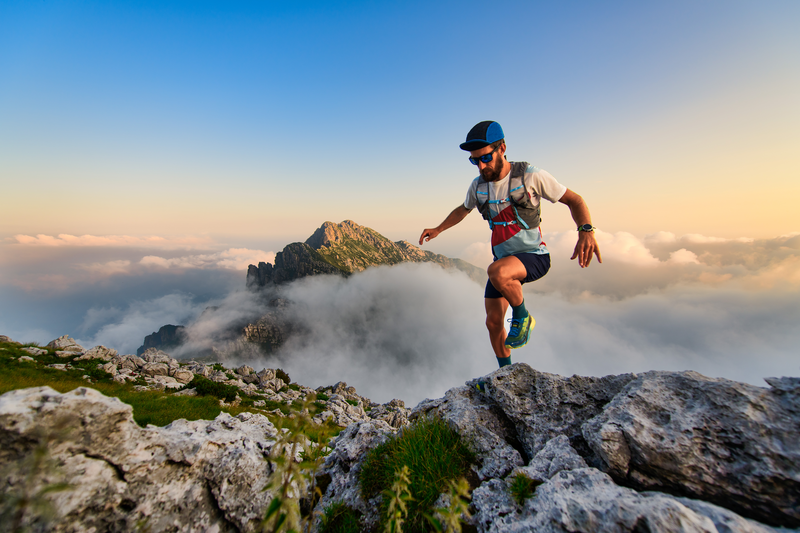
95% of researchers rate our articles as excellent or good
Learn more about the work of our research integrity team to safeguard the quality of each article we publish.
Find out more
ORIGINAL RESEARCH article
Front. Plant Sci. , 26 November 2024
Sec. Plant Abiotic Stress
Volume 15 - 2024 | https://doi.org/10.3389/fpls.2024.1468636
This article is part of the Research Topic Insights on Agricultural Modulators for Mitigating Water Stress in Cultivated Plants View all 6 articles
Drought is the one primary issue limiting peanut growth and productivity. The study aimed to investigate the effects of arbuscular mycorrhizal fungi (AMF), rhizobium (Rhi), and their combinations on phenolic content, proline content, growth, and yield of peanut under different soil water regimes. The pot experiments were carried out for two growing seasons under greenhouse conditions and designed based on a 2×3 factorial in randomized complete block design (RCBD) with four replications. Factor A comprised two soil water regimes: field capacity (FC) and 1/3 available soil water (1/3 AW), whereas factor B included three different types of microorganisms: (i) uninoculated control, (ii) arbuscular mycorrhiza (AMF), and (iii) a combination of AMF and rhizobium (Rhi) inoculations. Data were collected for growth, proline content, phenolic content, yield, and yield components. Drought stress significantly reduced in relative water content, leaf area, biomass, yield, and yield components of peanut, whereas leaf phenolic content was increased under drought stress. Higher pod dry weight was achieved under FC conditions (28.87 g plant-1), and it was reduced to 16.06 g plant-1 under 1/3 FC. Interestingly, AMF+Rhi synergistically increased the leaf area compared with non-incubated peanut under 1/3 FC conditions. AMF-inoculated peanut tended to increase biomass, while the combination of AMF+Rhi tended to have higher yield components compared with uninoculated control, especially for the weight of 100 seeds.
Peanut is mainly grown in tropics and semiarid tropics where drought is a major problem because of unpredictable rainfall and rain distribution (Rachaputia et al., 2021). Crop growth and productivity can be severely affected by drought, which is one of the most important abiotic stresses (Fathi and Tari, 2016). The peanut is typically grown in two main seasons: rainy season and dry season (Sukharomana and Dobkuntod, 2003).
In Thailand, peanut is grown under three production systems, namely, intercropping of peanut with other tree crops and field crops, growing as a major crop in the wet season, and growing after harvest of field crops in the late rainy season without irrigation or in the dry season with irrigation (Sukharomana and Dobkuntod, 2003). Peanut planted in the late rainy season without irrigation and in the dry season with irrigation can experience drought stress because of depletion of stored soil water and insufficient of irrigation water. As a major crop, peanut can be affected by drought due to unpredictable rainfall and distribution. Mid-season drought (Junjittakarn et al., 2016) and terminal drought (Girdthai et al., 2010; Koolachart et al., 2013; Aninbon et al., 2019) are particularly detrimental to pod yield of peanut because the drought events occur during reproductive phases.
Symbiotic nitrogen fixation of leguminous species by association with rhizobium is adopted widely in agriculture to reduce the excessive use of chemical nitrogen fertilizer (Abd-Alla et al., 2023). Peanut with rhizobium inoculation fixed 27.19 kg N ha−1. The Rhizobia inoculation increased the amount of nitrogen fixation up to 46% in North Eastern Nigeria (Yakubu et al., 2010), 42%–51% in Petrolina, and 43%–60% in Sergipe, Brazil (Jovino et al., 2022). According to Toomsan et al. (1995), peanut fixed nitrogen in the range of 150 kg N ha−1–200 kg N ha−1. When the stover was returned to the soil, the net contributions of N from N fixation ranged from 13 kg N ha−1–100 kg N ha−1. Therefore, peanut contributes to soil improvement, when plant parts of the peanut are added to the soil after harvesting.
The rhizobium directly supports the host plant by nitrogen fixation process including increased solubilization of nutrients via organic acid and siderophore production, and hormone production such as IAA and gibberellin. The Rhizobium also indirectly support the plant by production of antibiotics, catalase, and siderophores that control root pathogens (Jaiswal et al., 2021). The inoculation of Rhizobium isolated from cowpea increased the grain yield of peanut IAC505 (Guimaraes et al., 2019). Rhizobium application with 100% nitrogen recommended dose significantly improved peanut yield, yield-related traits, seed quality, and maximum uptake of major nutrients (nitrogen, phosphorus, and potassium) (Mondal et al., 2020). Moreover, Rhizobium leguminosarum could help to reduce drought effects in the two faba bean genotypes (Amine-Khodja et al., 2022).
Arbuscular mycorrhizal fungi (AMF) are now recognized as beneficial microorganisms that enhance plant growth and also reduce drought conditions by producing and extending the hyphae into dry soil and increasing surface contact for improved water absorption by host plants. Additionally, AMF enhance nutrient uptake and morphological adaptations and also influence physiological processes of host plants (Cheng et al., 2021). AMF-inoculated soybeans increase the relative water content (RWC) and photosynthetic rate compared with uninoculated plants, leading to higher dry weight of plants and seeds (Aliasgharzad et al., 2006; Oliveira et al., 2022). Inoculation peanut with AMF also enhances catalase and peroxidase activities in seeds (Sheteiwy et al., 2021). Similarly, inoculation of chickpea with AMF under drought conditions also improves the RWC, stomatal conductance, and photosynthetic rate compared with uninoculated plants (Hashem et al., 2018). Proline can help water absorption from the soil through the osmotic adjustment under drought conditions. Phenols also play an important role in regulating developmental mechanisms and tolerance to various stresses including drought stress. In addition, proline and phenolic contents in wheat also increase under drought stress, and this increase is more prominent in non-AMF inoculated plants than in AMF-inoculated plants. However, phenolic compounds do not show a significant difference between the inoculation methods of AMF.
