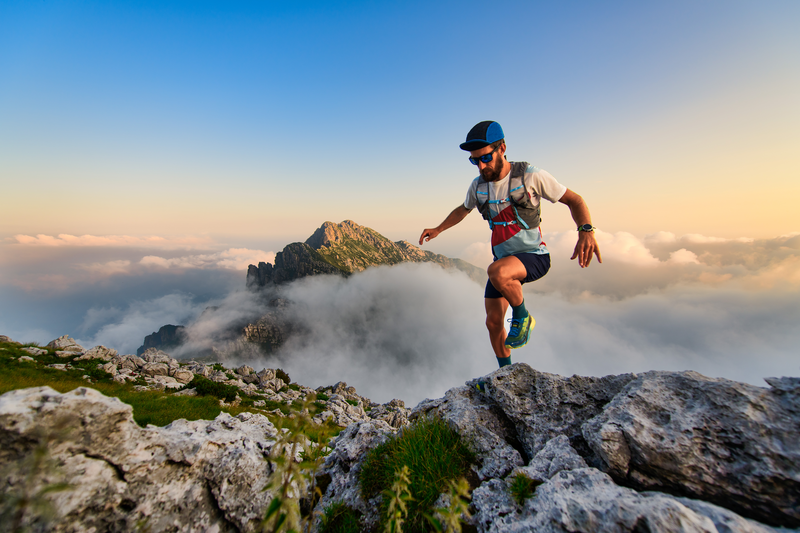
95% of researchers rate our articles as excellent or good
Learn more about the work of our research integrity team to safeguard the quality of each article we publish.
Find out more
MINI REVIEW article
Front. Plant Sci. , 07 January 2025
Sec. Plant Pathogen Interactions
Volume 15 - 2024 | https://doi.org/10.3389/fpls.2024.1518880
This article is part of the Research Topic Unraveling Molecular Mechanisms of Citrus Resistance to Huanglongbing View all 4 articles
Citrus Huanglongbing (HLB) represents a significant threat to the citrus industry, mainly caused by the phloem-limited bacterium Candidatus Liberibacter asiaticus (CLas). In this review, we summarize recent advances in understanding the relationship between citrus and CLas, particularly examining the functions of Sec-dependent effectors (SDEs) and non-classically secreted proteins (ncSPs) in virulence, as well as their targeted interactions with citrus. We further investigate the impact of SDEs on various physiological processes, including systemic acquired resistance (SAR), reactive oxygen species (ROS) accumulation, vesicle trafficking, callose deposition, cell death, autophagy, chlorosis and flowering. Additionally, we focus on the functional research on specific disease-resistant genes in citrus and the molecular mechanisms underlying disease resistance. Finally, we discuss the existing gaps and unresolved questions regarding citrus-CLas interactions, proposing potential solutions to facilitate the development of HLB-resistant citrus varieties.
Citrus Huanglongbing (HLB), caused by the phloem-restricted bacterium Candidatus Liberibacter asiaticus (CLas), americanus (CLam), and africanus (CLaf), is one of the most devastating diseases affecting the citrus industry (Bové, 2006; Wang, 2019; Sivager et al., 2021). Currently, CLas is most widely spread in citrus production areas in Asia and America (Zhou, 2020; Alquézar et al., 2021b). The disease is primarily transmitted in the field by the Asian citrus psyllid (Diaphorina citri Kuwayama). CLas proliferates within the psyllid and is subsequently transmitted to the phloem sieve elements of host plants through the insect’s saliva during feeding on young shoots (Hall et al., 2013; Alquézar et al., 2021a). CLas infects various citrus tissues, including stems, leaves, fruits, and roots, leading to phloem blockages that result in stunted growth, reduced fruit size, elevated juice acidity, and potentially tree mortality (Gottwald, 2010; Ma et al., 2022).
The genome of the CLas is approximately 1.2 ~ 1.3 Mb and lacks type III and type IV secretion systems, while containing a complete type I secretion system and the general secretory (Sec) pathway (Duan et al., 2009; Zheng et al., 2024). The Sec pathway is essential for the transmembrane transport of bacterial proteins, and the Sec-dependent effectors (SDEs) are important virulence factors of phloem-colonizing bacteria that cause plant diseases (Sugio et al., 2011; Tomkins et al., 2018). Thus, elucidating the biological functions of SDEs in the CLas infection process in citrus could provide valuable insights into the pathogenic mechanisms utilized by CLas.
To comprehensively elucidate the biological interactions between CLas and citrus, as well as to deepen our understanding of CLas pathogenic mechanisms and citrus immune responses, this review summarizes recent research on SDEs and non-classically secreted proteins (ncSPs) in CLas. Additionally, this review also emphasizes genes validated in vivo that contribute to enhancing citrus resistance to HLB.
