- 1Xianghu Laboratory, Hangzhou, China
- 2MEU Research Unit, Middle East University, Amman, Jordan
- 3Department of Life Sciences, Western Caspian University, Baku, Azerbaijan
- 4Institute of Nuclear Agricultural Sciences, Zhejiang University, Hangzhou, China
- 5Department of Plant Pathology, Faculty of Agriculture, University of Sargodha, Sargodha, Pakistan
- 6Department of Plant Sciences, College of Agricultural and Marine Sciences, Sultan Qaboos University, Muscat, Oman
- 7Department of Biology, College of Science, Sultan Qaboos University, Muscat, Oman
- 8Faculty of Agriculture, University of Zagreb, Zagreb, Croatia
Plant diseases caused by microbial pathogens pose a severe threat to global food security. Although genetic modifications can improve plant resistance; however, environmentally sustainable strategies are needed to manage plant diseases. Nano-enabled immunomodulation involves using engineered nanomaterials (ENMs) to modulate the innate immune system of plants and enhance their resilience against pathogens. This emerging approach provides unique opportunities through the ability of ENMs to act as nanocarriers for delivering immunomodulatory agents, nanoprobes for monitoring plant immunity, and nanoparticles (NPs) that directly interact with plant cells to trigger immune responses. Recent studies revealed that the application of ENMs as nanoscale agrochemicals can strengthen plant immunity against biotic stress by enhancing systemic resistance pathways, modulating antioxidant defense systems, activating defense-related genetic pathways and reshaping the plant-associated microbiomes. However, key challenges remain in unraveling the complex mechanisms through which ENMs influence plant molecular networks, assessing their long-term environmental impacts, developing biodegradable formulations, and optimizing targeted delivery methods. This review provides a comprehensive investigation of the latest research on nano-enabled immunomodulation strategies, potential mechanisms of action, and highlights future perspectives to overcome existing challenges for sustainable plant disease management.
1 Introduction
Agriculture is the most important sector that plays a crucial role in providing food, supporting economic stability, and maintaining ecological balance worldwide (Hartmann and Six, 2023). Plant pathogens, including fungi, bacteria, viruses, and other pathogens, pose a severe threat to agricultural productivity and food security worldwide (Kim et al., 2023). In the last few decades, due to trade globalization, climate change, and other factors, new pathogens are constantly emerging, and existing diseases are also spreading, posing a serious threat to agricultural production (Singh et al., 2023). Moreover, since the Green Revolution the global population has dramatically raised by more than 5 billion people, and the shortage of typical agricultural methods has critically restrained our capability to conserve food safety (Conway, 1998). Traditional methods such as broad-spectrum antibiotics and chemical pesticides provide some support; however, they also pose adverse effects to the environment and human health and can also lead to drug resistance in plant pathogens (Ahmed et al., 2022). To address these issues, traditional breeding and modern biotechnology are expected to significantly improve plant resilience to various pathogens by improving crop resistance genes and immune signaling pathways (Conway, 1998; Munaweera et al., 2022).
Although genetic modifications of plants offer promising advantages, these require risk assessment and careful consideration to confirm their safety and sustainability (Anders et al., 2021). Therefore, it is important to find new strategies for environmentally friendly and sustainable plant disease management. Enhancing crop resilience to pathogens is one of the key strategies to control plant diseases and maintain agricultural sustainability, while minimizing the reliance on traditional pesticides (Hannan Parker et al., 2022; Ngou et al., 2022). Notably, the innate immune system of plants provides a strong defense against pathogen invasion (Ma et al., 2021), but how to effectively regulate and enhance plant immunity remains to be established. In recent years, the application of nanotechnologies in agriculture has attracted increasing attention, due to their unique nanoscale-specific properties such as high efficiency, large surface area, small size, targeted delivery, and controlled release (Beckers et al., 2021).
Nano-enabled immunomodulation involves the use of engineered nanomaterials (ENMs) as nanoscale agrochemicals to modulate the immune response of plants to improve disease resistance (Ahmed et al., 2024; Zhang et al., 2024). ENMs can be used as nanocarriers to efficiently and precisely deliver immune signaling molecules or genes to specific locations in cells, thereby regulating the plant immune system (Zhang et al., 2021b). Additionally, nanoprobe technology is also used to monitor key biomolecular changes in plant immunity, and biotic and abiotic stress responses in real-time (Son et al., 2023). Recent studies have demonstrated that ENMs application strengthens the plant immunity and tolerance against biotic stress by enhancing systemic acquired resistance (SAR) and induced systemic resistance (ISR), and modulating antioxidative defense systems. For example, the foliar application of silica nanoparticles (SiNPs) 100 mg L−1 improved the disease resistance in Arabidopsis thaliana plants against bacterial pathogen Pseudomonas syringae by inducing the SAR in a dose-dependent manner. SAR-inducing phytohormone such as SA successfully enhances stress tolerance by upregulating the expression of pathogenesis-related genes (El-Shetehy et al., 2021). In another recent study, Noman et al. (2024) reported that salicylic acid (SA) coated biogenic iron nanoparticles (bio-FeNPs) at 100 mg Kg-1 concentration significantly suppressed Fusarium wilt disease in watermelon (Citrullus lanatus L.) caused by a fungal pathogen Fusarium oxysporum f. sp. niveum by improving SAR response via triggering antioxidative defense systems and SA signaling pathway genes. These findings suggest that nano-enabled immunomodulation might be an alternative way for enhancing plant resilience against phytopathogens; however, mechanistic insights and translation of these approaches from laboratory to the field scale involves significant challenges.
The aim of this review is to provide a comprehensive overview of the latest research progress in nano-enabled immunomodulation for enhancing plant resilience against phytopathogens threats. We aim to elucidate the potential mechanisms by which ENMs can modulate plant immune responses, critically evaluate the latest research advances, and highlight future challenges and opportunities for translating these approaches into sustainable agricultural practices.
2 Dynamics of immunomodulation in plants against phytopathogens
The coevolution of plants and microbial pathogens has led to an intricate interplay of defense and attack mechanisms (Fields and Friman, 2022; Harris and Mou, 2024). Microbial pathogens have developed strategies for evading or suppressing plant immune systems; however, plants employ various sophisticated defense mechanisms in response (Sun and Zhang, 2021). Notably, effector-triggered immunity (ETI) and pattern-triggered immunity (PTI) are two primary defense mechanisms of innate plant immunity against phytopathogens. In plants, NOD-like receptors (NLRs) constitute sensor and helper NLRs, which are responsible for ETI (Laflamme et al., 2020; Nabi et al., 2024). For example, Wang et al. (2023) reported that MPK3 and MPK6 suppression in Arabidopsis can potentially reduce pre-PTI-mediated ETI suppression (PES) through inhibition of two protein phosphatases genes (AP2C1 and PP2C5). Furthermore, recognition of conserved microbial features such as microbe-associated molecular patterns (MAMPs) through plant pattern recognition receptors (PRRs) can initiate PTI. However, phytopathogens can evade PTI by shielding or modifying MAMPs, inhibition of PRRs or downstream signaling components by secreting effectors (Lü et al., 2022; Loo et al., 2022; Totsline et al., 2023). Lee et al. (2024) reported that AVRblb2 pathogen effector forms a complex with calmodulin-like (CML) and calmodulin (CaM) proteins to interact with NbCNGC18 to disrupt PAMP-triggered immunity signaling. These mechanisms can potentially hijack plant defense systems, driven by the coevolutionary relationship between plants and phytopathogens (Sood et al., 2021; Harris and Mou, 2024).
Pathogen effectors can also play a central role in modulating plant immunity (Dou and Zhou, 2012), which target various components of plant immune system, from early recognition events to downstream signaling and defense responses (Figure 1). Effectors can prevent release, binding, or perception of MAMPs, or inhibit key signaling hubs such as receptor-like cytoplasmic kinases, interfere with PRR complexes, MAPK cascades, and phytohormone signaling pathways (Ceulemans et al., 2021; Iswanto et al., 2022). In a recent study, Qiu et al. (2023b) reported that inhibition of GmLHP1-2/GmPHD6 complex transcriptional activity in soybean due to suppressing effect of PsAvh110 nuclear effector from Phytophthora sojae can potentially evade plant immunity response. In another study, Shang et al. (2024) reported that CfEC12 (a fungal effector) from Colletotrichum fructicola binds to MdNIMIN2 and disrupting its interaction with MdNPR1 leading to suppression of salicylic acid defense pathway.
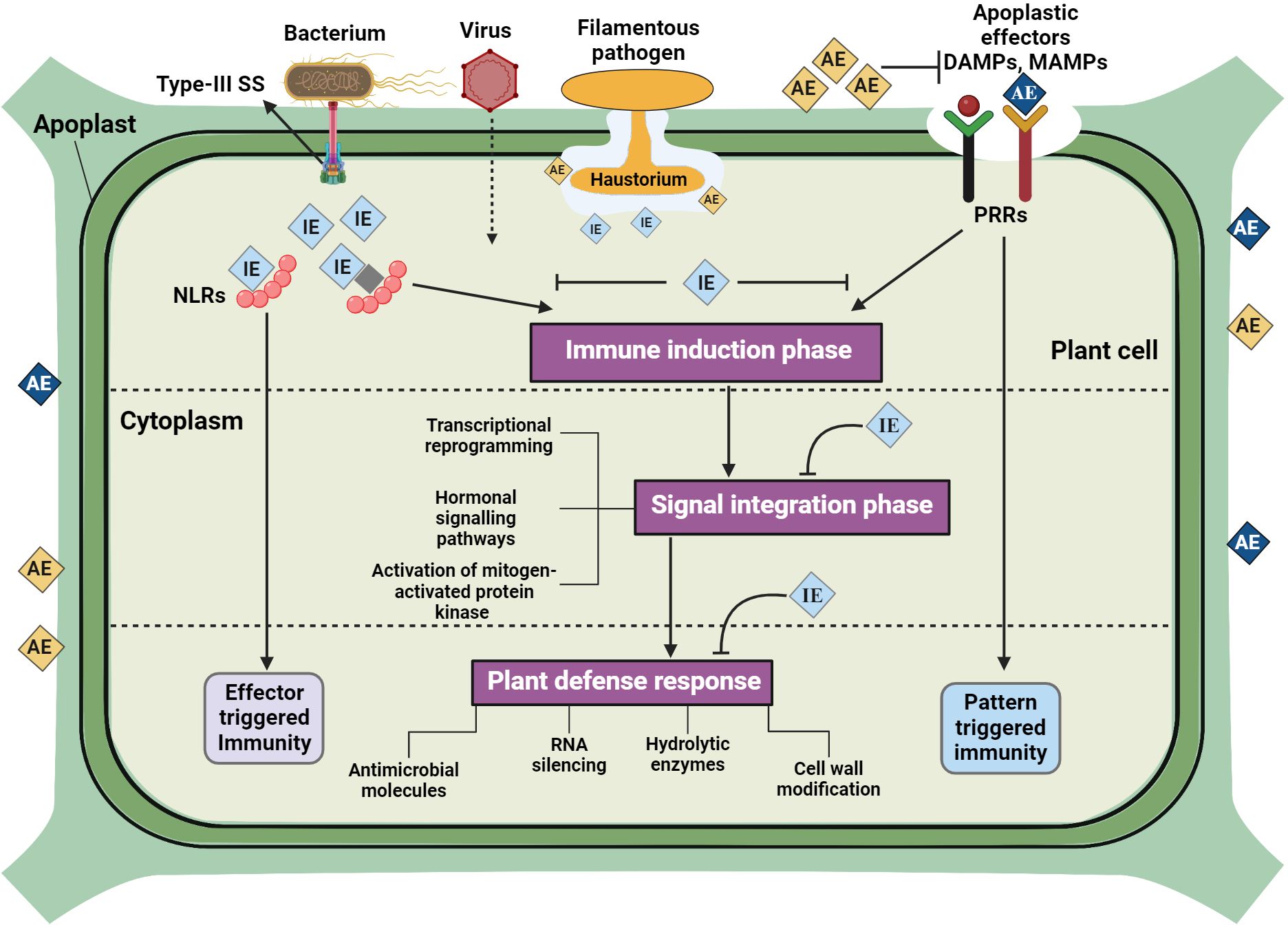
Figure 1 Schematic representation of plant immunomodulation against microbial pathogens. Microbial pathogens invasion initiates plant defense responses in three phases including immune recognition, signal integration, and defense response. Firstly, plants perceive pathogen-associated molecular patterns (PAMPs), damage-associated molecular patterns (DAMPs), and microbial effectors through intracellular and surface receptors. Secondly, various immune signaling events are activated, which involve the integration of immune signals from the recognition of diverse patterns and effectors. Finally, an effective and swift defense response is initiated in each cellular compartment of the plant cell, which leads to pattern-triggered immunity (PTI) and NLR-mediated pathways triggering the effector-triggered immunity (ETI) response. Host resistance is modulated by the cumulative action of effectors transmitted by microbial pathogens.
