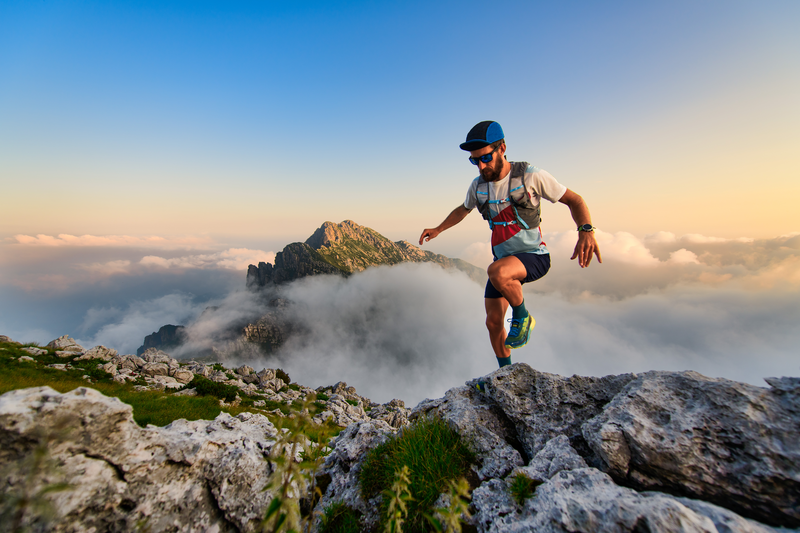
94% of researchers rate our articles as excellent or good
Learn more about the work of our research integrity team to safeguard the quality of each article we publish.
Find out more
ORIGINAL RESEARCH article
Front. Plant Sci. , 14 October 2024
Sec. Plant Abiotic Stress
Volume 15 - 2024 | https://doi.org/10.3389/fpls.2024.1482351
This article is part of the Research Topic Mechanisms of Stress Tolerance in Horticultural Crops: Physiological and Molecular Insights View all 11 articles
Nitrogen (N) is an essential element for plant growth, development, and metabolism. In apple production, the excessive use of N fertilizer may cause high N stress. Whether high N stress can be alleviated by regulating melatonin supply is unclear. The effects of melatonin on root morphology, antioxidant enzyme activity and 13C and 15N accumulation in apple rootstock M9T337 treated with high N were studied by soil culture. The results showed that correctly raising the melatonin supply level is helpful to root development of M9T337 rootstock under severe N stress. Compared with HN treatment, HN+MT treatment increased root and leaf growth by 11.38%, and 28.01%, respectively. Under high N conditions, appropriately increasing melatonin level can activate antioxidant enzyme activity, reduce lipid peroxidation in roots, protect root structural integrity, promote the transport of sorbitol and sucrose to roots, and promote further degradation and utilization of sorbitol and sucrose in roots, which is conducive to the accumulation of photosynthetic products, thereby reducing the inhibitory effect of high N treatment on root growth. Based on the above research results, we found that under high N stress, melatonin significantly promotes nitrate absorption, enhances N metabolism enzyme activity, and upregulates related gene expression, and regulate N uptake and utilization in the M9T337 rootstock. These results presented a fresh notion for improving N application and preserving carbon-nitrogen balance.
Nitrogen (N) is an essential nutrient for plants (Krapp, 2015). It has multiple functions in plant metabolic processes and is pivotal for plant growth (Cunha et al., 2015; Mu and Chen, 2021). The application of N fertilizer can greatly increase grain yield (Abbaraju et al., 2022). As the potential for N fertilizer to increase yield is high and the price of N fertilizer is relatively low, farmers often overuse N fertilizer to maintain high yields (Wang et al., 2021). However, at present, the irrational application of N fertilizer leads to a low fertilizer utilization rate, and 60% of the fertilizer cannot be absorbed by crops, which increases the emissions of methane (CH4) and nitrous oxide (N2O) (Tian et al., 2020). It is reported that the world uses about 110 Tg (1 Tg = 1 million tons) of chemical fertilizer every year to meet the growing demand for food (Chen et al., 2020). In the past half a century, the total amount of N discharged into the environment by humans exceeded that of any other major element (Deng et al., 2024). Excessive N fertilizer input will not only cause air, soil, and water pollution, global climatic change, and ecological environment change, but it will also have a negative impact on crop growth, yield, and quality (Liu et al., 2021). This has serious implications for international food security and natural ecosystem stability. In fact, the excessive application of N fertilizer cannot fundamentally increase crop yield, because once the N fertilizer rate exceeds a certain threshold, the yield no longer increases significantly (Zhang et al., 2021). China is the world’s largest N fertilizer user, using more than 30% of pesticides and fertilizers on 9% of the world’s arable land (Sun et al., 2012). Plant growth stress caused by high N has become increasingly serious.
Although soils contain different forms of N sources, including NO3-, NO2-, NH4+and various organic N sources, most terrestrial plants, including apples, mainly absorb NO3- (Feng et al., 2022). In addition to acting as a nutrient substance for plants, NO3- can also act as a signaling substance to trigger local and systemic signaling pathways that regulate gene expression, metabolism, physiology, and plant growth and developmental processes, including seed germination, root construction, branch growth, and flowering time (Fredes et al., 2019). However, excessive NO3- supply often has a negative impact on plant growth and development (Xu et al., 2016; Saiz-Fernández et al., 2015). It has demonstrated that excessive NO3–N reduces photosynthesis and enzyme activities, and increases ionic toxicity, osmotic stress and reactive oxygen species (ROS), further affecting crop quality and yields (Zhang et al., 2017). In previous studies, high concentrations of NO3- in plants were considered a signal of N sufficiency to directly inhibit root elongation or indirectly affect root growth and development in crosstalk with phytohormones (Vega et al., 2019). However, it is well known that N metabolism is closely linked to other metabolic pathways, especially carbon metabolism. Wang et al. also reported that high N levels increased the synthesis of amino acids and their derivatives in apple fruit, and inhibited carbohydrate accumulation (Wang et al., 2022). Therefore, excessive N supply often causes imbalance of carbon and N metabolism and affects plant growth.
As a multifunctional molecule, melatonin is present in nearly all living organism (Antoniou et al., 2017). It is now widely accepted as a new endogenous plant hormone having pleiotropic activities in trace amounts (Ding et al., 2017). Melatonin is generally produced in the chloroplast and mitochondria of roots and/or leaves, and further transported to the meristem, flowers and fruits in plants (Wang et al., 2016). Evidence have been found that melatonin has several physiological functions in regulating root development, seed germination, photosynthesis, flowering, leaf senescence, and fruit maturation (Back et al., 2016; Arnao and Hernández-Ruiz, 2019). The antioxidant properties of melatonin can significantly reduce plant damage due to environmental stress at specific concentrations (Gomes et al., 2014; Wei et al., 2015). For example, one study found that exogenous melatonin application improved photosynthetic performance, reduced leaf hydrogen peroxide accumulation, and alleviated plant growth inhibition due to drought in Malus hupehensis by promoting the absorption of nutrient elements (Liang et al., 2018). Du et al. (2022) found that melatonin can relieve nutrient stress caused by low concentrations of NH4+ through regulating the absorption and metabolism of N. Recently, the roles of melatonin in nutrient uptake has become a research focus, especially in terms of N absorption and utilization (Liu et al., 2020).
