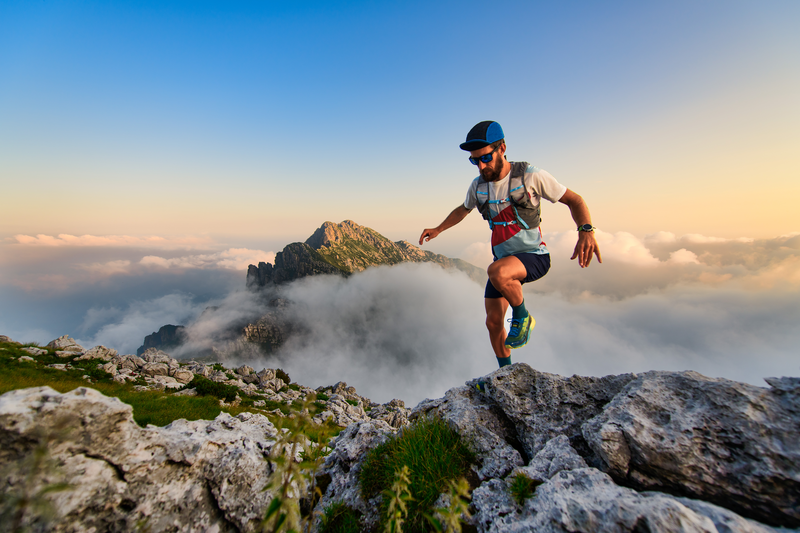
95% of researchers rate our articles as excellent or good
Learn more about the work of our research integrity team to safeguard the quality of each article we publish.
Find out more
MINI REVIEW article
Front. Plant Sci. , 05 December 2024
Sec. Plant Nutrition
Volume 15 - 2024 | https://doi.org/10.3389/fpls.2024.1465513
This article is part of the Research Topic Enhancing Plant Health Through Silicon Supplementation Under Nutritional Stress View all 6 articles
Manganese (Mn), a plant micronutrient element, is an important component of metalloprotein involved in multiple metabolic processes, such as photosynthesis and scavenging reactive oxygen species (ROS). Its disorder (deficiency or excess) affects the Mn-dependent metabolic processes and subsequent growth and development of plants. The beneficial element of Si has a variety of applications in agricultural fields for plant adaptation to various environmental stresses, including Mn disorder. The probable mechanisms for Si alleviation of Mn toxicity in plants are summarized as follows: (1) Si alters the rhizosphere acidification, root exudates and microorganisms to decrease the bioavailability of Mn in the rhizosphere; (2) Si down-regulates Mn transporter gene and reinforces the apoplastic barriers for inhibiting the Mn uptake and translocation; and (3) Si promotes the Mn deposition onto cell wall and Mn compartmentation into vacuole. Under Mn-deficient conditions, the probable mechanisms for Si promotion of Mn absorption in some plants remain an open question. Moreover, scavenging ROS is a common mechanism for Si alleviating Mn disorder. This minireview highlights the current understanding and future perspectives of Si regulation of manganese homeostasis in plants.
Manganese (Mn) is an essential element for plants, which is an integral part of the oxygen-evolving complex (OEC) of photosystem II (PSII) and serves as a cofactor for more than 30 enzymes, including Mn superoxide dismutase (MnSOD) and oxalate oxidase (Alejandro et al., 2020). Regardless of plant species, Mn should be accumulated at least 30 mg kg−1 dry weight in tissues to satisfy the demand for optimal growth and development (Broadley et al., 2012). If the accumulation of Mn in plants is below 10-20 mg kg−1 dry weight, Mn deficiency will occur (Broadley et al., 2012). Mn deficiency causes a lower net photosynthesis rate because the Mn-limited PSII is unstable and the development of chloroplast is inhibited (Schmidt et al., 2016). Under severe Mn-deficient conditions, leaves of plants will display brownish or necrotic spots in the tips, attributing to a decrease in MnSOD activity and thus their chloroplast impaired by the increased free oxygen radicals (Broadley et al., 2012; Hajiboland, 2012).
In contrast, high Mn causes a variety of symptoms in plants, and various plant species and genotypes have varying harmful Mn amounts (Fernando and Lynch, 2015). Generally, chlorotic leaves and necrotic spots are the most common symptoms of Mn toxicity among plant species (Millaleo et al., 2010), accompanied by decreased net photosynthetic efficiency and chlorophyll content (Alejandro et al., 2020). It is also observed that the uptake and translocation of other essential elements such as calcium (Ca), magnesium (Mg), iron (Fe), and phosphorus (P) are prevented in the Mn-stressed plants (Blamey et al., 2015; Lešková et al., 2017). Taken together, the maintenance of Mn homeostasis is required for normal plant growth and development.
