- 1Graduate School of Integrative Science and Engineering, Tokyo City University, Tokyo, Japan
- 2Department of Natural Sciences, Faculty of Knowledge Engineering, Tokyo City University, Tokyo, Japan
We conducted comparative analyses using an open-top chamber (OTC) to reduce wind stress to clarify the impact of decreased wind stress on the invasive species Bidens pilosa L. (Asteraceae), which ranks among the worst 100 species on the Invasive Alien Species List in Japan. Morphological analyses revealed that the number and size of leaves in the OTC group were significantly higher than those in the control group (wind). There was also a significantly higher investment in stems in the former than in the latter. No significant differences were observed in root dry mass; however, the resource allocation ratio to the roots was significantly higher in the wind group than in the OTC group. Although the total seed mass was greater in the OTC group, there were no significant differences in the ratio of resource allocation to seeds between the groups, and no significant differences were observed in the mass of each seed. However, the number of seeds was significantly higher in the OTC group. Adaptive changes in the leaves, stems, and roots to avoid and/or resist wind were reflected in differences in the number of seeds. In addition, a decrease in wind stress contributed to an increase in the number of seeds in B. pilosa. Such mechanisms are likely widespread because B. pilosa is often highly abundant in urban systems.
1 Introduction
Wind is one of the most ubiquitous environmental stressors that can strongly affect the development, growth, pollination, seed dispersal, insect herbivory, and reproductive yield of plants (Wilcock and Neiland, 2002; Hodges et al., 2004; Fernandez and Hilker, 2007; Turbill, 2008; Shiba et al., 2022c; Takizawa et al., 2022, 2023). Wind is an important factor at many levels in ecosystems, and avoiding wind stress using flexible and easily reconfigurable structures is an alternative strategy (Anten et al., 2009). How plants adapt to or acclimate to variable external forces depends on the intensity and frequency of stress and the plant structure.
Invasive alien plant species pose a serious threat to local biodiversity, ecosystem services, and environmental quality by replacing regionally unique native species with common nonnative species (Godefroid, 2001; Wania et al., 2006; Shochat et al., 2010; Rai and Singh, 2020). Bidens pilosa L. is an invasive plant in Japan and an annual species of herbaceous plants in the family Asteraceae that grows to approximately two meters tall (Figure 1). This species has pinnate leaves, heads consisting of approximately four or five broad white ray florets with many tubular yellow disc florets, and barbed fruits approximately 1 cm long. It is native to America but has expanded as an introduced species in other regions worldwide, including Eurasia, Africa, Australia, South America, and the Pacific Islands. It is widely used as a folk medicine in African and South American countries and is used to treat malaria (Adia et al., 2014), enteritis, nephritis, diarrhea (Chih et al., 1995), and gastric disorders, including peptic ulcers (Alvarez et al., 1999), antimalarial activity (Oliveira et al., 2004; Tobinaga et al., 2009), anti-inflammatory, anti-allergic, immunosuppressive activity (Pereira et al., 1999; Horiuchi and Seyama, 2006), antibacterial activity (Ashafa and Afolayan, 2009; Dagawal and Ghorpade, 2011), anti-leukemia activity (Chang et al., 2001), hypoglycemic activity (Ubillas et al., 2000), hepatoprotective effect (Yuan et al., 2008), and anticancer activity (Sundararajan et al., 2006). B. pilosa grows aggressively in cultivated fields, roadsides, and disturbed lands in the urban areas of Honshu, Kyushu, and Ryukyu, Japan, and often becomes weedy (Koyama, 1995; Asami et al., 1999). For example, in Japan, it has been reported that dragonflies become unable to move after their wings become stuck in the achenes of B. pilosa, resulting in their deaths (Fukasawa and Takahashi, 2017). The majority of the flying insects found on the achenes of B. pilosa were from the family Odonata, which were particularly frequently found was considered to be that their thin wings make it easy for them to pierce the achenes, and that many of them had the habit of using the tips of herbaceous stems as perches (Fukasawa and Takahashi, 2017). They use these perches not only to rest, but also to regulate their body temperature because they are cold-blooded animals that need to be exposed to sunlight to heat up during low temperatures, and they often rest in high places such as the tips of stems to heat up efficiently (e.g., Iwasaki et al., 2009). One of the reasons why many dragonflies found themselves on the achenes at high points of B. pilosa was that these places were easily exposed to sunlight. In addition, because B. pilosa inhibits the growth of other plants through allelopathy (Uraguchi et al., 2003), B. pilosa tends to be the only plant available for perching in the vicinity, which may increase the possibility of dragonflies becoming entangled in the achenes and dying. Therefore, the Ministry of the Environment has placed B. pilosa among the worst 100 species on the Invasive Alien Species List of Japan and urged caution against its growth. However, the barbed awns of fruits are stuck in the clothing, fur, fleeces, and socks of people who brush against this species and are dispersed by people (anthropochory), expanding their distribution in various parts of Japan.
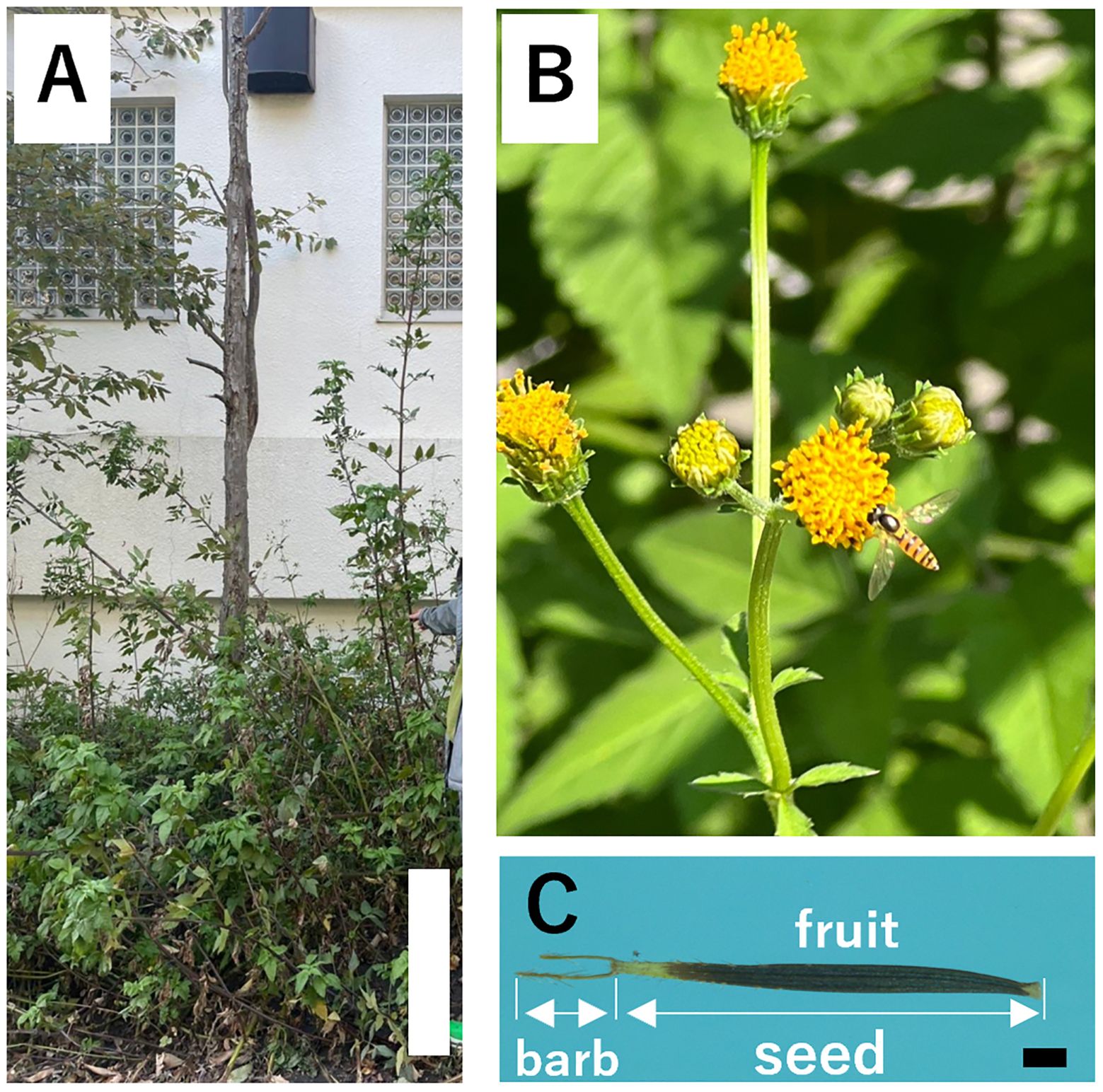
Figure 1. Bidens pilosa var. pilosa (bar = 50 cm) (A). Inflorescences (B) and seed (bar = 1 mm) (C).
