- 1Department of Agriculture, Medicinal Plants and Drugs Research Institute, Shahid Beheshti University, Tehran, Iran
- 2Faculty of Agriculture, Lorestan University, Khorramabad, Lorestan, Iran
Introduction: With increasing drought stress due to climate change and water scarcity, the agricultural sector has sought innovative strategies to mitigate the detrimental effects on crop productivity. One approach that has received significant attention is the use of fertilizers and biostimulants as potential means of alleviating drought stress.
Methods: In this study, five different irrigation levels including 100% (control), 80% (slight stress), 60% (mild stress), 40% (moderate stress), and 20% (severe stress) of field capacity (FC) and seaweed extract (SWE) at three concentrations (0, 5, and 10 g/L) were applied to the pots containing one-year-old licorice (Glycyrrhiza glabra L.) plants in a factorial completely randomized design experiment with three replications for eight weeks.
Results and discussion: The glycyrrhizic acid content increased with water stress intensity without the application of SWE until severe (20% FC) water stress treatment. The application of 10 g/L SWE under 100% FC led to a significant increase in the glycyrrhizic acid value (32.5±0.889 mg/g DW) compared with non-SWE application (30.0±1.040 mg/g DW). The maximum glabridin content (0.270±0.010 mg/g DW) was obtained under irrigation of 20% field capacity with 10 g/L SWE application. In addition, the activity of the all studied enzymes such as APX (ascorbate peroxidase), CAT (catalase), POD (peroxidase), and SOD (superoxide dismutase) were boosted by increasing the water stress levels. The use of SWE further enhanced the increase of some of these metabolites and enzymes, which, in turn, helped the plant to tolerate stress conditions through the scavenging of more ROS (Reactive oxygen species), wherein for this purpose, the SWE 10 g/L was more effective than other concentration. The plants efficiently eliminated ROS driven from drought stress by both non-enzymatic and enzymatic systems.
1 Introduction
Biostimulants are dose-dependent active ingredients that are classified in a group between plant growth regulators and fertilizers. Their benefits for plants include better absorption and assimilation of macro/micro elements, improvement in vegetative and reproductive growth, and the ability to withstand abiotic stresses (Franzoni et al., 2022). Various substances form the biostimulant complex, with the synergistic effect between the ingredients of the biostimulant leading to its effectiveness. One of the main classes of biostimulant based on the raw material source is algae extracts, which drive and stimulate some processes in plants, resulting in an improvement in the quantity and quality of the product. They also trigger the production of specialized (secondary) metabolites (SMs) in medicinal and aromatic plants (MAPs), which undeniably play a role in stress management.
Stress can cause an imbalance between the production of reactive oxygen species and their scavenging by intracellular production factors, leading to cell breakdown and, in severe cases, destruction (Najafi et al., 2021). According to the Intergovernmental Panel on Climate Change (IPCC) report, the Earth’s average temperature is increasing by 0.6° to 1° annually (Pant et al., 2021). One of the consequences of climate change is that plants are exposed to water stress and drought and uncultivable areas are increasing. Drought stress, one of the major abiotic stressors, forces MAPs to respond by producing SMs or radical-scavenging enzymes (Talbi et al., 2020). However, this stress reduces the growth and yield of and destroys their normal activities. MAPs can produce SMs in response to water deficit stress as one of their defense mechanisms. Studies have shown that specific transcription factors and regulatory genes play a mediating role in the response of MAPs to water deficit stress and the regulation of bioactive compound production (Hodaei et al., 2018; Qari and Tarbiyyah, 2021). As global climate change intensifies, the frequency, duration, and severity of drought events are expected to increase, posing substantial challenges to plant survival and agricultural sustainability (Tramblay et al., 2020). Plants respond to drought stress through a complex interplay of physiological, biochemical, and molecular mechanisms to conserve water, maintain cellular function, and ensure survival (Oguz et al., 2022).
Licorice (Glycyrrhiza glabra L.) is one of the important perennial MAPs of the legume family, whose raw materials are increasingly in demand in various industries due to its outstanding chemical compounds. Although licorice is known to be a tolerant plant to drought stress and is used to restore saline soils, the response to stress may vary depending on the plant genotype and developmental stage (Khaitov et al., 2021). Since many of this plant’s natural habitats in Iran have been destroyed by overharvesting, it is necessary to cultivate it due to the increasing demand for products derived from the plant (Esmaeili et al., 2020). On the other hand, many agricultural lands are facing water shortages due to successive droughts, and as farmers prefer to grow higher-yielding and annual crops, planning the cultivation of this valuable MAP requires scientific rehabilitation and utilization of poor and degraded pasture land.
Addressing the challenges posed by drought requires a focus on adaptation and resilience-building approaches in agricultural practice and policy. This includes promoting the use of drought-tolerant plant varieties, implementing water-efficient irrigation techniques, implementing agroforestry and soil conservation practices, and using biostimulants as a means of mitigating biotic and abiotic stress (Adhikari et al., 2019; Duygu, 2020; Abdoli and Ghassemi-Golezani, 2023; Bell et al., 2022).
Seaweed extracts (SWE) are natural biostimulants derived from various species of seaweed, such as brown algae (e.g., Ascophyllum nodosum, Sargassum spp., and Ecklonia maxima) and red algae (e.g., Kappaphycus alvarezii) (Ali et al., 2021). These extracts contain a diverse array of bioactive compounds, including plant growth regulators, amino acids, vitamins, and antioxidants, which can enhance plant tolerance to drought stress through multiple mechanisms (Khan et al., 2009). SWE promote the accumulation of osmolytes and osmoprotectants like proline, soluble sugars, and free amino acids in plant tissues (Ali et al., 2022). These compounds help maintain cellular turgor and membrane integrity and protect plants from dehydration under water-limited conditions (Chaves and Oliveira, 2004). SWE stimulate the synthesis of antioxidant enzymes and phenolic compounds, enhancing the plant’s ability to scavenge ROS and mitigate oxidative stress (Begum et al., 2021). The application of Ascophyllum nodosum extract in Arabidopsis upregulated genes involved in abscisic acid (ABA) signaling and antioxidant pathways, promoting drought tolerance (Santaniello et al., 2017). SWE represent a sustainable and effective approach to enhancing plant resilience to drought stress.
In previous studies, the response of licorice to water deficit has been investigated in different conditions (Xie et al., 2018; Hosseini et al., 2018; Khaitov et al., 2021; Haghighi et al., 2022; Zhang et al., 2023). However, no study has been conducted to uncover the effect of interaction between drought stress and seaweed extract on the phytochemical and biochemical characteristics of licorice. The results of this study can be exploited to grow this valuable medicinal plant in low-water areas using algae extract to prevent the harmful effects of drought on the plant.
2 Materials and methods
2.1 Chemicals and reagents
All the chemicals used, such as the reference standards of glabridin, glycyrrhizic acid, rutin, as well as Folin–Ciocalteu reagent, DPPH, and HPLC-graded solvents, were purchased from Sigma Aldrich Company. The soluble seaweed extract powder (Ascophyllum nodosum 90%) was prepared form Armansabz-Adine Company. The physical and chemical composition of the seaweed extract (SWE) employed in this study is presented in Table 1.
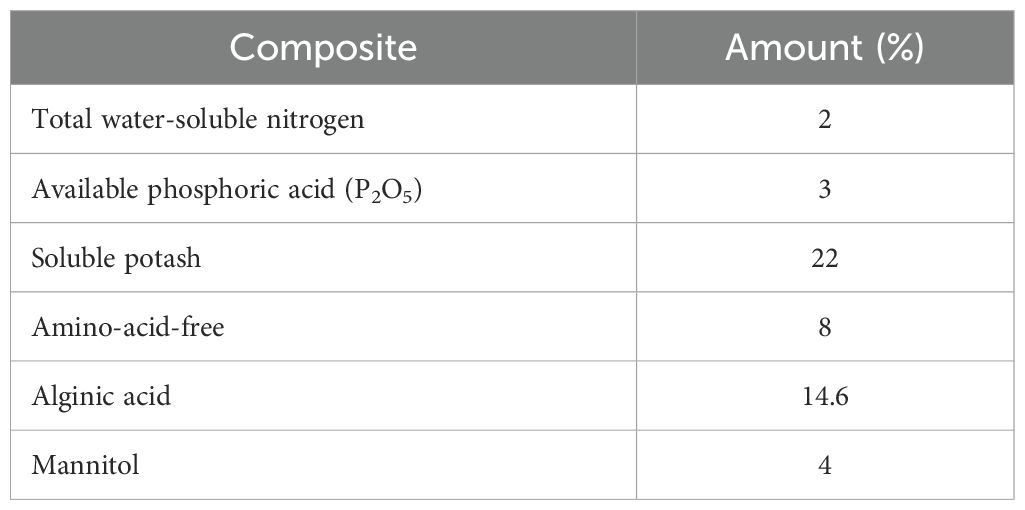
Table 1. Result of the seaweed extract (SWE) analysis derived from fresh Ascophyllum nodosum provided by the company.
2.2 Plant materials and treatments
The seeds of Khoram Abad (Lorestan province) ecotype of licorice were collected for the current study. The seeds’ dormancy were broken using sulfuric acid according to a previous method (Haghighi et al., 2022); then, these were planted into suitable pots (12 L in volume) containing leaf mulch, sand, and a soil mixture with a ratio of 1:1:2 and transported to the greenhouse with a controlled atmosphere. Afterward, five different irrigation levels including 100% (control), 80% (slight stress), 60% (mild stress), 40% (moderate stress), and 20% (severe stress) of field capacity (FC) and SWE at three concentrations (0, 5, and 10 g/L) were applied to the pots containing 1-year-old plants in a factorial completely randomized design experiment with three replications containing three plants in each for 8 weeks (during May to July 2022). The irrigation cycle was determined for each treatment by measuring the weight of the pots and evaporated water and applying it every other day. The SWE was applied twice a week through foliar application. The result of the SWE analysis derived from fresh Ascophyllum nodosum provided by the company is presented in Table 1. At the end of the experiment, all the plants were harvested and underwent biochemical and phytochemical analyses.
2.3 Extraction and HPLC analysis
All plant materials including aerial parts and roots were dried at room temperature in a shaded place after 2 months of experiment. Furthermore, 500 mg of root sample was extracted with 50 mL ethanol/water (70:30) using ultrasonic (30 min) in three replications. The same amount of dried leaf was extracted by using 50 mL methanol using the same ultrasonic procedures. To assess the contents of glabridin and glycyrrhizic acid in the root samples and rutin in the aerial parts, 20 μL of all extracts was subjected to high-performance liquid chromatography, Waters 2695, in three replications. The separation process for the root and leaf compounds was performed in a C18 column (Knauer, 25 cm × 4.6 mm Eurospher 100-5) and Sunfire C18 column (Waters, 15 cm × 4.6 mm), respectively, as stationary phases. The mobile phase and gradient elution program were adjusted according to Esmaeili et al. (2019, 2020). A photodiode array detector was used to detect glycyrrhizic acid glabridin and rutin at 250, 230, and 200 nm, respectively. The standard solutions of glycyrrhizic acid, glabridin, and rutin were prepared at concentrations of 5, 10, 100, 200, and 1,000 ppm and were subjected to HPLC (Waters 2695) to obtain the calibration curves and following calculations.