The metalloid of silicon (Si) is classified as a quasi-essential element because of its proven protective and beneficial effects during plant adaptation to the environmental stresses (Coskun et al., 2019; Mandlik et al., 2020; de Tombeur et al., 2023). Si can be absorbed and translocated by Si transporters in plants only in the form of monomeric silicic acid (H4SiO4) (Mitani-Ueno and Ma, 2021), which is an uncharged molecule in solutions with a pH below 9. After H4SiO4 enters the plant body, it will deposit as inorganic hydrated SiO2 by silicification and form an organosilicon by covalent crosslinking with cell wall components (Liang et al., 2015; Sheng and Chen, 2020). It was proposed that plants silicify with a role in alleviating the nutritional imbalances (Pavlovic et al., 2021), such as the regulation of C: N: P homeostasis (Costa et al., 2024) and mitigation of boron disorder (Sheng et al., 2024), as well as the alleviation of Mn deficiency and toxicity (Che et al., 2016; Oliveira et al., 2021, 2022). To date, Si alleviation of Mn disorder-induced symptoms has been reported in many plants, including rice, cucumber, sorghum, cowpea, bean, maize, barley, sunflower, sugarcane, and others (Table 1). In this minireview, we focus on the underlying mechanisms and future perspectives of Si regulation of Mn homeostasis in plants.
Plants grown in acidic soils may suffer from Mn toxicity, and the underlying mechanisms for the Si-reduced Mn toxicity seem to differ with plant species. In general, decreasing the bioavailability of Mn in soil, inhibiting the Mn uptake and translocation, and optimizing the distribution and allocation of Mn in plants are the main approaches to cope with Mn toxicity. Herein we will discuss the Si effects on the Mn immobilization in soil, and its uptake, translocation and subcellular compartmentation in plants (Figure 1).
Figure 1. Si effects on the Mn immobilization in soil, and its uptake, translocation and subcellular compartmentation in plants under Mn-excessive conditions.
The bioavailability of Mn in the rhizosphere mainly depends on the soil pH, root exudates, and microorganisms (Alejandro et al., 2020). In addition, the fate of Mn is closely interwoven with aluminum (Al) which is abundant in acidic soils (Shao et al., 2017). Traditional silicon fertilizers produced from wollastonite are the Lewis base, whose supply can consume the proton (H+) for the formation of H4SiO4 and interact with Al in the root apoplast (Hodson and Evans, 2020), leading to an increased pH of rhizosphere soil in theory. It has been reported that the uptake of Si by rice plants can significantly reduce the degree of root acidification (Ma et al., 2021; Pang et al., 2023) by inhibiting the expressions of proton pump and organic acid secretion genes (Pang et al., 2023), and the elevated soil pH decreases the Mn2+ availability.
Root exudation is a dynamic behavior that mediates interactions between plant roots and soil matrix. Interestingly, the leaf Mn concentrations can be used as a proxy for rhizosphere carboxylate concentrations because the root exudates mobilize the micronutrient Mn in the rhizosphere (Lambers et al., 2015, 2021; Yan et al., 2024). Under Mn-excessive conditions, root exudates of total phenolics are increased in Citrus sinensis, while the secretion of root total free amino acids, total soluble sugars, malate, and citrate are not altered (Zheng et al., 2024). Furthermore, a great number of researches do show that the application of Si fertilizer could reduce the secretion of organic acids by plant roots (e.g., Wu et al., 2015; Fan et al., 2018; Javed et al., 2020; Pang et al., 2023), which are observed under the normal and stress conditions. Based on these discoveries, it can be speculated that Si may immobilize the excessive micronutrient Mn by mediating the secretion of metabolites in the rhizosphere.
Moreover, root exudates have multiple effects on bacterial community composition and microbiome assembly (Vives-Peris et al., 2020; Qu et al., 2021; Li et al., 2022; Kabir et al., 2024; Shi et al., 2024), so does Si by altering root-released carboxylates and phenolics. It was proposed that Si fertilization influences microbial assemblages of rice roots in a five-season in-situ remediation field study (Gao et al., 2022a). In this study, Si improvement of microbial diversity and richness in the rhizosphere was detected after the third fertilization, suggesting a prolonged effect of Si fertilization on the microbiome in roots (Gao et al., 2022a). Besides working in the rhizosphere, Si enhances the abundance of reducing microbes in the rhizoplane as well (Gao et al., 2022b). Very recently, it has been reported that Si could regulate the reassembled microbial communities in soil (Gao et al., 2024).
Despite these results being involved in the Si-mediated arsenic uptake by rice plants, we still believe that Si may decrease the soil Mn2+ availability with microbial mechanisms. There are two reasons: (1) Mn functions as an electron donor and acceptor for microorganisms, and the anaerobic redox transition between Mn2+ and MnOx accelerates a dynamic biogeochemical cycle coupled to microorganisms (Wang et al., 2022); (2) Anaeromyxobacter is closely linked to the Si-regulated microbial interactions (Gao et al., 2024), which might be involved in Mn oxidation and is capable of bioremediation in the Mn-contaminated soil (Liu et al., 2024).
In mycotrophic plants, arbuscular mycorrhizas also modify rhizosphere chemistry and influence Mn bioavailability and uptake (Brito et al., 2014; Nazeri et al., 2014). According to reports, inoculating legumes with arbuscular mycorrhizal fungi (AMF) increased the pH of the rhizosphere by around 0.2–0.7 pH units, reduced the total amount of carboxylates in the rhizosphere by 52%, and reduced the concentration of Mn in the shoots by 38% (Nazeri et al., 2014). In wheat, AMF colonization that begins with the intact extraradical mycelium improves bio-protection by decreasing Mn uptake in roots (Brito et al., 2014). Recently, it was proposed that arbuscular mycorrhizal symbiosis alleviates Mn toxicity by downregulating Mn transporter genes in Eucalyptus tereticornis (De Oliveira et al., 2023). Moreover, the relationships between Si and arbuscular mycorrhizas were established: AMF improve Si uptake and translocation, meanwhile Si increases mycorrhizal effectiveness in plants (Etesami et al., 2022). These results suggest an AMF-related mechanism of Si-mitigating Mn toxicity.
