- 1School of Emergency Management, Jiangsu University, Zhenjiang, China
- 2Institute of Environment and Ecology, School of the Environment and Safety Engineering, Jiangsu University, Zhenjiang, China
- 3State Key Laboratory of Cotton Biology, Institute of Cotton Research, Chinese Academy of Agricultural Sciences, Anyang, China
- 4Department of Biology, College of Science and Arts, Najran University, Najran, Saudi Arabia
- 5Department of Biology, Faculty of Science, University of Tabuk, Tabuk, Saudi Arabia
- 6Department of Zoology, College of Science, King Saud University, Riyadh, Saudi Arabia
- 7School of Agricultural Engineering, Jiangsu University, Zhenjiang, China
- 8Jiangsu Collaborative Innovation Center of Technology and Material of Water Treatment, Suzhou University of Science and Technology, Suzhou, Jiangsu, China
- 9Department of Environmental Sciences, Government College University, Faisalabad, Pakistan
- 10Department of Biological Sciences and Technology, China Medical University, Taichung, Taiwan
- 11Jingjiang College, Jiangsu University, Zhenjiang, China
Ecosystem exposure to a biological invasion such as plant invasion could contribute to the extinction of native species and loss of productivity and ecosystem balance. Solidago canadensis (S. canadensis) is a highly invasive species that has formed monocultures in China, Europe, Asia, Australia, and New Zealand. It was designated as a notorious invasive species by the Chinese government. It has adversely affected the agroecosystem’s ability to germinate various plant seeds, including wheat, lettuce, and pepper, which could lead to food insecurity. This study was conducted to control the invasive species S. canadensis by utilizing a competitive species, Sorghum bicolor (S. bicolor) as a cover plant. Sorghum bicolor exudes allelochemicals such as sorgoleone from its roots which suppress the photosystem II activity of nearby plants. The synthesis of sorgoleone depends on a supply of nitrogen. The present study involved the cultivation of S. bicolor alongside the invasive species S. canadensis, with three different invasion levels (high, medium, and low) and three different nitrogen forms (ammonical, nitrate, and combined ammonical and nitrate nitrogen) applied as a modified Hogland solution. S. bicolor expressed higher performance over the invasive species under ammonical and combined nitrogen forms under low and medium invasion levels. Furthermore, even at greater levels of invasion, S. bicolor was not suppressed by S. canadensis. However, the plant height and dry biomass of S. bicolor were significantly high across both nitrogen forms. Leaf area, CO2 uptake, and photosystem II activity of S. canadensis were unable to sustain its growth under the low invasion condition. The plant biomass of S. canadensis was suppressed by up to 80% and the relative dominance index of S. bicolor was 5.22 over S. canadensis. There was a strong correlation between CO2 uptake, leaf area, and plant biomass. Principal component analysis showed that the first four components had a total variance of 96.89%, with principal component 1 (PC1) having the highest eigenvalue at 18.65. These promising findings suggested that S. bicolor, whose high intensity might be employed to control the invasion process for environmental safety, might be able to recover the barren ground that S. canadensis had invaded.
1 Introduction
Plant invasion is a major threat to biodiversity, ecosystem balance, and its management. The agroecosystem, which makes up over 40% of the world’s land surface, is extremely vulnerable to invasion (Frost et al., 2019; Batish et al., 2022; Allen et al., 2022). Solidago canadensis, native to North America, is the most important and economically significant invasive species in the agroecosystems of Central and Western Europe, Asia, Australia, New Zealand, and China (Anastasiu and Negrean, 2005; Szabó et al., 2020; Qi et al., 2022; Tian et al., 2023). Strong propagation strategies, such as producing a large number of seeds and growing from rhizomes, allow it to dominate an invaded ecosystem (Gazoulis et al., 2022; Khan et al., 2023a). The invasion of S. canadensis has put several native species in danger of going extinct (Wang et al., 2021; Gazoulis et al., 2022). It has decreased agricultural productivity, biodiversity, and medicinal plants, and the Chinese government has declared it an exotic invasive species (Wang et al., 2017; Mitryasova and Koszelnic, 2021; Kato-Noguchi, 2023). Thus, sustainable and environmentally friendly management of ecosystems is crucial.
Potential native resources may be sustainable and eco-friendly ways to manage an invaded ecosystem. Sorghum bicolor is a strong domesticated plant that can re-sprout and vigorously grow and is mainly grown for its grain and fodder (Venkateswaran et al., 2019). It uptakes nutrients efficiently and grows in diversified soil types such as salt-affected, drought-prone, and water-logged soils (Calone et al., 2020; Chen et al., 2020; Zhang et al., 2023). Crop rotation, root exudates, and water extracts of S. bicolor have all been shown to be effective weed control measures in agroecosystems (Zucareli et al., 2019). Because of its effective nutrient intake, allelopathic nature, ability to cover soil, and rapid growth rate during the invasive species’ vegetative growth cycle, it can inhibit S. canadensis (Werner et al., 1980; Afzal et al., 2023). It releases hydrophobic and hydrophilic allelochemicals. Sorgoleone is a unique hydrophobic allelochemical, a benzoquinone (2-hydroxy-5-methoxy-1,4-benzoquinone), that is secreted by root hairs, is present in the soil, and exhibits potent phytotoxicity towards a variety of nearby plants (Głąb et al., 2017; Besançon et al., 2020). It is produced throughout the growth period of S. bicolor. It inhibits several physiological functions, including the uptake of CO2, the transport of electrons in mitochondria, the activity of p-hydroxyphenylpyruvate dioxygenase (HPPD) and H+-ATPase in roots, and the uptake of water (Einhellig and Souza, 1992; Weidenhamer, 2005).
Plant-to-plant interaction is highly dependent on plant intensity. Many invasive species have been reported to exert high competition with native species and suppress them. For example, Erodium cicutarium invaded a granivore site and dominated all plots (Valone and Weyers, 2019). When invading species become more intense, they release more allelochemicals, which increases competition. S. canadensis suppresses Lactuca sativa and its suppression depends on the invasion intensity (Yu et al., 2022). Effective plant intensity of S. bicolor is important for successful competitiveness between invasive species and S. bicolor. It has not been tested against invasive species. However, it was studied to identify the effect of plant intensity on growth, forage, and grain yield and it was concluded that 88,888 plants ha−1 was the best intensity to plant at in Ethiopia (Bayu et al., 2005). Yan et al. (2023) reported that a change in plant intensity from 83,000 to 166,000 plants ha-1 increased yield by 188 kg ha-1 per 10,000 plants.
The root and shoot growth of S. bicolor is highly responsive to the form of nitrogen (N). Plants uptake N either in nitrate or ammonical forms (Smil, 1999; Crawford and Glass, 1998). These two forms of N in the soil are interconnected with each other through the ammonification and nitrification processes (Anas et al., 2020). Plants show different adaptations for the uptake of ammonical and nitrate nitrogen forms (Fisher et al., 2010; Du et al., 2020; Zhao et al., 2023). The production of sorgoleone is prolonged by root growth, proton gradient, and H-ATPase under ammonical nitrogen (Di et al., 2018; Afzal et al., 2023). In previous studies, the relation of sorghum root exudates is dependent on the available N form. Afzal et al. (2020) reported that the application of the ammonical form of N improved the production of root exudates. Ammonical nitrogen is biologically nitrified, releasing H+ ions and lowering the pH in the rhizosphere soil. Plasma membrane H+-ATPase activity increases in the presence of NH4+ (Zeng et al., 2016). Zhao et al. (2023) reported that sorgoleone had a negative charge which was exuded in the rhizosphere due to the activity of plasma membrane H+-ATPase. Sorgoleon production and H+-ATPase activity increase under a rhizosphere ammonium concentration of ≤ 0.1 mM (Zeng et al., 2016).
The environment and human health are harmed by the previous ineffective and unsustainable methods of controlling S. canadensis, which included burning, eradication, and the use of herbicides (Narwal and Haouala, 2013; Mohd Ghazi et al., 2023). The purpose of this study was to determine the influence of S. bicolor on invasive species under different invasion gradients and different nitrogen conditions. We wanted to evaluate to what extent the changes induced by S. bicolor are systematic and predictable, and to what extent they are manageable under controlled conditions. In order to investigate how the changes would affect ecosystem services, the productivity of potentially degraded land restored by covering the invasive species and the performance of S. bicolor were assessed in an outdoor experiment. It was hypothesized that S. bicolor would (a) decrease the growth of invasive S. canadensis aboveground and belowground, (b) dominate the invasive plant species by changing their physiological properties, and (c) reduce the dominance of invasive S. canadensis compared with sorghum grown under different nitrogen conditions.
2 Materials and methods
2.1 Experimental site and plant culture
The study was carried out in an outdoor experiment in a greenhouse at the Institute of Environment and Ecology, School of Environmental Science and Safety Engineering, Jiangsu University, Zhenjiang, China from April to September 2023. There is an average of 1,500 mm of precipitation every year in that region, with July having the greatest average of 179 mm and December having the lowest average of 24 mm. The annual maximum and minimum temperatures were recorded as 27.4°C in July and 2.7°C in January (Shen et al., 2023). The riverside is more vulnerable to invasion and sand collected at this site was sieved (2 mm), washed, and sterilized to remove stones, weeds, seeds, and impurities. The pots were filled with 2.5 kg of sand from the Yangtze River, stabilized for 1 week, and wet with double distilled water prior to the transplantation of the seedlings.
The S. canadensis seedlings were raised to obtain healthy and similarly sized seedlings. To avoid seed dispersal of the invasive species, S. canadensis, seeds of S. canadensis were planted in petri plates for germination. The seedlings were then transplanted in plastic trays after 15 days of germination for 2 months to obtain similar two-to-three-leaf seedlings. S. bicolor seeds were disinfected with 75% ethanol for 30 s and 10% NaOCl for 10 min and washed thoroughly in double distilled water. Seed disinfection treatment was performed to ensure seed germination and seedlings without seed-born disease. Sorghum bicolor seedlings were raised in plastic trays from the disinfected seeds. According to Oswald et al. (2001), the 10-day-old healthy S. bicolor seedlings (two to three leaves) and two-to-three-leaf S. canadensis seedlings were transplanted into pre-described pots with a 23 cm diameter with plant densities of four S. canadensis plants (100% S. canadensis: P) per pot, three S. canadensis plants and one S. bicolor plant (75% S. canadensis (H)+25% S. bicolor (L) per pot, two S. canadensis plants and two S. bicolor plants (50% S. canadensis (M) + 50% S. bicolor (M) per pot, one S. canadensis plant and three S. bicolor plants (25% S. canadensis (L)+75% S. bicolor (H) per pot, and four S. bicolor plants (100% S. bicolor: P) per pot. Following the transplantation of the seedlings into the pots, the pots were kept moist with water for a week before the Hogland solutions were applied. After 1 week after the transplantation of the seedlings, 100 times diluted modified Hogland solutions (Supplementary Table S1) with two different forms of available N and their combinations, i.e., 100% NO3, 100% NH4, and 50% NO3+50% NH4 concentrations, as well as a control (0% N; CK), were applied at a rate of 100 ml/pot two to three times per week. In total, there were 120 pots with six repeats for each plant density and form of N (Supplementary Figure S1).
2.2 Plant height, diameter, and root growth traits
The plants in the experiment were harvested at the panicle initiation stage of S. bicolor. Before the plants were harvested, the height of five plants was randomly measured using a measuring tape, the number of green leaves was counted, and the plant diameter was measured using a digital Vernier caliber scale (DL91200). The aboveground and belowground parts of the plants were harvested. The dry weight of leaf blades, stems, and roots were noted using a digital weighing balance after the plant tissues were dried at 120°C until constant weight. Root length and root branches were measured according to Khan et al. (2023b). The roots were carefully removed from the pots and washed gently to remove sand media and the root length was measured with measuring tape and root branches were counted.
2.3 Gaseous exchange traits
The gas exchange parameters CO2 uptake (Pn), transpiration rate (Tr), and stomatal conductance (gs) were measured with a Li-COR 6500 (Lincoln, NE, USA) on a full sunny day between 9:00 am and 11:30 am. The first fully expanded leaf from the top of the plant was selected from both the plant species and the conditions inside the chamber were 1000 µmol m-2s-1 photon flux density, 400 µmol mol-1 carbon dioxide, and a 25°C temperature (Anas et al., 2021b). Water use efficiency (WUE) was measured as a ratio of CO2 uptake and transpiration rate.
2.4 Chlorophyll fluorescence
The chlorophyll fluorescence of six different plant leaves was measured using a FluorPen (Photon Systems Instruments) for each treatment. Each leaf was clipped after a 20-min dark period. The clip was opened just before recording the electron transfer efficiency (Fm/Fo), potential photochemical activity (Fv/Fo) and maximum photochemical efficiency (Fv/Fm) readings (Davarzani et al., 2023).