To overcome the host’s defenses against bacterial proliferation, bacteria often deploy effectors to disrupt the host’s immune responses, thereby reducing the host’s resistance to infection (Shan et al., 2008; Wang et al., 2022). In CLas, there are 86 proteins that have been experimentally confirmed to have signal peptides (Prasad et al., 2016). Analyzing the biological processes that SDEs are involved in within plant cells can provide insights into the pathogenic mechanisms of CLas. It is observed that various SDEs are involved in the inhibition of specific processes in plant cells. When SDE15 (CLIBASIA_04025) interacts with ACCELERATED CELL DEATH 2 (ACD2), it has the effect of suppressing hypersensitive response (HR) cell death in plants (Pang et al., 2020). AGH17488 (a SDE in CLas strain gxpsy, its homologous protein in CLas strain psy62 is CLIBASIA_05590) is able to target and promote the enzyme activity of ascorbate peroxidase 6 (APX6) in citrus, ultimately leading to the inhibition of ROS accumulation (Du et al., 2023). Moreover, CLas0185 (CLIBASIA_00185) interacts with methionine sulphoxide reductase B1 (CsMsrB1) and boosts the enzyme activity of ascorbate peroxidase 1 (APX1) in citrus, resulting in a reduction of H2O2 content (Zhang et al., 2024). It is worth noting that SDE4310 (CLIBASIA_04310), SDE4435 (CLIBASIA_04435), and SDE4955 (CLIBASIA_04955), which are able to inhibit cell death and ROS accumulation, are discovered to interact with Arabidopsis thaliana CAT3 and GAPA (Li et al., 2024). Furthermore, m3875 (CLIBASIA_03875), m4405 (CLIBASIA_04405), and SECP8 (CLIBASIA_05330) are identified as suppressors of ROS accumulation (Zhang et al., 2019, 2020; Shen et al., 2022). Specifically, m4405, also known as SDE4405, is referred to the same SDE in different literatures (Zhang et al., 2020; Shi et al., 2023a). In addition, SDE3 (CLIBASIA_00420) interacts with citrus cytosolic glyceraldehyde-3-phosphate dehydrogenases (CsGAPCs) causing impairment of autophagy in citrus, consequently diminishing plant immunity (Shi et al., 2023b). SDE19 (CLIBASIA_05320) interacts with Sec12, causing disruption to vesicle trafficking and callose deposition in plants (Huang et al., 2024a). Furthermore, the overexpression of CaLasSDE115 (CLIBASIA_05115) impedes the citrus systemic acquired resistance (SAR) response and boosts the early establishment of CLas infection (Du et al., 2022).
However, some SDEs can greatly stimulate the host’s response. For instance, SDE1 (CLIBASIA_05315) has been shown to induce reactive oxygen species (ROS) accumulation, cell death, and chlorosis in plants (Clark et al., 2018; Pitino et al., 2018; Clark et al., 2020; Zhou et al., 2020). Additionally, studies have proven that SDEs like CaLasSDE460 (CLIBASIA_00460) can cause chlorosis and cell death (Liu et al., 2019; Wang et al., 2023), while FlgI (CLIBASIA_01305) can trigger callose deposition and cell death (Zuo et al., 2024). Moreover, m3915 (CLIBASIA_03915) and m4250 (CLIBASIA_04250) have been shown to induce cell death (Li et al., 2020), and CLas4425 (CLIBASIA_04425) can also result in cell death and the accumulation of ROS (Zhang et al., 2023). SDE4405 (CLIBASIA_04405) has been found to interact with ATG8-family proteins (ATG8s) which leads to the stimulation of autophagy in plants (Shi et al., 2023a). Furthermore, it is interesting to note that SDE1 interacts with various citrus papain-like cysteine proteases (PLCPs) and the DEAD-box RNA helicase (DDX3) (Clark et al., 2018; Zhou et al., 2020). Since PLCPs and DEAD-box RNA helicase have been demonstrated to play a role in defense mechanisms against pathogen invasion (Li et al., 2008; Misas-Villamil et al., 2016), it can be inferred that SDE1 weakens the immune response in citrus by interacting with PLCPs and DEAD-box RNA helicase, thereby promoting CLas infection. Consequently, the query of whether these SDEs are utilized as weapons by CLas to counteract the host’s defense system in the infection process remains disputed and calls for additional research.
Among ncSPs, SC2_gp095 (No annotated homologous proteins were identified in the CLas strain psy62 according to a BLASTp search conducted on October 5, 2024.), a nonclassical secreted peroxidase of CLas, is capable of reducing the accumulation of ROS, thereby suppressing HLB symptoms (Jain et al., 2015). LasBCP (CLIBASIA_00445), a peroxiredoxin secreted by CLas, has the ability to suppress the SAR response and inhibit callose deposition in plants (Jain et al., 2018, 2019, 2021). Furthermore, ncSPs LasRNHI (CLIBASIA_03435) suppresses plant flowering by interacting with a citrus B-box zinc finger protein CsBBX28 to inhibit CsBBX28’s regulation of FLOWERING LOCUS T expression (Du et al., 2024).