2.4 Total phenolic and flavonoid content
Total phenolic content (TPC) was quantified according to Lister and Wilson (2001), wherein 25 µL of each extract was mixed with 125 µL Folin–Ciocalteu reagent 10% and 100 µL sodium carbonate solution 7.5% (V/V). After incubation in a dark place for 90 min, the absorbance of all wells was detected using a Biotek plate reader at 760 nm. A standard curve was constructed based on the different concentrations of gallic acid, and ultimately, the TPC was quantified and reported as milligrams of gallic acid equivalent per gram of dry plant material.
The total flavonoid content (TFC) was measured using an aluminum chloride (AlCl3) reagent (Zhishen et al., 1999). To quantification, 25 μL of each extract was added to a mixture containing 100 μL of distilled water and 7.5 μL of NaNO2 solution in three replications in a 96-well plate. At 6 min after the reaction initiation, 7.5 μL of AlCl3, 100 μL of NaOH, and 10 μL of distilled water were added to the each well. After 15 min, the absorption was detected by using a Biotek plate reader (EPOCH 2, USA) at the wavelength of 510 nm. Different concentrations of rutin were considered for plotting the calibration curve. The following equations y = 0.0003x + 0.7282 (R2 = 0.989) and y = 0.0003x + 0.059 (R2 = 0.991) were used to measure TPC and TFC, respectively.
2.5 DPPH, antioxidant capacity
According to Blois (1958), the free radical scavenging capacity of the methanolic extract of plant material was assessed using 2,2′-diphenypicrylhydrazyl (DPPH). After measuring the absorbance of the extract at 515 nm, the obtained values were utilized to calculate the radical scavenging capacity of the extracts using the following equation: inhibition (%) is calculated as (Ac − As)/Ac * 100, where As and Ac represent the sample and control absorbances, respectively. With the curve showing the relationship between inhibition percentage and extract concentration, the 50% inhibition concentration (IC50) of the extracts was assessed.
2.6 Antioxidant enzymatic assessment
Enzymatic activities were assessed using a spectrophotometric method. Initially, 500 mg of fresh leaves underwent homogenization with an extraction buffer consisting of potassium phosphate at pH 7. Subsequent centrifugation led to the isolation of the supernatant for the evaluation of catalase (CAT), peroxidase (POD), ascorbate peroxidase (APX), and superoxide dismutase (SOD) activities and determination of total protein content. The activity of catalase (CAT) enzyme was assessed by monitoring the reduction of hydrogen peroxide at a wavelength of 240 nm. A reaction mixture consisting of 50 µM phosphate buffer with a pH value of 7 and 15 µM hydrogen peroxide was prepared. The enzyme extract (50 µL) was added to reach a final volume of 1.5 mL and initiate the reaction. Changes in absorbance were recorded over a period of 60 s at a wavelength of 240 nm. Finally, enzyme activity was determined as absorbance changes per minute per fresh weight. POD activity assessment was conducted using guaiacol as a substrate at 470 nm. APX activity was evaluated by measuring the absorbance at 290 nm, wherein it showed the enzyme’s capability to oxidize the ascorbate per minute. SOD activity was quantified at 560 nm by determining the enzyme’s capacity to cause 50% reduction of nitroblue tetrazolium (NBT) as the reaction mixture consisted of 50 µM phosphate buffer, 12 µM methionine, 75 μM nitrobuterazolium (NBT), and 0.1 µM EDTA. To measure SOD activity, 50 μM of the enzyme extract and 10 μL of riboflavin were added to 1 mL of the mixture. The solution was then shaken and exposed to light from a fluorescent lamp for 15 min. Absorbance was recorded at a wavelength of 560 nm, and the results were expressed in milligrams of protein (Elavarthi and Martin, 2010).
2.7 Statistical analysis
All data were subjected to statistical analysis and two-way ANOVA (factor 1, drought stress; factor 2, SEW treatment) using R (version 4.3.3). Tukey’s test was used to identify the significantly different means (P < 0.05). All values are shown as mean ± standard deviation. R software was used to perform PCA and correlation analysis as well as to draw figures and graphs.
3 Results
3.1 Phytochemical traits
The result of ANOVA indicated that the effects of drought stress, SWE, and their interaction were significant on the glycyrrhizic acid and glabridin contents (Supplementary Table S1). Drought stress and SWE significantly altered the glycyrrhizic acid content compared with the control, either through their sole effects or their interaction. The glycyrrhizic acid content increased with water /stress intensity without the application of SWE until severe (20% FC) water stress treatment. The application of 10 g/L SWE under 100% FC led to a significant increase in the glycyrrhizic acid value (32.536 ± 0.889 mg/g DW) compared with non-SWE application (29.996 ± 1.040 mg/g DW). The same trend was also observed in the slight (80% FC) to moderate (40% FC) stress. The application of SWE in the severe (20% FC) water stress did not lead to a significant increase in glycyrrhizic acid content. The greatest glycyrrhizic acid content (44.440 ± 0.608 mg/g DW(was obtained in the plants treated with 40% FC and using 10 g/L SWE, while its minimum content (21.033 ± 1.000 mg/g DW) was observed in the plants exposed to 20% FC without SWE (Table 2).
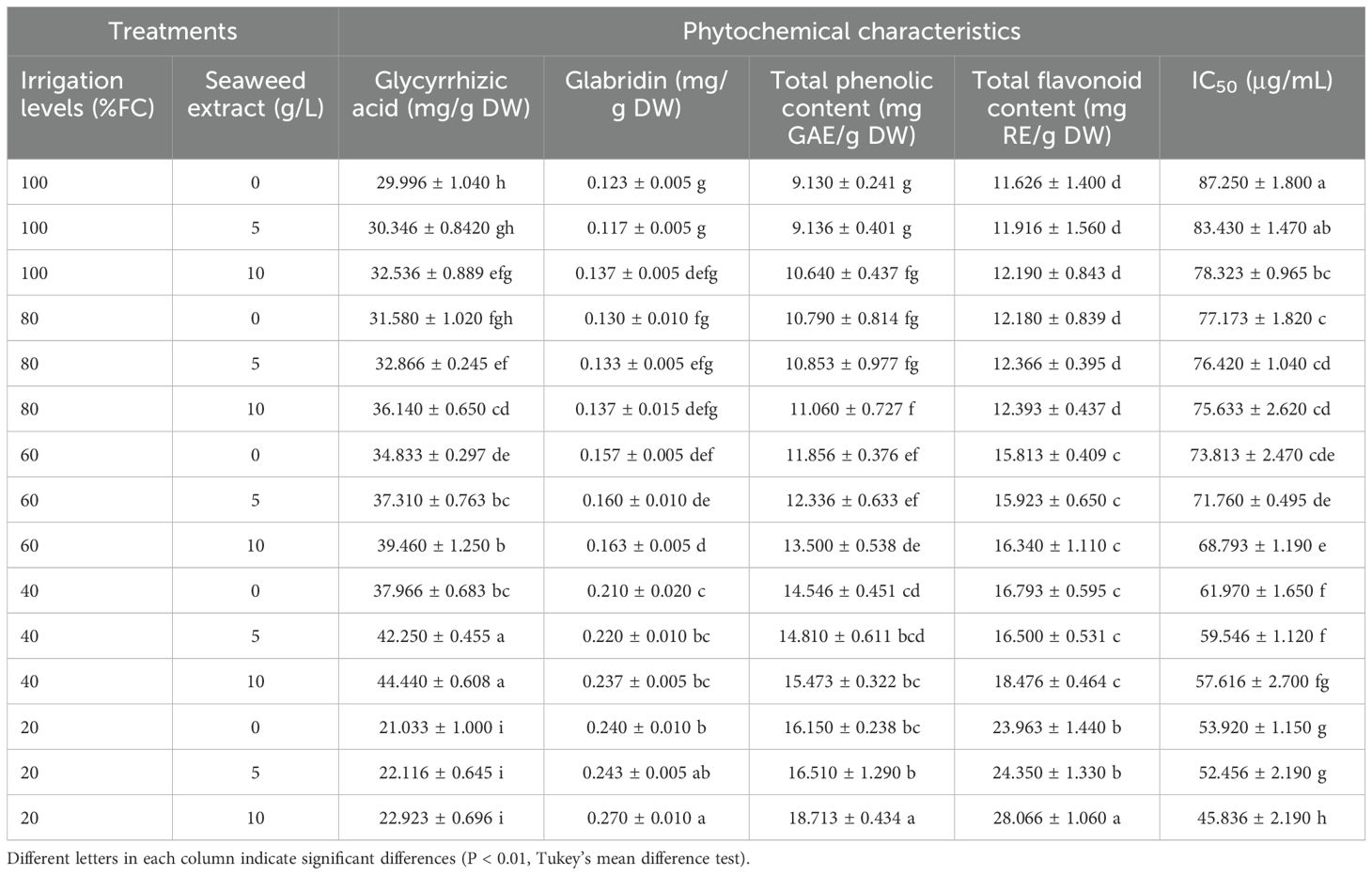
Table 2. The effect of different levels of drought stress and seaweed extract (SWE) on phytochemical and antioxidant characteristics of licorice (Glycyrrhiza glabra L.).
The glabridin content increased with the intensity of drought stress, wherein no significant difference was observed between the slight stress (80% FC) and the control (100% FC), but higher levels of stress led to a significant increase in the glabridin content. The maximum glabridin content (0.270 ± 0.010 mg/g DW) was obtained under irrigation of 20% field capacity with 10 g/L SWE application, while its minimum content (0.117 ± 0.005 mg/g DW) was recorded under 100% FC and 5 g/L SWE application. In the non-water stress (100% FC), the application of 10 g/L SWE increased the glabridin content (0.137 ± 0.005 mg/g DW), but its value was not significant compared with both 0 and 5 g/L SWE treatments (Table 2).
3.2 Total phenolic and total flavonoid contents
The result of ANOVA indicated that the effects of drought stress, SWE, and their interaction were significant on TPC and TFC (Supplementary Table S1). The root TPC significantly elevated with the intensification of drought stress compared with the control, except for the slight stress (80% FC). Irrigating the licorice plants with 20% FC and using 10 g/L SWE resulted in the highest c/ontent of root TPC (18.713 ± 0.434 mg GAE/g DW), while the lowest TPC (9.130 ± 0.241 mg GAE/g DW) was observed in the plants subjected to 100% field capacity (FC) without using SWE (Table 2).
The TFC was significantly influenced by drought stress under mild to severe water stress, wherein no significant difference was recorded for TFC between 100% and 80% FC. The application of 10 g/L SWE led to a significant increase in TFC only under severe (20% FC) drought stress, indicating the highest TFC (28.066 ± 1.060 mg RE/g DW) (Table 2).
3.3 Total antioxidant
The result of ANOVA showed that the total antioxidant activity was significantly influenced by drought, SWE, and their interaction (Supplementary Table S1). In this study, different levels of irrigation and SWE application significantly influenced the antioxidant capacity of the extracts as measured by IC50 values. However, among all treatments, the lowest IC50 value (45.836 ± 2.190 μg/mL) was obtained under irrigation of 20% field capacity (FC) and using 10 g/L SWE. Conversely, the highest IC50 value (87.250 ± 1.800 μg/mL) was observed under 100% FC without the use of SWE. A positive correlation was observed between the radical scavenging ability of extracts and the intensity of drought stress (Table 2).