2.5 Nitrogen content and leaf functions
Leaf N and greenness were measured according to Huang et al. (2022). Briefly, a portable handheld soil plant analysis development (SPAD) meter was used to measure leaf greenness and N content. Three distinct positions were used to assess the nitrogen content and leaf greenness of the first fully expanded leaf of six plants per treatment. Leaf area, perimeter, length, and width were measured using the YMJ-CH intelligent leaf area system (Topu Yunnong Technology, Zhejiang, China) (Cui et al., 2023).
2.6 Relative yield and relative dominance index
Relative yield and relative dominance index were measured by Equations 1, 2 respectively (Yau and Hamblin, 1994; Wang et al., 2020; Pan et al., 2023).
where RY is relative yield, Yi is the yield of species ‘i’ in an interculture, Ymono is the yield of a pure stand, and p is the proportion of species ‘i’.
where RDI is the relative dominance index, Yi is the yield of species ‘i’ in an interculture and Ytotal is the sum yield of all species in an interculture.
2.7 Statistical analysis
The mean value was calculated for plant height, diameter, root growth traits, gas exchange, chlorophyll fluorescence, nitrogen content, leaf functions, and relative yield and dominance index. The main effects and interactions for the computed mean values of the independent variables were examined using analysis of variance (ANOVA). The calculated mean values were also used as “Trait” values. For every treatment combination, these “Trait” values were converted to the natural log value as follows:
where Trait_x is the trait value of species x and Trait_y is the trait value of species y. A negative lnR value shows that species x is suppressed by the dominance of species y (Meng et al., 2015; Huang et al., 2021; Guo et al., 2023).
The lnR values were calculated using Microsoft Excel 2010 and further analysis was performed in RStudio using the “stat” and “ggplot2” packages for ANOVA and the scatter plot, respectively. Pearson’s correlation analysis shows a linear relationship among the variables and principal component analysis (PCA) visualizes the data to identify trends, patterns, or outliers. The “corrplot” and “fviz_pca” functions in R 4.3.2 were used to analyze the correlation and perform PCA, respectively, of the plant height, diameter, root growth traits, gas exchange, chlorophyll fluorescence, nitrogen contents, leaf functions, and relative yield and dominance index lnR values.
3 Results
3.1 Effect of invasion level of S. canadensis and nitrogen form on the phenotype and growth interaction of S. bicolor
Plant height, number of leaves, stem diameter, leaf greenness, and N content in the leaf were significantly influenced by the plant species and their interactions with invasion levels and available N forms (Table 1; Supplementary Table S2). However, the main effects of invasion level, nitrogen form, and interaction of invasion level with available N form were non-significant (Table 1; Supplementary Table S2).
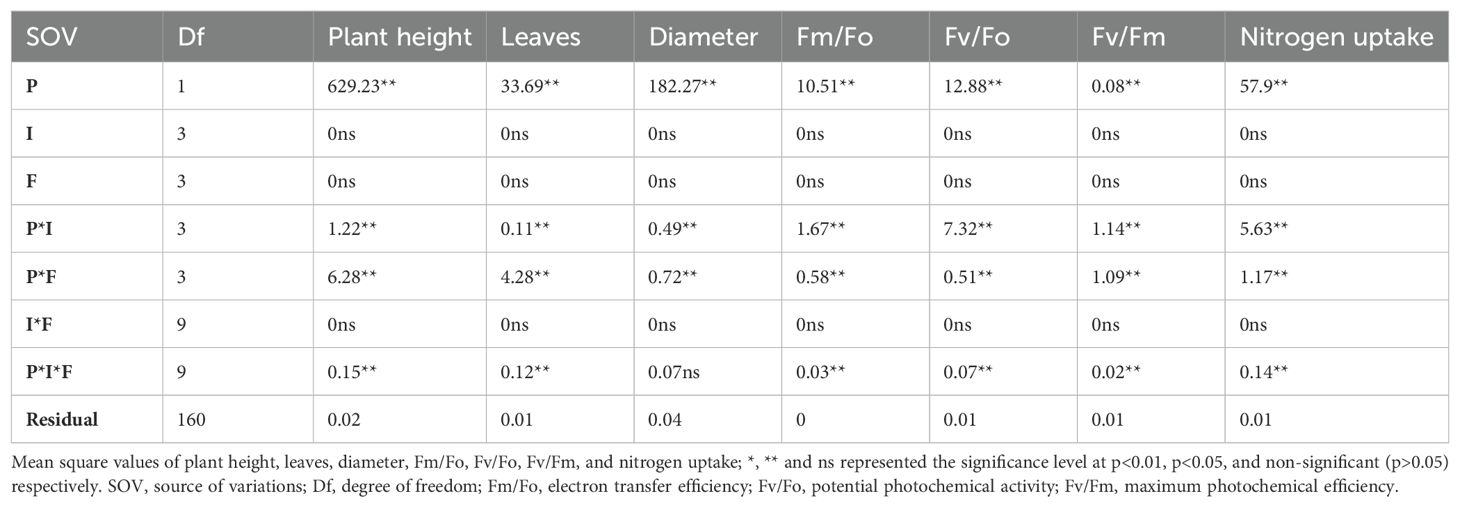
Table 1. Plant height, leaves, diameter, florescence traits, and nitrogen uptake of S. canadensis and S. bicolor under varied invasion levels and available nitrogen forms for plant species (P), invasion level (I), and nitrogen form (F).
The relative plant height of S. canadensis (-2.52 to -1.28) was significantly suppressed under the low invasion level of S. bicolor for all N forms in relation to the pure stand (Figure 1F). The growth of S. canadensis was higher under the low population of S. bicolor and the NO3- N form compared to the medium and high populations of S. bicolor (Figures 1A–C). However, the highest competitive ability of S. bicolor was observed under the combined N form (NH4++NO3-) and a low invasion level (Figure 1C). The pure stand of S. canadensis showed highest growth under nitrate nitrogen and S. bicolor showed under combined nitrogen form (Figures 1D, E). Maximum relative plant heights of 2.74 and -1.27 were observed for S. bicolor and S. canadensis, respectively. Furthermore, across the invasion levels, the height of S. bicolor (2.74) was higher under the low invasion level (Figure 1F). The respective mean plant heights of S. bicolor and S. canadensis varied from 61.8 to 15.9 cm and 12.36 to 0.98 cm, respectively (Supplementary Figure S2A).
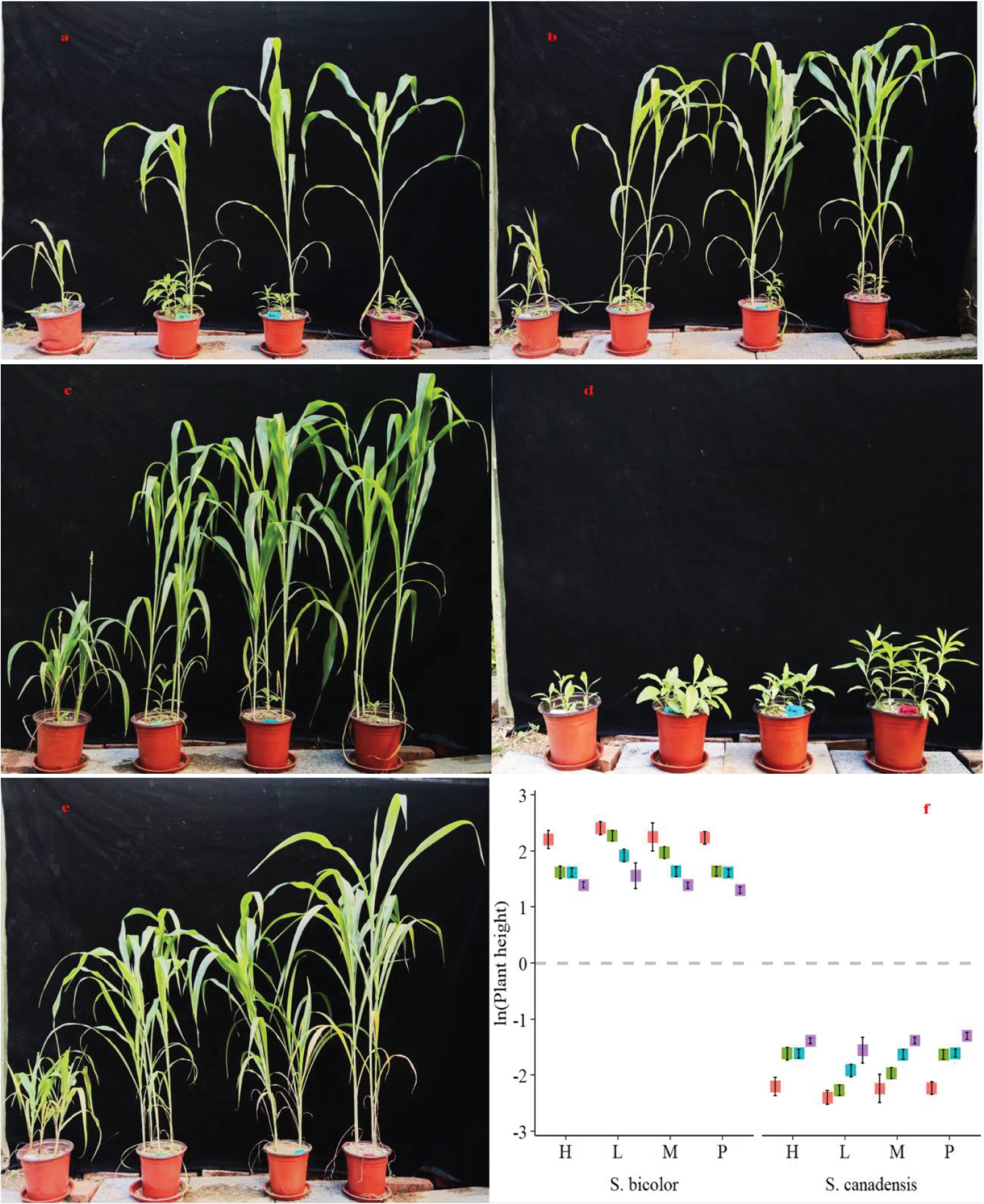
Figure 1. Phenotypic response of S. canadensis and S. bicolor under varied invasion levels and available nitrogen forms. (A) high invasion level; (B) medium invasion level; (C) low invasion level; (D) pure stand of invasive plant species (S. canadensis); (E) pure stand of domesticated plant (S. bicolor); (F) relative plant height of both plant species.
The relative number of green leaves of S. canadensis was higher than S. bicolor. The highest relative number of leaves (0.88) of S. canadensis was observed against the low invasion level and the NO3- N form (Figure 2A). The maximum number of green leaves per plant of S. canadensis was 16 under the pure culture. This was higher than the number of S. bicolor (Supplementary Figure S2B). The largest relative stem diameter of S. canadensis (-0.39) was noted under the high invasion levels, and the relative stem diameters of the two plant species differed significantly (Figure 2B). This was similar to plant height as it was higher under the low invasion levels (Supplementary Figure S2C). The leaf greenness (SPAD) values for S. bicolor were also higher (52.73) for the low invasion level and both nitrogen forms (Supplementary Figure S2D). The relative SPAD value (1.74 to -1.74) was generally influenced across the plant species but no significant difference was observed within a single plant species under all invasion levels and available N forms (Supplementary Figure S6A).
