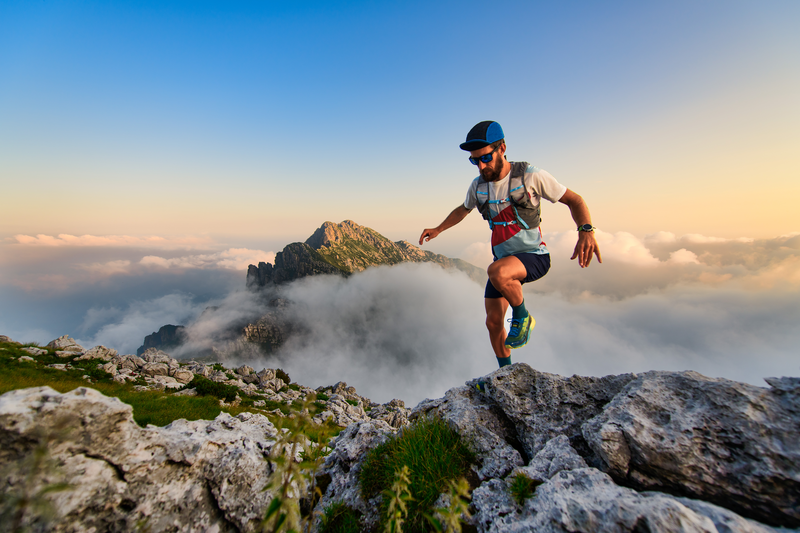
94% of researchers rate our articles as excellent or good
Learn more about the work of our research integrity team to safeguard the quality of each article we publish.
Find out more
ORIGINAL RESEARCH article
Front. Plant Sci. , 19 December 2024
Sec. Plant Symbiotic Interactions
Volume 15 - 2024 | https://doi.org/10.3389/fpls.2024.1500894
This article is part of the Research Topic Advancing Mycorrhizal Research for Sustainable Ecosystem and Agricultural Practices View all 4 articles
Introduction: Heat stress caused by global warming adversely affects wheat yield through declining most nutritional quality attributes in grains, excluding grain protein content.
Methods: This research investigated the biochemical, physiological, and antioxidant responses of wheat plants under heat stress, focusing on the role of plant growth-promoting bacteria (Micromonospora sp.). Two wheat genotypes were studied: one heat-sensitive and one heat-tolerant, examining their responses to heat stress with and without bacterial inoculation.
Results: Under heat stress, the sensitive cultivar experienced significant reductions in photosynthesis rate, chlorophyll content, and RuBisCO activity (57-61%), while the tolerant cultivar had milder reductions (24-28%). Micromonospora sp. treatment notably improved these parameters in the sensitive cultivar (+48-78%), resulting in a substantial increase in biomass production (+43-53%), which was not seen in the tolerant cultivar. Additionally, oxidative stress markers (H2O2 and MDA) were elevated more in the sensitive cultivar (82% and 90% higher) compared to the tolerant one. Micromonospora sp. treatment effectively reduced these markers in the sensitive cultivar (-28% and -27%). Enhanced activity of antioxidant enzymes and ASC-GSH pathway enzymes was particularly evident in Micromonospora sp.-treated sensitive plants. Carbohydrate metabolism shifted, with increased soluble sugars and significant rises in sucrose content in Micromonospora sp.-treated plants under stress.
Discussion: The higher soluble sugar levels facilitated amino acid synthesis, contributing to biosynthesis of secondary metabolites, including flavonoids, polyphenols, and anthocyanins. This was reflected in increased activity of phenylalanine ammonia-lyase, cinnamate (CA) 4-hydroxylase, and chalcone synthase enzymes, indicating the activation of phenylpropanoid pathways. Overall, the findings suggest that Micromonospora sp. can mitigate heat stress effects by enhancing photosynthetic efficiency, antioxidant defense, and metabolic adaptations in heat-sensitive wheat cultivars.
According to Intergovernmental Panel for Climate Change (IPCC), earth’s surface temperature from 2011-2020 increased by 1.1°C compared to the period of 1850-1900, primarily due to human activities through emissions of greenhouse gases (IPCC, 2023). Based on the present acceleration, the earth’s temperature is expected to increase by an additional 1.5°C by 2050 (IPCC, 2023). Over the past decade, most research in the agronomy section has emphasized understanding the effect of such deviations in normal temperature on plant growth and production, which can cause stress such as heat, salt, drought and water deficit (Gui et al., 2021; Sheteiwy et al., 2021). Previous research has indicated that heat stress caused by global warming adversely affected wheat yield through declining most nutritional quality attributes in grains, excluding grain protein content, which can improve under higher temperatures in some plants (Zhao et al., 2022; Kumar et al., 2023). In this regard, a reduction of about 6% in wheat grain yield is expected for every degree Celsius of temperature rise, based on a crop simulation modeling analysis (Asseng et al., 2015). Similarly, it has previously been reported that there is an unambiguous relationship between wheat production and air temperature during the growth season, so for the temperature rises by 1.5°C and 3°C, a predicted decline in wheat yields is expected at about 7% and 24%, respectively (Hussain and Mudasser, 2007). Moreover, changes in the morphologic and physiologic parameters of crops were reported when they were exposed to even short period of heat stress (Ullah et al., 2022). The production of free radicals in plant cells has been previously reported as a result of macromolecules oxidation (Notununu et al., 2022). Thus, detriment to photosynthetic systems and a decline in photosynthesis rate can occur in response to free radicals and their negative impacts on cell and thylakoid membranes (Kumar et al., 2023).
Plant-beneficial bacteria have garnered significant interest for their potential applications in sustainable agriculture as biofertilizers or biopesticides as well as phytoremediation (Zulfiqar et al., 2021; Sheteiwy et al., 2022; Yang et al., 2022; El-Sawah et al., 2023; Yaghoubi Khanghahi et al., 2024). Notably, certain plant growth-promoting bacteria (PGPB) have been proposed as stress-tolerant bioactive strains. These bacteria not only enhance plant growth but also mitigate the adverse effects of environmental stressors by bolstering plant resistance and productivity (Kumar and Dubey, 2020; Madnay et al., 2022). PGPB can stimulate plant growth, increase yield, and prevent pathogen infections by enhancing the synthesis of phytohormones and antioxidants, which consequently impact physiological and metabolic characteristics (Mandon et al., 2021; Pankievicz et al., 2021). Among PGPB, Micromonospora sp., which have been isolated from diverse environmental locations, have attracted attention due to their capacity to produce phytohormones (Trujillo et al., 2014; Ortúzar et al., 2020). Moreover, Micromonospora sp. are recognized as significant sources of antibiotics (El-Tarabily et al., 2008), making them potent biocontrol agents against pathogenic fungi and stress responses (Zadel et al., 2020).
The production of natural bioactive metabolites, particularly those derived from microorganisms such as bacteria can help plants tolerate stress (Yaghoubi Khanghahi et al., 2022a; Athukorala et al., 2023). Environmental stressors are known to stimulate antioxidant production in living organisms, among which, heat stress is a primary abiotic challenge for both eukaryotes and prokaryotes (Huang et al., 1998; Larkindale and Huang, 2004), particularly in the context of global environmental changes. Heat stress induces the production of reactive oxygen species (ROS), leading to oxidative stress (Foyer et al., 1997; Kocsy et al., 2004). While the heat stress mechanisms in eukaryotes and prokaryotes like cyanobacteria are well-studied, the responses of bacteria to stress are less understood (Hassan et al., 2020). Moreover, to our knowledge, no studies have investigated the use of Micromonospora species to enhance wheat growth under stress conditions.
Therefore, the aim of the current research was to study the modifications in the biochemical, physiological, and antioxidant parameters of wheat plants under heat stress through an examination of the potential of plant growth-promoting bacteria. Efforts were also undertaken to enhance our understanding of how two distinct genotypes, one sensitive to heat and the other tolerant, respond to heat stress when exposed to beneficial bacterial inoculation. This research seeks to further our understanding of these interactions and develop strategies to protect plants from the adverse effects of future temperature increases.
Eighteen actinobacterial strains were isolated from Deseret soil located at Riyadh, Saudi Arabia. Soil (2 g) was serially diluted (1:10–1:1000) in sterile saline and plated on starch-casein agar (Küster and Williams, 1964) and soil extract media (Barakate et al., 2022) with glycerol (carbon source). Nalidixic acid was also added to inhibit bacteria capable of overcrowding, without inhibiting the growth of actinobacteria. Antifungals such as cycloheximide and nystatin (0.05 mg mL-1) were added to soil. Plates were incubated at 28°C for 7-12 days, and colonies were then purified and stored in 20% glycerol at −20°C as stock cultures.