Pathogens create a favorable environment for infection by rewiring plant immune signaling networks. In addition to suppressing immune signaling pathways, pathogens also target and manipulate the downstream defense outputs of the plant immune system (Mishra et al., 2021; Singh et al., 2023). Effectors and secreted enzymes help pathogens overcome physical and chemical barriers in the plant, such as cell wall reinforcements, antimicrobial compounds, and hydrolytic enzymes. Some pathogens produce toxins or phytohormone mimics that further manipulate plant physiology and development to their advantage (Kaur et al., 2022; Wang et al., 2022). The dynamics of plant-pathogen interactions are further shaped by the spatiotemporal regulation of immunity and infection processes (König et al., 2021). Plants must balance the allocation of resources between growth and defense, while pathogens face the challenge of avoiding detection and preserving host viability. Therefore, timing and localization of immune responses and pathogen colonization are critical determinants of disease outcomes (Li et al., 2020; Monson et al., 2022). Taken together, the dynamics of plant immunomodulation against phytopathogens involve an intricate interplay of recognition mechanisms that detect PAMPs, DAMPs and effector molecules, subsequent signaling cascades that transmit this detection, and the activation of diverse defense responses. Furthermore, the application of ENMs to modulate plant immune responses has emerged as a promising alternative strategy for enhancing plant disease resistance and management (Liu et al., 2024; Zhang et al., 2024).
3 Nano-enabled technologies for plant immunomodulation
The plant immune system is essential for maintaining plant health and responding to pathogen invasions. However, the traditional methods (conventional breeding, crop rotation chemical fertilizers and pesticides) for activating or regulating plant immunity have shortcomings such as low efficiency and poor targeting (Raymaekers et al., 2020; Ma et al., 2021). Nano-enabled agriculture provides a new idea for the precise regulation of plant immunity and reducing the dependence on chemical pesticides (Fiol et al., 2021). Nano-enabled immunomodulation mechanisms lies in the ability of ENMs to act as nanoscale delivery platform for immunomodulatory agents (Noman et al., 2023b). Notably, ENMs as nanoscale carrier can efficiently carry immune signaling molecules (such as protein, enzymes, hormones, and RNA, etc.) into plant cells and activate immune responses (Vega-Vásquez et al., 2021; Liu et al., 2024). ENMs can be divided into different groups (metal based or inorganic ENMs, carbonaceous ENMs, polymer ENMs and hybrid ENMs) based on their unique nanoscale properties including size, shape, crystalline structure, chemical composition (Figure 2) (Saleh, 2020). Metal-based or inorganic ENMs mainly including zinc (Zn), gold (Au), silver (Ag), copper (Cu), titanium (Ti) and silica, which shown great potential for enhancing plant immunity against pathogens and environmental stresses (Mitchell et al., 2021). These inorganic ENMs can be produced to desired properties, geometries, sizes, and with desired functionalization/coatings to optimize benefit. Ma et al. (2020) reported that nanoscale Cu (250 mg L−1) amendments significantly suppressed soybean sudden death syndrome by activating plant immunity and enhancing the phytohormone contents, photosynthetic endpoints, antioxidant enzymes and nutritional status.
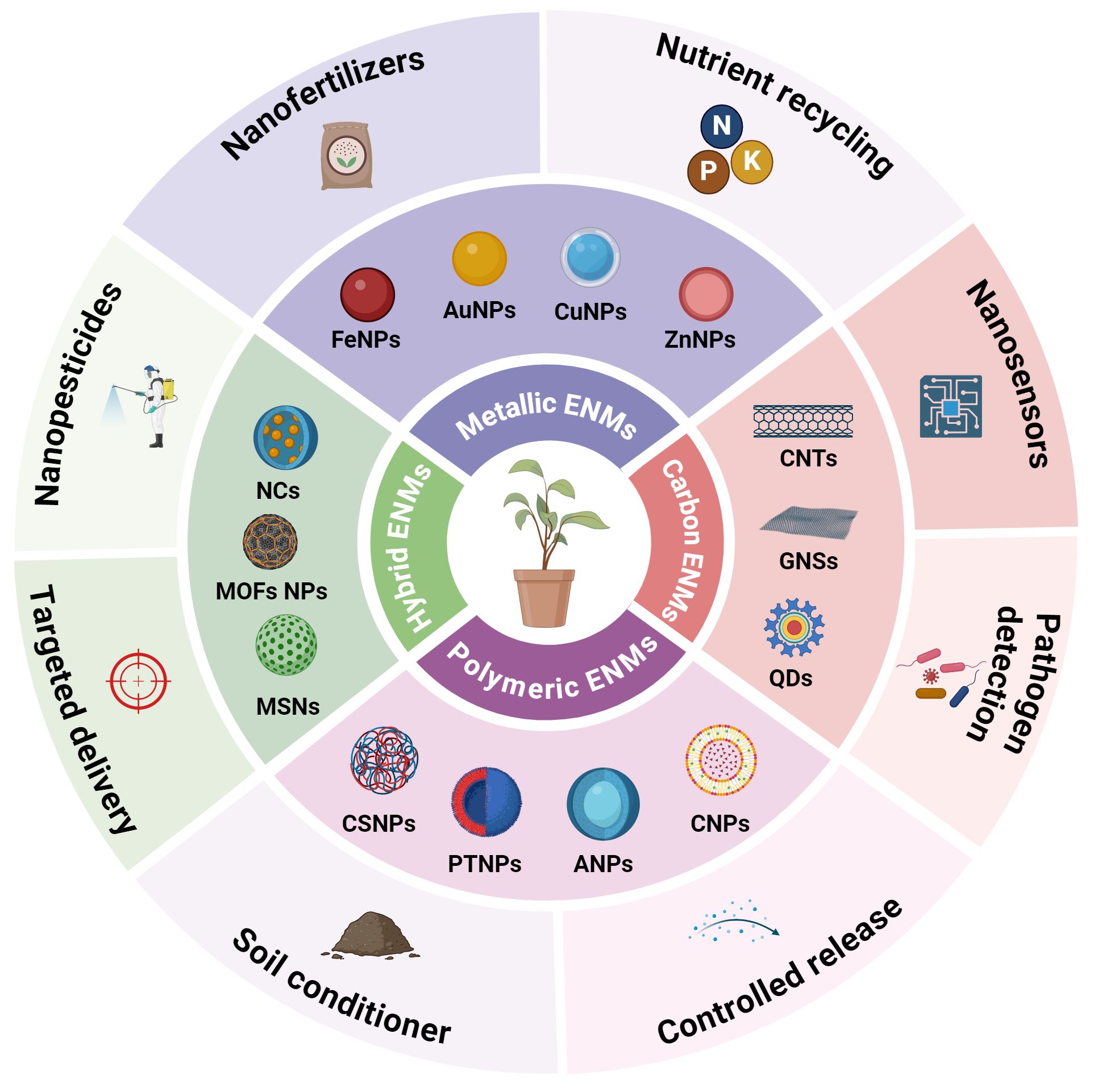
Figure 2 Schematic illustration of the current engineered nanomaterials (ENMs) toolbox to improve plant immunity against microbial pathogens. ENMs can be divided into different groups based on their unique physiochemical properties. nanoparticles (NPs), metal-organic framework (MOFs), nanocapsules (NCs), mesoporous silica nanoparticles (MSNs) chitosan (Cs), pectin (Pt), cellulose (Cl), alginate (A), quantum dots (QDs), graphene nanosheets (GNSs), carbon nanotubes (CNs), iron (Fe), copper (Cu), gold (Au) and zinc (Zn).
Carbon-based ENMs including carbon dots (CDs), carbon nanotubes (CNTs) and graphene NPs have been shown to promote plant growth and resilience against pathogens (Li et al., 2023). For example, Adeel et al. (2021) demonstrated that foliar exposure of CNTs at 200 mg L−1 significantly suppressed tobacco mosaic virus infection by activating of the defense system in tobacco (Nicotiana benthamiana) plants. Additionally, CNTs application enhanced the plant immunity by triggering defense-related phytohormones, antioxidant enzymes and improving photosynthetic performance. Polymeric ENMs such as polylactic acid, chitosan, pectin, carboxymethyl cellulose and alginate have attracted recent attention for controlled and sustained release of amicrobial agents and protect plants against pathogens (Shakiba et al., 2020; Vemula and Reddy, 2023). In a recent study, Hafeez et al. (2024) reveled that biologically produced chitosan NPs enhanced the rice blast disease resistance by improving antioxidant defense system (SOD, APX and CAT), nutrient uptake, photosynthesis efficiency and reducing the cellular oxidative stress (MDA and H2O2) in rice (Oryza sativa L.) plants. Nanohybrid, such as liposomes, nanocapsules (NCs), nanoemulsions and mesoporous silica nanoparticles (MSNs) can be engineered to carry pesticides, nutrients, enzymes and phytohormones for targeted delivery. Abdelrasoul et al. (2020) demonstrated that monoterpenes-based nanoemulsions at 100 mg L-1 concentration inhibited the Pectobacterium carotovorum and Ralstonia solanacearum pathogens growth and induced systemic resistance in potato (Solanum tuberosum L.) leaves by improving antioxidant enzymes activity. Overall, ENMs are able to modulate plant immune responses through multiple pathways, not only enhancing plant resistance to pathogens, but also promoting crop growth and increasing yields (Figure 2). However, there is still little understanding of the translocation, transformation, residue, and long-term environmental impact of ENMs in plants, and further research needs to be explored the potential mechanisms. In addition, the development of more environmentally friendly, efficient, and biodegradable ENMs formulations is also a key direction in future research.