Most of the studies so far have focused on individual inoculation of AMF and rhizobium (Rhi) on peanut crop, and information on co-inoculation of AMF and rhizobium is still limited. A recent greenhouse study indicated that combined inoculation of AMF and rhizobium on soybean favored the growth response in association with higher P and N uptake (Dobo, 2022). Similar results were also reported in Phaseolus vulgaris planted in low-fertility tropical soil (Razakatiana et al., 2020). It is a challenge to explore the co-inoculation of AMF and rhizobium on peanut, particularly under drought conditions. The hypothesis of this study was that application of AMF and/or co-inoculation of AMF and rhizobium would improve peanut proline content, phenolic content, growth, and yield under drought conditions. The aim of this study was to evaluate the effects of AMF, rhizobium (Rhi), and their combinations on phenolic content, proline content, growth, and yield of peanut under two different soil water regimes. The results of this study will provide information on the use of AMF and rhizobium for improving growth and yield of peanut under drought stress.
A pot experiment was conducted in a greenhouse at the faculty of Agricultural Technology, King Mongkut’s Institute of Technology Ladkrabang (13.7299° N, 100.7782° E, 0.5 m above sea level) for two growing seasons such as dry season (February–May 2023) and rainy season (August–November 2023). A 2×3 factorial experiment was arranged in a randomized complete block design (RCBD) with four replications. Six pots were used for each replication as subunits. Factor A comprised two different soil water regimes: field capacity (FC) and 1/3 available soil water (1/3 AW). Factor B included three inoculations of arbuscular mycorrhiza (AMF): (i) uninoculated control, (ii) inoculation with AMF, and (iii) a combination of AMF and rhizobium (Rhi). A peanut variety, KKU9, which is a newly released variety in Thailand and is recognized by its high pod yield and good plant architecture, was used in this study. The soil used in this study was Chakraja soil series, which is characterized as loamy sand. The chemical and physical properties of the soil are described in Table 1. The field capacity of the soil (FC) was 11.24%, and the permanent wilting point (PWP) was 5.27%.
The soil was air-dried and sieved through a 4-mm sieve to remove debris and homogenize the soil sample. Thirty six kg of the dry soil was evenly divided and filled into pots in two soil layers in each pot to ensure a uniform bulk density (1.56 g cm−3). Water was supplied to all the pots to obtain the soil moisture content at the field capacity level (11.24%).
The Rhi and AMF inoculants were commercial biofertilizers (Rhizobium: Bradyrhizobium sp. with 106 CFU g−1 and AMF: Glomus spp. with 25 spore g−1). The soil inoculation was done by putting the inoculum in a hole dug in each pot. The amount of inoculum, 10 g of AMF for AMF-alone treatment, and 10 g each of AMF and Rhizobium for combination were used. Healthy peanut seedlings at 15 days old were transplanted to the center of each pot (1 plant pot−1), and water was immediately applied to ensure good establishment.
Manual weeding was done whenever necessary, and compound fertilizer of N-P2O5-K2O (15-15-15) at the rate of 156.25 kg ha−1 was applied at 20 days after transplanting (DAT).
Water application was done from transplanting until 60 DAT to maintain the soil moisture content at field capacity for both well-watered and 1/3 AW treatments. After 60 DAT, field capacity treatment, water was maintained at field capacity level until harvest. In the 1/3 AW treatment, irrigation was stopped at 60 DAT, and the moisture content was allowed to decrease gradually to meet the level of 1/3 AW (7.26%), which was subsequently maintained until harvest. The amount of water applied to each pot was calculated according to crop water requirement described by Doorenbos and Pruitt (1992) and surface evaporation by Singh and Russel (1981).
Before planting, soil samples were randomly collected from 10 single samples, bulked for two samples, and examined for physicochemical properties, including soil texture (percentages of sand, silt, and clay), pH, organic matter (%), total nitrogen (N), available phosphorus (P), exchangeable potassium (K), exchangeable calcium (Ca), exchangeable magnesium (Mg), exchangeable manganese (Mn), sodium (Na), extractable iron (Fe), extractable copper (Cu), and extractable zinc (Zn) content, electrical conductivity (EC), and cation exchange capacity (CEC).
Soil moisture content (15 cm depth) was measured by the gravimetric method at 60, 75 and 90 DAT in both seasons. Briefly, soil samples were taken from each pot using a soil sampler throughout the entire column, and the soil wet weight was recorded. The soil samples were then oven-dried at 105°C for 72 h or until a constant weight was achieved, and the moisture percentage was calculated.
Relative water contents of three leaflets were measured from the second fully expanded leaf between 10:00 am and 2:00 pm at 60, 75 DAT, and harvest. The leaflets were cut, immediately placed in a plastic zip bag, and stored in an ice box to prevent water loss. The leaf samples were weighted once the samples were transported to the laboratory. Then, they were placed in distilled water for 8 h, and then the turgid weight was determined. Subsequently, the leaflets were oven-dried at 80°C for 48 h or until the weight remained constant, and the leaf dry weight was recorded. Relative water content was calculated using the formula suggested by Gonzalez and Gonzalez-Vilar (2001).
Three pots for each leaf area determination at 75 DAT, and leaf area and biomass determinations at final harvest, were separately harvested. After uprooting, the plant samples were separated into different plant parts, namely, leaves, stems, and pods. The fresh weights of each plant part were immediately recorded using an electronic balance. Leaf area was then measured using a leaf area meter (LI-3100C Area Meter, LI-COR Inc., USA). All samples were then oven-dried at 70°C for 72 h or until the weights were constant, and the dry weight was recorded. Total biomass was computed by summing the dry weights of all plant parts. Yield components were also recorded after harvesting, including number of pods per plant, pod fresh weight, pod dry weight, seed dry weight, and 100-seed weight.
Total phenolic content and proline content were measured in peanut leaves at 75 DAT and harvest stage. Liquid nitrogen was used to crush dry leaf samples. The total phenolic content was extracted from crushed dry leaf samples by dipping them in a beaker of pure methanol at room temperature on a magnetic stirrer. Each beaker was covered with aluminum foil. After 2 h, the extracted solutions were centrifuged at 5,000 rpm for 10 min, and the solutions were filtered through Whatman No. 4 paper. The solutions were then stored at 4°C until analysis. The total phenolic content was measured using the Folin-Ciocalteu’s reaction method (Kunnam et al., 2023), and the phenolic content was measured using a spectrophotometer (Fisher G10S UV-Vis Spectrophotometer 840-208200) at a 765-nm wavelength and expressed as gallic acid equivalent (mg of GAE 100 g−1 of dry samples).
Proline content was determined using the method of Bates et al. (1973). Firstly, 0.05 g of dried leaf sample was ground, and 5 ml of 3% aqueous sulfosalicylic acid solution was added into the ground sample. The solutions were then filtered through Whatman filter paper No. 1. The filtered solutions were mixed well with 2 ml glacial acetic acid and 2 ml acid ninhydrin using a vortex machine. The samples were boiled in boiling water at 98°C for 1 h, and the reaction was stopped by placing the hot samples in an ice bath. After cooling, 4 ml of toluene was added to the samples, mixed well, and set aside at room temperature to allow the solution to separate into two layers. The pink top layer of the solution was aspirated, and the absorbance was measured at 520 nm with a UV-Vis spectrophotometer.
