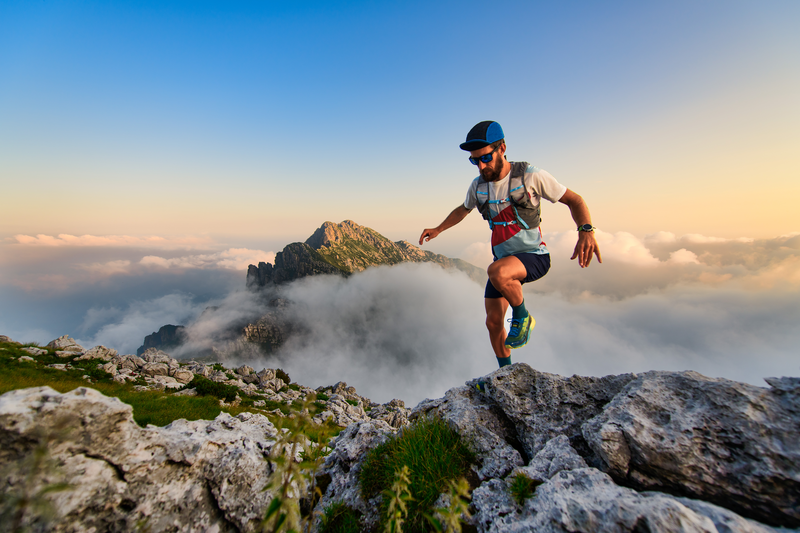
94% of researchers rate our articles as excellent or good
Learn more about the work of our research integrity team to safeguard the quality of each article we publish.
Find out more
ORIGINAL RESEARCH article
Front. Plant Sci. , 20 January 2025
Sec. Sustainable and Intelligent Phytoprotection
Volume 15 - 2024 | https://doi.org/10.3389/fpls.2024.1471706
Introduction: Piper kadsura is a well-known medicinal plant that belongs to woody liana, possessing high therapeutic and economic value. The market demand of P. kadsura is huge, but its wild resources are scarce and artificial cultivation methods have not been established, which leads to a situation with strong contradiction and imbalance between supply and demand.
Methods: In this study, 303 sample of distribution data for P. kadsura in China were collected, 33 environmental variables related to terrain, climate and soil were analyzed and the suitable habitats of P. kadsura during various periods were predicted by MaxEnt model and ArcGIS software, aiming to provide a basis for scientific cultivation and effective utilization of resources.
Results: The results indicated that precipitation and temperature were significant factors in the distribution of P. kadsura. The primary environmental variables influencing the potential distribution of P. kadsura were precipitation during the driest quarter (Bio17), annual precipitation (Bio12), mean diurnal range (Bio2), and annual temperature range (Bio7). Among them, precipitation of driest quarter (Bio17) was the most influential environmental variable for the distribution of P. kadsura with the range between 100.68 and 274.48 mm. The current distribution of P. kadsura is mainly located in the coastal areas of eastern and southern China, especially Guangxi, Guangdong, Zhejiang and Fujian, with a total area of 51.74 × 104 km2. Future climate change of global warming will lead to a reduction in the total suitable areas and high suitable areas under various climate scenarios. Especially in the SSP585 scenario, the total suitable area and the highly suitable area will be significantly reduced by 89.26% and 87.95% compared with the present during the 2090s.
Discussion: Overall, these findings can provide useful references for the suitable areas’ determination of wild resources, optimization of artificial cultivation and scientific selection of high quality medicinal materials on P. kadsura.
The global climate is continuously changing, impacting the Earth’s weather system in various ways, including seasonal patterns, extreme and unexpected weather events, temperature fluctuations, and changes in precipitation (Kunwar et al., 2023). The intensification of global warming, driven by human activities and natural disasters, is anticipated to result in a higher frequency and severity of climate change in the future (Wang Y. et al., 2024). Climate is a crucial factor influencing species distribution (Hou Z. et al., 2023) and plants are particularly sensitive to climate change, which may lead to the migration of plant habitats and alterations in their suitable areas (Duan et al., 2022; Nanda et al., 2021). Over time, the rates of shifts in species distribution, habitat loss and fragmentation, as well as species extinctions, are expected to increase (Subedi et al., 2024). Currently, many Chinese medicinal materials are primarily sourced from wild resources, which typically possess significant medicinal value, such as Pellionia scabra (Chen T. et al., 2022), Gentiana rhodantha (Zhang et al., 2022), Rheum nanum (Xu et al., 2022), and others. Current habitats that support wild populations may become unsuitable in the future due to rapidly changing climate conditions (Adhikari et al., 2023). Assessing the distributional changes of medicinal plants in relation to bioclimatic variables can provide valuable insights to specific variables that significantly influence their distribution (Subedi et al., 2023). This information is crucial for the proactive planning of protected areas, for warning against potential extinction events (Fan et al., 2022) and for offering guidance on the conservation, development, utilization, and artificial cultivation of medicinal plant resources. Currently, the application of climate data to construct species distribution models has been widely applied in the study of suitable habitats for plants (Sun et al., 2023; Yang et al., 2023). Several models are commonly used to analyze the potential suitable habitats of species, including genetic algorithm for rule set production (GARP), bioclimatic analysis and prediction system (BIOCLIM), random forests (RF), general additive model (GAM), general linear model (GLM), generalized boosting model (GBM), artificial neural network (ANN), multiple adaptive regression splines (MARS), and maximum entropy (MaxEnt) (Varela et al., 2011; Li and Wang, 2013; Melo-Merino et al., 2020). These models can comprehensively consider various environmental variables, including climate, terrain and soil, to accurately predict the potential distribution areas of species. Among them, the MaxEnt model constructs and predicts species distribution by calculating the probability distribution of maximum entropy, accurately identifying key variables affecting species distribution in complex environmental conditions (Cao et al., 2021). This model stands out due to its advantages such as requiring fewer samples, being less influenced by sample variation and providing precise predictions. It has been widely applied in various fields, including conservation biology and ecology (Kumar et al., 2022; Shen et al., 2023).