In brief, CLas uses SDEs and ncSPs as pathogen weapons to disrupt the regular functioning of citrus cells, suppress plant immune responses, and advance the infection process of CLas (Figure 1). Therefore, how can citrus combat CLas infection?
Figure 1. The interactions between Candidatus Liberibacter asiaticus (CLas) and citrus. The schematic diagram illustrates the virulence functions of Sec-dependent effectors (SDEs) and non-classically secreted proteins (ncSPs), along with their potential targets in citrus-CLas interactions. The dashed line ending with an arrow indicates activation, while the end marked with a diamond signifies suppression.
The nonexpressor of pathogenesis-related genes 1 (NPR1) is a crucial activator in salicylic acid (SA)-mediated immune responses, exhibiting diverse roles in plant resistance to various pathogens (Zavaliev and Dong, 2024). Overexpression of A. thaliana NPR1 (AtNPR1) significantly improved citrus resistance to CLas infection, likely by activating the citrus SA signaling pathway, which elevated the plant’s immune response (Qiu et al., 2020). Subsequently, overexpression of CiNPR4, an NPR1-like gene from Citrus paradisi, and CsNPR1 from C. sinensis indicated that the transgenic lines exhibited enhanced resistance to HLB (Peng et al., 2021; Wu et al., 2021). Given that activation of the SA signaling pathway can bolster citrus resistance to HLB, the overexpression of SA methyltransferase (CsSAMT1) in citrus elevated levels of SA and methyl salicylate (MeSA), thereby enhancing resistance to CLas infection (Zou et al., 2021). Notably, the introduction of transgenic SA binding protein 2 (NtSABP2) from tobacco, which plays a role in systemic acquired resistance (SAR), also markedly improved citrus resistance to HLB (Soares et al., 2022).
Antimicrobial peptides (AMPs) are essential components of the plant immune response against bacterial infections (Campos et al., 2018). Recently, an exciting study has found that stable antimicrobial peptides (SAMPs) derived from Microcitrus australiasica Australian finger lime (MaSAMP) can strongly inhibit the proliferation of CLas (Huang et al., 2021). Moreover, A chimeric peptide, UGK17, has demonstrated bactericidal activities against CLas in citrus (Basu et al., 2022; Choi et al., 2023). These findings suggest that AMPs are an effective strategy for the prevention and control of HLB. Additionally, the overexpression of the endolysin gene LasLYS2 (CLIBASIA_04800) in citrus provides significant dual resistance to both HLB and citrus canker, effectively preventing the colonization of CLas in transgenic plants (Xu et al., 2023).
In short, despite the fact that there are constrained resistance mechanisms to HLB, current research findings offer promising applications for the citrus industry. However, this approach faces considerable challenges and requires substantial effort.
The researchers have found that SDEs have an impact on various plant biological processes, including callose deposition, vesicle trafficking, SAR response, chlorosis, cell death, ROS accumulation, flowering, and autophagy (Clark et al., 2020; Pang et al., 2020; Du et al., 2023; Shi et al., 2023a, 2023; Du et al., 2024; Huang et al., 2024a; Zhang et al., 2024). It is interesting to note that certain SDEs can either promote or suppress ROS accumulation to facilitate CLas infection. For instance, SDE1 and CLas4425 can induce ROS accumulation, while CLas0185, AGH17488, m3875, m4405, SECP8, SDE4310, SDE4435, and SDE4955 can suppress it. The questions arise: why does both the induction and suppression of these biological processes benefit CLas infection in plants? Is CLas simultaneously regulating these processes during infection, or does it continuously change its strategies to alter the plant cell environment for its own survival throughout the infection process? Challenging work includes identifying which specific SDEs are critical for CLas infection and determining whether these SDEs operate independently or synergistically. If a synergistic interaction occurs, what regulatory mechanisms govern their interplay?
Although there are studies providing evidence that HLB is a pathogen immune-mediated disease (Ma et al., 2022), there are still many unanswered questions. For example, in the citrus-CLas interaction process, CLas induces or inhibits ROS accumulation through multiple SDEs or ncSPs (Jain et al., 2015; Clark et al., 2020; Du et al., 2023; Zhang et al., 2023; Li et al., 2024; Zhang et al., 2024). Additionally, in CLas infection samples, the expression trend of ROS metabolism-related genes is not completely reprogrammed. Out of the 91 ROS metabolism-related genes, 30 showed significant differential expression, with 16 being up-regulated and 14 down-regulated (Huang et al., 2024b). So, how does citrus trigger an ROS burst if it is not a result of CLas manipulating ROS accumulation during infection?