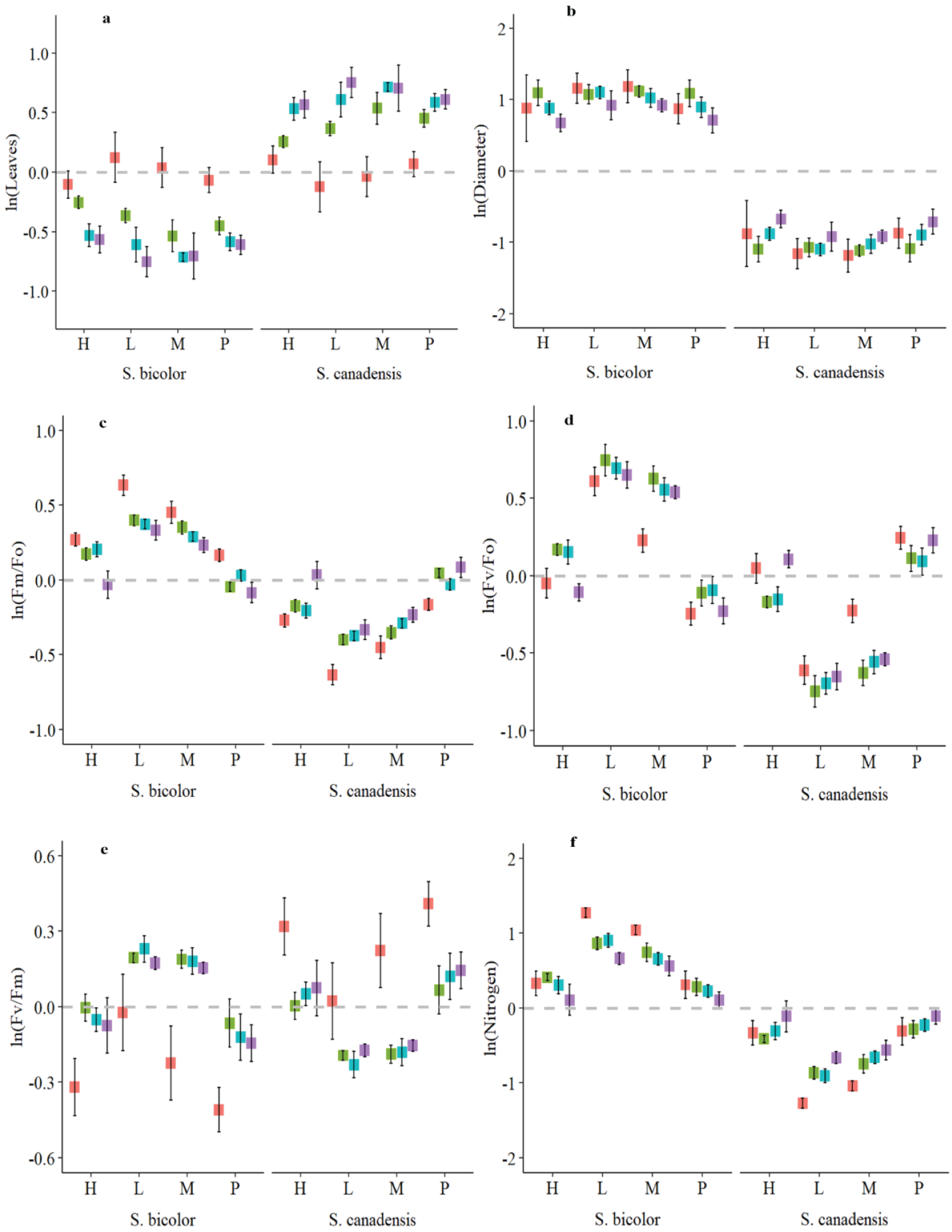
Figure 2. Relative number of leaves (A), stem diameter (B), chlorophyll fluorescence (C-E), and leaf nitrogen (F) characteristics of S. canadensis and S. bicolor under varied invasion levels and available nitrogen forms. Red color, control; green color, ammonical N; blue color, both nitrogen forms; purple color, nitrate N; H, high invasion level; L, low invasion level; M, medium invasion level; P, no invasion.
For all invasion levels, S. canadensis had the largest relative plant leaf N content (0.28 to -0.80) under the nitrate N form; however, at low invasion levels, S. canadensis displayed lower relative leaf N content under both N forms (Figure 2F). The highest leaf nitrogen content was attained by S. canadensis (2.78 mg/g) in the pure culture under both nitrogen forms (Supplementary Figure S3A).
3.2 Physiological interaction of S. bicolor and S. canadensis under different invasion and nitrogen conditions
Chlorophyll fluorescence describes photosystem II functionality and Fv/Fm is the ratio of the variable and maximum fluorescence of a dark-adapted leaf. The relative Fv/Fm ratio ranged from 0.18 to -0.28 and from 0.42 to -.26 for low and medium invasion levels for both plant species, respectively (Figures 2C–E). It was not significant within the species and was greatly impacted by the application of nitrogen, surpassing the control of both species (Supplementary Figures S2E, F).
The gaseous exchange parameters Pn, Tr, and gs were significantly different for the plant species, interactions of plant species with planting intensities, and N form (Table 2; Supplementary Table S2). The main effect of nitrogen and invasion and the interaction between these two variables were non-significant. However, the significance level of Tr and gs was less in the three-way interaction (Supplementary Table S2). Similarly, the fluorescence traits were significant for planting species, interaction of planting species with intensities, interaction of planting species with N forms, and their three-way interaction (Table 2; Table S2). However, these were unaffected by planting invasion levels and available N forms (Table 2, Supplementary Table S2).
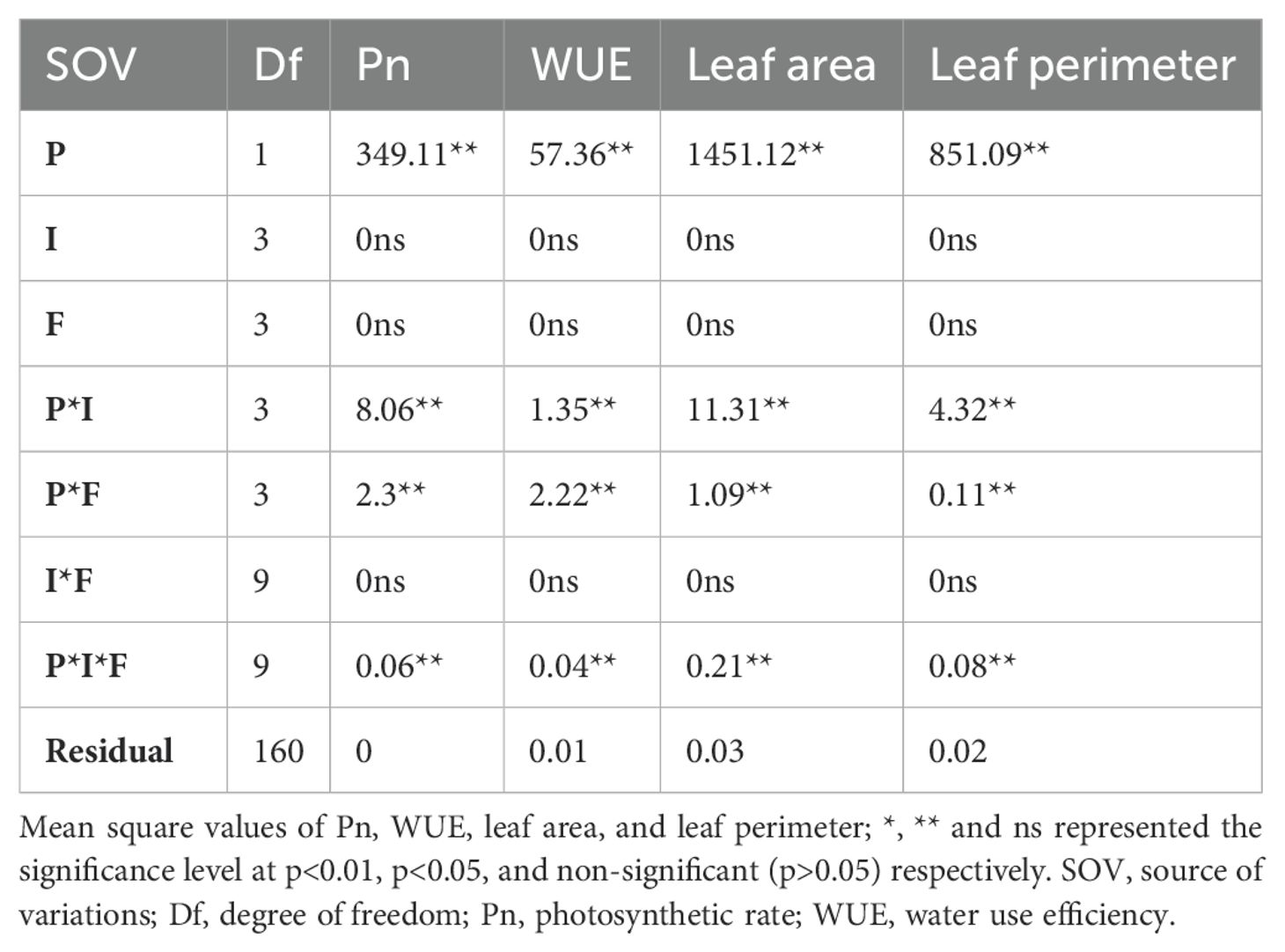
Table 2. Gaseous exchange, water use efficiency, leaf area, and leaf perimeter of S. canadensis and S. bicolor under varied invasion levels and available nitrogen forms for plant species (P), invasion level (I), and nitrogen form (F).
S. bicolor had higher relative Pn (2.11) and Tr (1.47) levels compared to S. canadensis but gs (-3.35 to -5) was the opposite and Ci was not significant (Figure 3A; Supplementary Figure S6B). WUE was higher for nitrate N across the plant species and all planting intensities (Figure 3B). However, the pure stand for both species and the high invasion level had similar water use efficiency across all the N forms except the no N condition (Figure 3B). S. bicolor showed non-significant differences for gas exchange parameters under ammonical and combined available nitrogen forms (Supplementary Figures S3B–F).
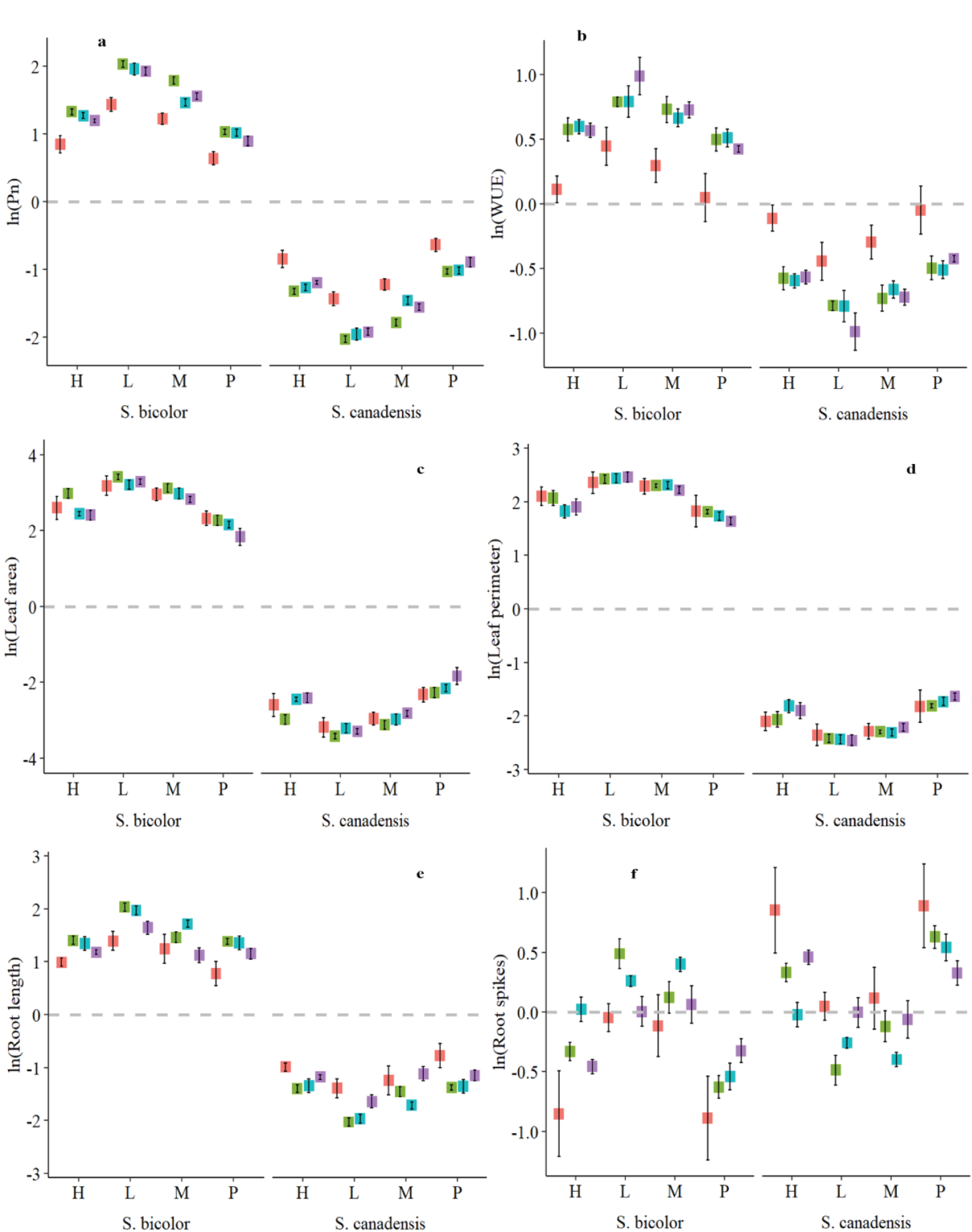
Figure 3. Relative photosynthetic rate (A), water use efficiency (B), leaf area (C), leaf perimeter (D), root dry weight (E), and root spikes (F) of S. canadensis and S. bicolor under varied invasion levels and available nitrogen forms. Red color, control; green color, ammonical N; blue color, both nitrogen forms; purple color, nitrate N; H, high invasion level; L, low invasion level; M, medium invasion level; P, no invasion.
