- 1Department of General and Molecular Genetics, Plant Breeding and Genetics Institute – National Center of Seed and Cultivar Investigation, Odesa, Ukraine
- 2Department of Molecular Biology, Biochemistry and Genetics, Odesa I.I. Mechnikov National University, Odesa, Ukraine
- 3Plant Cytogenetics and Molecular Biology Group, Institute of Biology, Biotechnology and Environmental Protection, Faculty of Natural Sciences, University of Silesia in Katowice, Katowice, Poland
- 4Institute of Agriculture, Lithuanian Research Centre for Agriculture and Forestry, Akademija, Lithuania
- 5Department of Plant Breeding, Swedish University of Agricultural Sciences, Alnarp, Sweden
Introduction: Emerging new races of leaf rust (Puccinia triticina Eriks) are threatening global wheat (Triticum aestivum L.) production. Identifying additional resistance genes from all available gene pools is crucial to expanding wheat resistance to these virulent leaf rust races. Siberian wild rye (Elymus sibiricus L.) possesses numerous beneficial traits that can be valuable in wheat improvement. Three new wheat-E. sibiricus addition lines, O27-2 (BC8), O27-3 (BC12) and O193-3 (BC12), were developed through a backcrossing scheme in this study, using leaf rust field evaluations, molecular marker assays and cytogenetic analysis.
Methods: These three lines were derived from progeny of the bread wheat cultivar ‘Obriy’ (2n = 6x = 42, AABBDD) and partial octoploid amphiploid wheat-E. sibiricus (2n = 8x = 56, AABBDDStSt).
Results and discussion: The lines (O27-2, O27-3 and O193-3) demonstrated strong specific leaf pubescence (hairiness) and resistance at the adult stage to a local population of leaf rust races. The response to leaf rust in these three lines significantly differed from that of the Lr24 gene, providing evidence for a distinct resistance mechanism associated with the 3St chromosome. This study is the first to report the transfer of an E. sibiricus chromosome into wheat that confers leaf rust resistance. Molecular marker analysis and genomic in situ hybridization confirmed that lines O27-2, O27-3 and O193-3 each possess one pair of E. sibiricus 3St chromosomes. The resistance gene was determined to be on the additional alien chromosome in these lines. Molecular markers (Xwmc221, Lr29F18, Sr24/Lr24) confirmed that the lines O27-2, O27-3, and O193-3 each contain a pair of E. sibiricus 3St chromosomes carrying leaf rust resistance genes. These findings demonstrate that the E. sibiricus 3St chromosome carries the leaf rust resistance gene and that the O27-2, O27-3, and O193-3 lines can serve as novel germplasm sources for introducing this resistance into wheat breeding programs. This study contributes to broadening the genetic diversity of resistance genes available for combating leaf rust in wheat.
Introduction
Bread wheat (Triticum aestivum L., 2n = 6x = 42, AABBDD) is one of the most important food crops, serving as a daily staple for human consumption worldwide (Pont et al., 2019; Shiferaw et al., 2013). According to the Food and Agriculture Organization (FAO), a 60% increase in food production is needed to feed a world population exceeding 10 billion by 2050 (Feeding the World Sustainably | United Nations). To meet this escalating demand for global food security, wheat yields need to show an annual 2% increase (Hickey et al., 2019). Unfortunately, wheat diseases are actually reducing the global wheat harvest by approximately 20% per year (Savary et al., 2019).
The genetic variation in bread wheat germplasm is limited, making it challenging to develop high-yielding, quality grain-producing and disease-resistant wheat varieties (Sansaloni et al., 2020). To harness genetic diversity in wheat breeding programs, it is necessary to access genetic diversity in wild relatives of wheat harboring beneficial traits that can contribute to wheat improvement (Molnár-Láng et al., 2015). This strategy involves introgression of novel genes from Aegilops spp., Elymus ssp., Secale cereale etc., into wheat, aiming to enhance productivity and stability (Johansson et al., 2020; Motsnyi et al., 2016; Simonenko et al., 1998). Hexaploid bread wheat was derived through spontaneous interspecific crosses between T. urartu (2n = 2x = 14, AA), Aegilops sp. (2n = 2x = 14, BB) and Ae. tauschii (2n = 2x = 14, DD) (Appels et al., 2024). As a result, homoeologous transfer of valuable genes from secondary (Aegilops spp.) and tertiary gene pools is challenging due to the presence of the Ph1 and Ph2 genes, which inhibit homoeologous pairing (Mello-Sampayo, 1971; Riley and Chapman, 1958; Sears, 1956). The ZIP4 gene located at the Ph1 locus has been identified as a key gene in controlling crossover recombination, preventing homoeologous chromosome pairing during meiosis, and restricting gene transfer from wild relatives (Rey et al., 2017).
Introgression hybridization is a technique for transferring genetic information from wild species to wheat, thereby increasing its genetic diversity, especially in terms of rare traits (Jiang et al., 1994). This method enables addition of individual alien chromosomes to the wheat genome, leading to the development of novel genetic resources (Wang et al., 2010). New sources of disease resistance have been identified in wild relatives of wheat (e.g. Aegilops spp., Elymus ssp., S. cereale, Thinopyrum spp. etc.), and resistance genes from these species have been successfully transferred into wheat, providing broad-spectrum resistance. Leaf rust, caused by Puccinia triticina Erikss., is one of the most prevalent wheat diseases globally, and severe outbreaks can lead to wheat yield losses of over 40% (Knott, 2012; Kolmer et al., 2013). More than 80 wheat leaf rust resistance genes have been identified in wheat and its relatives, and more than half of these genes originate from wild relatives such as Aegilops spp., S. cereale, Elytrigia spp., Elymus spp. etc (McIntosh et al., 2020). Many important genes have been successfully transferred from wild relatives to wheat, such as Sr24/Lr24 from Th. elongatum, Lr38 from Th. intermedium, Lr55 from E. trachycaulis, Fhb3 from Leymus racemosus and Fhb6 from E. tsukushiensis (Cainong et al., 2015; Friebe et al., 1996; McIntosh et al., 2020; Qi et al., 2008). Genetic diversity from Elymus sibiricus has also been successfully transferred into the wheat genome (Motsnyi et al., 2016; Motsnyi and Kulbida, 2018).
Siberian wild rye (Elymus sibiricus L., 2n = 2x = 28, StStHH) is an important source of new genetic diversity for wheat improvement (Klebesadel, 1969; Tsvelev, 1976). This crop exhibits high resilience, is distinguished by excellent palatability and adaptability, and shows outstanding tolerance to salinity, drought and cold growing conditions (Zhang et al., 2016). E. sibiricus also serves as a source of resistance to various wheat fungal and virus diseases (Braverman, 1986). This grass species is a promising gene pool source for wheat improvement and could greatly contribute to wheat breeding. However, it has rarely been hybridized with wheat, suggesting that there is significant untapped potential in this area (Wang, 2011). Scientists at the Plant Breeding and Genetics Institute-National Centre for Seed and Cultivar Investigation (PBGI-NCSCI) in Odesa, Ukraine, have developed an amphiploid line combining wheat and E. sibiricus genomes (Simonenko et al., 1998). The underlying aim was to enhance wheat-breeding programs by exploiting the unique genetic characteristics of E. sibiricus. Recent developments have resulted in alien disomic addition lines obtained by crossing the wheat cultivar Obriy with the amphiploid wheat-E. sibiricus line (Motsnyi and Kulbida, 2018). This represents a significant milestone in plant genetics, illustrating the potential of innovative combination of diverse genetic attributes to produce enhanced wheat varieties. The aim of the present study was to identify the alien addition in these lines, assess their cytological stability and investigate how this addition affects wheat disease resistance. The focus was on understanding genetic integration and its practical implications for enhancing wheat disease resistance.
Materials and methods
Plant materials
An amphiploid (2n = 8x = 56) wheat-E. sibiricus was provided by Dr. R. Franke from the Institute of Breeding Research, Quedlinburg, Germany (personal communication, 2024). E. sibiricus (genomic composition 2n = 2x = 28, StStHH) was crossed with bread wheat (genomic composition 2n = 6x = 42, AABBDD) to produce the partial amphiploid (2n = 8x = 56, AABBDDStSt) Elytricum fertile (hereafter called EF3). Throughout the process, cross-pollination was controlled, and careful screening and selection were performed to ensure the successful integration of genetic material from both parents. Genomic in situ hybridization (GISH) was conducted at the Eastern Cereal and Oilseed Research Centre, Agriculture and Agri-Food Canada, where the genomic composition of the amphiploid was determined to be 2n = 8x = 56, AABBDDStSt (Dr. G. Fedak, pers. comm. 2024). The SLU-9702 accession of E. sibiricus (2n = 4x = 28, StStHH) provided by Dr. A.V. Agafonov of the Central Botanic Garden of SB RAS, Novosibirsk, Russia, was used.
Elytricum fertile (EF3) was crossed with the cultivar Obriy, resulting in ~100 F1 seeds (Figure 1). These F1 plants were then self-pollinated or backcrossed to Obriy, producing about 170 F2 and 30 BC1F1 seeds. A total of 132 F2 and 29 BC1F1 plants were assessed at the adult stage for leaf rust reaction and leaf hairiness in the field, to select resistant plants that exhibited leaf hairiness (pubescence) for further backcrossing (Figure 1). The backcrossing process was carried out in the field, focusing on selecting leaf rust-resistant plants that exhibited leaf hairiness, with this approach continuing until the BC12F1 generation was obtained (Figure 1).
Evaluation of disease resistance and leaf pubescence in the field
From 2013 to 2024, the BC12F3 to BC12F∞ generations were grown in wide rows in the field PBGI-NCSCI. During this period, the lines were exposed each growing season to natural epidemics of leaf rust (Puccinia triticina Eriks.), yellow rust (Puccinia striiformis f. sp. tritici) and stem rust (Puccinia graminis f. sp. tritici). The level of natural infection by leaf rust was consistently observed every year, providing an ideal environment to evaluate resistance to this pathogen. Yellow rust epidemics occurred every 3 to 4 years throughout the study, while stem rust appeared less frequently every 4 to 5 years. We planted the highly susceptible wheat cultivars Odeska 267, Obriy, and Kuyalnik in the field nurseries for leaf rust and Michigan Amber and Odeska Polukarlicova for yellow rust and stem rust to assess natural disease pressure and monitor infection levels. These susceptible cultivars served as infection indicators, confirming the presence and spread of the pathogens for a reliable evaluation of rust resistance in the BC12F3 to BC12F∞ generations under natural infection conditions. In addition, the BC8F4 to BC8F9 and BC12F4 to BC12F9 generations were tested separately under artificial inoculation for leaf rust and stem rust, respectively. The plants were artificially inoculated with a mixture of talcum powder and urediniospores of leaf rust and stem rust using a knapsack sprayer. Artificial nurseries were mist-irrigated three times daily (morning, afternoon, and evening) to maintain a moist environment and stimulate rust development. Four susceptible wheat cultivars for leaf rust and six for stem rust were used as spreader rows, positioned perpendicular to the test plots in the main wind direction to facilitate disease spread. Disease severity was assessed at the booting, heading, and dough stages using the method described by Babayants and Babayants (2014) once the susceptible spreader plants reached full infection. The plants were consistently assessed for their resistance to three rust diseases (yellow rust, stem rust, and leaf rust), and leaf hairiness (pubescence) under both natural and artificial infection conditions. Adult plant infection responses (size and type of uredinia) (Roelfs et al., 1992; Babayants and Babayants, 2014) and leaf tissue infections by the three rust diseases (0–100%) were assessed in the field using the modified Cobb scale (Peterson et al., 1948) at Zadoks growth stages 45–60 (Zadoks et al., 1974).