The selected potential actinobacterial isolate was subjected to extract the genomic DNA according to the technique outlined by Hong et al (Hong et al., 2009). The 16S rRNA gene PCR amplification was conducted on the template using the universal primers 27F (5’-AGAGTTTGATC(AC)TGCCTCAG-3’) and 1498R (5-ACGGCTACCTTGTTACGACTT-3). All PCR amplification was performed in reactions buffer contains total DNA, Taq DNA polymerase, 3 mM of dNTP, and 3 mM MgCl2. The PCR condition was set on an initial denaturation (94°C for 5 min) and 35-40 cycles of amplification (95°C for 1.2 min, 55°C for 1.2 min, and 72°C for 2.5 min), followed by an extension step (73°C for 4 min). The amplicons were sequenced from both ends by MacroGen Company (South Korea; http://www.dna.macrogen.com). The bacteria isolate was identified by aligning the sequences with the BLASTn tool in the NCBI database (www.ncbi.nlm.nih.gov). More details on Abdelgawad et al (AbdElgawad et al., 2023). The MEGAX software program was used to carry out the cluster analysis.
Antioxidant activity of the selected isolate was assessed with the ferric reducing antioxidant power (FRAP) assay, measuring absorbance at 590 nm, based on Schlesier et al (Schlesier et al., 2002). The total phenolic and flavonoid content in bacterial isolates was analyzed according to Holder and Boyce (1994). A 24-hour culture was used to inoculate the medium, followed by incubation (37°C for 7 days) in darkness. After incubation, samples were centrifuged at 6000 rpm for 10 minutes. This acidified supernatant (by HCl to pH 2.8) was mixed with an equal volume of diethyl ether and left to incubate in the dark for 4 hours. After overnight incubation at 4°C, the upper solvent phase was evaporated, and 2–3 mL of HPLC-grade methanol was added. Quantification of indole-3-acetic acid (IAA) and gibberellic acid (GA3) was conducted using high-performance liquid chromatography (HPLC). Cytokinin (CK) production by actinobacteria was assessed via a cucumber cotyledon greening bioassay, as outlined by Fletcher et al (Fletcher et al., 1982).
Healthy uniform seeds of two genotypes of wheat (Triticum aestivum) including Giza-168 as a heat-tolerant genotype and Misr-3 as a heat-sensitive genotype (Omar et al., 2023) were planted in pots (with a diameter and height of 25 cm) containing sterilized clay soil (Tref EGO substrates, Netherland). The seeds were obtained from Agriculture Research Center, Giza, Egypt. The inoculation treatment is divided into two levels including (i) soil and seeds treatment with actinobacterial suspension and (ii) control treatment by adding sterile deionized water to the soil and priming the seeds with it. For biofertilization treatment, the bacterial strain was grown in Nutrient Broth medium at 28°C for 1 day and then was concentrated by centrifugation at 6500 rpm for 10 min, and the obtained pellet was washed and re-suspended in a sterile KCl solution (0.9%, w/v). The density of this bacterial suspension was adjusted to 10–7 CFU mL−1, approximating an optical density at 600 nm equivalent to 0.7–0.8, and used for seeds and soil inoculation before the initial planting and for subsequent additions to pots every 2 weeks (Yaghoubi Khanghahi et al., 2020). This suspension was applied to the soil before planting and added to the pot’s biweekly. Soil was autoclaved prior to bacterial inoculation treatment to eliminate unwanted microbial contaminants, thereby ensures that only targeted bacterial strains thrive during the incubation, minimizing interference from fast-growing or competing soil microorganisms.
Plants were also exposed to two levels of heat stress including controlled high-temperature conditions (38°C) and a recommended optimal temperature (24°C/18°C) for two weeks. The temperature applied was chosen after preliminary investigation, where initial tests using a wide range of temperatures and time period treatments reported 45°C to induce a progressively adverse impact with >50% dead plants after 14 days. The three pots represented three biological replicates for each treatment. All the pots were weighed daily to keep the water level (> 60% of field capacity). The shoots’ fresh weight (FW) and dry weight (DW) were gouged after seven weeks of growth and kept at -80°C for further examinations.
Some photosynthetic traits were assessed to investigate the impacts of beneficial actinobacteria and heat stress on plant biomass production. In this regard, the light-saturated photosynthetic rate of the most youthful, expanded leaves was analyzes by a LI-COR portable photosynthesis system. The concentration of chlorophyll a (Chl a) and chlorophyll b (Chl b) pigments were measured by reading the absorbance of the extracted samples at 665 and 652 nm, respectively (Lichtenthaler and Buschmann, 2001). The activity of RuBisCO enzyme was assessed based on the oxidation of nicotinamide adenine dinucleotide at wave length of 340 nm (Lobo et al., 2015).
To estimate malondialdehyde (MDA) concentration in the shoot tissues, the fresh samples were homogenized in ethanol (80% v/v) at 7000 g for in 60 second using a MagNALyser and reacted with thiobarbituric acid (TBA) reagent. The absorbance of the final product was measured at 450, 532, and 600 nm (Hodges et al., 1999). The content of hydrogen peroxide (H2O2) in the shoots was also measured in trichloroacetic acid (0.1% v/v) on the basis of peroxide-catalyzed oxidation of Fe2+, according to the xylenol orange method (AbdElgawad et al., 2016).
The sugars in the fresh shoot samples were extracted using a TAE buffer (50 mM, pH 7.5) supplemented with polyclar (0.15% v/v), sodium azide (0.02% v/v), PMSF (2 mM), sodium bisulfite (12 mM), mannitol (10 mM), and mercaptoethanol (1 mM). Following extraction, the samples were centrifuged, and the supernatant was passed through a mixed-bed Dowex column containing 300 µL each of Dowex H+ and Dowex Ac– (100–200 mesh) (AbdElgawad et al., 2023). Quantification of glucose, sucrose, and fructose was conducted using high-performance anion-exchange chromatography with pulsed amperometric detection (HPAEC-PAD) according to the methodology described by AbdElgawad et al (AbdElgawad et al., 2023). Total soluble sugars in the shoot were quantified using a spectrophotometric method. Initially, sugars were extracted with 80% ethanol (v/v) and reacted with anthrone reagent (anthrone dissolved in 72% v/v H2SO4), following the protocol outlined by Yaghoubi et al (Yaghoubi Khanghahi et al., 2022a). The absorbance of the samples was measured at 625 nm using a multi-mode microplate reader (Synergy Mx, Biotek, Santa Clara, USA) to determine the total soluble sugar content.
Total antioxidant capacity in the shoot samples was measured using ferric reducing/antioxidant power (FRAP) reagent, containing 0.3 M acetate buffer (pH 3.6), TPTZ (0.01) and FeCl3 (0.02 M), followed by reading the OD at 600 nm (Benzie and Strain, 1999). The standard was 6−hydroxy−2,5,7,8−tetramethylchromane−2−carboxylic acid (Trolox). The content of total polyphenols and flavonoids in the shoot samples, as described by Zhang et al (Zhang et al., 2006). and Chang et al (Chang et al., 2022), respectively. The total tocopherols by HPLC (SCL-10 AVP, Shimadzu Corporation) (AbdElgawad et al., 2015). HPLC method was employed to estimate reduced glutathione (GSH) and reduced ascorbate (ASC) (Hartley-Whitaker et al., 2001; Potters et al., 2004). The activities of antioxidant enzymes were assessed in a semi-high-throughput set-up (AbdElgawad et al., 2016; Shabbaj et al., 2022). The activity of superoxide dismutase (SOD) was measured by estimating the inhibition of nitro-blue tetrazolium reduction (Dhindsa et al., 1982). The oxidation of pyrogallol in phosphate buffer at 430 nm was analyzed to measure the activity of peroxidase (POX) (Kumar and Khan, 1983). The activity of catalase (CAT) was assessed by observing the H2O2 decomposition at 240 nm (Kumar and Khan, 1983). The estimation of ascorbate peroxidase (APX) activity was done by recording the decrease in absorbance at 290 nm as fully described by Nakano and Asada (1981). The decrease in NADPH at 340 nm was monitored to estimate the activity of glutathione reductase (GR) (Murshed et al., 2008). The reduction of 2-hydroxy-ethyl-disulfide by reduced glutathione was recorded to measure the activity of glutaredoxin (Grx) (Lundberg et al., 2001). The reduction in NADPH absorption was evaluated to measure the activity of glutathione peroxidase (GPX) (Drotar et al., 1985). Thioredoxin (Trx) content was assayed by recording NADPH oxidation at 340 nm (Wolosiuk et al., 1979).
To measure the anthocyanins content, methanol: HCl (99:1 v/v) was used for homogenizing the samples, followed by incubating the extracted samples at 25°C for 24 h in the dark conditions and centrifuging at 4000 g for 5 min. The absorbance of the extracted supernatant was read at 550 nm (ε550 = 233,000 M−1 cm−1) (Wagner, 1979).