With respect to seed germination after dispersal of B. pilosa, Chauhan et al. (2019) reported that germination was stimulated by light. Thus, its emergence was the greatest on the soil surface, indicating that seeds transported by people can easily germinate anywhere. Moreover, previous studies on the effects of this species on the environment indicate that dispersing seeds into cropping fields is becoming widely recognized as a major impediment to economic food production (Reddy and Singh, 1992). Even more concerning is that some biotypes of B. pilosa have acquired herbicide resistance. For example, López-Ovejero et al. (2006) reported that acetolactate synthase (ALS)-inhibiting herbicide-resistant biotypes of B. pilosa in Brazil and other biotypes in Mexico, were resistant to several herbicides, including glyphosate and paraquat (Alcántara-de la Cruz et al., 2016). The explosive spread of B. pilosa in urban cities may help it spread to various areas, including cropping fields, through seeds attached to people’s clothes. Therefore, an urgent examination of the reproductive strategies of B. pilosa in urban environments is important for developing an effective integrated management program for this species. In the present study, we examined the reverse chain of cause and effect and investigated how wind patterns alter B. pilosa productivity.
Which system best compares plant impacts on wind stress reduction? Experiments on the effects of wind on plant growth have been conducted using fans, wind tunnels, and artificial shelters (Cleugh et al., 1998). However, few field experiments have manipulated wind to determine its effects on wild plant growth (Bang et al., 2010). We selected a windbreak design to limit the impact of shading and enable direct measurement of the wind effect, although some wind turbulence is inevitable when constructing wind barriers (Moen, 1974). Open-top chambers (OTC) were first used to study the effects of air pollution and were later adapted for elevated CO2 research (Maggio et al., 2009), They are often used to warm aboveground systems, especially in Arctic and alpine ecosystems (Walker et al., 2006). However, as the temperature inside an OTC increases, it is necessary to consider the effects of temperature and wind. Bang et al. (2010) reported that leaving a 15–20 cm opening near the ground without a roof when protected by windbreak shields could prevent the greenhouse effect. This simple design is ideal for examining the effects of wind stress on plants. Therefore, we established an OTC (Figure 2) and conducted an experiment to compare the growth of B. pilosa. This comparison revealed the effect of wind on the growth and the morphology of B. pilosa in an environment with reduced wind stress. In addition to them, seed production plays a major role in determining the final plant fitness and is an important factor in the ecology and evolution of plant life histories (Donohue, 2005; Dwyer and Erickson, 2016; Ishii et al., 2022; Marui et al., 2023). Thus, consideration of the reproduction of invasive species in Japan requires attention to seed production. Many studies have been conducted on the number and size of seeds in seed production (e.g., LePage et al., 2000; Moles and Westoby, 2004; Jones and Muller-Landau, 2008; Muller-Landau et al., 2008). Qiu et al. (2021) reported that seed production varies depending on the size of the individual, and therefore the number of seeds can vary greatly within a species (Qiu et al., 2022), indicating that it is necessary to analyze the differences in seed production of B. pilosa. This study aimed to clarify the effects of stress reduction on the growth and production of B. pilosa using OTC.
2 Materials and methods
2.1 Cultivation conditions
Sprouts of Bidens pilosa var. pilosa was confirmed on April 18, 2022, in a hedge on the campus of Tokyo City University, and the plants were successively replanted in polypods (7.5 cm diameter, 220 mL volume) on May 26, when the true leaves were observed to have developed. Individuals collected at this site were confirmed to have grown and fruited with the parental individuals in the previous year. For cultivation, black soil was used, and plants were grown in polypods (7.5 cm diameter, 220 mL volume) and replanted into 12-cm (830 mL volume) and 18-cm (2900 mL volume) diameter pots when roots started to grow through the drainage holes to avoid root clogging during plant growth. Before the cultivation experiment, 36 B. pilosa var. pilosa plants grown in OTC without direct wind protection (hereafter referred to as the ‘OTC group’) and 36 plants grown without wind protection (hereafter referred to as the ‘wind group’) were sorted to ensure that individual size was not biased. In the OTC group, one individual died during cultivation, so the final number of plants was 35.
The cultivation experiment was conducted on June 20 on the rooftop of Building 6 of Tokyo City University. The plants in both groups were arranged in a 6 × 6 configuration. In this environment, wind speeds greater than 6 m/s were recorded using a handheld anemometer on cloudy days. The OTC group was not directly exposed to wind because the anemometer propeller did not rotate. However, an air inlet approximately the size of a pot is left open to eliminate the greenhouse effect. Although the outer pots are somewhat more exposed to external boundary effects than the inner pots, they are not directly subjected to wind loads by the plant body, which is not considered to be within the range of influence for this study. When typhoons passed, the plants were temporarily evacuated indoors to avoid damage from overtopping by strong winds. Watering during cultivation was performed until the water pool was filled at least once a day. Watering was not performed on days when the soil surface was not dry enough to prevent root rot.
For wind speed data during the cultivation period, historical weather data recorded by the Automated Meteorological Data Acquisition System (AMeDAS) set up by the Japan Meteorological Agency was used. AMeDAS data from nearby Fuchu and Tokyo were used as wind-speed data during the growing season. For wind speed data, the average and maximum wind speeds during a week were used for the period from June 21 to November 1 (Figure 3).
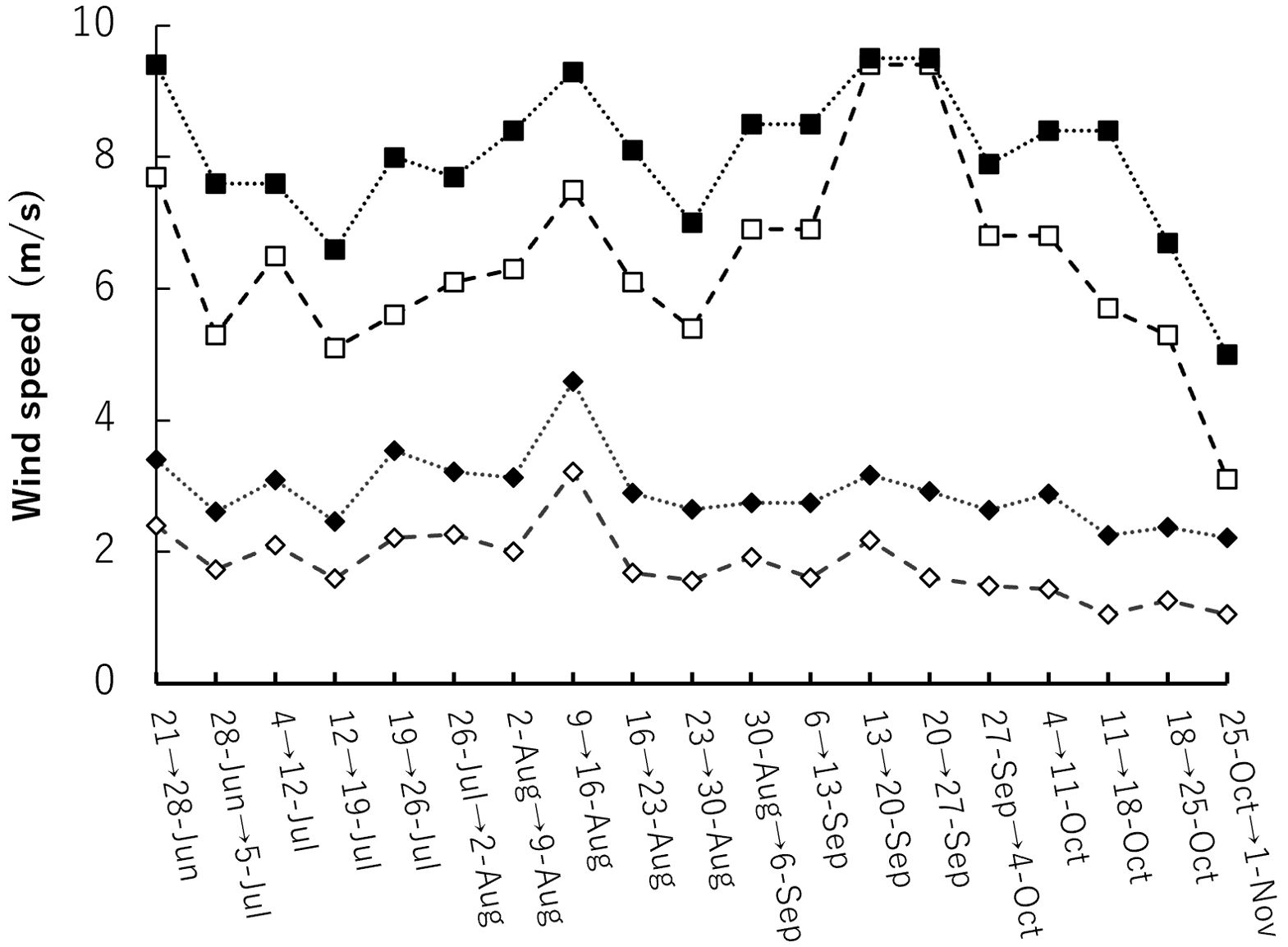
Figure 3. Wind speeds during the experimental period near the cultivation site. The data used were AMeDAS data at year 2022. Closed solid indicates the maximum weekly wind speed in Tokyo and open solid indicates the maximum weekly wind speed in Fuchu. Closed rhombus indicates the weekly average wind speed in Tokyo and open rhombus indicates the weekly average wind speed in Fuchu.