The apple industry is an important agricultural industry in China. In recent years, China’s apple cultivation area has stabilized, and the output has increased steadily, China has become the largest apple-producing country in the world (Faostat, 2018). However, the site conditions of most apple orchards are poor, as they are mainly distributed on hillsides, hills, and beaches with relatively poor soil nutrient levels and low soil organic matter (Ge et al., 2018). Given the poor soil conditions of apple orchards, chemical fertilizer plays an important role in increasing yields. The pursuit for high yield has led to the sustained and rapid growth of N fertilizer application in Chinese apple orchards, which has far exceeded the demand of the trees (Zhu et al., 2018).
There is currently a lack of research on how melatonin regulates carbon-nitrogen metabolism in apple rootstock M9T337 under high N stress. In this study, we used apple rootstocks M9T337 as test materials to explore the effects of melatonin on the roots and the photosynthetic carbon metabolism of rootstocks under high N conditions. Our results should provide a basis for the rational application of N fertilizer.
The pot experiment was carried out between April and July 2022 at the Agricultural Science Experimental Station of Weifang University (118°10’ E and 35°41’ N). The soil utilized in the experiment was loam, with 22.13 grams of organic matter per kilogram, 46.81 milligrams of N per kilogram, 33.25 milligrams of accessible phosphorus per kilogram, and 178.12 milligrams of potassium per kilogram. The test materials consisted of Malus domestica M9T337 dwarf rootstocks. Rootstocks were cultivated in a soil culture system within a growth chamber, exposed to natural light. The growth chamber maintained a temperature cycle of 28°C during the day and 18°C during the night, as well as a humidity level of 65%. On April 9th, when the seedlings reached 10 true leaves, they were moved into pots. The pots had a height of 17 cm and a diameter of 22 cm, and contained 2.5 kg of soil each and each treatment was repeated in 30 pots (replicates). Only one plant was placed in each pot. After a period of 14 days, rootstocks exhibiting consistent development were chosen for the purpose of conducting experiments (about 13 cm tall).
In the formal trial, Ca(NO3)2 was used as the only N source. The seedlings were treated for root application as follows: CK (Control, 10 mmol·L−1 NO3−-N), 100 μmol·L−1 melatonin (MT, Kangwei Century Technology Co., Ltd.), the experimental concentration is referred to the study of Liang et al (Liang et al., 2018), 30 mmol·L−1 NO3−-N (HN), the experimental concentration is referred to the study of Liu et al (Liu et al., 2022), 30 mmol·L−1 NO3−-N + 100 μmol·L−1 melatonin (HN+MT). For the 15N labeling of plants, we added 0.5 g (CK and MT) and 1.5 g (HN and HN+MT) Ca(15NO3)2 (10.22% abundance, Shanghai Yuan ye Biotechnology Co., Ltd.) to each pots, each pots was treated with 200ml, once every 2 days, for a total of three times. After 30 days of treatment, samples were obtained to determine different indicators.
The cleaned root systems that float on 5 mm of water in a 0.3 × 0.2 m Plexiglas tray. Roots be untangled and discrete with a brush to lessen root interlock. Greyscale root images got by tuning the parameters to ‘‘high’’ setting (resolution 300 by 300 dpi). Then WinRhizo software (WinRHIZO version 2012b, Regent Instruments Canada, Montreal, Canada) was using to analyze the whole root system (root length, Number of root tips, root surface area and root volume).
The root activity was determined by the 2,3,5-triphenyltetrazolium chloride (TTC) reduction method and expressed as the amount of TTC reduced by per gram of root (Gao et al., 2023).
The plant samples were washed and dried off with an absorbent paper. Then, the fresh weights of the aboveground and belowground parts were measured. The dry weights of the aboveground and below-ground parts were measured after drying the samples in an oven at 105°C for 30 min and at 80°C to constant weight (Sun et al., 2022).
The superoxide dismutase (SOD) activity was determined based on the ability to inhibit the photochemical reduction of nitroblue tetrazolium (NBT) (Meftahizadeh et al., 2023). The peroxidase (POD) activity was determined by a colorimetric method in a reaction mixture containing guaiacol as the substrate (Wu et al., 2023). The POD activity was determined based on the change in absorbance at 470 nm due to the oxidation of guaiacol to tetraguaiacol. The supernatant was mixed with and was allowed to run. The absorbance of the reaction mixture was read at 560 nm. The catalase (CAT) activity was determined by the ultraviolet spectrophotometric method (Ren et al., 2022) at 240 nm in a reaction mixture. The CAT activity was measured based on the decomposition of H2O2 with time. Ascorbate peroxidase (APX) activity was measured by a spectrophotometric method according to Sun et al (Sun et al., 2022).
The H2O2 was determined according to the methods described by Yan et al (Yan et al., 2023). Pre-cooled acetone was used to extract the H2O2 in the sample and detected by monitoring the absorbance of the titanium peroxide complex at 412 nm.
Sorbose (Sor), sucrose (Suc), glucose (Glu) and fructose (Fru) were performed as described by Aslam et al. (2019). The hexose phosphates glucose 6-phosphate (G6P) and fructose 6-phosphate (F6P) were extracted and assayed as described by Chen et al. (2023).
After 27 days of treatment, 13C isotope labeling was performed. Ba13CO3 (13C independence is 98%) was used as a selection marker, and the dosage was 0.2 g. The seedlings were placed together with the markers, fans, and reduced iron powder into a sealed marking room to make transparent film. The transmittance of sunlight in the labeling chamber was 95% of the natural light intensity. Labeling work started at 9:00 a.m., at which point the fan was turned on and the labeling chamber was sealed. One milliliter of hydrochloric acid (1 mol·L−1) was injected into the beaker with a syringe every 0.5 h in order to maintain the concentration of CO2, and the 13C labeling lasted for 4 h. In order to keep low temperature during the labeling process, an appropriate amount of ice was added to the bottom of the labeling chamber to limit the temperature within the range of 28–37°C. At the same time, three other plants were selected as the control (13C natural abundance), and destructive samples were taken on the third day after labeling and 13C was determined (Xu et al., 2020).
Biomass samples for 13C and 15N analyses were collected at the end of the labeling for 13C and 15N analyses. The samples were dried and then ground with an electric grinder and filtered with a 0.25 mm mesh screen. The abundance of 15N and 13C were measured with a ZHT-03 mass spectrometer from the Beijing Analytical Instrument Factory (Chinese Academy of Agricultural Sciences). Three replicates were conducted for each treatment. The formula is calculated according to Wang et al (Wang et al., 2020).
The NO3− flux at root surface were measured using a scanning non-invasive micro-test technique system (NMT 100 Series, United States) by Xuyue Sci. & Tech. Co., Ltd., Beijing, China. Net fluxes of NO3−. Each selected root was tested for 10 min, with 5 replicates per group. After the test, we analyzed the data with MageFlux (imFluxes V2.0; YoungerUSA LLC, Amherst, MA 01002, USA), a data analysis software provided by Xuyue company (Zheng et al., 2023).