In roots, Casparian bands intercept the apoplastic flow, resulting in the requirement of transporters for nutrient uptake and translocation. Thus, Mn uptake and translocation are governed by the expression of transporter genes and the development of apoplastic barriers. There are many Mn transporters in plants, including members of the natural resistance-associated macrophage protein (NRAMP) family, the ZRT/IRT-related protein (ZIP) family, the yellow stripe-like (YSL) family, the cation exchanger (CAX) family, the cation diffusion facilitator/metal tolerance protein (CDF/MTP) family, the vacuolar iron transporter (VIT) family and others (Shao et al., 2017; Alejandro et al., 2020).
Among the Mn transporters, OsNramp5 and OsMTP9 make up the Mn uptake system in rice roots, and OsNramp5 acts as a metal/H+ symporter and facilitates the Mn permeation into the root epidermis (Sasaki et al., 2012), while OsMTP9 is an efflux Mn transporter and mediates the export of Mn2+ into the stele (Ueno et al., 2015). Che et al. (2016) proposed that Si inhibits the Mn uptake in rice roots by down-regulating the expression of OsNramp5 gene after a relatively long-term exposure to Si. Furthermore, the Mn concentration can be decreased in the shoots but increased in the roots due to the Si effects, suggesting that Si can reduce the root-to-shoot translocation of Mn in rice plants (Che et al., 2016). The probable mechanism for Si-reduced translocation is the formation of the Mn-Si complex in root cells.
The development of apoplastic barriers, including Casparian bands and the suberin lamellae, has a role in controlling the radial fluxes of water and nutrients and preventing the uptake of toxicants (Kreszies et al., 2020). It is widely known that Si can enhance the formation of Casparian bands and suberin lamellae by forming phenol complexes (Vaculík et al., 2012; Wu et al., 2019; Kreszies et al., 2020). Moreover, Si-modified phenols have an excellent ability to bind metals (Sheng and Chen, 2020), thereby accomplishing the Mn retention in roots. Our previous study also found that Casparian bands and Si-lignin interactions promote the silica deposition in the inner tangential cell walls of endodermis (Sheng et al., 2023). Root silica gels may serve as a pool that can store the excess Mn through the formation of the Mn-Si complex.
In rice plants, most of the total Mn taken up by roots was translocated to the shoots, regardless of the Si presence in roots (Che et al., 2016). Additionally, Si tends to raise leaf tissue tolerance rather than induce root Mn exclusion in cucumbers (Dragišić Maksimović et al., 2007, 2012). Considering that symptoms of Mn excess mainly occur in leaves, Si alleviation of foliar Mn stress is a top priority. At the cellular level, the first strategy is that Si alters the cell wall chemistry to enhance the Mn-binding to the cell wall. For example, the lignin synthesis in cucumber leaves is altered by Si supply in response to Mn stress (Dragišić Maksimović et al., 2007), as well as the callose synthesis in maize (Doncheva et al., 2009). These results are in agreement with our assumption that Si crosslinks with different cell wall components in various plants (Sheng and Chen, 2020). In Si-accumulating plants, leaf apoplast is one of the main locations for silica deposition, relevant or irrelevant to the cell wall (Hodson, 2019). It is likely that the Mn-Si complex can form in the leaf apoplast of Si-accumulating plants to prevent the uptake of Mn into the cytoplasm. Taken together, cell wall-bound Si and silica mediate the Mn accumulation in leaf apoplast for reducing the Mn toxicity.
Once the excess Mn2+ ions enter the cytoplasm, the intracellular reactions will be triggered. To avoid metal toxicity, Mn compartmentation into vacuole is one of the most important ways (Sharma et al., 2016; He et al., 2021), a process which depends on the tonoplast stability and tonoplast-localized transporter. To our knowledge, there is no evidence to show that some tonoplast-localized Mn transporters are regulated by Si. Furthermore, Si significantly strengthens the capacity of antioxidant system to scavenge reactive oxygen species (ROS), which can harm the membrane system. It means that Si increases the stability of membrane system, including the tonoplast, under stress conditions (Sheng et al., 2024). This, in turn, enhances the activity of tonoplast-localized Mn transporters (Sheng et al., 2018, 2024). Thus, Si has the potential ability to mediate the Mn compartmentation into the vacuole, but this should still be elucidated. Similar results have been reported in Cd-stressed rice cells: In the case of Cd, Si addition can reduce the Cd toxicity by compartmentation of Cd into vacuoles (Ma et al., 2016). Overall, it can be concluded that Si optimizes the subcellular distribution of Mn in shoots.
