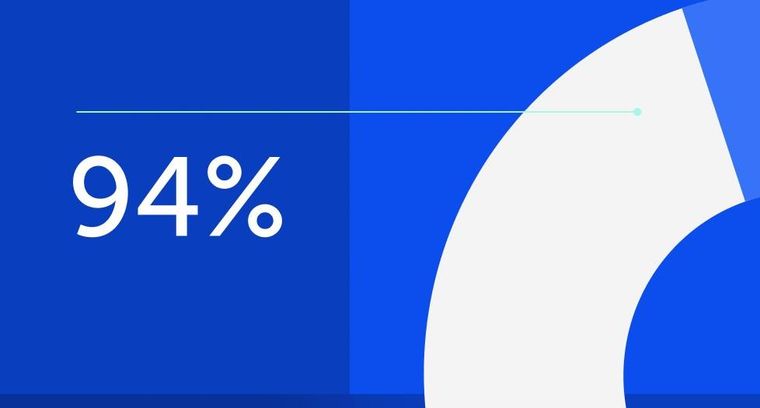
94% of researchers rate our articles as excellent or good
Learn more about the work of our research integrity team to safeguard the quality of each article we publish.
Find out more
ORIGINAL RESEARCH article
Front. Plant Sci., 27 January 2025
Sec. Plant Symbiotic Interactions
Volume 15 - 2024 | https://doi.org/10.3389/fpls.2024.1504650
This article is part of the Research TopicPlant-microbes Interactions and Resistance Against Abiotic StressView all 14 articles
Background and Aims: Arbuscular mycorrhizal fungi (AMF) and dark septate endophytes (DSE) are known to enhance the tolerance of host plants to biotic and abiotic stresses, but the mechanism of their interaction under natural conditions has not been extensively studied.
Methods: We analyzed the endophytic fungal diversity and colonization characteristics in the typical desert plants Populus euphratica and Haloxylon ammodendron and the relationship between them and environmental factors.
Results: Except for DSE in the roots of H. ammodendron, the colonization rates of AMF and DSE were significantly positively correlated with drought severity. The abundance of AMF and DSE under medium and mild drought conditions was greater than that under severe drought conditions. The root colonization rate and abundance of AMF were lower than those of DSE under the same drought conditions. The species diversity and abundance of AMF and DSE in P. euphratica were greater than those in H. ammodendron. AMF were more susceptible to soil factors such as soil water content, soil nitrogen and phosphorus content, and urease, whereas DSE were more affected by pH.
Conclusion: Drought stress has different effects on AMF and DSE in the roots of P. euphratica and H. ammodendron. DSE have a greater advantage in extremely arid environments. This study demonstrates the interaction between AMF and DSE with the host plants P. euphratica and H. ammodendron as well as their effects on the adaptation of host plants to the desert environment, which can provide a basis for strengthening desert vegetation management.
In natural ecosystems, most plants coexist with endophytic fungi, which can effectively promote plant growth and adaptation to stress (Rodriguez et al., 2009). During the long-term succession and evolution of arid desert plants, a series of special strategies for survival in arid environments have developed (Huo et al., 2022). The association between plants and endophytic fungi has also been recognized as a key strategy for desert plants to adapt to stressful environments (Liu et al., 2023), that is, endophytic fungi play an important role in host resistance to drought and other stresses.
Arbuscular mycorrhizal fungi (AMF) are widely distributed soil microorganisms that can form symbioses with a variety of plants (Gai et al., 2006), promote the growth of other soil microorganisms (Sun and Shahrajabian, 2023), transport mineral elements and water to the host plant, and improve the overall stress resistance of plants (Tariq et al., 2023), enabling plants to better survive in desert environments with scarce water and nutrient deficiency (Madouh and Quoreshi, 2023). Therefore, AMF play an important role in desert vegetation and ecological restoration (Alrajhi et al., 2024). As symbionts, dark septate endophytes (DSE) can enhance the absorption of nutrients—such as nitrate, phosphorus, and micronutrients—by plants (Farias et al., 2020; Wang et al., 2023), so that more nutrients are available to the host plants (Mandyam and Jumpponen, 2008). Moreover, the DSE are able to form the “microsclerotia” structure (Madouh and Quoreshi, 2023). Under adverse conditions, DSE increase host plants tolerance to biotic and abiotic stresses by activating physiological and biochemical responses (Ban et al., 2017). Their wide host range, especially its distribution in extreme environments, makes DSE no less ecologically significant than AMF and has potential applications value in ecological conservation and vegetation restoration and more (Malicka et al., 2022). Under natural conditions, AMF and DSE often co-infect plant roots, and their biological characteristics and ecological functions can play a positive role in ecological reconstruction and vegetation restoration after damage (Huo et al., 2021; Xie et al., 2024).
In arid and semiarid zones, changes in the colonization of plant roots by endophytes are mainly caused by changes in soil moisture (Ayala et al., 2023). P. euphratica and H. ammodendron are, respectively, typical arboreal and shrubby species in oases of arid regions (Tan et al., 2024). P. euphratica can regulate climate, prevent sandstorms and desert expansion, and protect oases (Yao et al., 2024). H. ammodendron can reduce wind speed, improve forest microclimate, and promote the settlement and growth of other desert plants (Yang and Lv, 2023). Therefore, they play an important role in maintaining the structure and function of the ecosystem. P. euphratica and H. ammodendron have formed stable colonization structures with AMF and DSE (Dang et al., 2022; Li et al., 2022). Moreover, the two plants have been extensively studied in previous studies, but there is a lack of comprehensive understanding of the colonization patterns of AMF and DSE fungi that colonize simultaneously in the roots of P. euphratica and H. ammodendron. Based on this, this study considers P. euphratica and H. ammodendron in the Ebinur Lake Basin as research objects to study the diversity of endophytic fungi, colonization status of the plant root system, and the relationship between colonization status and environmental factors under different moisture conditions. The following questions were raised: (1) What are the differences in the colonization rates and morphological structures of AMF and DSE under different drought conditions? Is there an interaction between these two mycorrhizal fungi, such as competition or mutualistic symbiosis? (2) What are the differences in AMF and DSE species compositions in rhizosphere and roots under different drought conditions? (3) What are the main influencing soil physical and chemical factors causing differences in AMF and DSE colonization across drought gradients?
Located in Xinjiang Uygur Autonomous Region in northwestern China, Ebinur Lake Wetland National Nature Reserve (EWNR) is the lowest depression and water-salt aggregation center of Ebinur Lake Wetland on the western edge of the Gurbantunggut Desert (44°30′-45°09′N, 82°36′-83°50′E), with a typical desert ecosystem. The annual maximum temperature is 44°C and the minimum temperature is -34°C. The average annual precipitation is less than 100 mm, whereas the annual evaporation is 16 times the average annual precipitation (Li et al., 2023). Ebinur Lake is the largest saltwater lake in Xinjiang. The soils in this basin are mostly grey desert soil, grey brown desert soil, and aeolian sandy soil with severe salinization (Chen et al., 2023). The dominant plant communities are the salt and drought stress tolerant plants such as P. euphratica, H. ammodendron, and Tamarix chinensis (Wang et al., 2022).