2.2 Open-top chamber
To reduce wind stress, a self-made OTC was created; the OTC was covered with horticultural plastic sheeting (0.1 mm × 1.85 × 5 m) on the lateral side of the frame and fixed to the floor with the canopy open. An air inlet for circulation was installed at a height that did not exceed the pot size in front of the floor to eliminate the greenhouse effect inside the OTC, as described by Bang et al. (2010). The air inlet eventually reached a height of approximately 15 cm.
The reduction in radiation and changes in spectral composition near the chamber walls of the OTC could affect plant production and morphology (Hollister et al., 2023). Some studies have reported that the chamber wall reduces photosynthetic photon flux density by more than 10% (Drake et al., 1989; Nakashima et al., 2018; May et al., 2022; Hollister et al., 2023). However, because the OTC is open at the top, the photosynthetic photon flux density reduces the light the most in the morning and evening when the sun is at a low angle. However, around noon, the rate of its reduction by the chamber walls becomes very small because sunlight directly reaches the plants inside the OTC.
2.3 Growth rate measurements of above ground morphology.
Stem and leaf measurements were performed to determine the morphogenetic patterns of the aboveground parts as they grew under different wind stress environments.
Node counts were used as indicators of leafing, and the number of nodes per individual was counted. The measurements were performed once a week between June 21 and November 2, 2022. Three fully expanded leaves, starting from the uppermost leaf, were selected and photographed to determine the leaf area. This was analyzed using “ImageJ” image analysis software, and the average of three values was calculated. The leaf area was measured once a week between July 8 and August 26, 2022. On September 16, inflorescences began to form, and leaf development decreased.
Stem length and diameter at the base were measured using digital calipers (CD-15APX; Mitutoyo, Japan). The stem diameter was measured three times, and the average of the three measurements was used as the representative value for each individual.
The growth rate of one individual was calculated using the following equation:
where G is the growth rate of elongation per day divided by the weekly elongation of the stem divided by seven days, l is the stem length on the day of measurement, and lweek is the stem length seven days after l.
The number of inflorescences per individual was measured between September 27 and December 6 The data from November 11, when the highest number of inflorescences developed in both groups, were compared.
Seeds were harvested by wrapping the fruits in nets to prevent seed loss from inflorescences. The number of seeds per individual and average seed mass were measured for the harvested seeds.
After November 1, morphometric measurements were terminated because the stems and leaves had stopped growing, and dead leaves were collected from November 1 onward. December 22, the absence of new organogenesis was confirmed, and the Biomass analysis was initiated.
2.4 Biomass allocation
After cultivation, the plants were cut into roots, stems, and leaves and dried in a dryer (DRM420DD; Advantec, Japan) set at 100°C for at least 72 h. After removing the dried samples from the dryer, dry mass was immediately measured using an electronic balance (ATX224R; Shimadzu, Japan). The masses of naturally dried seeds were also combined to calculate the resource-partitioning ratio of the roots, stems, leaves, and seeds in one individual using the following equation:
2.5 Statistical analysis
The R software was used for statistical analysis (R Core Team, 2024). After equal variances were obtained by comparing each measured item (F-test), t-tests were performed under the assumption of “equal variances” or “not equal variances.” The Student’s t-test was used when equal variances could be assumed, and Welch’s t-test was used when equal variances could not be assumed. For the comparison of Biomass allocation, which is a comparison of three or more groups, the Tukey–Kramer test was used.
3 Results
The results of the F-test analysis of variance showed that equal variances can be assumed for the number of inflorescences, number of seeds per individual, mass per seed, total seed mass per individual, dry mass of roots, and dry mass ratio above/underground, whereas equal variances cannot be assumed for the dry mass of stems and leaves.
3.1 Results of aboveground morphological measurements
The node count results are shown in Figure 4A. Significant differences were observed from June 28 to August 30, September 27 to October 4, and October 18 to November 1, with the node numbers tending to be higher in the OTC group during the cultivation period. Finally, the OTC group had 24.8 ± 0.24 (mean ± SE) nodes, and the Wind group had 23.9 ± 0.22 nodes, indicating that the OTC treatment significantly increased the number of nodes.
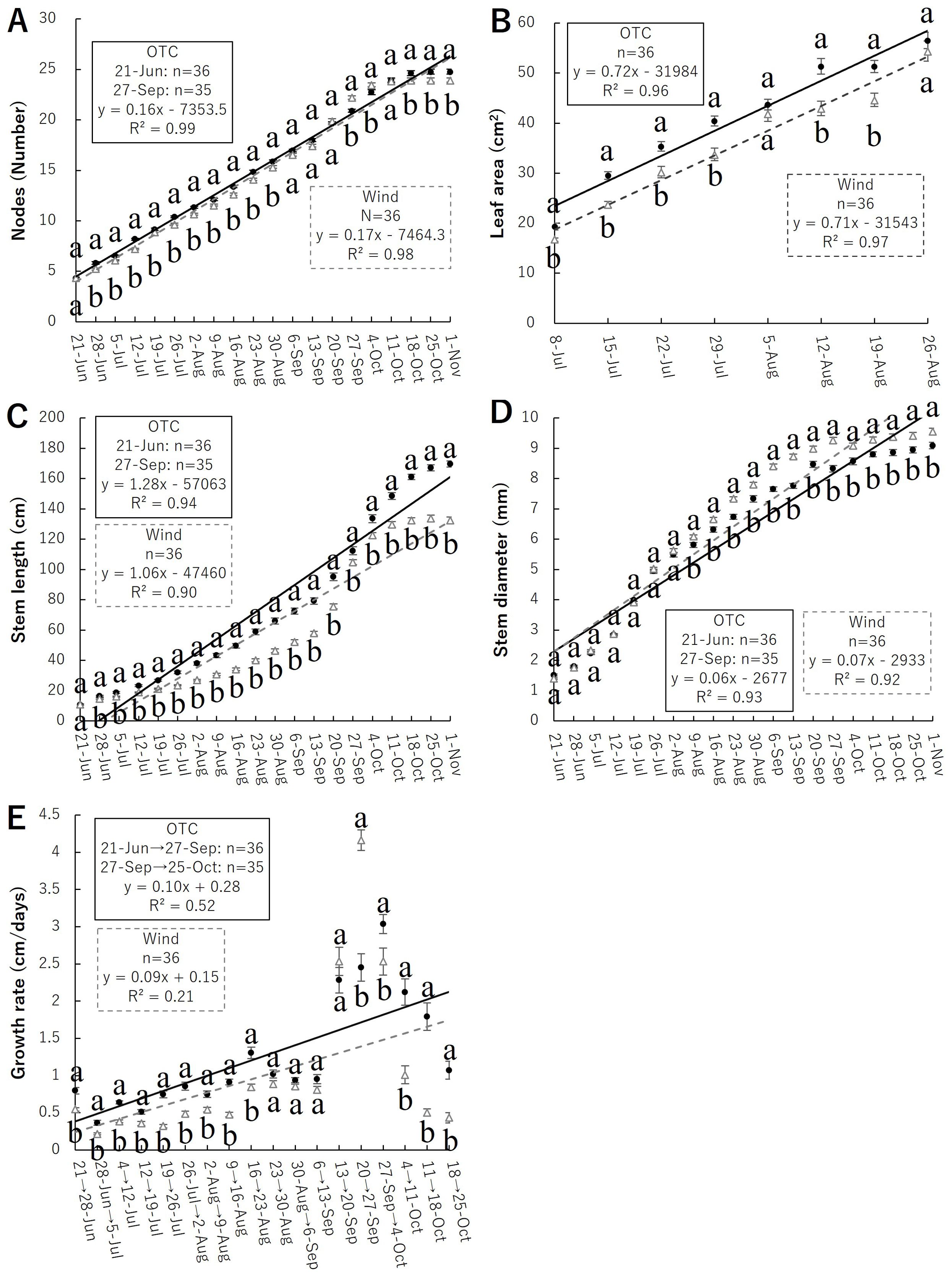
Figure 4. Relationship of morphological results; number of nodes (A) and leaf area (B), stem length (C), stem diameter (D), growth rate (E); closed circle indicate OTC and open triangle indicates wind. Plots marked with different letters differ significantly according to t-tests (p < 0.05). Linear approximations are indicated by solid lines for OTC and dashed lines for Wind.
Leaf area results are shown in Figure 4B. Measurements of leaf area to budding showed significant differences between July and August 12–19, with the leaf area tending to be larger in the OTC group during the cultivation period. Both groups displayed the maximum leaf area during the growing season when measured on August 26, with 56.53 ± 1.64 cm² for the OTC group and 54.40 ± 2.01 cm² for the Wind group. However, no significant differences were observed between groups.
