense response in tomatoes. SlMKK4 and SlMKK2 are the upstream MAPKKs that activate these MAPKs and induce cell death, contributing to plant defense signaling (Pedley and Martin, 2004). SlMAPK4 has pivotal functions in inducing resistance to Botrytis cinerea infection. Endogenous SlMAPK4 expression knockdown in tomato plants by virus-induced gene silencing (TRV-SlMAPK4) increased the plant’s susceptibility to B. cinerea. SlPR1a and SlPR1b, defense-related genes, showed upregulated expression in SlMAPK4-silenced plants. This increased expression might be due to the loss of SlMAPK4 function, which disrupts the SA-mediated signaling pathway (Nambeesan et al., 2012; Virk et al., 2013). SlMKK4 and SlMKK2 can activate SlMAPK1 and SlMAPK2 in vitro and enhance resistance to infections caused by B. cinerea. Additionally, SlMKK2 and SlMAPK2 show resistance to diseases caused by Xanthomonas campestris pv. vesicatoria in tomato plants (Melech-Bonfil and Sessa, 2011; Li et al., 2014). Tomato SlMAPK1, SlMAPK2, and SlMAPK3 are activated in response to fungal toxin fusicoccin, and have roles in HR and resistanceD (Higgins et al., 2007; Stulemeijer et al., 2007). SlMAPK1/2/3 inhibition disrupts the defense signaling pathways in tomato fruits and increases susceptibility to infection by B. cinerea; plant hormones and ROS are linked with defense signaling pathways related to SlMAPK1/2/3 (Zheng et al., 2015). The dual specificity of SlMAPK3 characterizes a convergence point for numerous signaling pathways that induce defense responses (Mayrose et al., 2004). Two MAPK pathways, MEK1-NTF6 and MEK2-WIPK, are involved in disease resistance mediated by Pto in tomatoes through the modulation of NPR1 expression, a crucial regulatory protein for systemic acquired resistance (Ekengren et al., 2003). SlMAPKKKϵ has a pivotal function in HR-stimulated cell death and confers tomato with resistance to gram-negative bacterial infections through the mediation of the SlMAPKKKϵ-MEK2-WIPK/SIPK signaling pathway. SlMAPKKKϵ silencing weakened the defense of tomato plants against P. syringae and X. campestris strains, leading to the onset of disease symptoms and increased bacterial proliferation (Melech-Bonfil and Sessa, 2010). Thus, MAPK cascades through several signal transduction pathways regulate disease resistance in tomato plants.
5 Conclusions and future prospects
MAPKs play a key role in tomato plants response to biotic and abiotic stresses. MAPKs regulate immune responses, overall development, and adaptation to environmental changes in tomatoes through various signaling pathways (Kong et al., 2012; Majeed et al., 2023). In particular, during abiotic stress events, for example, drought, high temperatures, and salinity, specific members of the MAPK family enhance tomato tolerance by modulating plant hormone signaling, activating antioxidant systems, and protecting cell membranes (Wang et al., 2017a). The MAPK cascade also has vital functions in plant defense mechanisms during biotic stress, including resistance to diseases such as gray mold (Meng and Zhang, 2013). Further elucidation of the pertinent functions of the MAPK cascade in the response of tomato plants to stress can clarify the underlying molecular mechanisms that could enable to develop tomato varieties with enhanced stress resistance.
At present, the functions of many protein kinases in the tomato MAPK cascade pathway are not yet fully understood, particularly those of MAPKK and MAPKK-related kinases, which should be further studied in the future. There are numerous members of the plant MAPK cascade protein kinase family, which can combine to form various levels of MAPK pathways to respond quickly to a variety of stressors (MAPK Group, 2002). It is now understood that a stimulus generally requires the participation of multiple cascades (Kumar et al., 2020). Therefore, the interrelationships between members at all levels and how these cascade pathways collaborate to maintain signal specificity in terms of functions and cross-talk still require substantial research to be fully understood. Similarly, since external stimuli are diverse and the MAPK resources of the plant itself are limited, a cascade pathway often plays a role in multiple stimulus responses (Wu and Wang, 2024). In these metabolic networks, they are usually connected in series through phosphorylation (Rodriguez et al., 2010). However, there are relatively few studies on phosphorylation and key phosphorylation sites, and further research is urgently needed.
