- 1School of Resources and Environmental Engineering, Anhui University, Hefei, China
- 2Anhui Shengjin Lake Wetland Ecology National Long-term Scientific Research Base, Dongzhi, China
- 3Anhui Province Key Laboratory of Wetland Ecosystem Protection and Restoration, Anhui University, Hefei, China
- 4Baiyangdian Basin Ecological Environment Monitoring Center, Baoding, China
Sediment accretion (burial) and nutrient enrichment may exert a synergistic influence on the growth and distribution of macrophytes in floodplain wetlands; however, this phenomenon has rarely been examined. In this study, we investigated the effects of sediment accretion and nutrient enrichment on the growth and vegetative propagation of Phalaris arundinacea within a Carex thunbergii stand (one P. arundinacea ramet within 25 C. thunbergii ramets) using a factorial sediment burial (0, 3, and 6 cm) and nutrient addition (low, medium, and high) experimental design. High sediment burial (6 cm) without nutrient addition decreased the aboveground and total biomass of C. thunbergii but did not affect P. arundinacea, indicating that P. arundinacea is more tolerant to sediment burial than C. thunbergii. Moderate sediment burial (3 cm) with nutrient addition enhanced the aboveground and total biomass of P. arundinacea but did not affect C. thunbergii, indicating that P. arundinacea may gain a growth advantage over C. thunbergii under moderate sedimentation. High sediment burial with nutrient addition increased the number of rhizomes and ramets produced by P. arundinacea but did not affect C. thunbergii, indicating that the relative abundance of P. arundinacea may increase within the Carex community under high sedimentation conditions. Based on these results, it can be concluded that an increased sedimentation rate facilitates the invasion of P. arundinacea into Carex grasslands, and this invasion is further enhanced by nutrient enrichment. Therefore, management measures should be taken to reduce the sediment load and nutrient input to prevent Carex grasslands invasion by P. arundinacea and maintain the ecological function of floodplain wetlands.
1 Introduction
Macrophyte vegetation in floodplain wetlands plays crucial ecological roles such as controlling sediment nutrients and providing food sources and habitats for wildlife (Dar et al., 2020; Dou et al., 2023). Macrophytic species are typically distributed along hydrological gradients and exhibit dynamic zonal patterns (Zhang et al., 2020). Hydrological conditions and sediment properties influence their growth and distribution, thereby influencing the ecological function of floodplain wetlands (Chen et al., 2014; Liu et al., 2018; Zhang et al., 2020; Jing et al., 2021; Moritz et al., 2022).
Flood regimes and sediment properties have changed considerably in many freshwater environments because of climatic changes and anthropogenic activities, which may influence the distribution patterns of macrophytes (Blakey et al., 2017; Chen et al., 2018; Ji et al., 2021; Huang et al., 2022). In floodplain wetlands, sedimentation co-occurs with flooding (Maun, 1998). After flooding, sediments are deposited on floodplain wetlands, ranging in depth from a few millimeters to several centimeters per year (Ou et al., 2019; Baniya et al., 2020; Geng et al., 2021; Kretz et al., 2021; Huang et al., 2022; Zeng et al., 2023). Wetland macrophytes with different growth forms respond differently to sediment accretion and have evolved various morphological and physiological strategies to acclimate to sediment burial stress (Li et al., 2015). Caulescent macrophytes, such as Phalaris arundinacea and Polygonum hydropiper, elongate the internode length, release dominant buds on their stems, and increase stem biomass to escape sediment accretion (Chen et al., 2014, 2017a). Non-caulescent macrophytes, such as Carex brevicuspis and Vallisneria natans, may not respond as successfully to sediment accretion as caulescent macrophytes (Pan et al., 2012). Thus, the differential responses to sediment accretion among macrophyte species may alter macrophyte distribution (Owen et al., 2004).
Nutrients in floodwater, such as nitrogen and phosphorus, may be retained in the sediment after flooding, facilitating the acclimation of wetland macrophytes to sediment stress (Ou et al., 2019; Huang et al., 2022; Zeng et al., 2023). Nutrients in the sediment may play an important role in stimulating plant growth following sediment burial. However, their effects on macrophyte growth vary from positive to negative, particularly following deep sediment burial (Chen et al., 2017b). Therefore, the effects of sediment nutrient enrichment on growth and vegetative reproduction may differ among macrophyte species.
Moreover, sediment accretion and nutrient enrichment are concurrent processes that may exert a synergistic influence on the growth and distribution of macrophytes but have rarely been examined. In this study, we investigated the effects of sediment accretion and nutrient enrichment on the growth and propagation of Phalaris arundinacea in a C. thunbergii stand. Carex is the dominant species in the floodplain wetlands of the Yangtze River basin, with P. arundinacea sparsely distributed within the Carex vegetation (Jing et al., 2017). Recently, the abundance and distribution of P. arundinacea has increased, especially in lacustrine wetlands with considerable sedimentation (Nelson and Anderson, 2015). Sediment accretion and nutrient enrichment are potential drivers of these changes, although empirical evidence supporting this is limited. We tested two hypotheses: (1) P. arundinacea can tolerate higher sediment accretion than C. thunbergii because it can escape sediment burial more efficiently than C. thunbergii, and (2) P. arundinacea may benefit more from nutrient enrichment than C. thunbergii because it can utilize nutrients more efficiently than C. thunbergii (Wetzel and van der Valk, 1998; Nelson and Anderson, 2015).
2 Materials and methods
2.1 Study site
Shengjin Lake (30°15′-30°30′N, 116°55′-117°15′E), located in the lower reaches of the Yangtze River, was listed as an internationally important wetland in 2015 (Wang et al., 2018). Influenced by the subtropical monsoon climate, wetlands tend to be inundated from June to October and are exposed from November to May. Carex thunbergii is the dominant plant community in the water-level fluctuation zone of this wetland.
2.2 Study species
Carex thunbergii is a rhizomatous sedge widely distributed in lakes and wet grasslands of eastern Asia (Dai et al., 2010). The pseudoculms of C. thunbergii have overlapping sheaths and are usually 40–100 cm high. Carex thunbergii often forms a monodominant community with a coverage of approximately 100% (Jia et al., 2020). The aboveground shoots of C. thunbergii are submerged and decompose during the flooding season, and new shoots emerge from the belowground rhizome buds immediately after the floodwaters recede (Chen et al., 2014).
Phalaris arundinacea is widely distributed in riparian and lacustrine wetlands in the subtropical and temperate regions of the Northern Hemisphere (Wu and Phillips, 2006). The erect culms are reed-like and 60–150 cm in height. They produce extensively spread rhizomes, enabling them to reproduce vigorously and spread aggressively (Wu and Phillips, 2006; Chen et al., 2017a). In the Carex community, P. arundinacea is a commonly observed companion species; however, its relative abundance and importance have increased considerably in recent times, which may affect the structure and function of Carex grasslands (Chen et al., 2017a).