The stem length results are shown in Figure 4C. The final stem length measurements showed that that of the OTC group was 1,696.54 ± 21.04 mm and that of the Wind group was 1,323.78 ± 22.48 mm, indicating that the OTC group was significantly taller than the Wind group.
Stem diameter at the base is shown in Figure 4D. The final results of stem diameter measurements showed that that of the OTC group was 9.09 ± 0.11 mm and that of the Wind group was 9.55 ± 0.12 mm, indicating that the stems of the Wind group was significantly thicker than the OTC group.
Growth rate results are shown in Figure 4E. The growth rate was significantly higher in the OTC group from June 21 to August 23. Subsequently, the growth rates were similar until September 20; however, from September 20 to 27, the growth rate was significantly higher in the wind group. After September 27, the growth rates of both groups decreased but were significantly higher in the OTC group.
The number of inflorescences per individual is shown in Figure 5. Finally, the total number of inflorescences per individual in the OTC group was 49.69 ± 2.83, while the total number of inflorescences per individual in the wind group was 43.94 ± 1.96, but no significant difference was observed.
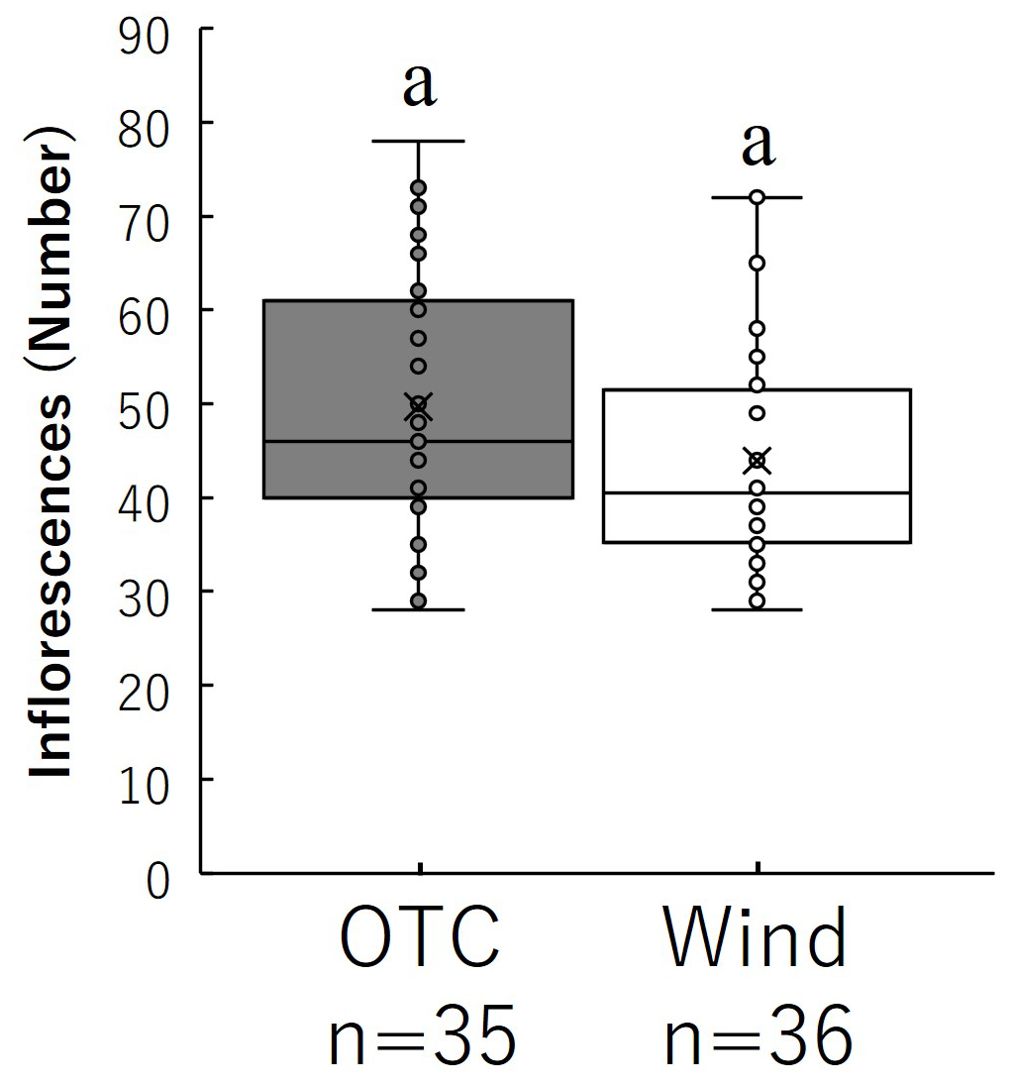
Figure 5. Comparison of the number of inflorescences during growth. Box whisker plots marked with identical letters were not significantly different according to t-test (p < 0.05). Crosses indicates position of the mean. Table 1 shows the average values.
The number of seeds per individual and average seed mass per seed are shown in Figure 6 and Table 1. The number of seeds per individual was significantly higher in the OTC group than in the WT-treated group (Figure 6A). Average seed mass per seed was not significantly different between the two groups (Figure 6B). Total seed mass per individual was significantly higher in the OTC group than in the Wind group (Figure 6C). These results indicate that the OTC group had a significantly higher total seed mass per individual because of the higher number of seeds.
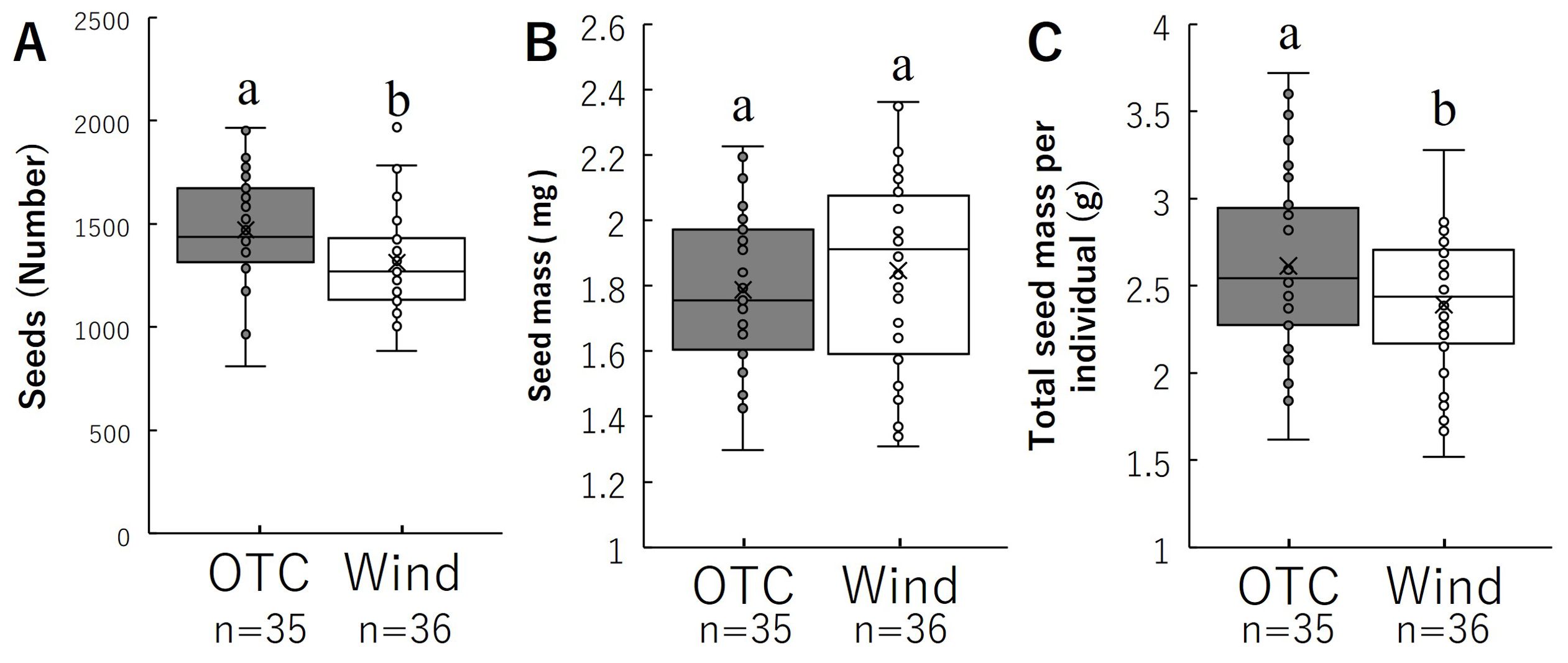
Figure 6. Comparison of number of seeds (A) and average seed mass (B), total seed mass per individual (C). Box whisker plots marked with different letters differ significantly according to t-test (p < 0.05). Crosses indicates position of the mean. Table 1 shows the average values.
3.2 Biomass and allocation rates for each organ
The dry mass results for each organ are shown in Figure 7 and Table 1. There was no significant difference in the root dry mass per individual between the two groups. However, the dry masses of stems and leaves per individual were significantly higher in the OTC group than in the wind group. Aboveground (stem and leaf) to belowground (root) dry mass ratios are shown in Figure 7D. The aboveground and underground dry mass ratios were significantly higher in the OTC group than in the wind group. These results indicate that the OTC group invested significantly more in aboveground areas than the wind group.