4 Mechanisms of nano-enabled immunomodulation in plants
4.1 Nano-enabled activation of phytohormone signaling
Phytohormone signaling pathways are involved in inducing a variety of defense responses against biotic and abiotic stresses. Plant hormones such as jasmonic acid (JA), salicylic acid (SA), ethylene (ET) and abscisic acid (ABA) play an important role in the plant immune response against pathogens (Huang et al., 2020; Zhao et al., 2021). In recent years, nano-enabled activation of plant hormone signaling represents an innovative approach in plant disease management (Figure 3). ENMs can design too slowly release hormones in a controlled manner, thus activating signaling pathways and enhancing the plant resistance to microbial pathogens (Tripathi et al., 2022; Liu et al., 2024). For example, Noman et al. (2024) observed that soil-application with SA-doped FeNPs suppressed the Fusarium wilt disease in watermelon through inhibiting the fungal invasive growth and improving the antioxidative capacity, and primed a SAR response via activating the SA signaling genes (Figure 4B). In another study, lanthanum oxide NMs at 200 mg L-1 with different surface modifications significantly suppressed cucumber wilt disease by 12.50–52.11% by improving total amino acids, vitamin contents, and activating SA-dependent systemic acquired resistance (Cao et al., 2023a). Similarly, elemental sulfur NPs increase resistance against Fusarium wilt disease, caused by a fungal pathogen Fusarium oxysporum f. sp. lycopersici. Notably, sulfur NPs 30–100 mg L-1 suppressed pathogen infection by regulating the SA-dependent systemic acquired resistance pathway and modulating of the expression of antioxidase-related and pathogenesis-related genes.
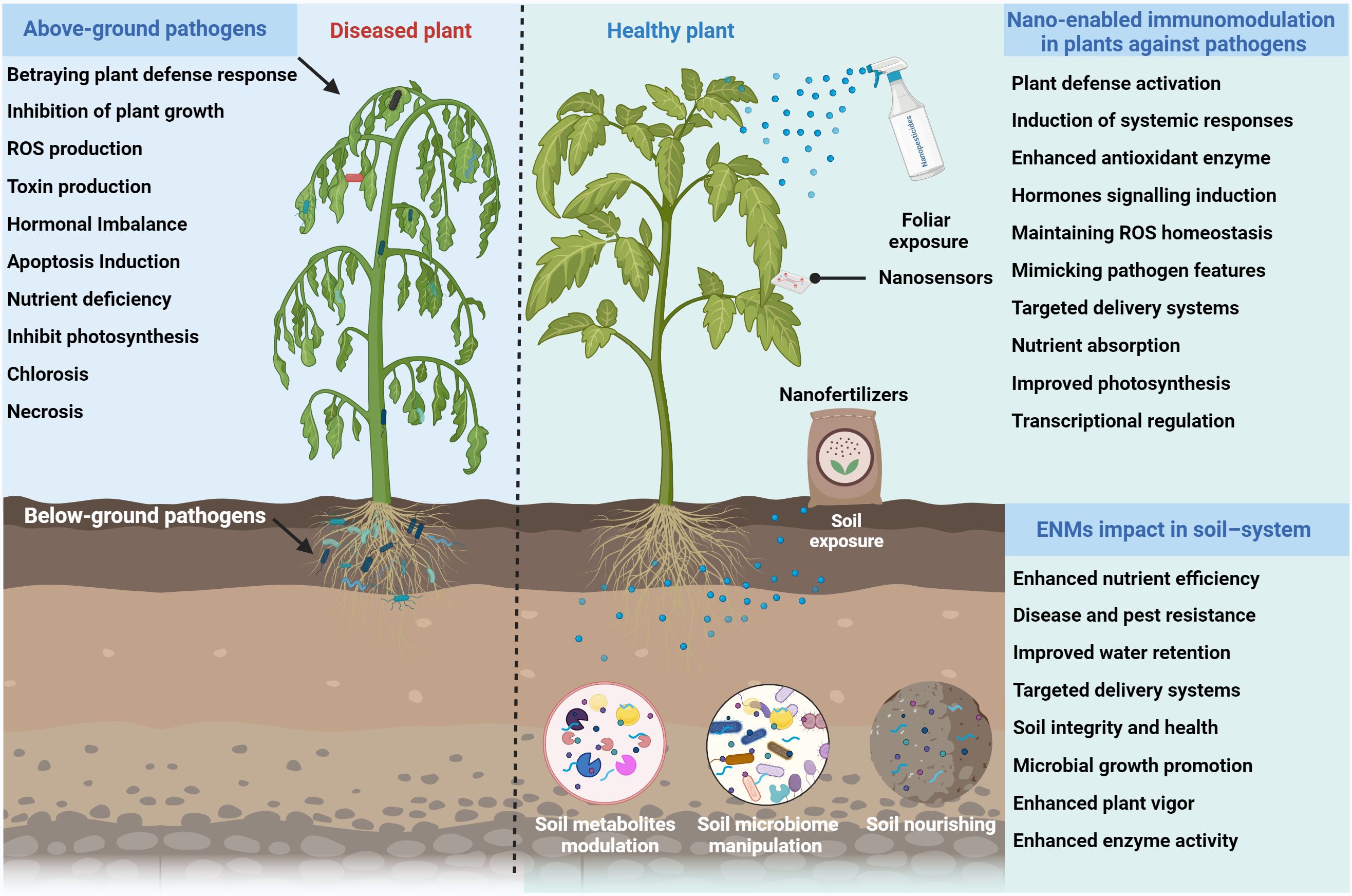
Figure 3 Schematic representation of nano-enabled immunomodulation to revolutionize plant health through several mechanisms. On the left, the diseased plant shows symptoms due to various pathogen-induced mechanisms, which inhibit plant growth. On the right, foliar and soil exposure to ENMs enhance plant growth by activating systemic responses, enhancing antioxidant enzyme activity, inducing hormone signaling, and maintaining ROS homeostasis.
Polymeric ENMs have also demonstrated excellent ability to regulate plant hormone signaling (Table 1). For example, Giri et al. (2023) reported that chitosan fabricated AgNPs control the bacterial leaf spot disease in tomato plants by inducing SAR mechanism through upregulating stress hormones responsive genes (PR1, NHO1, NPR1, MYC2, JAR1, ERF1). Taken together, these previous studies confirm that ENMs can precisely regulate plant immune responses by influencing hormone concentrations and regulating signaling pathways. However, the mechanism of action of different ENMs is different, and the specific effect may also vary depending on the plant species and the type of pathogen. In the future, it is necessary to study the interaction between ENMs and molecular networks in plants and explore new strategies to improve the specificity of immune mechanisms.
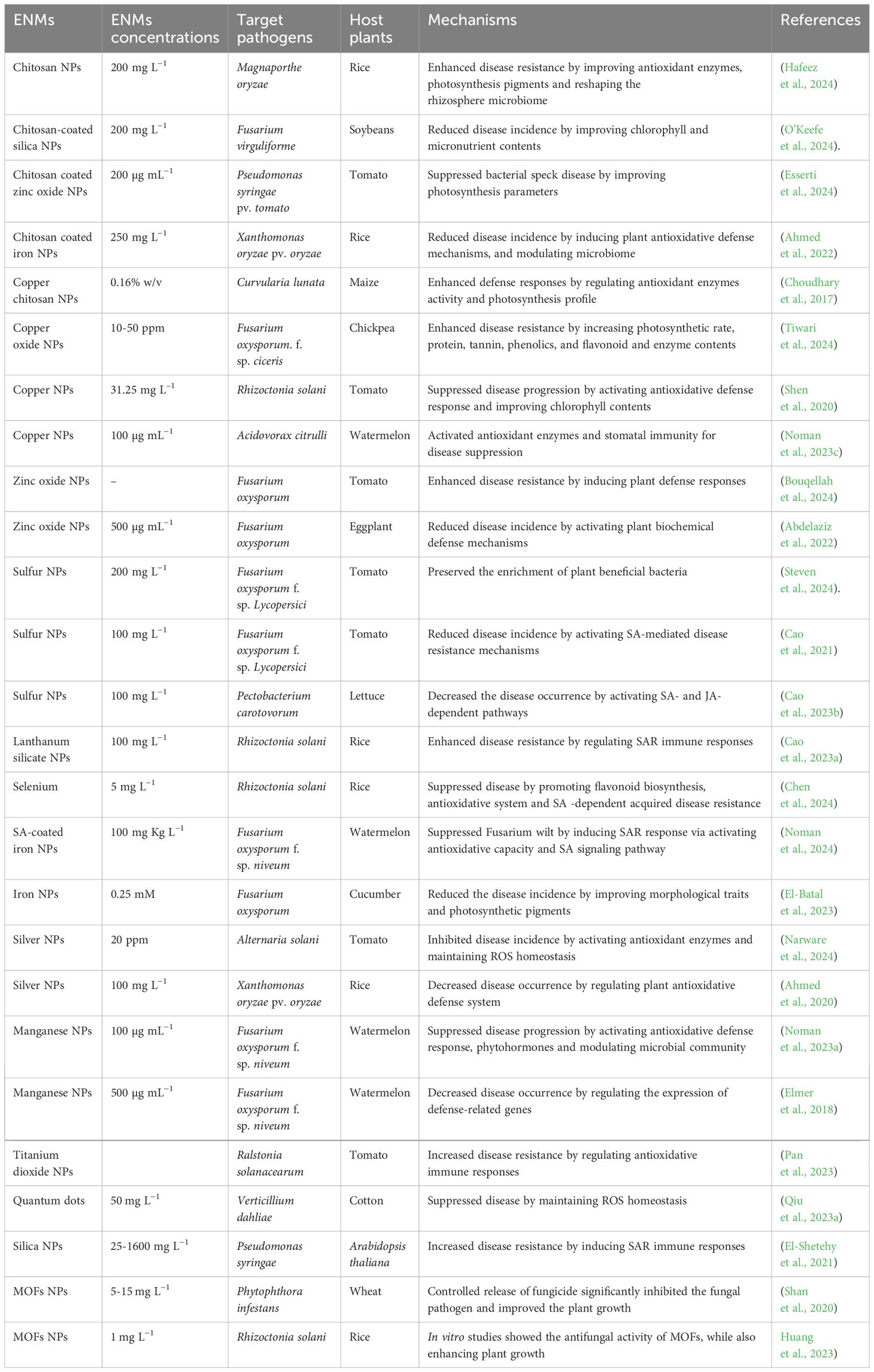
Table 1 Potential applications of engineered nanomaterials (ENMs) for improving immunomodulatory mechanisms and enhancing disease resilience in agricultural crops.