Fortunately, new technologies offer hope and illumination in tackling these scientific challenges. Despite the inability to culture CLas in vitro (Wang, 2019), spatial single-cell transcriptomics (Giacomello, 2021) can provide valuable insights into the CLas infection process in the citrus phloem tissues and the response of citrus to CLas infection. This can potentially address numerous unanswered questions. Additionally, due to the low abundance of CLas transcripts in citrus tissues, conventional sequencing methods may have limitations in obtaining sequencing reads (De Francesco et al., 2022). Higher-throughput sequencing instruments or methods, such as the NovaSeq X sequence platform and Data-independent acquisition (DIA) proteomics, can be utilized. Moreover, there have been 27 core SDEs identified in CLas (Thapa et al., 2020), but only 7 of them have been analyzed for their functions so far (Figure 1). The functions of most core SDEs remain unclear, so yeast two-hybrid and immunoprecipitation-mass spectrometry techniques can be used to identify targets of core SDEs in citrus, in order to obtain potential candidate susceptibility genes. Subsequently, by utilizing transgene-free CRISPR/Cas9 or Cas12a/crRNA technology to knock out these genes that interact with CLas SDEs in citrus (Wang, 2019; Alquézar et al., 2022; Su et al., 2023), it can be determined if disrupting the interaction can enhance citrus resistance to HLB and generate HLB-resistant citrus lines. Furthermore, it was observed that a full resistance to CLas was manifested in citrus relatives, such as Eremocitrus glauca, Microcitrus warburgiana, M. papuana, and M. australis, along with hybrids either among them or between them and Citrus (Alves et al., 2021, 2022). These germplasm resources offer a substantial genetic foundation for discerning citrus resistance to CLas infection and further bolster research pertaining to interactions between plants and phloem-invading pathogens. A prior study indicated that the overexpression of AtNRP1 can boost citrus resistance to CLas (Qiu et al., 2020), thus reinforcing our conviction that resistance genes sourced from these citrus relatives will substantially contribute to enhancing citrus resistance to CLas.
In conclusion, the pathogenic mechanism of CLas remains unclear, and many mysteries surrounding citrus-CLas interaction still need to be unraveled. However, with the ongoing accumulation of research findings and the development of new experimental methods, we remain optimistic that the eradication of HLB in the citrus industry is within reach.
YH: Investigation, Writing – original draft. NL: Investigation, Writing – original draft. KB: Investigation, Writing – original draft. SL: Investigation, Writing – original draft. RL: Conceptualization, Funding acquisition, Project administration, Writing – original draft, Writing – review & editing. GH: Investigation, Project administration, Writing – original draft, Writing – review & editing.
The author(s) declare financial support was received for the research, authorship, and/or publication of this article. This work was supported by the National Natural Science Foundation of China (grant numbers 32160621, 32260659), and the National Natural Science Foundation of Jiangxi Province (grant numbers 20242BAB20289).
The authors declare that the research was conducted in the absence of any commercial or financial relationships that could be construed as a potential conflict of interest.
The author(s) declare that no Generative AI was used in the creation of this manuscript.
All claims expressed in this article are solely those of the authors and do not necessarily represent those of their affiliated organizations, or those of the publisher, the editors and the reviewers. Any product that may be evaluated in this article, or claim that may be made by its manufacturer, is not guaranteed or endorsed by the publisher.
Alquézar, B., Bennici, S., Carmona, L., Gentile, A., Peña, L. (2022). Generation of transfer-DNA-free base-edited citrus plants. Front. Plant Sci. 13, 835282. doi: 10.3389/fpls.2022.835282
Alquézar, B., Carmona, L., Bennici, S., Miranda, M. P., Bassanezi, R. B., Peña, L. (2021a). Cultural management of Huanglongbing: current status and ongoing research. Phytopathology 112, 11–25. doi: 10.1094/PHYTO-08-21-0358-IA
Alquézar, B., Carmona, L., Bennici, S., Peña, L. (2021b). Engineering of citrus to obtain huanglongbing resistance. Curr. Opin. Biotechnol. 70, 196–203. doi: 10.1016/j.copbio.2021.06.003
Alves, M. N., Lopes, S. A., Raiol-Junior, L. L., Wulff, N. A., Girardi, E. A., Ollitrault, P., et al. (2021). Resistance to ‘Candidatus Liberibacter asiaticus,’ the Huanglongbing Associated Bacterium, in Sexually and/or Graft-Compatible Citrus Relatives. Front. Plant Sci. 11, 617664. doi: 10.3389/fpls.2020.617664
Alves, M. N., Raiol-Junior, L. L., Girardi, E. A., Miranda, M., Wulff, N. A., Carvalho, E. V., et al. (2022). Insight into resistance to ‘Candidatus Liberibacter asiaticus,’ associated with Huanglongbing, in Oceanian citrus genotypes. Front. Plant Sci. 13, 1009350. doi: 10.3389/fpls.2022.1009350
Basu, S., Sineva, E., Nguyen, L., Sikdar, N., Park, J. W., Sinev, M., et al. (2022). Host-derived chimeric peptides clear the causative bacteria and augment host innate immunity during infection: A case study of HLB in citrus and fire blight in apple. Front. Plant Sci. 13, 929478. doi: 10.3389/fpls.2022.929478
Bové, J. M. (2006). Huanglongbing: a destructive, newly-emerging, century-old disease of citrus. J. Plant Pathol. 88, 7–37.