2.3 Experimental design
The experiment was conducted at the Shengjin Lake Station for Wetland Ecosystem Research, Dongzhi County, Anhui Province, China (Figure 1). Before the experiment, we surveyed the ramet density of P. arundinacea in Carex grasslands in Shengjin Lake. The ramet density of P. arundinacea ranges from 1 to 112 ramets m-2, and density of C. thunbergii ranges from 400 to 2240 ramets m-2. Then, young ramets with soil from the Yaozui section of the lake were dug up and transported to the research station on April 3, 2023. Ramets were cultivated in a nursery bed containing a 10 cm soil/sand mixture (1:1 v/v) sourced from the Shengjin Lake. On April 10, one P. arundinacea ramet and 25 C. thunbergii ramets of similar heights (4–6 leaves and 14–20 cm in height) were planted in plastic buckets containing wetland soil 15 cm deep (each plastic bucket was 20 cm in diameter and 30 cm in height). There are many small holes (< 3 mm) at the bottom of the buckets, which allowed water to penetrate. The densities of C. thunbergii and P. arundinacea in the experiment were 796 and 32 ramets m-2, respectively, which were within the density range observed in the field.
The experimental design was a randomized block with five replicates (Figure 2). The experiment was conducted in separate outdoor water tanks (98 × 76 × 68 cm). Three sediment burial depths (0, 3, and 6 cm) and three levels of nutrient addition (low, medium, and high) were used in the experiment. The low-nutrient sediment was sand collected from Shengjin Lake, which contained 0.03 mg kg-1 total nitrogen, 0.02 mg kg-1 total phosphorus, and 0.48 mg kg-1 total potassium. The medium- and high-nutrient sediments were created by mixing lake sand homogeneously with 1 or 2 g of Osmocote slow-release fertilizer (501 Osmocote Plus [N-P-K, 15-10-12 + 2 MgO + TE ICL], Belgium N.V.). For the 3 and 6 cm sediment accretion with nutrient addition treatments, medium- or high-nutrient sand was added to the container to the corresponding depth. For the 0 cm sediment accretion with nutrient addition treatment, 1 or 2 g of slow-release fertilizer was added to the soil surface and covered with a thin layer of sand (< 0.2 cm). The water level in the tanks was maintained at 15 cm (0 cm for each plant) during the experiment. Plants were monitored weekly, and new ramets were marked with plastic labels.
2.4 Harvest and measurement
Plants were harvested on August 29, 2023, 20 weeks after treatment. The plants were carefully removed from the plastic containers to ensure the integrity of the aboveground and belowground parts. The plants were washed with tap water to remove attached sediment. Ramet height, root length, and number of ramets and rhizomes of each P. arundinacea parent ramet were measured. We also measured the number of rhizomes and ramets produced by each C. thunbergii parent ramet in the plastic containers. We then selected five C. thunbergii parent ramets from each container to measure the ramet height and root length. The ramets of P. arundinacea and C. thunbergii were separated into roots, shoots, and leaves. The biomass of each plant part was measured after drying at 85°C for 48 h in an oven.
2.5 Data analysis
Two-way ANOVA was performed to evaluate the main effects and interactions of sediment burial depth and nutrient addition levels on biomass accumulation, biomass allocation, ramet height, root length, and the number of new ramets and rhizomes. Multiple comparisons of the means were performed using Tukey’s test at a significance level of 0.05. Data were log10-transformed, if necessary, to reduce the heterogeneity of variance, and homogeneity was confirmed using Levene’s test. All analyses were performed using the “car” and “multcomp” package in R program (v. 4.1.2; R Core Team 2020).
3 Results
3.1 Biomass accumulation and allocation
Aboveground, belowground, and total biomass of P. arundinacea were significantly affected by burial depth and nutrient levels (P < 0.01; Table 1). Sediment accretion increased the aboveground, belowground, and total biomass of P. arundinacea growing in medium- and high-nutrient sediments (Figures 3A–C). Nutrient addition increased the aboveground and total biomass of P. arundinacea growing in the 3 and 6 cm sediment burial treatments (Figures 3A, B).
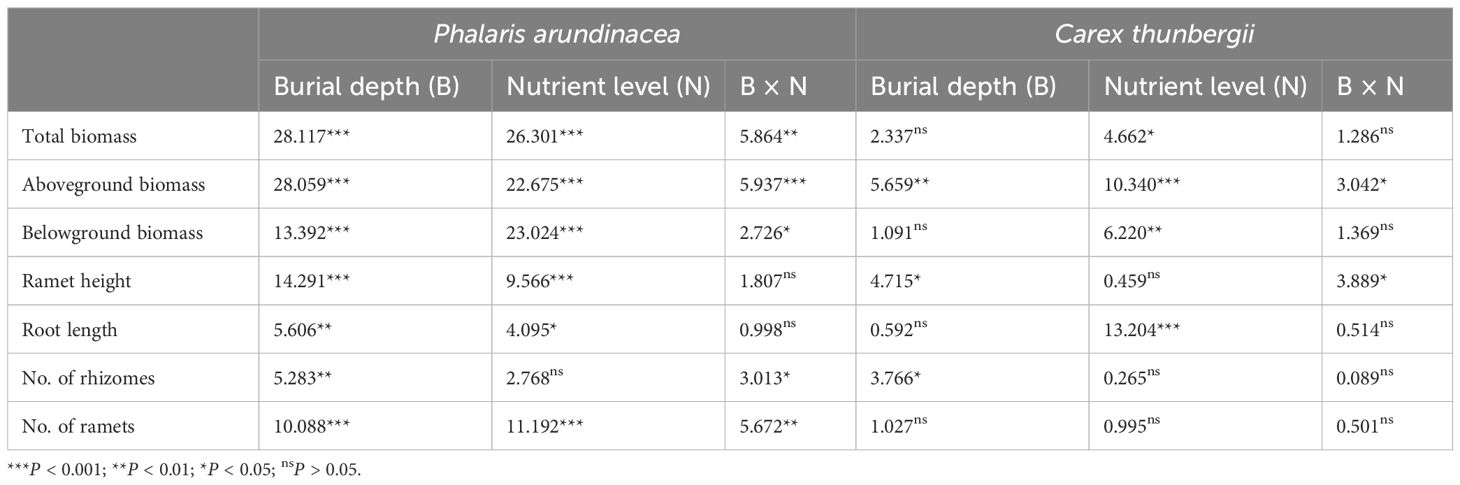
Table 1. Summary of two-way ANOVA (F-values) for total biomass, aboveground biomass, belowground biomass, ramet height, root length, and number of rhizomes and ramets in Phalaris arundinacea and Carex thunbergii grown at three sedimentation depths and three nutrient levels.