4.2 Nano-enabled stimulation of antioxidant defense system
Nano-enabled stimulation of the plant antioxidant defense system offers a promising approach to mitigating the deleterious effects of oxidative stress induced by pathogens (Pan et al., 2023). This system is designed to neutralize reactive oxygen species (ROS) generated during pathogen attacks or other stress conditions. ROS can cause oxidative damage to cellular components, including lipids, proteins, and DNA, ultimately leading to cell death and tissue dysfunction (Chen et al., 2023). The antioxidant defense enzymes (CAT, SOD, POD, APX, PPO) acts as a frontline defense, mitigating the detrimental effects of ROS and protecting plant cells from oxidative stress (Dvořák et al., 2021). ENMs with their unique physicochemical properties, have demonstrated the ability to modulate the plant antioxidant defense system. Notably, ENMs can interact with plant cells and trigger specific signaling pathways (Figure 3), leading to the upregulation of antioxidant enzymes and the biosynthesis of non-enzymatic antioxidants (Abdelrhim et al., 2021; Tiwari et al., 2024). For example, Noman et al. (2023c) investigated that foliar exposure of biogenic CuNPs at 100 µg mL−1 concentration substantially suppressed bacterial fruit blotch disease in watermelon plants by triggering antioxidants enzymes (CAT, SOD and POD), modulating stomatal immunity, and reducing the ROS activity.
In another study, biologically synthesized AgNPs improved early blight disease resistance by enhancing antioxidant enzymes (CAT, LPX, PO, SOD), and maintaining ROS (H2O2 and O−2) homeostasis in tomato plants (Narware et al., 2024). Similarly, FeO nanocomposites control the cucumber wilt disease caused by Fusarium oxysporum by stimulating morphological performances, total phenol, soluble protein contents, photosynthetic pigments and antioxidant enzymes (POD and PPO) (El-Batal et al., 2023). Liang et al. (2022) demonstrated that berberine loaded ZnO NMs at 100-1000 ug mL-1 significantly reduced the tomato bacterial wilt disease severity by 45.8% by improving the plant growth and antioxidant enzymes (SOD, PPO, PPO) (Figure 4A). Previous studies primarily focused on the mitigation of pathogen-induced oxidative stress by enhancing antioxidant activity (Table 1). However, ongoing research exploring biocompatible ENMs formulations and targeted delivery methods holds immense potential for sustainable agriculture and food security.
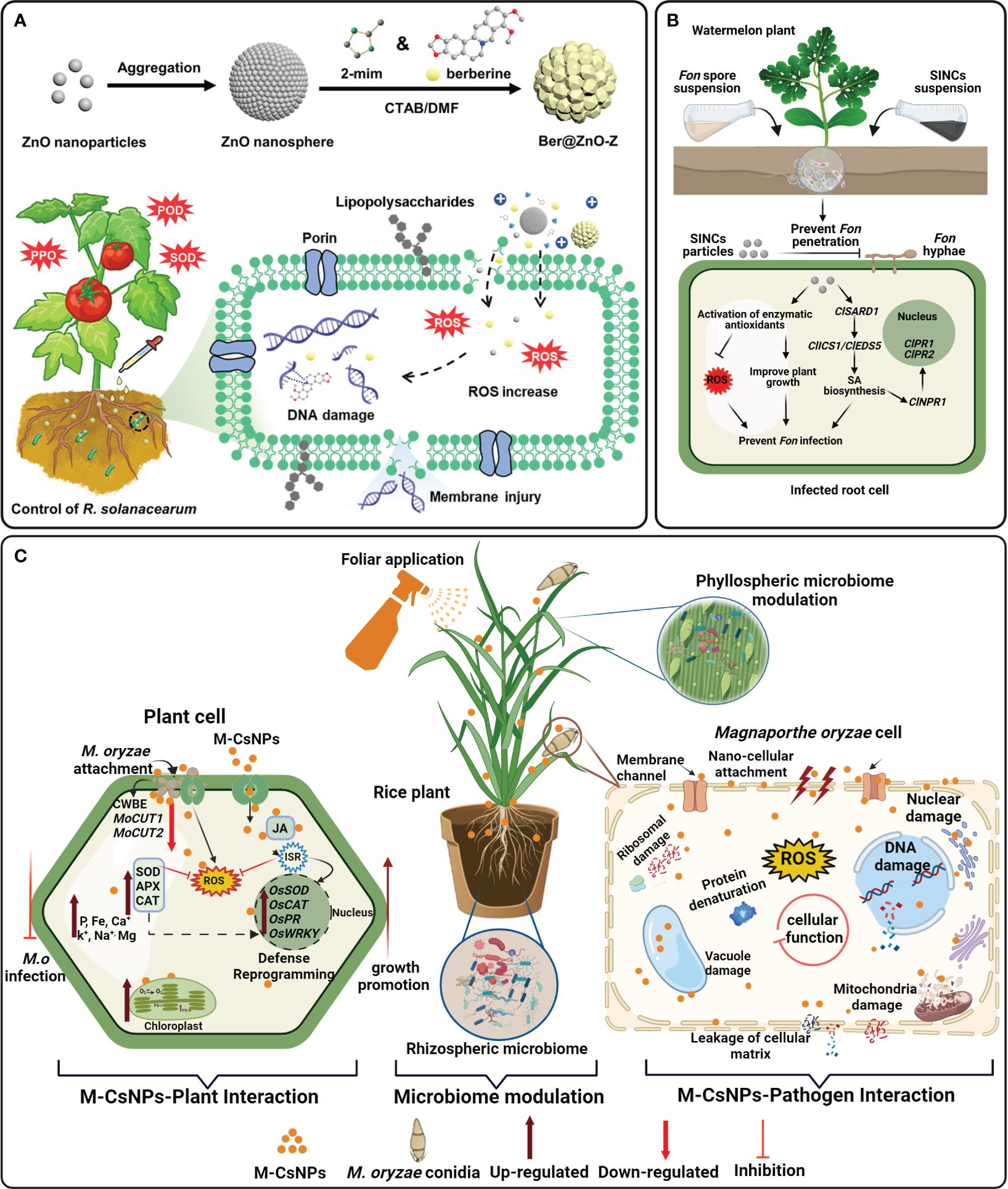
Figure 4 Examples of engineered nanomaterials (ENMs) used for plant disease management through immunomodulation. (A) The figure illustrates the synthesis of berberine-loaded ZnO-Z (Ber@ZnO-Z) nanosphere to synergistically control the bacterial wilt disease in tomato plants through direct pathogen inhibition and modulating antioxidant enzymes. Adapted with permission from reference (Liang et al., 2022). Copyright 2022 American Chemical Society. (B) Therapeutic delivery of salicylic acid-coated biogenic iron nanocomposites (BINCs) suppressed Fusarium wilt damages in watermelon plants by inducing systemic acquired resistance (SAR) and modulating antioxidative defense system. Adapted with permission from reference (Noman et al., 2024). Copyright 2024 Elsevier. (C) Schematic representation of the proposed mechanism of moringa chitosan nanoparticles (M-CsNPs) to control bacterial rice blast disease of rice. M-CsNPs act as nanofungicides that inhibit the pathogen Magnaporthe oryzae infection and improve defense responses by improving biochemical attributes and regulating transcriptional traits and modulating plant-associated microbiome. Adapted with permission from reference (Hafeez et al., 2024). Copyright 2024 Elsevier.
4.3 Nano-enabled regulation of photosynthesis and nutritional profile
Photosynthesis is an important process that drives plant growth and development, is often disrupted by pathogen infections (Yang and Luo, 2021). Phytopathogens can negatively impact photosynthesis by disrupting the structure and function of photosynthetic apparatus, reducing the efficiency of light harvesting, and impairing carbon fixation. Consequently, this can lead to reduced plant productivity, compromised nutrient acquisition, and an overall decline in plant health (Parveen and Siddiqui, 2021; Karpagam et al., 2023). In recent years, ENMs mediated regulation of photosynthesis and the nutritional profile of plants represents a promising strategy in sustainable agriculture, offering a multifaceted approach to mitigating the impacts of pathogens on crop productivity and quality (Figure 3). Importantly, ENMs can interact with plant cells and trigger specific signaling pathways, leading to the upregulation of photosynthetic enzymes, the biosynthesis of pigments, and the modulation of nutrient uptake and assimilation (Ahmed et al., 2022; Parveen and Siddiqui, 2022). For example, the foliar application of ZnONPs at 0.20 mL−1 concentrations significantly enhanced the plant growth and photosynthesis efficiency (Total chlorophyll and carotenoids contents) of tomato plants under several bacterial and fungal pathogens infection (Parveen and Siddiqui, 2021).
In another recent study, Esserti et al. (2024) chitosan embedded ZnONPs effectively control the bacterial speck disease of tomato by improving the plant growth, photosynthetic pigments (Chlorophyll a, Chlorophyll b, carotenoids), and gas exchange parameters such as internal CO2 concentration, net photosynthesis rate, transpiration rate and stomatal conductance. Notable, this study showed that bacterial speck disease significantly affected plant biomass and photosynthetic performance; however, NP applications mitigate the negative impact of phytopathogens. Likewise, Mogazy et al. (2022) reported that Ca and FeNPs at (100 and 200 ppm) positively regulate innate immune responses in strawberry (Fragaria ananassa) plants against gray mold disease caused by a fungal pathogen Botrytis cinerea. This study revealed that foliar exposure of NPs significantly increased the vitamin, phenolics, and flavonoids contents, and nutritional profile (Zn2+, Mg2+, Ca2+, Fe, N, P, and K+) in strawberry plants as compared to infected control. Likewise, chitosan coated mesoporous SiNPs treatment significantly reduced the sudden death syndrome by 30% and increased the micronutrient (Zn, Mn, Mg, K, B) content, and chlorophyll efficiency in soybean plants (O’Keefe et al., 2024). Taken together, previous studies demonstrated that nano-enabled regulation of nutritional profiles and photosynthesis shows great promise in mitigating pathogen impacts on crops (Table 1). However further research on ENMs toxicity, application methods, and biosafety is recommended for sustainable agricultural applications.