Campos, M. L., De Souza, C. M., De Oliveira, K. B. S., Dias, S. C., Franco, O. L. (2018). The role of antimicrobial peptides in plant immunity. J. Exp. Bot. 69, 4997–5011. doi: 10.1093/jxb/ery294
Choi, J., Basu, S., Thompson, A., Otto, K., Sineva, E. V., Kunta, M., et al. (2023). A host-derived chimeric peptide protects citrus against Huanglongbing without threatening the native microbial community of the phyllosphere. J. Sustain. Agric. Environ. 2, 489–499. doi: 10.1002/sae2.12089
Clark, K., Franco, J. Y., Schwizer, S., Pang, Z., Hawara, E., Liebrand, T. W. H., et al. (2018). An effector from the Huanglongbing-associated pathogen targets citrus proteases. Nat. Commun. 9, 1718. doi: 10.1038/s41467-018-04140-9
Clark, K. J., Pang, Z., Trinh, J., Wang, N., Ma, W. (2020). Sec-delivered effector 1 (SDE1) of ‘Candidatus Liberibacter asiaticus’ Promotes citrus huanglongbing. Mol. Plant-Microbe Interact. 33, 1394–1404. doi: 10.1094/MPMI-05-20-0123-R
De Francesco, A., Lovelace, A. H., Shaw, D., Qiu, M., Wang, Y., Gurung, F., et al. (2022). Transcriptome profiling of ‘Candidatus Liberibacter asiaticus’ in citrus and psyllids. Phytopathology 112, 116–130. doi: 10.1094/PHYTO-08-21-0327-FI
Du, J., Wang, Q., Shi, H., Zhou, C., He, J., Wang, X. (2023). A prophage-encoded effector from “Candidatus Liberibacter asiaticus” targets ASCORBATE PEROXIDASE6 in citrus to facilitate bacterial infection. Mol. Plant Pathol. 24, 302–316. doi: 10.1111/mpp.13296
Du, M., Wang, S., Dong, L., Qu, R., Zheng, L., He, Y., et al. (2022). Overexpression of a “Candidatus Liberibacter asiaticus” Effector gene caLasSDE115 contributes to early colonization in citrus sinensis. Front. Microbiol. 12. doi: 10.3389/fmicb.2021.797841
Du, P., Hu, J., Du, M., Gao, X., Yang, W., Zhang, C., et al. (2024). Interaction of a bacterial non-classically secreted RNase HI with a citrus B-Box zinc finger protein delays flowering in Arabidopsis thaliana and suppresses the expression of FLOWERING LOCUS T. Microbiological Res. 278, 127541. doi: 10.1016/j.micres.2023.127541
Duan, Y., Zhou, L., Hall, D. G., Li, W., Doddapaneni, H., Lin, H., et al. (2009). Complete genome sequence of citrus Huanglongbing bacterium, ‘Candidatus Liberibacter asiaticus’ Obtained through metagenomics. Mol. Plant-Microbe Interact. 22, 1011–1020. doi: 10.1094/MPMI-22-8-1011
Giacomello, S. (2021). A new era for plant science: spatial single-cell transcriptomics. Curr. Opin. Plant Biol. 60, 102041. doi: 10.1016/j.pbi.2021.102041
Gottwald, T. R. (2010). Current epidemiological understanding of citrus Huanglongbing. Annu. Rev. Phytopathol. 48, 119–139. doi: 10.1146/annurev-phyto-073009-114418
Hall, D. G., Richardson, M. L., Ammar, E.-D., Halbert, S. E. (2013). Asian citrus psyllid, iaphorina citri, vector of citrus huanglongbing disease. Entomologia Experimentalis Applicata 146, 207–223. doi: 10.1111/eea.2012.146.issue-2
Huang, C.-Y., Araujo, K., Sánchez, J. N., Kund, G., Trumble, J., Roper, C., et al. (2021). A stable antimicrobial peptide with dual functions of treating and preventing citrus Huanglongbing. Proc. Natl. Acad. Sci. 118, e2019628118. doi: 10.1073/pnas.2019628118
Huang, G., Chang, X., Hu, Y., Li, F., Wang, N., Li, R. (2024a). SDE19, a SEC-dependent effector from ‘Candidatus Liberibacter asiaticus’ suppresses plant immunity and targets Citrus sinensis Sec12 to interfere with vesicle trafficking. PloS Pathog. 20, e1012542. doi: 10.1371/journal.ppat.1012542
Huang, G., Li, F., Hu, Y., Ouyang, Z., Li, R. (2024b). Comprehensive Analysis of Genes Associated with the Reactive Oxygen Species Metabolism in Citrus sinensis during Pathogen Infection. Horticulturae 10, 625. doi: 10.3390/horticulturae10060625