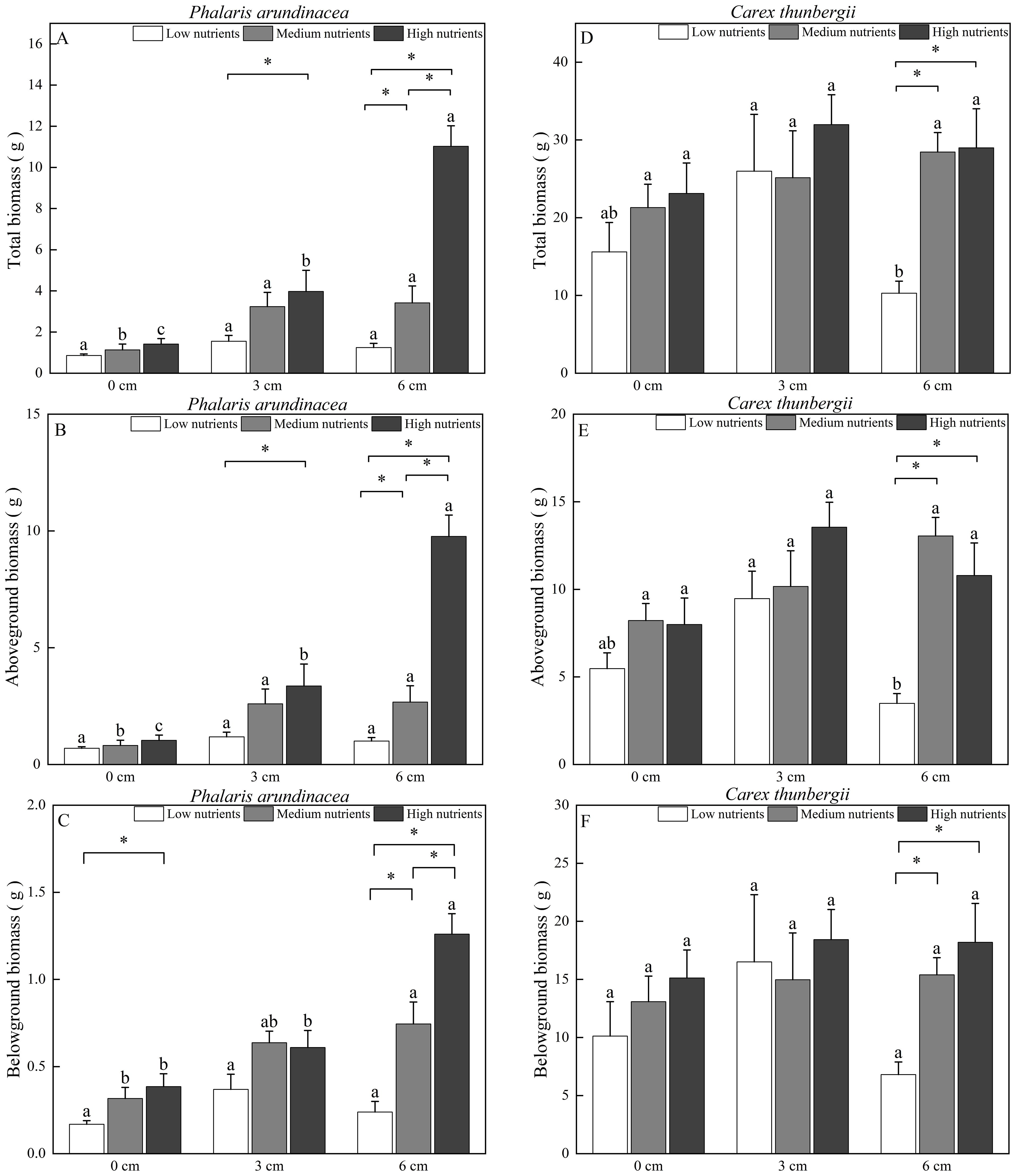
Figure 3. Biomass accumulation in Phalaris arundinacea and Carex thunbergii at three sediment burial depths with three nutrient levels. Total biomass, Aboveground biomass and Belowground biomass of Phalaris arundinacea (A–C), Total biomass, Aboveground biomass and Belowground biomass of Carex thunbergii (D–F). Different lowercase letters indicate significant differences among burial depth treatments at the same nutrient level (P < 0.05). * indicates significant differences among different nutrient level treatments at the same sediment burial depth (P < 0.05).
The total and belowground biomass of C. thunbergii were only affected by the nutrient levels (Table 1). Nutrient addition increased the total and belowground biomass of C. thunbergii growing in the 6 cm sediment burial treatment (Figures 3D, F). The aboveground biomass of C. thunbergii was significantly affected by burial depth and nutrient levels (P < 0.05; Table 1). When growing in low-nutrient sediment, the aboveground biomass of C. thunbergii was higher in the 3 cm sediment layer than in the 6 cm sediment layer (Figure 3E). Nutrient addition increased the aboveground biomass of C. thunbergii growing in the 6 cm sediment burial treatment (Figure 3E).
The ratio of shoot biomass of P. arundinacea to the shoot biomass of C. thunbergii ranges from 9.04% to 48.47% across treatments (Figure 4A). The ratio of root biomass of P. arundinacea to the root biomass of C. thunbergii ranges from 2.23% to 7.28% across treatments (Figure 4B). The ratio of total biomass of P. arundinacea to the total biomass of C. thunbergii ranges from 5.17% to 28.78% across treatments (Figure 4C). The ratios of the shoot, root, and total biomass of P. arundinacea to the shoot, root, and total biomass of C. thunbergii were higher in the 6 cm sediment burial treatment with high nutrient addition than that in the 0 cm burial depth treatment without nutrient addition (Figures 4A–C).