3.3 Effect of invasion level of S. canadensis and nitrogen form on the leaf area and root growth of S. bicolor
Leaf area and its components were also affected only across the plant species and by its interactions with invasion levels and available N forms. (Table 1; Supplementary Table S2). The maximum relative leaf area for S. bicolor (3.55) was observed under the high invasion level and ammonical N form. S. canadensis had a maximum relative leaf area (-2.10) under high invasion levels and the nitrate N form (Figures 3C, D; Supplementary Figures S6E, F). Leaf parameters were influenced by plant species, invasion levels, and nitrogen forms, and the maximum leaf width for S. bicolor (26.2 mm) was observed under both nitrogen forms and low invasion levels (Supplementary Figure S4).
Root length and root spikes were significantly different for plant species and their interactions with invasion levels and available N forms. However, the largest relative root spikes (1.39) were observed for S. canadensis (Figures 3E, F). The species-specific root length varied from 64.18 to 4.32 cm for both and it was not statistically significant (Supplementary Figure S5A).
3.4 Response of biomass and relative indices of S. bicolor under different invasion and nitrogen conditions
The root dry weight was significant against plant invasion levels, N forms, and their interaction (Table 3). The shoot/root weight was inversely correlated with the relative dry weight of the plant and, in comparison to ammonical N forms, it was comparable to the control and available N form under both low and high invasion levels (Figures 4A–C). The maximum relative plant biomass for S. bicolor (4.16) was observed under the high invasion level and ammonical N form and the lowest for S. canadensis (-4.16) was observed under the same conditions (Figure 4D). Shoot weight, root weight, and total plant dry biomass of S. bicolor were similar under the low and medium invasion conditions for the ammonical N form (Supplementary Figures S5C–F).
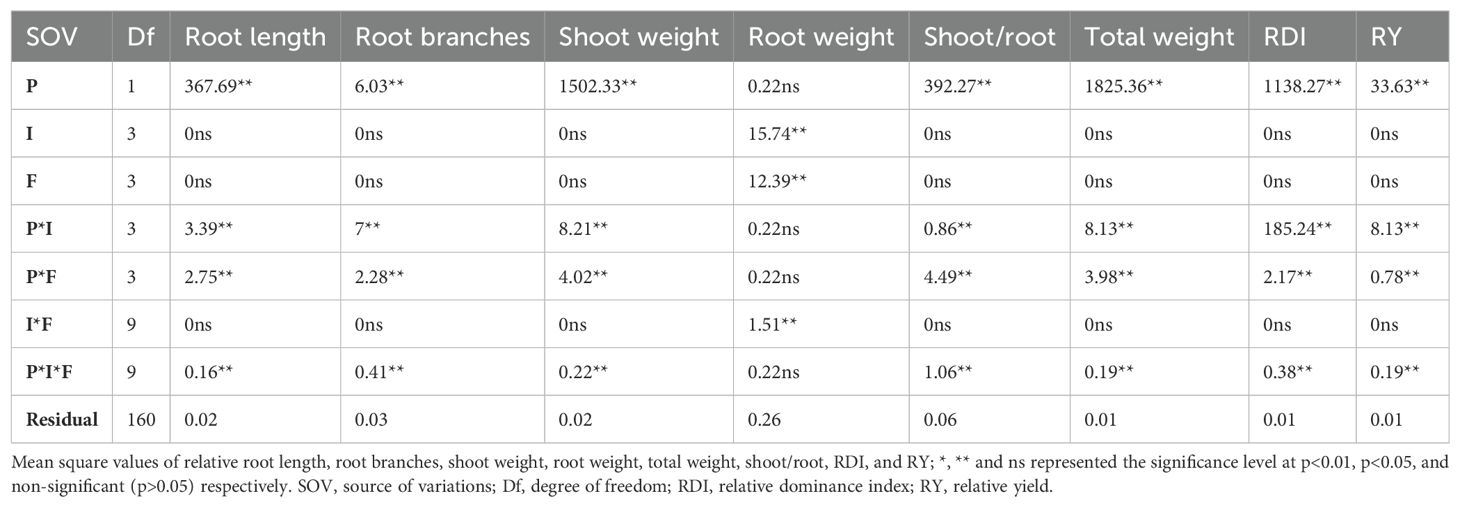
Table 3. Root length; root branches; dry biomass of roots, shoots, and total plant; shoot to root ratio; relative dominance index; and relative yield of S. canadensis and S. bicolor under varied invasion levels and available nitrogen forms for plant species (P), invasion levels (I), and nitrogen forms (F).
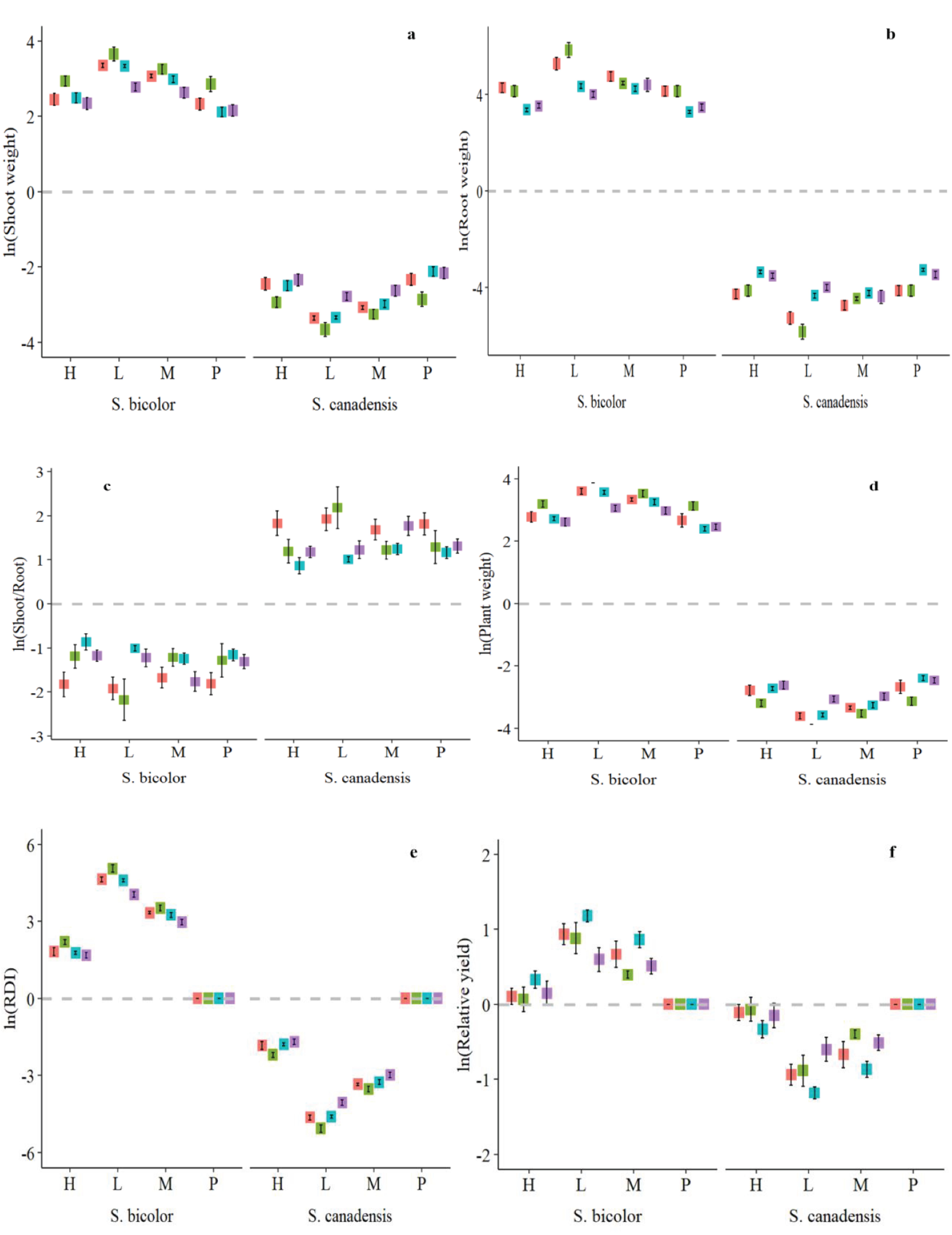
Figure 4. Relative shoot dry weight (A), root dry weight (B), plant dry weight (C), shoot to root ratio (D), relative yield (E), and relative dominance index (F) of S. canadensis and S. bicolor under varied invasion levels and available nitrogen forms. Red color, control; green color, ammonical N; blue color, both nitrogen forms; purple color, nitrate N; H, high invasion level; L, low invasion level; M, medium invasion level; P, no invasion.
The relative yield and RDI for the plant species and their interactions with invasion level and available N forms, and three-way interactions were significantly different (Table 3). S. bicolor had a higher RDI (5.22) compared to S. canadensis even under high invasion levels, and the ammonical and combined N forms (Figure 4E). Similarly, S. bicolor had a higher relative yield (1.29) under the combined N form, and the relative yield of S. canadensis under the low medium and medium invasion levels was lower compared to S. bicolor (Figure 4F).
3.5 Relationship between response variables of S. bicolor and S. canadensis under different invasion and nitrogen conditions
Pearson’s correlation coefficient determines the linear relationship between different datasets. A strong Pearson correlation coefficient was observed between Pn rate (0.98), plant height (0.98), and plant diameter (0.98; Figure 5). Plant dry biomass also showed a strong correlation with plant height (0.98), diameter (0.98), Pn rate (0.98), Tr rate (0.97), leaf area (0.99), and shoot weight (0.99). However, a weak correlation was observed between biomass and fluorescence parameters (0.67-0.82; Figure 5). The leaf N content had a strong correlation with RDI, SPAD, and relative yield, and negative correlations for gs and shoot-to-root ratio were observed against all the parameters (Figure 5).
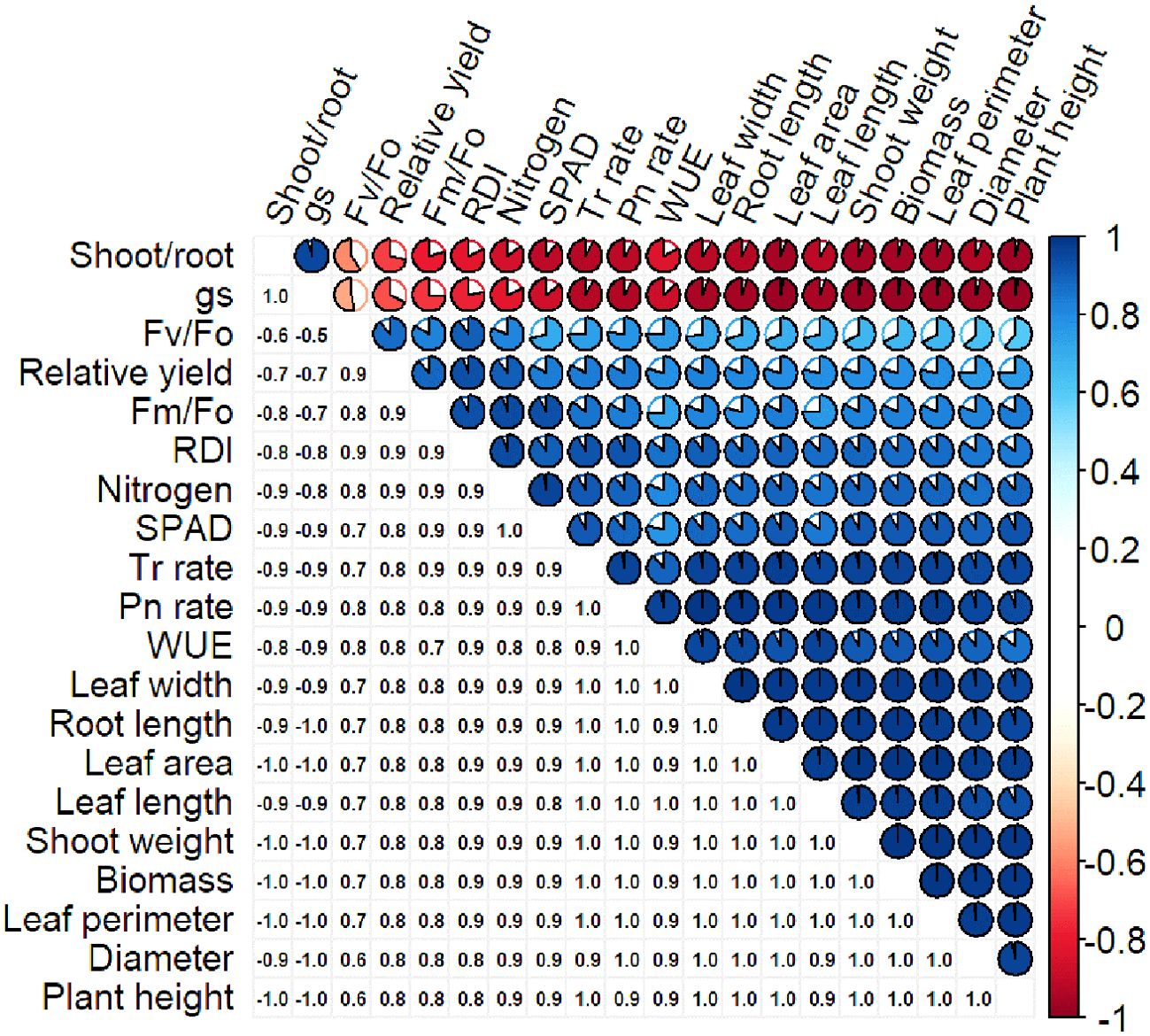
Figure 5. Correlation of different characteristics of S. canadensis and S. bicolor under varied invasion levels and available nitrogen forms.