In May 2023, a sample transect was established north of the Dongdaqiao Management Station within the typical arid desert reserve in the Ebinur Lake Basin, perpendicular to the bank of the Aqikesu River for approximately 500 m, towards the Kumtag Desert. Based on different natural drought conditions, we selected high, medium, and low moisture gradients from near to far at intervals of 1,200 m from the riverbank (representing mild, medium, and severe droughts, respectively), labelled W1, W2, and W3 from high to low moisture. All three sampling points are located in areas with gentle terrain. The main vegetation includes P. euphratica, H. ammodendron and other herbaceous plants such as Ceratocarpus arenarius and Nitraria tangutorum. From a high to a low moisture gradient, the height, crown width, and abundance of plants decrease. Three repeated plots were taken for each moisture gradient, and three P. euphratica and three H. ammodendron plants with similar growth conditions were selected as replicates from east to west in each plot, totaling nine plots, and 54 samples were collected.
Root samples were collected from the concentrated distribution area of fine roots within the 0-20 cm soil layer around the trunk of the tree (Xia et al., 2015), divided, labelled, collected in sterile bags, and immediately sealed and refrigerated. Among the 27 trees, two root samples were taken from each plant for a total of 54 fine root samples, of which 27 root samples were stored at -20°C for the determination of mycelial colonization rate, and the remaining 27 samples were stored at -80°C for DNA extraction. Due to scarce precipitation, local soil water and groundwater are mainly recharged by rivers, and the depth of groundwater decreases with distance from the Aqikesu River, resulting in significant changes in the soil water content gradient (Yang et al., 2022). We measured the water content of the 0-20 cm soil layer in the subsurface. The results showed that in May, the water content of W1, W2, and W3 was 16.54% ± 2.27%, 8.43% ± 1.61%, and 4.12% ± 1.54%, respectively.
The samples were taken to the laboratory, and the surface soil adhering to the roots was shaken off. The root samples were transferred to a sterile 50 ml centrifuge tubes, 20 ml of sterilized 10 mM PBS solution was added, the shaker speed was set to 120 rpm, and the centrifuge tubes were placed at room temperature for 20 min. Sterile tweezers were used to remove the root system, the centrifuge speed was set to 6,000 × g, and the remaining suspension was centrifuged at 4°C for 20 minutes. The precipitate obtained after centrifugation was the required rhizosphere soil (He et al., 2021). There were eighteen samples per moisture gradient, for a total of fifty-four soil samples. The shaken soil was then placed in numbered ziplock bags at room temperature.
The soil was collected in an empty aluminum box that had been weighed beforehand, weighed in the field, brought back to the laboratory, dried. The dry soil was weighed to calculate the soil moisture content (SWC). The shaken soil was dried naturally and then sieved for analysis of soil physical and chemical properties, including soil organic carbon (SOC), electrical conductivity (EC), soil pH (pH), total phosphorus (TP), total nitrogen (TN), available phosphorus (AP), ammonium nitrogen (NH4-N), nitrate nitrogen (NO3-N), urease (UA), and alkaline phosphatase (ALP). The specific methods were as follows (Bao, 2000): organic carbon content was determined by high temperature exothermic potassium dichromate oxidizing capacity method; soil conductivity was measured by precision conductivity meter; pH value was measured by a pH meter in the solution with a water soil ratio of 2.5:1; soil total phosphorus content was determined by HClO4-H2SO4 Mo-Sb colorimetric method; the content of soil total nitrogen was determined by H2SO4 mixed accelerant digestion; and available nitrogen content was determined by NaHCO3 leaching Mo-Sb colorimetric method; KCl leaching-indophenol blue colorimetric method for the determination of soil ammonium nitrogen content; colorimetry method with phenol disulfonic acid for the determination of soil nitrate nitrogen content; phenol-sodium hypochlorite colorimetric method for the determination of soil urease content; and alkaline phosphatase content in soil was determined by phenol-sodium hypochlorite colorimetric method.
Sufficient fine roots were randomly selected from the root samples. The cells were stained using the Phillips and Hayman staining method (Phillips and Hayman, 1970). The structures of AMF and DSE were observed under a microscope (Nikon ECLIPSE Ti2, Japan), and photographs of the arbuscules, hyphae, vesicles of AMF and hyphae, and microsclerotia of DSE were obtained. The root sample observation method and mycorrhizal colonization grading criteria were used to calculate the root colonization rate (F%), colonization density (M%), and arbuscule abundance(A%) (Trouvelot et al., 1986). Microsclerotia colonization rate (MS%) was measured and calculated using the cross method under a 200x microscope (McGONIGLE et al., 1990). F% = number of infected root segments/total number of microscopic examination root segments* 100%. M% = (0.95 * N5 + 0.7 * N4 + 0.3 * N3 + 0.05 * N2 + 0.01 * N1)/total number of root segments examined by microscopy* 100%, where 0.95, 0.7… respectively represent the weight of each level. N5=sum of fifth-level root segments, N4, N3, N2, and N1 have the same meaning. A% = sum of the intersection points of arbuscule colonization/sum of cross colonization points* 100%. MS% = number of cross-colonization points/sum of cross-colonization points * 100%.
Fungal DNA extraction and detection were completed, followed by PCR amplification using ITS1F (CTTGGTCATTTAGAGGAAGTAA) and ITS2R (GCTGCGTTCTTCATCGATGC) primers (Gardes and Bruns, 1993; Lekberg et al., 2018; Wang et al., 2024), and the PCR products were analysed using QuantiFluor ™ - ST blue fluorescence quantitative system (Promega Corporation), followed by the construction of an Illumina library and Illumina sequencing. DNA extraction and sequencing were performed by Shanghai Majorbio Bio-Pharm Technology Co., Ltd. (Shanghai, China) to rapidly detect target strains. Then we used UPARSE platform version 7.1 to analyze the obtained gene sequences. To improve the accuracy and stability of the results, we clustered the data sequences and eliminated all chimeric sequences, ensuring that those with 97% similarity were grouped into the same operational taxonomic unit (OTU) to optimize the features of these datasets. An in-depth comparative analysis of the SILVA rRNA database (Release 138 https://www.arb-silva.de/) with the Unite eukaryotic ITS region database (Release 8.0 http://unite.ut.ee/index.php) was performed. The Majorbio cloud computing platform (http://cloud.Majorbio.com) was used to analyze the microbial communities in the soil. The initial data was stored in NCBI Sequence Read Archive database with the accession number PRJNA1046269. The data were initially organized and statistically analysed. By integrating the AMF and DSE taxonomic information and references, we created a species abundance table using the Majorbio platform to screen and download biodiversity information and facilitate subsequent data analysis.
SPSS 27 (IBM Corporation, Armonk, NY, USA) was used to perform normality and homogeneity of variance tests on the colonization rates of AMF and DSE, and the colonization rates under different water gradients were compared using multiple group comparisons (Kruskal-Wallis test). Bar charts were plotted using Excel to represent the status of the rhizosphere soil physical and chemical factors in P. euphratica and H. ammodendron. The ggcor package for R (version 4.3.1) was used to analyze the correlation between fungal colonization in rhizosphere and roots of P. euphratica and H. ammodendron and soil physical and chemical factors. Heat maps were drawn using the ggpubr, corrplot, and ggplot2 packages to visualize the correlation between the AMF and DSE colonization status. AMF and DSE with different gradients in rhizosphere and roots were classified and visualized by genus using circular and statnet packages for R. Redundancy analysis (RDA) between AMF and DSE colonization status and soil physical and chemical factors in the roots of the two plants was performed using CANOCO 5. Prior to RDA analysis, a species-sample analysis was done using CANOCO 5 to determine the choice of RDA analysis. The collinearity degree between soil physical and chemical factors was assessed by variance inflation factor (VIF) analysis using the R package (version 4.3.0) to ensure that the respective VIF values for all factors were less than 10.