The ammonium-nitrogen (NH4+-N) concentration was quantified using Nessler’s spectrophotometric technique (Molins-Legua et al., 2006). The leaf extract was combined with hypochlorite and phenol in a very alkaline environment to produce a water-soluble blue indophenol, with its absorbance measured at 625 nm. The activity of nitrate reductase (NR) in the leaves was assessed using sulfanilamide colorimetry (Zhao and Cang, 2015). The leaf extract was combined with sulfanilamide in hydrochloric acid and N-1-naphtyl-ethylenediamine to produce a red azo dye, with absorbance measured at 520 nm. Glutamine synthetase (GS) activity was assessed using the plant GS enzyme activity assay kit, and glutamic acid synthetase (GOGAT) activity was evaluated using the plant GOGAT enzyme activity assay kit (Jiangsu Enzyme Label Biotechnology Co., Ltd, Jiangsu, China), in accordance with the manufacturer’s instructions (Sun et al., 2022).
Genes involved in N assimilation such as nitrate reductase (MdNR), glutamine synthetase 1 (MdGS1), and nicotinamide adenine dinucleotide-glutamate synthase (MdNADH-GOGAT); nitrate transporters such as nitrate transporter 1.1/1.2/1.5/2.1 (MdNRT1.1/1.2/1.5/2.1); Sucrose synthase (MdSUSY1); sucrose transporter (MdSUT1); Phosphosucrose synthase (MdSPS1) and hexokinase (MdHK6) were selected for transcript analysis by quantitative real-time PCR (qRT-PCR).
Total RNA was extracted using an RNAprep Pure Plant Kit (Tiangen, Beijing, China) according to the manufacturer’s instructions. The RNA was reverse-transcribed into cDNA using a RevertAid First Strand cDNA Synthesis Kit (TransGen) in a 20 mL reaction. The quantitative real-time PCR (qRT-PCR) was performed in a 20 mL reaction mixture containing 10 mL of Green qPCR SuperMix, 1 mL of cDNA, 2 mL (1 mL of upstream and 1 mL of downstream primers) of primers and 7 mL of ddH2O. The relative gene expression levels were calculated by the 2eDDCT method (Lv et al., 2012), and the MdActin gene was used as the internal control. These qRT-PCR experiments were performed with three technical replicates and three biological replicates. The primers used for qRT-PCR were listed in Supplementary Table S1.
The data presented as means (± SD) of three replicates. The data were subjected to one-way ANOVA and the least significant difference test (Duncan’s new multiple range method, p ≤ 0.05) using SPSS 20.0 software (Statistics software, version 20.0, IBM, USA). Significant differences were determined at a probability level of P ≤ 0.05.
Compared to CK, HN significantly inhibited the root growth of seedlings (Figure 1A). It was found that the MT treatment greatly improved the root morphology of plants that were given CK, and the HN+MT treatment greatly improved the effects of high N on plant root growth. For example, the root length, surface area, volume, and number of root tips were 14.79%, 27.29%, 46.53%, and 39.16% higher in the HN+MT treatment than in the HN treatment (Figures 1B–E). The root activity also showed the same trend (Figure 1F). Figure 1A shows that different treatments have different effects on M9T337 rootstock growth. The HN treatment can greatly lower the plant’s biomass in its roots and leaves. On the other hand, the HN+MT treatment can greatly improve M9T337 rootstock growth, which was slowed down by the high N treatment. Compared with HN treatment, HN+MT treatment increased root and leaf growth by 11.38% and 28.01%, respectively (Figures 1G, H).
Figure 1. Effects of different treatments on the growth of M9T337 rootstocks. (A) Root growth. (B) Root length. (C) The number of root tips. (D) Surface area of the root. (E) Volume of the root. (F) Root activity. (G) Root biomass. (H) Leaf biomass. The error bar represents the standard deviation of the mean (n=3). Different lowercase letters indicate significant differences among treatments (Duncan test, p < 0.05).
Compared to CK, MT treatment increases the net photosynthetic rate of M9T337 rootstocks, HN treatment reduces the net photosynthetic rate of M9T337, while high N treatment with the addition of melatonin can significantly reduce the decrease in the net photosynthesis rate caused by high N. The HN+MT treatment of M9T337 rootstocks increased the net photosynthetic rate by 19.65% compared to the HN treatment (Figure 2A). MT and HN treatment significantly increased the intercellular CO2 concentration (Ci) of M9T337 rootstocks and reduced the stomatal conductance (Gs) and transpiration rate (Tr) compared to the control. Compared to HN treatment, HN+MT treatment significantly reduces the intercellular CO2 concentration of M9T337 rootstocks and improves the stomatal conductance and transpiration rate. Intercellular CO2 concentration decreased by 12.94% compared to HN treatment, and stomatal conductance and transpiration rates increased by 18.08% and 11.20%, respectively (Figures 2B–D).
Figure 2. Effects of different treatments on photosynthetic parameter and the antioxidant enzyme activities in leaf of M9T337 rootstocks. (A) Net photosynthetic rate. (B) The intercellular CO2 concentration (Ci). (C) Stomatal conductance(Gs). (D) Transpiration rate (Tr). (E) SOD activity. (F) POD activity. (G) CAT activity. (H) APX activity. (I) H2O2 content. The error bar represents the standard deviation of the mean (n=3). Different lowercase letters indicate significant differences among treatments (Duncan test, p < 0.05).
MT treatment can significantly increase SOD activity, POD activity, and APX activity, contrary to HN treatment. Compared with CK treatment, SOD activity, POD activity, and APX activity of MT treatment were 12.56%, 19.45%, and 34.70% higher, respectively. However, compared with HN treatment, HN+MT treatment can significantly increase SOD activity, CAT activity, and APX activity under high N stress. Compared to HN treatment, HN+MT treatment had higher SOD activity, CAT activity, and APX activity (11.05%, 12.90%, and 5.92%, respectively) (Figures 2E–I).
The soluble sugar content in rootstock leaves and roots under different treatments were determined (Figure 3). The content of sorbitol and sucrose in leaves under HN+MT treatment was lower than that under HN treatment and higher than that under MT treatment, while the content of sorbitol and sucrose in leaves under HN+MT treatment was higher than that under HN treatment and lower than that under MT treatment. Compared to the MT treatment, the contents of sorbitol and sucrose in leaves treated with HN+MT increased by 18.18% and 23.77%, respectively. In roots treated with HN+MT, the content of sorbitol and sucrose increased by 20.71% and 30.91%, respectively, compared to HN treatment (Figures 3A, B, E, F).
Figure 3. Effect of different treatments on the soluble sugar in leaf and root of M9T337 rootstocks. (A) Leaf sorbitol content. (B) Leaf sucrose content. (C) Leaf fructose content. (D) Leaf glucose content. (E) Root sorbitol content. (F) Root sucrose content. (G) Root fructose content. (H) Root glucose content. (I) Photo of root and leaf. The error bar represents the standard deviation of the mean (n=3). Different lowercase letters indicate significant differences among treatments (Duncan test, p < 0.05).
Leaves and roots treated with HN+MT had significantly higher fructose and glucose contents than those treated with HN. Compared with HN treatment, the fructose and glucose contents in leaves under HN+MT treatment were increased by 58.33% and 4.83%, respectively, and the fructose and glucose contents in roots were increased by 28.88% and 17.19%, respectively (Figures 3C, D, G, H).