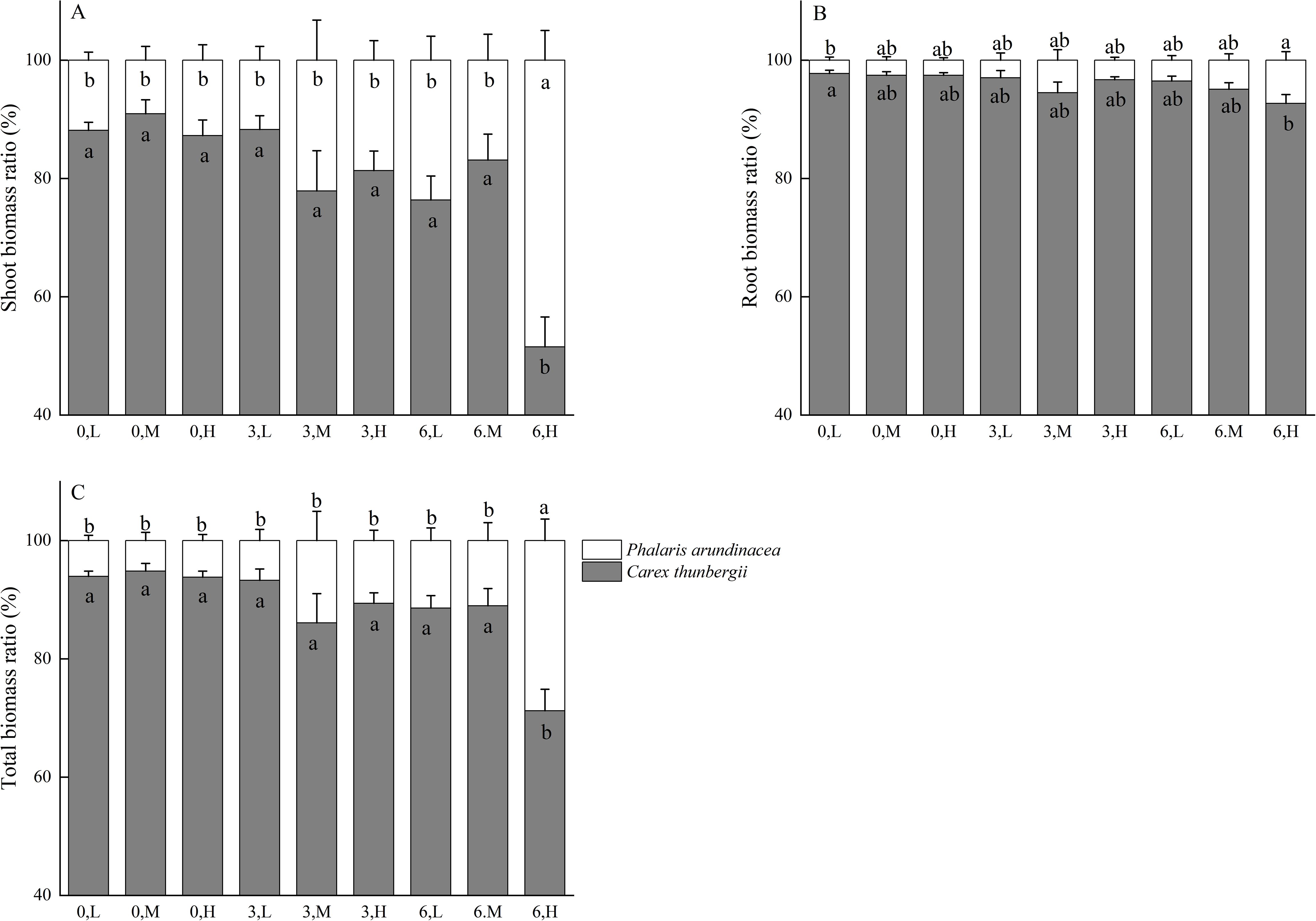
Figure 4. Shoot, root, and total mass ratio of Phalaris/Carex at three burial depths and three nutrient levels (A–C). Different lowercase letters indicate significant differences among the treatments (P < 0.05). Treatments: 0 cm burial depth with low nutrients (0, L); 0 cm burial depth with medium nutrients (0, M); 0 cm burial depth with high nutrients (0, H); 3 cm burial depth with low nutrients (3, L); 3 cm burial depth with medium nutrients (3, M); 3 cm burial depth with high nutrients (3, H); 6 cm burial depth with low nutrients (6, L); 6 cm burial depth with medium nutrients (6, M); and 6 cm burial depth with high nutrient levels (6, H).
3.2 Ramet height and root length
The ramet height of P. arundinacea was affected by burial depth and nutrient levels (P < 0.05; Table 1). When growing in high-nutrient sediments, the ramet height of P. arundinacea increased with sediment depth. Nutrient addition increased the ramet height of P. arundinacea in both the 3 and 6 cm burial depth treatments (Figure 5A). The ramet height of C. thunbergii was affected by burial depth and had a significant interaction with nutrient levels (Table 1). Without nutrient addition, the ramet height of C. thunbergii was shorter in the 6 cm burial depth treatment than that in the 3 cm burial depth treatment (Figure 5C). At a sediment burial depth of 6 cm, addition of nutrients increased the ramet height of C. thunbergii (Figure 5C).
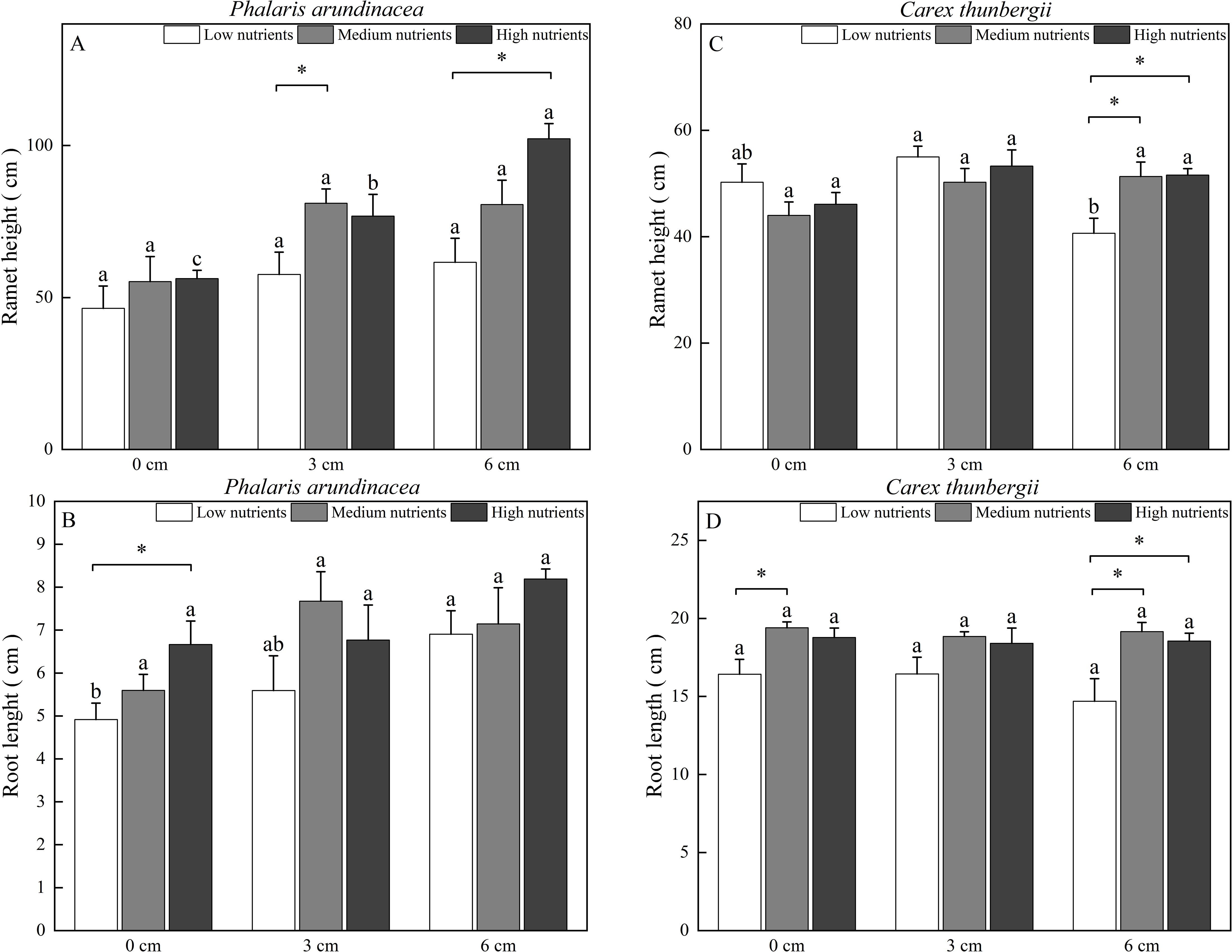
Figure 5. Ramet height and root length of Phalaris arundinacea (A, B) and Carex thunbergii (C, D) growing at three sediment burial depths with three nutrient levels. Different lowercase letters indicate significant differences among burial depth treatments at the same nutrient level (P < 0.05). * indicates significant differences among different nutrient level treatments at the same sediment burial depth (P < 0.05).