Future research should explore the crosstalk between the MAPK cascade and other signaling molecules, such as plant hormones and transcription factors, to better understand how these interactions influence tomato adaptability to stress (Amoroso et al., 2023). Additionally, by using gene editing technologies such as CRISPR/Cas9, we can precisely control the expression levels of specific genes linked with the MAPK cascade; this could enable to confirm their functions through molecular analysis and provide candidate genes to develop tomato varieties with enhanced stress resistance. The development and application of high-throughput screening technologies for identifying and validating new MAPK cascade members and their interacting proteins should also be focused. This could facilitate better understanding of the MAPK signaling network and provide molecular tools for designing new crop improvement strategies. Finally, with the increasingly severe impact of global climatic changes on agricultural production, studies on the influence of the MAPK cascade on tomato stress responses are not only scientifically significant but also practically valuable. As the signal transduction pathways and mechanisms mediated by MAPK under stress are revealed, this can provide a theoretical basis for tomato stress resistance breeding.
Author contributions
CL: Funding acquisition, Writing – original draft, Writing – review & editing, Supervision. YS: Funding acquisition, Writing – original draft, Writing – review & editing. ZZ: Conceptualization, Validation, Writing – original draft. ZY: Validation, Writing – original draft. HC: Methodology, Writing – original draft.
Funding
The author(s) declare financial support was received for the research, authorship, and/or publication of this article. This work was supported by grants from the National Natural Science Foundation of China (grant number 32460457), the Yunnan Fundamental Research Projects (grant number 202401AU070002), the Yunnan Provincial Department of Education Science Research Fund Project (grant number 2024J0939), and the Special Basic Cooperative Research Innovation Programs of Qujing Science and Technology Bureau & Qujing Normal University (grant numbers KJLH2024ZD04 and KJLH2023YB08).
Acknowledgments
We thank the editor and reviewers for their helpful comments and their input.
Conflict of interest
The authors declare that the research was conducted in the absence of any commercial or financial relationships that could be construed as a potential conflict of interest.
Generative AI statement
The author(s) declare that no Generative AI was used in the creation of this manuscript.
Publisher’s note
All claims expressed in this article are solely those of the authors and do not necessarily represent those of their affiliated organizations, or those of the publisher, the editors and the reviewers. Any product that may be evaluated in this article, or claim that may be made by its manufacturer, is not guaranteed or endorsed by the publisher.
References
Alfagham, A. T., Debnath, S., Perveen, K., Paul, A., Alsayed, M. F., Khanam, M. N. (2024). Computational analysis of albaflavenone interaction with SlMAPK1 for drought resistance in Tomato. Mol. Biotechnol. 2024. doi: 10.1007/s12033-024-01208-4
Amoroso, C. G., D’Esposito, D., Aiese Cigliano, R., Ercolano, M. R. (2023). Comparison of tomato transcriptomic profiles reveals overlapping patterns in abiotic and biotic stress responses. Int. J. Mol. Sci. 24, 4061. doi: 10.3390/ijms24044061