A one-way ANOVA was performed to analyze the significant differences in the physical and chemical properties of the soil of P. euphratica (Figure 1). SWC decreased with the intensification of drought, and there was a significant difference among the three gradients. Both NH4-N and UA decreased with increasing drought severity, and there were significant differences (P<0.05) between the contents of the high and low moisture gradients. There was no significant difference between the medium moisture gradient and the other two gradients. The NO3-N, content in the high-moisture gradient was significantly higher than those in the medium- and low-moisture gradients (P<0.05). EC, pH, SOC, and ALP decreased as the moisture content decreased, but these changes were not significant. TN, TP, and AP decreased with increasing drought severity and the content in the high water gradient were significantly higher than those in the other two gradients (P>0.05).
Figure 1. (A–K) Differences in physical and chemical properties of soil of Populus euphratica (mean ± SD, n=3). Different lowercase letters marked above the bars indicate significant differences in the physical and chemical properties of plant soil (P<0.05).
The result of one-way ANOVA in the H. ammodendron soil (Figure 2) showed that the variation patterns among SWC, TN, TP, NH4-N, and AP were the same with significantly greater content under high moisture gradient than in the remaining two gradients (P<0.05). There was a significant difference in the UA between the high and low moisture gradients (P<0.05), whereas there was no significant difference between the medium moisture gradient and the other two gradients. There were no significant differences in EC, pH, SOC, NO3-N, or ALP among the three moisture gradients.
Figure 2. (A–K) Differences in physical and chemical properties of soil of Haloxylon ammodendron (mean ± SD, n=3). Same as Figure 1.
In summary, all physical and chemical factors in soils of the two plants were higher in the high-moisture gradient than in the other two gradients. All physical and chemical factors decreased with a reduction in soil water content, except for NO3-N in the rhizosphere soils of P. euphratica. TP, TN, and AP were significantly higher (P<0.05) in the high moisture gradient than in the medium and low moisture gradients, whereas there were no significant differences between the medium and low moisture gradients.
AMF and DSE colonization structures were observed in P. euphratica and H. ammodendron (Figures 3, 4). AMF and DSE were widely present in the roots of P. euphratica and H. ammodendron. AMF formed typical hyphae, vesicles, and arbuscule. The size and shape of vesicles varied, with both circular and elliptical shapes appearing. DSE formed dark septate hyphae and microsclerotia. The mycelia were mostly brown, and obvious mycelial meshes formed along the longitudinal axis of the roots. Microsclerotia were formed by closely packed and enlarged cells with thickened cell walls, with varying degrees of shape, size, and density. The color of the same microsclerotial cluster was brown with varying shades.
Figure 3. AMF colonization structure in roots of Populus euphratica (A-F) and Haloxylon ammodendron (G-L). A, arbuscule; H, hypha; V, vesicle.
Figure 4. DSE colonization structure in roots of Populus euphratica (A-F) and Haloxylon ammodendron (G-L). H, hypha; M, microsclerotia.
It can be seen that the AMF colonization rate increased significantly from 13.89% to 71.83% in the roots of H. ammodendron from high to low water gradients (Table 1). The arbuscule abundance of P. euphratica decreased with a decrease in soil moisture, whereas that of H. ammodendron increased with a decrease in soil moisture. From high to low water gradients, the DSE colonization rate increased significantly from 53.24% to 92.41% in the roots of P. euphratica, and decreased significantly from 67.10% to 45.06% in the roots of H. ammodendron (Table 2). Similar to P. euphratica, the DSE colonization rate and density of H. ammodendron in the medium water gradient were the lowest among the three gradients, and the number of microsclerotia was the highest among the three gradients. In most cases, both AMF and DSE had higher colonization rates under low water gradient conditions, indicating that a certain degree of drought promotes the formation of mycorrhizal structures between AMF, DSE and plant roots.
Table 1. Differences in AMF colonization rates in the roots of Populus euphratica and Haloxylon ammodendron under different water gradients.
Table 2. Differences in DSE colonization rates in the roots of Populus euphratica and Haloxylon ammodendron under different water gradients.
Under the same water gradient, the colonization status of AMF and DSE in P. euphratica and H. ammodendron differed. Under a high water gradient, the AMF colonization rate of P. euphratica was significantly higher than that of H. ammodendron. AMF were not detected in the roots of P. euphratica under a medium water gradient, but were detected in all water gradients of H. ammodendron. The DSE colonization rate of P. euphratica in the medium water gradient was significantly higher than that of H. ammodendron. Under a low water gradient, the DSE colonization rate, colonization density, and number of microsclerotia in the roots of P. euphratica were significantly higher than in H. ammodendron.
Different interactions were observed between DSE and AMF in the roots of P. euphratica and H. ammodendron (Figure 5). For example, in the roots of P. euphratica, AMF colonization (AM) was significantly positively correlated with the DSE colonization rate (DF) (R=0.74), and DF was significantly positively correlated with the colonization rate of AMF (AF) roots (R=0.72).
Figure 5. Correlation between DSE and AMF colonization status of (A) Populus euphratica, (B) Haloxylon ammodendron. MS, the microsclerotia colonization rate; AF, the colonization rate of AMF; AM, the colonization density of AMF; A, the richness of root hyphae; DF, the colonization rate of DSE; DM, the colonization density of DSE; Dm, the colonization density of DSE in the infected root segment. The asterisk indicates significance p-value. No mark when P≥0.05; when 0.01<P<0.05, marked * ; when 0.001<P<0.01, marked ** ; when P≤0.001, marked ***.
Among all the 27 samples from the rhizosphere soil and roots of P. euphratica, 18 samples were detected in seven genera of AMF, with samples belonging to high and low water gradients in the rhizosphere and roots (Figure 6). More than one AMF genus coexisted in each of the 18 samples. At low water gradients, the dominant AMF genera from the rhizosphere to the root interior changed from Kamienskia to unclassified_f__glomerraceae. Under high water gradients, the dominant genus changed from unclassified_p__Glomeromycota in the rhizosphere to unclassified_f__Glomerraceae in the roots. Overall, Kamienskia had the highest abundance, followed by unclassified_f__Glomerraceae.
Figure 6. Differences in AMF diversity between Haloxylon ammodendron and Populus euphratica under different water gradients (A) Haloxylon ammodendron, (B) Populus euphratica. J, the sample taken from the rhizosphere; N, the sample taken from the plant roots.
Among the 27 samples in the rhizosphere soil and within the roots of H. ammodendron, 18 samples were detected in three genera of AMF, belonging to high water gradients in the rhizosphere and high and low water gradients in the roots, all of which had AMF coexisting in one or more genera. Only two types of AMF were detected in the roots of H. ammodendron under a high water gradient, with unclassified_p__Glomeromycota being the dominant genus. Under a low water gradient, the dominant genus in the rhizosphere was Kamienskia, and the dominant genus in the root was unclassified_p__Glomeromycota.