3.4 Antioxidant enzyme activity
The result of ANOVA revealed that both drought stress and SWE as well as their interaction significantly influenced the enzymatic activity of extracts based on the quantification of APX (ascorbate peroxidase), CAT (catalase), POD (peroxidase), and SOD (superoxide dismutase) (Supplementary Table S1).
It was found that the activity of all the studied enzymes was boosted by increasing the water stress levels (Figure 1). The plants exposed to 20% FC combined with 10 g/L SWE application exhibited the highest APX activity (36.0 ± 0.92 U/mg protein), which showed a significant difference with application of 5 g/L and 0 g/L SWE. The plants subjected to 100% FC and without SWE application showed the lowest APX activity (14.6 ± 0.38 U/mg protein). In the non-water stress condition, the plants treated with 5 g/L SWE did not cause a significant change in the activity level of the APX enzyme, while the effect of 10 g/L SWE was significant.
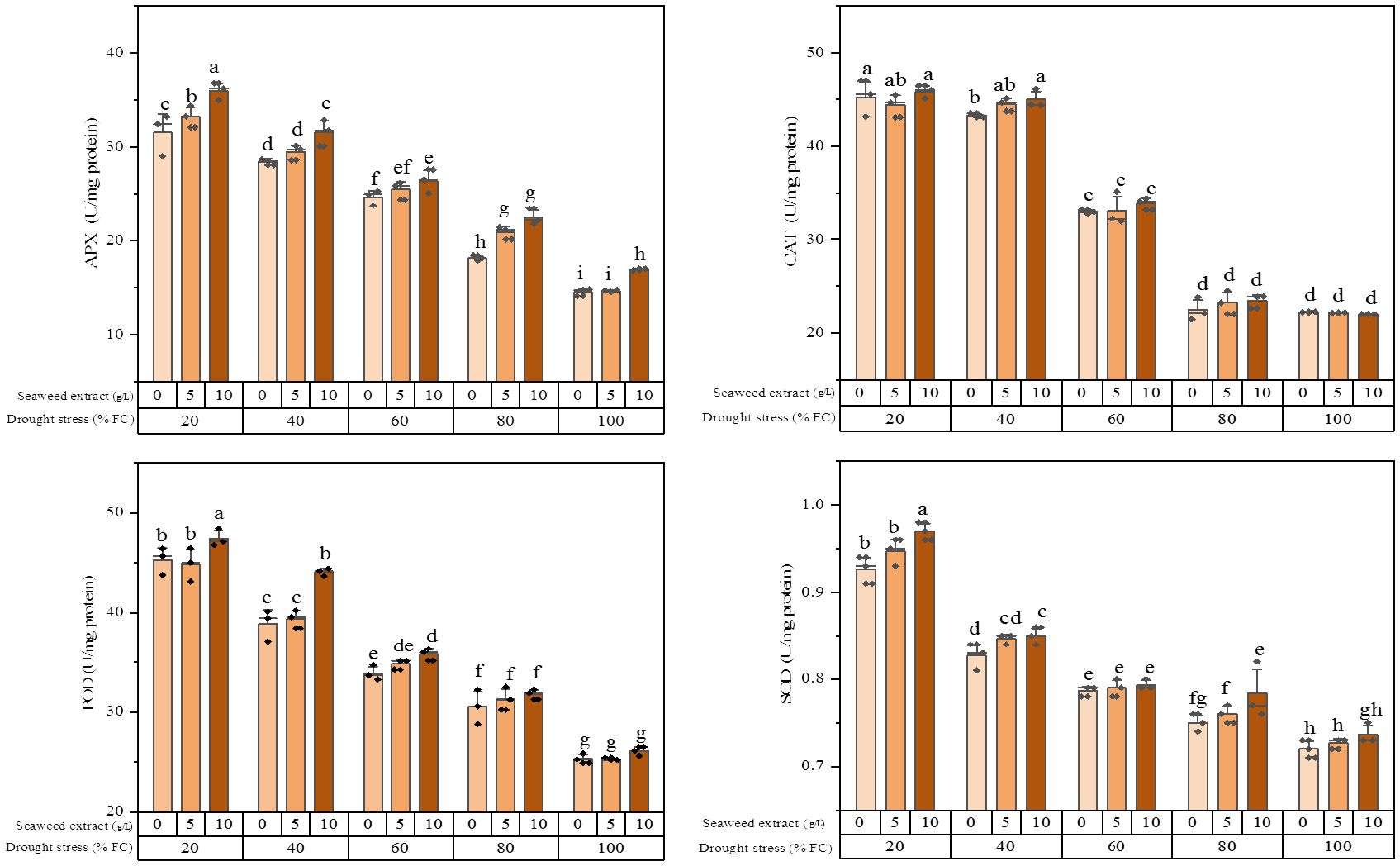
Figure 1. Antioxidant enzymes (CAT, POD, APX, and SOD) activity of the licorice (Glycyrrhiza glabra L.) plant under drought stress and seaweed extract (SWE) treatments.
The results of the CAT enzyme activity analysis showed that there was no significant difference between 100% FC and slight (80% FC) stress conditions. There was also no significant difference in the activity of this enzyme between moderate (40% FC) and severe (20% FC) stresses with 5 g/L and 10 g/L SWE application. The highest CAT activity (45.9 ± 0.69 U/mg protein) was recorded in the plants exposed to 20% FC and using 10 g/L SWE. Conversely, the minimum CAT activity (2/2.0 ± 0.02 U/mg protein) was recorded in the plants treated with 10 g/L SWE without water deficit stress.
An increment in the POD activity was observed with an increase in the level of water stress, as the highest enzyme activity was related to 20% FC. The use of SWE under 100% FC and 80% FC irrigation regimes did not change the POD activity, while utilizing 10 g/L SWE under 40% FC and 20% FC led to a higher POD enzyme activity. The minimum POD activity (25.3 ± 0.12 U/mg protein) was observed under full irrigation and 5 g/L SWE application.
The SOD activity showed an almost similar trend with the POD enzyme, wherein the plants subjected to 20% water stress and using 10 g/L SWE exhibited the highest SOD activity (0.97 ± 0.01 U/mg protein). Conversely, the minimum SOD activity (0.72 ± 0.01 U/mg protein) was observed with irrigation of 100% FC without SWE application.
3.5 Proline content
The result of ANOVA showed that drought stress, SWE, and their interaction significantly altered the proline content of the plant extracts. The proline content reached its maximum value equal to 41.5 ± 1.54 (mg/g DW) under 20% FC irrigation without SWE, while it dropped to 12.8 ± 2.20 (mg/g DW) under 100% FC irrigation and application of SWE 10 g/L. In the plants exposed to slight stress (80% FC) and full irrigation (100% FC), there was no difference in the proline level. In the mild (60% FC) and moderate (40% FC) stresses, the proline content was significantly decreased by SWE utilization (Figure 2). The growth status of the plants in some treatments is shown in Figure 3.
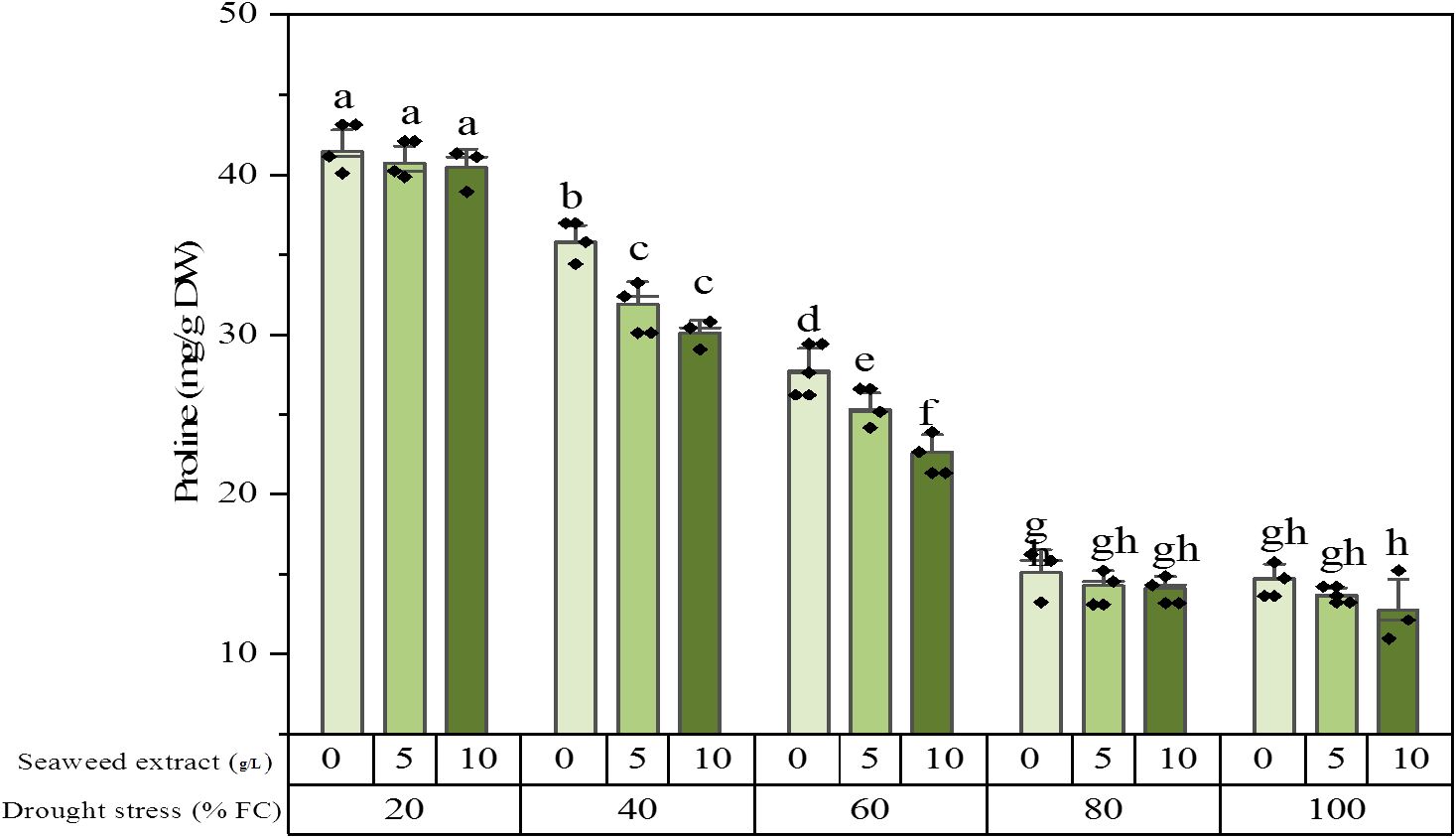
Figure 2. Proline content of the licorice (Glycyrrhiza glabra L.) plant under drought stress and seaweed extract (SWE) treatments.