Ba, Y., Zhai, J., Yan, J., Li, K., Xu, H. (2021). H2S improves growth of tomato seedlings involving the MAPK signaling. Sci. Hortic. 288, 110366. doi: 10.1016/j.scienta.2021.110366
Boyer, J. S., Byrne, P., Cassman, K. G., Cooper, M., Delmer, D., Greene, T., et al. (2013). The U.S. drought of 2012 in perspective: A call to action. Global Food Security. 2, 139–143. doi: 10.1016/j.gfs.2013.08.002
Chen, X., Ding, Y., Yang, Y., Song, C., Wang, B., Yang, S., et al. (2021). Protein kinases in plant responses to drought, salt, and cold stress. J. Integr. Plant Biol. 63, 53–78. doi: 10.1111/jipb.13061
Chisholm, S. T., Coaker, G., Day, B., Staskawicz, B. J. (2006). Hostmicrobe interactions: shaping the evolution of the plant immune response. Cell 124, 803–814. doi: 10.1016/j.cell.2006.02.008
Colcombet, J., Hirt, H. (2008). Arabidopsis MAPKs: a complex signaling network involved in multiple biological processes. Biochem. J. 413, 217–226. doi: 10.1042/BJ20080625
Collins, E. J., Bowyer, C., Tsouza, A., Chopra, M. (2022). Tomatoes: An extensive review of the associated health impacts of tomatoes and factors that can affect their cultivation. Biology 11, 239. doi: 10.3390/biology11020239
Danquah, A., de Zelicourt, A., Colcombet, J., Hirt, H. (2014). The role of ABA and MAPK signaling pathways in plant abiotic stress responses. Biotechnol. Adv. 32, 40–52. doi: 10.1016/j.bioteChadv.2013.09.006
de Zelicourt, A., Colcombet, J., Hirt, H. (2016). The role of MAPK modules and ABA during abiotic stress signaling. Trends Plant Sci. 21, 677–685. doi: 10.1016/j.tplants.2016.04.004
Dievart, A., Gottin, C., Périn, C., Ranwez, V., Chantret, N. (2020). Origin and diversity of plant receptor-like kinases. Annu. Rev. Plant Biol. 71, 131–156. doi: 10.1146/annurev-arplant-073019-025927
Ding, H., He, J., Wu, Y., Wu, X., Ge, C., Wang, Y., et al. (2018). The tomato mitogen activated protein kinase SlMPK1 is as a negative regulator of the high-temperature stress response. Plant Physiol. 177, 633–651. doi: 10.1104/pp.18.00067
Dóczi, R., Okrész, L., Romero, A. E., Paccanaro, A., Bögre, L. (2012). Exploring the evolutionary path of plant MAPK networks. Trends Plant Sci. 17, 518–525. doi: 10.1016/j.tplants.2012.05.009
Ekengren, S. K., Liu, Y. L., Schiff, M., Dinesh-Kumar, S. P., Martin, G. B. (2003). Two MAPK cascades, NPR1, and TGA transcription factors play a role in Pto-mediated disease resistance in tomato. Plant J. 36, 905–917. doi: 10.1046/j.1365-313X.2003.01944.x
Fujita, M., Fujita, Y., Noutoshi, Y., Takahashi, F., Narusaka, Y., Yamaguchi Shinozaki, K., et al. (2006). Crosstalk between abiotic and biotic stress responses: a current view from the points of convergence in the stress signaling networks. Curr. Opin. Plant Biol. 9, 436–442. doi: 10.1016/j.pbi.2006.05.014
Gao, W., Feng, Z., Bai, Q., He, J., Wang, Y. (2019). Melatonin-mediated regulation of growth and antioxidant capacity in salt-tolerant naked oat under salt stress. Int. J. Mol. Sci. 20, 1176. doi: 10.3390/ijms20051176
Gontia-Mishra, I., Sasidharan, S., Tiwari, S. (2014). Recent developments in use of 1-aminocyclopropane-1- carboxylate (ACC) deaminase for conferring tolerance to biotic and abiotic stress. Biotechnol. Lett. 36, 889–898. doi: 10.1007/s10529014-1458-9
Halim, V. A., Vess, A., Scheel, D., Rosahl, S. (2006). The role of salicylic acid and jasmonic acid in pathogen defence. Plant Biol. (Stuttg.) 8, 307–313. doi: 10.1055/s-2006-924025
Hazzalin, C. A., Mahadevan, L. C. (2002). MAPK-regulated transcription: A continuously variable gene switch? Nat. Rev. Mol. Cell Biol. 3, 30–40. doi: 10.1038/nrm715