Piper kadsura is a vine-like medicinal plant found mostly in the littoral regions of southern China (Liu et al., 2015). The stem part of P. kadsura is a traditional Chinese medicine called “haifengteng”. It serves as a key ingredient in the classical prescriptions of Juanbi decoction and Gunan-Yizhi decoction. These prescriptions have been widely used for the treatment of gout, rheumatoid arthritis and vascular dementia (Wang et al., 2020; Hu et al., 2022). According to the modern chemical and pharmacological studies, P. kadsura mainly comprises the compounds of terpenes, amide alkaloids and neolignans, which having the effects of anti-neuroinflammation, anti-oxidation and anti-inflammatory (Huang et al., 2021; Chen H. et al., 2022). A recent study shows that futoquinol from P. kadsura has the activity of nerve cell protection and is a potential drug for the treatment of Alzheimer’s disease (Zhang et al., 2024). The stems of P. kadsura have medicinal properties, while the entire plant is utilized as a food source. It is recognized as an important medicinal and edible plant, characterized by its extensive applications and significant potential market demand (Kim et al., 2010). Due to climate change and the thermophilic habit of Piper genus plants, the origins of P. kadsura gradually moved south from Qinling Mountains to coastal areas. Because P. kadsura is the only species source of “haifengteng” in all the versions of Chinese Pharmacopoeia, and the rapid growth of P. kadsura consumption and the current situation of resources highly dependent on the wild sources (Lee et al., 2016; Meng et al., 2023). However, the distribution area of P. kadsura is very limited at present, forming a situation with strong contradiction and imbalance between supply and demand.
Currently, there are few reports on the potential suitable areas for P. kadsura. This study is the first to conduct research on the potential suitable areas for P. kadsura. We collected and organized the distribution data of P. kadsura, combined it with three environmental factors: climate, soil, and terrain. The MaxEnt model and ArcGIS software were used for modeling to analyze the potential suitable areas for P. kadsura in the past (LGM, MH), present (1970-2000) and future (2050s, 2090s). This study has two objectives: (1) to evaluate the current distribution of P. kadsura and the factors influencing it and (2) to investigate how the range of this species may change under future climate scenarios. The distribution of P. kadsura is predominantly concentrated in the coastal regions of southern China. We hypothesize that its distribution will decline as a result of climate change.
By consulting online databases such as the Chinese Virtual Herbarium (http://www.cvh.ac.cn/) and the NSII-China National Specimen Resource Platform (http://www.nsii.org.cn/), a total of 303 records were collected nationwide and their distribution information was also obtained (Supplementary Table S1). Then, duplicate coordinate data and samples with unclear geographic distribution location information were removed. According to the reported method (Wu et al., 2024), the coordinate information of sample points with clear geographical locations was determined using baidu coordinate picker (https://api.map.baidu.com/lbsapi/getpoint/index.html), and 89 samples of P. kadsura were finally obtained (Supplementary Table S2). When utilizing this tool, if the provided location information was not accurate beyond the district or county level, automatic identification of coordinates became unattainable. In such cases, manual positioning was required. When manually locating an area, it was crucial to confine within the boundaries of the county. Otherwise, there might be significant deviations. Additionally, to reduce model overfitting caused by sampling bias, neighborhood analysis in ArcGIS 10.4.1 was used to set a buffer zone with a radius of 10 km, and one distribution point was randomly retained within a range of 20 km, eventually resulting in 65 valid distribution points (Supplementary Table S3; Figure 1). The species name, longitude, and latitude of these points were applied for subsequent analysis (Zhang et al., 2019).
Nineteen climate variables were obtained from the World Climate Database (http://www.worldclim.org) using current (1970-2000) climate data as the baseline, selecting the Last Glacial Maximum (LGM) and the Mid-Holocene (MH) for past climate data, as well as different scenarios for future climate (2041-2060, 2081-2100). Future climate data were determined based on the Shared Socioeconomic Pathways (SSPs) models released by the Sixth Coupled Model Intercomparison Project (CMIP6), with SSP126 (low emission scenario) and SSP585 (high emission scenario) reflecting the most optimistic and pessimistic greenhouse gas emission scenarios for the future, respectively (Riahi et al., 2017). Meanwhile, eleven soil variables and three topographic variables were obtained from the Food and Agriculture Organization of the United Nations World Soil Database (http://www.fao.org/soils-portal/data-hub/en/) and the WorldClim website (https://www.worldclim.org/) (Fan et al., 2022; Ouyang et al., 2022). A total of 33 environmental variables were applied to evaluate the impact on distribution of P. kadsura, and the most dominant environmental variables were found out after eliminating strongly correlation variables that are relatively minor (Table 1).
In order to reduce the high correlation and multicollinearity among environmental variables that cause model overfitting and ensure the accuracy of the prediction, this study used SPSS 26.0 software to perform Spearman correlation analysis on the above environmental variables (Supplementary Figure S1). When the correlation coefficient of two environmental variables was greater than |0.8|, the variables with small contribution rates were eliminated, thus minimizing the bias fitting of the MaxEnt mode (Yang et al., 2022). Ultimately, 18 environmental variables were retained to construct the prediction model for P. kadsura, including 8 climatic variables (Bio17, Bio12, Bio7, Bio2, Bio3, Bio18, Bio1, Bio15), 7 soil variables (t_ph_h2o, s_clay, s_oc, t_sand, s_caco3, s_ph_h2o, t_oc), and 3 topographic variables (aspect, elev, slope).
The distribution data of P. kadsura and effective environmental variables were imported into the MaxEnt software (V3.4.3) to predict its potential suitable habitat distribution. The following modeling parameters were used: sampling method was bootstrap, output format was logistic, and 75% of the distribution points were randomly selected as the training set, with the remaining 25% of the distribution points as the test set. For each training partition, after 106 iterations and 10 times model repetition, the average value of the calculations was taken as the final result of the model prediction (Huang et al., 2023). This study selected the area under the Receiver Operating Characteristic (ROC) curve (AUC) to evaluate the accuracy of the model prediction (Xu et al., 2020). At the same time, the Jackknife method was used to analyze the impact of each environmental variable on the distribution of P. kadsura and to plot the response curves of key environmental variables. In addition, in species distribution modeling, the Maximum Test Sensitivity Plus Specificity Logistic Threshold (MTSPS) was used as the dividing line between suitable and unsuitable areas, which is considered simple and effective (Aidoo et al., 2022; Huang R. et al., 2022). With reference to the methods of Yang et al. (2023) and Wu et al. (2024), we used MTSPS to classify their potential suitable habitats into the following four levels the MTSPS was used to divide its potential suitable habitat into the following four levels: unsuitable habitat (0~MTSPS), low suitability habitat (MTSPS~0.3), medium suitability habitat (0.3~0.5), and high suitability habitat (0.5~1), and the area of different suitability habitats was calculated (Yan et al., 2020).