Flavonoid compounds and phenolic acids were analyzed by HPLC with a Lichrosorb Si-60 column (7 μm, 3 × 150 mm) and DAD detector, as fully explained by Hamad et al (Hamad et al., 2015). Briefly, acetone:water (4:1, v/v) solution was used for homogenizing the shoot samples for 24 h. the final extraced samples were subjected to HPLC at 0.8 mL min-1 (flow rate), in which the mobile phase consisted of water:formic acid (90:10 v/v) and acetonitrile:water:formic acid (85:10:5 v/v/v). A calibration curve of the related standard was considered to estimate the content of each compound.
The activity of phenylalanine amino-layse (PAL) was measured by extracting the samples in sodium borate buffer and reading the absorbance of trans-cinnamic acid output at 290 nm (Hamad et al., 2015). Cinnamate (CA) 4-hydroxylase (C4H) activity was measured based on a method described by Jadhav et al (Jadhav et al., 2013). Briefly, shoot samples were homogenized in Tris buffer and its absorbance was recorded at 340 nm (ε340 = 6200 M−1 cm−1). 4-coumarate:coenzymeA ligase (4CL) activity was measured spectrophotometrically to measure formation of CoA esters of various phenolic acids (Allina et al., 1998).
Statistical analyses were performed using the SPSS statistical package (SPSS Inc., Chicago, IL, USA). One-way analysis of variance (ANOVA) and Tukey’s Honest Significant Difference (HSD) post-hoc test were conducted to determine significance at a 5% probability level (p<0.05). The values represent the mean of at five replicates.
Among several bacterial isolates from Riyadh, Saudi Arabia, one isolate that showed a great growing and colonizing ability under heat stress conditions (56°C) in the primary experiments was selected for further analysis. Based on the 16S rRNA gene sequencing, the actinobacteria isolate was closely related to Micromonospora sp, with similarity ≥ 100% (Figure 1). The NCBI and GenBank nucleotide sequence databases received the 16S rRNA gene data under the accession number (OQ978135) for deposit.
Figure 1. Phylogenetic analysis of JMG2. The tree was constructed by the neighbor-joining method based on 16S rRNA sequences. Numbers refer to bootstrap values for each node out of a total of 100 replicate resampling. The numbers in the bracket are the EMBL accession numbers of the 16S rRNA sequences of reference bacteria. Streptomyces megaspores, Bacillus subtilis and Escherichia coli were used as the outgroup.
Micromonospora sp. showed high antioxidant bioactivity as indicated by FRAP assay (Table 1). The strain can also produce high amounts of the total phenolic and flavonoid metabolites. This bioactive strain also showed a high potential for phytohormone production such as indole acetic acid (IAA), gibberellic acid (GA), and cytokinin (CK) (Table 1).
Plants exposed to stress had the lower biomass weight in both cultivars (p < 0.05), a clear improvement in fresh (+43%) and dry weights (+53%) was observed in sensitive cultivar when they were treated with Micromonospora sp. under heat stress conditions, the improvement that was not observed in the stress-tolerant line (p > 0.05) (Figure 2). Heat stress conditions led to a sharp decrease (p < 0.05) in the photosynthesis rate, Chl a+b content, and RuBisCO activity, equal to -57%, -58%, and -61% in the sensitive cultivar, and -26%, -24%, and -28% in the tolerant cultivar compared the control un-stressed plants, respectively (Figure 3). Nevertheless, Micromonospora sp. treatment improved (p < 0.05) the status of the mentioned parameters under stress in the sensitive line by +51%, +48%, and +78% compared to the non-inoculated plants, respectively. This increase in the inoculated tolerant cultivar was not significant (p > 0.05).
Figure 2. The effect of Micromonospora sp. and heat stress (HS) on plant biomass in two heat-sensitive (Misr-3) and tolerant (Giza-168) cultivars. The means in each parameter and each cultivar with similar small letter(s) are not significantly different at 5% probability level (Tukey HSD test). FW, Fresh weight; DW, Dry weight.
Figure 3. The effect of Micromonospora sp. and heat stress (HS) on the photosynthesis parameters (µmol CO2 m-2 s-1 for PN, µg g-1 FW for chlrophyll a+b content, and mg g-1 protein for RuBisCO activity) in two heat-sensitive (Misr-3) and tolerant (Giza-168) cultivars. The means in each parameter and each cultivar with similar small letter(s) are not significantly different at 5% probability level (Tukey HSD test). PN, Photosynthesis rate.
Oxidative stress was increased in both cultivars grown under heat stress conditions (p < 0.05), where the concentration of H2O2 and MDA were 82% and 90% in sensitive cultivar and 42% and 32% in tolerant cultivar higher than those un-stressed plants, respectively (Figure 4). The most interesting aspect of the results is the decreasing trend (p < 0.05) in H2O2 and MDA contents in response to bio-inoculation treatment in stressed plants about -28% and -27% in sensitive cultivar, respectively. This reduction was 16% (p < 0.05) and 6% (p > 0.05) in the stress-tolerant cultivar, respectively.
Figure 4. The effect of Micromonospora sp. and heat stress (HS) on the oxidative markers (represented as µmol g-1 FW for H2O2 and nmol g-1 FW for MDA) in two heat-sensitive (Misr-3) and tolerant (Giza-168) cultivars. The means in each parameter and each cultivar with similar small letter(s) are not significantly different at 5% probability level (Tukey HSD test). H2O2, Hydrogen peroxide; MDA, Malondialdehyde.
The activity of the direct ROS-detoxifying enzymes (POX, SOD, and CAT) and those enzymatic components of the ascorbate-glutathione (ASC-GSH) pathway (APX, GPX GR, and DHAR), as well as other antioxidant enzymes involved in the catalytic cycle (Prxs and Grxs) were investigated to unveil the plant mechanisms in promoting the toleration against oxidative damage induced by heat stress, and relieving the content of H2O2 and MDA in plants (Figures 5, 6). Accordingly, however, the activity of SOD, POX and CAT were significantly increased (except for CAT in tolerant cultivar) under stress conditions, they were more activated (p < 0.05) in Micromonospora sp.-treated plants in both cultivars (except for SOD in tolerant cultivar) compared to the no inoculation treatment in stressed plants (Figure 5). Similarly, the highest activity of APX, DHAR, GR, GPX, Grxs, and Prxs in both cultivars was observed in stressed plants treated with Micromonospora sp., which in the sensitive plants were 78, 30, 35, 42, 65, and 51% greater than those in non-inoculated stressed plants (p < 0.05). In contrast, this increase was more imperceptible in the tolerant cultivar, so only the activity of APX (+19%), GPX (+13%), and Prxs (+16%) was significantly higher than those non-inoculated plants under stress, and the increase in the activity of DHAR (+3%), GR (+10%), and Grxs (+16%) was not statistically significant (p > 0.05) (Figure 6).
Figure 5. The effect of Micromonospora sp. and heat stress (HS) on the antioxidant direct ROS-scavenging enzymes (represented as μmol min-1 mg-1 protein for POX and CAT, and mmol min-1 mg-1 protein for SOD) in two heat-sensitive (Misr-3) and tolerant (Giza-168) cultivars. The means in each parameter and each cultivar with similar small letter(s) are not significantly different at 5% probability level (Tukey HSD test). POX, Peroxidase; CAT, Catalase; SOD, Superoxide dismutases.
Figure 6. The effect of Micromonospora sp. and heat stress (HS) on the enzymatic components (μmol min-1 mg-1 protein) of the ascorbate-glutathione (ASC/GSH) and catalytic cycles in two heat-sensitive (Misr-3) and tolerant (Giza-168) cultivars. GPX, Glutathione peroxidase; APX, Ascorbate peroxidase; GR, Glutathione reductase; Grxs, Glutaredoxins; Prxs, Peroxiredoxins; DHAR, Dehydroascorbate reductase.
The content of antioxidant proteins in the ASC/GSH pathway (GSH and ASC) and redox-dependent signaling pathway (Trxs) in the sensitive cultivar were significantly increased in response to both bio-inoculation and stress treatments (p < 0.05) (Figure 7). Accordingly, the highest content of ASC, GSH, and Trxs in inoculated plants under stress was 76, 86, and 23% (p < 0.05) greater than those in non-inoculated stressed plants. On the other hand, the content of ASC and GSH in the tolerant cultivar was not affected by stress (p > 0.05), however, the values in Micromonospora sp.-treated plants under stress reached the highest and were about +34-61% higher than other treatments (p < 0.05). Similarly, Micromonospora sp. further improved the total antioxidant capacity (TAC) and antioxidants production (PP, Flav, TP), particularly under heat stress (Figure 8).
Figure 7. The effect of Micromonospora sp. and heat stress (HS) on non-enzymatic (µmol g-1 FW) component of the ascorbate-glutathione (ASC/GSH) cycle and redox-dependent signaling pathway in two heat-sensitive (Misr-3) and tolerant (Giza-168) cultivars. The means in each parameter and each cultivar with similar small letter(s) are not significantly different at 5% probability level (Tukey HSD test). ASC, Ascorbate; GSH, Gluthatione; Trx, Thioredoxin.