Principal component analysis separated three treatment factors from 23 components (Figure 6). Ellipse grouping showed two, four, and four groups for plant species, plant intensities, and available N forms, respectively (Figures 6A, C, E). The cumulative variance for PC1 to PC4 was distributed at 96.89%, and the eigenvalues of PC1, PC2, and PC3 were 18.65, 3.21, and 1.04, respectively (Figure 6B). The distribution of the cumulative variance of the top 10 components was displayed using a scree plot (Figure 6D). PC1 had the highest cumulative variance, at 77.7%, followed by PC2. The PCA plot showed the relationship of the most relevant principal components (Figure 6F). The components with less than a 90° angle were positively correlated with each other and the others were negatively correlated with each other. The PCA plot also showed the contribution of observations to PC1 and PC2 across all the treatment factors respectively (Figure 6F).
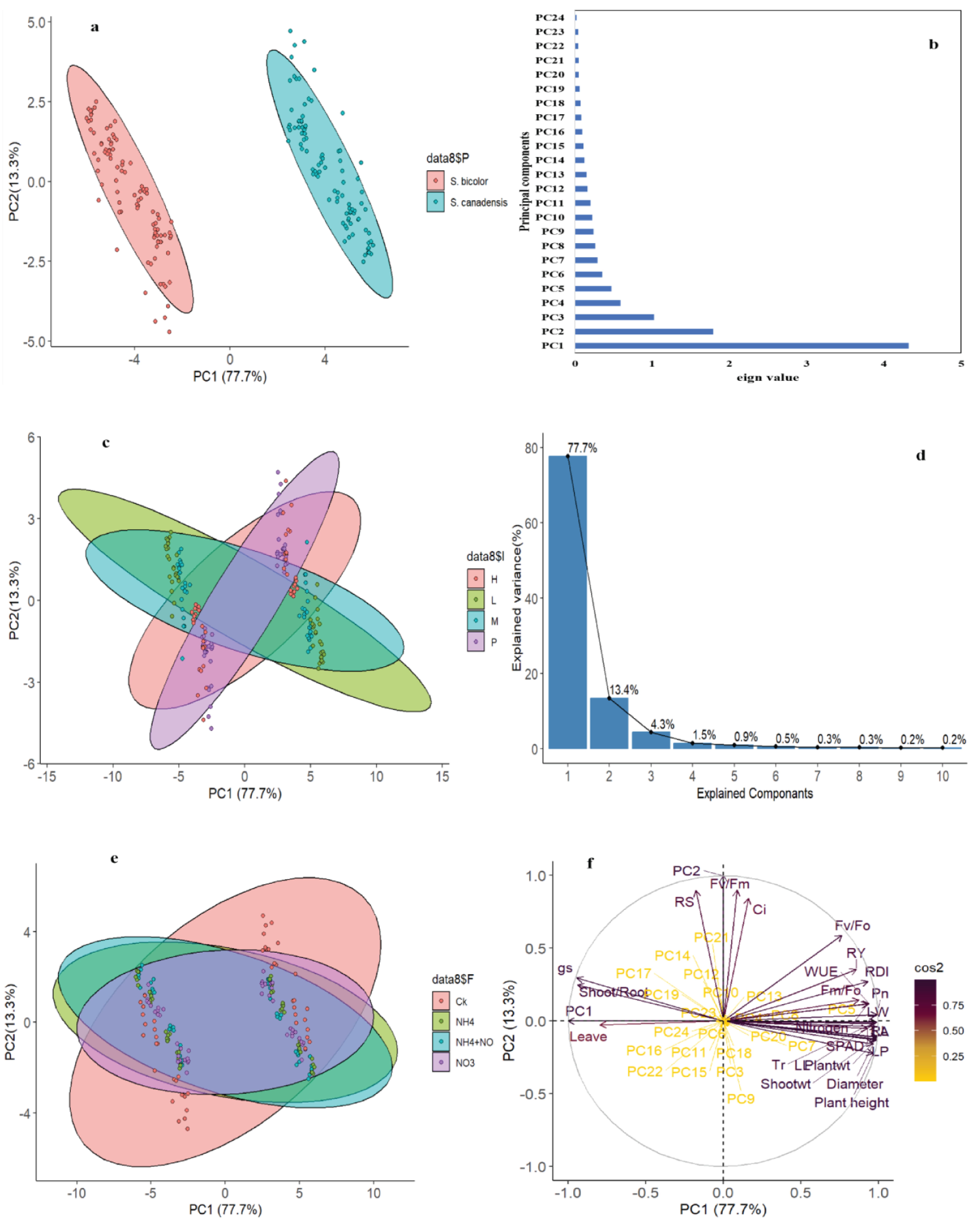
Figure 6. Principal component analysis of S. canadensis and S. bicolor under varied invasion levels and available nitrogen forms. Biplot of plant species (A), eigenvalue for principal component (B), biplot for invasion levels (C), variance distribution (D), biplot for available nitrogen forms (E), and principal component plot (F).
Except for the plant species, the main impacts of the treatment variables, invasion and N form, were not statistically significant. In the low to high invasion levels, S. bicolor exhibited a stronger dominance index over the invasive S. canadensis due to its higher plant height, leaf area, root length, and biomass values. Furthermore, the ammonical N form and the combined forms showed a positive relationship with the growth of S. bicolor which suggested that it was more responsive to the ammonical N form.
4 Discussion
4.1 Effect of S. bicolor on the growth of S. canadensis
S. bicolor suppressed the growth of invasive S. canadensis by obtaining more height and leaf area and covering the invasive species. Plant dry biomass is the ultimate indicator that describes the successful growth and development of a plant under the provided conditions. Our results showed that S. bicolor attained more biomass which supported our first hypothesis that S. bicolor would decrease the growth of invasive S. canadensis both above and belowground (Figure 4D). Allelopathy is an ecological phenomenon in which plants release secondary metabolites that suppress or promote nearby plants. S. bicolor is well-established for its allopathy (Farooq et al., 2013). Cheema and Khaliq (2000) reported that S. bicolor decreased weed dry biomass by up to 40% in a wheat field. The persistence of S. bicolor root exudates also decreased the growth of different weed species (Farooq et al., 2020; Roth et al., 2000). Plant dry biomass is also influenced by plant intensity, for example, the dry biomass of S. bicolor was higher in the low plant intensity treatments compared to the high plant intensity treatment but S. canadensis under a low intensity had lower plant dry biomass (Figure 4D). Furthermore, S. bicolor significantly suppressed S. canadensis in a combination of high and low S. bicolor and S. canadensis intensities. This supported our hypothesis that a higher intensity of S. bicolor suppresses the growth of S. canadensis by changing plant physiology. Similar results were reported by Guo et al. (2023) for Oenothera biennis, which could not compete with Artemisia argyi in a particular plant population. Previous studies reported that high intensity of S. bicolor reduced light interception by weeds (Contreras et al., 2022; Burnside, 1977; Gholami et al., 2013; Besançon et al., 2017a, b). This might be due to low CO2 uptake by S. canadensis underneath. Similarly, the allelochemicals of S. bicolor overcame a weed population by decreasing the production of chlorophyll and the photosynthetic rate (Gonzalez et al., 1997; Jabran and Farooq, 2012; Farooq et al., 2020).
S. bicolor is a C4 plant that uptakes CO2, water, and nitrogen more efficiently than the C3 S. canadensis plant (Zhao et al., 2005; Young and Long, 2000; Anten et al., 1995; Fay et al., 2006). Plant height and diameter have a direct relationship with the application of nitrogen (Anas et al., 2021b). The plant height of S. bicolor was also an indicator of its ability to overcome S. canadensis, because it covers S. canadensis underneath, captures more light and nutrients, and decreases the biomass of S. canadensis (Table 1; Liu et al., 2022; Ying et al., 2023). Plant diameter was significantly influenced by the planting intensity for both plant species under all N conditions. The results are in line with a previous study that found that a high-intensity plant population resulted in a thin plant stem compared to a low-intensity plant population (Anas et al., 2017).
The roots are responsible for the uptake of nutrients and water as well as interacting with soil bacteria. These secrete the secondary metabolites into the soil and have a direct relationship with the uptake process from the soil (Jiang et al., 2019). Plants produce more roots when grown together with other plants instead of alone and this phenomenon is known as self/non-self root discrimination (Hess and De Kroon, 2007). In this study, root length was found to be significantly different which may be due to the capacity to absorb more nutrients due to close contact with a greater volume of growing media (Table 3). Nutrient uptake is dependent on root length instead of root volume. Nutrients in the soil solution are taken up by roots either by mass flow or diffusion (Hodge, 2005). The use of photosynthates is defined by the shoots/roots (Anas et al., 2021a). Compared to S. canadensis, the shoots/roots of S. bicolor showed a substantial difference, indicating a superior usage of photosynthates (Figure 4C). These results were contrary to those of Ren et al. (2019) who found that S. canadensis had a greater shoot/root ratio and leaf area allocation with respect to non-invasive plant species. The reason might be that the growth of S. canadensis in the early stages was lower when compared to S. bicolor (Cox et al., 2018; Kelly et al., 2021). S. bicolor had more shoots/roots than Zea mays in an interculture with Panicum millet and Zea mays (Amanullah and Stewart, 2013). S. bicolor grew better in drought conditions than Zea mays (Danalatos et al., 2009).
4.2 Effect of available nitrogen forms on S. bicolor overcoming S. canadensis
Plant uptake N mainly in two available N forms, either nitrate (NO3-) or ammonium (NH4+), and they prefer a particular form according to their adaptation and environment (Sanchez-Zabala et al., 2015). It has been reported that S. bicolor prefers the NH4+ form of N and S. canadensis grew better under the NO3- form (de Souza Miranda et al., 2016; Miranda et al., 2013; Afzal et al., 2020; Wang et al., 2023). Leaf N content was higher in S. bicolor under the combined N and ammonical forms but S. canadensis positively responded to the nitrate N form. These results were the same as previous studies that showed that S. bicolor is ammonical N-loving and S. canadensis is nitrate-loving. S. bicolor growth was higher under ammonical N compared to nitrate N due to a low accumulation of H2O2 and improved K+/Na+ homeostasis under saline conditions (de Oliveira et al., 2020). Further, Mrid et al. (2016) reported that the application of ammonical N enhanced the growth of S. bicolor by improving the activities of glutamine synthetase and aspartate aminotransferase. These enzymes are important parts of the nitrogen metabolic pathway for protein synthesis. However, root growth and exudation of S. bicolor increase when ammonical N is present, releasing H+ into the rhizosphere and causing the pH to drop (Afzal et al., 2023; Di et al., 2018; Zhao et al., 2023). Root exudates of S. bicolor have the potential to retard the growth of nearby plants. The positive responses of S. bicolor under ammonical N for protein synthesis and root exudation might enhance its biomass and dominance over the invasive S. canadensis. However, another study showed that S. canadensis was flexible with regard to available N forms and it tended to take up dominant forms of available N (Guan et al., 2023). Although S. canadensis is independent of available N forms, in this study, it gained more biomass under the nitrate N form, similar to Wang et al. (2023) and Afzal et al. (2023). Guan et al. (2023) also used dry biomass as a reference to describe the uptake of a specific N form. Our result supported both arguments as S. bicolor acquired the ammonical N form either in the combined treatment or the ammonical form treatment and left less available N for S. canadensis due to its dominant growth (Figure 2F).