The colonization of AMF in plant roots was assessed according to Phillip and Hayman (1970) and Trouvelot et al. (1986) with some modifications. The roots were cleaned with distilled water before incubation at 90°C with 10% KOH for 30 min. Subsequently, the root samples were transferred to 1% HCl for 5 min and then incubated in trypan blue for 24 h at room temperature. The roots were cut into 1-cm segments, and 30 pieces were randomly sampled to assess AMF colonization under a microscope.
The data from all experiments were analyzed using Statistix software (version 8.0) (Statistix8, 2003). Homogeneity of variance was tested for all traits, and combined analysis of variance of two-season data was done. The means for all traits were separated by least significance difference (LSD) test at the 0.05 probability level (Gomez and Gomez, 1984). Correlation was calculated using Statistix 8 software.
The soil in this study was classified as non-saline and slightly alkaline (Table 1). The soil was low in organic matter, exchangeable potassium, exchangeable calcium, exchangeable magnesium, extractable copper, and sodium; medium in extractable zinc; and high in available phosphorus, extractable iron, and exchangeable manganese. Soil moisture contents were significantly different between FC and drought treatment at 75 DAT and harvest stage in both seasons (Figure 1).
Figure 1. Soil moisture content (%) of field capacity (FC) and drought treatments throughout the experiment in dry (A) and rainy seasons (B).
The plants grown under uninoculated treatment had lower AMF colonization than under inoculated treatments in both two water regimes and two seasons (Figure 2). Inoculation of AMF tended to have higher AMF colonization than co-inoculation of AMF and Rhizobium. Moreover, drought stress reduced AMF colonization in both seasons. The colonization of AMF ranged from 1.10% (uninoculated treatment under drought) to 12.48% (AMF inoculated under FC).
Figure 2. Arbuscular mycorrhizal fungi colonization (%) of peanut root growing under field capacity (FC) and drought condition in dry season (A) and rainy season (B). no, non-inoculation; Rhi +AMF, inoculation arbuscular mycorrhiza together with rhizobium; AMF, inoculation with arbuscular mycorrhiza. The means indicated by different capital letters are significantly different at P < 0.05.
Relative water content (RWC) decreased when plants were exposed to drought conditions in both growing seasons. The decrease in RWC was most pronounced in the rainy season (Figure 3).
Figure 3. Relative water content (RWC) of peanut leaves growing under field capacity (FC) and drought condition at 75 DAT in dry season (A) and rainy season (B), and, at harvest stage in dry season (C) and rainy season (D). No, non-inoculation; AMF+Rhi, inoculation arbuscular mycorrhiza with rhizobium; AMF, inoculation arbuscular mycorrhiza. The means indicated by different lowercase letters are significantly different at P < 0.05.
Under FC conditions, methods of fungi inoculation did not show a significant effect on relative water content at 75 DAT and harvest in both seasons; however, they had significant effects under drought conditions. The treated plants, AMF alone and rhizobium + AMF combination, were able to improve RWC in peanut leaves under drought conditions compared with no application in both seasons, although no significant difference was observed in the dry season. Non-inoculation had the lowest RWC under drought condition.
Water regime significantly affected leaf area per plant at 75 DAT and harvest in the dry season (Table 2). Drought stress reduced leaf area per plant from 4,650.3 cm3 to 4,045.6 cm3 at 75 DAT and 5,952.2 cm3 to 3,635.4 cm3 at harvest. The results in the rainy season were similar to those in the dry season, and drought reduced leaf area per plant from 4,739.9 cm3 to 3,026.9 cm3 at 75 DAT and from 5,826.6 cm3 to 3,022.3 cm3 at harvest.
Table 2. Leaf area (cm3 plant−1) per plant, leaf total phenolic content (TPC; mg 100g−1) and leaf proline content (mg g−1 dry weight) at 75 DAT and harvest stage, and biomass dry weight (g plant−1) at harvest stage of peanut growing under different water regimes and fungi applications in two seasons.
Application of biofertilizers also significantly affected leaf area per plant at 75 DAT, but it did not significantly affect leaf area per plant at harvest (Table 2). AMF alone and AMF + rhizobium significantly increased leaf area per plant from 4,131.6 cm3 of control to 4,440.9 cm3 and 4,471.3 cm3, respectively. However, the difference between AMF alone and AMF + rhizobium was not significant. AMF alone and AMF + rhizobium also increased leaf area per plant at harvest, but the increases in leaf area per plant were not significant.
Inoculation of biofertilizers also significantly increased the leaf area per plant at 75 DAT in the rainy season, but the increases in leaf area per plant at harvest were not significant. AMF + Rhizobium gave the highest leaf area per plant (4,339.3 cm3) followed by AMF alone (3,912.5 cm3), whereas non-inoculated control produced the lowest leaf area per plant (3,398.3 cm3). However, the leaf area of the plants treated by AMF alone and AMF + rhizobium were not significantly different from un-inoculated control at harvest.
For combined analysis of the interaction between water regime and biofertilizer, it is clear that biofertilizer did not have a significant effect on leaf area per plant at the time of harvest (Table 3). However, biofertilizer contributed to the significantly higher leaf area per plant than untreated control at 75 DAT. The effect of biofertilizer was not significant at harvest. However, the effect of drought was more pronounced as it drastically reduced the lead leaf area per plant compared with FC.
Table 3. Leaf area (cm3) per plant, leaf total phenolic content (TPC) (mg 100g−1), and leaf proline content (mg g−1 dry weight) at 75 DAT and biomass dry weight (g plant−1) at harvest of peanut growing under different water regimes and fungi applications in two seasons.
Drought stress was significantly higher than FC for total phenolic content in leaves of peanut at both 75 DAT and harvest (Table 2). Total phenolic contents of peanut grown under drought stress were 476.81 mg 100 g−1 at 75 DAT and 563.18 mg 100 g−1 at harvest, whereas total phenolic contents of peanut leaves grown under FC were 443.55 mg 100 g−1 at 75 DAT and 515.65 mg 100 g−1 at harvest. The results in the rainy season were in agreement with the results in the dry season, and total phenolic contents of peanut grown under drought were significantly higher than those of peanut grown under FC at 75 DAT and harvest.
The significantly higher total phenolic contents than untreated control was found in AMF at 75 DAT in both dry and rainy seasons. However, biofertilizer did not have significant effect on total phenolic content at harvest in the dry season, though the combined biofertilizer gave significantly lower total phenolic content (TPC) in the rainy season.
Under drought conditions, AMF-treated plants showed significantly higher total phenolic contents than untreated control at 75 DAT in both dry and rainy seasons, but the effect of biofertilizer was not significant at harvest in both seasons (Table 3).