By simulating and predicting the distribution area of the P. kadsura through MaxEnt software, the average ROC curve of 10 calculation results was finally obtained after 10 loops. The AUC value ranged from 0 to 1, and the closer it approached 1, the more accurate the prediction result of the model was. When AUC was more than 0.9, the model prediction was excellent (Ouyang et al., 2022). As shown in Figure 2, the average training value of the ROC curve in this study was 0.969, indicating that the construction of this model had a very high accuracy and could be used to study the potential suitable habitat of the P. kadsura.
To characterize the effects of various environmental variables on the construction results of the prediction model, we used the MaxEnt model to analyze the contribution rates and permutation importance of 18 environmental variables separately. The percent contribution represented the percentage of the impact of climatic factor on the model after all variables are considered, while permutation importance indicated the degree of impact on the model after the factor has been replaced (Hou J. et al., 2023). As shown in Table 2, Bio17 (precipitation of driest quarter) had the highest contribution rate at 52.0%, followed by Bio12 (annual precipitation) at 21.9%. The contribution rates of Bio7 (temperature annual range), slope, Bio2 (mean diurnal range), Bio3 (isothermality), aspect, and elev (elevation) were 5.8%, 4.5%, 2.8%, 2.8%, 2.6%, and 2.1% respectively. The contribution rates of the remaining environmental variables were all below 2.0%. Among them, Bio2, Bio7, and elev had relatively high confidence importance values of 38.8%, 17.1%, and 10.7% respectively, indicating a strong dependence of the model on these three variables (Wang E. et al., 2024).
Table 2. Percent contribution and permutation importance of dominant environmental variables of the MaxEnt model.
To characterize the importance of various environmental variables on the distribution of P. kadsura, we used the jackknife test to examine the impact of dominant environmental factors on the suitable distribution area of P. kadsura in China (Figure 3). The results indicated that Bio12, Bio17, and Bio2 had the greatest impact on the distribution of P. kadsura, suggesting that these three environmental variables contain more effective information compared to others (Deng et al., 2024). On the whole, the dominant environmental variables influencing the distribution of P. kadsura were precipitation of Bio17, Bio12, Bio2, and Bio7. Therefore, it could be inferred that temperature and precipitation are key factors affecting the distribution of P. kadsura.
Then, based on the response curves of the key environmental variables derived above, the relationship between the distribution probability of P. kadsura and the environmental variables can be determined. When the distribution probability of P. kadsura was greater than 0.5, the corresponding environmental variable values were favorable for the growth of P. kadsura. The response curves (Figures 4A–D) showed that the value ranges (and optimal values) of the key environmental variables that limited the distribution of P. kadsura were: Bio17 100.68-274.48 mm (153.24 mm), Bio12 1194.10-3898.20 mm (2190.12 mm), Bio7 7.82-28.00°C (12.66°C), Bio2 3.65-8.06°C (6.42°C). The distribution probability raised with the increase in the values of key environmental variables before the optimal values, and decreased with the increase in the environmental factor values after the optimal values.
Figure 4. Response curves of key influencing factors. (A) Mean diurnal range, Bio2 (°C); (B) Temperature annual range, Bio7 (°C); (C) Annual precipitation, Bio12 (mm); (D) Precipitation of driest quarter, Bio17 (mm).
According to the “Flora Reipulicae Popularis Sinicae”, P. kadsura was distributed along the coastal areas of China, especially in the provinces of Fujian and Zhejiang, growing in low-altitude forests, climbing on trees or rocks (Flora of China Editorial Committee of Chinese Academy of Sciences, 1982). The distribution records in the NSII-China National Specimen Resource Platform showed that P. kadsura is mainly distributed in Guangxi (129 distribution points), Guangdong (94 distribution points), Fujian (77 distribution points), Zhejiang (69 distribution points), Guizhou (42 distribution points), Taiwan (35 distribution points), Yunnan (22 distribution points), Sichuan (16 distribution points), Jiangxi (15 distribution points), and Hainan (12 distribution points), with sporadic distribution in other provinces. As shown in Figure 5, the white areas represented unsuitable zones of P. kadsura, the green areas represented low suitability zones, the yellow areas represented medium suitability zones, and red represents high suitability zones. The main distribution range of P. kadsura was between 105° E - 121° E and 18° N - 30° N, including medium and high suitability zones, with a total suitable area of 51.74 × 104 km², accounting for 5.39% of China’s land surface area, while the high suitability zone was accounting for only 22.32% of the total suitable area (Table 3). Currently, the distribution of the total suitable area for P. kadsura was relatively concentrated, mainly located in the coastal areas of East and South China, with rare distribution in inland regions, and none as it moved further north, which was highly consistent with the natural distribution area recorded in the “Flora Reipulicae Popularis Sinicae”. Among them, the high suitability zones were mainly distributed in Taiwan, Guangxi, Guangdong, Zhejiang, and Fujian provinces. The medium suitability zones were distributed around the high suitability zones, mainly covering Jiangxi, Hainan, southern Anhui, southern Hunan, southeastern Guizhou, and southeastern Yunnan. The low suitability zone area was 38.49 × 104 km², accounting for 4.01% of China’s land surface area (Table 3). The unsuitable zones were mostly located in the northern and southwestern regions of China, with large areas in Henan, Hubei, northern Jiangsu, and northern Anhui.