The rhizosphere NO3– velocity of M9T337 rootstocks was significantly different under different treatments (Figures 4A, B). Negative values indicate NO3– influx, i.e., NO3– absorption, and positive values indicate efflux. Compared to CK, MT treatment promoted NO3– absorption, with the average value changing from -26.11 pmol·cm–2·s–1 to -35.73 pmol·cm–2·s–1. In HN treatment, NO3– is effluxed by roots, while in HN+MT treatment, NO3– flow in rhiza is influxed, with an average rate of around -10.21 pmol·cm–2·s–1. The results showed that high N treatment was not conducive for NO3– absorption, while high N treatment after adding melatonin is favorable to NO3– absorption.
Figure 4. Effect of different treatment on N metabolism in leaf and root of M9T337 rootstocks. (A) NO3– fluxes detection of roots. (B) Net NO3– fluxes in the root of M9T337 rootstocks for 10 min. (C) NR activity. (D) GS activity. (E) GOGAT activity. (F) Nitrate nitrogen. (G) Amino acid content. (H) Soluble protein. The error bar represents the standard deviation of the mean (n=3). Different lowercase letters indicate significant differences among treatments (Duncan test, p < 0.05).
N-metabolizing enzymes play important roles in the process of N assimilation. Under different treatments, the activity trend of NR, GS, and GOGAT in leaves and roots remained the same. Compared to CK, MT treatment increased the activities of NR, GS, and GOGAT, while HN treatment inhibited these three enzymes. MT+HN treatment could reduce the inhibition of NR, GS, and GOGAT activities in high N treatment. Compared to HN treatment, NR, GS, and GOGAT in leaves increased by 12.84%, 20.49%, and 43.92%, respectively, while NR, GS, and GOGAT in roots increased by 12.84%, 34.44%, and 61.54%, respectively (Figures 4C–E). According to Figures 4F–H, it can be found that, compared with CK, the nitrate N and amino acid contents of leaves and roots under HN treatment significantly increased, while the soluble protein contents decreased. Compared with HN treatment, MT+HN treatment increased soluble protein content.
The expression changes of the N transporter gene and the N channel protein gene in the root and leaf systems are shown in Figures 5A–N. The transcription levels of NRT1.1, NRT1.2, and NRT2.1 in the roots of M9T337 stock treated by HN were significantly lower than those treated by HN+MT. Under MT treatment, the expression levels of MdNR, MdGS1, MdGOGAT, MdSUSY1, MdSUT1, and MdHK6 in the root were the highest. These results indicated that high N significantly inhibited the expression of the N transporter gene and the N channel protein gene. Compared with HN treatment, the expression of the N transporter gene and the N channel protein gene in the root and leaf was significantly up-regulated by high N treatment with melatonin.
Figure 5. Effect of different treatment on transcriptional regulation of genes involved in carbon and N metabolism of apple rootstock M9T337. (A–N) The expression changes of carbon and N metabolism genes in root and leaf. (Duncan test, *p ≤ 0.05) (O) Heat map of relative expression. Color scale represents relative intensity of gene expression. The qRT-PCR results were standardized by Z-score and the data represents the Z-score of gene expression. The red color represents upregulated expression, while blue represents downregulated expression.
According to the heat map of relative expression analysis in Figure 5O, the expression of the N transporter gene and the N channel protein gene in roots and leaves was significantly down-regulated under HN treatment, while HN+MT treatment could significantly up-regulate the expression of the N transporter gene and the N channel protein gene in roots and leaves.
Under different treatments conditions, 13C accumulation was highest in all of the organs under the MT treatment, followed by the HN+MT and CK treatments. The least 13C accumulated in all of the organs and the entire plant under the HN treatment (Figure 6A). Compared with HN treatment, the root, stem, leave and total 13C accumulation of HN+MT treatment were 23.85%, 7.63%, 20.44% and 17.53% higher, respectively (Figure 6A). The distribution rate of 13C was mainly concentrated in the root of HN treatment, the stem of MT treatment and the leaf of HN+MT treatment (Figure 6B). The 15N accumulation of CK and MT treatment was significantly higher than that of HN and HN+MT treatment. Compared with HN treatment, root, stem, leaf and total N accumulation of HN+MT treatment were 11.45%, 3.52%, 14.98% and 11.17% lower, respectively (Figure 6C). It can be seen from Figure 6D that the C/N accumulation rate of HN+MT treatment and MT treatment is higher than that of CK and HN treatment. Compared with HN treatment, the C/N accumulation rate of HN+MT treatment was 32.55% higher.
Figure 6. Effect of different treatments on 13C and 15N accumulation of M9T337 rootstocks. (A) 13C accumulation. (B) 13C distribution. (C) 15N accumulation. (D) C/N accumulation. The error bar represents the standard deviation of the mean (n=3). Different lowercase letters indicate significant differences among treatments (Duncan test, p < 0.05).
In order to study the effects of different treatments on the rootstock of seedling M9T337, correlation analysis was carried out on its growth conditions, antioxidant protective enzymes (SOD, POD, CAT, APX) activity, soluble sugar content, carbon and N accumulation and distribution. As can be seen from Figure 7, leaf sucrose content was significantly negatively correlated with root activity, leaf glucose content, root glucose content, volume of root, APX activity and POD activity. The was negatively correlated with SOD activity, root sorbitol content, root length and root activity. Leaf fructose content was positively correlated with 13C accumulation, root fructose content, CAT activity, number of root tips, APX activity and POD activity. Among them, 13C and 15N are negatively correlated.
Figure 7. Correlation between different indicators of M9T337 rootstocks. (Duncan test, *p ≤ 0.05, **p ≤ 0.01, ***p ≤ 0.001).
The major way that crops take in water and nutrients from the soil is through their roots. Proper root shape and structure are critical for plant growth and development as well as for the uptake of nutrients from the soil (Mulaudzi et al., 2020). Plants frequently allocate biomass preferentially to the roots in order to increase their capacity for nutrient uptake in response to nutritional deficiencies. For instance, low P stress encourages lateral root growth and increases the number of root hairs, while low N stress induces root elongation (Hu et al., 2024). The findings indicated that melatonin may successfully reduce the negative effects of excessive N on root development and vitality. The use of melatonin may effectively ameliorate the reduction of root length, root spots, root surface area, and root volume caused by high N pressure. Properly increasing the melatonin supply helps optimize the form and structure of roots in high-N environments by maximizing the absorption of N, which in turn encourages root development (Figures 1A–F). We conducted further measurements of the apple rootstocks’ biological mass. Our findings indicate that the application of high levels of N hindered plant development. Moreover, the addition of melatonin successfully mitigated the inhibitory effects of high levels of nitrate on plant growth (Figures 1G–I). These findings are concordant to the results of Zhang et al. (2017).