4.4 Nano-enabled modulation of microbiome and metabolites
The plant-associated microbiome (bacteria, fungi, viruses) plays a crucial role in plant health, growth, and resistance against pathogens (Trivedi et al., 2020; Steven et al., 2024). The plant-associated microbiome serves as a frontline defense against pathogens, conferring protection through various mechanisms, such as competitive exclusion, antimicrobial compound production, and the induction of systemic resistance pathways in plants (Fitzpatrick et al., 2020; Wang et al., 2022). Additionally, the intricate network of metabolites produced by plants and their associated microbiomes acts as a defensive arsenal against invading pathogens (Liu et al., 2020; Rangel and Bolton, 2022). However, microbial pathogens can disrupt the delicate balance of the plant microbiome, leading to dysbiosis and compromising plant health (Zhang et al., 2021a). Nano-enabled microbiome engineering has recently emerged as a powerful platform to enhance plant resilience against pathogenic threats (Ahmed et al., 2023; Hussain et al., 2023). In recent years, several studies have demonstrated the potential impact of ENMs plant-associated microbiome under biotic stress condition. The application of biogenic chitosan-Fe nanocomposite (BNCs) at 250 μg mL−1 concentration significantly reduced the bacterial leaf blight (BLB) disease incidence (67.1%) by enhancing the relative abundance of beneficial bacterial community such as Allorhizobium, Ochrobactrum, Pseudolabrys, Sphingomonas, Devosia, Bradyrhizobium and Methylobacterium in rice plants (Ahmed et al., 2022).
Additionally, BNCs amendments also enhanced the rice plant growth by modulating antioxidant enzymes, enhancing photosynthesis efficiency, and reducing ROS activity. Similarly, Noman et al. (2023a) revealed that soil application of biologically synthesized manganese (Mn) NPs control the Fusarium wilt disease in watermelon by enhancing SAR mechanism via triggering antioxidative defense machinery, SA signaling pathway, and modulating the soil bacterial community (Sphingomonas, Gemmatimonadaceae, Nocardioides, and Burkholderiaceae) and fungal community (Penicillium, Botryotrichum, Conocybe, and Mortierella). The foliar spray of nitrogen-doped CDs (10 mg L−1) alleviated tomato bacterial wilt disease induced damage by 71.2% through indirect resistance activation (SAR activation) and ROS scavenging. Moreover, metabolomics profile revealed that nitrogen doped CDs significantly improved the fatty acid and tricarboxylic acid synthesis in tomato plants (Luo et al., 2021). Similarly, the application of sulfur NMs at 10–100 mg L-1 significantly decreased the occurrence of bacterial soft rot disease in lettuce (Lactuca sativa L.) plants by improving the chlorophyll contents, antioxidant enzymes and regulating the defense-related genes expression. In addition, metabolomics analysis showed that sulfur NMs enhanced the tricarboxylic acid cycle and also regulated SA and JA metabolite biosynthesis, thereby enhancing the bacterial soft rot disease resistance in lettuce (Cao et al., 2023b). Taken together, nano-enabled modulation of microbiome and metabolites profile to enhance plant disease resistance has the potential to serve as highly sustainable, efficient, sustainable, and non-toxic alternative for the management of plant diseases (Figure 3).
4.5 Nano-enabled activation of defense related genetic pathways
Plants have evolved intricate defense mechanisms to protect themselves against a wide range of microbial pathogens. These defense responses are governed by complex genetic pathways that involve the coordinated expression of numerous genes encoding various proteins, enzymes, and signaling molecules (Kaur et al., 2022). After pathogens attack, specific defense-related genes are activated, triggering a cascade of events that ultimately lead to the production of antimicrobial compounds, the reinforcement of physical barriers, and the activation of systemic resistance pathways (Nishad et al., 2020; Dodds et al., 2024). Nano-enabled activation of defense-related genetic pathways represents a promising strategy in sustainable agriculture, offering a targeted and efficient approach to enhancing plant resilience against pathogenic threats (Cao et al., 2023a). For example, selenium (Se) NMs application 5 mg L-1 decreased the disease severity (68.8%) by enhancing the organic Se content (44.8%), nutritional quality by (7.2%) and rice yield up to (31.1%). Additionally, metabolomic and transcriptomic analyses confirmed that SeNMs simultaneously boosted the SA and JA dependent acquired disease resistance pathways, flavonoid biosynthesis and antioxidative defense system. Notably, Importantly, SeNMs significantly upregulated the expression of genes LOX2, LOX3, LOX6, OPR1, PR1, PR3, AOC, and JAR while reducing the expression level of genes POD, CAT, and SOD2 in rice plants compared to the infected controls, indicating overall stimulation of SAR in SeNM-treated rice (Chen et al., 2024).
Similarly, Hafeez et al. (2024) revealed that foliar exposure of biogenic chitosan NPs at 200 mg L−1 significantly control the rice blast disease by triggering defense related genes expression such as (OsNPP1, OsGRF9, WRKY71, OsAPX, OsSOD, OsCAT, OsNPR1, OsPR1, OsPR9, and MoCUT2) in rice plants (Figure 4C). In another study, seed primed with AgNPs enhanced the rice blast disease resistant by triggering transcriptional and metabolic reprogramming in rice seeds. In this study, KEGG pathway of transcriptomics data demonstrated that AgNPs-priming activated stress signaling and defense related pathways, such as MAPK signaling pathway, flavonol biosynthesis, glutathione metabolism, plant hormone signal transduction, and plant−pathogen interaction (Yan et al., 2022). Similarly, the application of ROS-generating AgNPs as nano-stimulants significantly triggered plant immune/stress responses against rice blast disease. The disease resilience mechanisms showed that AgNPs mediated “stress memory” induced considerable transcriptional reprogramming in rice leaves by modulating the expression of defense genes, including pathogen-plant interaction genes, cell membrane lipid metabolism genes, specialized metabolite biosynthesis-related genes, and other genes related to biosynthesis. These studies have demonstrated the potential of nanotechnology-mediated activation of defense-related gene expression in enhancing crop resilience against pathogens. However, further research is needed to explore the molecular signaling pathways involved in the interactions between ENMs and plant pathogens, as well as their co-stimulating impact on plant defense against phytopathogens.
5 Concluding remarks and future outlook
The promising field of nano-enabled immunomodulation has demonstrated exceptional opportunities to enhance plant resilience against a multitude of phytopathogenic threats (Zhang et al., 2024). The unique physicochemical properties of ENMs, coupled with their ability to interact with and modulate intricate plant defense mechanisms, have positioned them as promising tools in sustainable disease management strategies (Singh et al., 2024). However, as this domain continues to evolve, several critical considerations and future research directions must be addressed to harness the full potential of these innovative approaches. Firstly, while significant progress has been made in elucidating the underlying mechanisms through which ENMs influence plant immune responses, a comprehensive understanding of the complex interplay between ENMs and the intricate molecular networks governing plant defense remains elusive (Zhang et al., 2020; Kumar et al., 2024). Future endeavors should focus on unraveling the intricate signaling cascades, transcriptional regulation by ENMs, enabling the development of more targeted and efficient immunomodulatory strategies (Ma et al., 2023b; Zhang et al., 2024).
Additionally, the transformation of nano-enabled immunomodulation from laboratory-scale studies to field applications necessitates rigorous investigations into the environmental fate, behavior, and potential risks associated with the use of ENMs in agricultural settings (Ahmed et al., 2023; Ma et al., 2023a). Comprehensive assessments of the long-term impacts on soil health, nutrient cycling, and ecosystem dynamics are imperative to ensure the responsible and sustainable integration of these technologies into agricultural practices (Ijaz et al., 2023). Furthermore, the development of environmentally friendly, biodegradable, and biocompatible ENM formulations should be a priority, minimizing potential adverse effects on non-target organisms and ensuring compatibility with diverse plant species and environmental conditions (Balusamy et al., 2023; Wahab et al., 2024). Interdisciplinary collaborations between material scientists, plant biologists, and ecotoxicologists could facilitate the design and synthesis of tailored nanomaterials that balance efficacy, sustainability, and biosafety considerations (Shelar et al., 2023; Zain et al., 2023).
Another crucial aspect that warrants attention is the optimization of ENM delivery methods and application techniques. Developing efficient and targeted delivery systems, such as nanocarriers or nanoemulsions, could enhance the bioavailability and site-specific delivery of immunomodulatory agents, minimizing potential off-target effects and maximizing the desired immune responses (Ma et al., 2023c; Jeon et al., 2024). By addressing these critical considerations and leveraging the transformative potential of nanotechnology, researchers and agricultural stakeholders can revolutionize plant disease management practices, contributing to a more resilient, sustainable, and secure global food system.
Author contributions
HM: Investigation, Resources, Software, Validation, Visualization, Writing – original draft. YQ: Data curation, Investigation, Resources, Software, Visualization, Writing – original draft, Writing – review & editing. MZ: Conceptualization, Resources, Software, Validation, Visualization, Writing – original draft, Writing – review & editing. ZL: Formal analysis, Funding acquisition, Investigation, Software, Supervision, Validation, Writing – review & editing. SA: Conceptualization, Data curation, Investigation, Validation, Writing – review & editing. J-ML: Data curation, Formal analysis, Investigation, Software, Validation, Writing – review & editing. MS: Data curation, Formal analysis, Investigation, Validation, Writing – review & editing. HA: Conceptualization, Resources, Validation, Visualization, Writing – review & editing. GO: Data curation, Funding acquisition, Software, Validation, Visualization, Writing – review & editing. XQ: Conceptualization, Data curation, Funding acquisition, Investigation, Software, Supervision, Visualization, Writing – original draft, Writing – review & editing.
Funding
The author(s) declare financial support was received for the research, authorship, and/or publication of this article. This research was supported by the Project of Xianghu Laboratory under Grant No. 2023C02031 and 2023C1S02002, and the Scientific Research Foundation of Xianghu Laboratory. This study has been partly conducted under the IP-2022-10-7906 funded by the Croatian Science Foundation, and WAST2GROW project (NPOO.C3.2.R3-I1.04.0143) founded by the Ministry of Science and Education, R. of Croatia.
Acknowledgments
During the preparation of this work the author(s) used BioRender to make figures and used Claude tool to improve language and readability. After using this tool, the author(s) reviewed and edited the content as needed and take(s) full responsibility for the content of the publication.
Conflict of interest
The authors declare that the research was conducted in the absence of any commercial or financial relationships that could be construed as a potential conflict of interest.
Publisher’s note
All claims expressed in this article are solely those of the authors and do not necessarily represent those of their affiliated organizations, or those of the publisher, the editors and the reviewers. Any product that may be evaluated in this article, or claim that may be made by its manufacturer, is not guaranteed or endorsed by the publisher.