Jain, M., Cai, L., Black, I., Azadi, P., Carlson, R. W., Jones, K. M., et al. (2021). Candidatus Liberibacter asiaticus’-encoded BCP peroxiredoxin suppresses lipopolysaccharide-mediated defense signaling and nitrosative stress in planta. Mol. Plant-Microbe Interact. 35, 257–273. doi: 10.1094/MPMI-09-21-0230-R
Jain, M., Fleites, L. A., Gabriel, D. W. (2015). Prophage-encoded peroxidase in ‘Candidatus Liberibacter asiaticus’ Is a secreted effector that suppresses plant defenses. Mol. Plant-Microbe Interact. 28, 1330–1337. doi: 10.1094/MPMI-07-15-0145-R
Jain, M., Munoz-Bodnar, A., Gabriel, D. W. (2019). Candidatus Liberibacter asiaticus’ peroxiredoxin (LasBCP) suppresses oxylipin-mediated defense signaling in citrus. J. Plant Physiol. 236, 61–65. doi: 10.1016/j.jplph.2019.03.001
Jain, M., Munoz-Bodnar, A., Zhang, S., Gabriel, D. W. (2018). A secreted ‘Candidatus Liberibacter asiaticus’ Peroxiredoxin simultaneously suppresses both localized and systemic innate immune responses in planta. Mol. Plant-Microbe Interact. 31, 1312–1322. doi: 10.1094/MPMI-03-18-0068-R
Li, D., Liu, H., Zhang, H., Wang, X., Song, F. (2008). OsBIRH1, a DEAD-box RNA helicase with functions in modulating defence responses against pathogen infection and oxidative stress. J. Exp. Bot. 59, 2133–2146. doi: 10.1093/jxb/ern072
Li, H., Ying, X., Shang, L., Redfern, B., Kypraios, N., Xie, X., et al. (2020). Heterologous expression of CLIBASIA_03915/CLIBASIA_04250 by tobacco mosaic virus resulted in phloem necrosis in the senescent leaves of Nicotiana benthamiana. Int. J. Mol. Sci. 21, 1414. doi: 10.3390/ijms21041414
Li, X., Guo, Z., Zhou, Y., Zhang, B., Ruan, H., Chen, W. (2024). Three new discovery effector proteins from Candidatus Liberibacter asiaticus psy62 inhibit plant defense through interaction with AtCAT3 and AtGAPA. Plant Cell Rep. 43, 130. doi: 10.1007/s00299-024-03220-z
Liu, X., Fan, Y., Zhang, C., Dai, M., Wang, X., Li, W. (2019). Nuclear Import of a Secreted “Candidatus Liberibacter asiaticus” Protein is Temperature Dependent and Contributes to Pathogenicity in Nicotiana benthamiana. Front. Microbiol. 10. doi: 10.3389/fmicb.2019.01684
Ma, W. X., Pang, Z. Q., Huang, X. E., Xu, J., Pandey, S. S., Li, J., et al. (2022). Citrus Huanglongbing is a pathogen-triggered immune disease that can be mitigated with antioxidants and gibberellin. Nat. Commun. 13, 529. doi: 10.1038/s41467-022-28189-9
Misas-Villamil, J. C., van der Hoorn, R., Doehlemann, G. (2016). Papain-like cysteine proteases as hubs in plant immunity. New Phytol. 212, 902–907. doi: 10.1111/nph.14117
Pang, Z., Zhang, L., Coaker, G., Ma, W., He, S.-Y., Wang, N. (2020). Citrus csACD2 is a target of Candidatus Liberibacter asiaticus in Huanglongbing disease. Plant Physiol. 184, 792–805. doi: 10.1104/pp.20.00348
Peng, A., Zou, X., He, Y., Chen, S., Liu, X., Zhang, J., et al. (2021). Overexpressing a NPR1-like gene from Citrus paradisi enhanced Huanglongbing resistance in C. sinensis. Plant Cell Rep. 40, 529–541. doi: 10.1007/s00299-020-02648-3
Pitino, M., Allen, V., Duan, Y. (2018). LasΔ5315 Effector Induces Extreme Starch Accumulation and Chlorosis as Ca. Liberibacter asiaticus Infection in Nicotiana benthamiana. Front. Plant Sci. 9, 113. doi: 10.3389/fpls.2018.00113
Prasad, S., Xu, J., Zhang, Y., Wang, N. (2016). SEC-translocon dependent extracytoplasmic proteins of Candidatus Liberibacter asiaticus. Front. Microbiol. 7. doi: 10.3389/fmicb.2016.01989
Qiu, W., Soares, J., Pang, Z., Huang, Y., Sun, Z., Wang, N., et al. (2020). Potential mechanisms of atNPR1 mediated resistance against huanglongbing (HLB) in citrus. Int. J. Mol. Sci. 21, 2009. doi: 10.3390/ijms21062009
Shan, L., He, P., Li, J., Heese, A., Peck, S. C., Nürnberger, T., et al. (2008). Bacterial effectors target the common signaling partner BAK1 to disrupt multiple MAMP receptor-signaling complexes and impede plant immunity. Cell Host Microbe 4, 17–27. doi: 10.1016/j.chom.2008.05.017