The leaf rust reactions of BC12F2 populations, derived from plants with 43 chromosomes and resistant to leaf rust, were evaluated in the field, using Obriy as the susceptible control. These populations were exposed to natural infection pressure and, when the cultivar Obriy was fully infected, both the parent plants and the BC12F2 individuals were assessed for their resistance to leaf rust. Phenotypic evaluations were conducted during the heading and flowering stages (Zadoks stage 50-70). To determine leaf hairiness (pubescence), the upper (adaxial) surface of leaf blades was examined using a magnifying glass. This detailed field evaluation provided a comprehensive understanding of the resistance mechanisms and phenotypic traits in these wheat lines, contributing valuable information regarding leaf rust resistance in wheat.
Acetocarmine staining
The cytological examination was performed using standard acetocarmine staining techniques. After fixing the anthers in Carnoy fixative (containing 99% alcohol:chloroform:glacial acetic acid in a 6:3:1 ratio) and pre-treatment with 4% iron-ammonium alum, the anthers were stained with 2% acetocarmine. The acetocarmine staining analysis was performed on generations from BC1F1 through BC12F1, BC8F1 to BC8F6, and BC12F2 to BC12F6. All hybrid plants with the E. sibiricus traits of leaf hairiness and resistance to leaf rust, as well as some plants without the E. sibiricus traits were analyzed cytologically. At each plant, at least 30 distinct plates at the meiotic metaphase 1 (M1) stage were examined in pollen mother cells (PMCs). Univalent, open and closed bivalents were treated.
Statistical analysis
The data were analyzed using descriptive statistics and analysis of variance (ANOVA) with the STATISTICA 8 software package (Statsoft, 2005). Before statistical analysis, percentage values were converted to Fisher’s angular coefficient (φ) in radians to normalize the frequency distribution. A 95% confidence interval and the least significant difference at the p<0.05 level (LSD0.05) were employed to determine the differences’ significance. For comparing data expressed as percentages, confidence limits were calculated using the formula for alternative variability: t×Sp, where t – Student’s coefficient at p<0.05 = 1.96, and Sp – standard error for alternative variability, calculated as (Fomich, 1973).
Fluorescence in situ hybridization
Chromosome slide preparation
Mitotic cells from the 2- to 3-day-old root-tip meristems of BC12F6 (O27-2) and BC12F6 (O27-3 and O193-3) disomic lines were treated in ice-cold water for 24 hours, and used for chromosome slide preparation as described by Jenkins and Hasterok (2007). Seeds were germinated on moistened filter paper at 20-22°C in darkness until the roots reached 1.5-2 cm long. The seedlings were then fixed in a 3:1 (v/v) mixture of methanol and glacial acetic acid and stored at -20°C until needed. The excised roots were washed in 0.01 M citric acid-sodium citrate buffer (pH ~4.8) for 20 minutes and then subjected to enzymatic digestion for 1-1.5 hours at 37°C using a mixture of 1% (w/v) cellulose (Calbiochem), 1% (w/v) Onozuka R-10 cellulase (Serva) and 20% (v/v) pectinase (Sigma). After separating non-meristematic parts, the root tips were squashed in a drop of 45% acetic acid, and the preparations were rapidly frozen. Following the removal of coverslips, the slides were air-dried and stored in a refrigerator for future use.
DNA probes
The following probes were used: (1) For GISH, total nuclear DNA was extracted from E. sibiricus seeds (Saghai-Maroof et al., 1984) and then labeled by nick translation with digoxigenin-dUTP (Hasterok et al., 2002; Maluszynska, 2002). (2) The 25S rDNA probe was produced using nick translation with tetramethyl-rhodamine-dUTP on a 2.3 kb ClaI subclone of the 25S rDNA coding region from Arabidopsis thaliana (Unfried and Gruendler, 1990). This probe effectively identified the loci of the 18S-5.8S-25S rRNA genes in FISH experiments.
FISH procedure
The slides were initially treated with RNase (100 μg/mL) in 2×SSC at 37°C for one hour, followed by a wash in 2×SSC (saline sodium citrate) and dehydration in ethanol. The hybridization mixture for the cloned probes consisted of 50% deionized formamide, 10% dextran sulphate, 2× SSC, 0.5% SDS and approximately 3 ng/L (25-100 ng/slide) of each probe DNA. This mixture was pre-denatured at 75°C for 10 minutes before application to chromosome preparations. Slides and DNA probes were denatured at 75°C for 3 minutes in an in situ thermal cycler (Hybaid), and then hybridized overnight at 37°C in a humid chamber. Following stringent washing (10-20% formamide in 0.1×SSC at 42°C for 10 minutes), immunodetection of digoxigenated probes was performed using FITC-conjugated anti-digoxigenin antibodies (Roche). Finally, counterstaining and mounting were carried out using 2.5 g/mL DAPI in a Vectashield antifade buffer.
Image capturing and processing
Images were captured using two set-ups: an AxioCam MRm monochromatic camera (Zeiss) attached to a Zeiss AxioImager.Z.2 wide-field epifluorescence microscope and an Olympus Camedia C-4040Z digital camera connected to a Leica DMRB epifluorescence microscope. Image processing and superimposition were conducted using FIJI software. Three slides from different meristems were analyzed for each disomic line and its parental forms, including the amphiploid EF3 and T. aestivum.
Molecular marker analysis
DNA was extracted from young leaves, and PCR analysis and fragment detection were performed using the CTAB procedure (Saghai-Maroof et al., 1984). The DNA was isolated from six individual plants from each parental form, which included Elymus species, EF3, and cv. Obriy, and 10 individual plants from each experimental line. We also used lines containing the Lr34 resistance gene and Lr genes transferred from the St genome of Th. ponticum to the bread wheat genome, such as Lr19 (7DL-7Ae#1L), Lr24 (3DL-3Ae#1L), and Lr29 (DL-7Ae#1L·7Ae#1S). The molecular markers Xwmc221 for Lr19, Sr24/Lr24#12 for Lr24, Lr29F18 for Lr29, and csLV34 for Lr34 were assessed (Galaev and Sivolap, 2015; Gorash et al., 2014).
Field evaluations of agronomic performances
Field experiments were conducted at PBGI-NCSCI. Sowing was performed using a tractor-drawn breeding seed drill, SSFK-7, with each plot covering 10 m2 and a seed rate of 5 million germinating grains/ha. The number of spikes per m2 was assessed using a 0.5 m × 0.5 m frame placed in the middle of each plot, from which 25-30 plants were randomly selected for agronomic performance analysis. These traits encompassed heading date, plant height, spike length, spikelet number, spike density, number of kernels per spike and thousand kernel weight. Grain quality was evaluated using the SDS30′K sedimentation value, as described by Rybalka et al. (2006). Protein content was determined using the Kjeldahl method with a Kjeltec-Auto 1030 instrument (Foss Electric, Denmark), while the thousand kernel weight was measured according to the DSTU 4138-2002 method.
Results
Population development
In this study, addition lines were successfully developed through a backcrossing scheme using Elytricum fertile (EF3), and the recurrent cultivar Obriy (Figure 1). Selection of lines was based on a chromosome count of 2n = 43, determined by observing acetocarmine-stained chromosomes in pollen mother cells. Plants with a chromosome number of 2n = 43 (21wII+1eI), along with necrotic resistance to leaf rust and strong pubescence on the upper surface of the leaf blade, were selected through each backcross generation (BC1F1 to BC1F12). The development process involved two independent, parallel series of backcrosses initiated from two distinct second-generation plants that showed clear alien traits: 27-94 (BC1F1 Obriy/EF3//Obriy, 2n = 48) and 193-94 (F2 Obriy/EF3 self, 2n = 45+t). In the progeny derived from the plant 27-94, an individual plant BC8F1 with a chromosome count of 2n = 44; 22II, displaying distinct marker characteristics was identified after the eighth backcross without self-pollination. After completing 12 backcrosses (as shown in Tables 1, 2) and a final round of self-pollination involving 43 chromosome plants, two more independent disomic addition lines, O27-3 and O193-3, were developed. Each of the developed lines (O27-2, O27-3, and O193-3) was found to have one pair of intact E. sibiricus chromosomes in addition to the bread wheat genome. All three lines exhibited leaf pubescence (hairiness) and resistance to leaf rust, whereas the wheat cultivar Obriy showed glabrous leaves and was susceptible to the disease (Figure 2).
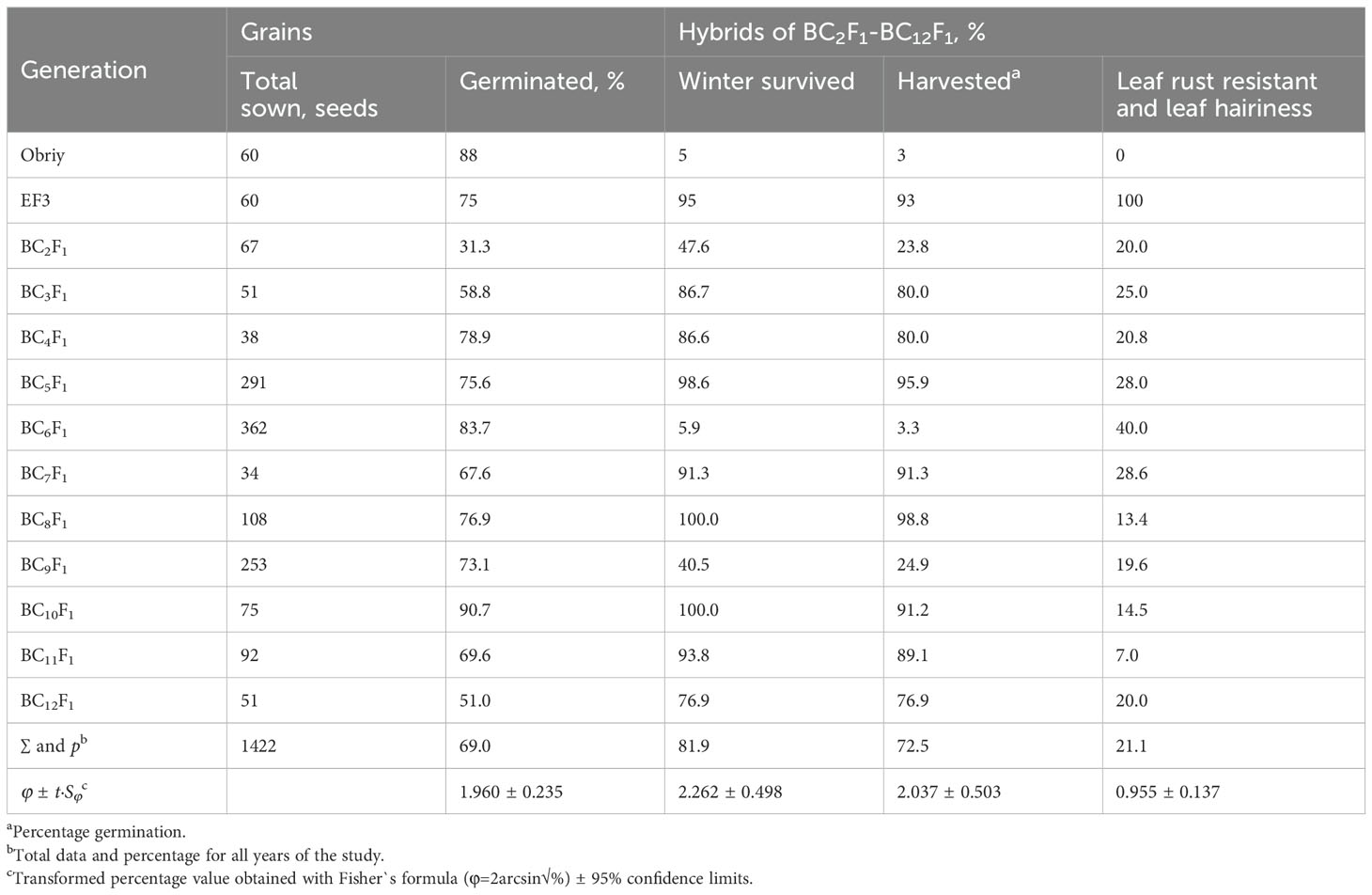
Table 1. Frequency of plants with the St chromosome of E. sibiricus transmitted through female gametes in the family 27-94 offspring of hybrids Obriy/EF3//Obriy*2-12.