Drought stress significantly increased proline contents at 75 DAT and harvest in both seasons (Table 2). Under the dry season, the proline contents under FC were 0.473 mg g−1 dry weight at 75 DAT and 0.421 mg g−1 dry weight at harvest, whereas the proline contents under drought were 0.590 mg g−1 dry weight at 75 DAT and 0.459 mg g−1 dry weight at harvest. The effect of drought stress on proline content in the rainy season provided similar information. Biofertilizer did not have significant effect on proline content at 75 DAT and harvest in both seasons.
For interaction effect, the treatment combinations under FC were not significantly different for proline content at 75 DAT in both dry and rainy seasons (Table 3). However, the AMF-treated plant under FC was significantly higher than untreated control at harvest in the dry season, whereas the AMF + rhizobium-treated plant under drought stress was significantly lower than untreated control at harvest in the dry season.
Fresh weight biomass and dry weight biomass provided similar information, and dry biomass was reported herein. Drought significantly reduced biomass of peanut grown in both seasons (Table 2). Biomass was reduced from 60.82 g plant−1 to 39.55 g plant−1 in the dry season and from 52.43 g plant−1 to 28.70 g plant−1 in the rainy season.
Application of AMF and AMF + rhizobium also significantly increased biomass in both seasons as they had significantly higher biomass than untreated control. However, the biomass of AMF and AMF + rhizobium were not significantly different.
Interaction effect showed that AMF and AMF + rhizobium were significantly higher than untreated control under FC in the rainy season (Table 3). However, they were not significantly different from untreated control under drought stress and FC in the dry season, and also under drought stress in the rainy season.
Drought stress significantly reduced all yield and yield-related traits of peanut in both seasons (Table 4). The reductions were from 22.89 pods to 17.21 pods for pod number, 52.23 g to 34.13 g for pod fresh weight, 26.87 g to 16.06 g for pod dry weight, 17.29 g to 9.58 g for seed dry weight, and 40.03 g to 34.27 g for 100-seed weight. Similar reductions were also found in the rainy season.
Table 4. Yield components of peanut growing under different water regimes and fungi applications in two seasons.
In the dry season, the plants treated with AMF and AMF + rhizobium were not significantly different from untreated control for most traits. The significant difference was observed only for pod number in the dry season in which AMF+ Rhizobium was significantly higher than untreated control. In the rainy season, biofertilizers were not significantly different for pod number, pod fresh weight, pod dry weight, and seed dry weight, but they were significantly different for 100-seed weight. AMF was significantly higher than untreated control for this trait.
For the interaction effect, there was no significant difference between biofertilizers and untreated control for all traits under the FC and drought stress in the dry season (Table 5). However, in the rainy season, significant differences of biofertilizers and untreated control were observed for pod number under drought stress, pod fresh weight and pod dry weight under FC, and seed dry weight and 100-seed weight under FC and drought stress. Although there were significant differences, both biofertilizer treatments were significantly lower than untreated control under FC. In contrast to under FC, both biofertilizer treatments were significantly higher than untreated control under drought stress.
Table 5. Yield components of peanut growing under different water regimes and fungal applications in two seasons.
Water regime changed the relationships among traits of peanut (Tables 6, 7), The correlation coefficients among pod number, pod fresh weight, pod dry weight, and seed dry weight were positive and significant (P ≤ 0.01), ranging from 0.60** to 0.96** under FC and 0.89** to 0.98** under drought stress. Biomass was more important for yield under drought stress than under FC as the relationships of biomass and yield-related traits were stronger under drought stress, whereas the importance of leaf area on yield-related traits was reduced under drought stress. AMF colonization had a significant contribution to biomass under FC (0.47**); moreover, it had significant contributions to pod number (0.47*) and 100-seed weight (0.49*) under drought stress. Leaf area was more important for biomass (0.79**) and pod dry weight (0.42*) under drought stress than under FC, whereas 100-seed weight had a significant contribution to seed dry weight (0.42*) under drought stress.
Table 6. Correlation coefficients among AMF colonization, growth, and yield-related traits of peanut grown under FC.
Table 7. Correlation coefficients among AMF colonization, growth, and yield-related traits of peanut grown under drought stress.
According to the findings of this study, AMF colonization was also detected in untreated control with a small percentage of colonization. This would be due to the natural occurrence of AMF in the soil. Johnson (1998) also mentioned that the colonization of AMF in the untreated control was due to the presence of the native AMF. AMF are soil-borne microbes that play a major role in improvement of plant nutrient uptake and resistance to several abiotic stresses (Sun et al., 2018). Inoculation of effective strains of AMF can increase cop productivity under drought; therefore, the effective strains should be selected for commercial use.
In this study, single inoculation of AMF had higher colonization than co-inoculation of AMF with rhizobium. Saxena et al. (1997) stated weaker effects on AMF colonization after dual inoculation with AMF and rhizobium versus single inoculation. Chalk et al. (2006) also noted that inoculation with AMF and rhizobium resulted in reduced AMF colonization than inoculation with simply AMF and that rhizobium and AMF compete for colonization sites in legume roots, which may decrease the symbiotic effect. However, co-inoculation of AMF and rhizobium performed better than single AMF or rhizobium inoculation, and it also exhibited synergistic effects on legume microbial colonization and nodulation (Marques et al., 2001), as well as white clover growth and yield (Xie et al., 2020).
In this study, drought stress reduced AMF colonization. The reduction in AMF colonization affected by drought stress has been reported in previous research. There was no arbuscular formation and low hyphal colonization of AMF on Populus cathayana seedlings under extreme drought conditions. Reduction of AMF colonization may be caused by AMF death or granule formation during the drought period (Han et al., 2022).
The change in moisture regimes affected AMF colonization in root and soil (Staddon et al., 2003; Clark et al., 2009). Water content was associated with AMF colonization in Lotus tenuis (Garcia and Mendoza, 2008). Moisture could hasten the spores to germinate and form colonization on the roots (Yuwati et al., 2020). Higher levels of germination could be obtained at low water potential when spores were incubated longer.
The length of germ tube was reduced at low water potential (Ajeesh et al., 2015). AMF development is favored when the moisture content of the medium is slightly less than optimal for plant growth. A moisture content of approximately 0.1–0.2 bars appears to be adequate for inoculum production (Habte and Osorio, 2001).
Another factor that reduced AMF colonization is soil available phosphorus. Carrenho et al. (2007) stated that application of phosphorus significantly reduced mycorrhizal colonization in peanut. The soil used in this study had a high level of phosphorus (Table 1). The gene expression regulating phosphorus homeostasis in fungi was also influenced by inorganic phosphorus (Ezawa and Saito, 2018). Under high phosphorus supply, the plant can uptake sufficient phosphorus through their root; therefore, the ability of plant to limit AMF colonization is considered a strategy (Balzaegue et al., 2010; Breuillin et al., 2010). Some studies have also confirmed that the abundance of AMF can be reduced by applying phosphorus (Shao et al., 2023). The application of AMF can be effective under the appropriate water and also phosphorus concentration.