Heng, Y. Q., Jiang, Y., Zhao, X. H., Zhou, H., Wang, X. C., Deng, X. W., et al. (2019). BBX4, a phyB-interacting and modulated regulator, directly interacts with PIF3 to fine tune red light-mediated photomorphogenesis. Proc. Natl. Acad. Sci. U.S.A. 116, 26049–26056. doi: 10.1073/pnas.1915149116
Higgins, R., Lockwood, T., Holley, S., Yalamanchili, R., Stratmann, J. (2007). Changes in extracellular pH are neither required nor sufficient for activa tion of mitogen-activated protein kinases (MAPKs) in response to systemin and fusicoccin in tomato. Planta 225, 1535–1546. doi: 10.1007/s00425-0060440-8
Holley, S. R., Yalamanchili, R. D., Moura, D. S., Ryan, C. A., Stratmann, J. W. (2003). Covergence of signaling pathways induced by systemin, oligosaccharide elicitors, and ultraviolet-B radiation at the level of mitogen-activated protein kinases in Lycopersicon Peruvianum suspension-cultured cells. Plant Physiol. 132, 1728–1738. doi: 10.1104/pp.103.024414
Huang, Z., Tian, Z., Zhao, Y., Zhu, F., Liu, W., Wang, X. (2022). MAPK signaling pathway is essential for female reproductive regulation in the cabbage beetle, colaphellus bowringi. Cells 11, 1602. doi: 10.3390/cells11101602
Huang, X., Wei, J. M., Feng, W. Z., Luo, Q., Tan, G. F., Li, Y. Z. (2023). Interaction between SlMAPK3 and SlASR4 regulates drought resistance in tomato (Solanum lycopersicum L.). Mol. Breed. 43, 73. doi: 10.1007/s11032-023-01418-9
Iba, K. (2002). Acclimative response to temperature stress in higher plants: approaches of gene engineering for temperature tolerance. Annu. Rev. Plant Biol. 53, 225–245. doi: 10.1146/annurev.arplant.53.100201.160729
Jiang, M., Zhang, Y. Z., Li, P., Jian, J. J., Zhao, C. L., Wen, G. S. (2022). Mitogen-activated protein kinase and substrate identification in plant growth and development. Int. J. Mol. Sci. 23, 2744. doi: 10.3390/ijms23052744
Kan, Y., Mu, X. R., Gao, J., Lin, H. X., Lin, Y. (2023). The molecular basis of heat stress responses in plants. Mol. Plant 16, 1612–1634. doi: 10.1016/j.molp.2023.09.013
Kandoth, P. K., Ranf, S., Pancholi, S. S., Jayanty, S., Walla, M. D., Miller, W., et al. (2007). Tomato MAPKs LeMPK1, LeMPK2, and LeMPK3 function in the systemin mediated defense response against herbivorous insects. Proc. Natl. Acad. Sci. U S A. 104, 12205–12210. doi: 10.1073/pnas.0700344104
Kissoudis, C., Chowdhury, R., van Heusden, S., van de Wiel, C., Finkers, R., Visser, R. G., et al. (2015). Combined biotic and abiotic stress resistance in tomato. Euphytica 202, 317–332. doi: 10.1007/s10681-015-1363-x
Kolbert, Z. (2019). Strigolactone-nitric oxide interplay in plants: the story has just begun. Physiol. Plant 165, 487–497. doi: 10.1111/ppl.12712
Kong, F., Wang, J., Cheng, L., Liu, S., Wu, J., Peng, Z., et al. (2012). Genome-wide analysis of the mitogen-activated protein kinase gene family in Solanum lycopersicum. Gene 499, 108–120. doi: 10.1016/j.gene.2012.01.048
Kumar, N., Pruthi, V. (2014). Potential applications of ferulic acid from natural sources. Biotechnol. Rep. 4, 86–93. doi: 10.1016/j.btre.2014.09.002
Kumar, K., Raina, S. K., Sultan, S. M. (2020). Arabidopsis MAPK signaling pathways and their cross talks in abiotic stress response. J. Plant Biochem. Biotechnol. 29, 700–714. doi: 10.1007/s13562-020-00596-3
Lee, S. C., Luan, S. (2012). ABA signal transduction at the crossroad of biotic and abiotic stress responses. Plant Cell Environ. 35, 53–60. doi: 10.1111/j.13653040.2011.02426.x
Lefebvre, V., North, H., Frey, A., Sotta, B., Seo, M. M., Okamoto, M., et al. (2006). Functional analysis of Arabidopsis NCED6 and NCED9 genes indicates that ABA synthesized in the endosperm is involved in the induction of seed dormancy. Plant J. 45, 309–319. doi: 10.1111/j.1365-313x.2005.02622.x