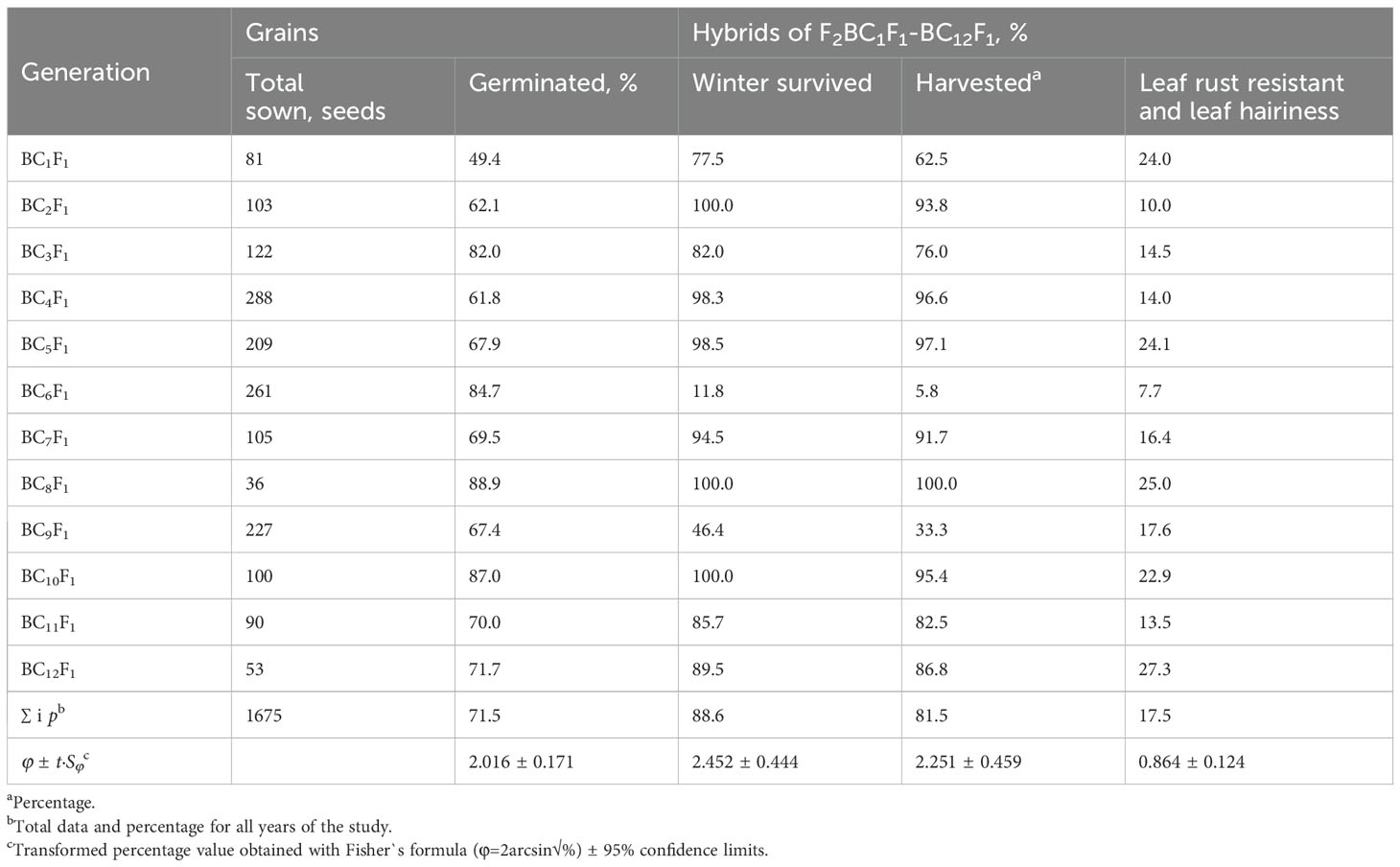
Table 2. Frequency of plants with the St chromosome of E. sibiricus transmitted through female gametes in the family 193-94 offspring of backcrossed hybrids Obriy/EF3 self//Obriy*2-12.
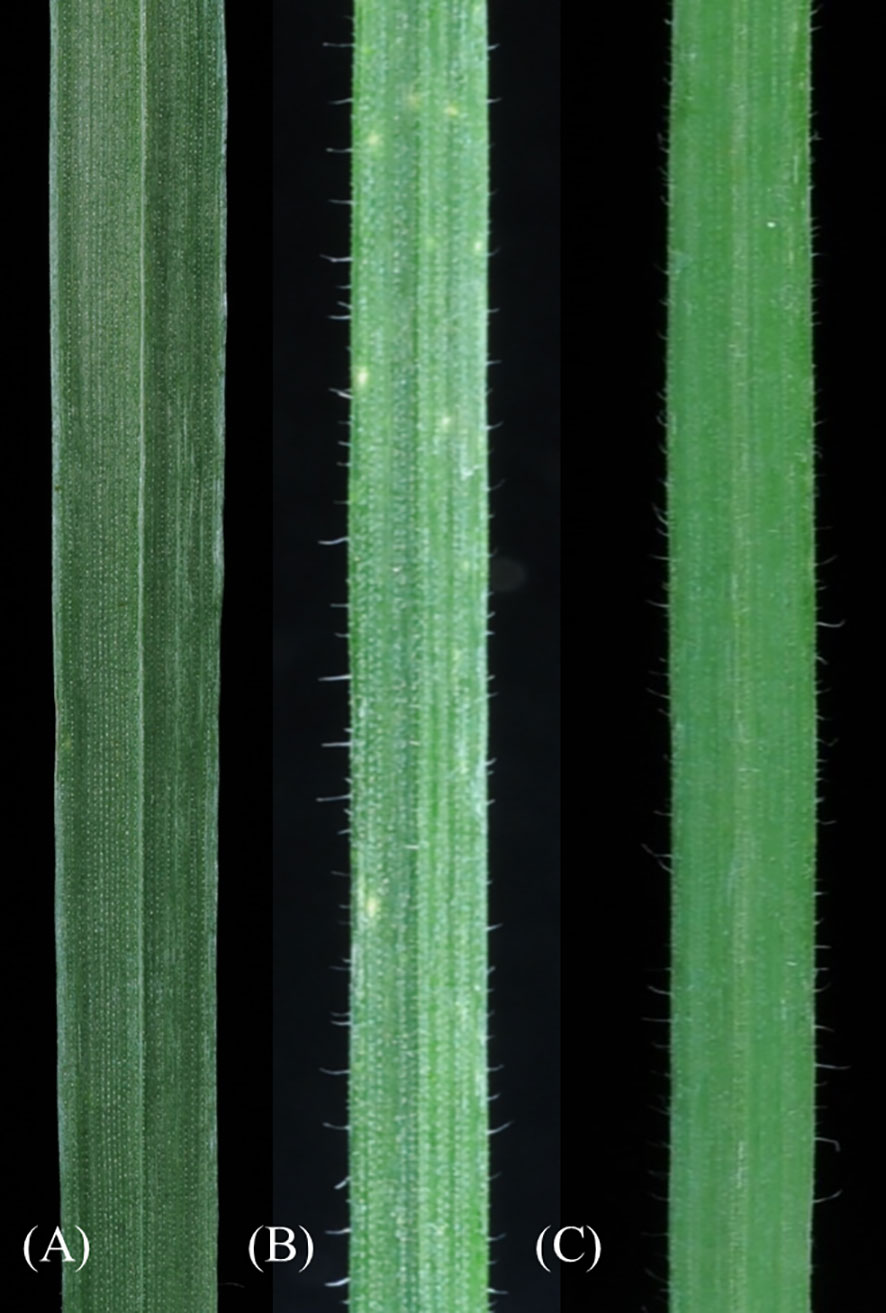
Figure 2. Leaf glabrous and pubescence (hairiness) morphologies. (A) Cultivar Obriy (2n = 42), showing glabrous leaves, (B) EF3 (2n = 8x = 56), exhibiting leaf pubescence, and (C) the addition line O27-3 (2n = 44) with leaf pubescence.
Leaf pubescence and resistance to leaf rust characteristics of addition lines
The EF3 amphiploid showed a spectrum of responses to leaf rust, yellow rust and stem rust, ranging from high to moderate resistance, whereas the cultivar Obriy displayed a range from moderately susceptible to very susceptible (Table 3). All three addition lines (O27-2, O27-3 and O193-3) showed high resistance to leaf rust, while they displayed moderate susceptibility to susceptibility to yellow rust and stem rust in field conditions (Table 3). The resistance responses to leaf rust were characterized by small to medium-sized necrotic flecks on wheat leaves, without any visible pustules (Figure 3). Recurrent wheat cultivar Obriy displayed the most severe disease symptoms, exhibiting complete susceptibility (S) to leaf rust (Figure 3). The addition lines and amphiploid EF3 exhibited leaf hairiness, whereas the cultivar Obriy was characterized by its lack of leaf hairiness (fully glabrous) (Figure 2). The observed leaf hairiness is associated with the leaf rust resistance gene identified in EF3, O27-2, O27-3 and O193-3 (Table 3).
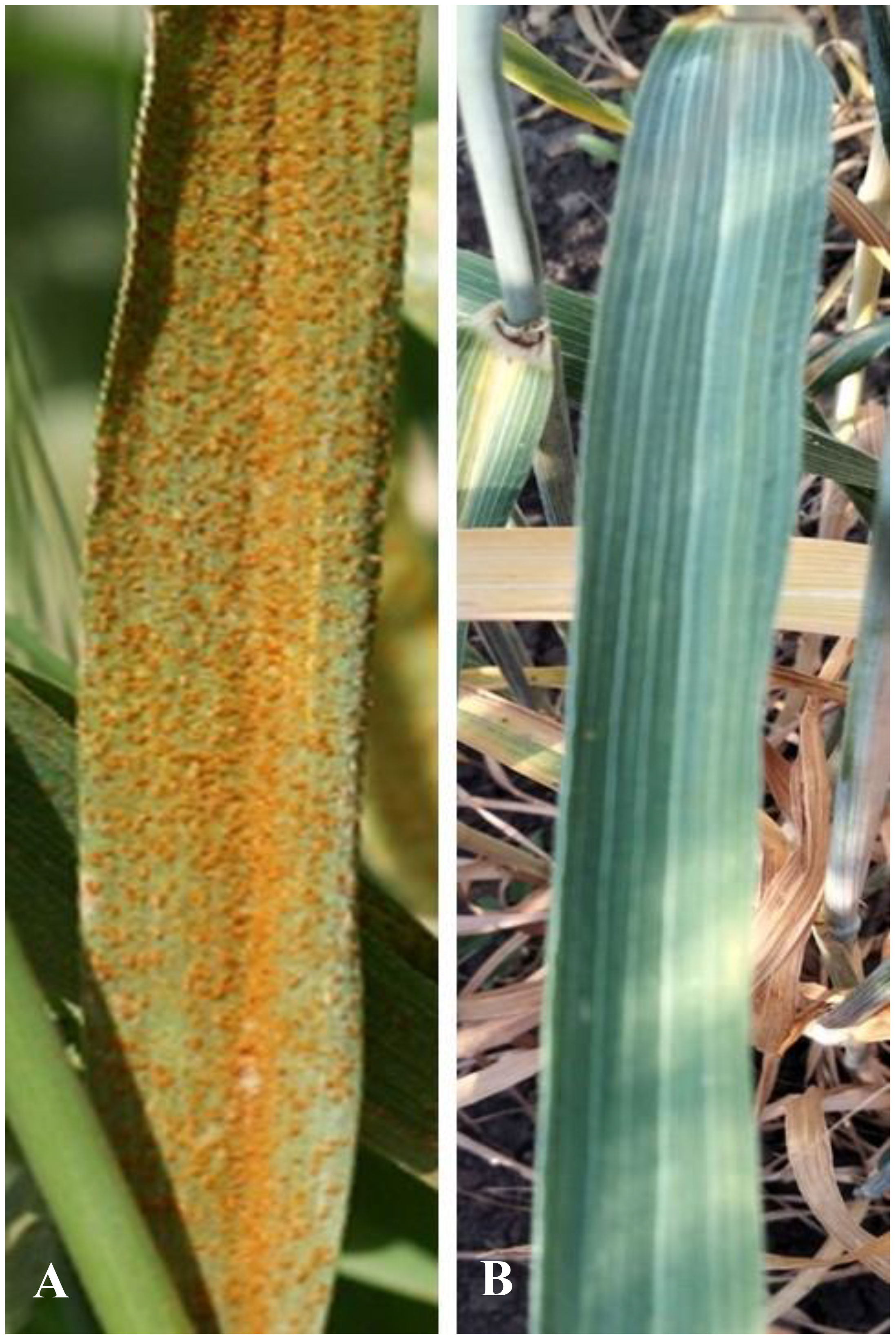
Figure 3. Field response to leaf rust. (A) Upper surface of the leaf blade of the bread wheat (Tr. aestivum) cultivar Obriy (2n = 42), showing leaf rust susceptible, and (B) the line О193-3 (2n = 44), showing resistance to leaf rust.