In this study, drought reduces RWC in peanut leaves, and AMF and AMF+Rhi applications can improve RWC under drought. The beneficial effect of AMF on alleviation of drought stress has been reported. Hyphae penetrate deep into the soil and provide moisture to the plants and have a positive effect on enzyme activities and protect plant cells from injury caused by drought (He et al., 2019). AMF produces an extensive mycelium which provides to absorb more nutrients and water by plant roots (Tang et al., 2022). Under drought stress, AMF also adjusted the accumulation of different hormones such as ABA, jasmonic acid (JA), and strigolactones which maintained the higher leaf relative water content and water use efficiency under drought stress. An increase in ABA level which performs as an anti-transpirant can reduce water loss by stomata closing and maintaining higher water use efficiency (Tang et al., 2022). Additionally, under stressful conditions, inoculation with AMF inhibited reactive oxygen species (ROS) accumulation of peanut; moreover, AMF-inoculated plants enhanced the activities of antioxidant enzymes (SOD, G-POD, CAT, and APX), total soluble sugar, sucrose, and free amino acids under stressful conditions (Liu et al., 2023).
In this study, AMF were higher than AMF + rhizobium for relative water content under drought stress. Under drought stress conditions, application of a mixture of bioinoculants may provide soybean plants to resist drought stress and also improve growth, productivity, and soil microbial activity (Nader et al., 2024). Ashwin et al. (2022) also stated that dual inoculation of AMF and rhizobium significantly enhanced physiological parameters and nutrient level under stress conditions. The interaction among soil microorganism is very complex and can cause the differences among studies.
Total phenolic content and proline content in peanut leaf were higher in drought treatment compared with well-watered conditions. Total phenolic content and proline content increased in response to drought stress. All Achillea species increased the proline content of the leaves, and the production of phenols in plants can be increased under drought stress (Gharibi et al., 2016). Drought stress reduces chlorophyll synthesis, increases proline contents, and causes oxidative damage by inducing the production of ROS. Under drought stress, plants accumulate different osmolytes; among them, proline is an important osmolyte that reduces the ROS by stimulating the activity of catalase (CAT), peroxidase (POD), superoxide dismutase (SOD), and other different antioxidant enzymes. Moreover, proline has a noticeable ability to bind and hydrate enzymes; thereby, it stabilizes and protects the macromolecules and maintains the structural integrity and function under drought stress (Tang et al., 2022).
In this study, AMF enhanced the total phenolic content while decreasing proline content in leaves under drought stress conditions at 75 DAT and harvest in both seasons, although there was no significant difference between AMF inoculation and non-inoculation. The proline content of AMF-inoculated plants is low, which may be due to the greater water content maintained by AMF. The finding was in agreement with previous research, which found that mycorrhiza inoculated Cupressus atlantica and Erythrina variegata and orange plants had a lower proline concentration in their leaves than non-mycorrhized plants growing under drought stress (Wu and Xia, 2006; Manoharan et al., 2010; Zarik et al., 2016).
The fungi, AMF, also improved the antioxidant defense system and the drought tolerance by increasing the accumulation of phenolic substances under drought conditions. It also considerably increases the amount of phenolic compounds by 50%–60% which substantially improves the drought stress tolerance (Tang et al., 2022).
In this study, drought reduces leaf area, biomass, and pod yield of peanut. Fungi application can improve leaf area at 75 DAT and biomass at harvest. Drought stress takes many morphological changes in plants such as reduction in size and area of leaf and growth of root and shoot due to activation of the abscisic acid (ABA) precursor (ACC) which prevents root growth (Hewedy et al., 2021; Sayer et al., 2021). Drought stress also lessens nodule growth and their N fixation and leads to a significant reduce in the growth and production of peanut (Furlan et al., 2012). Inoculation of AMF increased leaf area, shoot dry weight, root dry weight, total dry weight, and yield of soybean (Soretire et al., 2020). The colonization of AMF increases tomato vegetative growth for 50%–60% under reduced water (Leventis et al., 2021).
However, fungi application in both methods (AMF and Rhi + AMF) did not significantly affect yield and yield components of peanut in this study. The low effects of biofertilizer in this study might be caused by low AMF colonization. The colonization of AMF in this study ranged from 1.10% (under uninoculated treatment under drought) to 12.48% (AMF inoculated under FC). The colonization of AMF in this study was low compared with co-inoculation with rhizobium in other studies. Chotangui et al. (2022) reported the AMF colonization at 50% flowering growth from 25.33% to 63.11% in peanut var. Village and Garoua.
The relationships among agronomic traits and yield-related traits are well understood in peanuts. In this study, interest has been focused on the relationships between AMF colonization, and growth, yield, and yield parameters of peanut under FC and drought stress conditions.
In this study, AMF colonization could improve biomass, but it did not contribute to peanut yield under FC. However, AMF colonization could improve pod number and 100-seed weight resulting increase in seed yield under drought stress. Similar findings were reported by several former researchers. The improvement of plant performance and productivity and changes in the plant–water association can be caused by use of AMF under drought stress (Augé, 2001). Xiao et al. (2023) also indicated that AMF symbiosis proceeds drought stress tolerance of C. migao seedlings by improving water status, nutrient uptake, and growth. The plant under drought stress can acquire water and nutrients outside the root zone with the help of AMF (Begum et al., 2019). As a result, seed yield can be improved through the increases in pod number, 100-seed weight, and harvest index.
Drought reduced relative water content, leaf area, biomass, yield, and yield components in peanuts but increased proline and phenolic content in the leaves in both seasons. Application of AMF in both methods can increase relative water content, leaf area, and biomass under drought stress in both seasons; however, they had no effect on yield and yield components except pod number per plant in dry season. Fungal treatments increased phenolic levels in inoculated plants, but they did not have an effect on proline levels. It could be recommended that the use of AMF can help to preserve peanut biomass, and further research should be conducted in the fields in various growing areas.
The original contributions presented in the study are included in the article/supplementary material. Further inquiries can be directed to the corresponding authors.
CA: Conceptualization, Data curation, Formal analysis, Investigation, Methodology, Project administration, Writing – original draft, Writing – review & editing. PT: Formal analysis, Investigation, Methodology, Writing – original draft, Writing – review & editing. KB: Investigation, Writing – review & editing. TK: Methodology, Writing – review & editing. RR: Formal analysis, Writing – review & editing. AJ: Methodology, Writing – review & editing. YM: Formal analysis, Investigation, Writing – review & editing. PK: Methodology, Writing – review & editing.
The author(s) declare financial support was received for the research, authorship, and/or publication of this article. The research on “effect of arbuscular mycorrhiza and rhizobium on physiology and yield of peanut under drought conditions (FRB660065/0258-RE-KRIS/FF66/28)” by King Mongkut’s Institute of Technology Ladkrabang (KMITL) has received funding from the NSRF.
The authors declare that the research was conducted in the absence of any commercial or financial relationships that could be construed as a potential conflict of interest.
All claims expressed in this article are solely those of the authors and do not necessarily represent those of their affiliated organizations, or those of the publisher, the editors and the reviewers. Any product that may be evaluated in this article, or claim that may be made by its manufacturer, is not guaranteed or endorsed by the publisher.