The leaf is the main plant organ that provides the surface for sunlight absorption through chlorophyll pigments and starts photosynthetic metabolism. A high leaf area is the key factor for growth, development, and biomass, and an early development of leaf area is key to gaining more biomass (Liu et al., 2020). The higher leaf area of S. bicolor at the early stage might be responsible for its dominance over S. canadensis (Figure 3C). In this study, we found that leaf greenness was significantly higher in S. bicolor under low plant intensity, but higher in S. canadensis in the pure stands and at the high invasion level (Supplementary Figure S6A). However, it was higher for S. bicolor under the combined and ammonical N forms, and S. canadensis had higher leaf greenness under the nitrate N form (Supplementary Figure S6A). Plants that adopt an N acquisition strategy for the ammonical N form have increased leaf chlorophyll content but excessive application of that form of N might cause chlorosis (Sarasketa et al., 2014; Sanchez-Zabala et al., 2015) because a higher amount of ammonical N degrades the chloroplasts by triggering the ABA signaling pathway (Li et al., 2012). Tomato leaves showed leaf chlorosis through bursting H2O2 under a medium supply of ammonical N (Liu and von Wirén, 2017).
Plants transform light energy into chemical energy through a process called photosynthesis that occurs in green leaves when they get sunshine. Based on Li et al. (2013), chloroplasts account for 20–30% of the weight of leaves and are the photosynthetic site. One pigment in chloroplasts that absorbs light energy and transforms it into biomass is called leaf chlorophyll (Du et al., 2017). The structural component of proteins, such as chloroplast, is the N. In a previous study, it was reported that the coexistence of N forms had the highest photosynthetic rate which is in line with our results (Figure 2F) and followed by nitrate and ammonical N forms (Li et al., 2013). However, nitrate N form was suppressive for photosynthetic rate in this study which might be due to the different carbon fixation pathways of S. bicolor (C4) and S. canadensis (C3), and C4 plants have higher photosynthetic rates (Smart et al., 2012; Wang et al., 2012; Nawaz et al., 2023).
4.3 Dominance and suppressive effect of S. bicolor on S. canadensis
The relative yield of any plant species shows its position in an ecosystem because it has a direct relationship with successful growth (Leger and Rice, 2003). A change in the behavior of S. canadensis due to nearby other plant species describes the invasion level (Conti et al., 2018). The degree of invasion and the evolution of a native species in an invaded ecosystem can be described by an understanding of the adaption mechanism of the invading species (Ren et al., 2022). Relative yield in this study was significantly correlated with plant species, interactions with intensities, N forms, and their combined interaction (Table 3). Similarly, S. bicolor showed a higher relative yield under the combined N form and low planting intensity. S. canadensis did not attain a relative yield equivalent to S. bicolor in the intercultures and under all N forms (Figure 4F). Thus, choosing the correct plant species is crucial in light of planting intensities and available N sources; these findings are consistent with those of Ren et al. (2022). Agronomic yield is the sum of grains and total dry biomass of plants. It is also calculated on acreage for fodder crops or to calculate an economic return. In this case, ideal plant intensity per acre ensures successful crop growth. Recommended planting intensity utilizes all resources judicially and increases the cost-benefit ratio (Anas et al., 2017). The planting of S. bicolor plants in a medium to high intensity may be able to control invasive species according to our testing of plant intensity against various invasion levels in this study. Similarly, de Witt (1960) reported that the yield is dependent on the area available in a mixed culture.
The entry of a non-native species into an ecosystem may change its functions and reshape it for successful invasion and be either partially or fully dominant. The relative dominance of S. bicolor based on the plant’s dry biomass was higher than S. canadensis for the combined available N forms under low planting intensities (Figure 4E). It has been reported that S. canadensis may co-exist with non-invasive plant species without changing the species richness, diversity, and composition of the recipient ecosystem (Lai et al., 2015). Usually, invasive plant species change the diversity, species richness, and structure of an ecosystem after entry into that particular ecosystem (Powell et al., 2013; Florens et al., 2017). By developing robust physiological systems both above and below ground, native plant species have the potential to outcompete invasive species. Allelopathy is an ecological strategy used by plants to outcompete neighboring plants by releasing allelochemicals into the air, scattering them on the soil’s surface, and secreting these chemicals from their roots (Farooq et al., 2013; Cheema and Khaliq, 2000; Shan et al., 2023). Plants with greater height and leaf area may cover the vegetation underneath which results in low light penetration and decreases the photosynthetic process (Angadi et al., 2022). Dense populations of plants hinder weed growth in an agroecosystem by stifling their ability to capture resources (Anas et al., 2017). Moreover, plant adaptations for available nitrogen forms may contribute to a native species’ capacity to thrive successfully due to priority (Fisher et al., 2010; Zhao et al., 2023).
5 Conclusion
The competitiveness of the domesticated plant S. bicolor against the invasive species S. canadensis was first documented in this study. It was discovered that S. bicolor outcompeted S. canadensis because of its greater biomass yield, CO2 uptake, leaf area, and plant height. Furthermore, the two plant species exhibited distinct behaviors in response to various N sources. S. bicolor performed better under the ammonical N form and combined N form (ammonical+nitrate), and the growth of S. canadensis was better under the nitrate N form. S. canadensis was suppressed by S. bicolor under all interculture combinations and the results were more pronounced at a higher plant intensity of S. bicolor under the ammonical N form.
Data availability statement
The original contributions presented in the study are included in the article/Supplementary Material. Further inquiries can be directed to the corresponding authors.
Author contributions
MA: Conceptualization, Methodology, Writing – original draft. IK: Methodology, Writing – review & editing. SOA: Software, Writing – review & editing. MN: Data curation, Software, Writing – review & editing. ZYH: Visualization, Writing – review & editing. MAA: Data curation, Validation, Writing – review & editing. KAG: Methodology, Writing – review & editing. SSQ: Supervision, Validation, Writing – review & editing. JL: Supervision, Validation, Writing – review & editing. ZCD: Funding acquisition, Project administration, Supervision, Writing – review & editing. DDL: Project administration, Validation, Writing – review & editing. SA: Writing – review & editing, Software, Conceptualization.
Funding
The author(s) declare financial support was received for the research, authorship, and/or publication of this article. This work was supported by National Natural Science Foundation of China (32271587, 32171509, 32071521), Natural Science Foundation of Jiangsu (BK20211321), the Carbon Peak and Carbon Neutrality Technology Innovation Foundation of Jiangsu Province (BK20220030), and Jiangsu Funding Program for Excellent Postdoctoral Talent (2023ZB861). Part of the funding for this research was supported by the Priority Academic Program Development of Jiangsu Higher Education Institutions (PAPD) and the Special Scientific Research Project of the School of Emergency Management, Jiangsu University.
Acknowledgments
The authors would like to acknowledge all the team members of the Institute of Environment and Ecology, School of the Environment and Safety Engineering, Jiangsu University, Zhenjiang 212013, China and the researchers at King Saud University in Riyadh, Saudi Arabia, who are sponsoring project number (RSP2024R48).
Conflict of interest
The authors declare that the research was conducted in the absence of any commercial or financial relationships that could be construed as a potential conflict of interest.
Publisher’s note
All claims expressed in this article are solely those of the authors and do not necessarily represent those of their affiliated organizations, or those of the publisher, the editors and the reviewers. Any product that may be evaluated in this article, or claim that may be made by its manufacturer, is not guaranteed or endorsed by the publisher.
Supplementary material
The Supplementary Material for this article can be found online at: https://www.frontiersin.org/articles/10.3389/fpls.2024.1468816/full#supplementary-material
References
Afzal, M. R., Naz, M., Ullah, R., Du, D. (2023). Persistence of Root Exudates of Sorghum bicolor and Solidago canadensis: Impacts on Invasive and Native Species. Plants 13, 58. doi: 10.3390/plants13010058
Afzal, M. R., Zhang, M., Jin, H., Wang, G., Zhang, M., Ding, M., et al. (2020). Post-translational regulation of plasma membrane H+-ATPase is involved in the release of biological nitrification inhibitors from sorghum roots. Plant Soil 450, 357–372. doi: 10.1007/s11104-020-04511-6
Allen, W. J., Bufford, J. L., Barnes, A. D., Barratt, B. I., Deslippe, J. R., Dickie, I. A., et al. (2022). A network perspective for sustainable agroecosystems. Trends Plant Sci. 27, 769–780. doi: 10.1016/j.tplants.2022.04.002
Amanullah, A., Stewart, B. A. (2013). Shoot: root differs in warm season C4-cereals when grown alone in pure and mixed stands under low and high water levels. Pak. J. Bot. 45, 83–90. Available at: http://www.pakbs.org/pjbot/PDFs/45(S1)/12.pdf (Accessed June 28, 2024)
Anas, M., Jabbar, A., Sarwar, M. A., Ullah, R., Abuzar, M. K., Ijaz, A., et al. (2017). Intercropping sunflower with mungbean for improved productivity and net economic return under irrigated conditions. Pak. J. Agr. Res. 30, 338–345. doi: 10.17582/journal.pjar/2017/30.4.338.345
Anas, M., Liao, F., Verma, K. K., Sarwar, M. A., Mahmood, A., Chen, Z.-L., et al. (2020). Fate of nitrogen in agriculture and environment: agronomic, eco-physiological and molecular approaches to improve nitrogen use efficiency. Biol. Res. 53, 1–20. doi: 10.1186/s40659-020-00312-4
Anas, M., Verma, K. K., Haq, I. U., Naeem, M., Li, Q., Liao, F., et al. (2021a). Characterization of exotic, native and wild-type genotypes of sugarcane (Saccharum spp. Hybrids) for internal nitrogen use efficiency under different nitrogen levels. Sugar Tech 23, 1258–1267. doi: 10.1007/s12355-021-01014-1
Anas, M., Verma, K. K., Riaz, M., Qiang, L., Liao, F., Liu, Y., et al. (2021b). Physio-morphological and biochemical mechanism of nitrogen use efficiency in sugarcane (Saccharum spp.) genotypes under different growth stages and nitrogen levels. J. Plant Interact. 16, 332–343. doi: 10.1080/17429145.2021.1933224
Anastasiu, P., Negrean, G. (2005). Alien plants in Romania (I). Analele Stiintifice ale Universitatii “Al. I. Cuza” din Iasi Tomul LI, s. II a. Biol. Veget. 51, 87–96. Available at: http://cercetare.bio.uaic.ro/publicatii/anale_vegetala/issue/2005/13-2005.pdf.
Angadi, S. V., Umesh, M. R., Begna, S., Gowda, P. (2022). Light interception, agronomic performance, and nutritive quality of annual forage legumes as affected by shade. Field Crops Res. 275, 108358. doi: 10.1016/j.fcr.2021.108358
Anten, N. P., Schieving, F., Medina, E., Werger, M. J. A., Schuffelen, P. (1995). Optimal leaf area indices in C3 and C4 mono-and dicotyledonous species at low and high nitrogen availability. Physiologia plantarum 95, 541–550. doi: 10.1111/j.1399-3054.1995.tb05520.x
Batish, D. R., Jose, S., Kaur, S., Chauhan, B. S. (2022). Reducing the susceptibility of agroecosystems to invasion through sustainable weed management. Front. Agron. 4. doi: 10.3389/fagro.2022.1086681
Bayu, W., Rethman, N. F. G., Hammes, P. S. (2005). Growth and yield compensation in sorghum (Sorghum bicolor L. Moench) as a function of planting density and nitrogen fertilizer in semi-arid areas of northeastern Ethiopia. South Afr. J. Plant Soil 22, 76–83. doi: 10.1080/02571862.2005.10634685
Besançon, T. E., Dayan, F. E., Gannon, T. W., Everman, W. J. (2020). Conservation and divergence in sorgoleone production of sorghum species. J. Environ. Qual. 49, 368–377. doi: 10.1002/jeq2.20038
Besançon, T., Heiniger, R., Weisz, R., Everman, W. (2017a). Weed response to agronomic practices and herbicide strategies in grain sorghum. Agron. J. 109, 1642–1650. doi: 10.2134/agronj2016.06.0363
Besançon, T. E., Heiniger, R. W., Weisz, R., Everman, W. J. (2017b). Grain sorghum and Palmer amaranth (Amaranthus palmeri) response to herbicide programs and agronomic practices. Weed Tech. 31, 781–792. doi: 10.1017/wet.2017.53
Burnside, O. C. (1977). Control of weeds in non-cultivated, narrow-row sorghum 1. Agron. J. 69, 851–854. doi: 10.2134/agronj1977.00021962006900050031x
Calone, R., Sanoubar, R., Lambertini, C., Speranza, M., Vittori Antisari, L., Vianello, G., et al. (2020). Salt tolerance and Na allocation in Sorghum bicolor under variable soil and water salinity. Plants 9, 561. doi: 10.3390/plants9050561
Cheema, Z. A., Khaliq, A. (2000). Use of sorghum allelopathic properties to control weeds in irrigated wheat in a semi arid region of Punjab. Agric. Eco. Env. 79, 105–112. doi: 10.1016/S0167-8809(99)00140-1
Chen, X., Wu, Q., Gao, Y., Zhang, J., Wang, Y., Zhang, R., et al. (2020). The role of deep roots in sorghum yield production under drought conditions. Agronomy 10, 611. doi: 10.3390/agronomy10040611
Conti, L., Block, S., Parepa, M., Münkemüller, T., Thuiller, W., Acosta, A. T., et al. (2018). Functional trait differences and trait plasticity mediate biotic resistance to potential plant invaders. J. Ecol. 106, 1607–1620. doi: 10.1111/1365-2745.12928
Contreras, D., Leon, R. G., Post, A. R., Everman, W. J. (2022). Critical period of grass weed control in ALS-tolerant grain sorghum (Sorghum bicolor) is affected by planting date and environment. Front. Agron. 4. doi: 10.3389/fagro.2022.1014801
Cox, S., Nabukalu, P., Paterson, A. H., Kong, W., Nakasagga, S. (2018). Development of perennial grain sorghum. Sustainability 10, 172. doi: 10.3390/su10010172
Crawford, N. M., Glass, A. D. (1998). Molecular and physiological aspects of nitrate uptake in plants. Trends Plant Sci. 3, 389–395. doi: 10.1016/S1360-1385(98)01311-9
Cui, M., Yang, B., Ren, G., Yu, H., Dai, Z., Li, J., et al. (2023). Effects of Warming, Phosphorous Deposition, and Both Treatments on the Growth and Physiology of Invasive Solidago canadensis and Native Artemisia argyi. Plants 12, 1370. doi: 10.3390/plants12061370
Danalatos, N., Archontoulis, S. V., Tsiboukas, K. (2009). “Comparative analysis of sorghum vs corn growing under optimum and under water/nitrogen limited conditions in central Greece,” in 17th European Biomass Conference and Exhibition, Vol. 2. 538–544. Available at: https://www.researchgate.net/publication/235797946.