The diversity of AMF species inside and outside the roots of P. euphratica was greater than that in H. ammodendron. Only three of the seven AMF genera were detected inside and outside the roots of H. ammodendron, while P. euphratica detected all seven genera including unclassified_o__Glomerales, Rhizophagus, Glomus, and Dominikia, with a total number greater than that of H. ammodendron. Among all AMF-detected samples, the dominant genus was unclassified_f__Glomerraceae. The AMF in the rhizosphere soil of P. euphratica was approximately six times the amount of H. ammodendron, whereas the amount of AMF in H. ammodendron is 2 times more than that of P. euphratica.
In total, 25 DSE genera were detected in the rhizosphere soil and roots of P. euphratica, and more than one DSE genus coexisted in all 27 samples (Supplementary Table S2). The dominant genera were Cyphellophora, followed by Fusarium (Figure 7). The composition of DSE genera of P. euphratica varied among the different water gradients. Cyphellophora and Fusarium have been widely detected in low water gradients. However, Cadophora, the dominant genus in the high-water gradients were rarely detected in the low water gradients.
Figure 7. Differences in TOP 10 DSE genera diversity between Haloxylon ammodendron and Populus euphratica under different water gradients (A) Populus euphratica; (B) Haloxylon ammodendron. Same as Figure 6. J, the sample taken from the rhizosphere; N, the sample taken from the plant roots.
In total, 25 DSE genera were detected in the rhizosphere soil and roots of H. ammodendron, and more than one DSE genus coexisted in all 27 samples. The three most abundant genera were Neocamrosporium, Cyphellophora, and Preussia. Neochamrosporium was widely distributed in high water gradients in roots, whereas Scytalidium was mainly detected in the low water gradient rhizosphere soil. Other genera of DSE were detected in samples from multiple water gradients. The abundance of DSE in the roots of each water gradient was lower than that in the rhizosphere. But the quantities of DSE in the rhizosphere were greater than that in the rhizosphere.
The TOP 10 DSE genera inside and outside the roots of the two plant species were also different: Scytalidium and Microascus were not dominant in the roots of P. euphratica, and Cadophora, Phialophora, and Embellisia were not dominant in the collected samples of H. ammodendron roots.
A redundancy analysis was conducted on the relationship between AMF and DSE colonization status of the roots of P. euphratica and H. ammodendron and soil physical and chemical factors at the plots. The results showed that the eigenvalues of the first and second sorting axes were 0.5842 and 0.1294, respectively. The two axes explained 71.36% of the changes in endophytic fungi, indicating that the first and second sorting axes could better reflect the changes between endophytic fungi and soil physical and chemical factors (Figure 8). The first axis was positively correlated with soil physical and chemical factors TP, negatively correlated with other soil physical and chemical factors, such as pH, AP, NO3-N, ALP, UA, SOC, SWC, NH4-N, TN and EC. It was positively correlated with AA, AM, AF, DF, and DM and negatively correlated with MS. The second axis was negatively correlated with MS, DF, DM, and all environmental factors, and positively correlated with AM, AF, and AA.
Figure 8. Redundancy analysis of environmental factors and colonization status of Populus euphratica and Haloxylon ammodendron. AM, the colonization density of AMF; A, the richness of root hyphae; DF, the colonization rate of DSE; DM, the colonization density of DSE; MS, the microsclerotia colonization rate. S, Haloxylon ammodendron; H, Populus euphratica; the number represents the moisture gradient and sampling number, for example, S11, the No.1 Haloxylon ammodendron sample collected at a high moisture gradient.
The results indicated (Supplementary Table S1) that SWC, AP, TP, and TN were significantly and positively correlated with AM (P<0.01). UA levels were significantly positively correlated with AM (P<0.01), and significantly negatively correlated with AF (P<0.05). ALP levels were significantly positively correlated with AM (P<0.01). There was a significant negative correlation between NH4-N and AF (P<0.05). There was a highly significant negative correlation between NO3-N and AF (P<0.01) and a significant positive correlation with DM (P<0.05). PH was significantly and positively correlated with DF (P<0.05) and DM (P<0.05).
Soil moisture can directly affect the growth and development of mycorrhizal fungi and the formation of mycorrhizal symbioses (Liu et al., 2018). Under low water gradient, for example, endophytic fungi can more effectively connect the roots of H. ammodendron to the surrounding soil microenvironment, thus exhibiting more significant functions in absorbing and transporting water and nutrients. In the present study, the DSE colonization rate of H. ammodendron decreased with decreasing water content. We speculate that this may be due to the drought stress exceeding a certain limit, which may have resulted in the production of certain substances by fungal cells (Pegler and Allen, 1993), leading to changes in the cell membrane permeability of H. ammodendron (Dick et al., 1996) and affecting the growth of fungi and plants. The colonization rate of AMF in the roots of P. euphratica and H. ammodendron was negatively correlated with soil water content, consistent with previous findings (Shen and Zhu, 2021). It has been speculated that appropriate level of humidity is beneficial for AMF colonization and spore germination (Frew, 2023). In this study, it was also found that under the same drought stress conditions, host plant species have an impact on the biomass and colonization rate of mycorrhizal fungi (Ilyas et al., 2024). For example, in contrast to the changes in the DSE colonization rate of the roots of H. ammodendron mentioned earlier, the DSE colonization rate of P. euphratica was negatively correlated with soil moisture content. This may be because the soil moisture content of the W1 plot in this study was more suitable for DSE colonization of the roots of P. euphratica. Under these conditions, DSE can have a relatively tight colonization of the roots of H. ammodendron (Ge et al., 2018). In our study, we also found that the total colonization rate of DSE under a low water gradient was higher than that of AMF, which not only indicates that DSE has a stronger colonization ability than AMF, but also indicates that DSE has greater colonization advantages under extreme environments (Alberton et al., 2010; Postma et al., 2007). The changes in the colonization structure that occurred in AMF and DSE with increasing drought severity may be an adaptation of endophytic fungi to environmental influences (Xie et al., 2017).
Zhao et al. (2021) found a positive correlation between the colonization rate of DSE microsclerotia and the content of AMF groups, which is consistent with the results of this study. In the present study, we also found a correlation between DSE colonization and AMF in the roots of the two plants. The colonization rate and density of AMF in P. euphratica were significantly positively correlated with the colonization rate of DSE, whereas the colonization rate and density of AMF were negatively correlated with the DSE colonization rate and density in H. ammodendron. This indicates that AMF and DSE can form different colonization relationships in the roots of different species, and that the interaction between the two mycorrhizal fungi is complex and greatly influenced by the host plant and environmental conditions. This is consistent with previous research results. The study of Shang et al. (2021) study showed that the colonization of AMF and DSE in the roots of cultivated Vaccinium uliginosum in Guizhou is highly significantly negatively correlated. Seerangan and Thangavelu, (2014) found that there was no clear relationship between AMF colonization and DSE colonization when studying plants growing in aquatic and wetland habitats, and the co-occurrence of AMF and DSE is more common in terrestrial habitats.