Lesk, C., Rowhani, P., Ramankutty, N. (2016). Influence of extreme weather disasters on global crop production. Nature 529, 84–87. doi: 10.1038/nature16467
Li, Y., Cai, H., Liu, P., Wang, C., Gao, H., Wu, C., et al. (2017). Arabidopsis MAPKKK18 positively regulates drought stress resistance via downstream MAPKK3. Biochem. Biophys. Res. Commun. 484, 292–297. doi: 10.1016/j.bbrc.2017.01.104
Li, X., Zhang, Y., Huang, L., Ouyang, Z., Hong, Y., Zhang, H., et al. (2014). Tomato SlMKK2 and SlMKK4 contribute to disease resistance against Botrytis cinerea. BMC Plant Biol. 14, 166. doi: 10.1186/1471-2229-14-166
Lin, L., Wu, J., Jiang, M., Wang, Y. (2021). Plant mitogen-activated protein kinase cascades in environmental stresses. Int. J. Mol. Sci. 22, 1543. doi: 10.3390/ijms22041543
Link, V., Sinha, A. K., Vashista, P., Hofmann, M. G., Proels, R. K., Ehness, R., et al. (2002). A heat-activated MAP kinase in tomato: a possible regulator of the heat stress response. FEBS Lett. 531, 179–183. doi: 10.1016/S0014-5793(02)03498-1
Lu, F., Wang, Y., Li, K., Xu, H. (2024). NO alleviates oxidative damage and improves nitrogen metabolism in tomato under low nitrogen stress through the MAPK signaling pathway. Sci. Hortic. 2024, 338. doi: 10.1016/j.scienta.2024.113733
Luo, C., Bashir, N. H., Li, Z., Liu, C., Shi, Y., Chu, H. (2024). Plant microRNAs regulate the defense response against pathogens. Front. Microbiol. 15. doi: 10.3389/fmicb.2024.1434798
Majeed, Y., Zhu, X., Zhang, N., Ul-Ain, N., Raza, A., Haider, F. U., et al. (2023). Harnessing the role of mitogen-activated protein kinases against abiotic stresses in plants. Front. Plant Sci. 14. doi: 10.3389/fpls.2023.932923
Manasa, S. ,. L., Panigrahy, M., Panigrahi, K. C. S., Rout, G. R. (2022). Overview of cold stress regulation in plants. Bot. Rev. 88, 359–387. doi: 10.1016/j.envexpbot.2020.104243
MAPK Group (2002). Mitogen-activated protein kinase cascades in plants: a new nomenclature. Trends Plant Sci. 7, 301–308. doi: 10.1016/S1360-1385(02)02302-6
Matsuoka, D., Yasufuku, T., Furuya, T., Nanmori, T. (2015). An abscisic acid inducible Arabidopsis MAPKKK, MAPKKK18 regulates leaf senescence via its kinase activity. Plant Mol. Biol. 87, 565–575. doi: 10.1007/s11103-015-0295-0
Mayrose, M., Bonshtien, A., Sessa, G. (2004). LeMPK3 is a mitogen-activated protein kinase with dual specificity induced during tomato defense and wounding responses. J. Biol. Chem. 279, 14819–14827. doi: 10.1074/jbc.M313388200
Melech-Bonfil, S., Sessa, G. (2010). Tomato MAPKKKepsilon is a positive regulator of cell-death signaling networks associated with plant immunity. Plant J. 64, 379–391. doi: 10.1111/j.1365-313X.2010.04333.x
Melech-Bonfil, S., Sessa, G. (2011). The SlMKK2 and SlMPK2 genes play a role in tomato disease resistance to xanthomonas campestris pv. vesicatoria. Plant Signal. Behav. 6, 154–156. doi: 10.4161/psb.6.1.14311
Meng, X., Zhang, S. (2013). MAPK cascades in plant disease resistance signaling. Annu. Rev. Phytopathol. 51, 245–266. doi: 10.1146/annurev-phyto-082712-102314
Miura, K., Shiba, H., Ohta, M., Kang, S. W., Sato, A., Yuasa, T., et al. (2012). SlICE1 encoding a MYC-type transcription factor controls cold tolerance in tomato, Solanum lycopersicum. Plant Biotechnol. 29, 253–260. doi: 10.5511/plantbiotechnology.12.0303a
Mo, S., Qian, Y., Zhang, W., Qian, L., Wang, Y., Cailin, G., et al. (2021). Mitogen activated protein kinase action in plant response to high-temperature stress: a mini review. Protoplasma 258, 477–482. doi: 10.1007/s00709-020-01603-z
