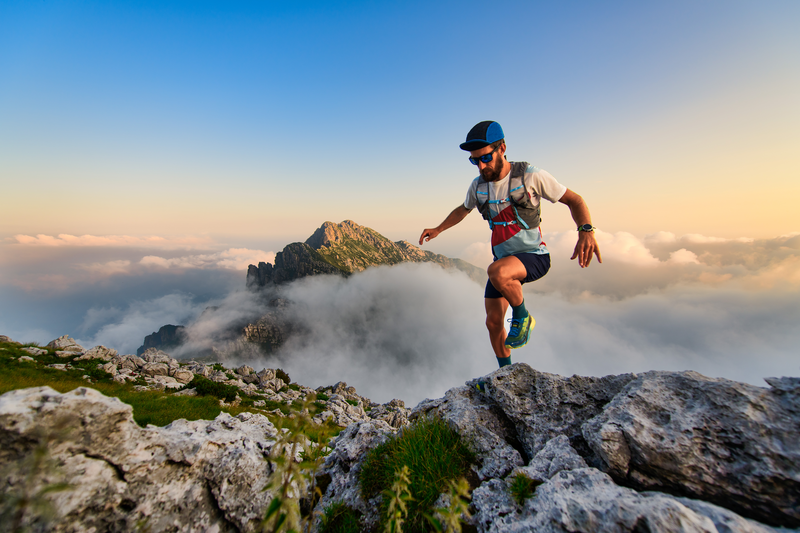
95% of researchers rate our articles as excellent or good
Learn more about the work of our research integrity team to safeguard the quality of each article we publish.
Find out more
ORIGINAL RESEARCH article
Front. Plant Sci. , 12 December 2024
Sec. Plant Genetics, Epigenetics and Chromosome Biology
Volume 15 - 2024 | https://doi.org/10.3389/fpls.2024.1501642
This article is part of the Research Topic Innovative Technologies of Molecular Cytogenetics and The Application in Plant Biology View all articles
Introduction: Rye (Secale cereale L.) played a very important role in wheat genetic improvement and forage production worldwide. However, since rye is a kind of cross-pollinated plant, high levels of genetic heterozygosity and heterogeneity existed in the genome. Genome-wide variation in repeat sequences is one of the most important reasons for chromosome evolution in rye. High-precision cytological identification can effectively identify the heterochromatin or repeat sequence variations in the rye genome, and the relationship between different rye varieties can be identified while obtaining the FISH-karyotype of different rye varieties. The evolution of rye chromosomes can be analyzed by the variation degree of different probes on rye chromosomes.
Methods: All materials were identified by non-denaturing fluorescence in situ hybridization (ND-FISH). Five probes, (AAC)6, Oligo-pSc119.2-1, Oligo-pTa71A-2, Oligo-pSc200, and Oligo-pSc250 were used to identify rye chromosomes.
Results: 15 rye varieties including S. cereale (cultivated rye and weedy rye), S. strictum (wild rye), S. sylvestre (wild rye), and S. vavilovii (wild rye) were examined by five oligonucleotides probes. 92 signal sites and 2074 signal patterns were observed, suggesting that high polymorphisms exist in the different rye genomes. The karyotypes of 15 rye varieties were obtained, the frequency of different signal types at each signal site was calculated and the model diagrams of probes (AAC)6, Oligo-pSc119.2-1, Oligo-pTa71A-2, Oligo-pSc200 + Oligo-pSc250 were drawn. The results showed that the rate of variation of different chromosomes of rye was not consistent. 1R, 6R, and 7R have higher variation and genetic diversity, while 2R and 3R have lower variation and are more conserved relative to other chromosomes. The results also indicated that S. sylvestre has a far genetic distance from other rye species, and S. vavilovii might be one of the ancestors of Chinese rye varieties.
Discussion: Results from this study confirmed rapid chromosome change and high levels of chromosome diversity in rye.
Rye (Secale cereale L, 2n=2x=14, RR genome) is a small but very important taxon in Triticeae. Rye is the secondary crop for food and feed, originated in the Near East, and was domesticated in Anatolia and Europe (Martis et al., 2013). Until now, Central and Eastern Europe is still the main producers of rye. Rye has great resistance to both biological and abiotic stresses and may carry a large number of disease-resistant, pest-resistant, and stress-resistant genes in the genome (Anderson et al., 2003; Li et al., 2021; Rakoczy-Trojanowska et al., 2021; Ren et al., 2022a). Therefore, rye was also used as one of the most important wheat-related species to improve the wheat genome (Ren et al., 2022a). Because rye is a cross-pollinated plant, there was a lot of gene exchange both within and between rye species, so the research on the genetic relationship between rye species has been inconsistent (Ren et al., 2011; Hagenblad et al., 2016; Guo et al., 2019; Skuza et al., 2019). However, according to different criteria, different rye species can be roughly divided into three categories, the wild species Secale sylvestre, the wild species Secale strictum, and Secale cereale (including cultivated and weedy rye) (Ren et al., 2011). Rye varieties were mainly cross-pollinated plants, and most of them have high genetic diversity within the species, which means there were high levels of genetic heterozygosity and heterogeneity in a rye variety (Ren et al., 2011; Bolibok-Brągoszewska et al., 2014; Guo et al., 2019). It can be seen that it is often possible to isolate different inbred lines of one rye variety by self-cross. These inbred lines from one rye variety may show different phenotypes and carry different benefit genes, thus providing different germplasm resources for the genetic improvement of wheat (Ren et al., 2022b).
There are a large number of repeated sequences in the rye genome, and the proportion of repeated sequences in the genome can reach 90% (Bauer et al., 2017). Some tandem repetitive sequences have been studied in detail based on sequence composition and genomic structure. For example, A repetition sequence of 118 bp length often appears in larger structural units, and it was named pSc119.2 (McIntyre et al., 1990). pSc200 and pSc250 have similar complex organization and contain many short direct, inverted repeats and stems (short palindromes). Therefore, for DNA containing pSc200 and pSc250, homologous recombination or chromosomal rearrangement may occur (Vershinin et al., 1995). There were many reports about the chromosome diversity within and between triticeae species or varieties from the results of fluorescence in situ hybridization (FISH) by using clones of these repetitive sequences as probes (Guo et al., 2019; Kroupin et al., 2023). Moreover, the application of oligonucleotides or multi-oligonucleotides has resulted in rapid development in the discovery of new genome- or chromosome-specific markers, such as Oligo-pSc119.2-1, Oligo-pTa71, Oligo-pSc200, and Oligo-pSc250, etc (Tang et al., 2014; Fu et al., 2015). These oligonucleotide probes have similar signal patterns to the repeat sequence probes and can be used for chromosome detection using non-denaturing fluorescence in situ hybridization (ND-FISH) (Tang et al., 2014; Fu et al., 2015). These oligonucleotide probes have obvious signals on the chromosomes of triticeae and have a very good recognition degree, which can identify different signals on chromosomes well, to quickly and accurately detect the polymorphism of signal patterns and judge the genetic differences between chromosomes of different species (Tang et al., 2014; Guo et al., 2019; Luo et al., 2022; Kroupin et al., 2023). More importantly, combining ND-FISH plus oligonucleotide probes is very cheap and much easier to operate than traditional FISH technology, making large-scale cytological identification possible.
Due to the limitation of traditional cytological markers, investigations on chromosome diversity have been limited to small numbers of individual plants (Vershinin et al., 1995; Guo et al., 2019). The research on the rye chromosomes was mainly concentrated on some cultivated rye originating from Europe and America. Genome sequencing results showed that Chinese rye (Weining) and European rye (Lo7) were very different on the genome level (Li et al., 2021; Rabanus-Wallace et al., 2021). Rye is not a major crop in China, and the relationship between Chinese rye, European rye, American rye, and wild rye is still unclear. The variation among rye varieties, as well as the evolution of rye chromosomes, remain largely unknown.
In this study, five oligonucleotide probes with high polymorphism were used with ND-FISH technology to detect the signal patterns with 15 rye varieties. High genetic diversity was observed in 15 rye varieties, and most of them have high levels of genetic heterozygosity and heterogeneity. The results also showed that S. sylvestre was very different from other rye, and S. vavilovii might be one of the ancestors of Chinese rye.