The root length of P. arundinacea was affected by burial depth and nutrient levels (P < 0.05; Table 1). Without nutrient addition, the root length of P. arundinacea was greater in the 6 cm sediment burial treatment than that in 0 cm burial depth treatment (Figure 5B). Without sediment burial, nutrient addition increased the root length of P. arundinacea (Figure 5B). The root length of C. thunbergii was only affected by nutrient levels (Table 1). At a sediment burial depth of 6 cm, nutrient addition increased the root length of C. thunbergii (Figure 5D).
3.3 Number of rhizomes and ramets
The number of rhizomes produced by P. arundinacea was significantly affected by the sediment burial depth, with significant interactions with nutrient levels (P < 0.05; Table 1). When grown under medium nutrient conditions, P. arundinacea produced more rhizomes in the 6 cm burial depth treatment than in the 0 cm burial depth treatment (Figure 6A). Nutrient addition increased the number of rhizomes produced by P. arundinacea when subjected to 3 and 6 cm sediment burial (Figure 6A). The number of rhizomes and ramets produced by C. thunbergii was neither affected by burial depth or nutrient levels (Figures 6C, D).
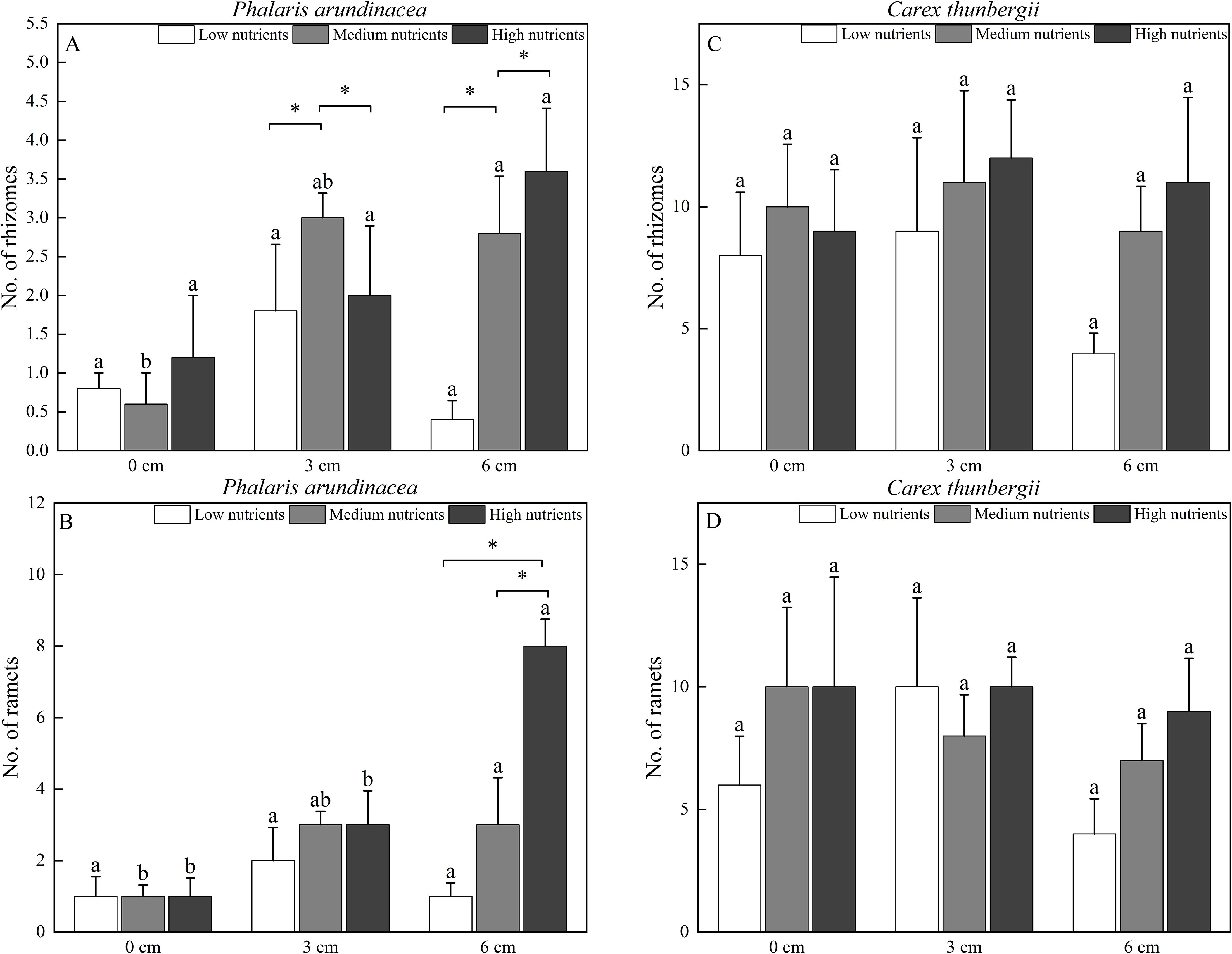
Figure 6. The number of rhizomes and ramets of Phalaris arundinacea (A, B) and Carex thunbergii (C, D) growing at three sediment burial depths with three nutrient levels. Different lowercase letters indicate significant differences among burial depth treatments at the same nutrient level (P < 0.05). * indicates significant differences among different nutrient level treatments at the same sediment burial depth (P < 0.05).