Shen, P., Li, X., Fu, S., Zhou, C., Wang, X. (2022). A “Candidatus Liberibacter asiaticus”-secreted polypeptide suppresses plant immune responses in Nicotiana benthamiana and Citrus sinensis. Front. Plant Sci. 13. doi: 10.3389/fpls.2022.997825
Shi, J., Gong, Y., Shi, H., Ma, X., Zhu, Y., Yang, F., et al. (2023b). Candidatus Liberibacter asiaticus’ secretory protein SDE3 inhibits host autophagy to promote Huanglongbing disease in citrus. Autophagy 19, 2558–2574. doi: 10.1080/15548627.2023.2213040
Shi, H., Yang, Z., Huang, J., Wu, H., Fu, S., Li, W., et al. (2023a). An effector of ‘Candidatus Liberibacter asiaticus’ manipulates autophagy to promote bacterial infection. J. Exp. Bot. 74, 4670–4684. doi: 10.1093/jxb/erad176
Sivager, G., Calvez, L., Bruyere, S., Boisne-Noc, R., Brat, P., Gros, O., et al. (2021). Specific physiological and anatomical traits associated with polyploidy and better detoxification processes contribute to improved huanglongbing tolerance of the persian lime compared with the Mexican lime. Front. Plant Sci. 12. doi: 10.3389/fpls.2021.685679
Soares, J. M., Weber, K. C., Qiu, W., Mahmoud, L. M., Grosser, J. W., Dutt, M. (2022). Overexpression of the salicylic acid binding protein 2 (SABP2) from tobacco enhances tolerance against Huanglongbing in transgenic citrus. Plant Cell Rep. 41, 2305–2320. doi: 10.1007/s00299-022-02922-6
Su, H., Wang, Y., Xu, J., Omar, A. A., Grosser, J. W., Calovic, M., et al. (2023). Generation of the transgene-free canker-resistant Citrus sinensis using Cas12a/crRNA ribonucleoprotein in the T0 generation. Nat. Commun. 14, 3957. doi: 10.1038/s41467-023-39714-9
Sugio, A., Maclean, A. M., Kingdom, H. N., Grieve, V. M., Manimekalai, R., Hogenhout, S. A. (2011). Diverse targets of phytoplasma effectors: from plant development to defense against insects. Annu. Rev. Phytopathol. 49, 175–195. doi: 10.1146/annurev-phyto-072910-095323
Thapa, S. P., De Francesco, A., Trinh, J., Gurung, F. B., Pang, Z., Vidalakis, G., et al. (2020). Genome-wide analyses of Liberibacter species provides insights into evolution, phylogenetic relationships, and virulence factors. Mol. Plant Pathol. 21, 716–731. doi: 10.1111/mpp.12925
Tomkins, M., Kliot, A., Marée, A. F. M., Hogenhout, S. A. (2018). A multi-layered mechanistic modelling approach to understand how effector genes extend beyond phytoplasma to modulate plant hosts, insect vectors and the environment. Curr. Opin. Plant Biol. 44, 39–48. doi: 10.1016/j.pbi.2018.02.002
Wang, N. (2019). The citrus huanglongbing crisis and potential solutions. Mol. Plant 12, 607–609. doi: 10.1016/j.molp.2019.03.008
Wang, S., Du, M., Dong, L., Qu, R., Ran, D., Ma, J., et al. (2023). Function and molecular mechanism analysis of CaLasSDE460 effector involved in the pathogenesis of “Candidatus Liberibacter asiaticus” in citrus. Mol. horticulture 3, 14. doi: 10.1186/s43897-023-00062-3
Wang, Y., Pruitt, R. N., Nürnberger, T., Wang, Y. (2022). Evasion of plant immunity by microbial pathogens. Nat. Rev. Microbiol. 20, 449–464. doi: 10.1038/s41579-022-00710-3
Wu, Q., Moniruzzaman, M., Yan, H., Lv, Y., Jiang, B., Jiang, N., et al. (2021). The CsNPR1 gene expression modulation in citrus and understanding the defense mechanism against Huanglongbing by screening CsNPR1-interacting proteins. Scientia Hortic. 288, 110375. doi: 10.1016/j.scienta.2021.110375
Xu, L., Mo, K., Ran, D., Ma, J., Zhang, L., Sun, Y., et al. (2023). An endolysin gene from Candidatus Liberibacter asiaticus confers dual resistance to huanglongbing and citrus canker. Horticulture Res. 10, uhad159. doi: 10.1093/hr/uhad159