Abd-Alla, M. H., Al-Amri, S. M., El-Enany, A. W. E. (2023). Enhancing rhizobium–legume symbiosis and reducing nitrogen fertilizer use are potential options for mitigating climate change. Agriculture. 13, 2092. doi: 10.3390/agriculture13112092
Ajeesh, R., Kumar, V., Santoshkumar, A. V., Surendra, G. K. (2015). Harnessing arbuscular mycorrhizal fungi (AMF) for quality seedling production. Res. J. Agric. Sci. 3, 22–40.
Aliasgharzad, N., Neyshabouri, M. R., Salimi, G. (2006). Effects of arbuscular mycorrhizal fungi and bradyrhizobium japonicum on drought stress of soybean. Biologia 61, S324–S328. doi: 10.2478/s11756-006-0182-x
Amine-Khodja, I. R., Boscari, A., Riah, N., Kechid, M., Maougal, R. T., Belbekri, N., et al. (2022). Impact of two strains of Rhizobium leguminosarum on the adaptation to terminal water deficit of two cultivars vicia faba. Plants 11, 515. doi: 10.3390/plants11040515
Aninbon, C., Jogloy, S., Vorasoot, N., NuChadomrong, S., Holbrook, C. C., Kvien, C., et al. (2019). Effect of terminal drought on arginine content in peanut genotypes with difference in levels of drought resistance. Int. J. Plant Prod. 13, 155–162. doi: 10.1007/s42106-019-00043-x
Ashwin, R., Bagyaraj, D. J., Raju, B. M. (2022). Dual inoculation with rhizobia and arbuscular mycorrhizal fungus improves water stress tolerance and productivity in soybean. Plant Stress. 4, 100084. doi: 10.1016/j.stress.2022.100084
Augé, R. M. (2001). Water relations, drought and vesicular-arbuscular mycorrhizal symbiosis. Mycorrhiza. 11, 3–42. doi: 10.1007/s005720100097
Balzaegue, C., Puech-Pagès, V., Bécard, G., Rochange, S. F. (2010). The regulation of arbuscular mycorrhizal symbiosis by phosphate in pea involves early and systemic signaling events. J. Exp. Bot. 62, 1049–1060. doi: 10.1093/jxb/erq335
Bates, L. S., Waldren, R. P., Teare, I. D. (1973). Rapid determination of free proline for water-stress studies. Plant Soil. 39, 205–207. doi: 10.1007/BF00018060
Begum, N., Ahanger, M. A., Su, Y., Lei, Y., Mustafa, N. S. A., Ahmad, P., et al. (2019). Improved drought tolerance by AMF inoculation in maize (Zea mays) involves physiological and biochemical implications. Plants 8 (12), 1–20. doi: 10.3390/plants8120579
Breuillin, F., Schramm, J., Hajirezaei, M., Ahkami, A., Favre, P., Druege, U., et al. (2010). Phosphate systemically inhibits development of arbuscular mycorrhiza in Petunia hybrida and represses genes involved in mycorrhizal functioning. Plant J. 64, 1002–1017. doi: 10.1111/j.1365-313X.2010.04385.x
Carrenho, R., Trufem, S. F. B., Bononi, V. L. R., Silva, E. S. (2007). The effect of different soil properties on arbuscular mycorrhizal colonization of peanuts, sorghum and maize. Acta Bot. Bras. 21, 723–730. doi: 10.1590/S0102-33062007000300018
Chalk, P. M., Souza, R. D. F., Urquiaga, S., Alves, B. J. R., Boddy, R. M. (2006). The role of arbuscular mycorrhiza in legume symbiotic performance. Soil Biol. Biochem. 38, 2944–2951. doi: 10.1016/j.soilbio.2006.05.005
Cheng, S., Zou, Y. H., Kuca, K., Hashem, A., Abd_Allah, E. F., Wu, Q. S. (2021). Elucidating the mechanisms underlying enhanced drought tolerance in plants mediated by arbuscular mycorrhizal fungi. Front. Microbiol. 12. doi: 10.3389/fmicb.2021.809473
Chotangui, A. H., Ndingadal, K. H., Adamou, S., Mandou, S. M., Solange, M. S., Djonko, H. B., et al. (2022). Growth and yield response of groundnut (Arachis hypogaea l.) to rhizobial and arbuscular mycorrhiza fungal inoculations in the western highlands of cameroon. Plant 10 (3), 69–75. doi: 10.11648/j.plant.20221003.11
Clark, N. M., Rillig, M. C., Nowak, R. S. (2009). Arbuscular mycorrhizal fungal abundance in the mojave desert: Seasonal dynamics and impacts of elevated CO2. J. Arid Environ. 73 (9), 834–843. doi: 10.1016/j.jaridenv.2009.03.004
Dobo, B. (2022). Effect of arbuscular mycorrhizal fungi (AMF) and rhizobium inoculation on growth and yield of Glycine max L. varieties. Int. J. Agron. 1, 9520091. doi: 10.1155/2022/9520091
Doorenbos, J., Pruitt, W. O. (1992). “Calculation of crop water requirement,” in Crop Water requirement (FAO of The United Nation, Rome, Italy), 1–65. Available at: https://www.fao.org/4/s8376e/s8376e.pdf.
Ezawa, T., Saito, K. (2018). How do arbuscular mycorrhizal fungi handle phosphate? New insight into fine-tuning of phosphate metabolism. New Phytol. 220, 1116–1121. doi: 10.1111/nph.15187
Fathi, A., Tari, D. B. (2016). Effect of drought stress and its mechanism in plants. Int. J. Life Sci. 10, 1–6. doi: 10.3126/IJLS.V10I1.14509
Furlan, A., Llanes, A., Luna, V., Castro, S. (2012). Physiological and biochemical responses to drought stress and subsequent rehydration in the symbiotic association peanut-Bradyrhizobium sp. Int. Sch Res. Notices. 366, 318083. doi: 10.5402/2012/318083
Garcia, I. V., Mendoza, R. E. (2008). Relationships among soil properties, plant nutrition and arbuscular mycorrhizal fungi-plant symbioses in a temperate grassland among hydrologic, saline and sodic gradients. FEMS Microbiol. Ecol. 63, 359–371. doi: 10.1111/j.1574-6941.2008.00441.x
Gharibi, S., Tabatabaei, B. E. S., Saeidi, G., Goli, S. A. H. (2016). Effect of drought stress on total phenolic, lipid peroxidation, and antioxidant activity of Achillea species. Appl. Biochem. Biotechnol. 178, 796–809. doi: 10.1007/s12010-015-1909-3
Girdthai, T., Jogloy, S., Vorasoot, N., Akkasaeng, C., Wongkaew, S., Holbrook, C. C., et al. (2010). Associations between physiological traits for drought tolerance and aflatoxin contamination in peanut genotypes under terminal drought. Plant Breed. 129, 693–699. doi: 10.1111/j.1439-0523.2009.01738.x
Gomez, K. A., Gomez, A. A. (1984). Statistical procedures for agricultural research. 2nd ed (New York: John Wiley & Sons).
Gonzalez, L., Gonzalez-Vilar, M. (2001). “Determination of relative water content,” in Handbook of Plant Ecophysiology Techniques (Springer, The Netherlands), 207–212, ISBN: 978-0-7923-7053-6.