References
Abdelaziz, A. M., Salem, S. S., Khalil, A. M., El-Wakil, D. A., Fouda, H. M., Hashem, A. H. (2022). Potential of biosynthesized zinc oxide nanoparticles to control Fusarium wilt disease in eggplant (Solanum melongena) and promote plant growth. BioMetals 35, 601–616. doi: 10.1007/s10534-022-00391-8
Abdelrasoul, M. A., Eid, A. R., Badawy, M. E. (2020). Preparation, characterizations and antibacterial activity of different nanoemulsions incorporating monoterpenes: in vitro and in vivo studies. Arch. Phytopathol. Plant Prot. 53, 310–334. doi: 10.1080/03235408.2020.1744977
Abdelrhim, A. S., Mazrou, Y. S., Nehela, Y., Atallah, O. O., El-Ashmony, R. M., Dawood, M. F. (2021). Silicon dioxide nanoparticles induce innate immune responses and activate antioxidant machinery in wheat against Rhizoctonia solani. Plants 10, 2758. doi: 10.3390/plants10122758
Adeel, M., Farooq, T., White, J. C., Hao, Y., He, Z., Rui, Y. (2021). Carbon-based nanomaterials suppress tobacco mosaic virus (TMV) infection and induce resistance in Nicotiana benthamiana. J. Hazard. Mater. 404, 124167. doi: 10.1016/j.jhazmat.2020.124167
Ahmed, T., Noman, M., Gardea-Torresdey, J. L., White, J. C., Li, B. (2023). Dynamic interplay between nano-enabled agrochemicals and the plant-associated microbiome. Trends Plant Sci. 28, 1310–1325. doi: 10.1016/j.tplants.2023.06.001
Ahmed, T., Noman, M., Jiang, H., Shahid, M., Ma, C., Wu, Z., et al. (2022). Bioengineered chitosan-iron nanocomposite controls bacterial leaf blight disease by modulating plant defense response and nutritional status of rice (Oryza sativa L.). Nano Today 45, 101547. doi: 10.1016/j.nantod.2022.101547
Ahmed, T., Noman, M., White, J. C., Ma, C., Wang, Q., Li, B. (2024). Quantum dots: next shift to combat plant diseases. Trends Plant Sci. doi: 10.1016/j.tplants.2024.02.006
Ahmed, T., Shahid, M., Noman, M., Niazi, M. B. K., Mahmood, F., Manzoor, I., et al. (2020). Silver nanoparticles synthesized by using Bacillus cereus SZT1 ameliorated the damage of bacterial leaf blight pathogen in rice. Pathogens 9, 160. doi: 10.3390/pathogens9030160
Anders, S., Cowling, W., Pareek, A., Gupta, K. J., Singla-Pareek, S. L., Foyer, C. H. (2021). Gaining acceptance of novel plant breeding technologies. Trends Plant Sci. 26, 575–587. doi: 10.1016/j.tplants.2021.03.004
Balusamy, S. R., Joshi, A. S., Perumalsamy, H., Mijakovic, I., Singh, P. (2023). Advancing sustainable agriculture: a critical review of smart and eco-friendly nanomaterial applications. J. Nanobiotechnol. 21, 372. doi: 10.1186/s12951-023-02135-3
Beckers, S. J., Staal, A. H., Rosenauer, C., Srinivas, M., Landfester, K., Wurm, F. R. (2021). Targeted drug delivery for sustainable crop protection: transport and stability of polymeric nanocarriers in plants. Adv. Sci. 8, 2100067. doi: 10.1002/advs.202100067
Bouqellah, N. A., El-Sayyad, G. S., Attia, M. S. (2024). Induction of tomato plant biochemical immune responses by the synthesized zinc oxide nanoparticles against wilt-induced Fusarium oxysporum. Int. Microbiol. 27, 435–448. doi: 10.1007/s10123-023-00404-7
Cao, X., Chen, X., Liu, Y., Wang, C., Yue, L., Elmer, W. H., et al. (2023a). Lanthanum silicate nanomaterials enhance sheath blight resistance in rice: mechanisms of action and soil health evaluation. ACS Nano. 17, 15821–15835. doi: 10.1021/acsnano.3c03701
Cao, X., Liu, Y., Luo, X., Wang, C., Yue, L., Elmer, W., et al. (2023b). Mechanistic investigation of enhanced bacterial soft rot resistance in lettuce (Lactuca sativa L.) with elemental sulfur nanomaterials. Sci. Total Environ. 884, 163793. doi: 10.1016/j.scitotenv.2023.163793
Cao, X., Wang, C., Luo, X., Yue, L., White, J. C., Elmer, W., et al. (2021). Elemental sulfur nanoparticles enhance disease resistance in tomatoes. ACS Nano. 15, 11817–11827. doi: 10.1021/acsnano.1c02917
Ceulemans, E., Ibrahim, H. M., De Coninck, B., Goossens, A. (2021). Pathogen effectors: exploiting the promiscuity of plant signaling hubs. Trends Plant Sci. 26, 780–795. doi: 10.1016/j.tplants.2021.01.005
Chen, S., Guo, X., Zhang, B., Nie, D., Rao, W., Zhang, D., et al. (2023). Mesoporous silica nanoparticles induce intracellular peroxidation damage of Phytophthora infestans: A new type of green fungicide for late blight control. Environ. Sci. Technol. 57, 3980–3989. doi: 10.1021/acs.est.2c07182
Chen, X., Jiang, Y., Wang, C., Yue, L., Li, X., Cao, X., et al. (2024). Selenium nanomaterials enhance sheath blight resistance and nutritional quality of rice: mechanisms of action and human health benefit. ACS Nano. doi: 10.1021/acsnano.4c01835
Choudhary, R. C., Kumaraswamy, R., Kumari, S., Sharma, S., Pal, A., Raliya, R., et al. (2017). Cu-chitosan nanoparticle boost defense responses and plant growth in maize (Zea mays L.). Sci. Rep. 7, 9754. doi: 10.1038/s41598-017-08571-0
Conway, G. (1998). The doubly green revolution: food for all in the twenty-first century (Ithaca, New York: Cornell University Press). doi: 10.7591/9781501722660
Dodds, P. N., Chen, J., Outram, M. A. (2024). Pathogen perception and signalling in plant immunity. Plant Cell., koae020. doi: 10.1093/plcell/koae020
Dou, D., Zhou, J.-M. (2012). Phytopathogen effectors subverting host immunity: different foes, similar battleground. Cell Host Microbe 12, 484–495. doi: 10.1016/j.chom.2012.09.003
Dvořák, P., Krasylenko, Y., Zeiner, A., Šamaj, J., Takáč, T. (2021). Signaling toward reactive oxygen species-scavenging enzymes in plants. Front. Plant Sci. 11, 618835. doi: 10.3389/fpls.2020.618835
El-Batal, A. I., El-Sayyad, G. S., Al-Shammari, B. M., Abdelaziz, A. M., Nofel, M. M., Gobara, M., et al. (2023). Protective role of iron oxide nanocomposites on disease index, and biochemical resistance indicators against Fusarium oxysporum induced-cucumber wilt disease: In vitro, and in vivo studies. Microb. Pathog. 180, 106131. doi: 10.1016/j.micpath.2023.106131
Elmer, W., de la Torre-Roche, R., Pagano, L., Majumdar, S., Zuverza-Mena, N., Dimkpa, C., et al. (2018). Effect of metalloid and metal oxide nanoparticles on Fusarium wilt of watermelon. Plant Dis. 102, 1394–1401. doi: 10.1094/PDIS-10-17-1621-RE
El-Shetehy, M., Moradi, A., Maceroni, M., Reinhardt, D., Petri-Fink, A., Rothen-Rutishauser, B., et al. (2021). Silica nanoparticles enhance disease resistance in Arabidopsis plants. Nat. Nanotechnol. 16, 344–353. doi: 10.1038/s41565-020-00812-0
Esserti, S., Billah, R. E. K., Venisse, J.-S., Smaili, A., Dich, J., Es-sahm, I., et al. (2024). Chitosan embedded with ZnO nanoparticles and hydroxyapatite: synthesis, antiphytopathogenic activity and effect on tomato grown under high density. Sci. Hortic. 326, 112778. doi: 10.1016/j.scienta.2023.112778
Fields, B., Friman, V.-P. (2022). Microbial eco-evolutionary dynamics in the plant rhizosphere. Curr. Opin. Microbiol. 68, 102153. doi: 10.1016/j.mib.2022.102153
Fiol, D. F., Terrile, M. C., Frik, J., Mesas, F. A., Álvarez, V. A., Casalongué, C. A. (2021). Nanotechnology in plants: recent advances and challenges. J. Chem. Technol. Biotechnol. 96, 2095–2108. doi: 10.1002/jctb.6741
Fitzpatrick, C. R., Salas-González, I., Conway, J. M., Finkel, O. M., Gilbert, S., Russ, D., et al. (2020). The plant microbiome: from ecology to reductionism and beyond. Annu. Rev. Microbiol. 74, 81–100. doi: 10.1146/annurev-micro-022620-014327
Giri, V. P., Pandey, S., Srivastava, S., Shukla, P., Kumar, N., Kumari, M., et al. (2023). Chitosan fabricated biogenic silver nanoparticles (Ch@BSNP) protectively modulate the defense mechanism of tomato during bacterial leaf spot (BLS) disease. Plant Physiol. Biochem. 197, 107637. doi: 10.1016/j.plaphy.2023.03.014
Hafeez, R., Guo, J., Ahmed, T., Jiang, H., Raza, M., Shahid, M., et al. (2024). Bio-formulated chitosan nanoparticles enhance disease resistance against rice blast by physiomorphic, transcriptional, and microbiome modulation of rice (Oryza sativa L.). Carbohydr. Polym. 334, 122023. doi: 10.1016/j.carbpol.2024.122023
Hannan Parker, A., Wilkinson, S. W., Ton, J. (2022). Epigenetics: a catalyst of plant immunity against pathogens. New Phytol. 233, 66–83. doi: 10.1111/nph.17699
Harris, F. M., Mou, Z. (2024). Damage-associated molecular patterns and systemic signaling. Phytopathology 114, 308–327. doi: 10.1094/PHYTO-03-23-0104-RVW
Hartmann, M., Six, J. (2023). Soil structure and microbiome functions in agroecosystems. Nat. Rev. Earth Environ. 4, 4–18. doi: 10.1038/s43017-022-00366-w
Huang, W., Wang, M., Hu, Z., Yang, T., Pei, H., Zhang, F. (2023). Multifunctional metal-organic framework with pH-response for co-delivery of prochloraz and siRNA to synergistic control pathogenic fungi. Colloids Surf. A: Physicochem. Eng. Aspects 670, 131563.