We also identified the antioxidant system’s metabolites and associated enzyme activity in the rootstock roots. The ROS equilibrium is maintained by plants by means of their inherent antioxidant systems (Boaretto et al., 2020). Among them, APX, CAT, POD, and SOD are crucial for sustaining and scavenging ROS (Choudhury et al., 2017). Under normal development circumstances, antioxidant protection enzyme activity is in a balanced state, but under high N suppression conditions, SOD activity, CAT activity and APX activity falls, weakening the function of root antioxidants. It lowers the efficiency of H2O2 clearance, promoting membrane lipid peroxidation, finally leading to root oxidation damage. This explains why excessive nitrate on produces greater oxidative damage to the root (Figures 2E–I). However, melatonin can significantly alleviate the peroxide damage caused by high N, and is conducive to removing hydrogen peroxide and reducing lipid peroxidation.
Plants convert CO2 dioxide into carbohydrates via photosynthesis, and these carbohydrates account for 90% of the biomass and agricultural productivity (Simkin et al., 2019). The carbohydrates essential for root development are transferred from the leaf, which shows that carbon partitioning is also a significant factor controlling root growth (Lambers et al., 2002). Some studies have shown that improving nitrogen (N) efficiency can lead to increased photosynthetic rates (Cechin and Valquilha, 2019). However, there is also evidence suggesting competition between nitrogen and carbon metabolism under high nitrogen conditions (Bloom, 2015). Our research indicates that under high nitrogen supply, the Pn, Gs, and Tr of apple rootstocks significantly decreased, while the addition of melatonin significantly enhanced photosynthetic capacity.
The effect of N in root growth promotion indicated via connections with carbohydrate content and metabolism (Li et al., 2018). In apples and certain other Rosaceae species, sorbitol and sucrose are the principal end-products of photosynthesis and are the main types of carbohydrates carried across large distances in the phloem (Sha et al., 2020). The findings revealed that high amounts of N compulsion and melatonin had a substantial influence on the accumulation and distribution of soluble sugar in M9T337 rootstock. Research has found that N fertilizer and melatonin have significant effects on plant carbon metabolism (Yan et al., 2023). In the absence of an increase in melatonin, high N coercion raised the level of peanut and mercury in the leaves and dramatically decreased the amounts of root-based peanut alcohol and mercury, showing that high nitrous coertion prevented the transfer of leaves from leaves to roots. This is comparable to the results of the research on apple (Malus hupehensis) (Zhao et al., 2020). Our results also show that high N stress limits the transport of photosynthetic products, as sorbitol content in leaves increases while sorbitol content and sucrose content in roots decreases. Increasing the supply of melatonin helps to alleviate the restriction of high N on the transport of photosynthetic products (Figure 3). Sorbitol and sucrose, as photosynthetic products can be degraded to fructose and glucose by SDH and SuSy after unloading to the root via long-distance transport in the phloem (Zhao et al., 2020). High N coercion reduces the content of fructose and glucose in the root. High nitrous coertion may reduce the activity of the related enzymes, resulting in a decrease in the concentration of frucose and glycine in the roots, thereby affecting the cycle of triglyceride and starch synthesis, inhibiting root growth (Li et al., 2012). Further analysis shows that the addition of melatonin in high N conditions promotes the phosphoridation of the fructose and glucose, thus enhancing the absorption and utilization of M9T337 rootstocks.
To further investigate the accumulation and distribution of photosynthetic products, M9T337 rootstock was tagged with 13C isotope. We found that the accumulation of 13C in leaves, stems and roots was the highest with melatonin administration, whereas the accumulation of 13C in roots under high N treatment was the lowest. High N treatment hindered N buildup and lowered C/N accumulation rate. Therefore, high N treatment inhibited the transport of photosynthetic products to roots, while appropriately increasing the melatonin supply level could increase and promote the distribution of sorbitol and sucrose from leaves to roots and increase the inhibition, thus reducing the inhibition of high N treatment on root growth.
The application of N is frequently used in excess to achieve increased crop yields (Sun et al., 2020). Nevertheless, this study discovered that an overabundance of nitrate supply hindered the growth of the M9T337 apple rootstocks’ roots and decreased the ratio of roots to shoots, which was unfavorable for the absorption of nutrients and the growth of the rootstocks. After nitrate is absorbed and transported by the roots, it is reduced to nitrite, glutamine, and glutamate in sequence under the action of NR, GS, and GOGAT, and then further metabolized into amino acids and nitrogen-containing compounds. We quantified the absorption of NO3− with the use of non-invasive micro measuring techniques. The net fluxes of NO3− at the root surface was effluxed under circumstances of elevated nitrate levels, suggesting that high N concentrations hindered the absorption of NO3−. This is due to when the intracellular N levels are too high, key enzymes in the metabolic pathways are inhibited to prevent further accumulation of N. N sensors, such as TARGET OF RAPAMYCIN (TOR) signaling pathway, are activated to reduce N uptake and utilization (Artins et al., 2024). This mechanism helps cells maintain N balance and avoid toxicity from excess N. It has been reported that genes encoding enzymes related to N assimilation are regulated by carbon and N interactions (Guti´errez et al., 2007). Our findings revealed that under circumstances of high N levels, the activities and expression of NR, GS, and GOGAT were dramatically suppressed, while melatonin can significantly relieve the symptoms, which may be related to the fact that melatonin changed the C/N ratio of seedlings.
The absorption of nutrients in plants is regulated by both internal signals inside the plant and external signals from the environment. When the intensity of nutrient supply surpasses the concentration needed for the plant to develop at its maximum rate, the uptake of nutrients is primarily controlled by the intensity of the nutrient pool. Nitrate uptake is mostly attributed to NRTs (Wang et al., 2012; Yuan et al., 2022). MdNRT1.1, MdNRT1.5 and MdNRT2.1 are significant NRTs that are predominantly engaged in NO3− absorption in the roots (Xuan et al., 2017). We observed that the expression levels of NRT1.1, NRT1.2 and NRT2.1 in the roots fell dramatically under high N conditions, which explained why high N stress hindered N uptake. However, it is not obvious if the reduced expression of these genes is regulated by internal or external N levels, and its precise mechanism requires additional exploration.
Excessive N application hampers the growth of M9T337 rootstocks by decreasing root-to-shoot ratios and nutrient absorption. High nitrate levels inhibit NO3− uptake, activate N sensors like the TOR pathway, suppress key N metabolism enzymes, and inhibit the synthesis of soluble proteins, while melatonin can alleviate these effects. Additionally, the expression of vital nitrate transporters decreases under high N conditions, highlighting the intricate relationship between nitrogen levels and nutrient absorption, with melatonin serving as a potential mitigator of high N stress.
In conclusion, correctly raising the melatonin supply level is helpful to root development of M9T337 rootstock under severe N stress. Under high N conditions, appropriately increasing melatonin level can activate antioxidant enzyme activity, reduce lipid peroxidation in roots, protect root structural integrity, promote the transport of sorbitol and sucrose to roots, and promote further degradation and utilization of sorbitol and sucrose in roots, which is conducive to the accumulation of photosynthetic products. At the same time, MT can also improve N metabolism, promote NO3− uptake by roots, and raise the buildup of 15N (Figure 8). In conclusion, these results offer new insights for optimizing N application and maintaining carbon-nitrogen balance, which can contribute to improving apple yield and quality.
Figure 8. Model of the effect of exogenous melatonin on carbon and N metabolism in apple rootstock under high N stress.
The original contributions presented in the study are included in the article/Supplementary Material. Further inquiries can be directed to the corresponding authors.