Fifteen rye varieties were used in this study, and they are listed in Table 1. The Germplasm Resources Information Network (GRIN) of the United States Department of Agriculture (USDA) kindly provided seeds of varieties with codes beginning with PI. Our laboratory collected and kept other rye varieties (Ren et al., 2011).
All materials were identified by ND-FISH. Five oligonucleotide probes, (AAC)6, Oligo-pSc119.2-1, Oligo-pTa71A-2, Oligo-pSc200, and Oligo-pSc250 were used to identify rye chromosomes. The sequences and the distribution of the signal patterns of the probes are listed in Supplementary Table S1. The details of the probes could be found in Tang et al. (2014); Cuadrado and Jouve (2002), and Luo et al. (2022). All probes were synthesized by Tsingke Biological Technology Co. Ltd. (Beijing, China). The pTa71A-2, Oligo-pSc200, and Oligo-pSc250 probes were 5’-end labeled with Cyanine Dye5 (Cy5), and the Oligo-pSc119.2-1 and (AAC)6 probes were 5’-end labeled with 6-carboxyfluorescein (6-FAM). Rye chromosomes were counterstained with 4’,6-diamidino-2-phenylindole (DAPI). The preparation of the experimental materials, probe labeling, in situ hybridization, and images captured were performed according to Tang et al. (2014) and Ren et al. (2019). Since S. cereale subsp. cereale Weining has been sequenced successfully and its mid-mitotic cytological identification results have been published, the FISH signal patterns of S. cereale subsp. cereale Weining were used as a control in this study (Li et al., 2021).
To more accurately compare the polymorphism, the signal patterns in this study were classified according to strengths, which were classified into six types: type 0 (no signals), type 1 (very weak signals), type 2 (obvious signals), type 3 (strong signals), type 4 (very strong signals) and type 5 (very strong signals and the signals radiates outside the chromosome). If there were multiple signal sites on one chromosome, these signal sites were named “probe name (chromosome-1, -2, -3, etc.)” according to the physical position from the end of the short arm to the end of the long arm. If the same chromosome of one rye variety has different probe signals, the chromosomes of this rye variety are recorded as “rye variety-1, -2, etc”.
The data of the signal types were recorded in Excel 2019. The heatmap was plotted at https://www.bioinformatics.com.cn, an online platform for data analysis and visualization (Tang et al., 2023).
A total of 30 Oligo-pSc119.2-1 signal sites and 748 signal patterns were shown on the chromosomes of 15 rye varieties (Supplementary Table S2). The Oligo-pSc119.2-1 signals showed very high polymorphism across different rye varieties. For example, different signal types of Oligo-pSc119.2-1 appeared on one signal site of 2R chromosomes of S. cereale subsp. cereale Weining (Figure 1). When compared with the signal patterns of S. cereale subsp. cereale Weining which was reported by Li et al. (2021), the Oligo-pSc119.2-1 signal patterns of S. cereale subsp. cereale Chile have four types of mutant at 3 signaling sites on the 1R chromosome, 2 types at 2 signaling sites on the 3R and 4R chromosome, 3 types at 3 signaling sites on the 5R chromosome, 4 types at 3 signaling sites on the 6R chromosome, and 4 types at 4 signaling sites on the 7R chromosome, respectively (Figure 1). All the types of signal patterns of the chromosomes of 15 rye varieties are listed in Supplementary Table S2. The statistics of different signal types at the same signal site were carried out, the proportion of each signal type on different signal sites was calculated (Supplementary Table S3), and the model of the signal patterns of Oligo-pSc119.2-1 was shown according to the types of signal patterns with the highest frequency (Figure 2). The distribution of the signal pattern types of different signal sites of Oligo-pSc119.2-1 on rye chromosome 1R to 7R and the frequency of different signal types were clearly shown in Figure 2. For example, among the five signal sites on the 1R chromosome, the most appeared signal types on Oligo-pSc119.2-1 (1RS-1), Oligo-pSc119.2-1(1RS-2), Oligo-pSc119.2-1(1RL-1), Oligo-pSc119.2-1(1RL-2), and Oligo-pSc119.2-1 (1RL-3) were type 2 (82.14%), type 1 (78.57%), type 1 (57.14%), type 1 (82.14%), and type 2 (60.71%), respectively (Figure 2; Supplementary Table S3). Compared with the signal patterns of S. cereale subsp. cereale Weining which was reported by Li et al. (2021), the mutant rates of five signal sites were higher than 60%, which were Oligo-pSc119.2-1(2RS) (62.07%), Oligo-pSc119.2-1(3RS) (62.07%), Oligo-pSc119.2-1(4RL-1) (82.14%), Oligo-pSc119.2-1(6RS-2) (60.71%), and Oligo-pSc119.2-1(7RS-2) (78.57%). The results of the mutant rates of all signal sites of Oligo-pSc119.2-1 are listed in Supplementary Table S4.
Figure 1. The ND-FISH results of Oligo-pSc119.2-1 and (AAC)6 of 1R TO 7R chromosomes for 15 rye varieties. Red signal patterns: (AAC)6. Green signal patterns: Oligo-pSc119.2-1. The names of rye were showed on the right.
Figure 2. The model of the signal patterns of Oligo-pSc119.2-1. In the figure, the green spots on the chromosomes are the signal patterns at the signal sites of Oligo-pSc119.2-1 with the highest frequency. The distribution of the probe at each signal site on the chromosome and the frequency of signal intensity types were shown, the red were the types with the highest frequency at this spot.
A total of 39 (AAC)6 signal sites and 816 signal patterns were shown on the chromosomes of 15 rye varieties (Supplementary Table S2). As shown in Figure 1, the (AAC)6 signals showed very high polymorphism across different rye varieties. (AAC)6 showed obvious signal patterns on the 1R to 7R chromosomes of S. cereale subsp. cereale Weining. However, the signal patterns were not identical, and different signal types of (AAC)6 at different signaling sites appeared on the 2R, 3R, 4R, and 7R chromosomes (Figure 1; Supplementary Table S2). The signal patterns of (AAC)6 of the other 14 rye varieties also showed a lot of variations on different chromosomes. For example, when compared with the signal patterns of S. cereale subsp. cereale Weining which was reported by Li et al. (2021), the (AAC)6 signal patterns of S. cereale subsp. cereale Chile have 2 types of the mutant at 2 signaling sites on the 1R chromosome, 3 types at 3 signaling sites on the 2R and 4R chromosomes, 5 types at 5 signaling sites on the 5R chromosome, 2 types at 2 signaling sites on the 6R chromosome, and 1 type at 1 signaling sites on the 7R chromosome, respectively (Figure 1; Supplementary Table S2). All the types of signal patterns of (AAC)6 of 15 rye varieties were listed in Supplementary Table S2. The distribution of the signal pattern types of different signal sites of (AAC)6 and the frequency of different signal types are shown in Figure 3 and Supplementary Table S3. For example, among the six signal sites on the 1R chromosome, the most appeared signal types on (AAC)6 (1R-1), (AAC)6 (1R-2), (AAC)6 (1R-3), (AAC)6 (1R-4), (AAC)6(1R-5), and (AAC)6 (1R-6) were type 1 (53.57%), type 1 (67.86%), type 3 (78.57%), type 2 (60.71%), type 0 (92.86%), and type 2 (57.14%), respectively (Figure 2; Supplementary Table S2). Compared with the signal patterns of S. cereale subsp. cereale Weining which was reported by Li et al. (2021), the mutant rates of seven signal sites were higher than 60%, which were (AAC)63R-4 (86.21%), (AAC)63R-5 (79.31%), (AAC)65R-4(75%), (AAC)65R-5 (82.14%), (AAC)65R-6 (75%), (AAC)66R-1(67.86%), and (AAC)67R-3 (62.07%). The results of the mutant rates of all signal sites of (AAC)6 are listed in Supplementary Table S4.
Figure 3. The model of the signal patterns of (AAC)6. In the figure, the red spots on the chromosomes are the signal patterns at the signal sites of (AAC)6 with the highest frequency. The distribution of the probe at each signal site on the chromosome and the frequency of signal intensity types were shown, the red were the types with the highest frequency at this spot.