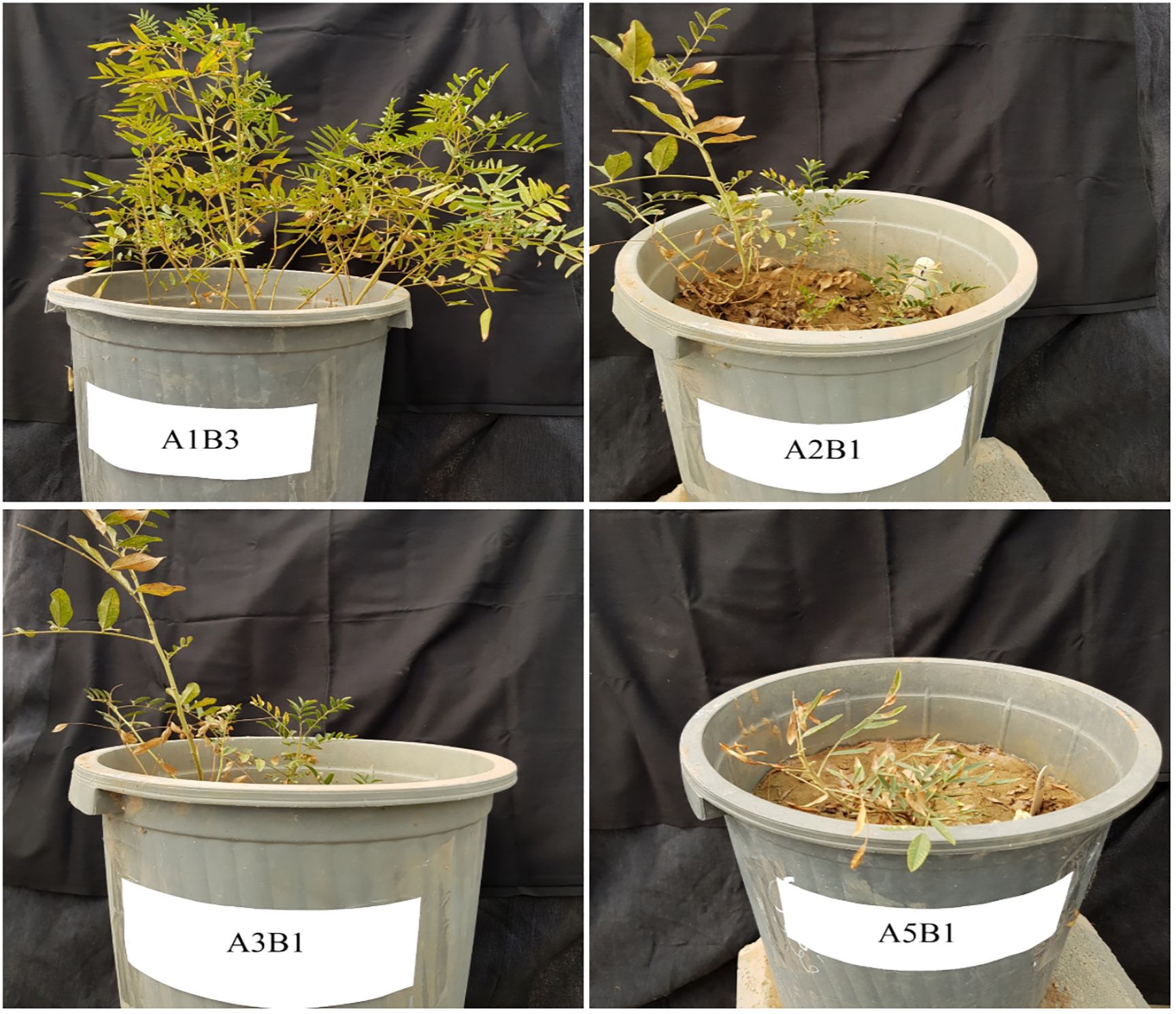
Figure 3. Growth status of licorice in some treatments (Glycyrrhiza glabra L.). A1B3: 100% FC irrigation and SWE 10 g/L. A2B1: 80% FC irrigation without SWE. A3B1: 60% FC irrigation without SWE. A5B1: 20% FC irrigation without SWE.
3.6 Correlation among traits
The pairwise matrix, density plots, and scatter plots among the studied traits are presented in Figure 4. The correlation between the traits measured in this experiment showed that glabridin content showed a strong and significant positive correlation with all the antioxidant enzymes, root TPC, and root TFC. The content of glabridin also had a significant negative relationship with IC50. In previous studies, the antioxidant role of glabridin has been mentioned as an important component of licorice root (Esmaeili et al., 2019). The negative correlation between total phenol and flavonoid content with IC50 was confirmed in this test, as expected. The glycyrrhizic acid (GA) content also had an inverse relationship with the measured antioxidant enzymes, which was not significant except in the case of SOD. The biplot diagram was drawn as a powerful tool to visualize the PCA results, which help to understand the internal structure of the data and the relationship between variables and samples (Figure 5). The biplot diagram based on PC1 and PC2 confirmed the results of the correlation analysis, wherein the total phenol and flavonoid contents and antioxidant enzymes were placed in one group and direction and the IC50 content was placed on the opposite side.
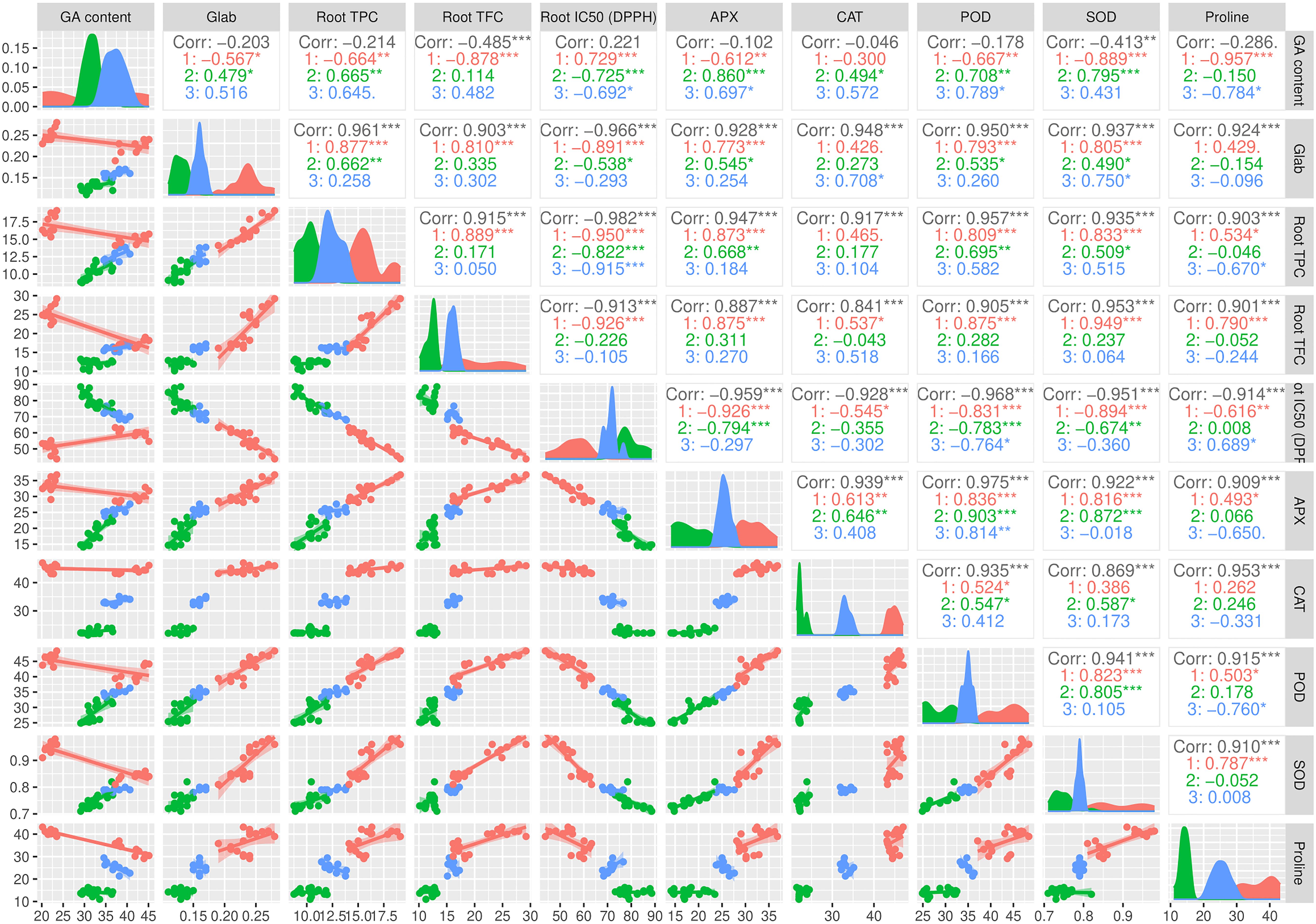
Figure 4. Pairwise matrix, density plots, and scatter plots among the studied traits of the licorice (Glycyrrhiza glabra L.) plant under drought stress and seaweed extract (SWE) treatments. *, **, ***, indicate significant levels at 0.05, 0.01, and 0.1, respectively.
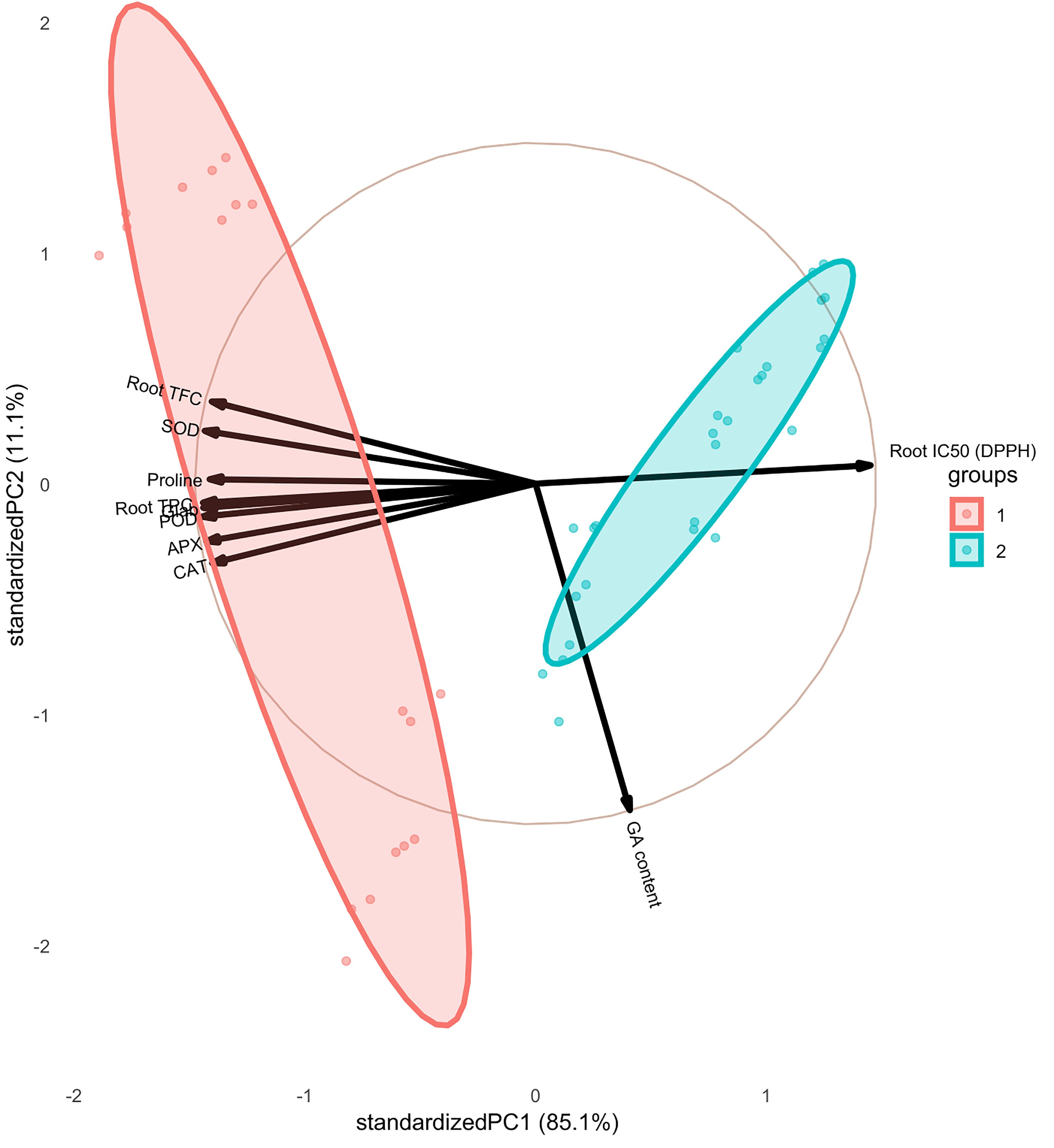
Figure 5. Biplot diagram based on PC1 and PC2 among the studied traits of the licorice (Glycyrrhiza glabra L.) plant under drought stress and seaweed extract (SWE) treatments.