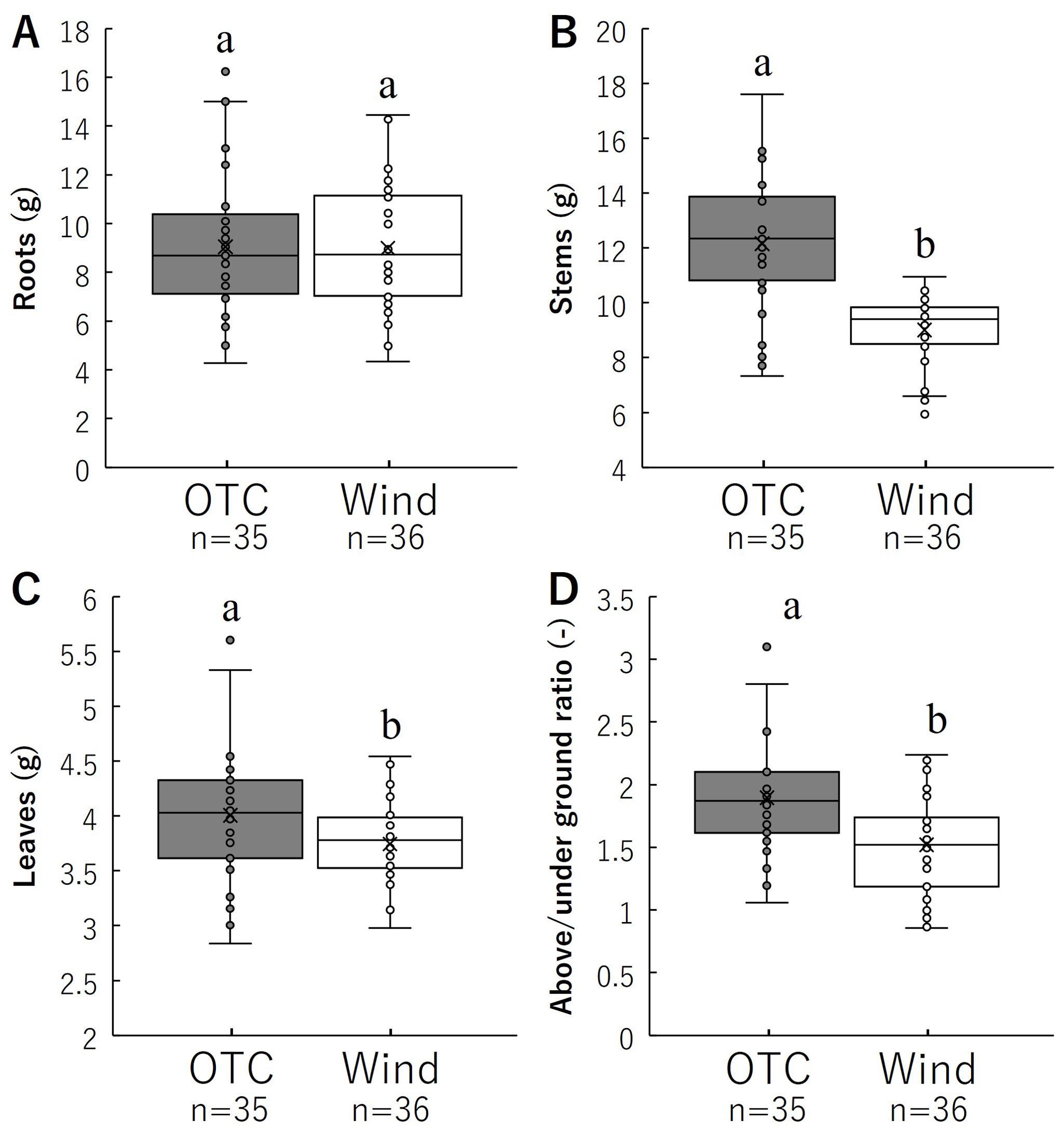
Figure 7. Comparison of roots (A) and stems (B), leaves (C), above/under ground ratio (D) for biomass. Box whisker plots marked with different letters differ significantly according to t-test (p < 0.05). Table 1 shows the average values.
The biomass allocation results are presented in Table 2. A comparison of the biomass allocation rates within the OTC group showed that the rates of investment in roots, stems, leaves, and seeds differed significantly, with the highest rates of investment in stems. Within the Wind treatment group, significantly different investment rates were observed for leaves and seeds than for roots and stems.
4 Discussion
Plants adapt to various environmental stressors by acquiring characteristics that allow them to avoid or tolerate them (e.g., Shiba and Fukuda, 2024). Wind is an environmental factor that has various effects on plants. Plant responses to wind typically include reduced photosynthesis (Clark et al., 2000; Burgess et al., 2016), decreased leaf area (Anten et al., 2010; Shiba et al., 2023), inhibition of stem elongation, and an increase in stem diameter to mechanically stabilize the plant (Coutand et al., 2000; Anten et al., 2010; Bang et al., 2010; Shiba et al., 2024b). Understanding the responses of each plant organ to external resources and the environment is central to studying plant diversification. Plant fitness is an important means of evaluating plant responses to environmental factors (Buonaiuto and Wolkovich, 2021; Creux et al., 2021) and serves as an index for evaluating the adaptability of plant characteristics to specific environments and the contribution of individual plants to the production of the next generation (Strauss, 1997). As the resources needed for the growth of roots and leaves, flowering, fruiting, and seed production are not the same, plant investment in these activities is not fixed (Ansaldi et al., 2018; Rusman et al., 2020), and resources are finite, resulting in life history trade-offs in plants (Li et al., 2015). Our results showed that the root mass of B. pilosa was not significantly different between the OTC and Wind treatments; however, the allocation ratio was significantly higher in the latter (Figures 7A, D; Table 2). Wind also improves plant anchorage by strengthening root development (Danjon et al., 2005; Tamasi et al., 2005; Štofko and Kodrík, 2008), and some studies have indicated that wind affects root growth and biomass allocation to roots (Cleugh et al., 1998; Poorter et al., 2012; Gardiner et al., 2016; Feng et al., 2019). Plant anchorage in the soil generally depends on the root system architecture, and the wind group of B. pilosa can acquire resistance to wind by allocating resources to the roots. Even the underground parts of B. pilosa were shown to be very different between the Wind and OTC groups, and this species also had a large morphological change in each aboveground organ compared to the roots. However, as B. pilosa is an annual herb with no significant difference in root mass (Figure 7A), it cannot be expected to actively establish itself in areas with extreme winds and will have difficulty growing in environments where it cannot resist this root mass.
Our results indicated that the size and number of leaves in the OTC group of B. pilosa were significantly larger and higher, respectively, than those in the wind group (Figures 4A, B). Regarding the relationship between wind and leaf size, Shiba et al. (2023) indicated that the leaf size of Farfugium japonicum (L.) Kitam. var. japonicum (Asteraceae) decreased with increasing wind speed based on wind speed data from the Automated Meteorological Data Acquisition System (AMeDAS) of the Japan Meteorological Agency. In this study, the decreased leaf size in the wind group was a strategy to minimize wind stress and avoid stem lodging, which leads to the physical collapse of photosynthetic capacity and can occur spontaneously owing to the mechanical instability of the stem structure. Therefore, the number of leaves in the wind group decreased to reduce the stress placed on the stem by the wind. In addition, our results indicated that the wind group had significantly shorter stems with increased diameters than the OTC group (Figures 4C, D), indicating that B. pilosa in the wind group was modified to gain resistance to strong winds. These results indicate the general trends in the effects of wind on plants. In addition, our growth results showed an increase in the stem diameter in the wind group after leaf dwarfing. With respect to these morphological differences, both stem and leaf resources were significantly lower in the Wind group, suggesting that wind stress hindered investment in stems and leaves (Figures 7B, C; Table 1). What is the relationship between the increase in the proportion of roots and the decrease in stem length, leaf area, and stem number in the wind group? Plants use many trade-off strategies for resource acquisition and distribution to achieve optimal fitness. A comparison of the allocation ratio between the belowground (roots) and aboveground (leaves and stems) parts of B. pilosa indicated a trade-off relationship (Figure 7D). In particular, the OTC group had the highest investment in stems in terms of resource investment ratio, while the Wind group had similar rates of investment in stems and roots (Table 2). This suggests that wind increased the resource allocation ratio of roots and decreased the resource allocation ratio of stems, resulting in individuals in the Wind group having increased resistance and avoidance to wind by reducing the moment on the stem. In addition, the Wind group has shown low leaf biomass and enlarged stems (Figures 4D, 7C), suggesting that resistance and avoidance of wind loads is also demonstrated in the above-ground morphology other than low stem length. Was the high investment in leaves during vegetative growth linked to the high assimilation of this plant, which affected reproductive growth in the OTC group of B. Pilosa, such as flowers and seeds? This is discussed as follows.