This study selected 6 periods to predict the potential distribution of P. kadsura in China. Based on the prediction results of the MaxEnt model, habitat suitability distribution maps of P. kadsura under two scenarios (SSP126, SSP585) in the 2050s and 2090s were obtained. As shown in Figures 6A, B, from the Last Glacial Maximum (LGM) to the Mid-Holocene (MH), P. kadsura had no suitable habitat, with a total suitable area of 0. From MH to the present, the suitable area increased to the maximum, with the current total suitable area being 51.74 × 104 km2. The high suitable area covered 11.55 × 104 km2, accounting for 1.20% of China’s land surface area (Table 3). This indicated that the current climate was more suitable for the survival of P. kadsura, while during the LGM and MH periods, P. kadsura could not survive, which might be related to the cold climate during the LGM and the unstable climate during the MH period.
Figure 6. Distribution of suitable habitats for P. kadsura under different climate scenarios. (A) Last Glacial Maximum (LGM); (B) Mid-Holocene (MH); (C) Average for 2041-2060 (2050S), SSP126; (D) Average for 2041-2060 (2050S), SSP585; (E) Average for 2081-2100 (2090S), SSP126; (F) Average for 2081-2100 (2090S), SSP585.
From the present to the future, the distribution range of P. kadsura would be reduced to varying degrees, showing a trend of initial decrease followed by an increase under the SSP126 scenario. However, compared to the current distribution range, there would still be a certain degree of reduction (Figures 6C–F). The future suitable habitats of P. kadsura shrinking and expanding were shown in Table 3 and Figure 7. Under the SSP126 scenario, the total suitable area from 2041 to 2060 was 14.03 × 104 km2, a decrease of 72.89% compared to the current climate scenario. The high suitable area decreased by 78.09%, while the low and medium suitable areas decreased by 17.72% and 71.39%, respectively. From 2081 to 2100, the total suitable area was 49.48 × 104 km2, a decrease of 4.37% compared to the current climate scenario. The low suitable area increased by 10.32%, while the high and medium suitable areas decreased by 14.69% and 1.40%, respectively. Under the SSP585 scenario, the total suitable area from 2041 to 2060 was 20.77 × 104 km2, a decrease of 59.85% compared to the current climate scenario. The low suitable area increased by 13.08%, while the high and medium suitable areas decreased by 74.86% and 55.54%, respectively. From 2081 to 2100, the total suitable area was 5.56 × 104 km2, a decrease of 89.26% compared to the current climate scenario. The high, medium, and low suitable areas decreased by 87.95%, 89.63%, and 51.55%, respectively.
Figure 7. Percentage of suitable area change of P. kadsura under future climate compared to current climate. At the top of the columns, the numbers on the left represented the potential distribution area of P. kadsura in different periods; the numbers on the right represented the proportion of change in distribution area compared to the current climate, with "+" and“-” indicating the percentage increase and decrease in potential distribution area.
Current and future climatic scenarios were modeled for the potential distribution of high-value and low-yield medicinal plant P. kadsura in the coastal areas of China. Precipitation contributed the most to the model scores, up to 73.9%, of which the precipitation of driest quarter (Bio17) accounted for 52%, and the annual precipitation (Bio12) accounted for 21.9%. Following was the temperature range that contributed 8.6% to the prediction scores, of which temperature annual range (Bio7) accounted for 5.8%, and the mean diurnal range (Bio2) accounted for 2.8%. These high-contribution variables were similar to those for Eremochloa ophiuroides (Xu et al., 2024). Specifically, P. kadsura maintained good growth efficiency when Bio17 was between 100.68-274.48 mm, Bio12 was between 1194.10-3898.20 mm, Bio7 was between 7.82-28.00°C, and Bio2 was between 3.65-8.06°C. Plants generally required sufficient water to meet transpiration needs and maintain normal physiological functions. When some researchers cultivated P. kadsuram, the precipitation of the selected locations as 1750.00-1800.00 mm (Jiang, 2017) and 1662.00 mm (Li, 2015), which was in line with the predicted range of 1194.10-3898.20 mm for Bio12. Additionally, the geographic distribution of P. kadsura was mainly in the coastal areas of eastern and southern China, which were characterized by a subtropical monsoon climate with distinct seasons and warm, humid conditions, also aligned well with the prediction results.
Besides precipitation, temperature played a significant role in the formation and distribution of plants. With the increase of temperature, the stomatal opening on the plant surface enlarged, the plant transpiration and respiratory rate increased significantly, and eventually lead to substantial losses of water, thereby inhibiting growth (Zhu et al., 2023). The primary temperature influence factors for P. kadsura were the Bio7 and Bio2, achieving optimal growth efficiency at 7.82-28.00°C and 3.65-8.06°C, respectively. This indicated that P. kadsura was not suitable for areas with large temperature differences and was not found in northern regions with significant temperature variations. Moreover, appropriate diurnal temperature variation can promote plant growth (Sun et al., 2000). In this study, the suitable value of Bio2 for the P. kadsura growth was 3.65-8.06°C, and exceeding this range was detrimental to its growth. Furthermore, numerous studies have also shown that precipitation and temperature were crucial variables affecting species distribution. For instance, Chang et al. (2020) studied the impact of climate change on the potential distribution of Anabasis aphylla in Northwestern China. Li et al. (2022) predicted the potential suitable areas for Glycyrrhiza uralensis, and Jiang et al. (2023) explored the potential suitable areas for Panicum milliaceum under climate change, all concluded that temperature and precipitation were major factors influencing the potential distribution of plants.
Therefore, in the future protection and cultivation of P. kadsura, the influence of temperature and precipitation should be fully considered. The results of this study can provide information for the suitable habitat of P. kadsura, but further practical exploration and summary are needed for subsequent practical applications.
As global climate continues to be warm and intensified, accompanied by frequent extreme events, the suitable habitats distribution of many species will be reduced, and the habitat fragmentation will be serious (Barbarossa et al., 2021). Sudden changes in the living environment will affect the migratory ability of species. If a species has weak migratory ability and its distribution speed is slower than the rate of climate change, it will not be able to adapt to the climate change quickly, making it easy for sensitive and ecologically poorly adaptable species to decline in distribution or become extinct (Zhang et al., 2019). Therefore, understanding the distribution of species’ survival under climate change is of great importance for assessing the impact of climate change on species and formulating conservation measures. Additionally, genetic diversity of species should also be fully considered. Potential suitable habitat simulations during different periods indicate that climate change significantly affects the species. The predicted results of this study show that P. kadsura did not have any distribution during the LGM and MH periods, possibly because the LGM was the most recent extremely cold period, with approximately 24% of the global land covered by ice and frequent extreme cold events (Zhan et al., 2022), which did not meet the survival conditions of P. kadsura. During the MH period, the climate was warmer and more humid than the present, with significant climate fluctuations. P. kadsura is a perennial vine, the instability and abrupt change of climate had a great impact on its growth in the next year, making this period also unsuitable for its survival. From the MH period to the present, P. kadsura transitioned from no distribution to having the largest total suitable habitat area, indicating that the current climate conditions favor the growth of P. kadsura.