A total of 22 Oligo-pSc200 + Oligo-pSc250 signal sites and 480 signal patterns were shown on the chromosomes of 15 rye varieties (Supplementary Table S2). As shown in Figure 4, the Oligo-pSc200 + Oligo-pSc250 signals showed very high polymorphism across different rye varieties, as well as the signal patterns of (AAC)6 and Oligo-pSc119.2-1. The signal patterns of Oligo-pSc200 + Oligo-pSc250 of S. cereale subsp. cereale Weining were different at the different signal sites on the 3R, 4R, and 7R chromosomes (Figure 4; Supplementary Table S2). The signal patterns of Oligo-pSc200 + Oligo-pSc250 of the other 14 rye varieties also showed a lot of variations on different chromosomes. For example, when compared with the signal patterns of S. cereale subsp. cereale Weining which was reported by Li et al. (2021), the Oligo-pSc200 + Oligo-pSc250 signal patterns of S. cereale subsp. cereale Chile have 3 types of the mutant at 2 signaling sites on the 1R chromosome, 2 types at 2 signaling sites on the 2R chromosome, 1 type at 1 signaling sites on the 3R chromosome, 3 types at 3 signaling sites on the 4R chromosome, 1 type at 1 signaling site on the 5R chromosome, 4 types at 4 signaling sites on the 6R chromosome, and 2 types at 2 signaling sites on the 7R chromosome (Supplementary Table S2; Figure 4). The signal patterns of Oligo-pSc200 + Oligo-pSc250 of S. sylvestre were completely different from other rye varieties. Most of the signal patterns of Oligo-pSc200 + Oligo-pSc250 were disappeared or very weak in S. sylvestre (Figure 4). All the types of signal patterns of Oligo-pSc200 + Oligo-pSc250 of 15 rye varieties were listed in Supplementary Table S2. The distribution of the signal pattern types of different signal sites of Oligo-pSc200 + Oligo-pSc250 and the frequency of different signal types are shown in Figure 5 and Supplementary Table S3. For example, among the two signal sites on the 1R chromosome, the most appeared signal types on Oligo-pSc200+Oligo-pSc250(1RS) and Oligo-pSc200+Oligo-pSc250(1RL) were type 4 (42.86%) and type 2 (39.29%), respectively (Figure 5; Supplementary Table S3). Compared with the signal patterns of S. cereale subsp. cereale Weining which was reported by Li et al. (2021), the mutant rates of 10 signal sites were higher than 60%, which were Oligo-pSc200 + Oligo-pSc250(1RL) (60.71%), Oligo-pSc200 + Oligo-pSc250(2RL-2) (85.71%), Oligo-pSc200 + Oligo-pSc250(4RS) (79.31%), Oligo-pSc200 + Oligo-pSc250(4RL-1) (72.41%), Oligo-pSc200 + Oligo-pSc250(5RS) (64.29%), Oligo-pSc200 + Oligo-pSc250(6RS-1) (82.14%), Oligo-pSc200 + Oligo-pSc250(6RL-1) (64.29%), Oligo-pSc200 + Oligo-pSc250(6RL-3) (89.29%), Oligo-pSc200 + Oligo-pSc250(7RL-1) (79.31%), and Oligo-pSc200 + Oligo-pSc250(7RL-2) (65.52%). The results of the mutant rates of all signal sites of Oligo-pSc200 + Oligo-pSc250 are listed in Supplementary Table S4.
Figure 4. The ND-FISH results of Oligo-pSc200 + Oligo-pSc250 for 15 rye varieties. Green signal patterns: Oligo-pSc200 + Oligo-pSc250. The names of rye were showed on the right.
Figure 5. The model of the signal patterns of Oligo-pSc200 + Oligo-pSc250 and Oligo-pTa71A-2. In the figure, the red spots on the chromosomes are the signal patterns at the signal sites of Oligo-pTa71A-2 with the highest frequency. The green spots on the chromosomes are the signal patterns at the signal sites of Oligo-pSc200 + Oligo-pSc250 with the highest frequency. The distribution of the probe at each signal site on the chromosome and the frequency of signal intensity types were shown. The red were the types with the highest frequency at this spot.
Oligo-pTa71A-2 has one signal site at the junction of the 1R short arm and the satellite, that is, the nucleolus organizer region. A total of 30 signal patterns were shown on the chromosomes of 15 rye varieties. In S. cereale subsp. cereale Weining, there was only one type of signal pattern of Oligo-pTa71A-2 (type 3). However, as shown in Figure 6, the Oligo-pTa71A-2 signals also showed high polymorphism across different rye varieties when they were compared with S. cereale subsp. cereale Weining. For example, the Oligo-pTa71A-2 signal patterns on the 1R chromosome of S. cereale subsp. cereale Chile (type 1) and S. cereale subsp. Dighoricum (type 1) were significantly weaker, while the signal patterns on the 1R chromosome of S. cereale subsp. Segetale (type 5) and S. sylvestre (type 4) were significantly stronger (Supplementary Table S4; Figure 6). The signal patterns of other rye varieties also showed many different types of variation, and the signal types of Oligo-pTa71A-2 of all 15 rye varieties were listed in Supplementary Table S2. The frequency of different signal types of Oligo-pTa71A-2 was type 1 (21.43%), type 2 (28.57%), type 3 (28.57), type 4 (10.71), and type 5 (10.71%), respectively (Figure 5; Supplementary Table S3).
Figure 6. The ND-FISH results of Oligo-pTa71A-2 for 15 rye varieties. The names of rye were showed on the top of the chromosomes. Green: Oligo-pTa71A-2.
Each chromosome showed a high level of genetic diversity. However, the degree of polymorphism of different probes on different chromosomes is different. Compared with the signal patterns of S. cereale subsp. cereale Weining which was reported by Li et al. (2021), the mutant rates based on all signal patterns of the chromosomes from high to low were: 6R (47.11%), 7R (43.94%), 5R (39%), 1R (35.48%), 4R (33.85%), 2R (28%), and 3R (25.15%) on average (Supplementary Table S4); based on the signal patterns of Oligo-pSc119.2-1 of the chromosomes from high to low were: 6R (48.33%), 4R (45%), 7R(44.17%), 3R(40%), 1R (28.67%), 5R (26%), and 2R (20%) on average (Supplementary Table S4); based on the signal patterns of (AAC)6 of the chromosomes from high to low were: 5R (45%), 7R (42.22%), 6R (34.17%), 1R (29.45%), 2R (22%), 4R(15.56%), and 3R (12.78) on average (Supplementary Table S4); based on the signal patterns of Oligo-pSc200 + Oligo-pSc250 of the chromosomes from high to low were: 6R (56%), 4R (55.56%), 1R (55%), 2R (53%), 7R (45%), 5R (42%), and 3R (40%) on average (Supplementary Table S4). Based on the signal patterns of Oligo-pTa71A-2 of the 1R chromosomes, the mutant rate of the signal patterns of Oligo-pTa71A-2 is as high as 71.43% (Supplementary Table S4).
The heat maps based on signal types of different chromosomes of different rye further illustrate the evolutionary relationship between different chromosomes of different rye varieties (Figure 7). For example, the 2R, 4R, and 5R chromosomes of S. sylvestre were divided into different clusters from other rye. On the other hand, the 1R, 3R, and 7R chromosomes of S. sylvestre were closely related to another wild rye S. strictum subsp. Anatolicum. Moreover, the 1R, 3R, and 6R of S. sylvestre were also closely related to S. cereale subsp. Segetale, Dighoricum-2 (S. cereale subsp. Dighoricum), and Vavilovii-1 (S. vavilovii), respectively (Figure 7).
Figure 7. Heatmap clustering analysis of 1R to 7R chromosomes of 15 rye varieties. (A) Heatmap clustering analysis of 1R chromosome. (B) Heatmap clustering analysis of 2R chromosome. (C) Heatmap clustering analysis of 3R chromosome. (D) Heatmap clustering analysis of 4R chromosome. (E) Heatmap clustering analysis of 5R chromosome. (F) Heatmap clustering analysis of 6R chromosome. (G) Heatmap clustering analysis of 7R chromosome. All signal patterns showed on the chromosomes were used for analysis.