In this study, we found that the majority of AMF and DSE were not unique to P. euphratica or H. ammodendron but were shared by both, indicating that AMF and DSE strains do not exhibit strong host specificity in arid areas (Liu et al., 2017; Bi and Xie, 2021). For example, unclassified_f:Glomeraceae, an unclassified member of the Glomeraceae family, was found in the roots of both H. ammodendron and P. euphratica. Glomeraceae is a widely distributed community in the AMF population currently discovered, and was found in both soil and plant roots (Stürmer et al., 2018). Unlike AMF, DSE exhibits host preference (Stroheker et al., 2021). For example, Cyphellophora, the dominant genus of DSE, had a significantly greater amount of colonization in the rhizosphere soil and roots of P. euphratica than H. ammodendron, whereas another genus, Neocamrosporium, had a higher number in the rhizosphere soil and roots of H. ammodendron, which also reflects the varying degrees of host preference for different mycorrhizal fungi (Joshee et al., 2009). Under the same drought conditions, there was a significant difference in the colonization status of AMF between the roots and rhizospheres of P. euphratica. The diversity and quantity of AMF in the rhizosphere soil were higher than in the roots, which is consistent with the results of Tian et al (2018). on AMF in the rhizosphere of potato roots. Differences in AMF communities between the soil and roots may be determined by the heterogeneity of the rhizosphere soil (Liu et al., 2009). Shi et al. (2022) and Wang et al. (2010) conducted a diversity survey of AMF in the Xinjiang region and found that Glomus was the dominant genus. In this study, Rhizophagus and Kamienskia were more dominant than Glomus, which may be due to differences in hosts.
Many of the AMF and DSE genera detected in this study are highly beneficial to the host. For example, Glomus genera are used for Se biofortification (Ye et al., 2020). The Phialocephala genus can promote the biological adaptability of Saussurea involucrata and Rhodiola rosea to extremely cold and strong radiation environments (Chen et al., 2018), and improve the gold tolerance properties of host plants. Thielavia in the soil provides plants with highly active endoglucanases, increases the accumulation of plant glucose, and promotes the absorption of soil nutrients by plants, thereby increasing the yields of Medicago sativa (Medicago sativa Linn) and Zea mays (Badhan et al., 2014; Shi et al., 2019). These findings demonstrated that endophytic fungi play an important role in improving plant adaptability to the environment.
Studies have shown that the distribution of endophytic fungi that colonize plant roots is related to soil physical and chemical factors (Wang et al., 2015). Both redundancy and correlation analyses in this study indicated that soil physical and chemical factors have different effects on fungal colonization within roots. Nitrogen deposition is one of the most important global change factors (Tang et al., 2023), and nitrogen content plays a role in AMF colonization. When the distribution ratio of nitrogen, phosphorus, and potassium in the soil is unbalanced, the infectivity of AMF is improved, helping plant roots absorb more nutrients from the soil to cope with adversity (Zhou et al., 2021). Increasing nitrogen fertilizer application can promote the proliferation of bacteria and actinomycetes in the rhizosphere soil of Oryza sativa, and the nitrogen application level is positively correlated with the number of bacteria and actinomycetes. TP and ALP were highly significantly positively correlated with AMF colonization density, which is consistent with the results of Li et al. (2021). Soil phosphatase can promote the hydrolysis of organic phosphorus compounds into inorganic phosphorus, thereby increasing the available phosphorus content in the soil (Luo et al., 2022). However, under high phosphorus levels, the colonization rate of AMF significantly decreases (Salmeron-Santiago et al., 2023), which is not conducive to plant growth. Therefore, controlling and managing soil phosphorus levels are beneficial for improving the richness and diversity of soil AMF, thereby facilitating the establishment of sustainable ecosystems (Qin et al., 2020). It is obvious that the soil moisture content was not the reason for the lack of AMF colonization in the roots of P. euphratica under a moderate water gradient, and the soil moisture content did not cause AMF colonization in the other two gradients. We speculate that this may be due to the aforementioned soil physical and chemical factors (Yan et al., 2023). Under nitrate-nitrogen conditions, endophytic fungal inoculation can promote seedling growth (Sun et al., 2022). Nitrate-nitrogen can reflect soil nitrogen levels, and nitrogen can accelerate the growth metabolism of soil microorganisms (Qu et al., 2022), strongly supporting the results of our study. In this study, there was a significant positive correlation between pH and the colonization status of the DSE communities, which is consistent with previous studies. Hou (2020) found that the colonization rate of DSE was positively correlated with pH in his research on various desert plants. This may be because the pH affects the soluble salt content in the soil and the material exchange capacity of DSE, thus affecting its growth status of DSE (Zhang et al., 2015).
Our study investigated the effects of soil factors on the colonization of AMF and DSE in the rhizosphere and roots of the desert plants P. euphratica and H. ammodendron in Xinjiang. This was achieved by observing their colonization status, community composition, morphological characteristics, and differences in morphological colonization structure. The results showed that drought stress increased the colonization rate of DSE in the roots of P. euphratica and AMF in the roots of P. euphratica and H. ammodendron but decreased the colonization rate of DSE in the roots of H. ammodendron. The DSE was more dominant in extreme drought environments. The AMF diversity decreased with increasing drought severity, whereas the DSE diversity increased. DSE showed stronger adaptability to drought-stressed environments. The dominant and endemic species of DSE vary in different environments and hosts. AMF were more susceptible to soil factors such as SWC, soil nitrogen and phosphorus content (including AP, TN, TP, NH4-N, and NO3-N), and UA, whereas DSE was more affected by pH. The results of this study are conducive to the development of targeted and biased protection strategies for desert plants, especially P. euphratica and H. ammodendron, and provide a basis for endophytic fungal species selection in ecological restoration.
The datasets presented in this study can be found in online repositories. The names of the repository/repositories and accession number(s) can be found below: https://www.ncbi.nlm.nih.gov/, PRJNA1046269.
HMW: Writing – original draft, Data curation, Visualization. HFW: Writing – review & editing, Conceptualization, Funding acquisition, Methodology, Visualization. SW: Data curation, Visualization, Investigation, Validation, Writing – original draft. LS: Data curation, Investigation, Supervision, Visualization, Writing – original draft. LC: Data curation, Writing – original draft.
The author(s) declare financial support was received for the research, authorship, and/or publication of this article. This research was supported by the National Natural Science Foundation of China (32360282) and the Natural Science Foundation of the Xinjiang Uyghur Autonomous Region (2022D01C042).
Thanks to Wenjing Li, Deyan Wu for their guidance on the experiment, and to Zhengxian Mo and Hao Huang for their help in sample collection and laboratory analysis.
The authors declare that the research was conducted in the absence of any commercial or financial relationships that could be construed as a potential conflict of interest.
The author(s) declare that no Generative AI was used in the creation of this manuscript.
All claims expressed in this article are solely those of the authors and do not necessarily represent those of their affiliated organizations, or those of the publisher, the editors and the reviewers. Any product that may be evaluated in this article, or claim that may be made by its manufacturer, is not guaranteed or endorsed by the publisher.