Moustafa, K., Abu-Qamar, S., Jarrar, M., Al-Rajab, J.-A., Tremouillaux-Guiller, J. (2014). MAPK cascades and major abiotic stresses. Plant Cell Rep. 33, 1217–1225. doi: 10.1007/s00299-014-1629-0
Muhammad, T., Zhang, J., Ma, Y., Li, Y., Zhang, F., Zhang, Y., et al. (2019). Overexpression of a mitogen-activated protein kinase SlMAPK3 positively regulates tomato tolerance to cadmium and drought stress. Molecules 24, 556. doi: 10.3390/molecules24030556
Nagy, S. K., Darula, Z., Kallai, B. M., Bogre, L., Banhegyi, G., Medzihradszky, K. F., et al. (2015). Activation of AtMPK9 through autophosphorylation that makes it independent of the canonical MAPK cascades. Biochem. J. 467, 167–175. doi: 10.1042/BJ20141176
Nambeesan, S., AbuQamar, S., Laluk, K., Mattoo, A. K., Mickelbart, M. V., Ferruzzi, M. G., et al. (2012). Polyamines attenuate ethylene-mediated defense responses to abrogate resistance to botrytis cinerea in tomato. Plant Physiol. 158, 1034–1045. doi: 10.1104/pp.111.188698
Ni, Z. J., Hu, K. D., Song, C. B., Ma, R. H., Li, Z. R., Zheng, J. L., et al. (2016). Hydrogen sulfide alleviates postharvest senescence of grape by modulating the antioxidant defenses. Oxid. Med. Cell. Longevity. 2016, 0–4715651. doi: 10.1155/2016/4715651
Noman, M., Ahmed, T., Ijaz, U., Shahid, M., Azizullah, Li, D., et al. (2021). Plant– Microbiome crosstalk: Dawning from composition and assembly of microbial community to improvement of disease resilience in plants. Int. J. Mol. Sci. 22, 6852. doi: 10.3390/ijms22136852
Osakabe, Y., Yamaguchi-Shinozaki, K., Shinozaki, K., Tran, L.-S. P. (2013). Sensing the environment: key roles of membrane-localized kinases in plant perception and response to abiotic stress. J. Exp. Bot. 64, 445–458. doi: 10.1093/jxb/ers354
Pedley, K., Martin, G. (2004). Identification of MAPKs and their possible MAPK kinase activators involved in the Pto-mediated defense response of tomato. J. Biol. Chem. 279, 49229–49235. doi: 10.1074/jbc.M410323200
Pitzschke, A., Djamei, A., Bitton, F., Hirt, H. (2009). A major role of the MEKK1- MKK1/2-MPK4 pathway in ROS signalling. Mol. Plant 2, 120–137. doi: 10.1093/mp/ssn079
Rodriguez, M. C., Petersen, M., Mundy, J. (2010). Mitogen-activated protein kinase signaling in plants. Annu. Rev. Plant Biol. 61, 621–649. doi: 10.1146/annurevarplant-042809-112252
Saijo, Y., Loo, E. P. (2020). Plant immunity in signal integration between biotic and abiotic stress responses. New Phytol. 225, 87–104. doi: 10.1111/nph.15989
Sangwan, V., Dhindsa, R. S. (2002). In vivo and in vitro activation of temperature-responsive plant map kinases. FEBS Lett. 531, 561–564. doi: 10.1016/s0014-5793(02)03626-8
Shi, H., Li, Q., Luo, M., Yan, H., Xie, B., Li, X., et al. (2022). BRASSINOSTEROID-SIGNALING KINASE1 modulates MAP KINASE15 phosphorylation to confer powdery mildew resistance in Arabidopsis. Plant Cell. 34, 1768–1783. doi: 10.1093/plcell/koac027
Shu, P., Li, Y., Li, Z., Xiang, L., Sheng, J., Shen, L. (2022). Ferulic acid enhances chilling tolerance in tomato fruit by up-regulating the gene expression of CBF transcriptional pathway in MAPK3-dependent manner. Postharvest Biol. Technol. 185, 11775. doi: 10.1016/j.postharvbio.2021.111775
Song, J., Lin, R., Tang, M., Wang, L., Fan, P., Xia, X., et al. (2023). SlMPK1- and SlMPK2-mediated SlBBX17 phosphorylation positively regulates CBF-dependent cold tolerance in tomato. New Phytol. 239, 1887–1902. doi: 10.1111/nph.19072