Davarzani, M., Aliniaeifard, S., Mehrjerdi, M. Z., Roozban, M. R., Saeedi, S. A., Gruda, N. S. (2023). Optimizing supplemental light spectrum improves growth and yield of cut roses. Sci. Rep. 13, 21381. doi: 10.1038/s41598-023-48266-3
de Oliveira, F. D. B., de Souza Miranda, R., dos Santos Araújo, G., Coelho, D. G., Lobo, M. D. P., de Oliveira Paula-Marinho, S., et al. (2020). New insights into molecular targets of salt tolerance in sorghum leaves elicited by ammonium nutrition. Plant Physiol. Biochem. 154, 723–734. doi: 10.1016/j.plaphy.2020.06.051
de Souza Miranda, R., Gomes-Filho, E., Prisco, J. T., Alvarez-Pizarro, J. C. (2016). Ammonium improves tolerance to salinity stress in Sorghum bicolor plants. Plant Growth Regul. 78, 121–131. doi: 10.1007/s10725-015-0079-1
de Witt, C. T. (1960). On competition. Verslagen Van Landouskundige Onderzoekingen 66, 1–82. Available at: https://edepot.wur.nl/187113.
Di, T., Afzal, M. R., Yoshihashi, T., Deshpande, S., Zhu, Y., Subbarao, G. V. (2018). Further insights into underlying mechanisms for the release of biological nitrification inhibitors from sorghum roots. Plant Soil. 423, 99–110. doi: 10.1007/s11104-017-3505-5
Du, E., Dong, D., Zeng, X., Sun, Z., Jiang, X., de Vries, W. (2017). Direct effect of acid rain on leaf chlorophyll content of terrestrial plants in China. Sci. Total Environ. 605, 764–769. doi: 10.1016/j.scitotenv.2017.06.044
Du, E., Terrer, C., Pellegrini, A. F., Ahlström, A., van Lissa, C. J., Zhao, X., et al. (2020). Global patterns of terrestrial nitrogen and phosphorus limitation. Nat. Geosci. 13, 221–226. doi: 10.1038/s41561-019-0530-4
Einhellig, F. A., Souza, I. F. (1992). Phytotoxicity of sorgoleone found in grain sorghum root exudates. J. Chem. Ecol. 18, 1–11. doi: 10.1007/BF00997160
Farooq, M., Khaliq, A., Cheema, Z., Cheema, S. (2013). Application of allelopathy in crop production: success story from Pakistan. Allelopath. Curr. Trends Futur. Appl., 113–143. doi: 10.1007/978-3-642-30595-5_6
Farooq, M., Khan, I., Nawaz, A., Cheema, M. A., Siddique, K. H. (2020). Using sorghum to suppress weeds in autumn planted maize. Crop Prot. 133, 105162. doi: 10.1016/j.cropro.2020.105162
Fay, P. A., Hui, D., Procter, A., Johnson, H. B., Polley, H. W., Jackson, R. B. (2006). “December. Photosynthetic Water Use Efficiency in it Sorghastrum nutans (C4) and it Solidago canadensis (C3) in Three Soils Along a CO2 Concentration Gradient,” in AGU Fall Meeting Abstracts, Vol. 2006. B41B–0187. Available at: https://ui.adsabs.harvard.edu/abs/2006AGUFM.B41B0187F/abstract.
Fisher, J. B., Sitch, S., Malhi, Y., Fisher, R. A., Huntingford, C., Tan, S.-Y. (2010). Carbon cost of plant nitrogen acquisition: A mechanistic, globally applicable model of plant nitrogen uptake, retranslocation, and fixation. Global Biogeochem Cycles 24, GB1014. doi: 10.1029/2009GB003621
Florens, F. V., Baider, C., Seegoolam, N. B., Zmanay, Z., Strasberg, D. (2017). Long-term declines of native trees in an oceanic island's tropical forests invaded by alien plants. Appl. Veg. Sci. 20, 94–105. doi: 10.1111/avsc.12273
Frost, C. M., Allen, W. J., Courchamp, F., Jeschke, J. M., Saul, W. C., Wardle, D. A. (2019). Using network theory to understand and predict biological invasions. Trends Ecol. Evol. 34, 831–843. doi: 10.1016/j.tree.2019.04.012
Gazoulis, I., Antonopoulos, N., Kanatas, P., Karavas, N., Bertoncelj, I., Travlos, I. (2022). Invasive alien plant species—Raising awareness of a threat to biodiversity and ecological connectivity (EC) in the adriatic-ionian region. Diversity 14, 387. doi: 10.3390/d14050387
Gholami, S., Minbashi, M., Zand, E., Noormohammadi, G. (2013). Non chemical management of weeds effects on forage sorghum production. Int. J. Adv. Biol. Biomed. Res. 1, 614–623. doi: 10.26655/ijabbr.2017.9.6
Głąb, L., Sowiński, J., Bough, R., Dayan, F. E. (2017). Allelopathic potential of sorghum (Sorghum bicolor (L.) Moench) in weed control: a comprehensive review. Adv. Agron. 145, 43–95. doi: 10.1016/bs.agron.2017.05.001
Gonzalez, V. M., Kazimir, J., Nimbal, C., Weston, L. A., Cheniae, G. M. (1997). Inhibition of a photosystem II electron transfer reaction by the natural product sorgoleone. J. Agric. Food Chem. 45, 1415–1421. doi: 10.1021/jf960733w
Guan, M., Pan, X.-C., Sun, J.-K., Chen, J.-X., Kong, D.-L., Feng, Y.-L. (2023). Nitrogen acquisition strategy and its effects on invasiveness of a subtropical invasive plant. Front. Plant Sci. 14. doi: 10.3389/fpls.2023.1243849
Guo, X., Ma, J.-Y., Liu, L.-L., Li, M.-Y., Wang, H., Sun, Y.-K., et al. (2023). Effects of salt stress on interspecific competition between an invasive alien plant Oenothera biennis and three native species. Front. Plant Sci. 14. doi: 10.3389/fpls.2023.1144511
Hess, L., De Kroon, H. (2007). Effects of rooting volume and nutrient availability as an alternative explanation for root self/non-self discrimination. J. Ecol. 95, 241–251. doi: 10.1111/j.1365-2745.2006.01204.x
Hodge, A. (2005). “Nitrogen in soil/plant uptake,” in Encyclopedia of Soils in the Environment, Elsevier, Radarweg 29, 1043 NX Amsterdam, The Netherlands. 00159–00154. doi: 10.1016/B0-12-348530-4/00159-4
Huang, P., Shen, F., Abbas, A., Wang, H., Du, Y., Du, D., et al. (2022). Effects of different nitrogen forms and competitive treatments on the growth and antioxidant system of Wedelia trilobata and Wedelia chinensis under high nitrogen concentrations. Front. Plant Sci. 13. doi: 10.3389/fpls.2022.851099
Huang, X., Yu, J., Liu, S., Xie, H., He, H., Li, K. (2021). Plant morphological traits and competition index comparisons of three invasive and native submerged plants. Knowledge Manage. Aquat. Ecosyst. 422, 11. doi: 10.1051/kmae/2021012
Jabran, K., Farooq, M. (2012). “Implications of potential allelopathic crops in agricultural systems,” in Allelopathy: Current trends and future applications (Springer Berlin Heidelberg, Berlin, Heidelberg), 349–385. doi: 10.1007/978-3-642-30595-5_15
Jiang, Z., Zhao, C., Yu, S., Liu, S., Cui, L., Wu, Y., et al. (2019). Contrasting root length, nutrient content and carbon sequestration of seagrass growing in offshore carbonate and onshore terrigenous sediments in the South China Sea. Sci. Total Environ. 662, 151–159. doi: 10.1016/j.scitotenv.2019.01.175
Kato-Noguchi, H. (2023). The Impact and Invasive Mechanisms of Pueraria montana var. lobata, One of the World’s Worst Alien Species. Plants 12, 3066. doi: 10.3390/plants12173066
Kelly, C. L., Schwarzkopf, L., Gordon, I. J., Hirsch, B. (2021). Population growth lags in introduced species. Ecol. Evol. 11, 4577–4587. doi: 10.1002/ece3.7352
Khan, I. U., Qi, S. S., Gul, F., Manan, S., Rono, J. K., Naz, M., et al. (2023a). A green approach used for heavy metals ‘Phytoremediation’ Via invasive plant species to mitigate environmental pollution: A review. Plants 12, 725. doi: 10.3390/plants12040725
Khan, I. U., Zhang, Y. F., Shi, X. N., Qi, S. S., Zhang, H. Y., Du, D. L., et al. (2023b). Dose dependent effect of nitrogen on the phyto extractability of Cd in metal contaminated soil using Wedelia trilobata. Ecotoxicol Environ. Saf. 264, 115419. doi: 10.1016/j.ecoenv.2023.115419
Lai, H. R., Mayfield, M. M., Gay-des-combes, J. M., Spiegelberger, T., Dwyer, J. M. (2015). Distinct invasion strategies operating within a natural annual plant system. Ecol. Lett. 18, 336–346. doi: 10.1111/ele.12414
Leger, E. A., Rice, K. J. (2003). Invasive California poppies (Eschscholzia californica Cham.) grow larger than native individuals under reduced competition. Ecol. Lett. 6, 257–264. doi: 10.1046/j.1461-0248.2003.00423.x
Li, B., Li, Q., Xiong, L., Kronzucker, H. J., Krämer, U., Shi, W. (2012). Arabidopsis plastid AMOS1/EGY1 integrates abscisic acid signaling to regulate global gene expression response to ammonium stress. Plant Physiol. 160, 2040–2051. doi: 10.1104/pp.112.206508
Li, S. X., Wang, Z. H., Stewart, B. (2013). Responses of crop plants to ammonium and nitrate N. Adv. Agron. 118, 205–397. doi: 10.1016/B978-0-12-405942-9.00005-0
Liu, D., Chen, L., Chen, C., Zhou, Y., Xiao, F., Wang, Y., et al. (2022). Effect of plant VOCs and light intensity on growth and reproduction performance of an invasive and a native Phytolacca species in China. Ecol. Evol. 12, e8522. doi: 10.1002/ece3.8522
Liu, P. C., Peacock, W. J., Wang, L., Furbank, R., Larkum, A., Dennis, E. S. (2020). Leaf growth in early development is key to biomass heterosis in Arabidopsis. J. Exp. Bot. 71, 2439–2450. doi: 10.1093/jxb/eraa006
Liu, Y., von Wirén, N. (2017). Ammonium as a signal for physiological and morphological responses in plants. J. Exp. Bot. 68, 2581–2592. doi: 10.1093/jxb/erx086
Meng, T. T., Wang, H., Harrison, S. P., Prentice, I. C., Ni, J., Wang, G. (2015). Responses of leaf traits to climatic gradients: adaptive variation versus compositional shifts. Biogeosci. 12, 5339–5352. doi: 10.5194/bg-12-5339-2015
Miranda, R. D. S., Alvarez-Pizarro, J. C., Araújo, C. M. S., Prisco, J. T., Gomes-Filho, E. (2013). Influence of inorganic nitrogen sources on K+/Na+ homeostasis and salt tolerance in sorghum plants. Acta Physiologiae Plantarum 35, 841–852. doi: 10.1007/s11738-012-1128-2
Mitryasova, O., Koszelnic, P. (2021). Climate Change & Sustainable Development: New Challenges of the Century. Available online at: https://dspace.chmnu.edu.ua/jspui/handle/123456789/1120. (Accessed June 30, 2024).