Figure 8. The effect of Micromonospora sp. and heat stress (HS) on the antioxidant metabolites in plant (µmol Torolex g-1 FW for TAC; mg GAE g-1 FW for PP; mg Quercetin g-1 FW for Flav; ng g-1 FW for TP) in two heat-sensitive (Misr-3) and tolerant (Giza-168) cultivars. The means in each parameter and each cultivar with similar small letter(s) are not significantly different at 5% probability level (Tukey HSD test). TAC, Total antioxidant capacity; PP, Polyphenols; Flav, Flavonoids; TP, Tocopherols.
To investigate carbon metabolism in wheat plants, the adaptation and modification in soluble sugars content were studied under heat stress and bio-inoculation treatment (Figure 9). In this regard, heat stress remarkably (p < 0.05) enhanced the content of total soluble sugar by 31% and 37% in the heat-sensitive and –tolerant cultivars compared to the control treatment, respectively. Moreover, Micromonospora sp. treatments greatly (p < 0.05) improved total soluble sugar content in both un-stressed and stressed plants, which were 26% and 45% in sensitive cultivar and 31% and 16% in tolerant cultivar higher than those non-inoculated plants under same stress level, respectively (Figure 9). Interestingly, by investigating the three main forms of soluble sugars in plants, a diverse reaction of fructose, glucose, and sucrose was found (Figure 9). Accordingly, fructose content did not show a specific response to stress and bio-inoculation in both cultivars. In contrast, the content of glucose in the sensitive cultivar was significantly (p < 0.05) increased in response to bio-inoculation in both stressed (+28%) and un-stressed (+15%) plants, while increasing only in un-stressed (+36%) tolerant cultivar (p < 0.05). The highest accumulation of sucrose was observed in bio-inoculated plants under stress in both cultivars, which were significantly higher than non-inoculated plants under stress and non-stress conditions, equal to +85% and +134% in sensitive cultivar and +20% and +127% in tolerant cultivar, respectively (Figure 9).
Figure 9. The effect of Micromonospora sp. and heat stress (HS) on sugar content (mg g-1 FW) in two heat-sensitive (Misr-3) and tolerant (Giza-168) cultivars. The means in each parameter and each cultivar with similar small letter(s) are not significantly different at 5% probability level (Tukey HSD test).
The modifications in the content of anthocyanin and the intermediates in their production, as well as some enzymes involved in their synthesis pathway in response to Micromonospora sp. and/or heat stress treatments were evaluated (Table 2). Interestingly, there were differences in the responses of anthocyanins to the treatments in each cultivar. Accordingly, anthocyanin content was increased by Micromonospora sp. in stressed sensitive cultivar (+28%; p < 0.05), while remained unchanged in the tolerant cultivar (+5%; p > 0.05) compared to no inoculation treatment under stress (Table 2). The content of individual phenolic acids, cinnamic acid and coumaric acid, in sensitive cultivar decreased in response to the inoculation treatment under both stress and control treatments, while only coumaric acid showed a similar reaction in the tolerant cultivar (p < 0.05) and no significant difference was observed in the content of cinnamic acid (p > 0.05).
Table 2. The effect of Micromonospora sp. and heat stress (HS) on some phenolic acids (mg g-1 DW), flavonoid compounds (mg g-1 DW), phenylalanine amino acid (mg g-1 protein), phenylalanine amnio lyase (PAL) enzyme activity (nkatal mg-1 protein) and enzymes involved in anthocyanin metabolism (nmol mg-1 protein min-1) in two heat-sensitive (Misr-3) and tolerant (Giza-168) cultivars.
No differences were found in the content of naringenin and the activity 4CL in both cultivars (p > 0.05) (Table 2). Nevertheless, heat stress significantly improved PAL (+15-27%), CHS (+18-21%), and C4H (+57-149%) activities in the tolerant cultivar in both Micromonospora sp. and no inoculation treatments. In contrast, Micromonospora sp. improved PAL and CHS activity in the sensitive cultivar under both stress (+14% and +28%, respectively) and non-stress conditions (+21% and +43%, respectively). Moreover, in addition to the stress, Micromonospora sp. inoculation also improved the C4H activity (+49-85%) in the sensitive cultivar under stress. The highest content of phenylalanine in both cultivars belonged to the bio-inoculation treatment under stress, which was significantly higher than that in no inoculation sensitive (+36%) and tolerant (+53%) cultivars under stress (Table 2).
The current research tried to clear some of the unanswered questions concerning the consequences of global warming on plants, one of which is the rising air temperature level. Accordingly, the responses of two different heat-sensitive and -tolerant cultivars of wheat to heat stress were investigated, especially to show whether the application of a heat-tolerant Micromonospora sp. strain could relieve the adverse effects of stress through the modifications of some of the plant’s biochemical and physiological traits. The results clearly showed that the plant biomass significantly decreased under stress in both cultivars. Moreover, the biomass accumulation in sensitive cultivar significantly improved when expose to Micromonospora sp. inoculation treatment under stress, while the improvement was not observed in the stress-tolerant line. To explain the utilized strategies by plants, we foremost debated the response of photosynthetic traits.
Decline in photo-assimilates synthesizing in the source tissue under heat stress and consequently their lower translocation and accumulation rates in the sink tissue have been considered as the main reason of decreasing in biomass (Kumar et al., 2023). The decrease in the photosynthesis rate under heat stress in both cultivars in the present research has been previously ascribed to the less activity of RuBisCO and consequently, disruption of the chloroplast structure and function (Ashraf, 2022). It has been reported that the rising chlorophyll concentration in stressed plants can protect the function and structure of the chlorophyll system from degradation and disruption (Albqmi et al., 2023). Thus, it can be pointed out that the higher chlorophyll a+b content in the Micromonospora sp.-inoculated plants under stress can be a reason for the higher photosynthesis rate and therefore biomass accumulation in heat-sensitive cultivar compared to that tolerant. Likewise, it has already been reported that more activation of RuBisCO in stressed plants is one of the functional and non-stomatal traits in the Calvin cycle (Acosta-Motos et al., 2017) which in turn can be one of the main causes of improved photosynthetic rate in the sensitive cultivar under stress when treated with Micromonospora sp. Similar findings have already proved that the Micromonospora sp. treatment can boost plant tolerant against stress systematically (Meena et al., 2019) by employing some strategies such as the shift in soil-plant system and affecting supplying/uptaking soil nutrients (Yaghoubi Khanghahi et al., 2018), synthesizing of phytohormones (Yaghoubi Khanghahi et al., 2022b), and modulating the expression of some specific genes (Hagaggi and Mohamed, 2020).
Shift in carbohydrate metabolism was another strategy of stressed plants, particularly when they were treated with Micromonospora sp. An increment in soluble sugar content in heat-stressed plants for osmotic adjustment has been already reported (Harsh et al., 2016). No significant changes in the content of fructose and glucose in the tolerant cultivar may be due to the milder heat stress for this cultivar compared to the sensitive cultivar, as previously reported by Sassi-Aydi et al (Sassi-Aydi et al., 2014). Likewise, differences in the synthesis of individual sugars compared to others under stress have previously been documented (Thompson et al., 2017). In this regard, different responses of hexose sugars (e.g., glucose) and sucrose to environmental stress were attributed to various source and sink activities in plants during the developing stages (Rogers et al., 2004). Glucose, sucrose, and fructose are produced in source organs as the main soluble sugars, among which sucrose is then forwarded to the sink organs (Ma et al., 2021). Nevertheless, the improvement in photosynthesis rate in plants treated with Micromonospora sp. under stress compared to those non-inoculated, as shown in Figure 2, maybe the primary reason for an increase in carbohydrate synthesis and accordingly for adaptations in carbon metabolism (Thompson et al., 2017). Similarly, higher photosynthesis rate and activation of RuBisCO are considered the main factors improving carboxylation rate under stress (Drake et al., 1997), as was observed in the present study in the Micromonospora sp.-inoculated plants (sensitive cultivar) in stress conditions.
More concentrations of oxidative damage markers, including MDA in heat-stressed plants can represent a high level of ROS and consequently oxidative stress (Liu et al., 2020). This finding confirms the reports of previous research, in which oxidative stress was observed in heat-stressed plants, principally since the high level of such oxidative markers can surpass the valence of the antioxidant enzymatic reservoir (Hassan et al., 2020; Fortunato et al., 2023). Moreover, an obvious reduction tendency in the production of these oxidative damage markers was observed in Micromonospora sp. inoculation under stress, particularly in the sensitive cultivar (Figure 4). This result confirms the results of a great deal of the earlier studies, in which the favorable consequences of Micromonospora sp. inoculation in diminishing the accumulation of oxidative markers in heat-stressed plants have been reported (Khan et al., 2020b; Khan et al., 2020a). It seems possible that these results are due to the higher detoxification of ROS in plants treated with Micromonospora sp. under stress, in which the antioxidant molecules and enzymes were more activated compared to non-inoculated stressed plants.