Compared to the present, the future high-temperature environment caused by carbon emissions showed an overall shrinking trend in the suitable habitat of P. kadsura. Under the low-emission SSP126 scenario, the future suitable habitat area of P. kadsura fluctuates significantly, with a sharp reduction in 2050S, shrinking by 72.89% compared to the current total suitable habitat area, and generally retreating to the southeastern coastal areas. This indicated that the environmental conditions during this period were not suitable for the growth of P. kadsura. In this scenario, the distribution of P. kadsura in the 2090S period was more optimistic than in the 2050S period but still reduced compared to the present. The total suitable habitat area was only reduced by 4.35% compared to the present, with an insignificant reduction degree, but the high suitability area was reduced by 14.69%. This indicated that the climate conditions in the high suitability area during this period did not provide a better growth environment for P. kadsura compared to the present, thus limiting its growth. Under the high-emission SSP585 scenario, the suitable habitat area of P. kadsura shrunk rapidly in the future, with a reduction of 89.25% by the 2090S period compared to the present. This indicated that under the high-emission scenario, P. kadsura cannot adapt to the changing climate environment, experiencing severe growth limitations, and might face endangerment and extinction in the future. The study by He and Ding (2023) showed that compared to the high-emission scenario, the low-emission scenario had a greater possibility of reducing future climate risks. In the face of climate change, the distribution of suitable habitats for plants will respond to varying degrees. However, in the context of continuous warming, the future distribution of P. kadsura is unfavorable, with a reduction in total suitable habitat area. This is consistent with research findings for Alternanthera philoxeroides (Yan et al., 2020), Dipteronia sinensis (Huang Y. et al., 2022) and Entodon challenger (Cong et al., 2023).
Many researches only use climate variables to predict suitable habitats for species, this study applies climate (Zhang et al., 2021; Zuo et al., 2022), soil and terrain as environmental variables, which can improve the accuracy of the suitable habitats prediction for P. kadsura. However, there are many models developed and ensembled to analyze potential suitable habitats. Kunwar et al. (2023) used ensemble model to predict the distribution of seven medicinal plant species of Nepal. Subedi et al. (2023) predicted the distribution of the endangered Maple Leaf oak (Quercus acerifolia) using an integrated model. MaxEnt model stands out for our study because it requires fewer samples and has the advantages of accurate prediction. The prediction of our model may not exactly match actual developments, because only a single MaxEnt model is applied, and the SSP model is based on assumptions of future conditions, rather than direct observation. In addition, model predictions alone are not sufficient to confirm claims about the evolutionary history or origin of P. kadsura. In the following study, we will try to use more models, increase sample quantity, apply R language method, and analyze actual distribution point samples to improve the accuracy of prediction. And consider incorporating phylogenetic evidence or fossil data to strengthen the inferences about the past distributions and evolutionary history of P. kadsura. However, it is important to note that despite some limitations, this study still provide reference value for the sustainable development and utilization of P. kadsura, as well as add literature support for applying species distribution models to assess the effects of climate change on the future distribution of species.
According to the above analysis, we need to implement protective measures for the resources with high-value and low-yield resources. First of all, for the areas that having distribution records or areas identified as high suitability, it is essential to clarify their specific geographic locations and growth patterns, carry out the continuous monitoring about the surrounding habitat and growth conditions, and strengthen the personalized protection of the environmental conditions. Furthermore, it’s essential to identify suitable locations for cultivation and conservation, and then conduct the necessary transplantation, cultivation, and breeding activities to establish a strong foundation for the responsible development and exploitation of P. kadsura.
P. kadsura is an important medicinal plant in China, but the shrinking suitable habitats lead to the serious imbalance in demand and resources. Our findings indicated P. kadsura will still face an obvious decrease in habitat suitability under different climate scenarios in the future. The suitable area of P. kadsura will gradually shrink to the southern coastal areas of China, in which precipitation and temperature range were the key environmental variables affecting the suitable habitats area. A predicted loss of more than 70% of current habitat was predicted by 2050 under the low-emission scenario, and even nearly 90% loss of suitable habitat is predicted by 2090 under the highest greenhouse gas emission scenario. P. kadsura will experience extreme vulnerability due to climate change. The large geographic shifts projected under very low to extreme climate change scenarios constitute a major threat for P. kadsura survival. And the restoration of degraded planting areas within high suitable habitats is essential for the sustainable protection of the P. kadsura. Our analysis contributes to the prediction of future distribution of P. kadsura, a precious medicinal plant with high-value and low-yield, and can be utilized as a valuable management and conservation planning basis for this important species.
The raw data supporting the conclusions of this article will be made available by the authors, without undue reservation.
SL: Formal Analysis, Investigation, Methodology, Software, Writing – original draft. YXL: Data curation, Investigation, Methodology, Software, Writing – original draft. MH: Data curation, Investigation, Methodology, Software, Writing – review & editing. YKL: Methodology, Validation, Writing – review & editing. MY: Methodology, Validation, Writing – review & editing. SW: Investigation, Methodology, Software, Validation, Writing – review & editing. WY: Investigation, Methodology, Software, Validation, Writing – review & editing. CC: Conceptualization, Funding acquisition, Project administration, Writing – review & editing. QC: Conceptualization, Funding acquisition, Investigation, Project administration, Visualization, Supervision, Writing – original draft, Writing – review & editing.
The author(s) declare that financial support was received for the research, authorship, and/or publication of this article. This work was supported by grants from National Natural Science Foundation of Hubei Province, China (2024AFB502). The authors gratefully acknowledge financial supports from Ph.D. Start-up Funding (BK202204 & BK202413), Medical Fund (2023YKY04) of Hubei University of Science and Technology and the Key Research Projects in Jiangxi Province (20223BBH8007 & 20232BBG70014).