4 Discussion
4.1 Interaction effect of drought and SWE on phytochemical traits
The biological and pharmaceuticals properties of G. glabra have been firstly attributed to two of its valuable compounds, triterpenoid saponins and flavonoids (Li et al., 2010). Glycyrrhizinic acid as a triterpenoid saponin and glabridin as a flavonoid are two valuable and biologically active compounds with extensive applications. Glycyrrhizin is an oleanane-type triterpenoid saponin found in the amount of 2% to 8% in the roots and stolons of Glycyrrhiza species, and the demand for its consumption in medicine, industry, cosmetics, health, and food is increasing day by day (Esmaeili et al., 2019). Glabridin, a prenylated isoflavan is another valuable phytochemical compound isolated from the plant’s underground parts which have received considerable attention in recent years. Glabridin is the most important flavonoid in licorice and is considered a phytoestrogen compound (Esmaeili et al., 2024). The SM content usually elevated during drought stress, which depends on the plant species and the intensity and duration of the stress period (Mahajan et al., 2020). In this regard, drought stress can effectively act as a controlling factor to increase the yield of compounds like alkaloids, phenols, and isoprenoids (Shil and Dewanjee, 2022). In a study, Khaitov et al. (2021) demonstrated that licorice roots produced the highest glycyrrhizic acid content in response to low or moderate water deficit, enhancing the quality of the raw materials, while this metabolite significantly decreased in the severe water stress condition. On the other hand, the findings of Nasrollahi et al. (2014) showed that extreme drought stress elevated the glycyrrhizic acid content in the G. glabra stolons through the expression of β-amyrin synthase (bAS) and sequalene synthase (SQS) genes. Sarker and Oba (2018) have demonstrated that the SM contents of Amaranthus tricolor plant were highly dependent on drought stress, wherein under control conditions the concentrations of all the flavonoids and phenolic acids were dramatically lower than those in the severe drought conditions. They suggested that the cultivation of this plant in arid and semi-arid areas can lead to the production of more bioactive compounds and thus a higher nutritional value for the human diet. In this study, the main therapeutic metabolite content of licorice, glycyrrhizic acid, significantly increased with the intensification of drought stress levels compared with the control (100% FC), except for the severe water stress (20% FC), while the glabridin content was positively correlated with an increase in stress level. The SMs such as glycyrrhizic acid and glabridin are known to play a significant role in alleviating abiotic stress, as a non-enzymatic plant defense system against stress (Mousavi et al., 2022). Abbasi and Mohammadi (2023) reported that moderate to intense drought stress can positively affect the accumulation of glycyrrhizic acid and glabridin in G. glabra. According to Yao et al. (2022), under drought stress, licorice underground parts exhibited a significant accumulation of glabridin and glycyrrhizic acid contents. A transcriptome analysis of drought-treated licorice revealed a significant enrichment of upregulated differentially expressed genes (UDEGs) involved in the synthesis of flavonoids in both the aerial and underground parts of the plant and glycyrrhizic acid in the latter. Furthermore, under drought stress, the accumulated JA content was found in both underground and aerial parts. Additionally, they reported that through the JA-mediated signaling pathway, drought stress may facilitate the accumulation of active ingredients in pharmaceuticals.
Although changes in plant metabolites in response to drought stress have been widely discussed, the interaction between drought stress and SWE has rarely been investigated. Our result indicated that under optimal water conditions (100% FC), the application of SWE 10 g/L resulted in a significant increase in glycyrrhizic acid content, while this effect was not significant in the case of glabridin. This aligns with the findings of Elansary et al. (2016) and Stirk et al. (2020) which indicate that the application of SWE on unstressed plants can stimulate the biosynthesis of saponins and isoflavones.
In this experiment, an increase in the production of glycyrrhizic acid when using SWE under slight to moderate water deficit stress is probably due to the role of this biostimulant in moderating the stress conditions for the plant. Our findings further revealed that only the application of SWE 10 g/L effectively raised the glabridin content in the severe drought stress condition. Glycyrrhizic acid and glabridin have been recognized as stress-dependent metabolites (Yao et al., 2022).
SWEs have been shown to increase plants’ resistance to both biotic and abiotic stress in numerous instances. More understanding of the mechanisms of action has been made possible by recent developments in the use of molecular tools. Evidence suggested that SWEs also contain molecules that stimulate plant metabolism and defense responses by up- and downregulating genes involved in the biosynthesis of hormones, even though some responses are attributed to different physiologically active compounds present in the complex mixture that alter physiological processes (Wally et al., 2013). Plants show different reactions to SWE depending on the method of application and dosage (Spann and Little, 2011). SWE treatment may influence the nutritional composition of vegetable crops by raising phenolics, flavonoids, and antioxidant capacity (Fan et al., 2011). According to a study on Calibrachoa x hybrida, SWE considerably raised the amount of tannin, flavonols, and total phenolic content in plant leaves, which, in turn, raised the antioxidant activity of leaf extracts (Elansary et al., 2016). Pelargonium cuttings treated with SWE showed increases in their leaves’ phenolic content (Krajnc et al., 2012), while in another study the effect of the extract on the increase of phenol and flavonoids was not observed (Xu and Leskovar, 2015). According to recent research, antioxidant enzymes and secondary metabolic pathways, particularly the biosynthesis of flavonoids (Santaniello et al., 2017), are activated during SWE treatments, which help plants adapt to stressful conditions.
4.2 Interaction effect of drought and SWE on TPC, TFC, and total antioxidant
Plant SMs, including phenolic and flavonoids compounds, which harbor antioxidant capacities, serve as primary molecules by which plants respond to abiotic stresses (Jan et al., 2021). Drought stress can alter the biosynthesis pathways of terpenoids and shikimate, leading to upregulation of isoprenoids, polyketides, and polyphenols and thus their increased accumulation (Park et al., 2023; Tahat and Al-Momany, 2021). Plants rely on SMs, such as phenols and flavonoids, to withstand drought conditions. Castellarin et al. (2007) reported a significant increase in flavonoids and phenolic compounds in grape and pea plants under drought stress. In the present study, mild to severe drought stress caused a significant increase in the accumulation of phenolics and flavonoids. The application of SWE 10 g/L on the plant under severe drought stress was associated with a remarkable increase in TPC and TFC. SWE has been extensively utilized to alleviate the damage caused by drought stress, which is primarily due to oxidative stress (Mansori et al., 2015). Ascophyllum nodosum extract significantly increased the amount of phenolics and flavonoids in two different broccoli cultivars under no stress condition (Lola-Luz et al., 2014). The nutrient profile of SWE, including macro-elements, microelements, vitamins, auxins, and phytohormones, affects plant growth and metabolism by transporting osmolytes, regulating membrane permeability, and promoting the production of stress-alleviating amino acids. These adjustments help regulate water capacity in plants (Khan et al., 2022).
The potential antioxidant activity of the licorice methanolic extracts was assessed using the DPPH method. The IC50 value represents the concentration of the extract required to scavenge 50% of the DPPH radicals. A lower IC50 value signifies a higher antioxidant potency. Esmaeili et al. (2019) reported an average IC50 value of 73.7 (μg/mL) for various populations of G. glabra collected from their natural habitats. In a similar study, Rebey et al. (2012) investigated the free radical scavenging capacity of Cuminum cyminum extract under drought conditions and demonstrated a significant increase in the DPPH scavenging activity by 17.40% and 64.05% under mild and severe water deficit stress, respectively, which is consistent with our results. They also reported a significant positive correlation (r = 0.92 at p < 0.05) between phenolic content and the capacity to scavenge the DPPH free radicals under drought conditions. This aligns with our findings, as the extract with the highest phenolics content possessed the highest antioxidant capacity. In this study, under non-drought stress condition, the lowest IC50 value was observed when utilizing SWE 10 g/L. Nikoogoftar-Sedghi et al. (2023) performed a similar study on foliar application of Ascophyllum nodosum extract in pistachio and reported that SWE was able to enhance the radical scavenging capacity by 22.53% under drought stress. This is also consistent with the study of Kumari et al. (2021), which demonstrated that, in a stress-free environment, SWE treatment increased the DPPH radical scavenging activity in Vigna radiata. Similarly, in our study, the antioxidant capacity increased with both intensifying drought stress and application of SWE. The phenolics and antioxidants in seaweed extract might alter the primary and secondary metabolic pathways in the treated plants, ultimately leading to improved tolerance toward abiotic stresses (Ammar et al., 2017; Kasim et al., 2016; Khan et al., 2009).
4.3 Interaction effect of drought and SWE on antioxidant enzyme activity
In plant cells, the ascorbate–glutathione cycle serves as a powerful hydrogen peroxide detoxifying system under abiotic stresses. In this cycle, ascorbate peroxidase (APX) isoenzymes catalyze the conversion of H2O2 into H2O, utilizing ascorbate as a specific electron donor in the chloroplast (Correa-Aragunde et al., 2013; Smirnoff, 2018; Wani et al., 2021). Within the context of cellular redox homeostasis and redox signaling, two non-enzymatic antioxidants play crucial roles: ascorbate (AsA) and glutathione (GSH). These antioxidants are regulated by genes encoding APXs, which directly or indirectly contribute to enhancing the photosynthetic rates in plants under adverse environmental conditions (de Pinto et al., 2013; Hasanuzzaman et al., 2019). There was an upregulation in the expression of the APX-coding gene in Phaseolus vulgaris under drought stress, indicating a key role of molecular regulation mechanisms induced by abiotic stresses (Nageshbabu and Jyothi, 2013). There was a significant increase in the activity of APX in soybeans under drought stress (Kausar et al., 2012). In the present study, the accumulation of APX enzyme significantly increased in the drought-stressed plants when treated with 10% SWE. Elansary et al. (2017) investigated the alleviation effect of Ascophyllum nodosum extract in a prolonged irrigation treatment in Salam Turfgrass, wherein the activity of APX(s) was significantly higher compared with the plant exposed to SWE in both stress and normal condition. The increase in APX activity following the use of seaweed extract contributed to enhancing drought stress tolerance by providing antioxidants (Frioni et al., 2018).