It has been proved that the detoxification of overproduced ROS in plants is attributed to the activation of the antioxidant defense systems (Zarbakhsh et al., 2020; Shabbaj et al., 2022), particularly in Micromonospora sp.-treated plants (Madnay et al., 2022). Similar reports indicated a greater activity of direct ROS-detoxifying enzymes (CAT, SOD and POX) and those metabolites and enzymes in the ASC/GSH and catalytic pathways (GSH, ASC, Trxs, APX, GR, DHAR, GPX, Grxs, and Prxs) can alleviate the adverse effects of heat stress (Albqmi et al., 2023; Zarbakhsh et al., 2024). In this regard, the response of non-enzymatic component of the ASC-GSH pathway (ASC and GSH) in Micromonospora sp. treatment in the current research, agreed with the activation of involved enzymes, mainly APX, which catalyze the reduction of H2O2 into H2O (Madnay et al., 2022; Zarbakhsh et al., 2024). Similarly, activating the ASC-GSH pathway in PGPB treatment was assumed a primary approach for detoxifying ROS and preserving plant cells from oxidative damage (Carreiras et al., 2023; Zarbakhsh et al., 2024).
Our results also indicated the highest levels of total antioxidant capacity and antioxidant molecules (total flavonoids, polyphenols and total tocopherols) in heat-stressed plants, particularly in Micromonospora sp. treatment. These findings agree with the findings of other research, in which a high content of antioxidant metabolites in Micromonospora sp.-inoculated plants under different environmental stress has been reported as an adaptation strategy of plants under stress conditions (Yaghoubi Khanghahi et al., 2022a; Sarraf et al., 2023; Hagagy and AbdElgawad, 2024), in particular via the preserving the photosynthetic apparatus (Albqmi et al., 2023). The observed increase in these antioxidant metabolites content in Micromonospora sp. -treated plants could be attributed to the higher content of phenylalanine. This amino acid has an essential role as a precursor of many molecules in defense systems against environmental stress such as flavonoids, polyphenols, and anthocyanins (Pascual et al., 2016; Shomali et al., 2022). Moreover, the responses of phenylalanine and anthocyanins in this study corroborate the shifts in the activity of the phenylalanine ammonia-lyase (PAL) enzyme, which is responsible for catalyzing the deamination of phenylalanine as the foremost and rate-limiting stage of the phenylpropanoid pathway at the regulation point between primary and secondary metabolites (Fan et al., 2022). The similar reaction of cinnamate (CA) 4-hydroxylase (C4H), the enzyme catalyzing the second step of the phenylpropanoid pathway (Schoch et al., 2002), to the Micromonospora sp. treatment in the sensitive cultivar, and the heat stress in the tolerant cultivar (Table 2) confirms the activation of this pathway in the synthesizing of defense molecules. Similarly, it is clear that Micromonospora sp. could improve chalcone synthase (CHS) activity in the sensitive cultivar, which is known for its function as a major hub for the employed enzymes in the flavonoid synthesis pathway (Crosby et al., 2011). On the other hand, it seems possible that the heat stress alone in the tolerant cultivar was enough stimulus to increase the activity of PAL and CHS enzymes, the stimulating effect that was not observed in the sensitive cultivar. The lack of significant changes in naringenin content, as a product of the flavonoid pathway, in both cultivars can indicate the lack of non-competitively inhibition by this pathway in increasing the enzyme activity (Hinderer and Seitz, 1985), since it is believed that high accumulation of naringenin in the cytosol can limit CHS activity to avoid toxic levels in the cells (Whitehead and Dixon, 1983). The findings represented the regulative role of these detoxifying metabolites in the present study, particularly in Micromonospora sp. treatment, as one of the leading defense mechanisms under heat stress.
In conclusion, this study demonstrates that plant growth-promoting bacteria (Micromonospora sp.), play a significant role in mitigating the adverse effects of heat stress on wheat plants by enhancing photosynthetic efficiency, antioxidant defense, and metabolic adaptations. The sensitive wheat cultivar, under heat stress, exhibited substantial improvements in photosynthesis rate, chlorophyll content, RuBisCO activity, and biomass production when treated with Micromonospora sp., compared to untreated stressed plants. Additionally, Micromonospora sp. treatment notably reduced oxidative stress markers and enhanced the activity of antioxidant enzymes and the ASC-GSH pathway enzymes. The increased levels of antioxidant proteins, metabolites, and the activation of the phenylpropanoid pathway further contributed to reducing oxidative damage. These findings underscore the potential of utilizing Micromonospora sp. to develop heat-resilient wheat cultivars, offering a promising strategy for sustainable agriculture in the face of rising global temperatures. However, the study has limitation such as the experiment was conducted in a lab setting using sterile soil, which does not fully replicate the complex microbial interactions present in natural soil environments. The use of sterilized soil may oversimplify the interactions between Micromonospora sp. and wheat plants, as native soil microorganisms could significantly impact the efficacy of Micromonospora sp. treatments in field conditions. Future studies should also consider employing advanced genomic and transcriptomic approaches to elucidate the pathways influenced by bacterial inoculation. Finally, long-term effects of bacterial treatments on wheat resilience under varying environmental conditions have not been fully explored, highlighting the importance of conducting extended field trials to assess the durability of these treatments.
The data presented in this study have been deposited in GenBank (NCBI) with the following accession number: PQ670967.
AH: Conceptualization, Methodology, Investigation, Formal analysis, Supervision, Writing – original draft, Writing – review & editing. EA: Conceptualization, Methodology, Investigation, Formal analysis, Supervision, Writing – original draft, Writing – review & editing. HA: Conceptualization, Methodology, Investigation, Formal analysis, Supervision, Writing – original draft, Writing – review & editing. MS: Investigation, Data curation, Software, Methodology, Resources, Writing – review & editing. YA: Investigation, Data curation, Software, Methodology, Resources, Writing – review & editing. MO: Investigation, Data curation, Software, Methodology, Resources, Writing – review & editing. AA: Data curation, Formal analysis, Project administration, Visualization, Validation, Supervision, Writing – review & editing. MM-Z: Data curation, Formal analysis, Project administration, Visualization, Validation, Supervision, Writing – review & editing. IA: Conceptualization, Data curation, Formal analysis, Methodology, Investigation, Funding acquisition, Validation, Writing – review & editing. AR: Conceptualization, Data curation, Formal analysis, Methodology, Investigation, Funding acquisition, Validation, Writing – review & editing.
The authors extend their appreciation to the Researchers Supporting Project number (RSP2024R176) King Saud University, Riyadh, Saud Arabia.
The authors extend their appreciation to the Researchers Supporting Project number (RSP2024R176) King Saud University, Riyadh, Saud Arabia.
The authors declare that the research was conducted in the absence of any commercial or financial relationships that could be construed as a potential conflict of interest.
All claims expressed in this article are solely those of the authors and do not necessarily represent those of their affiliated organizations, or those of the publisher, the editors and the reviewers. Any product that may be evaluated in this article, or claim that may be made by its manufacturer, is not guaranteed or endorsed by the publisher.