The results showed that the 1R chromosomes of different rye had significant differences, and all 15 rye varieties could be classified into 4 clusters (Figure 7A). Clusters 1 and 2 are more closely related, while clusters 3 and 4 are more closely related. In cluster 1, S. cereale subsp. cereale Qinling, S. cereale subsp. cereale Aigan, and S. cereale subsp. cereale Chile were included. In cluster 2, S. cereale subsp. Segetale, S. sylvestre, Vavilovii-2 (S. vavilovii), Shaanxi-1 (S. cereale subsp. cereale Shaanxi), AR106 BONE-2 (S. cereale subsp. cereale AR106BONE), and Anatonicum-1 (S. strictum subsp. Anatolicum) were included. In cluster 3, S. cereale subsp. cereale Baili, Anatonicum-2 (S. strictum subsp. Anatolicum), and Dalmaticum-2 (S. strictum subsp. strictum Dalmaticum) were included. In cluster 4, S. cereale subsp. cereale Weining, Vavilovii-1 (S. vavilovii), Shannxi-2 (S. cereale subsp. cereale Shaanxi), Dalmaticum-1 (S. strictum subsp. strictum Dalmaticum), S. cereale subsp. cereale Kustro, S. cereale subsp. cereale Jingzhou, S. cereale subsp. Dighoricum, and AR106 BONE-1 (S. cereale subsp. cereale AR106BONE) were included. S. vavilovii had a closer relationship with S. cereale subsp. cereale Weining, S. cereale subsp. cereale Shaanxi, and S. strictum subsp. Anatolicum.
The 2R chromosomes of different rye varieties also had significant differences, and all 15 rye varieties could be classified into 4 clusters (Figure 7B). Clusters 1 and 2 are more closely related, while clusters 3 and 4 are more closely related. In cluster 1, only S. sylvestre was included. In cluster 2, AR106 Bone-2 (S. cereale subsp. cereale AR106BONE), S. cereale subsp. cereale Aigan, S. cereale subsp. cereale Baili, Dalmaticum-1 (S. strictum subsp. strictum Dalmaticum), Qinling-1 (S. cereale subsp. cereale Qinling), Dighoricum-1 (S. cereale subsp. Dighoricum), and Chile-1 (S. cereale subsp. cereale Chile) were included. In cluster 3, S. cereale subsp. cereale Weining, AR106 BONE-1 (S. cereale subsp. cereale AR106BONE), Qinling-2 (S. cereale subsp. cereale Qinling), S. cereale subsp. cereale Kustro, S. cereale subsp. Segetale, Chile-2 (S. cereale subsp. cereale Chile), Dighoricum-2 (S. cereale subsp. Dighoricum) were included. In cluster 4, S. cereale subsp. cereale Shaanxi, S. strictum subsp. Anatolicum, S. vavilovii, S. cereale subsp. cereale Jingzhou, and Dalmaticum-2 (S. strictum subsp. strictum Dalmaticum) were included. S. vavilovii had a much closer relationship with S. cereale subsp. cereale Shaanxi, and S. strictum subsp. Anatolicum.
The 3R chromosomes also showed high differences among different rye, and 15 rye varieties could be classified into 4 clusters, and clusters 2, 3, and 4, are more closely related (Figure 7C). In cluster 1, S. cereale subsp. cereale Qinling, Dighoricum-2 (S. cereale subsp. Dighoricum), S. sylvestre, Aigan-1 (S. cereale subsp. cereale Aigan) and Anatolicum-1 (S. strictum subsp. Anatolicum) were included. In cluster 2, S. cereale subsp. Segetale, S. cereale subsp. cereale Kustro, Dighoricum-1 (S. cereale subsp. Dighoricum), Weining-1 (S. cereale subsp. cereale Weining), and Shaanxi-1 (S. cereale subsp. cereale Shaanxi) were included. In cluster 3, S. cereale subsp. cereale Chile, Anatolicum-2 (S. strictum subsp. Anatolicum), Jingzhou-1 (S. cereale subsp. cereale Jingzhou), and Vavilovii-1 (S. vavilovii) were included. In cluster 4, Jinzhou-2 (S. cereale subsp. cereale Jingzhou), Shaanxi-2 (S. cereale subsp. cereale Shaanxi), Aigan-2 (S. cereale subsp. cereale Aigan), Vavilovii-2 (S. vavilovii), S. cereale subsp. cereale AR106BONE, S. cereale subsp. cereale Baili, Weining-2 (S. cereale subsp. cereale Weining), and S. strictum subsp. strictum Dalmaticum were included. S. vavilovii had a much closer relationship with S. cereale subsp. cereale Jingzhou, S. cereale subsp. cereale Shaanxi, S. cereale subsp. cereale Aigan, and S. cereale subsp. cereale AR106BONE.
The 4R chromosomes showed higher differences among different rye, and 15 rye varieties could be classified into 7 clusters. Clusters 1, 2, and 3 are more closely related, while clusters 4, 5, 6, and 7 are more closely related (Figure 7D). In cluster 1, only S. sylvestre was included. In cluster 2, Aigan-1 (S. cereale subsp. cereale Aigan), Kustro-1 (S. cereale subsp. cereale Kustro), and Anatolicum-2 (S. strictum subsp. Anatolicum) were included. In cluster 3, S. cereale subsp. Segetale, S. cereale subsp. cereale AR106BONE, Kustro-1 (S. cereale subsp. cereale Kustro), and Dalmaticum-2 (S. strictum subsp. strictum Dalmaticum) were included. In cluster 4, only Aigan-2 (S. cereale subsp. cereale Aigan) was included. In cluster 5, S. cereale subsp. cereale Jingzhou, S. cereale subsp. cereale Shaanxi, and S. vavilovii were included. In cluster 5, only S. cereale subsp. cereale Chile was included. In cluster 6, Anatolicum-1 (S. strictum subsp. Anatolicum) and S. cereale subsp. Dighoricum were included. In cluster 7, S. cereale subsp. cereale Qinling, S. cereale subsp. cereale Baili, S. cereale subsp. cereale Weining, Dalmaticum-1 (S. strictum subsp. strictum Dalmaticum), and S. vavilovii were included. S. vavilovii had a closer relationship with Chinese rye landraces and wild rye.
Based on the signal patterns of 5R, 15 rye varieties could be classified into 5 clusters, and clusters 3, 4, and 5 are more closely related (Figure 7E). In cluster 1, only S. sylvestre was included. In cluster 2, Shaanxi-2 (S. cereale subsp. cereale Shaanxi), Aigan-1 (S. cereale subsp. cereale Aigan), Dalmaticum (S. strictum subsp. strictum Dalmaticum), and Kustro (S. cereale subsp. cereale Kustro) were included. In cluster 3, S. strictum subsp. Anatolicum and S. cereale subsp. Dighoricum were included. In cluster 4, Chile (S. cereale subsp. cereale Chile), Aigan-2 (S. cereale subsp. cereale Aigan), and Segetale-2 (S. cereale subsp. Segetale) were included. Cluster 5 could be divided into 4 sub-clusters. In sub-cluster 1, only Shaanxi-1 (S. cereale subsp. cereale Shaanxi) was included. In sub-cluster 2, AR106 BONE (S. cereale subsp. cereale AR106BONE), Vavilovii-1 (S. vavilovii), and S. cereale subsp. cereale Jingzhou were included. In sub-cluster 3, S. cereale subsp. cereale Weining and Qinling-2 (S. cereale subsp. cereale Qinling) were included. In sub-cluster 4, Vavilovii-2 (S. vavilovii), S. cereale subsp. cereale Baili, Segetale-1 (S. cereale subsp. Segetale), and Qinling-1 (S. cereale subsp. cereale Qinling) were included. S. vavilovii had a closer relationship with two Chinese rye S. cereale subsp. cereale Baili and S. cereale subsp. cereale Jingzhou.