The number of ramets produced by P. arundinacea was significantly affected by burial depth and nutrient levels (P < 0.05; Table 1). With the addition of nutrients, the number of ramets produced by P. arundinacea was higher in the 6 cm burial depth treatment than in the 0 cm burial depth treatment (Figure 6B). At a burial depth of 6 cm, addition of nutrients increased the number of ramets produced by P. arundinacea (Figure 6B). The number of ramets produced by C. thunbergii was neither affected by burial depth or nutrient levels (Table 1; Figure 6D).
4 Discussion
4.1 High sediment accretion inhibited C. thunbergii growth but did not affect P. arundinacea
In our study, 6 cm sediment burial without nutrient addition decreased the aboveground and total biomass of C. thunbergii but had no effect on P. arundinacea (Figures 3A–D). As a non-stem sedge, C. thunbergii can escape sediment burial by producing spreading ramets via rhizome elongation (Li et al., 2015). However, projecting new ramets onto a sediment surface consumes large quantities of carbohydrate reserves (Pan et al., 2012; Chen et al., 2017b). Consequently, the biomass of C. thunbergii decreases with heavy sediment burial. In contrast, P. arundinacea can escape sediment burial through rapid stem growth and internode elongation (Chen et al., 2014, 2017a). Therefore, P. arundinacea can tolerate higher sediment accretion than C. thunbergii, which is consistent with hypothesis 1. Hypothesis 1 was also supported by the higher proportional biomass of P. arundinacea to C. thunbergii in the heavy sedimentation treatment (Figure 4C).
4.2 Moderate sedimentation enhanced P. arundinacea growth but did not affect C. thunbergii
Our results showed that moderate sediment burial with nutrients enhanced the aboveground and total biomass of P. arundinacea but had no effect on C. thunbergii. Studies have indicated that moderate sedimentation stimulates macrophyte growth (Maun, 1998; Gilbert and Ripley, 2010; Chen et al., 2017a; Fan et al., 2018). For example, moderate sedimentation increases the growth of Spartina patens, Scirpus mariqueter, and S. alterniflora (Halun et al., 2002; Matzke and Elsey-Quirk, 2018; Xiao et al., 2023). The stimulation of macrophyte growth upon sedimentation may be attributed to increased soil volume, nutrients, moisture, and microbial activity (Maun, 1998). For P. arundinacea, increased nutrient availability may have been the primary contributor stimulating growth during sediment burial (Katagiri et al., 2011; Chen et al., 2017a).
Nevertheless, we did not observe a stimulatory effect of moderate sedimentation on C. thunbergii growth. Non-caulescent Carex species adapt to sedimentation by changing their clonal growth from economically clumped ramets to costively spreading ramets (Bernard, 1990; Chen et al., 2010; Li et al., 2015). Even with moderate sediment burial (3 cm), the proportion of spreading ramets produced by C. brevicuspis increased significantly, indicating that Carex species were sensitive to sediment burial. Furthermore, the increased growth of P. arundinacea after sediment deposition may have suppressed the growth of native species through light interception (Kercher and Zedler, 2004). Phalaris arundinacea can effectively utilize resources such as light and sediment nutrients to produce additional ramets, enhancing its competitive capacity (Martina and von Ende, 2012; Chen et al., 2017a; Winikoff et al., 2020). Therefore, P. arundinacea may have a growth advantage over C. thunbergii under moderate sedimentation conditions.
4.3 Nutrient enrichment increased the vegetative propagation of P. arundinacea but not C. thunbergii
Our results indicated that high sediment accretion with nutrients increased the number of rhizomes and ramets produced by P. arundinacea but did not affect C. thunbergii. This result is consistent with hypothesis 2: P. arundinacea benefits more from nutrient enrichment than C. thunbergii does. Other studies also found that nutrient addition increased the abundance and production of P. arundinacea in wet prairie assemblages during P. arundinacea invasion (Kercher and Zedler, 2004; Weston et al., 2021). As an opportunistic invader, P. arundinacea may efficiently utilize fluctuating resources and produce additional ramets to occupy its habitat (Davis et al., 2000; Kettenring et al., 2019). In contrast, Carex species may adopt a conservative reproductive strategy to manage resource availability, that is, produce a relatively constant number of rhizomes and ramets but change individual size (Chen et al., 2016). Therefore, with increasing nutrient input in floodplain wetlands, the abundance of P. arundinacea may increase in Carex grasslands.
5 Conclusion
Our study indicated that high sediment accretion inhibited the growth of C. thunbergii but had no effect on P. arundinacea. Moderate sedimentation enhanced the growth of P. arundinacea but did not affect C. thunbergii. Nutrient enrichment of sediments increased the vegetative propagation of P. arundinacea but did not affect C. thunbergii. From these results, we inferred that in floodplain wetlands, P. arundinacea may increase in abundance and coverage when growing in Carex grasslands subjected to sediment accretion and nutrient enrichment. Therefore, to maintain the ecological function of floodplain wetlands, management measures should be implemented to reduce sediment load and nutrient input to protect Carex grasslands from P. arundinacea invasion.
Data availability statement
The raw data supporting the conclusions of this article will be made available by the authors, without undue reservation.
Author contributions
XG: Data curation, Writing – original draft. JZ: Methodology, Writing – review & editing. WS: Data curation, Writing – review & editing. XS: Data curation, Investigation, Writing – review & editing. JL: Methodology, Writing – review & editing. XC: Conceptualization, Methodology, Writing – review & editing.
Funding
The author(s) declare financial support was received for the research, authorship, and/or publication of this article. This work was supported by the National Natural Science Foundation of China (32471657).
Conflict of interest
The authors declare that the research was conducted in the absence of any commercial or financial relationships that could be construed as a potential conflict of interest.
The author(s) declared that they were an editorial board member of Frontiers, at the time of submission. This had no impact on the peer review process and the final decision.
The reviewer ZD declared a past co-authorship with the author X-SC to the handling editor.
Publisher’s note
All claims expressed in this article are solely those of the authors and do not necessarily represent those of their affiliated organizations, or those of the publisher, the editors and the reviewers. Any product that may be evaluated in this article, or claim that may be made by its manufacturer, is not guaranteed or endorsed by the publisher.