Location of disease resistance and leaf pubescence genes on the additional chromosome
An analysis of the 27-3-94 BC12F2 segregation population, consisting of 158 individuals, revealed significant phenotypic and chromosomal differences (Table 3). Of these, 44 plants displayed resistance to leaf rust, and were pubescent, while the remaining 114 plants were susceptible to leaf rust, and had glabrous leaves. Meiotic analysis revealed that these groups exhibited a variety of chromosomal configurations. In the resistant-pubescent subset, two plants exhibited a chromosome count of 2n = 22II = 44 (Figure 4A), whereas the remaining 42 plants had a configuration of 2n = 21II+1I = 43 (Figure 4B). All 114 susceptible-glabrous plants consistently exhibited a chromosome configuration of 2n = 21II = 42 (Figure 4C). Analysis of the BC12F2 population of 193-3-94, comprising 385 individuals, provided a number of additional insights. A total of 71 plants containing marker characters had a chromosome set of 2n = 21II+1I = 43. Interestingly, only one plant was identified as a disomic addition with 2n = 22II = 44 chromosomes, while 313 plants without alien characters were confirmed to have 42 chromosomes (Table 4). The addition chromosome was not observed to pair with wheat chromosomes, based on the absence of multivalents. All resistant-pubescent plants possessed one or two E. sibiricus chromosomes, whereas none of the susceptible-glabrous plants possessed any E. sibiricus chromosomes. The correlation between leaf rust resistance and pubescence was determined by analyzing phenotypic and chromosomal differences in the segregated population. Meiotic observations revealed that the genes responsible for resistance and hairiness in lines O27-2, O27-3, and O193-3 are located on an additional St chromosome from E. sibiricus. These characteristics were validated in the analysis as potential phenotypic markers.
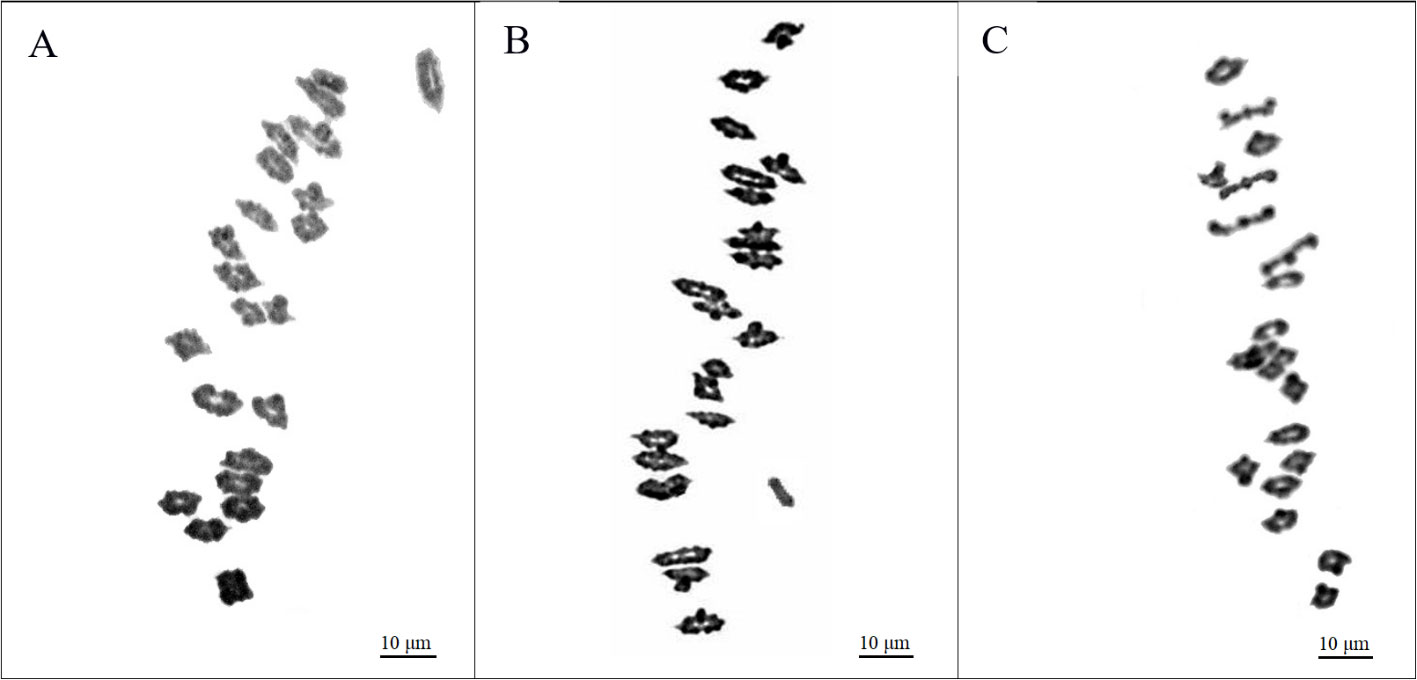
Figure 4. Chromosome behavior at meiotic metaphase I in pollen mother cells in typical resistant-pubescent and susceptible-glabrous plants from the ВС12F2 population of Obriy/EF3//Obriy*12. (A) Resistant-pubescent plant (2n = 22II = 44). (B) Plant with 2n = 21II + 1I = 43, the univalent being an E. sibiricus chromosome which carries leaf rust resistance and pubescence, indicated by an arrow. (C) Typical susceptible individual (2n = 21II = 42), no added E. sibiricus chromosome observed.
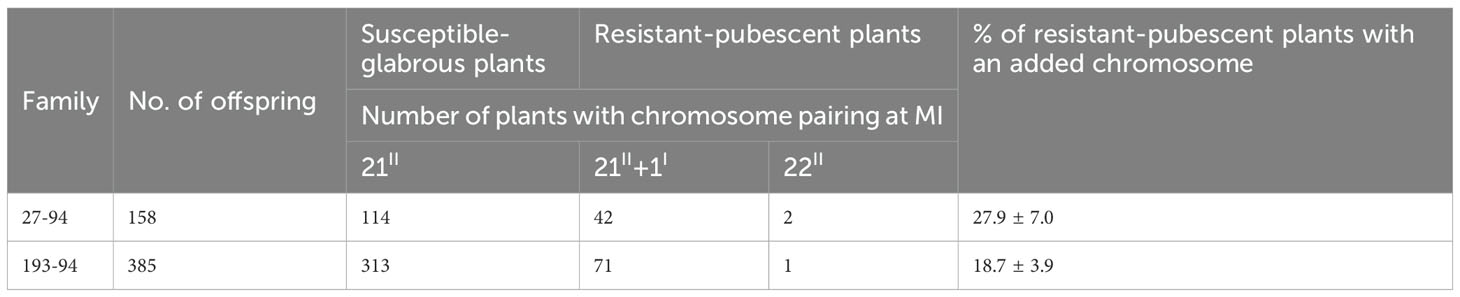
Table 4. Chromosome constitution of resistant-pubescent and susceptible-glabrous individuals obtained from self-pollination of BC12 43-chromosome plants Obriy/EF3//Obriy*12.
Acetocarmine-stained chromosomes in pollen mother cells
Most PMCs of lines O27-2, O27-3 and O193-3 showed 22 bivalents at meiotic metaphase I, indicating cytological stability. While 11.4 ± 4.1% of the cells had 21 bivalents and two univalents, 88.6 ± 4.1% maintained the full 22 bivalents. The presence of the alien chromosome in a disomic addition condition disrupted homologous chromosome pairing, resulting in aneuploidy. The result was that approximately 10% of the progeny from 44-chromosome plants resulted in aneuploids, mostly with 43 chromosomes. This disruption was seen at metaphase I as increased univalents and open (rod) bivalents. On average, the disomic addition lines had 0.26 ± 0.10 univalents and 1.75 ± 0.20 rod bivalents per PMC, compared to the recurrent variety Obriy, which had 0.13 ± 0.05 univalents and 1.12 ± 0.11 rod bivalents per cell. The addition lines also had slightly more closed (ring) bivalents, with 20.1 ± 0.2 compared to 19.8 ± 0.1 in Obriy. Although wheat and alien chromosomes couldn’t be distinguished directly in this analysis, a clear reduction in homologous wheat chromosome pairing was observed in aneuploid plants where the Elymus chromosome was always univalent (Figure 4B). This reduction was likely due to an unfavorable interaction between wheat and E. sibiricus genes, leading to partial or complete early bivalent splitting (desynapsis), which disrupted chromosome pairing stability. The impact of these genetic interactions was generally mild, but led to a slight increase univalents (on average 0.13 per PMC) and more frequent rod bivalent formations (on average 0.63 per PMC). Univalents were observed in around 11.4 ± 4.1% of PMCs, mainly with two observed per PMC. Only 1.3 ± 1.5% of PMCs contained four univalents, while cells containing more than four univalents were not identified. The recurrent cultivar Obriy, on the other hand, had 21 bivalents in 93.5 ± 1.7% of PMCs. Thus, disomic addition of the E. sibiricus chromosome, which determines leaf rust resistance and hairiness, to the karyotype of the cultivar Obriy most significantly negatively impacted homologous chromosome pairing.
Fluorescence in situ hybridization analysis of addition lines
Phenotypic analysis of the BC12F6 (O27-2) and BC12F6 (O27-3 and O193-3) stable disomic lines showed the presence of E. sibiricus chromosome, as indicated by two notable traits: necrotic resistance to leaf rust and specific pubescence of the leaf blades. FISH analysis was used to confirm the presence of genetic material from E. sibiricus at cytogenetic level. For analysis of partial amphiploid EF3 and all disomic lines, this technique used the total genomic DNA of E. sibiricus, which is fluorescent in green, and 25S ribosomal DNA (rDNA) probes, which are fluorescent in red. The results indicated presence of E. sibiricus genetic material in all investigated lines and parental forms (Figure 5). Each line contained 44 chromosomes, 42 of which were wheat chromosomes and two of which were E. sibiricus chromosomes (Figures 5A–C). Application of the 25S rDNA probe revealed the typical wheat distribution of 45S rDNA loci on the 1B and 6B satellite chromosomes (marked by red spots) and the 5D chromosomes (Figure 5D). The additional chromosomes in the disomic addition lines did not carry 45SrDNA loci and appeared submetacentric (Figures 5A, B), further substantiating their alien origins.