Mn deficiency often occurs in plants that grow in alkaline, well-aerated, and calcareous soils (Alejandro et al., 2020). In such soils, plant-available Mn2+ is readily oxidized and then converted to insoluble Mn oxides (MnOx). When sorghum and energy cane (Saccharum spontaneum L.) plants are exposed to Mn deficient environment, the Si fertilization of the root results in an increase in Mn use efficiency (Oliveira et al., 2021, 2022). Owing to a lack of investigations about the Si effects on Mn availability in the soil and the expression of Mn transporters, there is no way to know the underlying mechanisms for Mn uptake in sorghum and energy cane. In cucumber plant, Si application can prevent certain symptoms of Mn deficiency without any effects on the Mn uptake and accumulation (Bityutskii et al., 2014), suggesting a different mechanism in addition to Si enhancement of Mn uptake. It can be explained by Si-reducing the accumulation of ROS in plant tissues, which is a common mechanism among plant species and will be discussed in the next section.
The ROS burst is one of the most common phenomena in different cell compartments, resulting from the environment stress-induced disruption of cellular homeostasis. Both Mn deficiency and excess can trigger the ROS burst and then damage organelle membranes (Li et al., 2012; Oliveira et al., 2021, 2022). Many investigations indicate that Si mediates Mn disorder by scavenging reactive oxygen species in plants (Dragišić Maksimović et al., 2012; Oliveira et al., 2021, 2022). However, the effects of Si on the antioxidant defense system (including enzymatic and non-enzymatic antioxidants) differ from plant species under Mn excess (Dragišić Maksimović et al., 2007, 2012; Li et al., 2012). For instance, Si supply decreases the hydroxyl radical accumulation and suppresses the Mn-induced increased activity of peroxidase (POD) isoforms in cucumber (Dragišić Maksimović et al., 2012); while Si significantly counteracts high Mn-elevated malondialdehyde (MDA) and H2O2 concentrations and suppresses the Mn-induced increased activity of superoxide dismutase (SOD), catalase (CAT) and ascorbate peroxidase (APX) in Mn-sensitive rice plants (Li et al., 2012). Moreover, glutathione (GSH), non-protein thiols (NPT), and ascorbic acid (AsA) concentrations in rice are increased after Si addition (Li et al., 2012), as well as chlorogenic acid and caffeic acid in cucumber (Dragišić Maksimović et al., 2007). These results suggest that Si mainly influences non-enzymatic rather than enzymatic antioxidants in plants under high Mn stress.
Under Mn deficiency, Si reduces H2O2 and MDA contents and increases the SOD activity, phenol contents, thus improving the growth of energy cane (Oliveira et al., 2021, 2022). Similar results were also reported in sorghum plants, implying that Si enhances both enzymatic and non-enzymatic antioxidants (de Oliveira et al., 2019). In any case, Si accelerates the degradation of ROS and prevents the peroxidation of membrane systems under both Mn deficient and excessive conditions, with roles in attenuating the symptoms induced by Mn disorder and increasing photosynthetic activity in different plants (Li et al., 2015; de Oliveira et al., 2019).
Si regulates the Mn homeostasis in many plants with varying mechanisms. In sum, there are three approaches for Si to alleviate Mn stress: reducing the bioavailability of Mn in the rhizosphere, inhibiting Mn uptake and translocation, and optimizing the subcellular distribution of Mn in shoots. Furthermore, scavenging of reactive oxygen species is a common mechanism for Si alleviation of Mn disorder. Unfortunately, how Si promotes Mn uptake and accumulation in Mn-deficient plants is still unclear and the mechanisms for Si-attenuating high Mn stress are not fully known. To address these questions, the Si-Mn interactions with plant cell walls, ROS, and rhizosphere microorganisms should be considered in the future. If the Si-Mn interactions with plant cell walls are investigated in detail, how Si regulates the expression of Mn transporter genes will be addressed. Because the alterations of cell wall by Si, Mn, or both can be sensed by cell wall integrity sensors such as wall-associated kinases (WAKs) and FERONIA kinase family members (Wolf, 2022), which will trigger the cell wall integrity signaling and then reprogram the transcriptome of plants. Overall, these efforts will help improve our understanding of the Si roles in regulating Mn homeostasis.
YH: Writing – review & editing, Writing – original draft, Data curation. YuL: Writing – review & editing, Writing – original draft, Funding acquisition, Data curation. ZY: Writing – review & editing, Funding acquisition, Data curation. YiL: Funding acquisition, Data curation, Writing – review & editing. JF: Writing – review & editing, Visualization, Software. WL: Visualization, Software, Writing – review & editing, Data curation. HS: Writing – review & editing, Writing – original draft, Supervision, Funding acquisition, Data curation, Conceptualization.
The author(s) declare that financial support was received for the research, authorship, and/or publication of this article. This work was supported by the National Key Research and Development Program of China (Grant No.2018YFC1706101), the National Key Research and Development Program of Sichuan Province (Grant No. 2024YFFK0190), the first batch of Scientific and Technological Innovation Team for Qinghai-Tibetan Plateau Research in Southwest Minzu University (Grant No. 2024CXTD04) and the Fundamental Research Funds for the Central Universities, Southwest Minzu University (Grant No. ZYN2023099).
The authors declare that the research was conducted in the absence of any commercial or financial relationships that could be construed as a potential conflict of interest.
All claims expressed in this article are solely those of the authors and do not necessarily represent those of their affiliated organizations, or those of the publisher, the editors and the reviewers. Any product that may be evaluated in this article, or claim that may be made by its manufacturer, is not guaranteed or endorsed by the publisher.