Huang, S., Zhang, X., Fernando, W. D. (2020). Directing trophic divergence in plant-pathogen interactions: antagonistic phytohormones with NO doubt? Front. Plant Sci. 11, 600063. doi: 10.3389/fpls.2020.600063
Hussain, M., Shakoor, N., Adeel, M., Ahmad, M. A., Zhou, H., Zhang, Z., et al. (2023). Nano-enabled plant microbiome engineering for disease resistance. Nano Today 48, 101752. doi: 10.1016/j.nantod.2023.101752
Ijaz, M., Khan, F., Ahmed, T., Noman, M., Zulfiqar, F., Rizwan, M., et al. (2023). Nanobiotechnology to advance stress resilience in plants: Current opportunities and challenges. Mater. Today Bio. 9, 10075. doi: 10.1016/j.mtbio.2023.100759
Iswanto, A. B. B., Vu, M. H., Pike, S., Lee, J., Kang, H., Son, G. H., et al. (2022). Pathogen effectors: What do they do at plasmodesmata? Mol. Plant Pathol. 23, 795–804. doi: 10.1111/mpp.13142
Jeon, S. J., Zhang, Y., Castillo, C., Nava, V., Ristroph, K., Therrien, B., et al. (2024). Targeted delivery of sucrose-coated nanocarriers with chemical cargoes to the plant vasculature enhances long-distance translocation. Small 20, 2304588. doi: 10.1002/smll.202304588
Karpagam, T., Shanmugapriya, A., Suganya, V., Varalakshmi, B., Firdous, J. (2023). “Advanced study of plant-microbe interactions in photosynthesis,” in Plant-Microbe Interaction-Recent Advances in Molecular and Biochemical Approaches (Elsevier), 205–228. doi: 10.1016/B978-0-323-91875-6.00011-6
Kaur, S., Samota, M. K., Choudhary, M., Choudhary, M., Pandey, A. K., Sharma, A., et al. (2022). How do plants defend themselves against pathogens-Biochemical mechanisms and genetic interventions. Physiol. Mol. Biol. Plants. 28, 485–504. doi: 10.1007/s12298-022-01146-y
Kim, D.-Y., Patel, S. K., Rasool, K., Lone, N., Bhatia, S. K., Seth, C. S., et al. (2023). Bioinspired silver nanoparticle-based nanocomposites for effective control of plant pathogens: A review. Sci. Total Environ., 168318.
König, A., Müller, R., Mogavero, S., Hube, B. (2021). Fungal factors involved in host immune evasion, modulation and exploitation during infection. Cell. Microbiol. 23, e13272. doi: 10.1111/cmi.13272
Kumar, D., Singh, R., Upadhyay, S. K., Verma, K. K., Tripathi, R. M., Liu, H., et al. (2024). Review on interactions between nanomaterials and phytohormones: Novel perspectives and opportunities for mitigating environmental challenges. Plant Sci. 340, 111964. doi: 10.1016/j.plantsci.2023.111964
Laflamme, B., Dillon, M. M., Martel, A., Almeida, R. N., Desveaux, D., Guttman, D. S. (2020). The pan-genome effector-triggered immunity landscape of a host-pathogen interaction. Science 367, 763–768. doi: 10.1126/science.aax4079
Lee, S., Lee, H. Y., Kang, H. J., Seo, Y. E., Lee, J. H., Choi, D. (2024). Oomycete effector AVRblb2 targets cyclic nucleotide-gated channels through calcium sensors to suppress pattern-triggered immunity. New Phytol. 241, 1277–1291. doi: 10.1111/nph.19430
Li, P., Lu, Y.-J., Chen, H., Day, B. (2020). The lifecycle of the plant immune system. Crit. Rev. Plant Sci. 39, 72–100. doi: 10.1080/07352689.2020.1757829
Li, K., Tan, H., Li, J., Li, Z., Qin, F., Luo, H., et al. (2023). Unveiling the effects of carbon-based nanomaterials on crop growth: from benefits to detriments. J. Agric. Food Chem. 71, 11860–11874. doi: 10.1021/acs.jafc.3c02768
Liang, W., Cheng, J., Zhang, J., Xiong, Q., Jin, M., Zhao, J. (2022). pH-Responsive on-demand alkaloids release from core–shell ZnO@ ZIF-8 nanosphere for synergistic control of bacterial wilt disease. ACS Nano. 16, 2762–2773. doi: 10.1021/acsnano.1c09724
Liu, H., Brettell, L. E., Qiu, Z., Singh, B. K. (2020). Microbiome-mediated stress resistance in plants. Trends Plant Sci. 25, 733–743. doi: 10.1016/j.tplants.2020.03.014
Liu, Z., Wen, F., Cheng, X., Wu, Z. (2024). Nano-controlled release of phytohormones will broaden its application on plant protection. Adv. Agrochem. 3, 39–42. doi: 10.1016/j.aac.2023.11.004
Loo, E. P.-I., Tajima, Y., Yamada, K., Kido, S., Hirase, T., Ariga, H., et al. (2022). Recognition of microbe-and damage-associated molecular patterns by leucine-rich repeat pattern recognition receptor kinases confers salt tolerance in plants. Mol. Plant-Microbe Interact. 35, 554–566. doi: 10.1094/MPMI-07-21-0185-FI
Lü, P., Liu, Y., Yu, X., Shi, C.-L., Liu, X. (2022). The right microbe-associated molecular patterns for effective recognition by plants. Front. Microbiol. 13, 1019069. doi: 10.3389/fmicb.2022.1019069
Luo, X., Cao, X., Wang, C., Yue, L., Chen, X., Yang, H., et al. (2021). Nitrogen-doped carbon dots alleviate the damage from tomato bacterial wilt syndrome: systemic acquired resistance activation and reactive oxygen species scavenging. Environ. Sci.: Nano. 8, 3806–3819.
Ma, C., Borgatta, J., Hudson, B. G., Tamijani, A. A., de la Torre-Roche, R., Zuverza-Mena, N., et al. (2020). Advanced material modulation of nutritional and phytohormone status alleviates damage from soybean sudden death syndrome. Nat. Nanotechnol. 15, 1033–1042. doi: 10.1038/s41565-020-00776-1
Ma, C., Han, L., Shang, H., Hao, Y., Xu, X., White, J. C., et al. (2023a). Nanomaterials in agricultural soils: Ecotoxicity and application. Curr. Opin. Environ. Sci. Health 31, 100432. doi: 10.1016/j.coesh.2022.100432
Ma, K.-W., Niu, Y., Jia, Y., Ordon, J., Copeland, C., Emonet, A., et al. (2021). Coordination of microbe–host homeostasis by crosstalk with plant innate immunity. Nat. Plants. 7, 814–825. doi: 10.1038/s41477-021-00920-2
Ma, Y., Wang, Y., Zhao, R., Wang, Z., Li, S., Yu, M., et al. (2023c). pH-responsive ZIF-8 film-coated mesoporous silica nanoparticles for clean, targeted delivery of fungicide and environmental hazard reduction. J. Environ. Chem. Eng. 11, 111513. doi: 10.1016/j.jece.2023.111513
Ma, N. L., Zhang, N., Yong, W. T. L., Misbah, S., Hashim, F., Soon, C. F., et al. (2023b). Use, exposure and omics characterisation of potential hazard in nanomaterials. Mater. Today Adv. 17, 100341. doi: 10.1016/j.mtadv.2023.100341
Mishra, B., Kumar, N., Mukhtar, M. S. (2021). Network biology to uncover functional and structural properties of the plant immune system. Curr. Opin. Plant Biol. 62, 102057. doi: 10.1016/j.pbi.2021.102057
Mitchell, M. J., Billingsley, M. M., Haley, R. M., Wechsler, M. E., Peppas, N. A., Langer, R. (2021). Engineering precision nanoparticles for drug delivery. Nat. Rev. Drug Discovery 20, 101–124. doi: 10.1038/s41573-020-0090-8
Mogazy, A. M., Mohamed, H. I., El-Mahdy, O. M. (2022). Calcium and iron nanoparticles: A positive modulator of innate immune responses in strawberry against Botrytis cinerea. Process Biochem. 115, 128–145. doi: 10.1016/j.procbio.2022.02.014
Monson, R. K., Trowbridge, A. M., Lindroth, R. L., Lerdau, M. T. (2022). Coordinated resource allocation to plant growth–defense tradeoffs. New Phytol. 233, 1051–1066. doi: 10.1111/nph.17773
Munaweera, T., Jayawardana, N., Rajaratnam, R., Dissanayake, N. (2022). Modern plant biotechnology as a strategy in addressing climate change and attaining food security. Agric. Food Secur. 11, 1–28. doi: 10.1186/s40066-022-00369-2
Nabi, Z., Manzoor, S., Nabi, S. U., Wani, T. A., Gulzar, H., Farooq, M., et al. (2024). Pattern-Triggered Immunity and Effector-Triggered Immunity: crosstalk and cooperation of PRR and NLR-mediated plant defense pathways during host–pathogen interactions. Physiol. Mol. Biol. Plants., 1–18. doi: 10.1007/s12298-024-01452-7
Narware, J., Singh, S. P., Ranjan, P., Behera, L., Das, P., Manzar, N., et al. (2024). Enhancing tomato growth and early blight disease resistance through green-synthesized silver nanoparticles: Insights into plant physiology. S. Afr. J. Bot. 166, 676–689. doi: 10.1016/j.sajb.2024.01.059
Ngou, B. P. M., Jones, J. D., Ding, P. (2022). Plant immune networks. Trends Plant Sci. 27, 255–273. doi: 10.1016/j.tplants.2021.08.012
Nishad, R., Ahmed, T., Rahman, V. J., Kareem, A. (2020). Modulation of plant defense system in response to microbial interactions. Front. Microbiol. 11, 514909. doi: 10.3389/fmicb.2020.01298
Noman, M., Ahmed, T., Ijaz, U., Shahid, M., Nazir, M. M., Azizullah, et al. (2023a). Bio-functionalized manganese nanoparticles suppress Fusarium wilt in watermelon (Citrullus lanatus L.) by infection disruption, host defense response potentiation, and soil microbial community modulation. Small 19, 2205687. doi: 10.1002/smll.202205687
Noman, M., Ahmed, T., Shahid, M., Nazir, M. M., Li, D., Song, F. (2024). Salicylic acid-doped iron nano-biostimulants potentiate defense responses and suppress Fusarium wilt in watermelon. J. Adv. Res. 59, 19–33. doi: 10.1016/j.jare.2023.06.011
Noman, M., Ahmed, T., Wang, J., Ijaz, M., Shahid, M., Islam, M. S., et al. (2023b). Nano-enabled crop resilience against pathogens: potential, mechanisms and strategies. Crop Health 1, 15. doi: 10.1007/s44297-023-00015-8
Noman, M., Ahmed, T., White, J. C., Nazir, M. M., Azizullah, Li, D., et al. (2023c). Bacillus altitudinis-Stabilized Multifarious Copper Nanoparticles Prevent Bacterial Fruit Blotch in Watermelon (Citrullus lanatus L.): Direct Pathogen Inhibition, In Planta Particles Accumulation, and Host Stomatal Immunity Modulation. Small 19, 2207136. doi: 10.1002/smll.202207136
O’Keefe, T. L., Deng, C., Wang, Y., Mohamud, S., Torres-Gómez, A., Tuga, B., et al. (2024). Chitosan-Coated Mesoporous Silica Nanoparticles for Suppression of Fusarium virguliforme in Soybeans (Glycine max). ACS Agric. Sci. Technol.
Pan, X., Nie, D., Guo, X., Xu, S., Zhang, D., Cao, F., et al. (2023). Effective control of the tomato wilt pathogen using TiO 2 nanoparticles as a green nanopesticide. Environ. Sci.: Nano. 10, 1441–1452.