Song, J., Shang, L., Wang, X., Xing, Y., Xu, W., Zhang, Y., et al. (2021). MAPK11 regulates seed germination and ABA signaling in tomato by phosphorylating SnRKs. J. Exp. Bot. 72, 1677–1690. doi: 10.1093/jxb/eraa56
Stulemeijer, I. J. E., Stratmann, J. W., Joosten, M. H. A. J. (2007). Tomato mitogen-activated protein kinases LeMPK1, LeMPK2, and LeMPK3 are activated during the Cf-4/Avr4-induced hypersensitive response and have distinct phosphorylation specificities. Plant Physiol. 144, 1481–1494. doi: 10.1104/pp.107.101063
Tajdel-Zielińska, M., Janicki, M., Marczak, M., Ludwików, A. (2024). Arabidopsis HECT and RING-type E3 ligases promote MAPKKK18 degradation to regulate abscisic acid signaling. Plant Cell Physiol. 65, 390–404. doi: 10.1093/pcp/pcad165
Tena, G., Asai, T., Chiu, W. L., Sheen, J. (2001). Plant mitogen-activated protein kinase signaling cascades. Curr. Opin. Plant Biol. 4, 392–400. doi: 10.1016/S1369-5266(00)00191-6
Teshome, D. T., Zharare, G. E., Naidoo, S. (2020). The threat of the combined effect of biotic and abiotic stress factors in forestry under a changing climate. Front. Plant Sci. 11. doi: 10.3389/fpls.2020.601009
Thulasi Devendrakumar, K., Li, X., Zhang, Y. (2018). MAP kinase signalling: interplays between plant PAMP- and effector-triggered immunity. Cell Mol. Life Sci. 75, 2981–2989. doi: 10.1007/s00018-018-2839-3
Tian, B., Qiao, Z., Zhang, L., Li, H., Pei, Y. (2016). Hydrogen sulphide and proline cooperate to alleviate cadmium stress in foxtail millet seedlings. Plant Physiol. Biochem. 109, 293–299. doi: 10.1016/j.plaphy.2016.10.006
Virk, N., Liu, B., Zhang, H., Li, X., Zhang, Y., Li, D., et al. (2013). Tomato SlMPK4 is required for resistance against Botrytis cinerea and tolerance to drought stress. Acta Physiol. Plant 35, 1211–1221. doi: 10.1007/s11738-012-1160-2
Wang, L., Chen, L., Li, R., Zhao, R., Yang, M., Sheng, J., et al. (2017a). Reduced drought tolerance by CRISPR/Cas9-mediated SlMAPK3 mutagenesis in tomato plants. J. Agric. Food Chem. 65, 8674–8682. doi: 10.1021/acs.jafc.7b02745
Wang, Z., Li, X., Yao, X., Ma, J., Lu, K., An, Y., et al. (2023). MYB44 regulates PTI by promoting the expression of EIN2 and MPK3/6 in Arabidopsis. Plant Commun. 4, 100628. doi: 10.1016/j.xplc.2023.100628
Wang, L., Zhao, R., Li, R., Yu, W., Yang, M., Sheng, J., et al. (2018). Enhanced drought tolerance in tomato plants by overexpression of SlMAPK1. Plant Cell Tiss. Organ Cult. 133, 27–38. doi: 10.1007/s11240-017-1358-5
Wang, L., Zhao, R. R., Zheng, Y. Y., Chen, L., Li, R., Ma, J. F., et al. (2017b). SlMAPK1/2/3 and antioxidant enzymes are associated with H2O2-induced chilling tolerance in tomato plants. J. Agric. Food Chem. 65, 6812–6820. doi: 10.1021/acs.jafc.7b01685
Wei, L., Feng, L., Liu, Y., Liao, W. (2022). Mitogen-activated protein kinase is involved in salt stress response in tomato (Solanum lycopersicum) seedlings. Int. J. Mol. Sci. 23, 7645. doi: 10.3390/ijms23147645
Wu, J., Hettenhausen, C., Meldau, S., Baldwin, I. T. (2007). Herbivory rapidly activates MAPK signaling in attacked and unattacked leaf regions but not between leaves of Nicotiana attenuata. Plant Cell 19, 1096–1122. doi: 10.1105/tpc.106.049353
Wu, J., Nadeem, M., Galagedara, L., Thomas, R., Cheema, M. (2022). Recent insights into cell responses to cold stress in plants: Signaling, defence, and potential functions of phosphatidic acid. Environ. Exp. Bot. 203, 105068. doi: 10.1016/j.envexpbot.2022.105068
Wu, G., Wang, W. (2024). Recent advances in understanding the role of two mitogen-activated protein kinase cascades in plant immunity. J. Exp. Bot. 75, 2256–2265. doi: 10.1093/jxb/erae020