Drought-stressed plants maintain CAT activity in their leaves to scavenge the photorespiratory H2O2 produced as a result of drought stress, especially under severe water deficit stress (de Pinto et al., 2013). Plants, to a certain extent, are efficiently able to eliminate ROS by both non-enzymatic and enzymatic antioxidants under stress conditions. However, the oxidative stress will occur when the quantity of produced ROS surpasses the plant’s antioxidant system (Ajithkumar and Panneerselvam, 2014; Vanderauwera et al., 2012). It has been reported that CAT is an enzyme that reduces the damage caused by drought and salt stress through scavenging the ROS and preventing oxidative stress (Mittler et al., 2011; Sachdev et al., 2021). Our results showed that CAT activity and its accumulation are positively associated with the severity of stress, which is consistent with some other studies on drought stress (Faize et al., 2011; Mittler et al., 2011; Pinheiro and Chaves, 2011). Hosseini et al. (2018) reported a 1.6- to 3.7-fold increase in CAT activity in G. glabra plants under drought stress, which is consistent with our data showing about a twofold increase in CAT activity under stress conditions without SWE application. In the present study, CAT activity did not show a significant difference when drought-stressed plants were treated with SWE. Contrary to the results of this study, Hernández-Herrera et al. (2024), who conducted a study on the amelioration effect of another brown algae (Phaeophyceae) extract on tomato plants under salinity stress, reported that CAT activity nearly doubled under saline irrigation, while it decreased by approximately 6.63% with the application of seaweed extract.
The H2O2 released under abiotic stresses such as water deficiency on Glycyrrhiza uralensis is scavenged by peroxidase (POD), which reduces H2O2 using glutathione (GSH) and glutathione reductase (GR) (Zhang et al., 2017). Peroxidase, an iron heme protein, plays a key role in accelerating the reduction of H2O2 in the cell wall (Özdemir et al., 2004). POD is also an important enzyme in scavenging H2O2 produced in plants’ chloroplasts under abiotic stress (Dumanović et al., 2021). There was a significant increase in POX activity in three chickpea (Cicer arietinum) cultivars under drought stress (Mafakheri et al., 2011). Reducing the toxic levels of H2O2 during cell metabolism is entirely dependent on POX activity, representing an adaptation that effectively protects cells against oxidative damage (Ahmad et al., 2010). In the present study, drought stress significantly altered POD activity, resulting in a 1.53-fold increase in plants irrigated with 40% FC compared with the control. Application of SWE 10 g/L on stressed plants helped alleviate oxidative stress, with a significant increase observed in the POD levels under mild to severe stress. Interestingly, the higher concentrations of SWE were more effective in increasing the POD levels, which directly correlates with the maintenance of cellular homeostasis and scavenging of ROS. Similar results were reported by Nikoogoftar-Sedghi et al. (2023) in a study that investigated the effect of foliar application of Ascophyllum nodosum extract on pistachio in a stress condition.
In the present study, the plants subjected to 20% water stress and using 10 g/L SWE exhibited the highest SOD activity (0.97 ± 0.01 U/mg protein). A 1.7- to 4.9-fold increase in SOD accumulation in different wild populations of G. glabra under drought stress (Hosseini et al., 2018) has been reported. The primary scavenger of free radicals in plants is SOD, which produces H2O2. Then, CAT and POD remove the H2O2, leading to a homeostatic balance that contributes to plant survival (Golashan et al., 2011; Khatun et al., 2008). In a transgenic Arabidopsis, the enzymatic activity of superoxide dismutase and catalase was increased, resulting in reduced damage caused by H2O2 (Huang et al., 2015). There was a significant difference in SOD activity in drought-stressed plants compared with control plants (Mansori et al., 2015).
4.4 Interaction effect of drought and SWE on proline content
Drought stress is usually associated with proline accumulation in licorice (Haghighi et al., 2022). Hosseini et al. (2018) reported that intense drought stress was able to increase the proline concentration in the roots and leaves of licorice by 2.5 to 4.5 times, respectively. The increase in proline levels in cells is one of the important key factors in many plant species to reduce the oxidative effects of drought stress (Moreno-Galván et al., 2020). Healthy cell growth and effective photosynthesis are highly dependent on the presence of osmotic substances, which are responsible for adjusting the osmotic pressure in leaf cells and reducing water potential (Lin et al., 2024). However, applying SWE to the plants resulted in a decrease in the level of proline, indicating a reduction in stress levels. As an antioxidant, proline contributes to inhibiting lipid peroxidation and scavenging free radicals (Zulfiqar and Ashraf, 2023). In this study, plants accumulated a higher content of proline as the level of drought stress increased. Our results were aligned with the results of Hernández-Herrera et al. (2024), which reported that drought stress increased the proline content as a stress indicator. However, the utilization of seaweed extract was able to decrease the level of proline, alleviating the stress condition.
5 Conclusion
In this study, the phytochemical and biochemical response, respectively, of licorice plant to drought stress and seaweed extract (SWE) application were investigated. As the intensity of drought levels increased, the plant tried to overcome the stress conditions and maintain the homeostasis state of the cells by increasing the production of some metabolites like glycyrrhizic acid and glabridin and antioxidant enzymes such as APX, CAT, POD, and SOD. The use of SWE further enhanced the increase of some of these metabolites and enzymes, which, in turn, helped the plant to tolerate stress conditions through the scavenging of more ROS, wherein, for this purpose, SWE 10 g/L was more effective than the other concentrations. The results of this study can be exploited to grow this valuable medicinal plant in low-water areas using algae extract to prevent the harmful effects of drought on the plant.
Data availability statement
The original contributions presented in the study are included in the article/Supplementary Material. Further inquiries can be directed to the corresponding author.
Author contributions
VF: Formal analysis, Investigation, Writing – original draft. HE: Conceptualization, Investigation, Methodology, Supervision, Validation, Writing – review & editing. AA: Formal analysis, Methodology, Writing – original draft. GE: Validation, Writing – review & editing. MM: Validation, Writing – review & editing.
Funding
The author(s) declare that no financial support was received for the research, authorship, and/or publication of this article.
Conflict of interest
The authors declare that the research was conducted in the absence of any commercial or financial relationships that could be construed as a potential conflict of interest.
Publisher’s note
All claims expressed in this article are solely those of the authors and do not necessarily represent those of their affiliated organizations, or those of the publisher, the editors and the reviewers. Any product that may be evaluated in this article, or claim that may be made by its manufacturer, is not guaranteed or endorsed by the publisher.
Supplementary material
The Supplementary Material for this article can be found online at: https://www.frontiersin.org/articles/10.3389/fpls.2024.1474399/full#supplementary-material
References
Abbasi, S., Mohammadi, P. (2023). Improving drought tolerance and glycyrrhizin content of licorice plant by EPS-producing cyanobacteria. Ind. Crops Products 197, 116578. doi: 10.1016/j.indcrop.2023.116578
Abdoli, S., Ghassemi-Golezani, K. (2023). Emerging roles of nanomaterials in plant-salinity interaction. New Front. Plant-Environment Interactions: Innovative Technol. Developments, pp.93–pp119. doi: 10.1007/978-3-031-43729-8_5
Adhikari, M., Adhikari, N. R., Sharma, S., Gairhe, J., Bhandari, R. R., Paudel, S. (2019). Evaluation of drought tolerant rice cultivars using drought tolerant indices under water stress and irrigated condition. Am. J. Climate Change 8, pp.228–pp.236. doi: 10.4236/ajcc.2019.82013
Ahmad, P., Jaleel, C. A., Salem, M. A., Nabi, G., Sharma, S. (2010). Roles of enzymatic and nonenzymatic antioxidants in plants during abiotic stress. Crit. Rev. Biotechnol. 30, 161–175. doi: 10.3109/07388550903524243
Ajithkumar, I. P., Panneerselvam, R. (2014). ROS scavenging system, osmotic maintenance, pigment and growth status of Panicum sumatrense roth. under drought stress. Cell Biochem. biophysics 68, 587–595. doi: 10.1007/s12013-013-9746-x
Ali, O., Ramsubhag, A., Jayaraman, J. (2021). Biostimulant properties of seaweed extracts in plants: Implications towards sustainable crop production. Plants 10, 531. doi: 10.3390/plants10030531
Ali, A. H., Said, E. M., Abdelgawad, Z. A. (2022). The role of seaweed extract on improvement drought tolerance of wheat revealed by osmoprotectants and DNA (cpDNA) markers. Braz. J. Bot. 45, 857–867. doi: 10.1007/s40415-022-00820-5
Ammar, N., Ben Abdallah, A., Jabnoun-Khiareddine, H., Nefzi, A., Rguez, S., Daami-Remadi, M. (2017). Sargassum vulgare extracts as an alternative to chemical fungicide for the management of Fusarium dry rot in potato. J. Agric. Sci. Food Res. 8, 1000197.
Begum, R., Howlader, S., Mamun-Or-Rashid, A. N. M., Rafiquzzaman, S. M., Ashraf, G. M., Albadrani, G. M., et al. (2021). Antioxidant and signal-modulating effects of brown seaweed-derived compounds against oxidative stress-associated pathology. Oxid. Med. Cell. Longevity 2021, 9974890. doi: 10.1155/2021/9974890
Bell, J. C., Bound, S. A., Buntain, M. (2022). Biostimulants in agricultural and horticultural production. Hortic. Rev. 49, pp.35–pp.95. doi: 10.1002/9781119851981.ch2
Blois, M. S. (1958). Antioxidant determinations by the use of a stable free radical. Nature 181, 1199–1200. doi: 10.1038/1811199a0
Castellarin, S. D., Matthews, M. A., Di Gaspero, G., Gambetta, G. A. (2007). Water deficits accelerate ripening and induce changes in gene expression regulating flavonoid biosynthesis in grape berries. Planta 227, 101–112. doi: 10.1007/s00425-007-0598-8
Chaves, M. M., Oliveira, M. M. (2004). Mechanisms underlying plant resilience to water deficits: prospects for water-saving agriculture. J. Exp. Bot. 55, 2365–2384. doi: 10.1093/jxb/erh269
Correa-Aragunde, N., Foresi, N., Delledonne, M., Lamattina, L. (2013). Auxin induces redox regulation of ascorbate peroxidase 1 activity by S-nitrosylation/denitrosylation balance resulting in changes of root growth pattern in Arabidopsis. J. Exp. Bot. 64, 3339–3349. doi: 10.1093/jxb/ert172
de Pinto, M. C., Locato, V., Sgobba, A., Romero-Puertas, M., d., C., Gadaleta, C., et al. (2013). S-nitrosylation of ascorbate peroxidase is part of programmed cell death signaling in tobacco Bright Yellow-2 cells. Plant Physiol. 163, 1766–1775. doi: 10.1104/pp.113.222703
Dumanović, J., Nepovimova, E., Natić, M., Kuča, K., Jaćević, V. (2021). The significance of reactive oxygen species and antioxidant defense system in plants: A concise overview. Front. Plant Sci. 11, 552969. doi: 10.3389/fpls.2020.552969
Duygu, A. E. (2020). Some Methods of Water Conservation in Agriculture which may also be Integrated to Achieve Higher Productivity and Quality Ecologically I. Impacts of Global Warming and Climate Change on Agricultural Production. Int. J. Water Manage. Diplomacy 1, pp.71–pp.92.