Alejandro, S., Höller, S., Meier, B., Peiter, E. (2020). Manganese in plants: from acquisition to subcellular allocation. Front. Plant Sci. 11. doi: 10.3389/fpls.2020.00300
Bityutskii, N., Pavlovic, J., Yakkonen, K., Maksimović, V., Nikolic, M. (2014). Contrasting effect of silicon on iron, zinc and manganese status and accumulation of metal-mobilizing compounds in micronutrient-deficient cucumber. Plant Physiol. Biochem. 74, 205–211. doi: 10.1016/j.plaphy.2013.11.015
Blamey, F. P. C., Hernandez-Soriano, M., Cheng, M., Tang, C., Paterson, D., Lombi, E., et al. (2015). Synchrotron-based techniques shed light on mechanisms of plant sensitivity and tolerance to high manganese in the root environment. Plant Physiol. 169, 2006–2020. doi: 10.1104/pp.15.00726
Blamey, F. P. C., McKenna, B. A., Li, C., Cheng, M., Tang, C., Jiang, H., et al. (2018). Manganese distribution and speciation help to explain the effects of silicate and phosphate on manganese toxicity in four crop species. New Phytol. 217, 1146–1160. doi: 10.1111/nph.14878
Brito, I., Carvalho, M., Alho, L., Goss, M. J. (2014). Managing arbuscular mycorrhizal fungi for bioprotection: Mn toxicity. Soil Biol. Biochem. 68, 78–84. doi: 10.1016/j.soilbio.2013.09.018
Broadley, M., Brown, P., Cakmak, I., Rengel, Z., Zhao, F. (2012). “Function of nutrients: micronutrients,” in Marschner’s Mineral Nutrition of Higher Plants, 3rd Edn. Ed. Marschner, P. (Elsevier, Oxford), 191–249.
Che, J., Yamaji, N., Shao, J. F., Ma, J. F., Shen, R. F. (2016). Silicon decreases both uptake and root-to-shoot translocation of manganese in rice. J. Exp. Bot. 67, 1535–1544. doi: 10.1093/jxb/erv545
Coskun, D., Deshmukh, R., Sonah, H., Menzies, J. G., Reynolds, O., Ma, J. F., et al. (2019). The controversies of silicon’s role in plant biology. New Phytol. 221, 67–85. doi: 10.1111/nph.15343
Costa, M. G., de Mello Prado, R., Palaretti, L. F., de Souza Júnior, J. P. (2024). The effect of abiotic stresses on plant C: N: P homeostasis and their mitigation by silicon. Crop J. 12, 340–353. doi: 10.1016/j.cj.2023.11.012
de Oliveira, R. L. L., de Mello Prado, R., Felisberto, G., Checchio, M. V., Gratão, P. L. (2019). Silicon mitigates manganese deficiency stress by regulating the physiology and activity of antioxidant enzymes in sorghum plants. J. Soil Sci. Plant Nutr. 19, 524–534. doi: 10.1007/s42729-019-00051-w
De Oliveira, V. H., Montanha, G. S., Carvalho, H. W., Mazzafera, P., de Andrade, S. A. L. (2023). Mycorrhizal symbiosis alleviates Mn toxicity and downregulates Mn transporter genes in Eucalyptus tereticornis under contrasting soil phosphorus. Plant Soil 489, 361–383. doi: 10.1007/s11104-023-06024-4
de Tombeur, F., Raven, J. A., Toussaint, A., Lambers, H., Cooke, J., Hartley, S. E., et al. (2023). Why do plants silicify? Trends Ecol. Evol. 38, 275–288. doi: 10.1016/j.tree.2022.11.002
Doncheva, S. N., Poschenrieder, C., Stoyanova, Z., Georgieva, K., Velichkova, M., Barceló, J. (2009). Silicon amelioration of manganese toxicity in Mn-sensitive and Mn-tolerant maize varieties. Environ. Exp. Bot. 65, 189–197. doi: 10.1016/j.envexpbot.2008.11.006
Dragišić Maksimović, J., Bogdanović, J., Maksimović, V., Nikolic, M. (2007). Silicon modulates the metabolism and utilization of phenolic compounds in cucumber (Cucumis sativus L.) grown at excess manganese. J. Plant Nutr. Soil Sci. 170, 739–744. doi: 10.1002/jpln.200700101
Dragišić Maksimović, J., Mojović, M., Maksimović, V., Römheld, V., Nikolic, M. (2012). Silicon ameliorates manganese toxicity in cucumber by decreasing hydroxyl radical accumulation in the leaf apoplast. J. Exp. Bot. 63, 2411–2420. doi: 10.1093/jxb/err359
Etesami, H., Li, Z., Maathuis, F. J., Cooke, J. (2022). The combined use of silicon and arbuscular mycorrhizas to mitigate salinity and drought stress in rice. Environ. Exp. Bot. 201, 104955. doi: 10.1016/j.envexpbot.2022.104955
Fan, X. Y., Lin, W. P., Rui, L. I. U., Jiang, N. H., Cai, K. Z. (2018). Physiological response and phenolic metabolism in tomato (Solanum lycopersicum) mediated by silicon under Ralstonia solanacearum infection. J. Integr. Agr. 17, 2160–2171. doi: 10.1016/S2095-3119(18)62036-2
Fernando, D. R., Lynch, J. P. (2015). Manganese phytotoxicity: new light on an old problem. Ann. Bot. 116, 313–319. doi: 10.1093/aob/mcv111