The Supplementary Material for this article can be found online at: https://www.frontiersin.org/articles/10.3389/fpls.2024.1500894/full#supplementary-material
AbdElgawad, H., De Vos, D., Zinta, G. (2015). Grassland species differentially regulate proline concentrations under future climate conditions: An integrated biochemical and modelling approach. New Phytol. 208, 354–369. doi: 10.1111/nph.13481
AbdElgawad, H., Magdy Korany, S., Hagagy, N., Yaghoubi Khanghahi, M., Reyad, A. M., Crecchio, C., et al. (2023). Biochemical and pharmaceutical traits of Marrubium vulgare L. plants treated with plant growth-promoting bacteria and elevated CO2. 3 Biotech. 13, 412. doi: 10.1007/s13205-023-03836-0
AbdElgawad, H., Zinta, G., Hegab, M. M., Pandey, R., Asard, H., Abuelsoud, W. (2016). High salinity induces different oxidative stress and antioxidant responses in maize seedlings organs. Front. Plant Sci. 7. doi: 10.3389/fpls.2016.00276
Acosta-Motos, J. R., Ortuño, M. F., Bernal-Vicente, A., Diaz-Vivancos, P., Sanchez-Blanco, M. J., Hernandez, J. A. (2017). Plant responses to salt stress: Adaptive mechanisms. Agronomy 7, 18. doi: 10.3390/agronomy7010018
Albqmi, M., Selim, S., Yaghoubi Khanghahi, M., Crecchio, C., Al-Sanea, M. M., Alnusaire, T. S., et al. (2023). Chromium(VI) toxicity and active tolerance mechanisms of wheat plant treated with plant growth-promoting actinobacteria and olive solid waste. ACS Omega 8, 32458–32467. doi: 10.1021/acsomega.3c02447
Allina, S. M., Pri-Hadash, A., Theilmann, D. A., Ellis, B. E., Douglas, C. J. (1998). 4-Coumarate:coenzyme A ligase in hybrid poplar. Properties of native enzymes, cDNA cloning, and analysis of recombinant enzymes. Plant Physiol. 116, 743–754. doi: 10.1104/pp.116.2.743
Ashraf, M. (2022). Thermotolerance in plants: Potential physio-biochemical and molecular markers for crop improvement. Environ. Exp. Bot. 186, 104454. doi: 10.1016/j.envexpbot.2021.104454
Asseng, S., Ewert, F., Martre, P., Rotter, R. P., Lobell, D. B., Cammarano, D. (2015). Rising temperatures reduce global wheat production. Nat. Clim. Chang 5, 143–147. doi: 10.1038/nclimate2470
Athukorala, Y., Lee, K. W., Song, C., Ahn, C. B., Shin, T. S., Cha, Y. J., et al. (2023). Potential antioxidant activity of marine red alga Grateloupia filicina extracts. J. Food Lipids 10, 251–265. doi: 10.1111/j.1745-4522.2003.tb00019.x
Barakate, M., Ouhdouch, Y., Oufdou, K. (2022). Characterization of rhizospheric soil streptomycetes from Moroccan habitatsand their antimicrobial activities. World J. Microbiol. Biotechnol. 18, 49–54. doi: 10.1023/A:1013966407890
Benzie, I. F., Strain, J. (1999). Ferric reducing/antioxidant power assay: Direct measure of total antioxidant activity of biological fluids and modified version for simultaneous measurement of total antioxidant power and ascorbic acid concentration. Methods Enzymol. 299, 15–27. doi: 10.1016/S0076-6879(99)99005-5
Carreiras, J., Cruz-Silva, A., Fonseca, B., Carvalho, R. C., Cunha, J. P., Proença Pereira, J., et al. (2023). Improving grapevine heat stress resilience with marine plant growth-promoting rhizobacteria consortia. Microorganisms 11, 856. doi: 10.3390/microorganisms11040856
Chang, C.-C., Yang, M.-H., Wen, H.-M., Chern, J.-C. (2022). Estimation of total flavonoid content in propolis by two complementary colorimetric methods. J. Food Drug Anal. 10, 3. doi: 10.38212/2224-6614.2748
Crosby, K. C., Pietraszewska-Bogiel, A., Gadella, T. W., Winkel, B. S. (2011). Förster resonance energy transfer demonstrates a flavonoid metabolon in living plant cells that displays competitive interactions between enzymes. FEBS Lett. 585, 2193–2198. doi: 10.1016/j.febslet.2011.05.066
Dhindsa, R. S., Plumb-Dhindsa, P. L., Reid, D. M. (1982). Leaf senescence and lipid peroxidation: Effects of some phytohormones and scavengers of free radicals and singlet oxygen. Physiol. Plant 56, 453–457. doi: 10.1111/j.1399-3054.1982.tb04539.x
Drake, B. G., Gonzàlez-Meler, M. A., Long, S. P. (1997). More efficient plants: A consequence of rising atmospheric CO2? Annu. Rev. Plant Physiol. Plant Mol. Biol. 48, 609–639. doi: 10.1146/annurev.arplant.48.1.609
Drotar, A., Phelps, P., Fall, R. (1985). Evidence for glutathione peroxidase activities in cultured plant cells. Plant Sci. 42, 35–40. doi: 10.1016/0168-9452(85)90025-1
El-Sawah, A. M., Abdel-Fattah, G. G., Holford, P., Korany, S. M., Alsherif, E. A., AbdElgawad, H., et al. (2023). Funneliformis constrictum modulates polyamine metabolism to enhance tolerance of Zea mays L. @ to salinity. Microbiol. Res. 266, 127254. doi: 10.1016/j.micres.2022.127254
El-Tarabily, K. A., Nassar, A. H., Sivasithamparam, K. (2008). Promotion of growth of bean (Phaseolus vulgaris L.) in a calcareous soil by a phosphate-solubilizing, rhizosphere-competent isolate of Micromonospora endolithica. Appl. Soil Ecol. 39, 161–171. doi: 10.1016/j.apsoil.2007.12.005
Fan, L., Shi, G., Yang, J., Liu, G., Niu, Z., Ye, W., et al. (2022). A protective role of phenylalanine ammonia-lyase from Astragalus membranaceus against saline-alkali stress. Int. J. Mol. Sci. 23, 15686. doi: 10.3390/ijms232415686
Fletcher, R. A., Kallumbil, V., Steele, P. (1982). An improved bioassay for cytokinins using cucumber cotyledons. Plant Physiol. 69, 675–677. doi: 10.1104/pp.69.3.675
Fortunato, S., Lasorella, C., Dipierro, N., Vita, F., de Pinto, M. C. (2023). Redox signaling in plant heat stress response. Antioxidants 12, 605. doi: 10.3390/antiox12030605
Foyer, C. H., Lopez-Delgado, H., Dat, J. F., Scott, I. M. (1997). Hydrogen peroxide-and glutathione-associated mechanisms of acclimatory stress tolerance and signalling. Physiol. Plant 100, 241–254. doi: 10.1111/j.1399-3054.1997.tb04780.x
Gui, Y., Sheteiwy, M. S., Zhu, S., Zhu, L., Batool, A., Jia, T., et al. (2021). Differentiate responses of tetraploid and hexaploid wheat (Triticum aestivum L.) to moderate and severe drought stress: A cue of wheat domestication. Plant Signal. Behav. 16, 1839710. doi: 10.1080/15592324.2020.1839710
Hagaggi, N. S. A., Mohamed, A. A. A. (2020). Enhancement of Zea mays (L.) growth performance using indole acetic acid producing endophyte Mixtatheicola isolated from Solenostemma argel (Hayne). S. Afr. J. Bot. 134, 64–71. doi: 10.1016/j.sajb.2020.02.034
Hagagy, N., AbdElgawad, H. (2024). Rapeseed plant: Biostimulation effects of plant growth-promoting Actinobacteria on metabolites and antioxidant defense system under elevated CO2 conditions. J. Sci. Food Agric. 104, 51–62. doi: 10.1002/jsfa.12909
Hamad, I., AbdElgawad, H., Al-Jaouni, S., Zinta, G., Asard, H., Hassan, S., et al. (2015). Metabolic analysis of various date palm fruit (Phoenix dactylifera L.) cultivars from Saudi Arabia to assess their nutritional quality. Molecules 20, 13620. doi: 10.3390/molecules200813620
Harsh, A., Sharma, Y. K., Joshi, U., Rampuria, S., Singh, G., Kumar, S., et al. (2016). Effect of short-term heat stress on total sugars, proline and some antioxidant enzymes in moth bean (Vigna aconitifolia). Ann. Agric. Sci. 61, 57–64. doi: 10.1016/j.aoas.2016.02.001
Hartley-Whitaker, J., Ainsworth, G., Vooijs, R., Bookum, W. T., Schat, H., Meharg, A. A. (2001). Phytochelatins are involved in differential arsenate tolerance in Holcus lanatus. Plant Physiol. 126, 299–306. doi: 10.1104/pp.126.1.299
Hassan, A. H. A., Hozzein, W. N., Mousa, A. S. M. (2020). Heat stress as an innovative approach to enhance the antioxidant production in Pseudooceanicola and Bacillus isolates. Sci. Rep. 10, 15076. doi: 10.1038/s41598-020-72054-y
Hinderer, W., Seitz, H. U. (1985). Chalcone synthase from cell suspension cultures of Daucus carota L. Arch. Biochem. Biophys. 240, 265–272. doi: 10.1016/0003-9861(85)90032-3
Hodges, D. M., Delong, J. M., Forney, C. F., Prange, R. K. (1999). Improving the thiobarbituric acid-reactive-substances assay for estimating lipid peroxidation in plant tissues containing anthocyanin and other interfering compounds. Planta 207, 604–611. doi: 10.1007/s004250050524
Holder, I., Boyce, S. (1994). Agar well diffusion assay testing of bacterial susceptibility to various antimicrobials in concentrations non-toxic for human cells in culture. Burns 20, 426–429. doi: 10.1016/0305-4179(94)90035-3
Hong, K., Gao, A. H., Xie, Q. Y. (2009). Actinomycetes for marine drug discovery isolated from mangrove soils and plants in China. Mar. Drugs 7, 24–44. doi: 10.3390/md7010024
Huang, B., Liu, X., Fry, J. D. (1998). Shoot physiological responses of two bentgrass cultivars to high temperature and poor soil aeration. Crop Sci. 38, 1219–1224. doi: 10.2135/cropsci1998.0011183X003800050018x
Hussain, S. S., Mudasser, M. (2007). Prospects for wheat production under changing climate in mountain areas of Pakistan—An econometric analysis. Agric. Syst. 94, 494–501. doi: 10.1016/j.agsy.2006.12.001
IPCC (2023). “Summary for Policymakers,” in Climate Change 2023: Synthesis Report; Core Writing Team. Eds. Lee, H., Romero, J. (IPCC, Geneva, Switzerland). doi: 10.59327/IPCC/AR6-9789291691647.001
Jadhav, P. R., Mahatma, M. K., Jha, S., Mahatma, L., Parekh, V. B., Jha, S. K. (2013). Changes in the phenylpropanoid pathway during the compatible and incompatible interaction of Ricinus communis-Fusarium oxysporum f.sp. ricini. Ind. J. Agric. Biochem. 26, 56–60.