The authors declare that the research was conducted in the absence of any commercial or financial relationships that could be construed as a potential conflict of interest.
All claims expressed in this article are solely those of the authors and do not necessarily represent those of their affiliated organizations, or those of the publisher, the editors and the reviewers. Any product that may be evaluated in this article, or claim that may be made by its manufacturer, is not guaranteed or endorsed by the publisher.
The Supplementary Material for this article can be found online at: https://www.frontiersin.org/articles/10.3389/fpls.2024.1471706/full#supplementary-material
Adhikari, B., Subedi, S. C., Bhandari, S., Baral, K., Lamichhane, S., Maraseni, T. (2023). Climate-driven decline in the habitat of the endemic spiny babbler (Turdoides nipalensis). Ecosphere 14, e4584. doi: 10.1002/ecs2.4584
Aidoo, O. F., Souza, P. G. C., Da Silva, R. S., Santana, P. A., Picanço, M. C., Kyerematen, R., et al. (2022). Climate-induced range shifts of invasive species (Diaphorina citri Kuwayama). Pest Manage. Sci. 78, 2534–2549. doi: 10.1002/ps.6886
Barbarossa, V., Bosmans, J., Wanders, N., King, H., Bierkens, M. F. P., Huijbregts, M. A. J., et al. (2021). Threats of global warming to the world’s freshwater fishes. Nat. Commun. 12, 1701. doi: 10.1038/s41467-021-21655-w
Cao, Z., Zhang, L., Zhang, X., Guo, Z. (2021). Predicting the potential distribution of Hylomecon japonica in China under current and future climate change based on maxent model. Sustainability 13, 11253. doi: 10.3390/su132011253
Chang, Y. L., Xia, Y., Peng, M. W., Chu, G. M., Wang, M. (2020). Maxent modelling for predicting impacts of climate change on the potential distribution of Anabasis aphylla in northwestern China. Appl. Ecol. Env. Res. 18, 1637–1648. doi: 10.15666/aeer/1801_16371648
Chen, H., Zhu, Y., Zhang, Y.-L., Zeng, M.-N., Cao, Y.-G., Sun, P.-T., et al. (2022). Neolignans and amide alkaloids from the stems of Piper kadsura and their neuroprotective activity. Phytochemistry 203, 113336. doi: 10.1016/j.phytochem.2022.113336
Chen, T., Acma, F. M., Amoroso, V. B., Medecilo Guiang, M. M., Huang, B. (2022). Distribution of climatic suitability of Pellionia scabra benth. (urticaceae) in China. Appl. Ecol. Env. Res. 20, 4489–4498. doi: 10.15666/aeer/2005_44894498
Cong, M., Li, Y., Yang, W. (2023). Potential distribution of bryophyte, Entodon challengeri (Entodontaceae), under climate warming in China. Australas. I. Min. Met. 15, 871. doi: 10.3390/d15070871
Deng, C., Zhong, Q., Shao, D., Ren, Y., Li, Q., Wen, J., et al. (2024). Potential suitable habitats of chili pepper in China under climate change. Plants 13, 1027. doi: 10.3390/plants13071027
Duan, X., Li, J., Wu, S. (2022). Maxent modeling to estimate the impact of climate factors on distribution of Pinus densiflora. Forests 13, 402. doi: 10.3390/f13030402
Fan, Z., Zhou, B., Ma, C., Gao, C., Han, D., Chai, Y. (2022). Impacts of climate change on species distribution patterns of Polyspora sweet in China. Ecol. Evol. 12, e9516. doi: 10.1002/ece3.9516
Flora of China Editorial Committee of Chinese Academy of Sciences (1982). The Flora of China Vol. 20 (Beijing: Science Press), 046.
He, B., Ding, K. J. (2021). Localize the impact of global greenhouse gases emissions under an uncertain future: a case study in Western Cape, South Africa. Earth 2, 111–123. doi: 10.3390/earth2010007
Hou, Z., Sun, Z., Du, G., Shao, D., Zhong, Q., Yang, S. (2023). Assessment of suitable cultivation region for Pepino (Solanum muricatum) under different climatic conditions using the MaxEnt model and adaptability in the Qinghai–Tibet plateau. Heliyon 9, e18974. doi: 10.1016/j.heliyon.2023.e18974
Hou, J., Xiang, J., Li, D., Liu, X. (2023). Prediction of potential suitable distribution areas of Quasipaa spinosa in China based on MaxEnt optimization model. Biology 12, 366. doi: 10.3390/biology12030366
Hu, Y., Zhang, L., Guan, X., Hou, H., Bi, S., Liu, C., et al. (2022). Gunao-Yizhi decoction combined with donepezil for vascular dementia: A systematic review and meta-analysis. Medicine 101, e30971. doi: 10.1097/MD.0000000000030971
Huang, R., Du, H., Wen, Y., Zhang, C., Zhang, M., Lu, H., et al. (2022). Predicting the distribution of suitable habitat of the poisonous weed Astragalus variabilis in China under current and future climate conditions. Front. Plant Sci. 13. doi: 10.3389/fpls.2022.921310
Huang, B., Hu, G., Jiang, H., Wu, K. (2023). Potential distribution and climatic suitability of Kadsura coccinea (magnoliaceae) in China. Appl. Ecol. Env. Res. 21, 2657–2669. doi: 10.15666/aeer/2103_26572669
Huang, T.-Y., Wu, C.-C., Su, W.-T. (2021). Biological and cytoprotective effect of piper kadsura ohwi against hydrogen-peroxide-induced oxidative stress in human SW1353 cells. Molecules 26, 6287. doi: 10.3390/molecules26206287
Huang, Y., Zeng, Y., Jiang, P., Chen, H., Yang, J. (2022b). Prediction of potential geographic distributionof endangered relict tree species Dipteronia sinensis in China based on MaxEnt and GIS. Pol. J. Environ. Stud. 31, 3597–3609. doi: 10.15244/pjoes/146936
Jiang, X. (2017). Investigation on growing regularity of cutting seedlings and seedling nursing technology of Piper kadsura. Fujian Agric. Sci. Technology. 04), 23–27. doi: 10.13651/j.cnki.fjnykj.2017.04.008