Based on the signal patterns of 6R, 15 rye varieties could be classified into 3 clusters, and clusters 2 and 3 are more closely related (Figure 7F). In cluster 1, S. cereale subsp. cereale Qinling, S. sylvestre, Vavilovii-1 (S. vavilovii), S. cereale subsp. cereale Jingzhou, and Chile-1 (S. cereale subsp. cereale Chile) were included. Cluster 2 could be divided into 2 sub-clusters. In sub-cluster 1, Baili-2 (S. cereale subsp. cereale Baili), Anatolicum-2 (S. strictum subsp. Anatolicum), and Dalmaticum-2 (S. strictum subsp. strictum Dalmaticum) were included. In sub-cluster 2, Vavilovii-2 (S. vavilovii), S. cereale subsp. Segetale, Baili-1 (S. cereale subsp. cereale Baili), Dighoricum-1 (S. cereale subsp. Dighoricum), and Shaanxi-1 (S. cereale subsp. cereale Shaanxi) were included. Cluster 3 could be divided into 3 sub-clusters. In sub-cluster 1, Anatolicum-1 (S. strictum subsp. Anatolicum), Chile-2 (S. cereale subsp. cereale Chile), Shaanxi-2 (S. cereale subsp. cereale Shaanxi), and Aigan-1(S. cereale subsp. cereale Aigan) were included. In sub-cluster 2, Kustro-2 (S. cereale subsp. cereale Kustro) and AR106 BONE-1 (S. cereale subsp. cereale AR106BONE) were included. In sub-cluster 3, Aigan-2 (S. cereale subsp. cereale Aigan), Dalmaticum-1 (S. strictum subsp. strictum Dalmaticum), AR106 BONE-2 (S. cereale subsp. cereale AR106BONE), Kustro-1 (S. cereale subsp. cereale Kustro), S. cereale subsp. cereale Weining, and Dighoricum-2 (S. cereale subsp. Dighoricum), were included. S. vavilovii had a closer relationship with three Chinese rye S. cereale subsp. cereale Qinling, S. cereale subsp. cereale Jingzhou, and S. cereale subsp. cereale Baili.
Based on the signal patterns of 7R, 15 rye varieties could be classified into 3 clusters, and clusters 2 and 3 are more closely related (Figure 7G). In cluster 1, Vavilovii-1 (S. vavilovii), Dighoricum-1 (S. cereale subsp. Dighoricum), Dalmaticum-1 (S. strictum subsp. strictum Dalmaticum), Baili-2 (S. cereale subsp. cereale Baili), and S. cereale subsp. cereale Jingzhou were included. Cluster 2 could be divided into 4 sub-clusters. In sub-cluster 1, S. sylvestre and S. cereale subsp. cereale Kustro were included. In sub-cluster 2, Aigan-2 (S. cereale subsp. cereale Aigan) and Dalmaticum-2 (S. strictum subsp. strictum Dalmaticum) were included. In sub-cluster 3, S. cereale subsp. cereale Chile and Shaanxi-1 (S. cereale subsp. cereale Shaanxi) were included. In sub-cluster 4, Vavilovii-2 (S. vavilovii), S. strictum subsp. Anatolicum, AR106 BONE-1 (S. cereale subsp. cereale AR106BONE), and Kustro-1 (S. cereale subsp. cereale Kustro) were included. Cluster 3 could be divided into 2 sub-clusters. In sub-cluster 1, S. cereale subsp. Segetale, Dighoricum-2 (S. cereale subsp. Dighoricum), and Baili-1 (S. cereale subsp. cereale Baili) were included. In sub-cluster 2, S. cereale subsp. cereale Qinling, S. cereale subsp. cereale Weining, Shaanxi-2 (S. cereale subsp. cereale Shaanxi), Aigan-2 (S. cereale subsp. cereale Aigan), and S. cereale subsp. cereale AR106BONE were included. S. vavilovii had a closer relationship with S. cereale subsp. cereale Jingzhou.
Cytogenetics enables the simultaneous of the genome and comparison of the chromosomes between different species. Therefore, cytogenetics has been widely used in wheat improvement programs (Gill, 2022; Kroupin et al., 2023). As one of the most valuable tools of cytogenetics, FISH is a powerful tool that enables to performance of systematic, evolutionary, and population studies of wheat wild relatives as well as to characterize alien introgression into the wheat genome (Kroupin et al., 2023). Triticeae probes based on satellite repeats have been widely used for chromosome analysis, and many new probes, especially for oligonucleotide and multi-oligonucleotides were developed and were quickly used in chromosome identification. For example, to verify the distribution of satellite DNA in Thinopyrum bessarabicum chromosomes, new oligonucleotides were designed based on the sequences of satellite clusters or the coding sequences of 5S rDNA. These probes can distinguish all wheat and Th. bessarabicum chromosomes after one round of FISH (Chen et al., 2019). The genetic polymorphisms among different Dasypyrum villosum accessions were also determined by cytological analysis. One multi-oligonucleotides probe ONPM4# (contained six oligonucleotides: pAs1-1, pAs1-3, pAs1-4, pAs1-6, AFA-3, and AFA-4), and two oligonucleotides probes (GAA)10 and pSc119.2-1 were used, and a total of 106 polymorphic chromosomes were identified (Wu et al., 2023). The chromosomes of Agropyron cristatum were identified by five tandem repeat probes, in addition to 5S and 45S ribosomal DNA and rye sub-telomeric repeats pSc119.2 and pSc200. As a result, structural rearrangements were observed for chromosomes 2P, 4P, 5P, 6P, and 7P of A. cristatum, and chromosomal inversions were also found for the pericentric region of 4P and whole chromosome arm 6PL (Said et al., 2018). Chromosome-specific FISH markers for Psathyrostachys huashanica were developed by Zhang et al. (2022). The results indicated that the combination of pSc200, pTa71A-2, and Oligo-44 can distinguish all Ns chromosomes from wheat chromosomes in the wheat background. Structural chromosome variations (SCVs) are large-scale genomic variations that can be also detected by FISH (Zhao et al., 2022; Ren et al., 2016). For example, 543 wheat accessions from China were identified by oligonucleotide probe multiplex FISH, and 139 SCVs including translocations, pericentric inversions, presence/absence variations, and copy number variations were identified at 230 loci (Zhao et al., 2022). A complex chromosome rearrangement line with balanced reciprocal translocations 1RS.3BL and 3BS.1BL, and a complex chromosome translocation line with 3DS.4BSDS and 3DL-4BSPS.4BL was also identified by FISH (Ren et al., 2016; Li et al., 2022). Ren et al. (2017) found mutants on the 4A and 5A chromosomes of wheat in a newly developed 1RS.1BL translocation line when compared with the wheat parent. Five different oligonucleotide probes (Oligo-pSc119.2-1, Oligo-pTa535-1, Oligo-Ku, Oligo-pSc200, and Oligo-pSc250) were used with ND-FISH to examine 21 wheat cultivars, and 17.6% of the A-genome chromosomes, 25.9% of the B-genome chromosomes, and 8.9% of the D-genome chromosomes showed obvious mutations when they were compared to the standard signal patterns (Ren et al., 2019). The results of cytological identification can also shed light on some related theories such as recombination, species evolution, and gene functions. For example, to precisely identify Triticum timopheevii chromosomes and to trace the evolution of Triticum zhukovskyi, several probes, such as pSc119.2, pTa71, pAs1, pTa535-1, (GAA)9, and (CTT)10 were used. As a result, the origin of T. zhukovskyi from the hybridization of T. timopheevii with T. monococcum was confirmed (Badaeva et al., 2016). To study the effects of structural variations of chromosomes during the meiotic recombination, the wheat lines with different 5A structures were used to investigate their meiotic recombination by ND-FISH. The results showed that the smaller structural difference between the 5A in the distal regions resulted in a higher recombination frequency in the interstitial region (Zou et al., 2022). ND-FISH with oligonucleotide probes derived from tandem repeats and single-copy FISH were used to investigate recombination in three kinds of 5AL, and the variations of the signal patterns of Oligo-pSc119.2-1, Oligo-pTa535–1, Oligo-713, Oligo-275.1, Oligo-18, and the variations of the signal patterns of the single-copy FISH probes showed the structural variations caused by tandem repeats might be one of the factors affecting meiotic recombination in wheat (Zou et al., 2021). The FISH results of the localization of CENH3 in the centromere of soybean, emphasize the role of centromere satellites in maintaining stable positions, underscoring their importance in centromere organization (Liu et al., 2023).