Guimaraes, S. L., Bonfim-Silva, E. M., de Souza, A. C. P., Simeon, B. G. (2019). Efficiency of inoculation with rhizobium in peanuts (Arachis hypogaea L.) grown in Brazilian Cerrado soil. Agric. Sci. 10, 948–956. doi: 10.4236/as.2019.107072
Habte, M., Osorio, N. W. (2001). Arbuscular mycorrhizas: Producing and applying arbuscular mycorrhizal inoculum (University of Hawaii at Manoa: Department of Tropical Plant and Soil Sciences).
Han, Y., Lou, X., Zhang, W., Xu, T., Tang, M. (2022). Arbuscular mycorrhizal fungi enhanced drought resistance of populus cathayana by regulating the 14-3-3 family protein genes. Microbiol. Spectr. 10 (3), 1–18. doi: 10.1128/spectrum.02456-21
Hashem, A., Kumar, A., Al-Dbass, A., Alqarawi, A., Al-Arjani., A. F., Singh, G., et al. (2018). Saudi J. Biol. 26 (3), 614–624. doi: 10.1016/j.sjbs.2018.11.005
He, J. D., Dong, T., Wu, H. H., Zou, Y. N., Wu, Q. S., Kuca, K. (2019). Mycorrhizas induce diverse response of root TIP aquaporin gene expression to drought stress in trifoliate orange. Sci. Hortic. (Amsterdam). 234, 64–69. doi: 10.1016/j.scienta.2018.08.010
Hewedy, O. A., Abdel Lateif, K. S., Seleiman, M. F. (2021). Phylogenetic diversity of Trichoderma strains and their antagonistic potential against soil-borne pathogens under stress conditions. Biology. 9, 189. doi: 10.3390/biology9080189
Jaiswal, S. K., Mustapha, M., Ibny, F. Y. I. (2021). Rhizobia as a source of plant growth-promoting molecules: Potential applications and possible operational mechanisms. Front. Sustain Food Syst. 4. doi: 10.3389/fsufs.2020.619676
Johnson, N. C. (1998). Responses of Salsola kali and Panicum virgatum to mycorrhizal fungi, phosphorus and soil organic matter: implications for reclamation. J. Appl. Ecol. 35, 86–94. doi: 10.1046/j.1365-2664.1998.00277.x
Jovino, R. S., da Silva, T. R., Rodrigues, R. T., de Sá Carvalho, J. R., Cunha, J. B. A., de Lima, L. M., et al. (2022). Elite Bradyrhizobium strains boost biological nitrogen fixation and peanut yield in tropical drylands. Braz. J. Microbiol. 53, 1623–1632. doi: 10.1007/s42770-022-00792-4
Junjittakarn, J., Jogloy, S., Vorasoot, N., Jongrungklang, N. (2016). Effect of mid-season drought and recovery on physiological traits and root system in peanut genotypes (Arachis hypogaea L.). Sabrao. J. Breed Genet. 48, 318–331.
Koolachart, R., Jogloy, S., Vorasoot, N., Wongkaew, S., Holbrook, C. C., Jongrungklang, N., et al. (2013). Rooting traits of peanut genotypes with different yield responses to terminal drought. Field Crops Res. 149, 366–378. doi: 10.1016/j.fcr.2013.05.024
Kunnam, J., Teamkao, P., Aninbon, C. (2023). Chemical composition and bioactive compounds of Riceberry rice produced under organic and conventional practices. J. Agric. Sci. 161, 13–21. doi: 10.1017/S0021859622000648
Leventis, G., Tsiknia, M., Feka, M., Ladikou, E. V., Papadakis, I. E., Chatzipavlidis, I., et al. (2021). Arbuscular mycorrhizal fungi enhance growth of tomato under normal and drought conditions, via different water regulation mechanisms. Rhizosphere. 19, 100394. doi: 10.1016/j.rhisph.2021.100394
Liu, Y., Lu, J., Cui, L., Tang, Z., Ci, D., Zou, X., et al. (2023). The multifaceted roles of arbuscular mycorrhizal fungi in peanut responses to salt, drought, and cold stress. BMC Plant Biol. 23, 36. doi: 10.1186/s12870-023-04053-w
Manoharan, P., Shanmugaiah, V., Balasubramanian, N., Gomathinayagam, S., Sharma, M. P., Muthuchelian, K. (2010). Influence of AM fungi on the growth and physiological status of Erythrina variegata Linn. grown under different water stress conditions. Eur. J. Soil Biol. 46, 151–156. doi: 10.1016/j.ejsobi.2010.01.001
Marques, M., Pagano, M., Scotti, M. R. (2001). Dual inoculation of a woody legume (Centrolobium tomentosum) with rhizobia and mycorrhizal fungi in south-eastern Brazil. Agrofor. Syst. 50, 107–117. doi: 10.1023/A:1010637401475
Mondal, M., Skalicky, M., Garai, S., Hossain, A., Sarkar, S., Banerjee, H., et al. (2020). Supplementing nitrogen in combination with rhizobium inoculation and soil mulch in peanut (Arachis hypogaea L.) production system: Part II. Effect on phenology, growth, yield attributes, pod quality, profitability and nitrogen use efficiency. Agronomy. 10, 1513. doi: 10.3390/agronomy10101513
Nader, A. A., Fathi, I., Hauka, A., Afify, A. H., El-Sawah, A. M. (2024). Drought-tolerant bacteria and arbuscular mycorrhizal fungi Mitigate the detrimental effects of drought stress induced by withholding irrigation at critical growth stages of soybean (Glycine max, L.). microorganisms. 12, 1123. doi: 10.3390/microorganisms12061123
Oliveira, T. C., Cabral, J. S., Santana, L. R., Tavares, G. G., Santos, L. D., Paim, T. P., et al. (2022). The arbuscular mycorrhizal fungus rhizophagus clarus improves physiological tolerance to drought stress in soybean plants. Sci. Rep. 12 (1), 1–15. doi: 10.1038/s41598-022-13059-7
Phillip, J. M., Hayman, D. S. (1970). Improve procedures for cleaning roots and staining parasitic and vesicular-arbuscular mycorrhizal fungi for rapid assessment of infection. Trans. Brit Mycologia. 53, 158–161. doi: 10.1016/S0007-1536(70)80110-3
Rachaputia, R., Chauhana, Y. S., Wrightb, G. C. (2021). “Chapter 11 - Peanut,” in Crop Physiology Case Histories for Major Crops. Academic Press., 360–382. doi: 10.1016/B978-0-12-819194-1.00011-6
Razakatiana, A. T. E., Trap, J., Baohanta, R. H., Raherimandimby, M., Roux, C. L., Duponnois, R., et al. (2020). Benefits of dual inoculation with arbuscular mycorrhizal fungi and rhizobia on Phaseolus vulgaris planted in a low-fertility tropical soil. Pedobiologia. 83, 150685. doi: 10.1016/j.pedobi.2020.150685
Saxena, A. K., Rathi, S. K., Tilak, K. V. B. R. (1997). Differential effect of various endomycorrhizal fungi on nodulating ability of green gram by Bradyrhizobium sp. (Vigna) strain S24. Biol. Fertil Soils 24, 175–178. doi: 10.1007/s003740050227
Sayer, J. E., Crawford, A. J., Edgerley, J., Askew, P. A., Hahn, Z. C., Whitlock R. and Dodd, C. I. (2021). Adaptation to chronic drought modifies soil microbial community responses to phytohormones. Commun. Biol. 4, 1–9. doi: 10.1038/s42003-021-02037-w
Shao, Y., Wang, Z., Liu, W., Zhang, X., Wang, J., Guo, P. (2023). Effects of variations in soil moisture and phosphorus concentrations on the diversity of the arbuscular mycorrhizal fungi community in an agricultural ecosystem. Agriculture. 13, 1272. doi: 10.3390/agriculture13061272
Sheteiwy, M. S., Ali, D. F. I., Xiong, Y. C., Brestic, M., Skalick, M., Hamoud, Y. A., et al. (2021). Physiological and biochemical responses of soybean plants inoculated with arbuscular mycorrhizal fungi and Bradyrhizobium under drought stress. BMC Plant Biol. 21, 195. doi: 10.1186/s12870-021-02949-z
Singh, S., Russel, M. B. (1981). “Water use by maize/pigeonpea intercrop on a deep Vertisol,” in Proceedings of International Workshop on Pigeonpeas, vol. 1. (ICRISAT Center Patancheru, India), 271–282. Available at: https://oar.icrisat.org/1956/1/Proceedings_of_the_international_workshop_on_pigeonpeasV1.pdf.