Zavaliev, R., Dong, X. (2024). NPR1, a key immune regulator for plant survival under biotic and abiotic stresses. Mol. Cell 84, 131–141. doi: 10.1016/j.molcel.2023.11.018
Zhang, C., Du, P., Yan, H., Zhu, Z., Wang, X., Li, W. (2020). A sec-dependent secretory protein of the huanglongbing-associated pathogen suppresses hypersensitive cell death in Nicotiana benthamiana. Front. Microbiol. 11. doi: 10.3389/fmicb.2020.594669
Zhang, S., Wang, X., He, J., Zhang, S., Zhao, T., Fu, S., et al. (2023). A Sec-dependent effector, CLIBASIA_04425, contributes to virulence in ‘Candidatus Liberibater asiaticus’. Front. Plant Sci. 14. doi: 10.3389/fpls.2023.1224736
Zhang, C., Wang, X., Liu, X., Fan, Y., Zhang, Y., Zhou, X., et al. (2019). A Novel ‘Candidatus Liberibacter asiaticus’-Encoded Sec-Dependent Secretory Protein Suppresses Programmed Cell Death in Nicotiana benthamiana. Int. J. Mol. Sci. 20, 5802. doi: 10.3390/ijms20225802
Zhang, S., Wang, X., Zhao, T., Zhou, C. (2024). Effector Las0185 targets methionine sulphoxide reductase B1 of Citrus sinensis to promote multiplication of ‘Candidatus Liberibacter asiaticus’ via enhancing enzymatic activity of ascorbate peroxidase 1. Mol. Plant Pathol. 25, e70002. doi: 10.1111/mpp.70002
Zheng, Y., Li, J., Zheng, M., Li, Y., Deng, X., Zheng, Z. (2024). Whole genome sequences of 135 “Candidatus Liberibacter asiaticus” strains from China. Sci. Data 11, 1018. doi: 10.1038/s41597-024-03855-3
Zhou, C. (2020). The status of citrus Huanglongbing in China. Trop. Plant Pathol. 45, 279–284. doi: 10.1007/s40858-020-00363-8
Zhou, Y., Wei, X., Li, Y., Liu, Z., Duan, Y., Zou, H. (2020). Candidatus Liberibacter asiaticus’ SDE1 effector induces Huanglongbing chlorosis by downregulating host DDX3 gene. Int. J. Mol. Sci. 21, 7996. doi: 10.3390/ijms21217996
Zou, X., Zhao, K., Liu, Y., Du, M., Zheng, L., Wang, S., et al. (2021). Overexpression of salicylic acid carboxyl methyltransferase (CsSAMT1) enhances tolerance to Huanglongbing disease in Wanjincheng orange (Citrus sinensis (L.) osbeck). Int. J. Mol. Sci. 22, 2803. doi: 10.3390/ijms22062803
Keywords: Citrus sinensis, Candidatus Liberibacter asiaticus, Sec-dependent effectors, interaction, virulence
Citation: Hu Y, Lu N, Bao K, Liu S, Li R and Huang G (2025) Swords and shields: the war between Candidatus Liberibacter asiaticus and citrus. Front. Plant Sci. 15:1518880. doi: 10.3389/fpls.2024.1518880
Received: 29 October 2024; Accepted: 12 December 2024;
Published: 07 January 2025.
Edited by:
Eduardo Augusto Girardi, Brazilian Agricultural Research Corporation (EMBRAPA), BrazilReviewed by:
Eduardo Andrade, Brazilian Agricultural Research Corporation (EMBRAPA), BrazilCopyright © 2025 Hu, Lu, Bao, Liu, Li and Huang. This is an open-access article distributed under the terms of the Creative Commons Attribution License (CC BY). The use, distribution or reproduction in other forums is permitted, provided the original author(s) and the copyright owner(s) are credited and that the original publication in this journal is cited, in accordance with accepted academic practice. No use, distribution or reproduction is permitted which does not comply with these terms.
*Correspondence: Ruimin Li, bGlydWltaW5AZ25udS5lZHUuY24=; Guiyan Huang, aHVhbmdndWl5YW5AZ25udS5lZHUuY24=
Disclaimer: All claims expressed in this article are solely those of the authors and do not necessarily represent those of their affiliated organizations, or those of the publisher, the editors and the reviewers. Any product that may be evaluated in this article or claim that may be made by its manufacturer is not guaranteed or endorsed by the publisher.
Research integrity at Frontiers
Learn more about the work of our research integrity team to safeguard the quality of each article we publish.