Khan, M. A., Asaf, S., Khan, A. L. (2020a). Thermotolerance effect of plant growth-promoting Bacillus cereus SA1 on soybean during heat stress. BMC Microbiol. 20, 175. doi: 10.1186/s12866-020-01822-7
Khan, M. A., Asaf, S., Khan, A. L., Jan, R., Kang, S.-M., Kim, K.-M. (2020b). Extending thermotolerance to tomato seedlings by inoculation with SA1 isolate of Bacillus cereus and comparison with exogenous humic acid application. PloS One 15, e0232228. doi: 10.1371/journal.pone.0232228
Kocsy, G., Szalai, G., Sutka, J., Páldi, E., Galiba, G. (2004). Heat tolerance together with heat stress-induced changes in glutathione and hydroxymethylglutathione levels is affected by chromosome 5A of wheat. Plant Sci. 166, 451–458. doi: 10.1016/j.plantsci.2003.10.011
Kumar, S., Bhushan, B., Wakchaure, G. C., Dutta, R., Jat, B. S., Meena, K. K., et al. (2023). Unveiling the impact of heat stress on seed biochemical composition of major cereal crops: Implications for crop resilience and nutritional value. Plant Stress 9, 100183. doi: 10.1016/j.stress.2023.100183
Kumar, A., Dubey, A. (2020). Rhizosphere microbiome: Engineering bacterial competitiveness for enhancing crop production. J. Adv. Res. 24, 337–352. doi: 10.1016/j.jare.2020.04.014
Kumar, K., Khan, P. (1983). Age-related changes in catalase and peroxidase activities in the excised leaves of Eleusine coracana Gaertn. cv PR 202 during senescence. Exp. Gerontol. 18, 409–417. doi: 10.1016/0531-5565(83)90019-0
Küster, E., Williams, S. T. (1964). Selection of media for isolation of streptomycetes. Nature 202, 928–929. doi: 10.1038/202928a0
Larkindale, J., Huang, B. (2004). Changes of lipid composition and saturation level in leaves and roots for heat-stressed and heat-acclimated creeping bentgrass (Agrostis stolonifera). Environ. Exp. Bot. 51, 57–67. doi: 10.1016/S0098-8472(03)00060-1
Lichtenthaler, H. K., Buschmann, C. (2001). Chlorophylls and carotenoids: Measurement and characterization by UV‐VIS spectroscopy. Curr. Protoc. Food Anal. Chem. 1 (1), F4–3. doi: 10.1002/0471142913.faf0403s01
Liu, Z., Li, G., Zhang, H., Zhang, Y., Zhang, Y., Duan, S., et al. (2020). TaHsfA2-1, a new gene for thermotolerance in wheat seedlings: Characterization and functional roles. J. Plant Physiol. 246–247, 153135. doi: 10.1016/j.jplph.2020.153135
Lobo, A. K. M., de Oliveira Martins, M., Neto, M. C. L., MaChado, E. C., Ribeiro, R. V., Silveira, J. A. G. (2015). Exogenous sucrose supply changes sugar metabolism and reduces photosynthesis of sugarcane through down-regulation of Rubisco abundance and activity. J. Plant Physiol. 179, 113–121. doi: 10.1016/j.jplph.2015.03.007
Lundberg, M., Johansson, C., Chandra, J., Enoksson, M., Jacobsson, G., Ljung, J., et al. (2001). Cloning and expression of a novel human glutaredoxin (Grx2) with mitochondrial and nuclear isoforms. J. Biol. Chem. 276, 26269–26275. doi: 10.1074/jbc.M011605200
Ma, Y., Xie, Y., Ha, R., Cao, B., Song, L. (2021). Effects of elevated CO2 on photosynthetic accumulation, sucrose metabolism-related enzymes, and genes identification in Goji berry (Lycium barbarum L.). Front. Plant Sci. 12. doi: 10.3389/fpls.2021.643555
Madnay, M., Obaid, W., Selim, S., Alsherif, E., Korany, S., Abdel-Mawgoud, M., et al. (2022). Rhodospirillum sp. JY3: An innovative tool to mitigate the phytotoxic impact of galaxolide on wheat (Triticum aestivum) and faba bean (Vicia faba) plants. Front. Plant Sci. 13, 1037474. doi: 10.3389/fpls.2022.1037474
Mandon, K., Nazaret, F., Farajzadeh, D., Alloing, G., Frendo, P. (2021). Redox regulation in diazotrophic bacteria in interaction with plants. Antioxidants 10, 880. doi: 10.3390/antiox10060880
Meena, M. G., Yadav, R. K., Narjary, B., Yadav, G., Jat, H. S., Sheoran, P., et al. (2019). Municipal solid waste (MSW): Strategies to improve salt affected soil sustainability: A review. Waste Manage. 84, 38–53. doi: 10.1016/j.wasman.2018.11.020
Murshed, R., Lopez-Lauri, F., Sallanon, H. (2008). Microplate quantification of enzymes of the plant ascorbate–glutathione cycle. Anal. Biochem. 383, 320–322. doi: 10.1016/j.ab.2008.07.020
Nakano, Y., Asada, K. (1981). Hydrogen peroxide is scavenged by ascorbate-specific peroxidase in spinach chloroplasts. Plant Cell Physiol. 22, 867–880.
Notununu, I., Moleleki, L., Roopnarain, A., Adeleke, R. (2022). Effects of plant growth-promoting rhizobacteria on the molecular responses of maize under drought and heat stresses: A review. Pedosphere 32, 90–106. doi: 10.1016/S1002-0160(21)60051-6
Omar, A. A., Heikal, Y. M., Zayed, E. M., Shamseldin, S. A. M., Salama, Y. E., Amer, K. E., et al. (2023). Conferring of drought and heat stress tolerance in wheat (Triticum aestivum L.) genotypes and their response to selenium nanoparticles application. Nanomaterials 13, 998. doi: 10.3390/nano13060998
Ortúzar, M., Trujillo, M. E., Román-Ponce, B., Carro, L. (2020). Micromonospora metallophores: A plant growth promotion trait useful for bacterial-assisted phytoremediation? Sci. Total Environ. 739, 139850. doi: 10.1016/j.scitotenv.2020.139850
Pankievicz, V. C. S., Do Amaral, F. P., Ané, J.-M., Stacey, G. (2021). Diazotrophic bacteria and their mechanisms to interact and benefit cereals. Mol. Plant-Microbe Interact. 34, 491–498. doi: 10.1094/MPMI-11-20-0316-FI
Pascual, M. B., El-Azaz, J., de la Torre, F. N., Cañas, R. A., Avila, C., Cánovas, F. M. (2016). Biosynthesis and metabolic fate of phenylalanine in conifers. Front. Plant Sci. 7. doi: 10.3389/fpls.2016.01030
Potters, G., Horemans, N., Bellone, S., Caubergs, R. J., Trost, P., Guisez, Y., et al. (2004). Dehydroascorbate influences the plant cell cycle through a glutathione-independent reduction mechanism. Plant Physiol. 134, 1479–1487. doi: 10.1104/pp.103.033548
Rogers, A., Allen, D. J., Davey, P. A. (2004). Leaf photosynthesis and carbohydrate dynamics of soybeans grown throughout their life cycle under free-air carbon dioxide enrichment. Plant Cell Environ. 27, 449–458. doi: 10.1111/j.1365-3040.2004.01163.x
Sarraf, M., Janeeshma, E., Arif, N., QudratUllahFarooqi, M., Kumar, V., Ansari, N. A., et al. (2023). Understanding the role of beneficial elements in developing plant stress resilience: Signalling and crosstalk with phytohormones and microbes. Plant Stress 10, 100224. doi: 10.1016/j.stress.2023.100224
Sassi-Aydi, S., Aydi, S., Abdelly, C. (2014). Inorganic nitrogen nutrition enhances osmotic stress tolerance in Phaseolus vulgaris: Lessons from a drought-sensitive cultivar. Horticulturae 49, 550–555.