As one of the most valuable wheat-related species, the precise identification of the rye chromosomes and precise recognition of the rye chromosomes in the wheat genetic background were important goals for wheat distance hybridization breeding programs. Several FISH probes were developed based on the repetitive sequences, such as pSc119.2, pAs1, pTa-535, pTa71, CCS1, and PAWRC.1 (Tang et al., 2014). These probes can distinguish wheat and rye chromosomes in one cell and play important roles in wheat-rye cytogenetics identification studies (An et al., 2019; Ren et al., 2017, Ren et al., 2022a; Ren et al., 2022b; Han et al., 2020, 2023; Kroupin et al., 2023). Several new oligonucleotide probes that can be used with ND-FISH were developed in recent years and quickly used in rye chromosome identification due to their significant advantages, such as being more convenient, precise, and cheap (Tang et al., 2014; Fu et al., 2015). For example, six rye cultivars were analyzed by using oligonucleotides TAMRA-oligo-6 and FAM-pSc119.2-1 to represent the signal patterns of pSc200 and pSc119.2, and 73 types of heterochromatin blocks were identified on all seven chromosomes (Guo et al., 2019).
In this study, seven oligonucleotide probes were employed to identify the genetic polymorphisms among different rye varieties. In previous studies, these probes have been proven can replace the roles of repetitive sequences pAs1, pSc119.2, pTa-535, pTa71, and rye genomic DNA in FISH analysis of wheat, rye, and hybrids derived from wheat × rye by DN-FISH (Tang et al., 2014; Fu et al., 2015; Ren et al., 2019; Ren et al., 2022a; Ren et al., 2022b; Luo et al., 2022). The results showed that the oligonucleotide probes Oligo-pSc119.2-1, Oligo-pTa71A-2, Oligo-pSc200 + Oligo-pSc250, and (AAC)6 produced high-resolution signal patterns not only showed different signal patterns in the rye chromosomes but also revealed the varied distribution of these probes among chromosomes and varieties (Figures 1, 4, 6). A total of 92 polymorphic signal patterns were identified from 15 rye varieties and high levels of chromosomal heterozygosity and heterogeneity were observed. The results showed that these oligonucleotide probes could effectively detect the differences in the signal patterns in rye chromosomes, and the polymorphisms of the chromosomes could be easily observed according to these probes by the ND-FISH method. Compared with the signal patterns of S. cereale subsp. cereale Werining, which was reported by Li et al. (2021), the total mutant rates of the other 14 rye varieties ranged from 25.15% to 47.11% (Supplementary Table S4). The results suggested that 6R and 7R have the highest variation and the genetic diversity, while 2R and 3R have the lowest variation and are more conserved relative to other chromosomes. Oligo-pTa71A-2 had the highest variation rate among all probes, suggesting that the distal of the 1RS chromosome may have more variation and higher genetic diversity (Luo et al., 2022).
The phylogenetic relationships and taxonomy within the genus Secale have long been the subject of controversy (Ren et al., 2011; Skuza et al., 2019). In the beginning, according to the results of the morphological, ecological, and earlier cytological investigations, scientists believed that S. vavilovii should be an ancestor of the cultivated rye (Vavilov, 1926). Several useful genes, such as self-fertility, high protein content, resistance to diseases, and resistance to sprouting were successfully used for the improvement of cultivated rye in Europe (Meier et al., 1996). The analyses of RFLP of mitochondrial DNA (mtDNA) indicated that S. vavilovii had a close genetic relationship with S. strictum and S. cereale, but a far genetic relationship with S. sylvestre and S. cereale subsp. Segetale (Skuza et al., 2007). The analysis of ISSR also indicated that the genetic distance of S. vavilovii was closer to S. cereale but far from S. sylvestre and S. strictum subsp. Anatolicum (Ren et al., 2011). Based on the analysis of noncoding regions of the chloroplast (cpDNA) and mtDNA, the results indicated that S. Vavilovii was very similar to S. cereale (Skuza et al., 2019). Schreiber et al. (2019) found that there was only weak genetic differentiation between S. vavilovii and domesticated rye with likely gene flow. All the results indicated that S. vavilovii has a very close genetic relationship with cultivated rye, which supported the assumption that S. vavilovii might share a common ancestor with cultivated rye, or S. vavilovii was one of the ancestors of cultivated rye (Skuza et al., 2019). China has no history of growing and domesticating rye. Therefore, these undomesticated or artificially selected rye in China tend to have richer genetic resources. In recent years, a large number of disease-resistance genes have been found in these Chinese rye varieties and used in wheat breeding (Ren et al., 2017; Ren et al., 2022a; Ren et al., 2022b). The previous molecular analysis suggested that rye had been introduced from its origin center to northwest China and then spread to southwest China (Ren et al., 2011). During the spread process, genetic differentiation likely occurred. There were big differences between the genomes of Chinese rye (Weining) and European rye (Lo7) (Rabanus-Wallace et al., 2021; Li et al., 2021). The rye varieties from northwestern China were more genetically similar to the rye varieties of western countries than the rye varieties from southwestern China (Ren et al., 2011). In this study, based on the signal patterns of different probes, it looked like the Chinese rye had a closer genetic relationship with S. vavilovii (Figure 7), which suggested that S. vavilovii might be one of the ancestors of Chinese rye.
S. sylvestre was another wild rye and had a far genetic distance from other rye varieties. The lowest call rates were observed on the Rye600k array in samples from S. vavilovii (87.5%) and S. sylvestre (84.3%), consistent with their evolutionary distance from cultivated rye (Bauer et al., 2017). Based on the results characteristics of internal transcribed spacer (ITS) rDNA sequences suggested that S. sylvestre is the most distant taxonomic unit (De Bustos and Jouve, 2002). Analyses of RFLP, AFLP, SSR, and ISSR also indicated that S. sylvestre is one of the most ancient species (Chikmawati et al., 2005; Shang et al., 2006; Skuza et al., 2007; Ren et al., 2011). The analysis of noncoding regions of the cpDNA and mtDNA also indicated the divergence of S. sylvestre from other species and subspecies of rye (Skuza et al., 2019). ND-FISH results of (AAC)6 showed different signal patterns between S. sylvestre and other rye varieties (He et al., 2021). In this study, the signal patterns of (AAC)6, Oligo-pSc119.2-1, Oligo-pTa71A-2, Oligo-pSc200 + Oligo-pSc250 showed different signal patterns between S. Sylvestre and other 14 rye varieties (Figures 1, 4, 6). The signal patterns of Oligo-pSc200 + Oligo-pSc250 on 7 chromosomes of S. sylvestre were completely different from other rye varieties (Figure 4). And the results of the cluster analysis also showed that S. sylvestre was highly divergent from other rye varieties, which was consistent with the results of previous studies (Ren et al., 2011; Tang et al., 2011; Cuadrado and Jouve, 2002).
Several studies proposed that cultivated rye had been domesticated from weedy rye, rather than directly from wild species (Sun et al., 2022). The RFLP analysis of mtDNA showed that S. cereale subsp. Segetale had a closer genetic relationship with S. sylvestre among the taxa (Skuza et al., 2007). The ISSR analysis also indicated greater similarity among the weedy subspecies than among different varieties of cultivated rye subspecies (Ren et al., 2011), which meant the leave of genetic differentiation among weedy rye was smaller than that of cultivated rye varieties. In this study, lower polymorphisms in S. cereale subsp. Segetale were observed (Figure 7). However, another weedy rye S. cereale subsp. Dighoricum showed higher chromosome differentiation than that of S. cereale subsp. Segetale (Figure 7). Sun et al. (2022) indicated that interspecific introgression serves as one of the likely causes of obscure species taxonomy of rye. In this study, the cluster analysis based on FISH signal patterns of cultivated rye, weedy rye, and wild rye (except S. sylvestre) also showed the same conclusion as Sun et al. (2022). All results from this study confirmed chromosome differentiation and high levels of chromosome diversity in different rye varieties.
In this study, 15 rye varieties including S. cereale (9 cultivated rye and 2 weedy rye), S. strictum (wild rye), S. sylvestre (wild rye), and S. vavilovii (wild rye) were examined by oligonucleotides probes. 30 signal sites and 748 signal patterns, 39 signal sites, and 816 signal patterns, 22 signal sites and 480 signal patterns, 1 signal site and 30 signal patterns were detected by Oligo-pSc119.2-1, (AAC)6, Oligo-pSc200 + Oligo-pSc250, and Oligo-pTa71A-2, respectively. The results indicated that high polymorphism occurs in the genomes of different rye species. The degree of variation of signal patterns on different chromosomes was not consistent, indicating that different chromosomes have different degrees of evolutionary conservation. 1R, 6R, and 7R have the highest variation rate and the fastest evolution, while 2R and 3R have the least variation rate and tend to be conservative in evolution. Moreover, the cluster analysis also indicated that S. sylvestre has the most far genetic distance from other rye species, and the ancestor of Chinese rye varieties may be S. vavilovii. This also suggested that these undomesticated and unselected Chinese rye varieties may contain more favorable genes in the genome, which may provide more genetic resources for distant hybridization breeding of wheat in the future.