Floral display is fundamental to plant fitness and can affect pollinator visitation rate and total seed production (Bell, 1985). A common feature of plant reproduction is the production of many more flowers than mature fruits (Stephenson, 1981; Sutherland and Delph, 1984; Sutherland, 1986a, b). Our results indicated that the number of inflorescences did not differ significantly between the OTC and Wind groups (Figure 5), suggesting that B. pilosa had a similar number of inflorescences regardless of vegetative growth. How can small wind groups produce many inflorescences during vegetative growth? Our results showed that the wind treatment group experienced a rapid acceleration in stem elongation when petioles and inflorescences began to emerge (Figure 4E). As the peduncle of B. pilosa emerges from nodes with leaves, the wind group could have exhibited rapid stem elongation to increase the number of nodes to form many peduncles. The pool allocated to floral function is limited, and there should be some trade-offs among the behaviors in floral displays (Worley and Barrett, 2000; Caruso, 2004). For example, there are trade-offs in reproductive allocation between flower size and number (Sargent et al., 2007), fruit mass, and seed number for optimal fitness (Sun et al., 2011; Atlan et al., 2015). Our results indicate that the amount of resources allocated to seeds was greater in the OTC group than in the wind group, but the resource allocation ratio in the latter was higher than in the former (Table 2). However, there was no significant difference in the number of inflorescences between groups. Why was the Wind group able to form as many inflorescences as the OTC group despite having fewer leaves for photosynthate acquisition? Although the answer to this question could not be determined from the results of this study, B. pilosa is often seen on leaves, even in the cold winter months in Japan. Therefore, this species seems to continue to invest the resources acquired through photosynthesis into seed production, even during that period.
The number and size of seeds are important evolutionary traits that affect the reproductive outcomes of many plant species and have been found to vary between and within plant species (Leishman et al., 1995; Silvertown and Bullock, 2003; Moles and Westoby, 2006). The number of seeds is the most direct measure of fitness (Thomson and Hadfield, 2017), and seed size directly influences germination time, germination percentage, and seedling vigor and indirectly determines plant distribution and abundance across different habitats (van Mölken et al., 2005; Silveira et al., 2012). Therefore, it is important to determine the effects of decreased wind stress on B. pilosa. The effects of wind stress on seed production have been studied using several methods. Our results showed that the number of seeds in the OTC treatment was significantly higher than that in the wind treatment (Figure 6A); however, the mass per seed was not significantly different between the OTC and wind treatments (Figure 6B). These results showed that both groups had similar seeds, but the OTC group produced more seeds than the wind group. This significantly affected the formation of offspring, suggesting that the OTC group could produce more offspring than the wind group of B. pilosa. The question arose as to why seed number increased in OTC but seed size remained unchanged. It has been reported that increases in weight per seed in animal-adhesive seeds such as B. pilosa often have the negative effect on seed dispersal (Kiviniemi and Telenius, 1998). Recently, Qiu et al. (2022) indicated that seed number could vary by more than ten orders of magnitude between different environments. In addition, seeds of B. pilosa already have high germination and growth rates easily anywhere (Chauhan et al., 2019), so increasing seed size, which would have a positive effect on germination rates, was not necessary for this species. Considering these, B. pilosa had increased only seed number without changing the weight per seed in order to maintain dispersal for successful widespread distribution.
The OTC group may have had many seeds because they were able to increase the amount of resource investment in leaves; that is, adaptive changes in leaves and roots to avoid and/or resist wind stress caused the wind group to decrease the amount of resource investment in leaves, which was reflected in the number of seeds. Many studies have reported that plants adapt to avoid environmental stress by changing the morphological and anatomical traits of their leaves in various environments (Yamada et al., 2011; Hayakawa et al., 2012; Ohga et al., 2012a, b, 2013; Ueda et al., 2012; Yokoyama et al., 2012; Kumekawa et al., 2013; Matsui et al., 2013; Sunami et al., 2013; Shiba et al., 2021, 2022a, 2022b, 2022c, 2024a; Takizawa et al., 2022, 2023). These changes in leaves are related to photosynthetic efficiency; therefore, adaptation processes in these environments may also be involved in reducing the number of seeds. Disc florets of B. pilosa have self-compatibility, but insects such as hymenopterans and lepidopterans frequently visit and pollinate this species (Grombone-Guaratini et al., 2004; Budumajji and Solomon-Raju, 2018). However, wind significantly influences flying insects and is an important factor in the spatiotemporal heterogeneity of pollinator flights, influencing insect dispersal and movement. For example, Lewis and Smith (1969) reported that installing windbreaks in orchards tripled the number of insects visiting, and Koga and Namai (2001) revealed the influence of wind on insect pollination of carrot (Daucus carota L.) plants. Wind currents are also highly variable and have been reported to affect the stability of pollinator flight and flower landing (Combes and Dudley, 2009; Ravi et al., 2013; Chang et al., 2016). In particular, Chang et al. (2016) indicated that the entry path of honeybees to flowers shifted from multiple directions in still air to one direction in the wind, regardless of flower orientation. In addition, honeybees attempting to land in stronger headwinds did not decelerate smoothly but maintained high flight speeds until contact, resulting in higher peak decelerations upon impact (Chang et al., 2016). These studies showed that wind had a strong influence on the behavior of pollinators and that the decrease in the number of seeds in the wind-affected population of B. pilosa may also be related to the influence of wind on the behavior of pollinators. Recently, Budumajji and Solomon-Raju (2018) reported that most foraging visits to the flowers of B. pilosa were by butterflies and bees, although wasps and honeybees were also involved in foraging visits. In the future, it will be necessary to compare the number of visits between the OTC and Wind populations of B. pilosa to determine the effects of wind on pollinators.
The reproduction of invasive plants in urban areas is an important new area of research that will improve the prediction and management of further spread. Urban environments support the invasive species B. pilosa, which ranks among the worst 100 species on the Invasive Alien Species List in Japan. Such mechanisms are likely widespread because B. pilosa is often highly abundant in urban systems. Thus, B. pilosa and other invasive alien species can exploit urban environments and invest in traits that enhance their reproductive success. The Ministry of the Environment has designated 233 taxa in Asteraceae as invasive species, six species other than B. pilosa (i.e., B. aristosa (Michx.) Britton, B. aurea (Aiton) Sherff, B. bipinnata L., B. frondosa L., B. laevis (L.) Britton, Sterns et Poggenb., and B. parviflora Willd.) has been designated as an invasive species in Japan. Future studies should synthesize and use the results of this study as a basis for enhancing the colonization, dispersal, and ecological effects on reproduction.
Data availability statement
The datasets presented in this study can be found in online repositories. The names of the repository/repositories and accession number(s) can be found in the article/Supplementary Material.
Author contributions
MS: Writing – review & editing, Writing – original draft, Software, Methodology, Investigation, Data curation, Conceptualization, Funding acquisition. NK: Writing – review & editing, Writing – original draft, Project administration, Methodology, Investigation, Data curation, Conceptualization. SH: Writing – review & editing, Writing – original draft, Software. TF: Writing – review & editing, Writing – original draft, Supervision, Funding acquisition.
Funding
The author(s) declare financial support was received for the research, authorship, and/or publication of this article. This work was supported by JSPS KAKENHI Grant Numbers JP21K06324,JP24KJ2045.
Acknowledgments
We thank Drs. Kawate T, Kondo S, Sato N, Sato R, Takashima K, Yasuda T, Noriyasu R, Watanabe H, Takizawa E, and Tsubouchi H for their advice regarding this study.
Conflict of interest
The authors declare that the research was conducted in the absence of any commercial or financial relationships that could be construed as a potential conflict of interest.
Publisher’s note
All claims expressed in this article are solely those of the authors and do not necessarily represent those of their affiliated organizations, or those of the publisher, the editors and the reviewers. Any product that may be evaluated in this article, or claim that may be made by its manufacturer, is not guaranteed or endorsed by the publisher.