Elansary, H. O., Norrie, J., Ali, H. M., Salem, M. Z., Mahmoud, E. A., Yessoufou, K. (2016). Enhancement of Calibrachoa growth, secondary metabolites and bioactivity using seaweed extracts. BMC Complementary Altern. Med. 16, 1–11. doi: 10.1186/s12906-016-1332-5
Elansary, H. O., Yessoufou, K., Abdel-Hamid, A. M., El-Esawi, M. A., Ali, H. M., Elshikh, M. S. (2017). Seaweed extracts enhance salam turfgrass performance during prolonged irrigation intervals and saline shock. Front. Plant Sci. 8, 830. doi: 10.3389/fpls.2017.00830
Elavarthi, S., Martin, B. (2010). Spectrophotometric assays for antioxidant enzymes in plants. Plant Stress tolerance: Methods Protoc. 639, pp.273–pp.280. doi: 10.1007/978-1-60761-702-0_16
Esmaeili, H., Karami, A., Hadian, J., Ebrahimi, S. N., Otto, L. G. (2020). Genetic structure and variation in Iranian licorice (Glycyrrhiza glabra L.) populations based on morphological, phytochemical and simple sequence repeats markers. Ind. Crops Products 145, 112140. doi: 10.1016/j.indcrop.2020.112140
Esmaeili, H., Karami, A., Hadian, J., Saharkhiz, M. J., Ebrahimi, S. N. (2019). Variation in the phytochemical contents and antioxidant activity of Glycyrrhiza glabra populations collected in Iran. Ind. Crops Products 137, 248–259. doi: 10.1016/j.indcrop.2019.05.034
Esmaeili, H., Mirjalili, M. H., Karami, A., Nejad Ebrahimi, S. (2024). Introducing the glycyrrhizic acid and glabridin rich genotypes from the cultivated Iranian licorice (Glycyrrhiza glabra L.) populations to exploit in production systems. Sci. Rep. 14, 11034. doi: 10.1038/s41598-024-61711-1
Faize, M., Burgos, L., Faize, L., Piqueras, A., Nicolas, E., Barba-Espin, G., et al. (2011). Involvement of cytosolic ascorbate peroxidase and Cu/Zn-superoxide dismutase for improved tolerance against drought stress. J. Exp. Bot. 62, 2599–2613. doi: 10.1093/jxb/erq432
Fan, D., Hodges, D. M., Zhang, J., Kirby, C. W., Ji, X., Locke, S. J., et al. (2011). Commercial extract of the brown seaweed Ascophyllum nodosum enhances phenolic antioxidant content of spinach (Spinacia oleracea L.) which protects Caenorhabditis elegans against oxidative and thermal stress. Food Chem. 124, 195–202. doi: 10.1016/j.foodchem.2010.06.008
Franzoni, G., Cocetta, G., Prinsi, B., Ferrante, A., Espen, L. (2022). Biostimulants on crops: Their impact under abiotic stress conditions. Horticulturae 8, 189. doi: 10.3390/horticulturae8030189
Frioni, T., Sabbatini, P., Tombesi, S., Norrie, J., Poni, S., Gatti, M., et al. (2018). Effects of a biostimulant derived from the brown seaweed Ascophyllum nodosum on ripening dynamics and fruit quality of grapevines. Scientia Hortic. 232, 97–106. doi: 10.1016/j.scienta.2017.12.054
Golashan, M., Habibi, D., Beladi, S. M., Maleki, M. J. (2011). Copper and Lead Tolerance Strategies in Mustard (Sinapis arvensis), Egyptian Clover (Trifolium alexandrinum) and Hairy Vetch (J'icia villosa): Role of Some Antioxidant Enzymes. American-Eurasian J. Agric. Environ. Sci. 11, 122–128.
Haghighi, T. M., Saharkhiz, M. J., Kavoosi, G., Jowkar, A. (2022). Monitoring amino acid profile and protein quality of Licorice (Glycyrrhiza glabra L.) under drought stress, silicon nutrition and mycorrhiza inoculation. Scientia Hortic. 295, 110808. doi: 10.1016/j.scienta.2021.110808
Hasanuzzaman, M., Bhuyan, M. B., Anee, T. I., Parvin, K., Nahar, K., Mahmud, J. A., et al. (2019). Regulation of ascorbate-glutathione pathway in mitigating oxidative damage in plants under abiotic stress. Antioxidants 8, 384. doi: 10.3390/antiox8090384
Hernández-Herrera, R. M., Gómez-Leyva, J. F., Sánchez-Hernández, C. V., Ocampo-Álvarez, H., Ramírez-Romero, R., Palmeros-Suárez, P. A. (2024). Seaweed extract ameliorates salt stress in tomato plants by enhancing the antioxidant system and expression of stress-responsive genes. J. Appl. Phycology 36, 1–14. doi: 10.1007/s10811-024-03236-8
Hodaei, M., Rahimmalek, M., Arzani, A., Talebi, M. (2018). The effect of water stress on phytochemical accumulation, bioactive compounds and expression of key genes involved in flavonoid biosynthesis in Chrysanthemum morifolium L. Ind. Crops Products 120, pp.295–pp.304. doi: 10.1016/j.indcrop.2018.04.073
Hosseini, M. S., Samsampour, D., Ebrahimi, M., Abadía, J., Khanahmadi, M. (2018). Effect of drought stress on growth parameters, osmolyte contents, antioxidant enzymes and glycyrrhizin synthesis in licorice (Glycyrrhiza glabra L.) grown in the field. Phytochemistry 156, 124–134. doi: 10.1016/j.phytochem.2018.08.018
Huang, Q., Wang, Y., Li, B., Chang, J., Chen, M., Li, K., et al. (2015). TaNAC29, a NAC transcription factor from wheat, enhances salt and drought tolerance in transgenic Arabidopsis. BMC Plant Biol. 15, 1–15. doi: 10.1186/s12870-015-0644-9
Jan, R., Asaf, S., Numan, M., Lubna, Kim, K.-M. (2021). Plant secondary metabolite biosynthesis and transcriptional regulation in response to biotic and abiotic stress conditions. Agronomy 11, 968. doi: 10.3390/agronomy11050968
Kasim, W. A. E.-A., Saad-Allah, K. M., Hamouda, M. (2016). Seed priming with extracts of two seaweeds alleviates the physiological and molecular impacts of salinity stress on radish (Raphanus sativus). Int. J. Agricul. Biol. 18, 653‒660.
Kausar, R., Hossain, Z., Makino, T., Komatsu, S. (2012). Characterization of ascorbate peroxidase in soybean under flooding and drought stresses. Mol. Biol. Rep. 39, 10573–10579. doi: 10.1007/s11033-012-1945-9
Khaitov, B., Urmonova, M., Karimov, A., Sulaymonov, B., Allanov, K., Israilov, I., et al. (2021). Licorice (Glycyrrhiza glabra)—Growth and phytochemical compound secretion in degraded lands under drought stress. Sustainability 13, 2923. doi: 10.3390/su13052923
Khan, Z., Gul, H., Rauf, M., Arif, M., Hamayun, M., Ud-Din, A., et al. (2022). Sargassum wightii aqueous extract improved salt stress tolerance in Abelmoschus esculentus by mediating metabolic and ionic rebalance. Front. Mar. Sci. 9, 853272. doi: 10.3389/fmars.2022.853272
Khan, W., Rayirath, U. P., Subramanian, S., Jithesh, M. N., Rayorath, P., Hodges, D. M., et al. (2009). Seaweed extracts as biostimulants of plant growth and development. J. Plant Growth Regul. 28, 386–399. doi: 10.1007/s00344-009-9103-x
Khatun, S., Ali, M. B., Hahn, E.-J., Paek, K.-Y. (2008). Copper toxicity in Withania somnifera: growth and antioxidant enzymes responses of in vitro grown plants. Environ. Exp. Bot. 64, 279–285. doi: 10.1016/j.envexpbot.2008.02.004
Krajnc, A. U., Ivanus, A., Kristl, J., Susek, A. (2012). Seaweed extract elicits the metabolic responses in leaves and enhances growth of Pelargonium cuttings. Eur. J. Hortic. Sci. 77, pp.170–pp.181.
Kumari, S., Phogat, D., Sehrawat, K. D., Choudhary, R., Rajput, V. D., Ahlawat, J., et al. (2021). The Effect of Ascophyllum nodosum Extract on the Nutraceutical Antioxidant Potential of Vigna radiata Sprout under Salt Stress. Plants 10, 1216. Available at: https://www.mdpi.com/2223-7747/10/6/1216.
Li, Y., Luo, H. M., Sun, C., Song, J. Y., Sun, Y. Z., Wu, Q., et al. (2010). EST analysis reveals putative genes involved in glycyrrhizin biosynthesis. BMC Genomics 11, 1–11. doi: 10.1186/1471-2164-11-268
Lin, S., Zhang, W., Wang, G., Hu, Y., Zhong, X., Tang, G. (2024). Physiological regulation of photosynthetic-related indices, antioxidant defense, and proline anabolism on drought tolerance of wild soybean (Glycine soja L.). Plants 13, 880. doi: 10.3390/plants13060880
Lister, E., Wilson, P. (2001). Measurement of total phenolics and ABTS assay for antioxidant activity (personal communication) Vol. 7 (Lincoln, New Zealand: Crop Research Institute), pp.235–pp.239.
Lola-Luz, T., Hennequart, F., Gaffney, M. (2014). Effect on yield, total phenolic, total flavonoid and total isothiocyanate content of two broccoli cultivars (Brassica oleraceae var italica) following the application of a commercial brown seaweed extract (Ascophyllum nodosum). Agric. Food Sci. 23, 28–37. doi: 10.23986/afsci.8832
Mafakheri, A., Siosemardeh, A., Bahramnejad, B., Struik, P., Sohrabi, Y. (2011). Effect of drought stress and subsequent recovery on protein, carbohydrate contents, catalase and peroxidase activities in three chickpea ('Cicer arietinum') cultivars. Aust. J. Crop Sci. 5, 1255–1260.
Mahajan, M., Kuiry, R., Pal, P. K. (2020). Understanding the consequence of environmental stress for accumulation of secondary metabolites in medicinal and aromatic plants. J. Appl. Res. Medicinal Aromatic Plants 18, p.100255. doi: 10.1016/j.jarmap.2020.100255
Mansori, M., Chernane, H., Latique, S., Benaliat, A., Hsissou, D., El Kaoua, M. (2015). Seaweed extract effect on water deficit and antioxidative mechanisms in bean plants (Phaseolus vulgaris L.). J. Appl. phycology 27, pp.1689–1698. doi: 10.1007/s10811-014-0455-7
Mittler, R., Vanderauwera, S., Suzuki, N., Miller, G., Tognetti, V. B., Vandepoele, K., et al. (2011). ROS signaling: the new wave? Trends Plant Sci. 16, 300–309.