Gao, Z., Chen, H., Zhang, X., Xiao, Z., Fan, X., Yin, C., et al. (2022b). Silicon enhances abundances of reducing microbes in rhizoplane and decreases arsenite uptake by rice (Oryza sativa L.). Environ. pollut. 306, 119405. doi: 10.1016/j.envpol.2022.119405
Gao, Z., Jiang, Y., Li, W., Chen, H., Ye, M., Liang, Y. (2024). Evidence for the role of microbes in the silicon-regulated arsenic concentrations of rice roots in the soil environment. Sci. Total Environ. 908, 168321. doi: 10.1016/j.scitotenv.2023.168321
Gao, Z., Jiang, Y., Yin, C., Zheng, W., Nikolic, N., Nikolic, M., et al. (2022a). Silicon fertilization influences microbial assemblages in rice roots and decreases arsenic concentration in grain: A five-season in-situ remediation field study. J. Hazard. Mater. 423, 127180. doi: 10.1016/j.jhazmat.2021.127180
Hajiboland, R. (2012). “Effect of micronutrient deficiencies on plants stress responses,” in Abiotic Stress Responses in Plants. Eds. Ahmad, P., Prasad, M. (Springer, New York, NY), 283–329. doi: 10.1007/978-1-4614-0634-1n_16
He, J., Rössner, N., Hoang, M. T., Alejandro, S., Peiter, E. (2021). Transport, functions, and interaction of calcium and manganese in plant organellar compartments. Plant Physiol. 187, 1940–1972. doi: 10.1093/plphys/kiab122
Hodson, M. J. (2019). The relative importance of cell wall and lumen phytoliths in carbon sequestration in soil: A hypothesis. Front. Earth Sci. 7. doi: 10.3389/feart.2019.00167
Hodson, M. J., Evans, D. E.. (2020). Aluminium-silicon interactions in higher plants: an update. J. Exp. Bot. 71, 6719–6729. doi: 10.1093/jxb/eraa024
Horst, W. J., Marschner, H. (1978). Effect of silicon on manganese tolerance of bean plants (Phaseolus vulgaris L.). Plant Soil 50, 287–303. doi: 10.1007/BF02107179
Iwasaki, K., Maier, P., Fecht, M., Horst, W. J. (2002a). Effects of silicon supply on apoplastic manganese concentrations in leaves and their relation to manganese tolerance in cowpea (Vigna unguiculata (L.) Walp.). Plant Soil 238, 281–288. doi: 10.1023/A:1014482911196
Iwasaki, K., Maier, P., Fecht, M., Horst, W. J. (2002b). Leaf apoplastic silicon enhances manganese tolerance of cowpea (Vigna unguiculata). J. Plant Physiol. 159, 167–173. doi: 10.1078/0176-1617-00691
Javed, M. T., Saleem, M. H., Aslam, S., Rehman, M., Iqbal, N., Begum, R., et al. (2020). Elucidating silicon-mediated distinct morpho-physio-biochemical attributes and organic acid exudation patterns of cadmium stressed Ajwain (Trachyspermum ammi L.). Plant Physiol. Biochem. 157, 23–37. doi: 10.1016/j.plaphy.2020.10.010
Kabir, A. H., Baki, M. Z. I., Ahmed, B., Mostofa, M. G. (2024). Current, faltering, and future strategies for advancing microbiome-assisted sustainable agriculture and environmental resilience. New Crops 1, 100013. doi: 10.1016/j.ncrops.2024.100013
Kreszies, T., Kreszies, V., Ly, F., Thangamani, P. D., Shellakkutti, N., Schreiber, L. (2020). Suberized transport barriers in plant roots: the effect of silicon. J. Exp. Bot. 71, 6799–6806. doi: 10.1093/jxb/eraa203
Lambers, H., Hayes, P. E., Laliberte, E., Oliveira, R. S., Turner, B. L. (2015). Leaf manganese accumulation and phosphorus-acquisition efficiency. Trends Plant Sci. 20, 83–90. doi: 10.1016/j.tplants.2014.10.007
Lambers, H., Wright, I. J., Guilherme Pereira, C., Bellingham, P. J., Bentley, L. P., Boonman, A., et al. (2021). Leaf manganese concentrations as a tool to assess belowground plant functioning in phosphorus-impoverished environments. Plant Soil 461, 43–61. doi: 10.1007/s11104-020-04690-2
Lešková, A., Giehl, R. F. H., Hartmann, A., Fargašová, A., von Wirén, N. (2017). Heavy metals induce iron deficiency responses at different hierarchic and regulatory levels. Plant Physiol. 174, 1648–1668. doi: 10.1104/pp.16.01916
Li, P., Song, A., Li, Z., Fan, F., Liang, Y. (2012). Silicon ameliorates manganese toxicity by regulating manganese transport and antioxidant reactions in rice (Oryza sativa L.). Plant Soil 354, 407–419. doi: 10.1007/s11104-011-1076-4
Li, P., Song, A., Li, Z., Fan, F., Liang, Y. (2015). Silicon ameliorates manganese toxicity by regulating both physiological processes and expression of genes associated with photosynthesis in rice (Oryza sativa L.). Plant Soil 397, 289–301. doi: 10.1007/s11104-015-2626-y