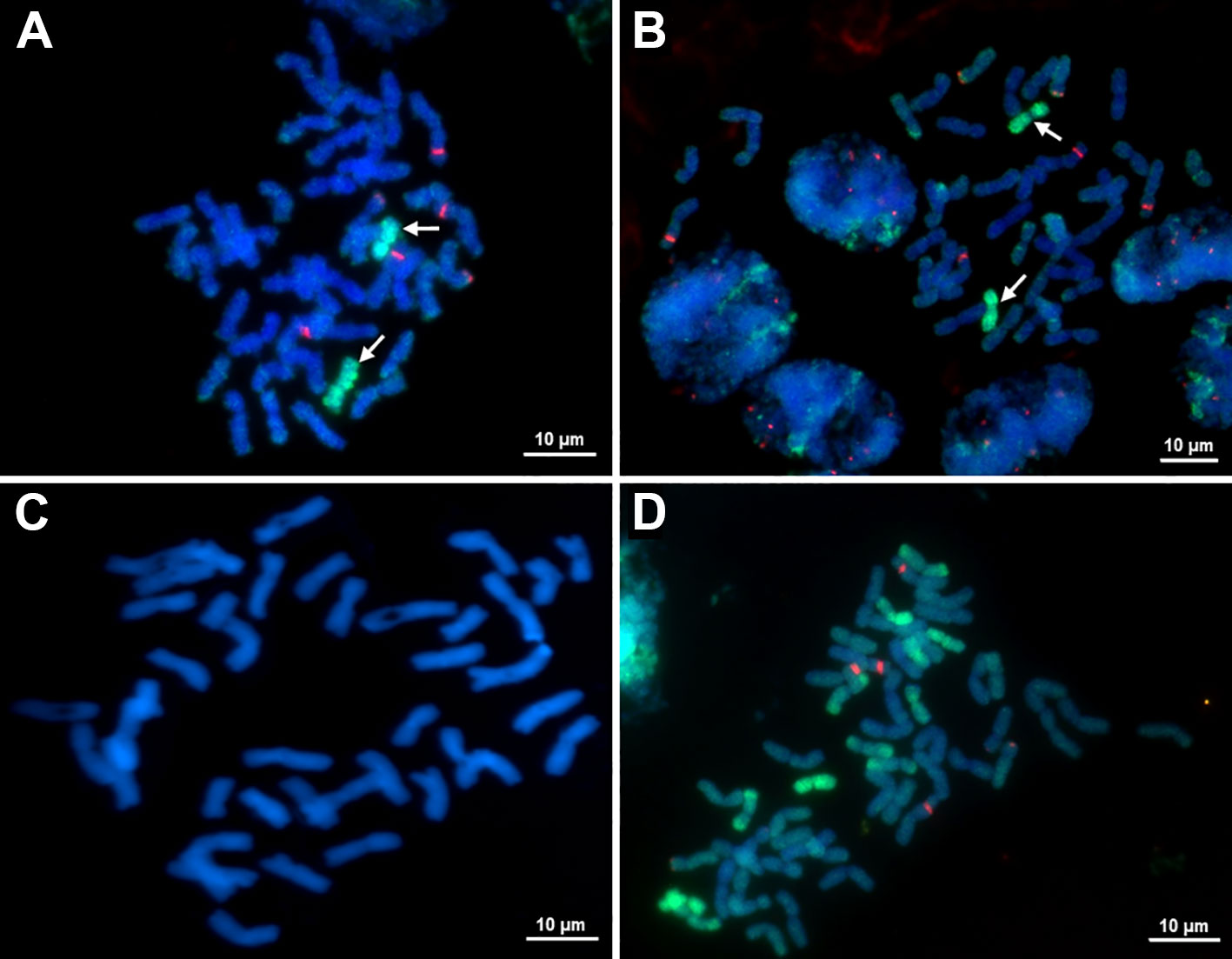
Figure 5. Metaphase chromosomes of (A) O27-2 BC8F6 disomic additional lines (2n = 44), (B) O27-3 BC12F6 disomic additional lines 2n = 44), (C) Mitotic metaphase chromosomes of the recurrent parent cultivar Obriy (2n = 42) stained with DAPI, and (D) amphiploid EF3 (2n = 56). Green fluorescence indicates hybridization of E. sibiricus genomic DNA, red fluorescence indicates hybridization of 25S rDNA.
Molecular markers specific to the alien additional chromosomes
The markers Xwmc221 (7DL-7Ae#1L) and Lr29F18 (DL-7Ae#1L·7Ae#1S) were not detected in lines O27-2, O27-3 and O193-3. In contrast, the Sr24/Lr24#12 marker (3DL-3Ae#1L) with 500 bp size was positively amplified in EF3, O27-2, O27-3 and O193-3, but not in the cultivar Obriy (Table 5 and Figure 6). Hence, the presence of the Sr24/Lr24#12 marker, which is located on the 3St chromosome, indicates that the chromosome added from E. sibiricus in these lines is in fact the 3St chromosome. These specific markers made it possible to determine the location of the genetic material from E. sibiricus in the added alien chromosome, enhancing understanding of the genomic composition of these addition lines. It was found that three Elymus species from the PBGI-NCSCI collection did not carry the 500 bp locus of the Sr24/Lr24#12 marker (Figure 6). This finding indicates that the 3St chromosome in the amphiploid EF3 karyotype may have originated from other species or samples within the genus Elymus (or Agropyrum), unlike E. sibiricus. Alternatively, it could point to heterogeneity in E. sibiricus, suggesting its potential role as a diverse alien genome donor species in the amphiploid. The absence of the 500 bp locus in certain plants underscores the complexity and variability of genetic contributions in these hybrid lines, highlighting the necessity of further exploring the genetic composition and origins of the 3St chromosome in EF3.
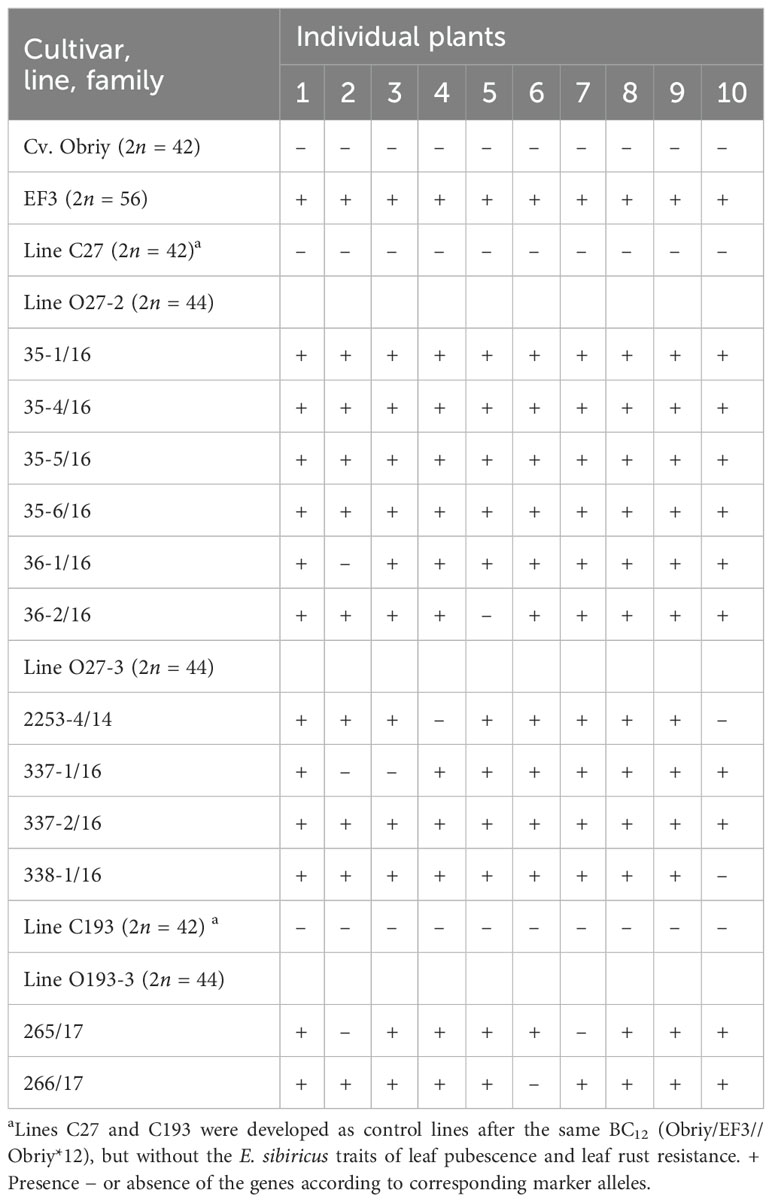
Table 5. Results obtained using the Sr24/Lr24#12 PCR marker to detect the Lr24 (3St) leaf rust resistance gene from Th. ponticum in paternal forms and disomic addition wheat lines.
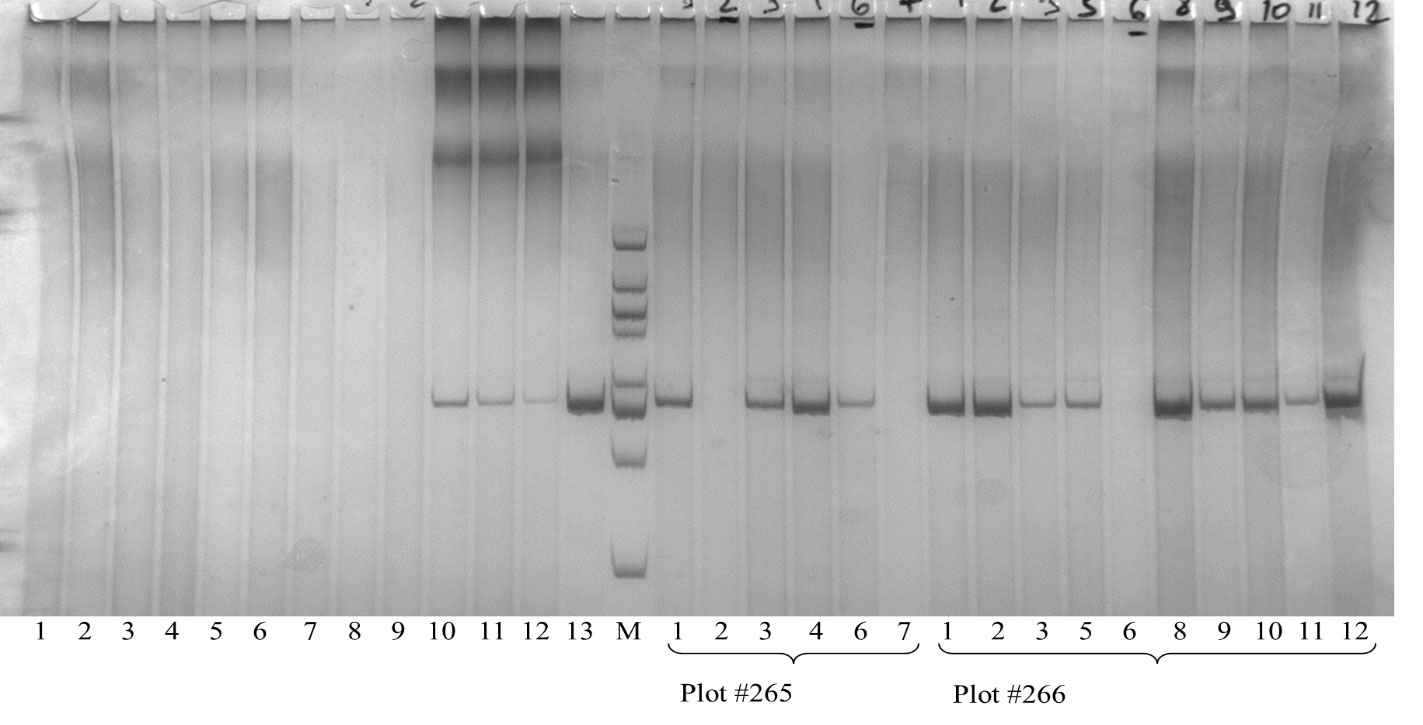
Figure 6. DNA amplification products (500 bp) of Elymus species and disomic addition lines at the microsatellite locus Sr24/Lr24#12. M – molecular weight marker 20 bp ladder; 1-3 – E. canadensis; 4-6 – E. dahuricus; 7-9 – E. sibiricus; 10-12 – amphiploid EF3; 13 – Agent (T3DS.3DL-3Ae # 1, cultivar-carrier of Lr24/Sr24 genes); 1-7 – individual plants from plot #265 (according to Table 5); 1-12 – individual plants from plot #266 (line O193-3).