Supplementary material
The Supplementary Material for this article can be found online at: https://www.frontiersin.org/articles/10.3389/fpls.2024.1445437/full#supplementary-material
References
Adia, M. M., Anywar, G., Byamukama, R., Kamatenesi-Mugisha, M., Sekagya, Y., Kakudidi, E. K., et al. (2014). Medicinal plants used in malaria treatment by Prometra herbalists in Uganda. J. Ethnopharmacol. 155, 580–588. doi: 10.1016/j.jep.2014.05.060
Alcántara-de la Cruz, R., Fernández-Moreno, P. T., Ozuna, C. V., Rojano-Delgado, A. M., Cruz-Hipolito, H. E., Domínguez-Valenzuela, J. A., et al. (2016). Target and non-target site mechanisms developed by glyphosate-resistant hairy beggarticks (Bidens pilosa L.) groups from Mexico. Front. Plant Sci. 7. doi: 10.3389/fpls.2016.01492
Alvarez, A., Pomar, F., Sevilla, M. A., Montero, M. J. (1999). Gastric antisecretory and antiulcer activities of an ethanolic extract of Bidens pilosa L. var. radiata Schult. Bip. J. Ethnopharmacol. 67, 333–340. doi: 10.1016/s0378-8741(99)00092-6
Ansaldi, B. H., Weber, J. J., Franks, S. J. (2018). The role of phenotypic plasticity and pollination environment in the cleistogamous, mixed mating breeding system of Triodanis perfoliata. Plant Biol. (Stuttg). 20, 1068–1074. doi: 10.1111/plb.12877
Anten, N. P. R., Alcalá-Herrera, R., Schieving, F., Onoda, Y. (2010). Wind and mechanical stimuli differentially affect leaf traits in Plantago major. New Phytol. 188, 554–564. doi: 10.1111/j.1469-8137.2010.03379.x
Anten, N. P. R., von Wettberg, E. J., Pawlowski, M., Huber, H. (2009). Interactive effects of spectral shading and mechanical stress on the expression and costs of shade avoidance. Am. Nat. 173, 241–255. doi: 10.1086/595761
Asami, K., Yamato, M., Hattori, T., Akamatsu, H., Takeda, Y. (1999). Floristic composition and process of establishment of the Bldens pilosa var. minor-Imperata cyilndrica community maintained by cutting on nonarable land in Okinawa Prefecture. J. Veg. Sci. 16, 1–11. doi: 10.15031/vegsci.16.1
Ashafa, A. O. T., Afolayan, A. J. (2009). Screening the root extracts from Bidens pilosa L. var. radiata (Asteraceae) for antimicrobial potentials. J. Med. Plants Res. 3, 568–572.
Atlan, A., Hornoy, B., Delerue, F., Gonzalez, M., Pierre, J. S., Tarayre, M. (2015). Phenotypic plasticity in reproductive traits of the perennial shrub Ulex europaeus in response to shading: A multi-year monitoring of cultivated clones. PloS One 10, e0137500. doi: 10.1371/journal.pone.0137500
Bang, C., Sabo, J. L., Faeth, S. H. (2010). Reduced wind speed improves plant growth in a desert city. PloS One 5, e11061. doi: 10.1371/journal.pone.0011061
Bell, G. (1985). On the function of flowers. Proc. R. Soc Lond. B. 22, 223–265. doi: 10.1098/rspb.1985.0031
Budumajji, U., Solomon-Raju, A. J. (2018). Pollination ecology of Bidens pilosa L. (Asteraceae). Taiwania 63, 89–100. doi: 10.6165/tai.2018.63.89
Buonaiuto, D. M., Wolkovich, E. M. (2021). Differences between flower and leaf phenological responses to environmental variation drive shifts in spring phenological sequences of temperate woody plants. J. Ecol. 109, 2922–2933. doi: 10.1111/1365-2745.13708
Burgess, A. J., Retkute, R., Preston, S. P., Jensen, O. E., Pound, M. P., Pridmore, T. P., et al. (2016). The 4-dimensional plant: effects of wind-induced canopy movement on light fluctuations and photosynthesis. Front. Plant Sci. 7. doi: 10.3389/fpls.2016.01392
Caruso, C. M. (2004). The quantitative genetics of floral trait variation in Lobelia: potential constraints on adaptive evolution. Evolution 58, 732–740. doi: 10.1111/j.0014-3820.2004.tb00406.x
Chang, J. J., Crall, J. D., Combes, S. A. (2016). Wind alters landing dynamics in bumblebees. J. Exp. Biol. 219, 2819–2822. doi: 10.1242/jeb.137976
Chang, J. S., Chiang, L. C., Chen, C. C., Liu, L. T., Wang, K. C., Lin, C. C. (2001). Antileukemic activity of Bidens pilosa L. var. minor (Blume) sherff and Houttuynia cordata Thunb. Am. J. Chin. Med. 29, 303–312. doi: 10.1142/S0192415X01000320
Chauhan, B. S., Ali, H. H., Florentine, S. (2019). Seed germination ecology of Bidens pilosa and its implications for weed management. Sci. Rep. 9, 16004. doi: 10.1038/s41598-019-52620-9
Chih, H. W., Lin, C. C., Tang, K. S. (1995). Anti-inflammatory activity of Taiwan folk medicine “ham-hong-chho” in rats. Am. J. Chin. Med. 23, 273–278. doi: 10.1142/S0192415X95000328
Clark, A. J., Landolt, W., Bucher, J. B., Strasser, R. J. (2000). How wind affects the photosynthetic performance of trees: quantified with chlorophyll a fluorescence and open-top chambers. Photosynthetica 38, 349–360. doi: 10.1023/A:1010909201307
Cleugh, H. A., Miller, J. M., Böhm, M. (1998). Direct mechanical effects of wind on crops. Agrofor. Syst. 41, 85–112. doi: 10.1023/A:1006067721039
Combes, S. A., Dudley, R. (2009). Turbulence-driven instabilities limit insect flight performance. Proc. Natl. Acad. Sci. U.S.A. 106, 9105–9108. doi: 10.1073/pnas.0902186106
Coutand, C., Julien, J. L., Moulia, B., Mauget, J. C., Guitard, D. (2000). Biomechanical study of the effect of a controlled bending on tomato stem elongation: global mechanical analysis. J. Exp. Bot. 51, 1813–1824. doi: 10.1093/jexbot/51.352.1813
Creux, N. M., Brown, E. A., Garner, A. G., Saeed, S., Scher, C. L., Holalu, S. V., et al. (2021). Flower orientation influences floral temperature, pollinator visits and plant fitness. New Phytol. 232, 868–879. doi: 10.1111/nph.17627
Dagawal, M. J., Ghorpade, D. S. (2011). Antimicrobial activity of an ethnomedicinal plant Bidens pilosa L. Int. J. Pharm. Sci. Res. 2, 2237–2238. doi: 10.13040/IJPSR.0975-8232.2(8).2237-38
Danjon, F., Fourcaud, T., Bert, D. (2005). Root architecture and wind-firmness of mature Pinus pinaster. New Phytol. 168, 387–400. doi: 10.1111/j.1469-8137.2005.01497.x
Donohue, K. (2005). Niche construction through phenological plasticity: life history dynamics and ecological consequences. New Phytol. 166, 83–92. doi: 10.1111/j.1469-8137.2005.01357.x
Drake, B. G., Leadley, P. W., Arp, W. J., Nassiry, D., Curtis, P. S. (1989). An Open Top Chamber for field studies of elevated atmospheric CO2 concentration on saltmarsh vegetation. Funct. Ecol. 3, 363–371. doi: 10.2307/2389377
Dwyer, J. M., Erickson, T. E. (2016). Warmer seed environments increase germination fractions in Australian winter annual plant species. Ecosphere 7, 1–14. doi: 10.1002/ecs2.1497
Feng, J., Huang, P., Wan, X. (2019). Interactive effects of wind and light on growth and architecture of poplar saplings. Ecol. Res. 34, 94–105. doi: 10.1111/1440-1703.1013
Fernandez, P., Hilker, M. (2007). Host plant location by Chrysomelidae. Basic Appl. Ecol. 8, 97–116. doi: 10.1016/j.baae.2006.05.001
Fukasawa, K., Takahashi, D. (2017). Effects of seeds in an exotic plant Bidens pilosa L. on endemic flying insects. Bull. Nagano Univ. 43, 57–62.
Gardiner, B., Berry, P., Moulia, B. (2016). Review: wind impacts on plant growth, mechanics and damage. Plant Sci. 245, 94–118. doi: 10.1016/j.plantsci.2016.01.006
Godefroid, S. (2001). Temporal analysis of the Brussels flora as indicator for changing environmental quality. Landsc. Urban Plan. 52, 203–224. doi: 10.1016/S0169-2046(00)00117-1
Grombone-Guaratini, M. T., Solferini, V. N., Semir, J. (2004). Reproductive biology in species of Bidens L. (Asteraceae). Sci. Agric. 61, 185–189. doi: 10.1590/S0103-90162004000200010
Hayakawa, H., Tunala, M. Y., Ito, K., Gale, S., Yokoyama, J., Arakawa, R., et al. (2012). Comparative Study of leaf morphology in Aster hispidus Thunb. var. leptocladus (Makino) Okuyama (Asteraceae). Am. J. Plant Sci. 3, 110–113. doi: 10.4236/ajps.2012.31011
Hodges, L., Suratman, M. N., Brandle, J. R., Hubbard, K. G. (2004). Growth and yield of snap beans as affected by wind protection and microclimate changes due to shelterbelts and planting dates. HortSci. 39, 996–1004. doi: 10.21273/HORTSCI.39.5.996
Hollister, R. D., Elphinstone, C., Henry, G. H. R., Bjorkman, A. D., Klanderude, K., Björk, R. G., et al. (2023). A review of open top chamber (OTC) performance across the ITEX Network. Arct. Sci. 9, 331–344. doi: 10.1139/as-2022-0030
Horiuchi, M., Seyama, Y. (2006). Antiinflammatory and antiallergic activity of Bidens pilosa L. var. radiata Scherff. J. Health Sci. 52, 711–717. doi: 10.1248/jhs.52.711
Ishii, C., Shiba, M., Kumekawa, Y., Fukuda, T. (2022). Seed germination and seedling emergence of Canavalia lineata (Thunb.) DC. (Fabaceae). Int. J. Biol. 14, 8–18. doi: 10.5539/ijb.v14n1p8
Iwasaki, H., Suda, D., Watanabe, M. (2009). Foraging activity of Sympetrum infuscatum (Selys) adults living in Satoyama forest gaps (Odonata: Libellulidae). Japanese J. Appl. Entomology Zoology 53, 165–171. doi: 10.1303/jjaez.2009.165
Jones, F. A., Muller-Landau, H. C. (2008). Measuring long-distance seed dispersal in complex natural environments: An evaluation and integration of classical and genetic methods. J. Ecol. 96, 642–652. doi: 10.1111/j.1365-2745.2008.01400.x
Kiviniemi, K., Telenius, A. (1998). Experiments on adhesive dispersal by wood mouse: seed shadows and dispersal distances of 13 plant species from cultivated areas in southern Sweden. Ecography 21, 108–116. doi: 10.1111/j.1600-0587.1998.tb00664.x
Koga, S., Namai, H. (2001). Effect of wind direction on pollen flow depending on insect pollinators in carrot (Daucus carota L.) F1 seed production. Breed. Sci. 3, 81–86. doi: 10.1270/jsbbr.3.81
Koyama, H. (1995). “Flora of Japan IIIb,” in B. L. Eds. Iwatsuki, K., Yamazaki, T., Boufford, D. E., Ohba, H. (Kodansha, Tokyo), 29–31.