The Supplementary Material for this article can be found online at: https://www.frontiersin.org/articles/10.3389/fpls.2024.1504650/full#supplementary-material
Alberton, O., Kuyper, T. W., Summerbell, R. C. (2010). Dark septate root endophytic fungi increase growth of Scots pine seedlings under elevated CO2 through enhanced nitrogen use efficiency. Plant Soil 328, 459–470. doi: 10.1007/s11104-009-0125-8
Alrajhi, K., Bibi, S., Abu-Dieyeh, M. (2024). Diversity, Distribution, and applications of arbuscular mycorrhizal fungi in the Arabian Peninsula. Saudi J. Biol. Sci. 31, 103911. doi: 10.1016/j.sjbs.2023.103911
Ayala, S., Thomas, V., Nelson, G., Soti, P. (2023). Soil conditions regulate the patterns of root colonization by fungal endophytes in Guinea grass (Megathyrsus maximus). Symbiosis 91, 101–105. doi: 10.1007/s13199-023-00944-6
Badhan, A., Wang, Y., Gruninger, R., Patton, D., Powlowski, J., Tsang, A., et al. (2014). Formulation of enzyme blends to maximize the hydrolysis of alkaline peroxide pretreated alfalfa hay and barley straw by rumen enzymes and commercial cellulases. BMC Biotechnol. 14, 31. doi: 10.1186/1472-6750-14-31
Ban, Y., Xu, Z., Yang, Y., Zhang, H., Chen, H., Tang, M. (2017). Effect of Dark Septate Endophytic Fungus Gaeumannomyces cylindrosporus on Plant Growth, Photosynthesis and Pb Tolerance of Maize (Zea mays L.). Pedosphere 27, 283–292. doi: 10.1016/S1002-0160(17)60316-3
Bi, Y., Xie, L. (2021). Functions of arbuscular mycorrhizal fungi and dark septate endophytes in ecological restoration. Acta Microbiologica Sin. 61, 58–67. doi: 10.13343/j.cnki.wsxb.20200171
Chen, Y., Wang, J., Jiang, L., Li, H., Wang, H., Lv, G., et al. (2023). Prediction of spatial distribution characteristics of ecosystem functions based on a minimum data set of functional traits of desert plants. Front. Plant Sci. 14. doi: 10.3389/fpls.2023.1131778
Chen, J., Zhu, J., Yan, B., Li, J., Guo, S. (2018). Preliminary identification of endophytic fungi colonized in the root of Saussurea involucrata and Rhodiola rosea from Xinjiang region. Mycosystema 37, 110–119. doi: 10.13346/j.mycosystema.170221
Dang, H., Zhao, W., Zhang, T., Cheng, Y., Dong, J., Zhuang, L. (2022). Great gerbil burrowing-induced microbial diversity shapes the rhizosphere soil microenvironments of Haloxylon ammodendron in temperate deserts. Front. Microbiol. 13. doi: 10.3389/fmicb.2022.960594/full
Dick, R. P., Breakwell, D. P., Turco, R. F. (1996). Soil enzyme activities and biodiversity measurements as integrative microbiological indicators. Available online at: http://dl.sciencesocieties.org/publications/books/abstracts/sssaspecialpubl/methodsforasses/247/preview.
Farias, G. C., Nunes, K. G., Soares, M. A., De Siqueira, K. A., Lima, W. C., Neves, A. L. R., et al. (2020). Dark septate endophytic fungi mitigate the effects of salt stress on cowpea plants. Braz. J. Microbiol. 51, 243–253. doi: 10.1007/s42770-019-00173-4
Frew, A. (2023). Water availability alters the community structure of arbuscular mycorrhizal fungi and determines plant mycorrhizal benefit. Plants People Planet 5, 683–689. doi: 10.1002/ppp3.10372
Gai, J. P., Christie, P., Feng, G., Li, X. L. (2006). Twenty years of research on community composition and species distribution of arbuscular mycorrhizal fungi in China: a review. Mycorrhiza 16, 229–239. doi: 10.1007/s00572-005-0023-8
Gardes, M., Bruns, T. D. (1993). ITS primers with enhanced specificity for basidiomycetes–application to the identification of mycorrhizae and rusts. Mol. Ecol. 2, 113–118. doi: 10.1111/j.1365-294X.1993.tb00005.x
Ge, J., Zhang, L., Li, X., Qiang, W., He, X. (2018). Colonization of dark septate endophytes in roots of different plants and its correlation with soil factors in extreme arid desert environment. J. Hebei University(Natural Sci. Edition) 38, 73. doi: 10.3969/j.issn.1000-1565.2018.01.012
He, X., Yang, Y., Yuan, Z. (2021). Protocol for sampling of root and rhizosphere soils from trees in natural fields. BIO-PROTOCOL. doi: 10.21769/BioProtoc.2003655
Hou, L. (2020). Species Diversity and Salt Tolerance of Dark Septate Endophytes in Three Desert Plants. (Hebei University). doi: 10.27103/d.cnki.ghebu.2020.000030
Huo, L., Gao, R., Hou, X., Yu, X., Yang, X. (2021). Arbuscular mycorrhizal and dark septate endophyte colonization in Artemisia roots responds differently to environmental gradients in eastern and central China. Sci. Total Environ. 795, 148808. doi: 10.1016/j.scitotenv.2021.148808
Huo, J., Shi, Y., Chen, J., Zhang, H., Feng, L., Zhao, Y., et al. (2022). Hydraulic trade-off and coordination strategies mediated by leaf functional traits of desert shrubs. Front. Plant Sci. 13. doi: 10.3389/fpls.2022.938758
Ilyas, U., Du Toit, L. J., Hajibabaei, M., McDonald, M. R. (2024). Influence of plant species, mycorrhizal inoculant, and soil phosphorus level on arbuscular mycorrhizal communities in onion and carrot roots. Front. Plant Sci. 14, 1324626. doi: 10.3389/fpls.2023.1324626
Joshee, S., Paulus, B. C., Park, D., Johnston, P. R. (2009). Diversity and distribution of fungal foliar endophytes in New Zealand Podocarpaceae. Mycological Res. 113, 1003–1015. doi: 10.1016/j.mycres.2009.06.004
Lekberg, Y., Vasar, M., Bullington, L. S., Sepp, S.-K., Antunes, P. M., Bunn, R., et al. (2018). More bang for the buck? Can arbuscular mycorrhizal fungal communities be characterized adequately alongside other fungi using general fungal primers? New Phytol. 220, 971–976. doi: 10.1111/nph.2018.220.issue-4
Li, Y., Dang, H., Lv, X., Pu, X., Li, Z. (2022). High-throughput sequencing reveals rhizosphere fungal community composition and diversity at different growth stages of Populus euphratica in the lower reaches of the Tarim River. PeerJ. 10, e13552. doi: 10.7717/peerj.13552
Li, R., Jing, Y., Mao, J., Ning, D., Li, Y., Li, S., et al. (2021). Correlation between arbuscular mycorrhizal in walnut orchards under different intercropping with soil factors. J. West China Forestry Sci. 5071–78, 84. doi: 10.16473/j.cnki.xblykx1972.2021.01.010
Liu, Y., He, L., An, L., Helgason, T., Feng, H. (2009). Arbuscular mycorrhizal dynamics in a chronosequence of Caragana korshinskii plantations. FEMS Microbiol. Ecol. 67, 81–92. doi: 10.1111/j.1574-6941.2008.00597.x
Li, Z., Gong, X., Wang, J., Chen, Y., Liu, F., Li, H., et al. (2023). Foliar water uptake improves branch water potential and photosynthetic capacity in Calligonum mongolicum. Ecological Indicators. 146, 109825. doi: 10.1016/j.ecolind.2022.109825