The karyotype of the specified disomic addition lines contains an additional pair of chromosomes from the St genome of the tetraploid E. sibiricus (2n = 4x = 28, StStHH). We also observed phenotypic differences between disomic addition lines, where leaf rust resistance is characterized by necrosis without stem rust resistance (Figure 3, Table 3), and cultivars and lines containing the typical Lr24 gene. Lines O27-2, O27-3, and O193-3 were confirmed to be homozygous for the E. sibiricus 3St chromosome and showed high levels of leaf rust resistance (Table 3). These lines consistently exhibited strong resistance across multiple growing seasons under natural and artificial leaf rust infection conditions. As no leaf rust resistance genes have been previously reported from E. sibiricus, this study is the first to successfully transfer and integrate an E. sibiricus chromosome into the wheat genome that confers leaf rust resistance. This finding opens new avenues for understanding the genetic basis of disease resistance in wheat and highlights the complexity of resistance mechanisms involving multiple genes and alleles.
Some plants in the three-disomic addition line families did not exhibit DNA amplification products at the Sr24/Lr24#12 marker locus (Table 5 and Figure 6). The absence of these traits cannot be attributed to cross-pollination or contamination, as that would result in plants lacking the marker traits with 43 or 42 chromosomes. We employed a combination of molecular markers, phenotypic traits and cytological analysis to select 44-chromosome plants that exhibited leaf pubescence, resistance to leaf rust and the presence of a 500 bp amplicon at the Sr24/Lr24#12 microsatellite marker locus. This rigorous selection process was crucial for maintaining line constancy and ensuring genetic material integrity in subsequent generations. A focus on these specific characteristics led to isolation and propagation of plants retaining the desired phenotypic characteristics, confirming the presence of the target genetic segment and contributing significantly to the understanding and development of wheat varieties resistant to leaf rust.
Agronomic performance
Lines containing the E. sibiricus 3St chromosome with Lre (resistance to leaf rust) and Hle (leaf hairiness) genes were found to exhibit significantly lower fertility (averaging 46.6 ± 2.6 grains per main spike) compared with the recurrent cultivar Obriy (66.4 ± 2.9 grains per main spike). The chromosome with Lre and Hle genes from E. sibiricus, associated with leaf rust resistance and increased hairy leaves, may contribute to the reduced fertility observed in these addition lines. The introgressed E. sibiricus chromosome lowered seed set and negatively affected traits such as plant height, productive tillering, spikelet count in the main spike, main spike density and thousand kernel weight, which varied annually (Table 6). Other characteristics, such as heading date, number of seeds per spikelet and sedimentation values, did not differ significantly (Table 6). Only the protein content increased in these lines, with O27-3 showing 15.8% compared to 13.4% in Obriy (Table 6). Disomic-addition line O27-3 displayed shorter growth, reduced spike density (310/m2) and lower grain yield = (2.86 t/ha) compared with the recurrent parent (Table 6).
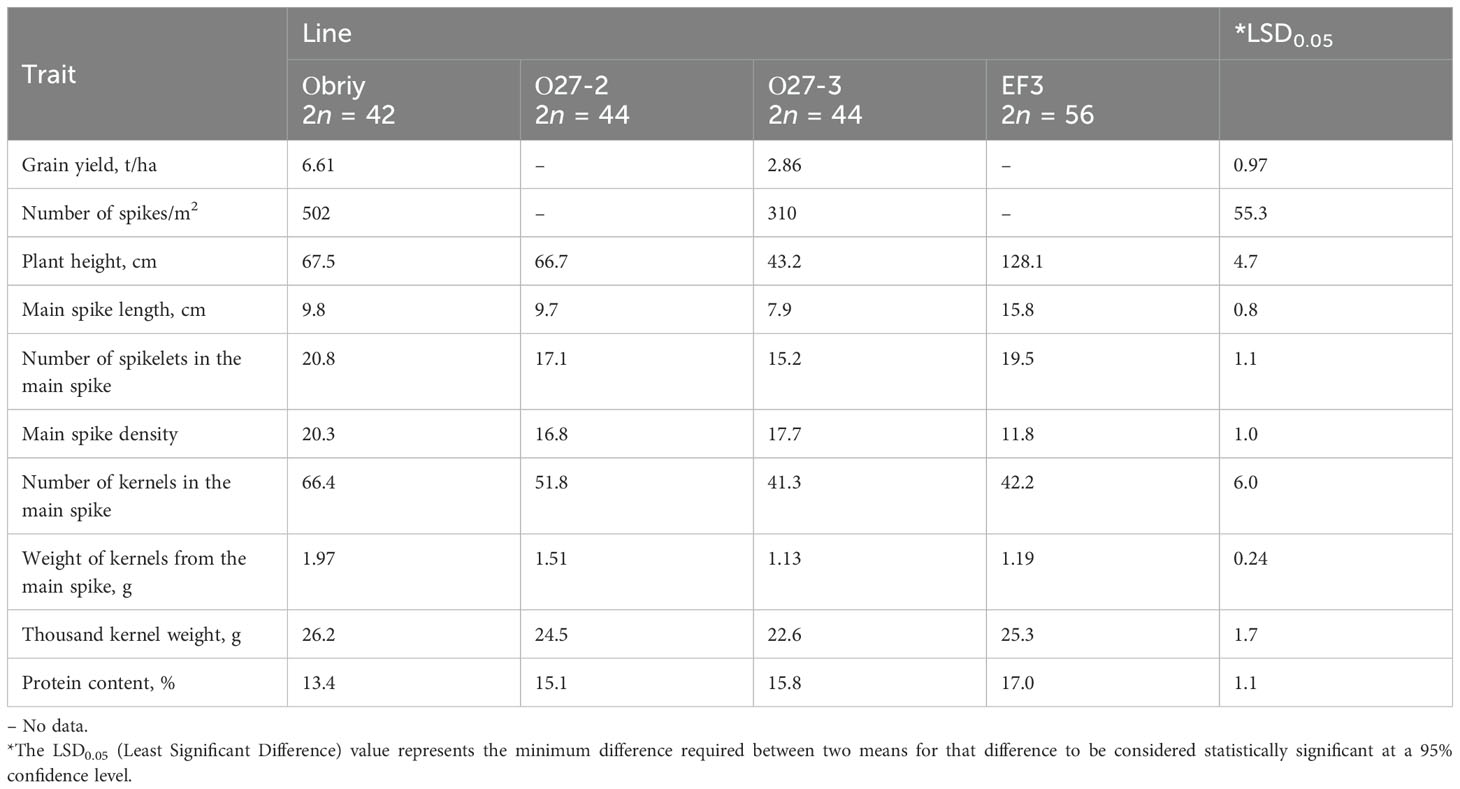
Table 6. Average values of traits characterizing the development of plants of Obriy, O27-2, O27-3 and Elytricum fertile (EF3) lines in the period 2022-2023.
Discussion
In this study, three wheat-E. sibiricus disomic addition lines (O27-2, O27-3 and O193-3) were successfully identified. They were all based on E. sibiricus chromosome (St), as confirmed by leaf rust field evaluations, molecular marker assays and cytogenetic analysis (GISH, FISH and acetocarmine staining). The process involved either eight (O27-2) or twelve (O27-3 and O193-3) backcross generations, allowing for the gradual restoration of the recurrent parent genome (cultivar Obriy) in these addition lines. Each successive backcross increased the proportion of the recurrent parent’s genetic material while retaining the E. sibiricus 3St chromosome. This ensured that the desired alien chromosome segment carrying leaf rust resistance was retained, while minimizing unwanted genetic material from the donor species, enhancing wheat’s genetic integrity. Evaluation of EF3 and its derived lines O27-2, O27-3, and O193-3, all carrying the E. sibiricus 3St chromosome with leaf rust resistance gene(s), showed effectiveness against the local Ukrainian leaf rust population. Since no previous studies have identified leaf rust resistance genes from E. sibiricus, this study reports the first successful transfer of the 3St chromosome from E. sibiricus to wheat. E. sibiricus is known for its rich gene pool, which can potentially enhance biotic and abiotic stress tolerance in cereal crops like wheat and barley (Zheng et al., 2022; Yu et al., 2022). The GISH/FISH analyses in this study confirmed that the additional alien chromosome in lines O27-2, O27-3 and O193-3 originated from E. sibiricus.
The presence of the St genome chromosome in these wheat lines indicates that there is a complex interaction at the genomic level, which might provide an opportunity to develop disease-resistant or stress-tolerant wheat varieties derived from E. sibiricus. The variation observed in the alien chromosome among different lines also indicates a diversity of genetic potential for wheat breeding. Agronomic performance data indicate that E. sibiricus is a valuable source of genetic resources, especially leaf rust resistance genes, which can be exploited for wheat genetic improvement. These findings support the potential of E. sibiricus to contribute desirable traits to wheat breeding programs focused on disease resistance. The rRNA genes were chosen as probe sites because they are highly replicated, occupy distinct chromosomal locations and have high degrees of sequence conservation across plant groups (Liu et al., 2005; Maluszynska et al., 1998). FISH, GISH and classical cytological analysis are powerful and efficient tools for determining the extent of alien chromatin introgression. In this study, E. sibericus genomic DNA and 25S rDNA were employed to identify E. sibiricus chromatin in the wheat genome through GISH/FISH (Hasterok et al., 2002; Maluszynska, 2002; Unfried and Gruendler, 1990). Using multicolor FISH/GISH, novel wheat-E. sibiricus addition lines exhibiting leaf pubescence and resistance to leaf rust were characterized (Figure 2, Figure 3). On the alien chromosomes (green) as shown in Figure 5, there was no red fluorescence, which indicates the absence of 45S rDNA on this chromosome. Furthermore, some slides displayed “green shadows” along some wheat chromosomes. This observation led to the hypothesis that these might be instances of nonspecific hybridization or due to synteny in the cereal group, although the exact causes remain unclear.
Thus, all lines contain a pair of intact Elymus chromosomes in addition to the bread wheat genome. Notably, the identified chromosome (3St) significantly enhanced resistance to leaf rust, although no similar effect was observed for other diseases (Table 3). This aligns with previous findings by Cauderon et al. (1973) in development of an addition line with Th. intermedium chromosome homoeologues for the third group of wheat homoeologues, specifically determining leaf rust resistance and leaf hairiness. The PMCs of lines O27-2, O27-3 and O193-3 displayed 22 bivalents during meiotic metaphase I, which indicates cytological stability. However, it was observed that the introduction of the alien chromosome from E. sibiricus in a disomic addition condition led to a low frequency (10%) of non-paired chromosomes, resulting in aneuploidy. This resulted in turn in a slight increase in the frequency of rod bivalents (0.63 per PMC) and univalents (0.13 per PMC). In contrast, the recurrent cultivar Obriy typically exhibited 21 bivalents in 93.5 ± 1.7% of PMCs, highlighting the significant impact of this chromosomal addition on homologous chromosome pairing in these lines. Chromosome rearrangements, such as inversions, translocations, fusions and fissions, significantly affect hybrid fitness, chromosome-pairing behavior and pollen fertility (Heslop-Harrison and Schwarzacher, 2011). On the other hand, addition or deletion of heterochromatin does not significantly affect these factors (Rieseberg, 2001). The GISH/FISH and cytological analysis in this study made it possible to distinguish E. sibiricus chromosomes from those of wheat origin, thus providing a clear understanding of the genetic composition of these disomic addition lines. These findings have important implications for understanding the genetic and cytogenetic relationships between wheat and E. sibiricus, providing a potential avenue for future breeding and genetic research.
This study successfully identified lines that exhibit pronounced leaf pubescence (hairiness) and resistance to leaf rust at the adult stage. This finding is significant because leaf pubescence could serve as a valuable phenotypic marker for selection in the field. The presence of pseudo-black chaff, associated with the Sr2/Yr30/Lr27 resistance genes, and leaf tip necrosis, linked to the Lr34/Yr18/Sr57 genes (Rahmatov et al., 2019), are also used as phenotypic markers for field selection at PBGI-NCSCI. Leaf pubescence phenotypes provide a practical and efficient means of identifying and selecting leaf rust-resistant plants for PBGI-NCSCI breeding programs. There is a strong correlation between leaf pubescence and leaf rust resistance gene(s), which indicates good potential for using these markers to rapidly screen large populations in order to develop wheat varieties with enhanced leaf rust resistance. This approach is especially helpful if molecular markers are not readily available or cannot be used in breeding programs.