The datasets presented in this study can be found in online repositories. The names of the repository/repositories and accession number(s) can be found in the article/Supplementary Material.
ZL: Data curation, Formal analysis, Writing – original draft, Writing – review & editing. ZS: Data curation, Formal analysis, Writing – review & editing. TR: Data curation, Formal analysis, Funding acquisition, Investigation, Methodology, Project administration, Writing – original draft, Writing – review & editing.
The author(s) declare financial support was received for the research, authorship, and/or publication of this article. This study was funded by the National Natural Science Foundation of China (32401797), and the Foundation of Sichuan Province Science and Technology Support Program (2024NSFSC0316).
The authors declare that the research was conducted in the absence of any commercial or financial relationships that could be construed as a potential conflict of interest.
All claims expressed in this article are solely those of the authors and do not necessarily represent those of their affiliated organizations, or those of the publisher, the editors and the reviewers. Any product that may be evaluated in this article, or claim that may be made by its manufacturer, is not guaranteed or endorsed by the publisher.
The Supplementary Material for this article can be found online at: https://www.frontiersin.org/articles/10.3389/fpls.2024.1501642/full#supplementary-material
An, D. G., Ma, P. T., Zheng, Q., Fu, S. L., Li, L. H., Han, F. P., et al. (2019). Development and molecular cytogenetic identification of a new wheat-rye 4R chromosome disomic addition line with resistances to powdery mildew, stripe rust and sharp eyespot. Theor. Appl. Genet. 132, 257–272. doi: 10.1007/s00122-018-3214-3
Anderson, G. R., Papa, D., Peng, J., Tahi, M., Lapitan, N. L. V. (2003). Genetic mapping of Dn7, a rye gene conferring resistance to the Russian wheat aphid in wheat. Theor. Appl. Genet. 107, 1297–1303. doi: 10.1007/s00122-003-1358-1
Badaeva, E. D., Ruban, A. S., Zoshchuk, S. A., Surzhikov, S. A., Knupffer, H., Kilian, B. (2016). Molecular cytogenetic characterization of Triticum timopheevii chromosomes provides new insight on genome evolution of T. zhukovskyi. Plant Syst. Evol. 302, 943–956. doi: 10.1007/s00606-016-1309-3
Bauer, E., Schmutzer, T., Barilar, I., Mascher, M., Gundlach, H., Martis, M. M., et al. (2017). Towards a whole-genome sequence for rye (Secale cereale L.). Plant J. 89, 853–869. doi: 10.1111/tpj.2017.89.issue-5
Bolibok-Brągoszewska, H., Targońska, M., Bolibok, L., Kilian, A., Rakoczy-Trojanowska, M. (2014). Genome-wide characterization of genetic diversity and population structure in Secale. BMC Plant Biol. 14, 184. doi: 10.1186/1471-2229-14-184
Chen, J., Tang, Y., Yao, L., Wu, H., Tu, X., Zhuang, L., et al. (2019). Cytological and molecular characterization of Thinopyrum bessarabicum chromosomes and structural rearrangements introgressed in wheat. Mol. Breed. 39, 146. doi: 10.1007/s11032-019-1054-8
Chikmawati, T., Skovmand, B., Gustafson, J. P. (2005). Phylogenetic relationships among Secale species revealed by amplified fragment length polymorphisms. Genome 48, 792–801. doi: 10.1139/g05-043
Cuadrado, A., Jouve, N. (2002). Evolutionary trends of different repetitive DNA sequences during speciation in the genus Secale. J. Hered. 93, 339–345. doi: 10.1093/jhered/93.5.339
De Bustos, A., Jouve, N. (2002). Phylogenetic relationships of the genus Secale based on the characterisation of rDNA ITS sequences. Plant Syst. Evol. 235, 147–154. doi: 10.1007/s00606-002-0215-z
Fu, S., Chen, L., Wang, Y., Li, M., Yang, Z., Qiu, L., et al. (2015). Oligonucleotide probes for ND-FISH analysis to identify rye and wheat chromosomes. Sci. Rep. 5, 10552. doi: 10.1038/srep10552
Gill, B. S. (2022). A century of cytogenetic and genome analysis: impact on wheat crop improvement. Wheat Improvement (Cham: Springer International Publishing), 277–297.
Guo, J., Lei, Y., Zhang, H., Song, D., Liu, X., Cao, Z., et al. (2019). Frequent variations in tandem repeats pSc200 and pSc119.2 cause rapid chromosome evolution of open-pollinated rye. Mol. Breed. 39, 133. doi: 10.1007/s11032-019-1033-0
Hagenblad, J., Oliveira, H. R., Forsberg, N. E. G., Leino, M. W. (2016). Geographical distribution of genetic diversity in Secale landrace and wild accessions. BMC Plant Biol. 16, 23. doi: 10.1186/s12870-016-0710-y
Han, G. H., Liu, S. Y., Wang, J., Jin, Y. L., Zhou, Y. L., Luo, Q. L., et al. (2020). Identification of an elite wheat-rye T1RS·1BL translocation line conferring high resistance to powdery mildew and stripe rust. Plant Dis. 104, 2940–2948. doi: 10.1094/PDIS-02-20-0323-RE
Han, G., Liu, H., Zhu, S., Gu, T., Cao, L., Yan, H., et al. (2023). Two functional CC-NBS-LRR proteins from rye chromosome 6RS confer differential age-related powdery mildew resistance to wheat. Plant Biotechnol. J. 22, 66–81. doi: 10.1111/pbi.14165
He, H., Du, H., Liu, R., Liu, T., Yang, L., Gong, S., et al. (2021). Characterization of a new gene for resistance to wheat powdery mildew on chromosome 1RL of wild rye Secale sylvestre. Theor. Appl. Genet. 134, 887–896. doi: 10.1007/s00122-020-03739-1
Kroupin, P. Y., Ulyanov, D. S., Karlov, G. I., Divashuk, M. G. (2023). The launch of satellite: DNA repeats as a cytogenetic tool in discovering the chromosomal universe of wild Triticeae. Chromosoma 132, 65–88. doi: 10.1007/s00412-023-00789-4
Li, Z., Ren, Z., Tan, F., Luo, P., Ren, T. (2022). Molecular cytogenetic characterization of novel 1RS.1BL translocation and complex chromosome translocation lines with stripe rust resistance. Int. J. Mol. Sci. 23, 2731. doi: 10.3390/ijms23052731
Li, G., Wang, L., Yang, J., He, H., Jin, H., Li, X., et al. (2021). A high-quality genome assembly highlights rye genomic characteristics and agronomically important genes. Nat. Genet. 53, 574. doi: 10.1038/s41588-021-00808-z
Liu, Y., Yi, C., Fan, C., Liu, Q., Liu, S., Shen, L., et al. (2023). Pan-centromere reveals widespread centromere repositioning of soybean genomes. Proc. Natl. Acad. Sci. U.S.A. 120, e2310177120. doi: 10.1073/pnas.2310177120
Luo, J., Liao, R., Duan, Y., Fu, S., Tang, Z. (2022). Variations of subtelomeric tandem repeats and rDNA on chromosome 1RS arms in the genus Secale and 1BL.1RS translocations. BMC Plant Biol. 22, 212. doi: 10.1186/s12870-022-03598-6
Martis, M. M., Zhou, R., Haseneyer, G., Schmutzer, T., Vrána, J., Kubaláková, M., et al. (2013). Reticulate evolution of the rye genome. Plant Cell 25, 3685–3698. doi: 10.1105/tpc.113.114553
McIntyre, C. L., Pereira, S., Moran, L. B., Appels, R. (1990). New Secale cereale (rye) DNA derivatives for the detection of rye chromosome segments in wheat. Genome 33, 635–640. doi: 10.1139/g90-094
Meier, S., Kunzmann, R., Zeller, F. J. (1996). Genetic variation in germplasm accessions of Secale vavilovii Grossh. Genet. Resour. Crop Evol. 43, 91–96. doi: 10.1007/BF00126946
Rabanus-Wallace, M. T., Hackauf, B., Mascher, M., Lux, T., Wicher, T., Gundlash, H., et al. (2021). Chromosome-scale genome assembly provides insights into rye biology, evolution and agronomic potential. Nat. Genet. 53, 564–573. doi: 10.1038/s41588-021-00807-0
Rakoczy-Trojanowska, M., Bolibok-Brągoszewska, H., Myśków, B., Dzięgielewska, M., Stojałowski, S., Grądzielewska, A., et al. (2021). “Genetics and genomics of stress tolerance,” in The rye genome, compendium of plant genomes; rabanusWallace. Ed. Stein, N. (Springer Nature Switzerland AG, Cham, Switzerland), 213–236.