Wu, M., Wang, S., Ma, P., Li, B., Hu, H., Wang, Z., et al. (2024). Dual roles of the MPK3 and MPK6 mitogen-activated protein kinases in regulating Arabidopsis stomatal development. Plant Cell. 36, 4576–4593. doi: 10.1093/plcell/koae225
Xing, Y., Jia, W., Zhang, J. (2008). AtMKK1 mediates ABA-induced CAT1 expression and H2O2 production via AtMPK6-coupled signaling in Arabidopsis. Plant J. 54, 440–451. doi: 10.1111/j.1365-313X.2008.03433.x
Yamasaki, H., Cohen, M. F. (2016). Biological consilience of hydrogen sulfide and nitric oxide in plants: gases of primordial earth linking plant, microbial and animal physiologies. Nitric. Oxide 55, 91–100. doi: 10.1016/j.niox.2016.04.002
Yu, W., Wang, L., Zhao, R., Sheng, J., Zhang, S., Li, R., et al. (2019). Knockout of SlMAPK3 enhances tolerance to heat stress involving ROS homeostasis in tomato plants. BMC Plant Biol. 19, 1–13. doi: 10.1186/s12870-019-1939-z
Yu, L., Yan, J., Yang, Y., He, L., Zhu, W. (2015b). Enhanced tolerance to chilling stress in tomato by overexpression of a mitogen-activated protein kinase, SlMPK7. Plant Mol. Biol. Rep. 34, 76–88. doi: 10.1007/s11105-015-0897-3
Yu, L., Yan, J., Yang, Y., Zhu, W. (2015a). Overexpression of tomato mitogen-activated protein kinase SlMPK3 in tobacco increases tolerance to low temperature stress. Plant Cell Tiss. Organ. Cult. 121, 21–34. doi: 10.1007/s11240-014-0675-1
Zhang, X., Du, H., Shi, Q., Gong, B. (2022b). Loss of GSNOR increases abiotic stress sensitivity via regulating MAPK-ethylene cascade signaling in Solanum lycopersicum L. Environ. Exp. Bot. 199, 104872. doi: 10.1016/j.envexpbot.2022.104872
Zhang, S., Klessig, D. F. (2001). MAPK cascades in plant defense signaling. Trends Plant Sci. 6, 520–527. doi: 10.1016/s1360–1385(01)02103–3
Zhang, M., Zhang, S. (2022). Mitogen-activated protein kinase cascades in plant signaling. J. Integr. Plant Biol. 64, 301–341. doi: 10.1111/jipb.13215
Zhang, H., Zhu, J., Gong, Z., Zhu, J. K. (2022a). Abiotic stress responses in plants. Nat. Rev. Genet. 23, 104–119. doi: 10.1038/s41576-021-00413-0
Zheng, Y., Yang, Y., Liu, C., Chen, L., Sheng, J., Shen, L. (2015). Inhibition of SlMPK1, SlMPK2, and SlMPK3 disrupts defense signaling pathways and enhances tomato fruit susceptibility to Botrytis cinerea. J. Agric. Food Chem. 63, 5509–5517. doi: 10.1021/acs.jafc.5b00437
Keywords: mitogen-activated protein kinases, biotic stress, abiotic stress, signal transduction, tomato
Citation: Shi Y, Zhang Z, Yan Z, Chu H and Luo C (2025) Tomato mitogen-activated protein kinase: mechanisms of adaptation in response to biotic and abiotic stresses. Front. Plant Sci. 16:1533248. doi: 10.3389/fpls.2025.1533248
Received: 23 November 2024; Accepted: 20 January 2025;
Published: 03 February 2025.
Edited by:
Wajid Zaman, Yeungnam University, Republic of KoreaReviewed by:
Qiuling Wang, Northwest A&F University, ChinaCiro Gianmaria Amoroso, University of Naples Federico II, Italy
Dongbao Li, Nanjing University, China
Ayesha Khan, Lithuanian Research Centre for Agriculture and Forestry, Lithuania
Copyright © 2025 Shi, Zhang, Yan, Chu and Luo. This is an open-access article distributed under the terms of the Creative Commons Attribution License (CC BY). The use, distribution or reproduction in other forums is permitted, provided the original author(s) and the copyright owner(s) are credited and that the original publication in this journal is cited, in accordance with accepted academic practice. No use, distribution or reproduction is permitted which does not comply with these terms.
*Correspondence: Changxin Luo, bHVvY2hhbmd4aW5AbWFpbC5xam51LmVkdS5jbg==