Soretire, A. A., Adeyemi, N. O., Atayese, M. O., Olubode, A. A., Adewunmi, A. (2020). Inoculation of arbuscular mycorrhizal fungi improve soil chemical properties, growth and symbiotic N2 -fixation in soybean (Glycine max L.) cultivars under field condition with low phosphorus availability. Acta Fytotechn Zootechny. 23, 182–191. doi: 10.15414/afz.2020.23.04.182-191
Staddon, P. L., Thompson, K., Jakobsen, I., Grime, J. P., Askew, P., A and Fitter, H. A. (2003). Mycorrhizal fungal abundance is affected by long-term climatic manipulations in the field. Glob.Change Biol. 9 (2), 186–194. doi: 10.1046/j.1365-2486.2003.00593.x
Sukharomana, S., Dobkuntod, B. (2003). Peanut and the Thai food system: macro study. University of Georgia, United States, 1–54.
Sun, Z., Song, J., Xin, X., Xie, X., Zhao, B. (2018). Arbuscular mycorrhizal fungal proteins 14-3-3- are involved in arbuscule formation and responses to abiotic stresses during AM symbiosis. Front. Microbiol. 5. doi: 10.3389/fmicb.2018.00091
Tang, H., Hassan, M. U., Feng, L., Nawaz, M., Shah, A. N., Qari, S. H., et al. (2022). The critical role of arbuscular mycorrhizal fungi to improve drought tolerance and nitrogen use efficiency in crops. Front. Plant Sci. 13. doi: 10.3389/fpls.2022.919166
Toomsan, B., McDonagh, J. F., Limpinuntana, V., Giller, K. E. (1995). Nitrogen fixation by groundnut and soyabean and residual nitrogen benefits to rice in farmers' fields in Northeast Thailand. Plant Soil. 175, 45–56. doi: 10.1007/BF02413009
Trouvelot, A., Kough, J. L., Gianinazzi-Pearson, V. (1986). “Mesure du taux de mycorhization VA d’un systeme radiculaire. Recherche de methods d’estimation ayant une signification fonctionnelle,” in Physiological and Genetical Aspects of Mycorrhizae (INRA, Paris), 217–221. Available at: https://www.scirp.org/reference/referencespapers?referenceid=1398644.
Wu, Q., Xia, R. (2006). Effects of arbuscular mycorrhizal fungi on leaf solutes and root absorption areas of trifoliate orange seedlings under water stress conditions. Front. Forest. China. 1, 312–317. doi: 10.1007/s11461-006-0035-3
Xiao, X., Liao, X., Yan, Q., Xie, Y., Chen, J., Liang, G., et al. (2023). Arbuscular mycorrhizal fungi improve the growth, water Status, and nutrient uptake of Cinnamomum migao and the soil nutrient stoichiometry under drought stress and recovery. J. Fungi. 9, 321. doi: 10.3390/jof9030321
Xie, M. M., Zou, Y. N., Wu, Q. S., Zhang, Z. Z., Kuca, K. (2020). Single or dual inoculation of arbuscular mycorrhizal fungi and rhizobia regulates plant growth and nitrogen acquisition in white clover. Plant Soil Environ. 66, 287–294. doi: 10.17221/234/2020-PSE
Yakubu, H., Kwari, J. D., Ngala, A. L. (2010). N2 fixation by grain legume varieties as affected by rhizobia inoculation in the sandy loam soil of Sudano-Sahelian zone of North Eastern Nigeria. Nig J. Basic Appl. Sci. 18, 229–236. doi: 10.4314/njbas.v18i2.64325
Yuwati, T. W., Rahmi, A. N. S., Hakim, S. S., Badruzsaufari (2020). The abundance of arbuscular mycorrhiza infective propagules under Galam stand at shallow peat of South Kalimantan. Bio Web Conf. 20, 3008. doi: 10.1051/bioconf/20202003008
Keywords: drought tolerance crop, groundnut, microorganism, phenols, AMF
Citation: Aninbon C, Teamkao P, Buram K, Kaewnoo T, Ruttanaprasert R, Janket A, Mon YY and Kaewtaphan P (2024) Effect of arbuscular mycorrhiza and rhizobium on physiology and yield of peanut under drought conditions. Front. Plant Sci. 15:1468636. doi: 10.3389/fpls.2024.1468636
Received: 22 July 2024; Accepted: 30 October 2024;
Published: 26 November 2024.
Edited by:
Dominik K. Großkinsky, Austrian Institute of Technology (AIT), AustriaReviewed by:
Saeed Ur Rahman, Shanghai Jiao Tong University, ChinaCopyright © 2024 Aninbon, Teamkao, Buram, Kaewnoo, Ruttanaprasert, Janket, Mon and Kaewtaphan. This is an open-access article distributed under the terms of the Creative Commons Attribution License (CC BY). The use, distribution or reproduction in other forums is permitted, provided the original author(s) and the copyright owner(s) are credited and that the original publication in this journal is cited, in accordance with accepted academic practice. No use, distribution or reproduction is permitted which does not comply with these terms.
*Correspondence: Chorkaew Aninbon, Y2hvcmthZXcuYW5Aa21pdGwuYWMudGg=; Pattrarat Teamkao, cGF0dHJhcmF0LnRlQGttaXRsLmFjLnRo
Disclaimer: All claims expressed in this article are solely those of the authors and do not necessarily represent those of their affiliated organizations, or those of the publisher, the editors and the reviewers. Any product that may be evaluated in this article or claim that may be made by its manufacturer is not guaranteed or endorsed by the publisher.
Research integrity at Frontiers
Learn more about the work of our research integrity team to safeguard the quality of each article we publish.