Liu, Z., Wang, C., Yang, X., Liu, G., Cui, Q., Indree, T., et al. (2023). The relationship and influencing factors between endangered plant tetraena mongolica and soil microorganisms in west ordos desert ecosystem, northern China. Plants 12. doi: 10.3390/plants12051048
Liu, C., Zhang, F., Zhang, D., Srivastava, A., Wu, Q., Zou, Y. (2018). Mycorrhiza stimulates root-hair growth and IAA synthesis and transport in trifoliate orange under drought stress. Sci. Rep. 8, 1978. doi: 10.1038/s41598-018-20456-4
Liu, M., Zheng, R., Bai, S., Zhu, Y. (2017). Vertical distribution of arbuscular mycorrhizal fungal community in the rhizosphere of Spiraea pubescens in Daqingshan Mountains, Inner Mongolia. Mycosystema 36, 292–301. doi: 10.13346/j.mycosystema.160033
Luo, M., Moorhead, D. L., Ochoa-Hueso, R., Mueller, C. W., Ying, S. C., Chen, J. (2022). Nitrogen loading enhances phosphorus limitation in terrestrial ecosystems with implications for soil carbon cycling. Funct. Ecol. 36, 2845–2858. doi: 10.1111/1365-2435.14178
Madouh, T. A., Quoreshi, A. M. (2023). The function of arbuscular mycorrhizal fungi associated with drought stress resistance in native plants of arid desert ecosystems: A review. Diversity 15, 391. doi: 10.3390/d15030391
Malicka, M., Magurno, F., Piotrowska-Seget, Z. (2022). Plant association with dark septate endophytes: When the going gets tough (and stressful), the tough fungi get going. Chemosphere 302, 134830. doi: 10.1016/j.chemosphere.2022.134830
Mandyam, K., Jumpponen, A. (2008). Seasonal and temporal dynamics of arbuscular mycorrhizal and dark septate endophytic fungi in a tallgrass prairie ecosystem are minimally affected by nitrogen enrichment. Mycorrhiza 18, 145–155. doi: 10.1007/s00572-008-0165-6
McGONIGLE, T. P., Miller, M. H., Evans, D. G., Fairchild, G. L., Swan, J. A. (1990). A new method which gives an objective measure of colonization of roots by vesicular—arbuscular mycorrhizal fungi. New Phytol. 115, 495–501. doi: 10.1111/j.1469-8137.1990.tb00476.x
Pegler, D. N., Allen, M. F. (1993).The ecology of mycorrhizae. In: Kew bulletin. Available online at: https://www.jstor.org/stable/4110216?origin=crossref.
Phillips, J. M., Hayman, D. S. (1970). Improved procedures for clearing roots and staining parasitic and vesicular-arbuscular mycorrhizal fungi for rapid assessment of infection. Trans. Br. Mycological Soc. 55, 158–IN18. doi: 10.1016/S0007-1536(70)80110-3
Postma, J. W. M., Olsson, P. A., Falkengren-Grerup, U. (2007). Root colonisation by arbuscular mycorrhizal, fine endophytic and dark septate fungi across a pH gradient in acid beech forests. Soil Biol. Biochem. 39, 400–408. doi: 10.1016/j.soilbio.2006.08.007
Qin, Z., Zhang, H., Feng, G., Christie, P., Zhang, J., Li, X., et al. (2020). Soil phosphorus availability modifies the relationship between AM fungal diversity and mycorrhizal benefits to maize in an agricultural soil. Soil Biol. Biochem. 144, 107790. doi: 10.1016/j.soilbio.2020.107790
Qu, W., Xie, B., Hua, H., Bohrer, G., Penuelas, J., Wu, C., et al. (2022). Long-term nitrogen enrichment accelerates soil respiration by boosting microbial biomass in coastal wetlands. Soil Biol. Biochem. 175, 108864. doi: 10.1016/j.soilbio.2022.108864
Rodriguez, R. J., White, J. F., Arnold, A. E., Redman, R. S. (2009). Fungal endophytes: diversity and functional roles. New Phytol. 182, 314–330. doi: 10.1111/j.1469-8137.2009.02773.x
Salmeron-Santiago, I. A., Martínez-Trujillo, M., Valdez-Alarcón, J. J., Pedraza-Santos, M. E., Santoyo, G., López, P. A., et al. (2023). Carbohydrate and lipid balances in the positive plant phenotypic response to arbuscular mycorrhiza: increase in sink strength. Physiologia Plantarum 175, e13857. doi: 10.1111/ppl.v175.1
Seerangan, K., Thangavelu, M. (2014). Arbuscular mycorrhizal and dark septate endophyte fungal associations in south Indian aquatic and wetland macrophytes. J. Bot. 2014, 173125. doi: 10.1155/2014/173125
Shang, X., Zhang, F., Li, S., Hou, R. (2021). Spatial distribution of dark septate endophytes, arbuscular mycorrhiza fungi and ericoid mycorrhiza fungi in cultivated blueberries from Guizhou Province, Southwest China. Mycosystema 40, 2752–2770. doi: 10.13346/j.mycosystema.210220
Shen, Y., Zhu, B. (2021). Arbuscular mycorrhizal fungi reduce soil nitrous oxide emission. Geoderma 402, 115179. doi: 10.1016/j.geoderma.2021.115179
Shi, Y., Bayandala, Y. L., Adeli Jiang, I., Yeledenbarth (2022). Study on the AMF diversity of the soils adjacent to two tree species in gongliu wild fruit forest of xinjiang. Hunan Agric. Sci. 7), 38–41. doi: 10.16498/j.cnki.hnnykx.2022.007.010
Shi, Y. J., Wang, J., Jin, X., Wang, Z. L., Pan, D. D., Zhuang, Y., et al. (2019). Effects of intercropping of wheat on composition of cucumber seedling rhizosphere fungal community. Allelopathy J. 46, 241–249. doi: 10.26651/allelo.j/2019-46-2-1212
Stroheker, S., Dubach, V., Vögtli, I., Sieber, T. N. (2021). Investigating Host Preference of Root Endophytes of Three European Tree Species, with a Focus on Members of the Phialocephala fortinii—Acephala applanata Species Complex (PAC). J. Fungi 7, 317. doi: 10.3390/jof7040317
Stürmer, S. L., Oliveira, L. Z., Morton, J. B. (2018). Gigasporaceae versus Glomeraceae (phylum Glomeromycota): A biogeographic tale of dominance in maritime sand dunes. Fungal Ecol. 32, 49–56. doi: 10.1016/j.funeco.2017.11.008
Sun, K., Lu, F., Huang, P., Tang, M., Xu, F., Zhang, W., et al. (2022). Root endophyte differentially regulates plant response to NO 3– and NH 4+ nutrition by modulating N fluxes at the plant–fungal interface. Plant Cell Environ. 45, 1813–1828. doi: 10.1111/pce.14304
Sun, W., Shahrajabian, M. H. (2023). The application of arbuscular mycorrhizal fungi as microbial biostimulant, sustainable approaches in modern agriculture. Plants 12. doi: 10.3390/plants12173101
Tan, Y., Lv, Y., Xv, M., Qu, L., Wang, W. (2024). Differences in metabolic characteristics of rhizosphere fungal community of typical arboreal, shrubby and herbaceous species in oasis of arid region. J. Fungi 10, 565. doi: 10.3390/jof10080565
Tang, B., Man, J., Lehmann, A., Rillig, M. C. (2023). Arbuscular mycorrhizal fungi benefit plants in response to major global change factors. Ecol. Lett. 26, 2087–2097. doi: 10.1111/ele.v26.12
Tariq, A., Ullah, I., Sardans, J., Graciano, C., Mussarat, S., Ullah, A., et al. (2023). Strigolactones can be a potential tool to fight environmental stresses in arid lands. Environ. Res. 229, 115966. doi: 10.1016/j.envres.2023.115966
Tian, Y. (2018). The Study on The AMF Populations in Roots and Rhizosphere of Potato Samples Collected form The Middle Region of Inner Mongolia. (Inner Mongolia Agricultural University).