Jiang, P., Jiang, J., Yang, C., Gu, X., Huang, Y., Liu, L. (2023). Climate change will lead to a significant reduction in the global cultivation of Panicum milliaceum. Atmosphere 14, 1297. doi: 10.3390/atmos14081297
Kim, K. H., Choi, J. W., Ha, S. K., Kim, S. Y., Lee, K. R. (2010). [amp]]ldquo;Neolignans from Piper kadsura and their anti-neuroinflammatory activity”. Bioorganic Medicinal Chem. Lett. 20, 3186–3187. doi: 10.1016/j.bmcl.2010.04.003
Kumar, D., Pandey, A., Rawat, S., Joshi, M., Bajpai, R., Upreti, D. K., et al. (2022). Predicting the distributional range shifts of Rhizocarpon geographicum (L.) DC. @ in Indian Himalayan Region under future climate scenarios. Environ. Sci. pollut. Res. 29, 61579–61593. doi: 10.1007/s11356-021-15624-5
Kunwar, R. M., Thapa-Magar, K. B., Subedi, S. C., Kutal, D. H., Baral, B., Joshi, N. R., et al. (2023). Distribution of important medicinal plant species in Nepal under past, present, and future climatic conditions. Ecol. Indic. 146, 109879. doi: 10.1016/j.ecolind.2023.109879
Lee, J.-H., Choi, I.-S., Choi, B.-H., Yang, S., Choi, G. (2016). The complete plastid genome of Piper kadsura (Piperaceae), an East Asian woody vine. Mitochondrial DNA Part A 27, 3555–3556. doi: 10.3109/19401736.2015.1074216
Li, Z. (2015). The selection of excellent provenance of Piper kadsura. J. Fujian Agric. Forestry Univ. (Natural Sci. Edition) 44, 34–39. doi: 10.13323/j.cnki.j.fafu(nat.sci.).2015.01.007
Li, X., Wang, Y. (2013). Applying various algorithms for species distribution modelling. Integr. Zoology 8, 124–135. doi: 10.1111/1749-4877.12000
Li, Q., Wang, X., Yan, K., Liang, Z., Xia, P. (2022). Based on multiple environmental factors to explore the habitat distribution of licorice (Glycyrrhiza uralensis) in different time and space. Biochem. Syst. Ecol. 105, 104490. doi: 10.1016/j.bse.2022.104490
Liu, Y., Huang, T., Ba, W.-J. (2015). Chemical composition of essential oils from piper kadsura. Chem. Nat. Compd 51, 583–585. doi: 10.1007/s10600-015-1354-0
Melo-Merino, S. M., Reyes-Bonilla, H., Lira-Noriega, A. (2020). Ecological niche models and species distribution models in marine environments: A literature review and spatial analysis of evidence. Ecol. Model. 415, 108837. doi: 10.1016/j.ecolmodel.2019.108837
Meng, J., Wang, L., Zhuang, Y., Zhan, Z., Yang, C. (2023). Herbal textual research on Piperis Kadsurae caulis in famous classical formulas. Chin. J. Exp. Traditional Med. Formulae 29, 93–102. doi: 10.13422/j.cnki.syfjx.20220756
Nanda, S. A., Haq, M., Singh, S. P., Reshi, Z. A., Rawal, R. S., Kumar, D., et al. (2021). Species richness and β-diversity patterns of macrolichens along elevation gradients across the Himalayan Arc. Sci. Rep. 11, 20155. doi: 10.1038/s41598-021-99675-1
Ouyang, X., Pan, J., Wu, Z., Chen, A. (2022). Predicting the potential distribution of Campsis grandiflora in China under climate change. Environ. Sci. pollut. R. 29, 63629–63639. doi: 10.1007/s11356-022-20256-4
Riahi, K., Van Vuuren, D. P., Kriegler, E., Edmonds, J., O’Neill, B. C., Fujimori, S., et al. (2017). The Shared Socioeconomic Pathways and their energy, land use, and greenhouse gas emissions implications: An overview. Glob. Environ. Change 42, 153–168. doi: 10.1016/j.gloenvcha.2016.05.009
Shen, L., Deng, H., Zhang, G., Ma, A., Mo, X. (2023). Effect of climate change on the potentially suitable distribution pattern of Castanopsis hystrix Miq. In China. Plants 12, 717. doi: 10.3390/plants12040717
Subedi, S. C., Drake, S., Adhikari, B., Coggeshall, M. V. (2024). Climate-change habitat shifts for the vulnerable endemic oak species (Quercus arkansana Sarg.). J. For. Res. 35, 23. doi: 10.1007/s11676-023-01673-8
Subedi, S. C., Ruston, B., Hogan, J. A., Coggeshall, M. V. (2023). Defining the extent of suitable habitat for the endangered Maple-Leaf oak (Quercus acerifolia). Front. Biogeography 15. doi: 10.21425/F5FBG58763
Sun, C., Mao., J., Bai, B., Bai, Y. (2000). Studies of enzymology on diurnal change of temperature accelerating the rate of wheat seedling growth. J. Jilin Agric. Univ. 22, 30–33. doi: 10.13327/j.jjlau.2000.01.007
Sun, R., Tong, G., Zhang, Q., Xu, L., Sang, Z., Li, Y. (2023). A study on the suitable areas for growing apricot kernels in China based on the Maxent model. Sustainability 15, 9635. doi: 10.3390/su15129635
Varela, S., Lobo, J. M., Hortal, J. (2011). Using species distribution models in paleobiogeography: A matter of data, predictors and concepts. Palaeogeography Palaeoclimatology Palaeoecol. 310, 451–463. doi: 10.1016/j.palaeo.2011.07.021
Wang, T., Jia, Q., Chen, T., Yin, H., Tian, X., Lin, X., et al. (2020). Alleviation of synovial inflammation of Juanbi-Tang on collagen-induced arthritis and TNF-Tg mice model. Front. Pharmacol. 11. doi: 10.3389/fphar.2020.00045
Wang, E., Lu, Z., Rohani, E. R., Ou, J., Tong, X., Han, R. (2024). Current and future distribution of Forsythia suspensa in China under climate change adopting the MaxEnt model. Front. Plant Sci. 15. doi: 10.3389/fpls.2024.1394799
Wang, Y., Wu, K., Zhao, R., Xie, L., Li, Y., Zhao, G., et al. (2024). Prediction of potential suitable habitats in the 21st century and GAP analysis of priority conservation areas of Chionanthus retusus based on the MaxEnt and Marxan models. Front. Plant Sci. 15. doi: 10.3389/fpls.2024.1304121