In early studies, Sears (1956) and Knott (1961) developed alien addition lines that were combined with irradiation or cytogenetic manipulation of pairing control mechanisms and induced translocations to improve wheat genetics (Cauderon et al., 1973; Khush and Brar, 1992; Rey and Prieto, 2017). Among various sources, perennial wild species carrying the St genome remain valuable for their agronomic performance (Ceoloni et al., 2015; Curwen-McAdams and Jones, 2017). Agropyron and Elymus species provide important genetic resources for improving disease resistance in wheat (Han et al., 2014; Li and Wang, 2009; Qi et al., 2015). Specifically, Th. ponticum is known to have contributed the following Lr genes to bread wheat: Lr19 (7DL-7Ae#1L), Lr24 (3DL-3Ae#1L) and Lr29 (DL-7Ae#1L·7Ae#1S) (Wang, 2011). The gene Lr24, derived from the 3St chromosome of Th. ponticum, is known to be linked to Sr24 (McIntosh et al., 2020). Lines carrying Lr24 exhibit high resistance to leaf rust and stem rust at the adult stage. Moreover, lines containing Lr24 exhibit high resistance to leaf rust and stem rust without chlorosis or necrosis on the flag leaves of adult plants (Bolton et al., 2008; McIntosh et al., 1977). We found that lines O27-2, O27-3 and O193-3 exhibited distinct differences in their reaction to leaf rust and stem rust compared with accessions known to possess Lr24/Sr24 resistance genes. Chromosomal rearrangements, such as translocations, inversions, and fusions, can alter chromosome structure and result in the loss of specific marker loci (Przewieslik-Allen et al., 2021). Similar phenomena have been observed in wheat-Th. ponticum substitution lines, where certain marker loci fail to amplify due to structural changes in the alien chromosome or instability during integration (Wang et al., 2020).
This study assigned the molecular marker Sr24/Lr24# to homologous group 3, specific to the additional chromosomes of the O27-2, O27-3 and O193-3 lines. Therefore, the leaf rust resistance gene in lines O27-2, O27-3 and O193-3 is a novel resistance gene from E. sibiricus. Chromosome translocation lines are being developed to transfer the leaf rust resistance gene into the bread wheat genome. Further research is required to determine whether the leaf rust resistance gene on the 3St chromosome is a single dominant gene. The unique molecular marker Sr24/Lr24# found on the additional chromosomes of O27-2, O27-3 and O193-3 can also detect and select alien chromosomes or their segments carrying resistance genes to leaf rust. This study broadens the range of valuable genetic resources for discovering novel genes and analyzing genetic diversity in E. sibiricus, thus providing breeders with additional tools to develop leaf rust-resistant wheat varieties. Current efforts by our research group are concentrating on developing interstitial recombinants with shortened St fragments from E. sibiricus, a strategy to enhance wheat genetic foundation by incorporating desirable traits from this wild species. Through this approach, the genetic diversity available for wheat improvement will be expanded and specific resistance genes will be transferred, potentially leading to the development of more robust and resilient wheat varieties.
Conclusions
By combining the bread wheat cultivar Obriy and a partial wheat-E. sibiricus octoploid amphiploid, we successfully developed three wheat-E. sibiricus disomic addition lines, O27-2, O27-3 and O193-3. These lines exhibit distinct leaf hairiness linked to the 3St chromosome and are resistant to leaf rust, representing a significant achievement in unlocking the potential of this valuable gene(s) for wheat improvement. Molecular marker assays and cytogenetic analyses identified wheat-E. sibiricus disomic addition lines carrying the 3St chromosome, which exhibit leaf hairiness and resistance to leaf rust. Our research also encompasses ongoing chromosome engineering endeavors, with a primary focus on reducing the E. sibiricus segment through ph1b-induced homoeologous recombination. This process involves manipulating the ZIP4 gene at the Ph1 locus, which regulates homologous chromosome pairing in wheat, thus facilitating recombination between wheat and E. sibiricus chromosomes (Rey et al., 2017). Furthermore, we have identified leaf hairiness as a valuable phenotypic field marker related to leaf rust resistance. In this study, we confirmed the importance of the E. sibiricus St genome as a valuable genetic resource, providing promising solutions to address challenges related to leaf rust in wheat production. Developed lines provide a novel germplasm resource, which offers a promising pathway for incorporating leaf rust resistance genes into wheat improvement efforts.
Data availability statement
The datasets presented in this study are available in online repositories. The names of the repositories and accession numbers are in the article/supplementary material.
Author contributions
IM: Conceptualization, Investigation, Methodology, Supervision, Data curation, Writing – original draft. OH: Writing – review & editing. TA: Writing – review & editing. GC: Writing – review & editing. SC: Supervision, Project administration, Validation, Writing – review & editing. RH: Supervision, Writing – original draft, Writing – review & editing. AB: Supervision, Writing – review & editing. RA: Writing – review & editing, Validation. MR: Investigation, Writing – original draft, Writing – review & editing, Supervision, Validation, Funding acquisition.
Funding
The author(s) declare financial support was received for the research, authorship, and/or publication of this article. We acknowledge financial support from the Swedish Institute through the "Academic Collaboration in the Baltic Sea Region" funding program for the project "Portfolio of Technology Transfer for Acceleration and Improvement of Wheat Breeding Activities in Ukraine, 01132-2022" and from FORMAS (2018-01029) for Mahbubjon Rahmatov. We also thank the Plant Breeding and Genetics Institute - National Center of Seed and Cultivar Investigation, and Research Excellence Initiative of the University of Silesia in Katowice for supporting this research. We thank Dr. R. Franke, Institute of Breeding Research, Quedlinburg, Germany, for providing a wheat-E. sibiricus introgression line.
Conflict of interest
The authors declare that the research was conducted in the absence of any commercial or financial relationships that could be construed as a potential conflict of interest.
Publisher’s note
All claims expressed in this article are solely those of the authors and do not necessarily represent those of their affiliated organizations, or those of the publisher, the editors and the reviewers. Any product that may be evaluated in this article, or claim that may be made by its manufacturer, is not guaranteed or endorsed by the publisher.
References
Appels, R., Eversole, K., Feuillet, C., Gallagher, D. (2024). “The wheat genome,” in Compendium of plant genomes (Springer, Cham). doi: 10.1007/978-3-031-38294-9_1
Babayants, O. V., Babayants, L. T. (2014). Bases of breeding and methodology of resistance evaluations of wheat to pathogens of diseases Vol. 401 (Odessa, Ukraine: VMV, Odessa, Ukraine).
Bolton, M. D., Kolmer, J. A., Garvin, D. F. (2008). Wheat leaf rust caused by Puccinia triticina. Mol. Plant Pathol. 9, 563–575. doi: 10.1111/j.1364-3703.2008.00487.x
Braverman, S. W. (1986). “Disease resistance in cool-season forage range and turf grasses II,” in The botanical review, vol. 52. (Pennsylvania State University, Pennsylvania, US), 28–36. Available at: https://www.jstor.org/stable/4354068.
Cainong, J. C., Bockus, W. W., Feng, Y., Chen, P., Qi, L., Sehgal, S. K., et al. (2015). Chromosome engineering, mapping, and transferring of resistance to Fusarium head blight disease from Elymus tsukushiensis into wheat. Theor. Appl. Genet. 128, 1019–1027. doi: 10.1007/s00122-015-2485-1
Cauderon, Y., Saigne, B., Dauge, M. (1973). The resistance to wheat rust of Agropyrum intermedium and its use in wheat improvement. In: Proc. 4th Int. Wheat Genet. Symp. Columbia U.S.A. pp, 401–407.
Ceoloni, C., Kuzmanovic, L., Forte, P., Virili, M. E., Bitti, A. (2015). “Wheat-perennial Triticeae introgressions: Major achievements and prospects,” in Alien introgression in wheat: Cytogenetics, molecular biology, and genomics. Eds. Molnar-Lang, M., Ceoloni, C., Doležel, J. (Springer, New York), 273–313. doi: 10.1007/978-3-319-23494-6
Curwen-McAdams, C., Jones, S. S. (2017). Breeding perennial grain crops based on wheat. Crop Sci. 57, 1–17. doi: 10.2135/cropsci2016.10.0869
Fomich, R. P. (1973). “Biological statistics,” in Textbook(Minsk: Visheya Shkola), 320. in Russian. Available online at: https://libarch.nmu.org.ua/handle/GenofondUA/43982.
Friebe, B., Jiang, J., Raupp, W. J., McIntosh, R. A., Gill, B. S. (1996). Characterization of wheat-alien translocations conferring resistance to diseases and pests: current status. Euphytica 91, 59–87. doi: 10.1007/BF00035277
Galaev, A. V., Sivolap, Y. M. (2015). Description of the soft wheat varieties of Ukrainian and Russian breeding by alleles of locus csLV34 closely connected with multipathogen resistance gene Lr34/Yr18/Pm38. Cytol. Genet. (Ukraine) 49, 13–19. doi: 10.3103/S0095452715010041
Gorash, A., Galaev, A., Babayants, O., Babayants, L. (2014). Leaf rust resistance of bread wheat (Triticum aestivum L.) lines derived from interspecific crosses. Zemdirbyste-Agriculture 101, 295–302. doi: 10.13080/z-a.2014.101.038
Han, H., Bai, L., Su, J., Zhang, J., Song, L., Gao, A., et al. (2014). Genetic rearrangements of six wheat–Agropyron cristatum 6P addition lines revealed by molecular markers. PloS One 9, e91066. doi: 10.1371/journal.pone.0091066
Hasterok, R., Langdon, T., Taylor, S., Jenkins, G. (2002). Combinatorial labelling of DNA probes enables multicolour fluorescence in situ hybridisation in plants. Folia Histochemica Cytobiologica 40, 319–323. Available at: https://www.researchgate.net/publication/11169291.
Heslop-Harrison, J. S. P., Schwarzacher, T. (2011). Organisation of the plant genome in chromosomes. Plant J. 66, 18–33. doi: 10.1111/j.1365-313x
Hickey, L. T., Hafeez, A. N., Robinson, H., Jackson, S. A., Leal-Bertioli, S. C. M., Tester, M., et al. (2019). Breeding crops to feed 10 billion. Nat. Biotechnol. 37, 744–754. doi: 10.1038/s41587-019-0152-9
Jenkins, G., Hasterok, R. (2007). BAC ‘landing’ on chromosomes of brachypodium distachyon for comparative genome alignment. Nat. Protoc. 2, 88–98. doi: 10.1038/nprot.2006.490
Jiang, J. M., Friebe, B., Gill, B. S. (1994). Recent advances in alien gene transfer in wheat. Euphytica 73, 199–212. doi: 10.1007/BF00036700
Johansson, E., Henriksson, T., Prieto-Linde, M. L., Andersson, S., Ashraf, R., Rahmatov, M. (2020). Diverse wheat-alien introgression lines as a basis for durable resistance and quality characteristics in bread wheat. Front.Plant Sci. 11. doi: 10.3389/fpls.2020.01067
Khush, G. S., Brar, D. S. (1992). “Overcoming the barriers in hybridisation,” in Distant hybridisation of crop plants. (Monographs on theoretical and applied genetics, vol. 16 . Eds. Kalloo, G., Chowdhury, J. B. (Springer-Verlag, Berlin Heidelberg), 47–61. doi: 10.1007/978-3-642-84306-8_4
Klebesadel, L. J. (1969). Siberian Wildrye (Elymus sibiricus L.): agronomic characteristics of a potentially valuable forage and conservation grass for the North. Agron. J. 61, 855–859. doi: 10.2134/agronj1969.00021962006100060008x
Knott, D. R. (2012). The wheat rusts–breeding for resistance (Vol. 12) (Springer Science & Business Media).