MS: Writing – original draft, Conceptualization, Data curation, Methodology, Software. CW: Investigation, Software, Writing – original draft. GZ: Investigation, Software, Writing – original draft. HC: Methodology, Supervision, Writing – original draft. FW: Conceptualization, Funding acquisition, Methodology, Software, Supervision, Writing – review & editing. ML: Methodology, Visualization, Writing – review & editing. SG: Funding acquisition, Investigation, Software, Writing – review & editing.
The author(s) declare that financial support was received for the research, authorship, and/or publication of this article. This work was supported by the National Natural Science Foundation of China (32302471 and 32072518), Ministry of Finance and Ministry of Agriculture and Rural Affairs: The China Agriculture Research System (CARS-27), Shandong Provincial Natural Science Foundation (ZR2023QC020), Weifang University Doctor Initiation Fund Project (2022BS15; 2024056) and Weifang science and technology development program (2023GX006).
The authors declare that the research was conducted in the absence of any commercial or financial relationships that could be construed as a potential conflict of interest.
All claims expressed in this article are solely those of the authors and do not necessarily represent those of their affiliated organizations, or those of the publisher, the editors and the reviewers. Any product that may be evaluated in this article, or claim that may be made by its manufacturer, is not guaranteed or endorsed by the publisher.
The Supplementary Material for this article can be found online at: https://www.frontiersin.org/articles/10.3389/fpls.2024.1482351/full#supplementary-material
Abbaraju, H. K., Gupta, R., Appenzeller, L. M., Fallis, L. P., Hazebroek, J., Zhu, G., et al. (2022). A vegetative storage protein improves drought tolerance in maize. Plant Biotechnol. J. 20, 374–389. doi: 10.1111/pbi.13720
Antoniou, C., Chatzimichail, G., Xenofontos, R., Pavlou, J. J., Panagiotou, E., Christou, A., et al. (2017). Melatonin systemically ameliorates drought stress-induced damage in Medicago sativa plants by modulating nitro-oxidative homeostasis and proline metabolism. J. Pineal Res. 62, e12401. doi: 10.1111/jpi.12401
Arnao, M. B., Hernández-Ruiz, J. (2019). Melatonin: a new plant hormone and/or a plant master regulator? Trends Plant Sci. 24, 38–48. doi: 10.1016/j.tplants.2018.10.010
Artins, A., Martins, M. C. M., Meyer, C., Fernie, A. R., Caldana, C. (2024). Sensing and regulation of C and N metabolism – novel features and mechanisms of the TOR and SnRK1 signaling pathways. Plant J. 118, 1268–1280. doi: 10.1111/tpj.16684
Aslam, M. M., Deng, L., Wang, X., Wang, Y., Pan, L., Liu, H., et al. (2019). Expression patterns of genes involved in sugar metabolism and accumulation during peach fruit development and ripening. Scientia Hortic. 257, 108633. doi: 10.1016/j.scienta.2019.108633
Back, K., Tan, D. X., Reiter, R. J. (2016). Melatonin biosynthesis in plants: multiple pathways catalyze tryptophan to melatonin in the cytoplasm or chloroplasts. J. Pineal Res. 61, 426–437. doi: 10.1111/jpi.12364
Bloom, A. J. (2015). Photorespiration and nitrate assimilation: a major intersection between plant carbon and nitrogen. Photosynth. Res. 123, 117–128. doi: 10.1007/s11120-014-0056-y
Boaretto, R. M., Hippler, F. W., Ferreira, G. A., Azevedo, R. A., Quaggio, J. A., Mattos, D. (2020). The possible role of extra magnesium and nitrogen supply to alleviate stress caused by high irradiation and temperature in lemon trees. Plant Soil 457, 57–70. doi: 10.1007/s11104-020-04597-y
Cechin, I., Valquilha, ´E.M. (2019). Nitrogen effect on gas exchange characteristics, dry matter production and nitrate accumulation of Amaranthus cruentus L. Braz. J. Bot. 42, 373–381. doi: 10.1007/s40415-019-00542-1
Chen, K.-E., Chen, H.-Y., Tseng, C.-S., Tsay, Y.-F. (2020). Improving nitrogen use efficiency by manipulating nitrate remobilization in plants. Nat. Plants 6, 1126–1135. doi: 10.1038/s41477-020-00758-0
Chen, X., Ji, Y., Zhao, W., Niu, H., Yang, X., Jiang, X., et al. (2023). Fructose-6-phosphate-2-kinase/fructose-2, 6-bisphosphatase regulates energy metabolism and synthesis of storage products in developing rice endosperm. Plant Sci. 326, 111503. doi: 10.1016/j.plantsci.2022.111503
Choudhury, F. K., Rivero, R. M., Blumwald, E., Mittler, R. (2017). Reactive oxygen species, abiotic stress and stress combination. Plant J. 90, 856–867. doi: 10.1111/tpj.13299
Cunha, M., Cavalcante, Í.H.L., Mancin, A. C., Albano, F. G., Marques, A. S. (2015). Impact of humic substances and nitrogen fertilising on the fruit quality and yield of custard apple. Acta Scientiarum. Agron. 37, 211–218. doi: 10.4025/actasciagron.v37i2.19511
Deng, C., Zhang, Z., Song, X., Peng, D., Zhao, C., Chen, C., et al. (2024). Nitrogen-derived environmental behavior, economic performance, and regulation potential by human production and consumption in a mega river basin. J. Cleaner Production 434, 140279. doi: 10.1016/j.jclepro.2023.140279
Ding, F., Liu, B., Zhang, S. (2017). Exogenous melatonin ameliorates cold-induced damage in tomato plants. Scientia Hortic. 219, 264–271. doi: 10.1016/j.scienta.2017.03.029
Du, P., Yin, B., Zhou, S., Li, Z., Zhang, X., Cao, Y., et al. (2022). Melatonin and dopamine mediate the regulation of nitrogen uptake and metabolism at low ammonium levels in Malus hupehensis. Plant Physiol. Biochem. 171, 182–190. doi: 10.1016/j.plaphy.2022.01.004
Faostat. (2018). Statistical Database of the Food and Agricultural Organization of the United Nations. Available online: https:///www.faostat.fao.org.