Mohd Ghazi, R., Nik Yusoff, N. R., Abdul Halim, N. S., Wahab, I. R., Ab Latif, N., Hasmoni, S. H., et al. (2023). Health effects of herbicides and its current removal strategies. Bioengineered 14, 2259526. doi: 10.1080/21655979.2023.2259526
Mrid, R. B., Omari, R. E., Nhiri, M. (2016). Effect of nitrogen source and concentration on growth and activity of nitrogen assimilation enzymes in roots of a moroccan sorghum ecotype. Plant 4, 71. doi: 10.11648/j.plant.20160406.14
Narwal, S., Haouala, R. (2013). Role of allelopathy in weed management for sustainable agriculture. Allelopathy. Curr. Trends Future Appl., 217–249. doi: 10.1007/978-3-642-30595-5
Nawaz, M., Sun, J., Shabbir, S., Khattak, W. A., Ren, G., Nie, X., et al. (2023). A review of plants strategies to resist biotic and abiotic environmental stressors. Sci. Total Environ., 165832. doi: 10.1016/j.scitotenv.2023.165832
Oswald, A., Ransom, J. K., Kroschel, J., Sauerborn, J. (2001). Transplanting maize and sorghum reduces Striga hermonthica damage. Weed Sci. 49, 346–353. Available at: http://www.jstor.org/stable/4046316.
Pan, L., He, F., Liang, Q., Bo, Y., Lin, X., Javed, Q., et al. (2023). Allelopathic Effects of Caffeic Acid and Its Derivatives on Seed Germination and Growth Competitiveness of Native Plants (Lantana indica) and Invasive Plants (Solidago canadensis). Agric. 13, 1719. doi: 10.3390/agriculture13091719
Powell, K. I., Chase, J. M., Knight, T. M. (2013). Invasive plants have scale-dependent effects on diversity by altering species-area relationships. Science 339, 316–318. doi: 10.1126/science.1226817
Qi, S., Wang, J., Wan, L., Dai, Z., da Silva Matos, D. M., Du, D., et al. (2022). Arbuscular mycorrhizal fungi contribute to phosphorous uptake and allocation strategies of Solidago canadensis in a phosphorous-deficient environment. Front. Plant Sci. 13. doi: 10.3389/fpls.2022.831654
Ren, G.-Q., Li, Q., Li, Y., Li, J., Adomako, M. O., Dai, Z.-C., et al. (2019). The enhancement of root biomass increases the competitiveness of an invasive plant against a co-occurring native plant under elevated nitrogen deposition. Flora 261, 151486. doi: 10.1016/j.flora.2019.151486
Ren, G., Yang, B., Cui, M., Yu, H., Fan, X., Dai, Z., et al. (2022). Additive effects of warming and nitrogen addition on the performance and competitiveness of invasive Solidago canadensis L. Front. Plant Sci. 13. doi: 10.3389/fpls.2022.1017554
Roth, C. M., Shroyer, J. P., Paulsen, G. M. (2000). Allelopathy of sorghum on wheat under several tillage systems. Agron. J. 92, 855–860. doi: 10.2134/agronj2000.925855x
Sanchez-Zabala, J., González-Murua, C., Marino, D. (2015). Mild ammonium stress increases chlorophyll content in Arabidopsis thaliana. Plant Signal Behav. 10, e991596. doi: 10.4161/15592324.2014.991596
Sarasketa, A., González-Moro, M. B., González-Murua, C., Marino, D. (2014). Exploring ammonium tolerance in a large panel of Arabidopsis thaliana natural accessions. J. Exp. Bot. 65, 6023–6033. doi: 10.1093/jxb/eru342
Shan, Z., Zhou, S., Shah, A., Arafat, Y., Rizvi, S. A. H., Shao, H. (2023). Plant allelopathy in response to biotic and abiotic factors. Agronomy 13, 2358. doi: 10.3390/agronomy13092358
Shen, G., Song, Z., Xu, J., Zou, L., Huang, L., Li, Y. (2023). Are ecosystem services provided by street trees at parcel level worthy of attention? A case study of a campus in Zhenjiang, China. Int. J. Environ. Res. Public Health 20, 880. doi: 10.3390/ijerph20010880
Smart, A. J., Larson, G. E., Bauman, P. J. (2012). Grass and Canada goldenrod (Solidago canadensis) competition and implications for management in the northern tallgrass Prairie. Prairie Nat. 45, 4–12. Available at: https://digitalcommons.unl.edu/cgi/viewcontent.cgi?article=1125&context=tpn.
Smil, V. (1999). Nitrogen in crop production: An account of global flows. Global biogeochemical cycles 13, 647–662. doi: 10.1029/1999GB900015
Szabó, A. K., Várallyay, É., Demian, E., Hegyi, A., Galbács, Z. N., Kiss, J., et al. (2020). Local aphid species infestation on invasive weeds affects virus infection of nearest crops under different management systems–a preliminary study. Front. Plant Sci. 11. doi: 10.3389/fpls.2020.00684
Tian, Z., Cheng, J., Xu, J., Feng, D., Zhong, J., Yuan, X., et al. (2023). Cytogeography of naturalized Solidago canadensis populations in Europe. Plants 12, 1113. doi: 10.3390/plants12051113
Valone, T. J., Weyers, D. P. (2019). Invasion intensity influences scale-dependent effects of an exotic species on native plant diversity. Sci. Rep. 9, 18769. doi: 10.1038/s41598-019-55165-z
Venkateswaran, K., Elangovan, M., Sivaraj, N. (2019). “Origin, domestication and diffusion of Sorghum bicolor,” in Breeding Sorghum for diverse end uses (Radarweg 29, 1043 NX Amsterdam, The Netherlands: Elsevier), 15–31. doi: 10.1016/B978-0-08-101879-8.00002-4
Wang, C., Cheng, H., Wang, S., Wei, M., Du, D. (2021). Plant community and the influence of plant taxonomic diversity on community stability and invasibility: A case study based on Solidago canadensis L. Sci. Total Environ. 768, 144518. doi: 10.1016/j.scitotenv.2020.144518
Wang, S., Cheng, H. Y., Wei, M., Wu, B. D., Wang, C. Y. (2020). Litter decomposition process dramatically declines the allelopathy of Solidago canadensis L. on the seed germination and seedling growth of Lactuca sativa L. Int. J. Phytoremed. 22, 1295–1303. doi: 10.1080/15226514.2020.1765140
Wang, C., Guo, L., Li, Y., Wang, Z. (2012). Systematic comparison of C3 and C4 plants based on metabolic network analysis. BMC Syst. Biol. 6, 1–14. doi: 10.1186/1752-0509-6-S2-S9
Wang, R., Wan, F., Li, B. (2017). Roles of Chinese government on prevention and management of invasive alien species. Biol. Invasions Its Manage. China 1, 149–156. doi: 10.1007/978-94-024-0948-2_7
Wang, W., Zhu, Q., Dai, S., Meng, L., He, M., Chen, S., et al. (2023). Effects of Solidago canadensis L. @ on mineralization-immobilization turnover enhance its nitrogen competitiveness and invasiveness. Sci. Total Environ. 882, 163641. doi: 10.1016/j.scitotenv.2023.163641
Weidenhamer, J. D. (2005). Biomimetic measurement of allelochemical dynamics in the rhizosphere. J. Chem. Ecol. 31, 221–236. doi: 10.1007/s10886-005-1337-x
Werner, P. A., GROSS, R. S., BRADBURY, I. K. (1980). The biology of canadian weeds.: 45. Solidago canadensis L. Can. J. Plant Sci. 60, 1393–1409. doi: 10.4141/cjps80-194
Yan, P., Song, Y. H., Zhang, K. Y., Zhang, F., Tang, Y. J., Zhao, X. N., et al. (2023). Interaction of genotype-ecological type-plant spacing configuration in sorghum [Sorghum bicolor (L.) Moench] in China. Front. Plant Sci. 13. doi: 10.3389/fpls.2022.1076854
Yau, S. K., Hamblin, J. (1994). Relative yield as a measure of entry performance in variable environments. Crop Sci. 34, 813–817. doi: 10.2135/cropsci1994.0011183X003400030038x
Ying, L., Maohua, M., Zhi, D., Bo, L., Ming, J., Xianguo, L., et al. (2023). Light–acquisition traits link aboveground biomass and environment in inner saline–alkaline herbaceous marshes. Sci. Total Environ. 857, 159660. doi: 10.1016/j.scitotenv.2022.159660
Young, K. J., Long, S. P. (2000). Crop ecosystem responses to climatic change: maize and sorghum. Climate Change Global Crop productivity, 107–131. doi: 10.1079/9780851994390.0107
Yu, Y., Cheng, H., Xu, Z., Zhong, S., Wang, C., Guo, E. (2022). Invasion intensity modulates the allelopathic impact of Solidago canadensis L. leaves and roots against Lactuca sativa L. during germination and early seedling stage. Internat. J. Environ. Res. 16, 48. doi: 10.1007/s41742-022-00428-3
Zeng, H., Di, T., Zhu, Y., Subbarao, G. V. (2016). Transcriptional response of plasma membrane H+-ATPase genes to ammonium nutrition and its functional link to the release of biological nitrification inhibitors from sorghum roots. Plant Soil 398, 301–312. doi: 10.1007/s11104-015-2675-2
Zhang, R., Yue, Z., Chen, X., Huang, R., Zhou, Y., Cao, X. (2023). Effects of waterlogging at different growth stages on the photosynthetic characteristics and grain yield of sorghum (Sorghum bicolor L.). Sci. Rep. 13, 7212. doi: 10.1038/s41598-023-32478-8
Zhao, D., Reddy, K. R., Kakani, V. G., Reddy, V. R. (2005). Nitrogen deficiency effects on plant growth, leaf photosynthesis, and hyperspectral reflectance properties of sorghum. Europ. J. Agron. 22, 391–403. doi: 10.1016/j.eja.2004.06.005
Zhao, Q., Wang, P., Smith, G. R., Hu, L., Liu, X., Tao, T., et al. (2023). Nitrogen redistribution and seasonal trait fluctuation facilitate plant N conservation and ecosystem N retention. J. Ecol. 00, 1–13. doi: 10.1111/1365-2745.14246
Keywords: ecosystem, S. bicolor, S. canadensis, nitrogen, invasion
Citation: Anas M, Khan IU, Alomrani SO, Nawaz M, Huang Z-Y, Alshehri MA, Al-Ghanim KA, Qi S-S, Li J, Dai Z-C, Ali S and Du D-L (2024) Evaluating Sorghum bicolor resistance to Solidago canadensis invasion under different nitrogen scenarios. Front. Plant Sci. 15:1468816. doi: 10.3389/fpls.2024.1468816
Received: 22 July 2024; Accepted: 30 September 2024;
Published: 28 October 2024.
Edited by:
Xiang Liu, Lanzhou University, ChinaReviewed by:
Yuping Hou, Ludong University, ChinaMing-Chao Liu, Shenyang Agricultural University, China
Copyright © 2024 Anas, Khan, Alomrani, Nawaz, Huang, Alshehri, Al-Ghanim, Qi, Li, Dai, Ali and Du. This is an open-access article distributed under the terms of the Creative Commons Attribution License (CC BY). The use, distribution or reproduction in other forums is permitted, provided the original author(s) and the copyright owner(s) are credited and that the original publication in this journal is cited, in accordance with accepted academic practice. No use, distribution or reproduction is permitted which does not comply with these terms.
*Correspondence: Zhi-Cong Dai, ZGFpemhpY29uZ0AxNjMuY29t; Shan-Shan Qi, cWlzaGFuc2hhbjE5ODYxMjBAMTYzLmNvbQ==; Shafaqat Ali, c2hhZmFxYXRhbGlnaWxsQHlhaG9vLmNvbQ==; Dao-Lin Du, ZGRsQHVqcy5lZHUuY24=