Ren, T. H., Chen, F., Zou, Y. T., Jia, Y. H., Zhang, H. Q., Yan, B. J., et al. (2011). Evolutionary trends of microsatellites during the speciation process and phylogenetic relationships within the genus Secale. Genome 54, 316–326. doi: 10.1139/g10-121
Ren, T., He, M., Sun, Z., Tan, F., Luo, P., Tang, Z., et al. (2019). The polymorphisms of oligonucleotide probes in wheat cultivars determined by ND-FISH. Molecules 24, 1126. doi: 10.3390/molecules24061126
Ren, T., Jiang, Q., Sun, Z., Zhao, L., Peng, W., Ren, Z., et al. (2022b). Development and molecular cytogenetic characterization of novel primary wheat-rye 1RS.1BL translocation lines from multiple rye sources with resistance to stripe rust. Plant Dis. 106, 2191–2200. doi: 10.1094/PDIS-11-21-2605-RE
Ren, T., Li, Z., Yan, B., Tan, F., Tang, Z., Fu, S., et al. (2016). De novo balanced complex chromosome rearrangements involving chromosomes 1B and 3B of wheat and 1R of rye. Genome 59, 1076–1084. doi: 10.1139/gen-2016-0112
Ren, T., Sun, Z., Hu, Y., Ren, Z., Tan, F., Luo, P., et al. (2022a). Molecular cytogenetic identification of new wheat-rye 6R, 6RS, and 6RL addition lines with resistance to stripe rust and powdery mildew. Front. Plant Sci. 13, 992016. doi: 10.3389/fpls.2022.992016
Ren, T., Tang, Z., Fu, S., Yan, B., Tan, F., Ren, Z., et al. (2017). Molecular cytogenetic characterization of novel wheat-rye T1RS.1BL translocation lines with high resistance to diseases and great agronomic traits. Front. Plant Sci. 8, 799. doi: 10.3389/fpls.2017.00799
Said, M., Hřibová, E., Danilova, T. V., Karafátová, M., Čížková, J., Friebe, B., et al. (2018). The Agropyron cristatum karyotype, chromosome structure and cross−genome homoeology as revealed by fuorescence in situ hybridization with tandem repeats and wheat single−gene probe. Theor. Appl. Genet. 131, 2213–2227. doi: 10.1007/s00122-018-3148-9
Schreiber, M., Himmelbach, A., Börner, A., Mascher, M. (2019). Genetic diversity and relationship between domesticated rye and its wild relatives as revealed through genotyping- by-sequencing. Evolutionary Appl. 12, 66–77. doi: 10.1111/eva.2019.12.issue-1
Shang, H. Y., Wei, Y. M., Wang, X. R., Zheng, Y. L. (2006). Genetic diversity and phylogenetic relationships in the rye genus Secale L. (rye) based on Secale cereale microsatellite markers. Genet. Mol. Biol. 29, 685–691. doi: 10.1590/S1415-47572006000400018
Skuza, L., Rogalska, S. M., Bocianowski, J. (2007). RFLP analysis of mitochondrial DNA in the genus Secale. Acta Biol. Crac. Bot. 49, 77–87.
Skuza, L., Szućko, I., Filip, E., Strzała, T. (2019). Genetic diversity and relationship between cultivated, weedy and wild rye species as revealed by chloroplast and mitochondrial DNA non-coding regions analysis. PloS One 14, e0213023. doi: 10.1371/journal.pone.0213023
Sun, Y., Shen, E., Hu, Y., Wu, D., Feng, Y., Lao, S., et al. (2022). Population genomic analysis reveals domestication of cultivated rye from weedy rye. Mol. Plant 15, 552–561. doi: 10.1016/j.molp.2021.12.015
Tang, D., Chen, M., Huang, X., Zhang, G., Zeng, L., Zhang, G., et al. (2023). Rplot: A free online platform for data visualization and graphing. PloS One 18, e0294236. doi: 10.1371/journal.pone.0294236
Tang, Z. X., Ross, K., Ren, Z. L., Yang, Z. J., Zhang, H. Y., Chikmawati, T., et al. (2011). “Secale, chapter 8,” in Wild crop relatives: genomic and breeding resources. Ed. Kole, C. (Cereals, Springer, Berlin), 367–396.
Tang, Z., Yang, Z., Fu, S. (2014). Oligonucleotides replacing the roles of repetitive sequences pAs1, pSc119.2, pTa-535, pTa71, CCS1, and pAWRC.1 for FISH analysis. J. Appl. Genet. 55, 313–318. doi: 10.1007/s13353-014-0215-z
Vershinin, A. V., Schwarzacher, T., Heslop-Harrison, J. S. (1995). The large-scale genomic organization of repetitive DNA families at the telomeres of rye chromosomes. Plant Cell 71823–1833. doi: 10.1105/tpc.7.11.1823
Wu, N., He, Z., Fang, J., Liu, X., Shen, X., Zhang, J., et al. (2023). Chromosome diversity in Dasypyrum villosum, an important genetic and trait resource for hexaploid wheat engineering. Ann. Bot. 131, 185–197. doi: 10.1093/aob/mcac054
Zhang, H., Wang, F., Zeng, C., Zhu, W., Xu, L., Wang, Y., et al. (2022). Development and application of specific FISH probes for karyotyping Psathyrostachys huashanica chromosomes. BMC Genomics 22, 309. doi: 10.1186/s12864-022-08516-6
Zhao, J., Zheng, X., Qiao, L., Yang, C., Wu, B., He, Z., et al. (2022). Genome-wide association study reveals structural chromosome variations with phenotypic effects in wheat (Triticum aestivum L.). Plant J. 112, 1447–1461. doi: 10.1111/tpj.v112.6
Zou, Y., Luo, J., Tang, Z., Fu, S. (2022). Variations of wheat (Triticum aestivum L.) chromosomes caused by the 5A chromosomes with complex cytological structure. Front. Plant Sci. 13, 992934. doi: 10.3389/fpls.2022.992934
Keywords: rye, FISH, genetic diversity, oligonucleotides, evolution
Citation: Li Z, Sun Z and Ren T (2024) Frequent variations and phylogenetic relationships within the genus Secale identified by ND-FISH according to the genome-wide universal oligonucleotides chromosome probes. Front. Plant Sci. 15:1501642. doi: 10.3389/fpls.2024.1501642
Received: 26 September 2024; Accepted: 22 November 2024;
Published: 12 December 2024.
Edited by:
Richard Wang, United States Department of Agriculture, United StatesReviewed by:
Handong Su, Huazhong Agricultural University, ChinaCopyright © 2024 Li, Sun and Ren. This is an open-access article distributed under the terms of the Creative Commons Attribution License (CC BY). The use, distribution or reproduction in other forums is permitted, provided the original author(s) and the copyright owner(s) are credited and that the original publication in this journal is cited, in accordance with accepted academic practice. No use, distribution or reproduction is permitted which does not comply with these terms.
*Correspondence: Tianheng Ren, cmVudGhAc2ljYXUuZWR1LmNu
†These authors have contributed equally to this work
Disclaimer: All claims expressed in this article are solely those of the authors and do not necessarily represent those of their affiliated organizations, or those of the publisher, the editors and the reviewers. Any product that may be evaluated in this article or claim that may be made by its manufacturer is not guaranteed or endorsed by the publisher.
Research integrity at Frontiers
Learn more about the work of our research integrity team to safeguard the quality of each article we publish.