References
Baniya, M. B., Asaeda, T., Fujino, T., Jayasanka, S., Muhetaer, G., Li, J. H. (2020). Mechanism of riparian vegetation growth and sediment transport interaction in floodplain: A dynamic riparian vegetation model (DRIPVEM) approach. Water 12, 77. doi: 10.3390/w12010077
Bernard, J. M. (1990). Life history and vegetative reproduction in Carex. Botany 68, 1441–1448. doi: 10.1139/B90-182
Blakey, R. V., Kingsford, R. T., Law, B. S., Stoklosa, J. (2017). Floodplain habitat is disproportionately important for bats in a large river basin. Biol. Conserv. 215, 1–10. doi: 10.1016/j.biocon.2017.08.030
Chen, X. S., Deng, Z. M., Xie, Y. H., Li, F., Hou, Z. Y., Li, X., et al. (2014). Effects of sediment burial disturbance on the vegetative propagation of Phalaris arundinacea with different shoot statuses. Aquat. Ecol. 48, 409–416. doi: 10.1007/s10452-014-9493-0
Chen, X. S., Deng, Z. M., Xie, Y. H., Li, F., Hou, Z. Y., Wu, C. (2016). Consequences of repeated defoliation on belowground bud banks of carex brevicuspis (Cyperaceae) in the dongting lake wetlands, China. Front. Plant Sci. 7. doi: 10.3389/fpls.2016.01119
Chen, M. S., Ding, S. M., Chen, X., Sun, Q., Fan, X. F., Lin, J., et al. (2018). Mechanisms driving phosphorus release during algal blooms based on hourly changes in iron and phosphorus concentrations in sediments. Water Res. 133, 153–164. doi: 10.1016/j.watres.2018.01.040
Chen, J. S., Lei, N. F., Dong, M. (2010). Clonal integration improves the tolerance of Carex praeclara to sand burial by compensatory response. Acta Oecologica-International J. Ecol. 36, 23–28. doi: 10.1016/j.actao.2009.09.006
Chen, X. S., Liao, Y. L., Xie, Y. H., Li, F., Deng, Z. M., Hou, Z. Y., et al. (2017b). Concurrent effects of sediment accretion and nutrient availability on the clonal growth strategy of Carex brevicuspis-A wetland sedge that produces both spreading and clumping ramets. Front. Plant Sci. 8. doi: 10.3389/fpls.2017.01685
Chen, X. S., Liao, Y. L., Xie, Y. H., Wu, C., Li, F., Deng, Z. M., et al. (2017a). The combined effects of sediment accretion (burial) and nutrient enrichment on the growth and propagation of Phalaris arundinacea. Sci. Rep. 7, 39963. doi: 10.1038/srep39963
Dai, L. K., Liang, S. Y., Zhang, S. R., Tang, Y. C., Koyama, T., Tucker, G. C., et al. (2010). Flora of China (Cyperaceae) Vol. 23 (Beijing: Science Press).
Dar, S. A., Bhat, S. U., Rashid, I., Dar, S. A. (2020). Current status of wetlands in srinagar city: threats, management strategies, and future perspectives. Front. Environ. Sci. 7. doi: 10.3389/fenvs.2019.00199
Davis, M., Grime, J., Thompson, K. (2000). Fluctuating resources in plant communities: A general theory of invasibility. J. Ecol. 88, 528–536. doi: 10.1046/j.1365-2745.2000.00473.x
Dou, X. Y., Guo, H. D., Zhang, L., Liang, D., Zhu, Q., Liu, X. T., et al. (2023). Dynamic landscapes and the influence of human activities in the Yellow River Delta wetland region. Sci. Total Environ. 899, 166239. doi: 10.1016/j.scitotenv.2023.166239
Fan, B. L., Zhao, C. M., Zhang, X. W., Sun, K. (2018). Impacts of sand burial and wind erosion on regeneration and growth of a desert clonal shrub. Front. Plant Sci. 9. doi: 10.3389/fpls.2018.01696
Geng, M. M., Wang, K. L., Yang, N., Li, F., Zou, Y. A., Chen, X. S., et al. (2021). Spatiotemporal water quality variations and their relationship with hydrological conditions in Dongting Lake after the operation of the Three Gorges Dam, China. J. Cleaner Production 283, 124644. doi: 10.1016/j.jclepro.2020.124644
Gilbert, M. E., Ripley, B. S. (2010). Resolving the differences in plant burial responses. Austral Ecol. 35, 53–59. doi: 10.1111/j.1442-9993.2009.02011.x
Halun, Z., Terrados, J., Borum, J., Kamp-Nielsen, L., Duarte, C. M., Fortes, M. D. (2002). Experimental evaluation of the effects of siltation-derived changes in sediment conditions on the Philippine seagrass Cymodocea rotundata. J. Exp. Mar. Biol. Ecol. 279, 73–87. doi: 10.1016/S0022-0981(02)00366-0
Huang, Y., Chen, X. S., Li, F., Hou, Z. Y., Li, X., Zeng, J., et al. (2022). Concurrent effects of flooding regimes and floodwater quality on sediment properties in a Yangtze River-connected floodplain wetland: Insights from field investigations during 2011–2020. Sci. Total Environ. 827, 154225. doi: 10.1016/j.scitotenv.2022.154225
Ji, Z. H., Long, Z. W., Zhang, Y., Wang, Y. K., Qi, X. Y., Xia, X. H., et al. (2021). Enrichment differences and source apportionment of nutrients, stable isotopes, and trace metal elements in sediments of complex and fragmented wetland systems. Environ. pollut. 289, 117852. doi: 10.1016/j.envpol.2021.117852
Jia, X., Huangfu, C. H., Hui, D. F. (2020). Nitrogen uptake by two plants in eesponse to plant competition as regulated by neighbor density. Front. Plant Sci. 11. doi: 10.3389/fpls.2020.584370
Jing, L., Lu, C., Xia, Y., Shi, L. L., Zuo, A. J., Lei, J. L., et al. (2017). Effects of hydrological regime on development of Carex wet meadows in East Dongting Lake, a Ramsar Wetland for wintering waterbirds. Sci. Rep. 7, 41761. doi: 10.1038/srep41761
Jing, C., Yunliang, L., Junfeng, Z., Jingyuan, L., Li, W., Yuyin, G. (2021). Assessing surface water-groundwater interactions in the seasonal lake-wetland system of Lake Poyang. J. Lake Sci. 33, 842–853. doi: 10.18307/2021.0317
Katagiri, K., Yabe, K., Nakamura, F., Sakurai, Y. (2011). Factors controlling the distribution of aquatic macrophyte communities with special reference to the rapid expansion of a semi-emergent Phalaris arundinacea L. @ in Bibi River, Hokkaido, northern Japan. Limnology 12, 175–185. doi: 10.1007/s10201-010-0335-z
Kercher, S. M., Zedler, J. B. (2004). Multiple disturbances accelerate invasion of reed canary grass (Phalaris arundinacea L.) in a mesocosm study. Oecologia 138, 455–464. doi: 10.1007/s00442-003-1453-7