Parveen, A., Siddiqui, Z. A. (2021). Zinc oxide nanoparticles affect growth, photosynthetic pigments, proline content and bacterial and fungal diseases of tomato. Arch. Phytopathol. Plant Prot. 54, 1519–1538. doi: 10.1080/03235408.2021.1917952
Parveen, A., Siddiqui, Z. A. (2022). Impact of silicon dioxide nanoparticles on growth, photosynthetic pigments, proline, activities of defense enzymes and some bacterial and fungal pathogens of tomato. Vegetos 35, 83–93. doi: 10.1007/s42535-021-00280-4
Qiu, X., Kong, L., Chen, H., Lin, Y., Tu, S., Wang, L., et al. (2023b). The Phytophthora sojae nuclear effector PsAvh110 targets a host transcriptional complex to modulate plant immunity. Plant Cell 35, 574–597. doi: 10.1093/plcell/koac300
Qiu, P., Li, J., Zhang, L., Chen, K., Shao, J., Zheng, B., et al. (2023a). Polyethyleneimine-coated MXene quantum dots improve cotton tolerance to Verticillium dahliae by maintaining ROS homeostasis. Nat. Commun. 14, 7392. doi: 10.1038/s41467-023-43192-4
Rangel, L. I., Bolton, M. D. (2022). The unsung roles of microbial secondary metabolite effectors in the plant disease cacophony. Curr. Opin. Plant Biol. 68, 102233. doi: 10.1016/j.pbi.2022.102233
Raymaekers, K., Ponet, L., Holtappels, D., Berckmans, B., Cammue, B. P. (2020). Screening for novel biocontrol agents applicable in plant disease management–a review. Biol. Control 144, 104240. doi: 10.1016/j.biocontrol.2020.104240
Saleh, T. A. (2020). Nanomaterials: Classification, properties, and environmental toxicities. Environ. Technol. Innov. 20, 101067. doi: 10.1016/j.eti.2020.101067
Shakiba, S., Astete, C. E., Paudel, S., Sabliov, C. M., Rodrigues, D. F., Louie, S. M. (2020). Emerging investigator series: polymeric nanocarriers for agricultural applications: synthesis, characterization, and environmental and biological interactions. Environ. Sci.: Nano. 7, 37–67. doi: 10.1039/C9EN01127G
Shan, Y., Cao, L., Muhammad, B., Xu, B., Zhao, P., Cao, C., et al. (2020). Iron-based porous metal–organic frameworks with crop nutritional function as carriers for controlled fungicide release. J. colloid Interface Sci. 566, 383–393. doi: 10.1016/j.jcis.2020.01.112
Shang, S., Liu, G., Zhang, S., Liang, X., Zhang, R., Sun, G. (2024). A fungal CFEM-containing effector targets NPR1 regulator NIMIN2 to suppress plant immunity. Plant Biotechnol. J. 22, 82–97. doi: 10.1111/pbi.14166
Shelar, A., Nile, S. H., Singh, A. V., Rothenstein, D., Bill, J., Xiao, J., et al. (2023). Recent advances in nano-enabled seed treatment strategies for sustainable agriculture: challenges, risk assessment, and future perspectives. Nano-Micro Lett. 15, 54. doi: 10.1007/s40820-023-01025-5
Shen, Y., Borgatta, J., Ma, C., Elmer, W., Hamers, R. J., White, J. C. (2020). Copper nanomaterial morphology and composition control foliar transfer through the cuticle and mediate resistance to root fungal disease in tomato (Solanum lycopersicum). J. Agric. Food Chem. 68, 11327–11338. doi: 10.1021/acs.jafc.0c04546
Singh, R., Choudhary, P., Kumar, S., Daima, H. K. (2024). Mechanistic approaches for crosstalk between nanomaterials and plants: plant immunomodulation, defense mechanisms, stress resilience, toxicity, and perspectives. Environ. Sci.: Nano.
Singh, B. K., Delgado-Baquerizo, M., Egidi, E., Guirado, E., Leach, J. E., Liu, H., et al. (2023). Climate change impacts on plant pathogens, food security and paths forward. Nat. Rev. Microbiol. 21, 640–656. doi: 10.1038/s41579-023-00900-7
Son, W. K., Choi, Y. S., Han, Y. W., Shin, D. W., Min, K., Shin, J., et al. (2023). In vivo surface-enhanced Raman scattering nanosensor for the real-time monitoring of multiple stress signalling molecules in plants. Nat. Nanotechnol. 18, 205–216. doi: 10.1038/s41565-022-01274-2
Sood, M., Kapoor, D., Kumar, V., Kalia, N., Bhardwaj, R., Sidhu, G. P., et al. (2021). Mechanisms of plant defense under pathogen stress: A review. Curr. Protein Pept. Sci. 22, 376–395. doi: 10.2174/1389203722666210125122827
Steven, B., Hassani, M. A., LaReau, J. C., Wang, Y., White, J. C. (2024). Nanoscale sulfur alters the bacterial and eukaryotic communities of the tomato rhizosphere and their interactions with a fungal pathogen. NanoImpact 33, 100495. doi: 10.1016/j.impact.2024.100495
Sun, T., Zhang, Y. (2021). Short-and long-distance signaling in plant defense. Plant J. 105, 505–517. doi: 10.1111/tpj.15068
Tiwari, V., Bambharoliya, K. S., Bhatt, M. D., Nath, M., Arora, S., Dobriyal, A. K., et al. (2024). Application of green synthesized copper oxide nanoparticles for effective mitigation of Fusarium wilt disease in roots of Cicer arietinum. Physiol. Mol. Plant Pathol., 102244. doi: 10.1016/j.pmpp.2024.102244
Totsline, N., Kniel, K. E., Bais, H. P. (2023). Microgravity and evasion of plant innate immunity by human bacterial pathogens. NPJ Microgravity. 9, 71. doi: 10.1038/s41526-023-00323-x
Tripathi, D., Singh, M., Pandey-Rai, S. (2022). Crosstalk of nanoparticles and phytohormones regulate plant growth and metabolism under abiotic and biotic stress. Plant Stress. 6, 100107. doi: 10.1016/j.stress.2022.100107
Trivedi, P., Leach, J. E., Tringe, S. G., Sa, T., Singh, B. K. (2020). Plant–microbiome interactions: from community assembly to plant health. Nat. Rev. Microbiol. 18, 607–621. doi: 10.1038/s41579-020-0412-1
Vega-Vásquez, P., Mosier, N. S., Irudayaraj, J. (2021). Nanovaccine for plants from organic waste: D-limonene-loaded chitosan nanocarriers protect plants against Botrytis cinerea. ACS Sustain. Chem. Eng. 9, 9903–9914.
Vemula, M., Reddy, A. V. B. (2023). Polymeric nanoparticles as effective delivery systems in agriculture sustainability. Nanotechnol. Environ. Eng. 8, 805–814. doi: 10.1007/s41204-023-00319-8
Wahab, A., Muhammad, M., Ullah, S., Abdi, G., Shah, G. M., Zaman, W., et al. (2024). Agriculture and environmental management through nanotechnology: Eco-friendly nanomaterial synthesis for soil-plant systems, food safety, and sustainability. Sci. Total Environ., 171862.
Wang, Y., Pruitt, R. N., Nuernberger, T., Wang, Y. (2022). Evasion of plant immunity by microbial pathogens. Nat. Rev. Microbiol. 20, 449–464. doi: 10.1038/s41579-022-00710-3
Wang, D., Wei, L., Liu, T., Ma, J., Huang, K., Guo, H., et al. (2023). Suppression of ETI by PTI priming to balance plant growth and defense through an MPK3/MPK6-WRKYs-PP2Cs module. Mol. Plant 16, 903–918. doi: 10.1016/j.molp.2023.04.004
Yan, X., Chen, S., Pan, Z., Zhao, W., Rui, Y., Zhao, L. (2022). AgNPs-triggered seed metabolic and transcriptional reprogramming enhanced rice salt tolerance and blast resistance. ACS Nano. 17, 492–504. doi: 10.1021/acsnano.2c09181
Yang, H., Luo, P. (2021). Changes in photosynthesis could provide important insight into the interaction between wheat and fungal pathogens. Int. J. Mol. Sci. 22, 8865. doi: 10.3390/ijms22168865
Zain, M., Ma, H., Chaudhary, S., Nuruzaman, M., Azeem, I., Mehmood, F., et al. (2023). Nanotechnology in precision agriculture: Advancing towards sustainable crop production. Plant Physiol. Biochem., 108244. doi: 10.2139/ssrn.4663507
Zhang, J., Cook, J., Nearing, J. T., Zhang, J., Raudonis, R., Glick, B. R., et al. (2021a). Harnessing the plant microbiome to promote the growth of agricultural crops. Microbiol. Res. 245, 126690. doi: 10.1016/j.micres.2020.126690
Zhang, P., Guo, Z., Ullah, S., Melagraki, G., Afantitis, A., Lynch, I. (2021b). Nanotechnology and artificial intelligence to enable sustainable and precision agriculture. Nat. Plants. 7, 864–876. doi: 10.1038/s41477-021-00946-6
Zhang, P., Guo, Z., Zhang, Z., Fu, H., White, J. C., Lynch, I. (2020). Nanomaterial transformation in the soil–plant system: implications for food safety and application in agriculture. Small 16, 2000705. doi: 10.1002/smll.202000705
Zhang, P., Jiang, Y., Schwab, F., Monikh, F. A., Grillo, R., White, J. C., et al. (2024). Strategies for enhancing plant immunity and resilience using nanomaterials for sustainable agriculture. Environ. Sci. Technol. doi: 10.1021/acs.est.4c03522
Keywords: agriculture, nanomaterials, immunomodulation, phytopathogens, disease resistance
Citation: Masood HA, Qi Y, Zahid MK, Li Z, Ahmad S, Lv J-M, Shahid MS, Ali HE, Ondrasek G and Qi X (2024) Recent advances in nano-enabled immunomodulation for enhancing plant resilience against phytopathogens. Front. Plant Sci. 15:1445786. doi: 10.3389/fpls.2024.1445786
Received: 08 June 2024; Accepted: 22 July 2024;
Published: 07 August 2024.
Edited by:
Yasser Nehela, University of Florida, United StatesReviewed by:
Fasih Ullah Haider, Chinese Academy of Sciences (CAS), ChinaAmir Raza, University of Arizona, United States
Irfan Manzoor, Government College University, Faisalabad, Pakistan
Copyright © 2024 Masood, Qi, Zahid, Li, Ahmad, Lv, Shahid, Ali, Ondrasek and Qi. This is an open-access article distributed under the terms of the Creative Commons Attribution License (CC BY). The use, distribution or reproduction in other forums is permitted, provided the original author(s) and the copyright owner(s) are credited and that the original publication in this journal is cited, in accordance with accepted academic practice. No use, distribution or reproduction is permitted which does not comply with these terms.
*Correspondence: Xingjiang Qi, cWl4akB6YWFzLmFjLmNu; Zhitao Li, SXp0OTI2MTZAc2luYS5jbg==
†These authors have contributed equally to this work