Feng, Z.-Q., Li, T., Wang, X., Sun, W.-J., Zhang, T.-T., You, C.-X., et al. (2022). Identification and characterization of apple MdNLP7 transcription factor in the nitrate response. Plant Sci. 316, 111158. doi: 10.1016/j.plantsci.2021.111158
Fredes, I., Moreno, S., Díaz, F. P., Gutiérrez, R. A. (2019). Nitrate signaling and the control of Arabidopsis growth and development. Curr. Opin. Plant Biol. 47, 112–118. doi: 10.1016/j.pbi.2018.10.004
Gao, M., Gai, C., Li, X., Feng, X., Lai, R., Song, Y., et al. (2023). Waterlogging tolerance of Actinidia valvata Dunn is associated with high activities of pyruvate decarboxylase, alcohol dehydrogenase and antioxidant enzymes. Plants 12, 2872. doi: 10.3390/plants12152872
Ge, S. F., Zhu, Z. L., Peng, L., Chen, Q., Jiang, Y. M. (2018). Soil nutrient status and leaf nutrient diagnosis in the main apple producing regions in China. Hortic. Plant J. 4, 89–93. doi: 10.1016/j.hpj.2018.03.009
Gomes, B. R., Siqueira-Soares, R., Santos, W., Marchiosi, R., Soares, A. R., Ferrarese-Filho, O. (2014). The effects of dopamine on antioxidant enzymes activities and reactive oxygen species levels in soybean roots. Plant Signaling Behav. 9, e977704. doi: 10.4161/15592324.2014.977704
Guti´errez, R. A., Lejay, L. V., Dean, A., Chiaromonte, F., Shasha, D. E., Coruzzi, G. M. (2007). Qualitative network models and genome-wide expression data define carbon/ nitrogen-responsive molecular machines in Arabidopsis. Genome Biol. 8, R7. doi: 10.1186/gb-2007-8-1-r7
Hu, F., Fang, D., Zhang, W., Dong, K., Ye, Z., Cao, J. (2024). Lateral root primordium: Formation, influencing factors and regulation. Plant Physiol. Biochem. 207, 108429. doi: 10.1016/j.plaphy.2024.108429
Krapp, A. (2015). Plant nitrogen assimilation and its regulation: a complex puzzle with missing pieces. Curr. Opin. Plant Biol. 25, 115–122. doi: 10.1016/j.pbi.2015.05.010
Lambers, H., Atkin, O. K., Millenaar, F. F. (2002). Respiratory patterns in roots in relation to their functioning. Plant roots: hidden half 3, 521–552. doi: 10.1201/9780203909423.pt6
Li, G., Dong, G., Li, B., Li, Q., Kronzucker, H. J., Shi, W. (2012). Isolation and characterization of a novel ammonium overly sensitive mutant, amos2, in Arabidopsis thaliana. Planta 235, 239–252. doi: 10.1007/s00425-011-1504-y
Li, M., Li, P., Ma, F., Dandekar, A. M., Cheng, L. (2018). Sugar metabolism and accumulation in the fruit of transgenic apple trees with decreased sorbitol synthesis. Horticulture Res. 5, 60. doi: 10.1038/s41438-018-0064-8
Liang, B., Ma, C., Zhang, Z., Wei, Z., Gao, T., Zhao, Q., et al. (2018). Long-term exogenous application of melatonin improves nutrient uptake fluxes in apple plants under moderate drought stress. Environ. Exp. Bot. 155, 650–661. doi: 10.1016/j.envexpbot.2018.08.016
Liu, X. M., Gao, T. T., Zhang, Z. J., Tan, K. X., Jin, Y. B., Zhao, Y. J., et al. (2020). The mitigation effects of exogenous dopamine on low nitrogen stress in Malus hupehensis. J. Integrat. Agric. 19, 2709–2724. doi: 10.1016/S2095-3119(20)63344-5
Liu, J., Lyu, M., Xu, X., Liu, C., Qin, H., Tian, G., et al. (2022). Exogenous sucrose promotes the growth of apple rootstocks under high nitrogen supply by modulating carbon and nitrogen metabolism. Plant Physiol. Biochem. 192, 196–206. doi: 10.1016/j.plaphy.2022.10.005
Liu, D., Song, C., Fang, C., Xin, Z., Xi, J., Lu, Y. (2021). A recommended nitrogen application strategy for high crop yield and low environmental pollution at a basin scale. Sci. Total Environ. 792, 148464. doi: 10.1016/j.scitotenv.2021.148464
Lv, S. L., Jiang, P., Chen, X. Y., Fan, P. X., Wang, X. C., Li, Y. X. (2012). Multiple compartmentalization of sodium conferred salt tolerance in Salicornia europaea. Plant Physiol. Biochem. 51, 47–52. doi: 10.1016/j.plaphy.2011.10.015
Meftahizadeh, H., Baath, G. S., Saini, R. K., Falakian, M., Hatami, M. (2023). Melatonin-mediated alleviation of soil salinity stress by modulation of redox reactions and phytochemical status in guar (Cyamopsis tetragonoloba L.). J. Plant Growth Regul. 42, 4851–4869. doi: 10.1007/s00344-022-10740-z
Molins-Legua, C., Meseguer-Lloret, S., Moliner-Martinez, Y., Campíns-Falcó, P. (2006). A guide for selecting the most appropriate method for ammonium determination in water analysis. TrAC, Trends Anal. Chem. 25, 282–290. doi: 10.1016/j.trac.2005.12.002
Mu, X., Chen, Y. (2021). The physiological response of photosynthesis to nitrogen deficiency. Plant Physiol. Biochem. 158, 76–82. doi: 10.1016/j.plaphy.2020.11.019
Mulaudzi, T., Hendricks, K., Mabiya, T., Muthevhuli, M., Ajayi, R. F., Mayedwa, N., et al. (2020). Calcium improves germination and growth of Sorghum bicolor seedlings under salt stress. Plants 9, 730. doi: 10.3390/plants9060730
Ren, J., Yang, X., Zhang, N., Feng, L., Ma, C., Wang, Y., et al. (2022). ). Melatonin alleviates aluminum-induced growth inhibition by modulating carbon and nitrogen metabolism, and reestablishing redox homeostasis in Zea mays L. J. Hazardous Materials 423, 127159. doi: 10.1016/j.jhazmat.2021.127159
Saiz-Fernández, I., De Diego, N., Sampedro, M. C., Mena-Petite, A., Ortiz-Barredo, A., Lacuesta, M. (2015). High nitrogen supply reduces growth in maize, from cell to whole plant. J. Plant Physiol. 173, 120–129. doi: 10.1016/j.jplph.2014.06.018
Sha, J., Wang, F., Xu, X., Chen, Q., Zhu, Z., Jiang, Y., et al. (2020). Studies on the translocation characteristics of 13C-photoassimilates to fruit during the fruit development stage in ‘Fuji’apple. Plant Physiol. Biochem. 154, 636–645. doi: 10.1016/j.plaphy.2020.06.044
Simkin, A. J., López-Calcagno, P. E., Raines, C. A. (2019). Feeding the world: improving photosynthetic efficiency for sustainable crop production. J. Exp. Bot. 70, 1119–1140. doi: 10.1093/jxb/ery445
Sun, C., Chen, L., Zhai, L., Liu, H., Wang, K., Jiao, C., et al. (2020). National assessment of nitrogen fertilizers fate and related environmental impacts of multiple pathways in China. J. Clean Prod 277, 123519. doi: 10.1016/j.jclepro.2020.123519
Sun, M., Li, S., Gong, Q., Xiao, Y., Peng, F. (2022). Leucine contributes to copper stress tolerance in peach (Prunus persica) seedlings by enhancing photosynthesis and the antioxidant defense system. Antioxidants 11, 2455. doi: 10.3390/antiox11122455