Wu, X. P., Song, L. H., Yang, Q., Yang, Y., Hu, M. L. (2024). Assessing the impact of climate change on the habitat dynamics of Magnolia Biondii in China: a MaxEnt modelling approach. Appl. Ecol. Env. Res. 22, 2241–2255. doi: 10.15666/aeer/2203_22412255
Xu, J., Liu, T., Zang, G., Zheng, Y. (2024). Prediction of suitable areas of Eremochloa ophiuroides in China under different climate scenarios based on MaxEnt model. J. Beijing Forestry University. 46, 91–102. doi: 10.12171/i.1000-1522.20230022
Xu, N., Meng, F., Zhou, G., Li, Y., Wang, B., Lu, H. (2020). Assessing the suitable cultivation areas for Scutellaria baicalensis in China using the Maxent model and multiple linear regression. Biochem. Syst. Ecol. 90, 104052. doi: 10.1016/j.bse.2020.104052
Xu, W., Zhu, S., Yang, T., Cheng, J., Jin, J. (2022). Maximum Entropy Niche-Based Modeling for predicting the potential suitable habitats of a traditional medicinal plant (rheum nanum) in Asia under climate change conditions. Agriculture-london. 12, 610. doi: 10.3390/agriculture12050610
Yan, H., Feng, L., Zhao, Y., Feng, L., Wu, D., Zhu, C. (2020). Prediction of the spatial distribution of Alternanthera philoxeroides in China based on ArcGIS and MaxEnt. Global Ecol. Conserv. 21, e00856. doi: 10.1016/j.gecco.2019.e00856
Yang, Y., He, J., Liu, Y., Zeng, J., Zeng, L., He, R., et al. (2023). Assessment of Chinese suitable habitats of Zanthoxylum nitidum in different climatic conditions by Maxent model, HPLC, and chemometric methods. Ind. Crops Prod. 196, 116515. doi: 10.1016/j.indcrop.2023.116515
Yang, J., Jiang, P., Huang, Y., Yang, Y., Wang, R., Yang, Y. (2022). Potential geographic distribution of relict plant Pteroceltis tatarinowii in China under climate change scenarios. PloS One 17, e0266133. doi: 10.1371/journal.pone.0266133
Zhan, P., Wang, F., Xia, P., Zhao, G., Wei, M., Wei, F., et al. (2022). Assessment of suitable cultivation region for Panax notoginseng under different climatic conditions using MaxEnt model and high-performance liquid chromatography in China. Ind. Crops Prod. 176, 114416. doi: 10.1016/j.indcrop.2021.114416
Zhang, Y., Chen, H., Zeng, M., Guo, P., Liu, M., Cao, B., et al. (2024). Futoquinol improves Aβ 25–35 -induced memory impairment in mice by inhibiting the activation of p38MAPK through the glycolysis pathway and regulating the composition of the gut microbiota. Phytotherapy Res. 38, 1799–1814. doi: 10.1002/ptr.8136
Zhang, J., Song, M., Li, Z., Peng, X., Su, S., Li, B., et al. (2021). Effects of climate change on the distribution of akebia quinata. Front. Ecol. Evol. 9. doi: 10.3389/fevo.2021.752682
Zhang, H., Sun, X., Zhang, G., Zhang, X., Miao, Y., Zhang, M., et al. (2022). Potential global distribution of the habitat of endangered Gentiana rhodantha franch: predictions based on Maxent ecological niche modeling. Sustainability 15, 631. doi: 10.3390/su15010631
Zhang, K., Zhang, Y., Zhou, C., Meng, J., Sun, J., Zhou, T., et al. (2019). Impact of climate factors on future distributions of Paeonia ostii across China estimated by MaxEnt. Ecol. Inform. 50, 62–67. doi: 10.1016/j.ecoinf.2019.01.004
Zhu, B., Cheng, Y., Hu, X., Chai, Y., Berghuijs, W. R., Borthwick, A. G. L., et al. (2023). Constrained tropical land temperature-precipitation sensitivity reveals decreasing evapotranspiration and faster vegetation greening in CMIP6 projections. NPJ Clim Atmos Sci. 6, 91. doi: 10.1038/s41612-023-00419-x
Keywords: Piper kadsura, environmental variable, habitat suitability, species distribution, ArcGIS
Citation: Li S, Li Y, Hu M, Li Y, Yang M, Wang S, Yu W, Cheng C and Cheng Q (2025) Ecological risk assessment of future suitable areas for Piper kadsura under the background of climate change. Front. Plant Sci. 15:1471706. doi: 10.3389/fpls.2024.1471706
Received: 28 July 2024; Accepted: 26 December 2024;
Published: 20 January 2025.
Edited by:
Orhun Aydin, Saint Louis University, United StatesReviewed by:
Aseesh Pandey, Govind Ballabh Pant National Institute of Himalayan Environment and Sustainable Development, IndiaCopyright © 2025 Li, Li, Hu, Li, Yang, Wang, Yu, Cheng and Cheng. This is an open-access article distributed under the terms of the Creative Commons Attribution License (CC BY). The use, distribution or reproduction in other forums is permitted, provided the original author(s) and the copyright owner(s) are credited and that the original publication in this journal is cited, in accordance with accepted academic practice. No use, distribution or reproduction is permitted which does not comply with these terms.
*Correspondence: Qiqing Cheng, Y2hlbmdxaXFpbmcwOTE3QDE2My5jb20=
†These authors have contributed equally to this work
Disclaimer: All claims expressed in this article are solely those of the authors and do not necessarily represent those of their affiliated organizations, or those of the publisher, the editors and the reviewers. Any product that may be evaluated in this article or claim that may be made by its manufacturer is not guaranteed or endorsed by the publisher.
Research integrity at Frontiers
Learn more about the work of our research integrity team to safeguard the quality of each article we publish.