Li, M., Song, Z., Li, Z., Qiao, R., Zhang, P., Ding, C., et al. (2022). Populus root exudates are associated with rhizosphere microbial communities and symbiotic patterns. Front. Microbiol. 13. doi: 10.3389/fmicb.2022.1042944
Liang, Y., Nikolic, M., Bélanger, R., Gong, H., Song, A. (2015). “Silicon uptake and transport in plants: physiological and molecular aspects,” in Silicon in Agriculture (Springer, Dordrecht), 69–82. doi: 10.1007/978-94-017-9978-2_4
Liu, Y., Song, X., Xu, Z., Wang, Y., Hou, X., Wang, Y., et al. (2024). Biomineralized manganese oxide mediated nitrogen-contained wastewater treatment. Biores. Technol. 400, 130689. doi: 10.1016/j.biortech.2024.130689
Ma, C., Ci, K., Zhu, J., Sun, Z., Liu, Z., Li, X., et al. (2021). Impacts of exogenous mineral silicon on cadmium migration and transformation in the soil-rice system and on soil health. Sci. Total Environ. 759, 143501. doi: 10.1016/j.scitotenv.2020.143501
Ma, J., Sheng, H., Li, X., Wang, L. (2016). iTRAQ-based proteomic analysis reveals the mechanisms of silicon-mediated cadmium tolerance in rice (Oryza sativa) cells. Plant Physiol. Biochem. 104, 71–80. doi: 10.1016/j.plaphy.2016.03.024
Mandlik, R., Thakral, V., Raturi, G., Shinde, S., Nikolić, M., Tripathi, D. K., et al. (2020). Significance of silicon uptake, transport, and deposition in plants. J. Exp. Bot. 71, 6703–6718. doi: 10.1093/jxb/eraa301
Millaleo, R., Reyes-Diaz, M., Ivanov, A. G., Mora, M. L., Alberdi, M. (2010). Manganese as essential and toxic element for plants: transport, accumulation and resistance mechanisms. J. Soil Sci. Plant Nutr. 10, 470–481. doi: 10.4067/s0718-95162010000200008
Mitani-Ueno, N., Ma, J. F. (2021). Linking transport system of silicon with its accumulation in different plant species. Soil Sci. Plant Nutr. 67, 10–17. doi: 10.1080/00380768.2020.1845972
Nazeri, N. K., Lambers, H., Tibbett, M., Ryan, M. H. (2014). Moderating mycorrhizas: arbuscular mycorrhizas modify rhizosphere chemistry and maintain plant phosphorus status within narrow boundaries. Plant Cell Environ. 37, 911–921. doi: 10.1111/pce.12207
Oliveira, K. S., de Mello Prado, R., Checchio, M. V., Gratão, P. L. (2021). Silicon via nutrient solution modulates deficient and sufficient manganese sugar and energy cane antioxidant systems. Sci. Rep. 11, 16900. doi: 10.1038/s41598-021-96427-z
Oliveira, K. S., de Mello Prado, R., Checchio, M. V., Gratão, P. L. (2022). Interaction of silicon and manganese in nutritional and physiological aspects of energy cane with high fiber content. BMC Plant Biol. 22, 374. doi: 10.1186/s12870-022-03766-8
Pang, Z., Yin, W., Wang, Y., Zeng, W., Peng, H., Liang, Y. (2023). Silicon-phosphorus pathway mitigates heavy metal stress by buffering rhizosphere acidification. Sci. Total Environ. 904, 166887. doi: 10.1016/j.scitotenv.2023.166887
Pavlovic, J., Kostic, L., Bosnic, P., Kirkby, E. A., Nikolic, M. (2021). Interactions of silicon with essential and beneficial elements in plants. Front. Plant Sci. 12. doi: 10.3389/fpls.2021.697592
Qu, Q., Li, Y., Zhang, Z., Cui, H., Zhao, Q., Liu, W., et al. (2021). Effects of S-metolachlor on wheat (Triticum aestivum L.) seedling root exudates and the rhizosphere microbiome. J. Hazard. Mater. 411, 125137. doi: 10.1016/j.jhazmat.2021.125137
Sasaki, A., Yamaji, N., Yokosho, K., Ma, J. F. (2012). Nramp5 is a major transporter responsible for manganese and cadmium uptake in rice. Plant Cell 24, 2155–2167. doi: 10.1105/tpc.112.096925
Schmidt, S. B., Jensen, P. E., Husted, S. (2016). Manganese deficiency in plants: The impact on photosystem II. Trends Plant Sci. 21, 622–632. doi: 10.1016/j
Shao, J. F., Yamaji, N., Shen, R. F., Ma, J. F. (2017). The key to Mn homeostasis in plants: regulation of Mn transporters. Trends Plant Sci. 22, 215–224. doi: 10.1016/j.tplants.2016.12.005
Sharma, S. S., Dietz, K. J., Mimura, T. (2016). Vacuolar compartmentalization as indispensable component of heavy metal detoxification in plants. Plant Cell Environ. 39, 1112–1126. doi: 10.1111/pce.12706
Sheng, H., Chen, S. (2020). Plant silicon-cell wall complexes: Identification, model of covalent bond formation and biofunction. Plant Physiol. Biochem. 155, 13–19. doi: 10.1016/j.plaphy.2020.07.020
Sheng, H., Lei, Y., Wei, J., Yang, Z., Peng, L., Li, W., et al. (2024). Analogy of silicon and boron in plant nutrition. Front. Plant Sci. 15. doi: 10.3389/fpls.2024.1353706