Moreno-Galván, A. E., Cortés-Patiño, S., Romero-Perdomo, F., Uribe-Vélez, D., Bashan, Y., Bonilla, R. R. (2020). Proline accumulation and glutathione reductase activity induced by drought-tolerant rhizobacteria as potential mechanisms to alleviate drought stress in Guinea grass. Appl. Soil Ecol. 147, 103367. doi: 10.1016/j.apsoil.2019.103367
Mousavi, S. S., Karami, A., Saharkhiz, M. J., Etemadi, M., Zarshenas, M. M. (2022). Evaluation of metabolites in Iranian Licorice accessions under salinity stress and Azotobacter sp. inoculation. Sci. Rep. 12, p.15837. doi: 10.1038/s41598-022-20366-6
Nageshbabu, R., Jyothi, M. (2013). Profile of small interfering RNAs from French bean Phaseolus vulgaris under abiotic stress conditions. Int. J. Pharm. Biol. Sci. 4, 176–185.
Najafi, S., Nazari Nasi, H., Tuncturk, R., Tuncturk, M., Sayyed, R. Z., Amirnia, R. (2021). Biofertilizer application enhances drought stress tolerance and alters the antioxidant enzymes in medicinal pumpkin (Cucurbita pepo convar. pepo var. Styriaca). Horticulturae 7, 588. doi: 10.3390/horticulturae7120588
Nasrollahi, V., Mirzaie-Asl, A., Piri, K., Nazeri, S., Mehrabi, R. (2014). The effect of drought stress on the expression of key genes involved in the biosynthesis of triterpenoid saponins in liquorice (Glycyrrhiza glabra). Phytochemistry 103, pp.32–pp.37. doi: 10.1016/j.phytochem.2014.03.004
Nikoogoftar-Sedghi, M., Rabiei, V., Razavi, F., Molaei, S., Khadivi, A. (2023). The effect of foliar application of Ascophyllum nodosum (L.) Le Jol. seaweed extract on biochemical traits related to abiotic stresses in pistachio (Pistacia vera L. cv. Kaleh-Ghoochi). BMC Plant Biol. 23, 635. doi: 10.1186/s12870-023-04654-5
Oguz, M. C., Aycan, M., Oguz, E., Poyraz, I., Yildiz, M. (2022). Drought stress tolerance in plants: Interplay of molecular, biochemical and physiological responses in important development stages. Physiologia 2, 180–197. doi: 10.3390/physiologia2040015
Özdemir, F., Bor, M., Demiral, T., Türkan, İ. (2004). Effects of 24-epibrassinolide on seed germination, seedling growth, lipid peroxidation, proline content and antioxidative system of rice (Oryza sativa L.) under salinity stress. Plant Growth Regul. 42, 203–211. doi: 10.1023/B:GROW.0000026509.25995.13
Pant, P., Pandey, S., Dall'Acqua, S. (2021). The influence of environmental conditions on secondary metabolites in medicinal plants: A literature review. Chem. Biodiversity 18, e2100345. doi: 10.1002/cbdv.202100345
Park, Y. J., Kwon, D. Y., Koo, S. Y., Truong, T. Q., Hong, S.-C., Choi, J., et al. (2023). Identification of drought-responsive phenolic compounds and their biosynthetic regulation under drought stress in Ligularia fischeri. Front. Plant Sci. 14, 1140509. doi: 10.3389/fpls.2023.1140509
Pinheiro, C., Chaves, M. M. (2011). Photosynthesis and drought: can we make metabolic connections from available data? J. Exp. Bot. 62, 869–882. doi: 10.1093/jxb/erq340
Qari, S. H., Tarbiyyah, I. (2021). The genetic regulation of secondary metabolic pathways in response to salinity and drought as abiotic stresses. Appl. Sci. 11, p.6668. doi: 10.3390/app11156668
Rebey, I. B., Jabri-Karoui, I., Hamrouni-Sellami, I., Bourgou, S., Limam, F., Marzouk, B. (2012). Effect of drought on the biochemical composition and antioxidant activities of cumin (Cuminum cyminum L.) seeds. Ind. Crops Products 36, 238–245. doi: 10.1016/j.indcrop.2011.09.013
Sachdev, S., Ansari, S. A., Ansari, M. I., Fujita, M., Hasanuzzaman, M. (2021). Abiotic stress and reactive oxygen species: Generation, signaling, and defense mechanisms. Antioxidants 10, 277. doi: 10.3390/antiox10020277
Santaniello, A., Scartazza, A., Gresta, F., Loreti, E., Biasone, A., Di Tommaso, D., et al. (2017). Ascophyllum nodosum seaweed extract alleviates drought stress in Arabidopsis by affecting photosynthetic performance and related gene expression. Front. Plant Sci. 8, p.1362. doi: 10.3389/fpls.2017.01362
Sarker, U., Oba, S. (2018). Drought stress enhances nutritional and bioactive compounds, phenolic acids and antioxidant capacity of Amaranthus leafy vegetable. BMC Plant Biol. 18, 1–15. doi: 10.1186/s12870-018-1484-1
Shil, S., Dewanjee, S. (2022). Impact of drought stress signals on growth and secondary metabolites (SMs) in medicinal plants. J. Phytopharmacol 11, pp.371–pp.376. doi: 10.31254/phyto
Smirnoff, N. (2018). Ascorbic acid metabolism and functions: A comparison of plants and mammals. Free Radical Biol. Med. 122, 116–129. doi: 10.1016/j.freeradbiomed.2018.03.033
Spann, T. M., Little, H. A. (2011). Applications of a commercial extract of the brown seaweed Ascophyllum nodosum increases drought tolerance in container-grown ‘Hamlin’sweet orange nursery trees. HortScience 46, pp.577–pp.582. doi: 10.21273/HORTSCI.46.4.577
Stirk, W. A., Rengasamy, K. R., Kulkarni, M. G., van Staden, J. (2020). Plant biostimulants from seaweed: An overview. Chem. Biol. Plant biostimulants, 31–55. doi: 10.1002/9781119357254.ch2
Tahat, M. M., Al-Momany, A. M. (2021). Phytochemical contents in cucumber tissues influenced by enomycorrhizal fungi and verticillium dahliae. Fresenius Environ. Bull. 30, 4262–4269.
Talbi, S., Rojas, J. A., Sahrawy, M., Rodríguez-Serrano, M., Cárdenas, K. E., Debouba, M., et al. (2020). Effect of drought on growth, photosynthesis and total antioxidant capacity of the saharan plant Oudeneya africana. Environ. Exp. Bot. 176, 104099. doi: 10.1016/j.envexpbot.2020.104099
Tramblay, Y., Koutroulis, A., Samaniego, L., Vicente-Serrano, S. M., Volaire, F., Boone, A., et al. (2020). Challenges for drought assessment in the Mediterranean region under future climate scenarios. Earth-Science Rev. 210, 103348. doi: 10.1016/j.earscirev.2020.103348
Vanderauwera, S., Vandenbroucke, K., Inzé, A., Van De Cotte, B., Mühlenbock, P., De Rycke, R., et al. (2012). AtWRKY15 perturbation abolishes the mitochondrial stress response that steers osmotic stress tolerance in Arabidopsis. Proc. Natl. Acad. Sci. 109, 20113–20118. doi: 10.1073/pnas.1217516109
Wally, O. S., Critchley, A. T., Hiltz, D., Craigie, J. S., Han, X., Zaharia, L. I., et al. (2013). Regulation of phytohormone biosynthesis and accumulation in Arabidopsis following treatment with commercial extract from the marine macroalga Ascophyllum nodosum. J. Plant Growth Regul. 32, pp.324–pp.339. doi: 10.1007/s00344-012-9301-9
Wani, S. H., Anand, S., Singh, B., Bohra, A., Joshi, R. (2021). WRKY transcription factors and plant defense responses: latest discoveries and future prospects. Plant Cell Rep. 40, 1071–1085. doi: 10.1007/s00299-021-02691-8
Xie, W., Hao, Z., Zhou, X., Jiang, X., Xu, L., Wu, S., et al. (2018). Arbuscular mycorrhiza facilitates the accumulation of glycyrrhizin and liquiritin in Glycyrrhiza uralensis under drought stress. Mycorrhiza 28, pp.285–pp.300. doi: 10.1007/s00572-018-0827-y
Xu, C., Leskovar, D. I. (2015). Effects of A. nodosum seaweed extracts on spinach growth, physiology and nutrition value under drought stress. Scientia Hortic. 183, pp.39–pp.47. doi: 10.1016/j.scienta.2014.12.004
Yao, H., Wang, F., Bi, Q., Liu, H., Liu, L., Xiao, G., et al. (2022). Combined analysis of pharmaceutical active ingredients and transcriptomes of glycyrrhiza uralensis under PEG6000-induced drought stress revealed glycyrrhizic acid and flavonoids accumulation via JA-mediated signaling. Front. Plant Sci. 13, 920172. doi: 10.3389/fpls.2022.920172
Zhang, D., Liu, Y., Yang, Z., Song, X., Ma, Y., Zhao, J., et al. (2023). Widely target metabolomics analysis of the differences in metabolites of licorice under drought stress. Ind. Crops Products 202, p.117071. doi: 10.1016/j.indcrop.2023.117071
Zhang, W., Xie, Z., Wang, L., Li, M., Lang, D., Zhang, X. (2017). Silicon alleviates salt and drought stress of Glycyrrhiza uralensis seedling by altering antioxidant metabolism and osmotic adjustment. J. Plant Res. 130, 611–624. doi: 10.1007/s10265-017-0927-3
Zhishen, J., Mengcheng, T., Jianming, W. (1999). The determination of flavonoid contents in mulberry and their scavenging effects on superoxide radicals. Food Chem. 64, pp.555–pp.559. doi: 10.1016/S0308-8146(98)00102-2
Keywords: antioxidant, phytochemical response, drought stress, licorice, seaweed extract
Citation: Fozi V, Esmaeili H, Alizadeh A, Eghlima G and Mirjalili MH (2024) The interaction effect of water deficit stress and seaweed extract on phytochemical characteristics and antioxidant activity of licorice (Glycyrrhiza glabra L.). Front. Plant Sci. 15:1474399. doi: 10.3389/fpls.2024.1474399
Received: 01 August 2024; Accepted: 17 September 2024;
Published: 07 October 2024.
Edited by:
Inês Maria Valente, LAQV Network of Chemistry and Technology, PortugalReviewed by:
Muzaffer Ipek, University of Selçuk, TürkiyeWalid Zorrig, Center of Biotechnology of Borj Cedria (CBBC), Tunisia
Copyright © 2024 Fozi, Esmaeili, Alizadeh, Eghlima and Mirjalili. This is an open-access article distributed under the terms of the Creative Commons Attribution License (CC BY). The use, distribution or reproduction in other forums is permitted, provided the original author(s) and the copyright owner(s) are credited and that the original publication in this journal is cited, in accordance with accepted academic practice. No use, distribution or reproduction is permitted which does not comply with these terms.
*Correspondence: Hassan Esmaeili, h.esmaili_6007@yahoo.com