Knott, O. R. (1961). The inheritance of rust resistance. IV. The transfer of stem rust resistance from Agropyron elongatum to common wheat. Can. J. Plant Sci. 41, 109–123. doi: 10.4141/cjps61-014
Kolmer, J. A., Hanzalova, A., Goyeau, H., Bayles, R., Morgounov, A. (2013). Genetic differentiation of the wheat leaf rust fungus Puccinia triticina in Europe. Plant Pathol. 62, 21–31. doi: 10.1111/j.1365-3059.2012.02626.x
Li, H., Wang, X. (2009). Thinopyrum ponticum and Th. intermedium: the promising source of resistance to fungal and viral diseases of wheat. J. Genet. Genomics 36, 557–565. doi: 10.1016/S1673-8527(08)60147-2
Liu, B., Chen, C., Li, X., Chen, R., Song, W. (2005). Physical mapping of 45S rDNA to metaphase chromosomes in 30 taxonomically diverse plant species. J. Hortic. Sci. Biotechnol. 80, 287–290. doi: 10.1080/14620316.2005.11511931
Maluszynska, J., Hasterok, R., Weiss, H. (1998). “rRNA genes – their distribution and activity in plants,” in Plant cytogenetics, proc. Symp. Ed. Maluszynska, J. (Katowice, Poland: Wydawnictwo Uniwersytetu Śląskiego), 75–95.
Maluszynska, J. (2002). “In situ hybridisation in plants – methods and application,” in Molecular techniques in crop improvement. Eds. Jain, S. M., Brar, D. S., Ahloowalia, B. S. (Springer, Dordrecht), 299–326. doi: 10.1007/978-94-017-2356-5_11
McIntosh, R. A., Dubcovsky, J., Rogers, W. J., Xia, X. C., Raupp, W. J. (2020). Catalogue of gene symbols for wheat: 2020 supplement. Manhattan: Ann. Wheat Newslett. 66, 109–149.
McIntosh, R. A., Dyck, P. L., Green, G. J. (1977). Inheritance of leaf rust and stem rust resistances in wheat cultivars Agent and Agatha. Aust. J. Agric. Res. 28, 37–45. doi: 10.1071/AR9770037
Mello-Sampayo, T. (1971). Genetic regulation of meiotic chromosome pairing by chromosome 3D of triticum aestivum. Nat. New Biol. 230, 22–23. doi: 10.1038/newbio230022a0
Molnár-Láng, M., Ceoloni, C., Doležel, J. (2015). Alien introgression in wheat: cytogenetics, molecular biology, and genomics (Cham: Springer International Publishing). Available online at: https://www.springer.com/gp/book/9783319234939 (Accessed January 30, 2019).
Motsnyi, I. I., Petrova, I. S., Chebotar, S. V. (2016). “Inheritance and effects of addition chromosome of elymus sibiricus st genome with alien characters from the amphiploid elytricum fertile,” in Factors in experimental evolution of organisms. collection of scientific papers of USGB, vol. 18 . Ed. Kunakh, V. A. (, Kyiv: Logos), 121–126. (in Ukrainian with English summary).
Motsnyi, I. I., Kulbida, M. P. (2018). Inheritance of traits controlled by odd chromosomes using data on transmission of monosomic addition St chromosome of the Elymus sibiricus genome. Cytol. Genet. (Ukraine) 52, 260–268. doi: 10.3103/S0095452718040072
Peterson, R. F., Campbell, A. B., Hannah., A. E. (1948). A diagrammatic scale for estimating rust severity on leaves and stems of cereals. Can. J. Res. 26, 496–500. doi: 10.1139/cjr48c-033
Pont, C., Leroy, T., Seidel, M., Tondelli, A., Duchemin, W., Armisen, D., et al. (2019). Tracing the ancestry of modern bread wheats. Nat. Genet. 51, 905–911. doi: 10.1038/s41588-019-0393-z
Przewieslik-Allen, A. M., Wilkinson, P. A., Burridge, A. J., Winfield, M. O., Dai, X., Beaumont, M., et al. (2021). The role of gene flow and chromosomal instability in shaping the bread wheat genome. Nat. Plants 7, 172–183. doi: 10.1038/s41477-020-00845-2
Qi, L., Pumphrey, M., Friebe, B., Chen, P., Gill, B. (2008). Molecular cytogenetic characterization of alien introgressions with gene Fhb3 for resistance to Fusarium head blight disease of wheat. Theor. Appl. Genet. 117, 1155–1166. doi: 10.1007/s00122-008-0853-9
Qi, X. L., Li, X. F., He, F., Hu, L. Q., Bao, Y. G., Gao, J. R., et al. (2015). Cytogenetic and molecular identification of a new wheat-Thinopyrum intermedium addition line with resistance to powdery mildew. Cereal Res. Commun. 43, 353–363. doi: 10.1556/0806.43.2015.004
Rahmatov, M., Otambekova, M., Muminjanov, H., Rouse, M. N., Hovmøller, M. S., Nazari, K., et al. (2019). Characterization of stem, stripe and leaf rust resistance in Tajik bread wheat accessions. Euphytica 215, 1–22. doi: 10.1007/s10681-019-2377-6
Rey, M.-D., Martín, A. C., Higgins, J., Swarbreck, D., Uauy, C., Shaw, P., et al. (2017). Exploiting the ZIP4 homologue within the wheat Ph1 locus has identified two lines exhibiting homoeologous crossover in wheat-wild relative hybrids. Mol. Breed. 37, 95. doi: 10.1007/s11032-017-0700-2
Rey, M.-D., Prieto, P. (2017). Detection of alien genetic introgressions in bread wheat using dot-blot genomic hybridisation. Mol. Breed. 37, 1–11. doi: 10.1007/s11032-017-0629-5
Rieseberg, L. H. (2001). Chromosomal rearrangements and speciation. Trends Ecol. Evol. 16, 351–358. doi: 10.1016/S0169-5347(01)02187-5
Riley, R., Chapman, V. (1958). Genetic control of the cytologically diploid behaviour of hexaploid wheat. Nature 182, 713–715. doi: 10.1038/182713a0
Roelfs, A. P., Singh, R. P., Saari, E. E. (1992). Rust diseases of wheat: concepts and methods of disease management. (Mexico, DF: CIMMIYT), 81, ISBN: 968-6127-70-4.
Rybalka, A.І., Chervonis, М.V., Тоpоrаsh, І.G., Surzhenko, І.О., Bodelan, О.P., Shchеrbina, Z. V. (2006). Scientific rationale of new methods development of wheat flour bread-making quality estimation. Storage Process. Grain 79, 43–48.
Saghai-Maroof, M. A., Soliman, K. M., Jorgensen, R. A., Allard, R. W. (1984). Ribosomal DNA spacer-length polymorphisms in barley: Mendelian inheritance, chromosomal location, and population dynamics. Proc. Natl. Acad. Sci. U.S.A. 549, 8014–8018. doi: 10.1073/pnas.81.24.8014
Sansaloni, C., Franco, J., Santos, B., Percival-Alwyn, L., Sukhwinder, S., Petroli, C., et al. (2020). Diversity analysis of 80,000 wheat accessions reveals consequences and opportunities of selection footprints. Nat. Commun. 4572, 1–12. doi: 10.1038/s41467-020-18404-w
Savary, S., Willocquet, L., Pethybridge, S. J., Esker, P., McRoberts, N., Nelson, A. (2019). The global burden of pathogens and pests on major food crops. Nat. Ecol. Evol. 3, 430–439. doi: 10.1038/s41559-018-0793-y
Sears, E. R. (1956). The transfer of leaf-rust resistance from Ae. umbellulata to wheat. Brookhaven Symp. Biology: Genet. Plant Breed. 9, 1–22.
Shiferaw, B., Smale, M., Braun, H. J., Duveiller, E., Reynolds, M., Muricho, G. (2013). Crops that feed the world 10. Past successes and future challenges to the role played by wheat in global food security. Food Sec. 5, 291–317. doi: 10.1007/s12571-013-0263-y
Simonenko, V. K., Motsny, I. I., Sechnyak, A. L., Kulbida, M. P. (1998). The use of wheat-alien and Aegilops-rye amphiploids for introgression of genetic material to wheat. Euphytica 100, 313–321. doi: 10.1023/A:1018309802377
Tsvelev, N. N. (1976). Poaceae USSR. (Leningrad (St. Petersburg), Russia: Academy of Sciences), 788. (in Russian).
Unfried, I., Gruendler, P. (1990). Nucleotide sequence of the 5.85 and 25S rRNA genes and of the internal transcribed spacers from Arabidopsis thaliana. Nuc. Acids Res. 18, 4011. doi: 10.1093/nar/18.13.4011
Wang, R. R.-C. (2011). “Agropyron and psathyrostachys,” in Wild crop relatives: genomic and breeding resources, cereals. Ed. Kole, C. (Springer-Verlag, Berlin and Heidelberg), 77–108. doi: 10.1007/978-3-642-14228-4
Wang, Y., Cao, Q., Zhang, J., Wang, S., Chen, C., Wang, C., et al. (2020). Cytogenetic analysis and molecular marker development for a new wheat–Thinopyrum ponticum 1Js (1D) disomic substitution line with resistance to stripe rust and powdery mildew. Front. Plant Sci. 11, 1282. doi: 10.3389/fpls.2020.01282
Wang, S., Yin, L., Tanaka, H., Tanaka, K., Tsujimoto, H. (2010). Identification of wheat alien chromosome addition lines for breeding wheat with high phosphorus efficiency. Breed. Sci. 60, 371–379. doi: 10.1270/jsbbs.60.371
Yu, Q., Xiong, Y., Su, X., Xiong, Y., Dong, Z., Zhao, J., et al. (2022). Comparative metabolomic studies of siberian wildrye (Elymus sibiricus L.): a new look at the mechanism of plant drought resistance. Int. J. Mol. Sci. 24, 452. doi: 10.3390/ijms24010452
Zadoks, J. C., Chang, T. T., Konzak, C. F. (1974). A decimal code for the growth stages of cereals. Weed Res. 14, 415–421. doi: 10.1111/j.1365-3180.1974.tb01084.x
Zhang, Z., Zhang, J., Zhao, X., Xie, W., Wang, Y. (2016). Assessing and broadening genetic diversity of Elymus sibiricus germplasm for the improvement of seed shattering. Molecules 21, 869–883. doi: 10.3390/molecules21070869
Keywords: genomic in situ hybridization, alien introgression, leaf hairiness, molecular markers, resistance genes, Triticum aestivum, wild relatives
Citation: Motsnyi II, Halaiev OV, Alіeksіeіeva TG, Chebotar GO, Chebotar SV, Betekhtin A, Hasterok R, Armonienė R and Rahmatov M (2024) Cytogenetic and molecular identification of novel wheat-Elymus sibiricus addition lines with resistance to leaf rust and the presence of leaf pubescence trait. Front. Plant Sci. 15:1482211. doi: 10.3389/fpls.2024.1482211
Received: 17 August 2024; Accepted: 03 October 2024;
Published: 12 November 2024.
Edited by:
Jian Zeng, Sichuan Agricultural University, ChinaReviewed by:
Zujun Yang, University of Electronic Science and Technology of China, ChinaMaría-Dolores Rey, University of Cordoba, Spain
Copyright © 2024 Motsnyi, Halaiev, Alіeksіeіeva, Chebotar, Chebotar, Betekhtin, Hasterok, Armonienė and Rahmatov. This is an open-access article distributed under the terms of the Creative Commons Attribution License (CC BY). The use, distribution or reproduction in other forums is permitted, provided the original author(s) and the copyright owner(s) are credited and that the original publication in this journal is cited, in accordance with accepted academic practice. No use, distribution or reproduction is permitted which does not comply with these terms.
*Correspondence: Mahbubjon Rahmatov, Mahbubjon.Rahmatov@slu.se
†Present address: Galyna O. Chebotar, Leibniz Institute of Plant Genetics and Crop Plant Research, Seeland, Germany