Sheng, H., Li, Y., Feng, J., Liu, Y. (2023). Regulation of thermodynamics and kinetics of silica nucleation during the silicification process in higher plants. Plant Physiol. Biochem. 198, 107674. doi: 10.1016/j.plaphy.2023.107674
Sheng, H., Ma, J., Pu, J., Wang, L. (2018). Cell wall-bound silicon optimizes ammonium uptake and metabolism in rice cells. Ann. Bot. 122, 303–313. doi: 10.1093/aob/mcy068
Shi, X., Zhao, Y., Xu, M., Ma, L., Adams, J. M., Shi, Y. (2024). Insight into Plant-Microbe Interactions in rhizosphere for Sustainable Agriculture in the New Crops Era. New Crops 1, 100004. doi: 10.1016/j.ncrops.2023.11.002
Ueno, D., Sasaki, A., Yamaji, N., Miyaji, T., Fujii, Y., Takemoto, Y., et al. (2015). A polarly localized transporter for efficient manganese uptake in rice. Nat. Plants 1, 1–8. doi: 10.1038/nplants.2015.170
Vaculík, M., Landberg, T., Greger, M., Luxová, M., Stoláriková, M., Lux, A. (2012). Silicon modifies root anatomy, and uptake and subcellular distribution of cadmium in young maize plants. Ann. Bot. 110, 433–443. doi: 10.1093/aob/mcs039
Van der Ent, A., Casey, L. W., Blamey, F. P. C., Kopittke, P. M. (2020). Time-resolved laboratory micro-X-ray fluorescence reveals silicon distribution in relation to manganese toxicity in soybean and sunflower. Ann. Bot. 126, 331–341. doi: 10.1093/aob/mcaa081
Vives-Peris, V., De Ollas, C., Gómez-Cadenas, A., Pérez-Clemente, R. M. (2020). Root exudates: from plant to rhizosphere and beyond. Plant Cell Rep. 39, 3–17. doi: 10.1007/s00299-019-02447-5
Wang, X., Xie, G. J., Tian, N., Dang, C. C., Cai, C., Ding, J., et al. (2022). Anaerobic microbial manganese oxidation and reduction: a critical review. Sci. Total Environ. 822, 153513. doi: 10.1016/j.scitotenv.2022.153513
Wolf, S. (2022). Cell wall signaling in plant development and defense. Ann. Rev. Plant Biol. 73, 323–353. doi: 10.1146/annurev-arplant-102820-095312
Wu, J., Guo, J., Hu, Y., Gong, H. (2015). Distinct physiological responses of tomato and cucumber plants in silicon-mediated alleviation of cadmium stress. Front. Plant Sci. 6. doi: 10.3389/fpls.2015.00453
Wu, J., Mock, H. P., Giehl, R. F., Pitann, B., Mühling, K. H. (2019). Silicon decreases cadmium concentrations by modulating root endodermal suberin development in wheat plants. J. Hazard. Mater. 364, 581–590. doi: 10.1016/j.jhazmat.2018.10.052
Yan, L., Tang, D., Pang, J., Lambers, H. (2024). Root carboxylate release is common in phosphorus-limited forest ecosystems in China: using leaf manganese concentration as a proxy. Plant Soil 1-16. doi: 10.1007/s11104-024-06791-8
Keywords: beneficial element, Mn disorder, Mn bioavailability, subcellular distribution, scavenging ROS
Citation: Hailai Y, Liu Y, Yang Z, Li Y, Feng J, Li W and Sheng H (2024) Silicon regulation of manganese homeostasis in plants: mechanisms and future prospective. Front. Plant Sci. 15:1465513. doi: 10.3389/fpls.2024.1465513
Received: 16 July 2024; Accepted: 20 November 2024;
Published: 05 December 2024.
Edited by:
Renato De Mello Prado, São Paulo State University, BrazilReviewed by:
Vinicius De Oliveira, University of Reading, United KingdomCopyright © 2024 Hailai, Liu, Yang, Li, Feng, Li and Sheng. This is an open-access article distributed under the terms of the Creative Commons Attribution License (CC BY). The use, distribution or reproduction in other forums is permitted, provided the original author(s) and the copyright owner(s) are credited and that the original publication in this journal is cited, in accordance with accepted academic practice. No use, distribution or reproduction is permitted which does not comply with these terms.
*Correspondence: Zhengming Yang, NzkwNzIxOTE4QHFxLmNvbQ==; Huachun Sheng, aHVhY2h1bnNoZW5nOTlAMTI2LmNvbQ==
†These authors share first authorship
‡ORCID: Huachun Sheng, orcid.org/0000-0002-4684-5055
Disclaimer: All claims expressed in this article are solely those of the authors and do not necessarily represent those of their affiliated organizations, or those of the publisher, the editors and the reviewers. Any product that may be evaluated in this article or claim that may be made by its manufacturer is not guaranteed or endorsed by the publisher.
Research integrity at Frontiers
Learn more about the work of our research integrity team to safeguard the quality of each article we publish.