Sun, B., Zhang, L., Yang, L., Zhang, F., Norse, D., Zhu, Z. (2012). Agricultural non-point source pollution in China: causes and mitigation measures. Ambio 41, 370–379. doi: 10.1007/s13280-012-0249-6
Tian, H., Xu, R., Canadell, J. G., Thompson, R. L., Winiwarter, W., Suntharalingam, P., et al. (2020). A comprehensive quantification of global nitrous oxide sources and sinks. Nature 586, 248–256. doi: 10.1038/s41586-020-2780-0
Vega, A., O’Brien, J. A., Gutiérrez, R. A. (2019). Nitrate and hormonal signaling crosstalk for plant growth and development. Curr. Opin. Plant Biol. 52, 155–163. doi: 10.1016/j.pbi.2019.10.001
Wang, Q., An, B., Wei, Y., Reiter, R. J., Shi, H., Luo, H., et al. (2016). Melatonin regulates root meristem by repressing auxin synthesis and polar auxin transport in Arabidopsis. Front. Plant Sci. 7. doi: 10.3389/fpls.2016.01882
Wang, F., Ge, S., Lyu, M., Liu, J., Li, M., Jiang, Y., et al. (2022). DMPP reduces nitrogen fertilizer application rate, improves fruit quality, and reduces environmental cost of intensive apple production in China. Sci. Total Environ. 802, 149813. doi: 10.1016/j.scitotenv.2021.149813
Wang, F., Ge, S., Xu, X., Xing, Y., Du, X., Zhang, X., et al. (2021). Multiomics analysis reveals new insights into the apple fruit quality decline under high nitrogen conditions. J. Agric. Food Chem. 69, 5559–5572. doi: 10.1021/acs.jafc.1c01548
Wang, Y. Y., Hsu, P. K., Tsay, Y. F. (2012). Uptake, allocation and signaling of nitrate. Trends Plant Sci. 17, 458–467. doi: 10.1016/j.tplants.2012.04.006
Wang, H., Tahir, M. M., Nawaz, M. A., Mao, J., Li, K., Wei, Y., et al. (2020). Spermidine application affects the adventitious root formation and root morphology of apple rootstock by altering the hormonal profile and regulating the gene expression pattern. Scientia Hortic. 266, 109310. doi: 10.1016/j.scienta.2020.109310
Wei, W., Li, Q.-T., Chu, Y.-N., Reiter, R. J., Yu, X.-M., Zhu, D.-H., et al. (2015). Melatonin enhances plant growth and abiotic stress tolerance in soybean plants. J. Exp. Bot. 66, 695–707. doi: 10.1093/jxb/eru392
Wu, C., Hao, W., Yan, L., Zhang, H., Zhang, J., Liu, C., et al. (2023). Postharvest melatonin treatment enhanced antioxidant activity and promoted GABA biosynthesis in yellow-flesh peach. Food Chem. 419, 136088. doi: 10.1016/j.foodchem.2023.136088
Xu, X., Du, X., Wang, F., Sha, J., Chen, Q., Tian, G., et al. (2020). Effects of potassium levels on plant growth, accumulation and distribution of carbon, and nitrate metabolism in apple dwarf rootstock seedlings. Front. Plant Sci. 11. doi: 10.3389/fpls.2020.00904
Xu, H., Zhao, X., Guo, C., Chen, L., Li, K. (2016). Spinach 14-3-3 protein interacts with the plasma membrane H+-ATPase and nitrate reductase in response to excess nitrate stress. Plant Physiol. Biochem. 106, 187–197. doi: 10.1016/j.plaphy.2016.04.043
Xuan, W., Beeckman, T., Xu, G. (2017). Plant nitrogen nutrition: sensing and signaling. Curr. Opin. Plant Biol. 39, 57–65. doi: 10.1016/j.pbi.2017.05.010
Yan, D., Wang, J., Lu, Z., Liu, R., Hong, Y., Su, B., et al. (2023). Melatonin-mediated enhancement of photosynthetic capacity and photoprotection improves salt tolerance in wheat. Plants 12, 3984. doi: 10.3390/plants12233984
Yuan, G. P., Sun, D. X., Wang, Y. F., An, G. L., Li, W. H., Si, W. J., et al. (2022). Genome-wide identification and expression analysis of NIN-like Protein (NLP) genes reveals their potential roles in the response to nitrate signaling in watermelon. Hortic. Plant J. 8, 602–614. doi: 10.1016/j.hpj.2022.06.010
Zhang, J., He, P., Ding, W., Ullah, S., Abbas, T., Li, M., et al. (2021). Identifying the critical nitrogen fertilizer rate for optimum yield and minimum nitrate leaching in a typical field radish cropping system in China. Environ. pollut. 268, 115004. doi: 10.1016/j.envpol.2020.115004
Zhang, R., Sun, Y., Liu, Z., Jin, W., Sun, Y. (2017). Effects of melatonin on seedling growth, mineral nutrition, and nitrogen metabolism in cucumber under nitrate stress. J. Pineal Res. 62, e12403. doi: 10.1111/jpi.12403
Zhao, S. J., Cang, J. (2015). Experimental Instruction in Plant Physiology (Beijing, China: Agricultural Science and Technology Press).
Zhao, H., Sun, S., Zhang, L., Yang, J., Wang, Z., Ma, F., et al. (2020). Carbohydrate metabolism and transport in apple roots under nitrogen deficiency. Plant Physiol. Biochem. 155, 455–463. doi: 10.1016/j.plaphy.2020.07.037
Zheng, J., Fujii, K., Koba, K., Wanek, W., Müller, C., Jansen-Willems, A. B., et al. (2023). Revisiting process-based simulations of soil nitrite dynamics: Tighter cycling between nitrite and nitrate than considered previously. Soil Biol. Biochem. 178, 108958. doi: 10.1016/j.soilbio.2023.108958
Keywords: apple rootstock, high N, melatonin, carbon-nitrogen metabolism, 13C, 15N
Citation: Sun M, Wang C, Zhang G, Cao H, Wang F, Li M and Ge S (2024) Melatonin mitigates root growth inhibition and carbon-nitrogen metabolism imbalance in apple rootstock M9T337 under high nitrogen stress. Front. Plant Sci. 15:1482351. doi: 10.3389/fpls.2024.1482351
Received: 18 August 2024; Accepted: 30 September 2024;
Published: 14 October 2024.
Edited by:
Ravinder Kumar, Indian Agricultural Research Institute (ICAR), IndiaReviewed by:
Honghai Luo, Shihezi University, ChinaCopyright © 2024 Sun, Wang, Zhang, Cao, Wang, Li and Ge. This is an open-access article distributed under the terms of the Creative Commons Attribution License (CC BY). The use, distribution or reproduction in other forums is permitted, provided the original author(s) and the copyright owner(s) are credited and that the original publication in this journal is cited, in accordance with accepted academic practice. No use, distribution or reproduction is permitted which does not comply with these terms.
*Correspondence: Fen Wang, ZmVud2FuZ0BzZGF1LmVkdS5jbg==; Ming Li, bG0xMWFAMTYzLmNvbQ==; Shunfeng Ge, Z2VzaHVuZmVuZzIxMEAxMjYuY29t
Disclaimer: All claims expressed in this article are solely those of the authors and do not necessarily represent those of their affiliated organizations, or those of the publisher, the editors and the reviewers. Any product that may be evaluated in this article or claim that may be made by its manufacturer is not guaranteed or endorsed by the publisher.
Research integrity at Frontiers
Learn more about the work of our research integrity team to safeguard the quality of each article we publish.