Trouvelot, A., Kough, J. L., Gianinazzi-Pearson, V. (1986).Mesure du taux de mycorhization VA d’un systeme radiculaire. Recherche de methodes d’estimation ayant une significantion fonctionnelle. In: Gianinazzi-Pearson, V., Gianinazzi, S.. Eds, Physiological and Genetical Aspects of Mycorrhizae (Paris: NRA), 217–221.
Wang, Y., Chen, L., Zhang, S., Liu, Y. (2010). Biodiversity of Arbuscular Mycorrhizal Fungi in the Natural Forests of Populus euphratica and Alhagi sparsifolia in Xinjiang. Arid Zone Res. 27, 927–932. doi: 10.13866/j.azr.2010.06.018
Wang, W., Xu, B., Hu, J., Pna, G., Liu, W. (2024). Diversity of arbuscular mycorrhizal fungi in the soil of Lespedeza davidii scrub in manganese mining area. Acta Ecologica Sin. 12), 1–11. doi: 10.20103/j.stxb.202301190124
Wang, H., Zhang, R., Cai, Y., Yang, Q., Lv, G. (2022). Ecological uniqueness and the determinants in arid desert ecosystems of Northwest China. Global Ecol. Conserv. 34, e02005. doi: 10.1016/j.gecco.2022.e02005
Wang, J., Zhen, J., Hu, W., Chen, S., Lizaga, I., Zeraatpisheh, M., et al. (2023). Remote sensing of soil degradation: Progress and perspective. Int. Soil Water Conserv. Res. 11, 429–454. doi: 10.1016/j.iswcr.2023.03.002
Wang, N. F., Zhang, T., Zhang, F., Wang, E. T., He, J. F., Ding, H., et al. (2015). Diversity and structure of soil bacterial communities in the Fildes Region (maritime Antarctica) as revealed by 454 pyrosequencing. Front. Microbiol. 6. doi: 10.3389/fmicb.2015.01188
Xia, Y., Dong, F., Lv, S., Wang, J., Jing, J., Li, J. (2015). Vertical distribution and seasonal dynamics of fine roots in Populus euphratica plantation in the extremely drought area. J. Beijing Forestry Univ. 37, 37–44. doi: 10.13332/j.1000--1522.20150082
Xie, L., Bi, Y., Ma, S., et al. (2021). Combined inoculation with dark septate endophytes and arbuscular mycorrhizal fungi: synergistic or competitive growth effects on maize?. BMC Plant Biol. 21, 498 doi: 10.1186/s12870-021-03267-0
Xie, L., He, X., Wang, K., Hou, L., Sun, Q. (2017). Spatial dynamics of dark septate endophytes in the roots and rhizospheres of Hedysarum scoparium in northwest China and the influence of edaphic variables. Fungal Ecol. 26, 135–143. doi: 10.1016/j.funeco.2017.01.007
Yan, P., Hou, H., Lv, Y., Zhang, H., Li, J., Shao, L., et al. (2023). Diversity characteristics of arbuscular mycorrhizal fungi communities in the soil along successional altitudes of Helan Mountain, arid, and semi-arid regions of China. Front. Microbiol. 14. doi: 10.3389/fmicb.2023.1099131
Yang, X. D., Anwar, E., Zhou, J., He, D., Gao, Y. C., Lv, G. H., et al. (2022). Higher association and integration among functional traits in small tree than shrub in resisting drought stress in an arid desert. Environ. Exp. Bot. 201, 104993. doi: 10.1016/j.envexpbot.2022.104993
Yang, F., Lv, G. (2023). Metabolomic Analysis of the Response of Haloxylon ammodendron and Haloxylon persicum to Drought. Int. J. Mol. Sci. 24. doi: 10.3390/ijms24109099
Yao, S., Wang, J., Huang, W., Jiao, P., Peng, C., Li, Y., et al. (2024). Adaptation strategies of Populus euphratica to arid environments based on leaf trait network analysis in the mainstream of the tarim river. Forests 15, 437. doi: 10.3390/f15030437
Ye, Y., Qu, J., Pu, Y., Rao, S., Xu, F., Wu, C. (2020). Selenium biofortification of crop food by beneficial microorganisms. J. Fungi 6, 59. doi: 10.3390/jof6020059
Zhang, J., He, X., Zhao, L., Xu, W., Yan, J. (2015). Responses of desert soil factors and dark septate endophytes colonization to clonal plants invasion. Acta Ecologica Sin. 35, 1095–1103. doi: 10.5846/stxb201403200490
Zhao, X., Gao, H., Long, J., Liu, Y., Li, X., He, X. (2021). Spatial and temporal distribution and the response to rhizosphere soil environment of dark septate endophyte and other microorganisms in roots of Haloxylon ammodendron in sand area of Northwest China. Mycosystema 40, 2716–2734. doi: 10.13346/j.mycosystema.210109
Keywords: arbuscular mycorrhizal fungi, dark septate endophytes, colonization strategy, colonization status, rhizosphere effect
Citation: Wang H, Wang H, Wei S, Sun L and Cheng L (2025) Interaction between arbuscular mycorrhizal fungi and dark septate endophytes in the root systems of Populus euphratica and Haloxylon ammodendron under different drought conditions in Xinjiang, China. Front. Plant Sci. 15:1504650. doi: 10.3389/fpls.2024.1504650
Received: 01 October 2024; Accepted: 30 December 2024;
Published: 27 January 2025.
Edited by:
Marzena Sujkowska-Rybkowska, Warsaw University of Life Sciences, PolandCopyright © 2025 Wang, Wang, Wei, Sun and Cheng. This is an open-access article distributed under the terms of the Creative Commons Attribution License (CC BY). The use, distribution or reproduction in other forums is permitted, provided the original author(s) and the copyright owner(s) are credited and that the original publication in this journal is cited, in accordance with accepted academic practice. No use, distribution or reproduction is permitted which does not comply with these terms.
*Correspondence: Hengfang Wang, d2FuZ2hmQHhqdS5lZHUuY24=
Disclaimer: All claims expressed in this article are solely those of the authors and do not necessarily represent those of their affiliated organizations, or those of the publisher, the editors and the reviewers. Any product that may be evaluated in this article or claim that may be made by its manufacturer is not guaranteed or endorsed by the publisher.
Research integrity at Frontiers
Learn more about the work of our research integrity team to safeguard the quality of each article we publish.