Kettenring, K. M., Menuz, D. R., Mock, K. E. (2019). The nativity and distribution of the cryptic invader phalaris arundinacea (Reed canarygrass) in riparian areas of the Columbia and missouri river basins. Wetlands 39, 55–66. doi: 10.1007/s13157-018-1074-x
Kretz, L., Bondar-Kunze, E., Hein, T., Richter, R., Schulz-Zunkel, C., Seele-Dilbat, C., et al. (2021). Vegetation characteristics control local sediment and nutrient retention on but not underneath vegetation in floodplain meadows. PloS One 16, e0252694. doi: 10.1371/journal.pone.0252694
Li, F., Xie, Y. H., Zhu, L. L., Jiang, L., Chen, X. S., Pan, B. H., et al. (2015). Changed clonal growth form induced by sand burial facilitates the acclimation of carex brevicuspis to competition. PloS One 10, e0121270. doi: 10.1371/journal.pone.0121270
Liu, Z. Y., Zhao, L. N., Xu, T., Bu, F., Liu, X. M., Zhou, D. M. (2018). Quantification of potential flood inundation areas in the marsh wetland of Honghe National Natural Reserve, Northeast China. Ecohydrology Hydrobiology 18, 355–364. doi: 10.1016/j.ecohyd.2018.10.005
Martina, J. P., von Ende, C. N. (2012). Highly plastic response in morphological and physiological traits to light, soil-N and moisture in the model invasive plant, Phalaris arundinacea. Environ. Exp. Bot. 82, 43–53. doi: 10.1016/j.envexpbot.2012.03.010
Matzke, S., Elsey-Quirk, T. (2018). Spartina patens productivity and soil organic matter response to sedimentation and nutrient enrichment. Wetlands 38, 1233–1244. doi: 10.1007/s13157-018-1030-9
Maun, M. A. (1998). Adaptations of plants to burial in coastal sand dunes. Can. J. Bot. 76, 713–738. doi: 10.1139/b98-058
Moritz, C. M., Vepraskas, M. J., Ricker, M. C. (2022). Hydrology and vegetation relationships in a Carolina Bay Wetland 15 years after restoration. Wetlands 42, 8. doi: 10.1007/s13157-022-01530-0
Nelson, M. F., Anderson, N. O. (2015). Variation among genotypes and source habitats in growth and fecundity of the wetland invasive plant Phalaris arundinacea L. Wetlands 35, 1175–1184. doi: 10.1007/s13157-015-0704-9
Ou, Y., Rousseau, A. N., Wang, L. X., Yan, B. X., Gumiere, T., Zhu, H. (2019). Identification of the alteration of riparian wetland on soil properties, enzyme activities and microbial communities following extreme flooding. Geoderma 337, 825–833. doi: 10.1016/j.geoderma.2018.10.032
Owen, N. W., Kent, M., Dale, D. M. (2004). Plant species and community responses to sand burial on the machair of the Outer Hebrides, Scotland. J. Vegetation Sci. 15, 669–678. doi: 10.1111/j.1654-1103.2004.tb02309.x
Pan, Y., Xie, Y. H., Chen, X. S., Li, F. (2012). Effects of flooding and sedimentation on the growth and physiology of two emergent macrophytes from Dongting Lake wetlands. Aquat. Bot. 100, 35–40. doi: 10.1016/j.aquabot.2012.03.008
R Core Team (2020). R: A Language and Environment for Statistical Computing. In: R Version, 4.0.1. R Foundation for Statistical Computing, Vienna, Austria.
Wang, C., Dong, B., Zhu, M., Huang, H., Zhao, K. K. (2018). Habitat selection of wintering crane in Shengjin Lake wetland. Chin. J. Ecol. 37, 810. doi: 10.13292/j.1000-4890.201803.020
Weston, L. M., Mattingly, K. Z., Day, C. T. C., Hovick, S. M. (2021). Potential local adaptation in populations of invasive reed canary grass (Phalaris arundinacea) across an urbanization gradient. Ecol. Evol. 11, 11457–11476. doi: 10.1002/ece3.7938
Wetzel, P. R., van der Valk, A. G. (1998). Effects of nutrient and soil moisture on competition between Carex stricta, Phalaris arundinacea, and Typha latifolia. Plant Ecol. 138, 179–190. doi: 10.1023/A:1009751703827
Winikoff, S. G., Larkin, D. J., Meier, S. L., Finlay, J. C. (2020). Vegetation trajectories of restored agricultural wetlands following sediment removal. Restor. Ecol. 28, 612–622. doi: 10.1111/rec.13128
Wu, Z. L., Phillips, S. (2006). Phalaris linnaeus, sp. Pl. 1:54. 1753. (Flora of China) 22, 335–336.
Xiao, M., Cai, T. L., Wang, X. K., Cheng, J., Liu, B., Xia, X. M., et al. (2023). Response of native and exotic saltmarsh species to sediment deposition addition. Sci. Total Environ. 888, 164271. doi: 10.1016/j.scitotenv.2023.164271
Zeng, L. H., Ji, J., Xu, S. Y., Cao, Y. M., Chen, X. (2023). Decoupling of nitrogen, phosphorus and biogenic silica in floodplain sediments in response to land use change and hydrological alterations. J. Hydrology 623, 129833. doi: 10.1016/j.jhydrol.2023.129833
Keywords: Carex grasslands, floodplain wetlands, macrophyte distribution, sediment deposition, vegetative propagation
Citation: Guo X-z, Zhong J-s, Sun W-j, Song X-r, Liu J and Chen X-s (2024) Sediment accretion and nutrient enrichment enhance the growth and vegetative propagation of Phalaris arundinacea growing within a Carex thunbergii stand. Front. Plant Sci. 15:1459663. doi: 10.3389/fpls.2024.1459663
Received: 04 July 2024; Accepted: 24 September 2024;
Published: 14 October 2024.
Edited by:
Igor Zelnik, University of Ljubljana, SloveniaReviewed by:
Zhengmiao Deng, Chinese Academy of Sciences (CAS), ChinaLi Wen, NSW Department of Planning, Industry and Environment, Australia
Copyright © 2024 Guo, Zhong, Sun, Song, Liu and Chen. This is an open-access article distributed under the terms of the Creative Commons Attribution License (CC BY). The use, distribution or reproduction in other forums is permitted, provided the original author(s) and the copyright owner(s) are credited and that the original publication in this journal is cited, in accordance with accepted academic practice. No use, distribution or reproduction is permitted which does not comply with these terms.
*Correspondence: Xin-sheng Chen, MjEyMjJAYWh1LmVkdS5jbg==