Schlesier, K., Harwat, M., Böhm, V. (2002). Assessment of antioxidant activity by using different in vitro methods. Free Radical Res. 36, 177–187. doi: 10.1080/10715760290006411
Schoch, G. A., Nikov, G. N., Alworth, W. L., Werck-Reichhart, D. (2002). Chemical inactivation of the cinnamate 4-hydroxylase allows for the accumulation of salicylic acid in elicited cells. Plant Physiol. 130, 1022–1031. doi: 10.1104/pp.004309
Shabbaj, I. I., Abdelgawad, H., Balkhyour, M. A., Tammar, A., Madany, M. M. Y. (2022). Elevated CO2 differentially mitigated oxidative stress induced by indium oxide nanoparticles in young and old leaves of C3 and C4 crops. Antioxidants 11, 308. doi: 10.3390/antiox11020308
Sheteiwy, M. S., El-Sawah, A. M., Korany, S. M., Alsherif, E. A., Mowafy, A. M., Chen, J., et al. (2022). Arbuscular mycorrhizal fungus “Rhizophagus irregularis” impacts on physiological and biochemical responses of ryegrass and chickpea plants under beryllium stress. Environ. pollut. 315, 120356. doi: 10.1016/j.envpol.2022.120356
Sheteiwy, M. S., Shaghaleh, H., Hamoud, Y. A., Holford, P., Shao, H., Qi, W., et al. (2021). Zinc oxide nanoparticles: Potential effects on soil properties, crop production, food processing, and food quality. Environ. Sci. pollut. Res. 28, 36942–36966. doi: 10.1007/s11356-021-14542-w
Shomali, A., Das, S., Arif, N., Sarraf, M., Zahra, N., Yadav, V., et al. (2022). Diverse physiological roles of flavonoids in plant environmental stress responses and tolerance. Plants 11, 3158. doi: 10.3390/plants11223158
Thompson, M., Gamage, D., Hirotsu, N. (2017). Effects of elevated carbon dioxide on photosynthesis and carbon partitioning: A perspective on root sugar sensing and hormonal crosstalk. Front. Physiol. 8. doi: 10.3389/fphys.2017.00578
Trujillo, M. E., Bacigalupe, R., Pujic, P., Igarashi, Y., Benito, P., Riesco, R., et al. (2014). Genome features of the endophytic actinobacterium Micromonospora lupini strain Lupac 08: On the process of adaptation to an endophytic life style? PloS One 9, e108522. doi: 10.1371/journal.pone.0108522
Ullah, A., Nadeem, F., Nawaz, A., Siddique, K. H., Farooq, M. (2022). Heat stress effects on the reproductive physiology and yield of wheat. J. Agron. Crop Sci. 208, 1–17. doi: 10.1111/jac.v208.1
Wagner, G. J. (1979). Content and vacuole/extravacuole distribution of neutral sugars, free amino acids, and anthocyanin in protoplasts. Plant Physiol. 64, 88–93. doi: 10.1104/pp.64.1.88
Whitehead, J. M., Dixon, R. A. (1983). Chalcone synthase from cell suspension cultures of Phaseolus vulgaris L. Biochim. Biophys. Acta 747, 298–303. doi: 10.1016/0167-4838(83)90109-7
Wolosiuk, R. A., Crawford, N. A., Yee, B. C., Buchanan, B. B. (1979). Isolation of three thioredoxins from spinach leaves. J. Biol. Chem. 254, 1627–1632. doi: 10.1016/S0021-9258(17)37818-3
Yaghoubi Khanghahi, M., AbdElgawad, H., Verbruggen, E., Korany, S. M., Alsherif, E. A., Beemster, G. T. S., et al. (2022a). Biofertilisation with a consortium of growth-promoting bacterial strains improves the nutritional status of wheat grain under control, drought, and salinity stress conditions. Physiol. Plant 174 (6), e13800. doi: 10.1111/ppl.13800
Yaghoubi Khanghahi, M., Crecchio, C., Verbruggen, E. (2022b). Shifts in the rhizosphere and endosphere colonizing bacterial communities under drought and salinity stress as affected by a biofertilizer consortium. Microb. Ecol. 84, 483–495. doi: 10.1007/s00248-021-01856-y
Yaghoubi Khanghahi, M., Pirdashti, H., Rahimian, H., Nematzadeh, G. A., Ghajar Sepanlou, M. (2018). Nutrient use efficiency and nutrient uptake promoting of rice by potassium solubilizing bacteria (KSB). Cereal Res. Commun. 46, 739–750. doi: 10.1556/0806.46.2018.042
Yaghoubi Khanghahi, M., Strafella, S., Crecchio, C. (2020). Changes in photo-protective energy dissipation of photosystem II in response to beneficial bacteria consortium in durum wheat under drought and salinity stresses. Appl. Sci. 10, 5031. doi: 10.3390/app10155031
Yaghoubi Khanghahi, M., Strafella, S., Filannino, P., Minervini, F., Crecchio, C. (2024). Importance of lactic acid bacteria as an emerging group of plant growth-promoting rhizobacteria in sustainable agroecosystems. Appl. Sci. 14, 1798. doi: 10.3390/app14051798
Yang, H., Fang, C., Li, Y., Wu, Y., Fransson, P., Rillig, M. C., et al. (2022). Temporal complementarity between roots and mycorrhizal fungi drives wheat nitrogen use efficiency. New Phytol. 236, 1168–1181. doi: 10.1111/nph.1841
Zadel, U., Nesme, J., Michalke, B., Vestergaard, G., Płaza, G. A., Schröder, P., et al. (2020). Changes induced by heavy metals in the plant-associated microbiome of Miscanthus x giganteus. Sci. Total Environ. 711, 134433. doi: 10.1016/j.scitotenv.2019.134433
Zarbakhsh, S., Kazemzadeh-Beneh, H., Rastegar, S. (2020). Quality preservation of minimally processed pomegranate cv. Jahrom arils based on chitosan and organic acid edible coatings. J. Food Saf. 40, e12752. doi: 10.1111/jfs.12752
Zarbakhsh, S., Shahsavar, A. R., Afaghi, A., Hasanuzzaman, M. (2024). Predicting and optimizing reactive oxygen species metabolism in Punica granatum L. through machine learning: Role of exogenous GABA on antioxidant enzyme activity under drought and salinity stress. BMC Plant Biol. 24, 65. doi: 10.1186/s12870-024-04740-2
Zhang, Q., Zhang, J., Shen, J., Silva, A., Dennis, D. A., Barrow, C. J. (2006). A simple 96-well microplate method for estimation of total polyphenol content in seaweeds. J. Appl. Phycol 18, 445–450. doi: 10.1007/s10811-006-9048-4
Zhao, K., Tao, Y., Liu, M., Yang, D., Zhu, M., Ding, J., et al. (2022). Does temporary heat stress or low temperature stress similarly affect yield, starch, and protein of winter wheat grain during grain filling? J. Cereal Sci. 103, 103408. doi: 10.1016/j.jcs.2021.103408
Keywords: wheat cultivars, heat stress, sugars, anthocyanin metabolism, phenylpropanoid pathways, ASC/GSH cycle 2
Citation: Hassan AHA, Ahmed ES, Sheteiwy MS, Alhaj Hamoud Y, Okla MK, AlGarawi AM, Maridueña-Zavala MG, Alaraidh IA, Reyad AM and Abdelgawad H (2024) Inoculation with Micromonospora sp. enhances carbohydrate and amino acid production, strengthening antioxidant metabolism to mitigate heat stress in wheat cultivars. Front. Plant Sci. 15:1500894. doi: 10.3389/fpls.2024.1500894
Received: 24 September 2024; Accepted: 15 November 2024;
Published: 19 December 2024.
Edited by:
Sabine Dagmar Zimmermann, IPSiM Institute of Plant Science in Montpellier CNRS UMR5004, FranceReviewed by:
Jonathan Rojas, Instituto Tecnológico de Sonora (ITSON), MexicoCopyright © 2024 Hassan, Ahmed, Sheteiwy, Alhaj Hamoud, Okla, AlGarawi, Maridueña-Zavala, Alaraidh, Reyad and Abdelgawad. This is an open-access article distributed under the terms of the Creative Commons Attribution License (CC BY). The use, distribution or reproduction in other forums is permitted, provided the original author(s) and the copyright owner(s) are credited and that the original publication in this journal is cited, in accordance with accepted academic practice. No use, distribution or reproduction is permitted which does not comply with these terms.
*Correspondence: Maria Gabriela Maridueña-Zavala, Z21hcmlkdWVAZXNwb2wuZWR1LmVj
Disclaimer: All claims expressed in this article are solely those of the authors and do not necessarily represent those of their affiliated organizations, or those of the publisher, the editors and the reviewers. Any product that may be evaluated in this article or claim that may be made by its manufacturer is not guaranteed or endorsed by the publisher.
Research integrity at Frontiers
Learn more about the work of our research integrity team to safeguard the quality of each article we publish.