Kumekawa, Y., Miyata, H., Ohga, K., Hayakawa, H., Yokoyama, J., Ito, K., et al. (2013). Comparative analyses of stomatal size and density among ecotypes of Aster hispidus (Asteraceae). Am. J. Plant Sci. 4, 524–527. doi: 10.4236/ajps.2013.43067
Leishman, M. R., Westoby, M., Jurado, E. (1995). Correlates of seed size variation: a comparison among five temperate floras. J. Ecol. 83, 517–529. doi: 10.2307/2261604
LePage, P. T., Canham, C. D., Coates, K. D., Bartemucci, P. (2000). Seed abundance versus substrate limitation of seedling recruitment in northern temperate forests of British Columbia. Can. J. For. Res. 30, 415–427. doi: 10.1139/x99-223
Lewis, T., Smith, B. D. (1969). The Insect faunas of pear and apple orchards and the effect of windbreaks on their distribution. Ann. Appl. Biol. 64, 11–20. doi: 10.1111/j.1744-7348.1969.tb02850.x
Li, X. M., She, D. Y., Zhang, D. Y., Liao, W. J. (2015). Life history trait differentiation and local adaptation in invasive groups of Ambrosia artemisiifolia in China. Oecologia 177, 669–677. doi: 10.1007/s00442-014-3127-z
López-Ovejero, R. F., Carvalho, S. J., Nicolai, M., Abreu, A. G., Grombone-Guaratini, M. T., Toledo, R. E. B., et al. (2006). Resistance and differential susceptibility of Bidens pilosa and B. subalternans biotypes to ALS-inhibiting herbicides. Sci. Agric. 63, 139–145. doi: 10.1590/S0103-90162006000200005
Maggio, A., Chiarandà, F. Q., Cefariello, R., Fagnano, M. (2009). Responses to ozone pollution of alfalfa exposed to increasing salinity levels. Environ. pollut. 157, 1445–1452. doi: 10.1016/j.envpol.2008.09.013
Marui, Y., Takizawa, E., Shiba, M., Yoshizaki, S., Fukuda, T. (2023). Seed germination and seedling emergence of Lysimachia mauritiana Lam. (Primulaceae). Int. J. Biol. 15, 13–23. doi: 10.5539/ijb.v15n1p13
Matsui, R., Takei, S., Ohga, K., Hayakawa, H., Yoshida, M., Yokoyama, J., et al. (2013). Morphological and anatomical variations in rheophytic ecotype of violet, Viola mandshurica var. ikedaeana (Violaceae). Am. J. Plant Sci. 4, 859–865. doi: 10.4236/ajps.2013.44106
May, J. L., Oberbauer, S. F., Unger, S. L., Simon, M. J., Betway, K. R., Hollister, R. D. (2022). Shading decreases and delays NDVI and flowering of prostrate Arctic shrubs. Arct. Sci. 8, 967–978. doi: 10.1139/as-2020-0043
Moen, A. N. (1974). Turbulence and the visualization of wind flow. Ecology 55, 1420–1424. doi: 10.2307/1935471
Moles, A. T., Westoby, M. (2004). Seedling survival and seed size: a synthesis of the literature. J. Ecol. 92, 372–383. doi: 10.1111/j.0022-0477.2004.00884.x
Moles, A. T., Westoby, M. (2006). Seed size and plant strategy across the whole life cycle. Oikos 113, 91–105. doi: 10.1111/j.0030-1299.2006.14194.x
Muller-Landau, H. C., Wright, S. J., Calderon, O., Condit, R., Hubbell, S. P. (2008). Interspecific variation in primary seed dispersal in a tropical forest. J. Ecol. 96, 653–667. doi: 10.1111/j.1365-2745.2008.01399.x
Nakashima, K., Nishi, Y., Kawada, S., Yamaguchi, M. (2018). Assessment of ambient air pollution in Nagasaki City, Japan, based on ozone impacts on growth of radish (Raphanus sativus L.) using the Open-Top Chamber method. J. Jpn. Soc Atmos. Environ. 53, 186–193. doi: 10.11298/taiki.53.186
Ohga, K., Muroi, M., Hayakawa, H., Yokoyama, J., Ito, K., Tebayashi, S., et al. (2012a). Comparative morphology and anatomy of non-rheophytic and rheophytic types of Adenophora triphylla var. japonica (Campanulaceae). Am. J. Plant Sci. 3, 805–809. doi: 10.4236/ajps.2012.36097
Ohga, K., Muroi, M., Hayakawa, H., Yokoyama, J., Ito, K., Tebayashi, S., et al. (2012b). Morphological and anatomical analyses of the serpentine ecotype of Adenophora triphylla var. japonica (Campanulaceae). J. Plant Stud. 1, 180–187. doi: 10.5539/jps.v1n2p180
Ohga, K., Muroi, M., Hayakawa, H., Yokoyama, J., Ito, K., Tebayashi, S., et al. (2013). Coastal adaptation of Adenophora triphylla (Thunb.) A. DC. var. japonica (Regel) H. Hara (Campanulaceae). Am. J. Plant Sci. 4, 596–601. doi: 10.4236/ajps.2013.43078
Oliveira, F. Q., Andrade-Neto, V., Krettli, A. U., Brandão, M. G. (2004). New evidences of antimalarial activity of Bidens pilosa roots extract correlated with polyacetylene and flavonoids. J. Ethnopharmacol. 93, 39–42. doi: 10.1016/j.jep.2004.03.026
Pereira, R. L., Ibrahim, T., Lucchetti, L., da Silva, A. J., Gonçalves de Moraes, V. L. (1999). Immunosuppressive and anti-inflammatory effects of methanolic extract and the polyacetylene isolated from Bidens pilosa L. Immunopharmacology 43, 31–37. doi: 10.1016/s0162-3109(99)00039-9
Poorter, H., Niklas, K. J., Reich, P. B., Oleksyn, J., Poot, P., Mommer, L. (2012). Biomass allocation to leaves, stems and roots: meta-analyses of interspecific variation and environmental control. New Phytol. 193, 30–50. doi: 10.1111/j.1469-8137.2011.03952.x
Qiu, T., Andrus, R., Aravena, M. C., Ascoli, D., Bergeron, Y., Berretti, R., et al. (2022). Limits to reproduction and seed size-number trade-offs that shape forest dominance and future recovery. Nat. Commun. 13, 2381. doi: 10.1038/s41467-022-30037-9
Qiu, T., Aravena, M. C., Andrus, R., Ascoli, D., Bergeron, Y., Berretti, R., et al. (2021). Is there tree senescence? the fecundity evidence. Proc. Natl. Acad. Sci. U.S.A. 118, e2106130118. doi: 10.1073/pnas.2106130118
Rai, P. K., Singh, J. S. (2020). Invasive alien plant species: Their impact on environment, ecosystem services and human health. Ecol. Indic. 111, 106020. doi: 10.1016/j.ecolind.2019.106020
Ravi, S., Crall, J. D., Fisher, A., Combes, S. A. (2013). Rolling with the flow: bumblebees flying in unsteady wakes. J. Exp. Biol. 216, 4299–4309. doi: 10.1242/jeb.090845
R Core Team (2024). R: A Language and Environment for Statistical Computing (Vienna, Austria: R Foundation for Statistical Computing). Available at: https://www.r-project.org/.
Reddy, K. N., Singh, M. (1992). Germination and emergence of hairy beggarticks (Bidens pilosa). Weed Sci. 40, 195–199. doi: 10.1017/S0043174500057210
Rusman, Q., Lucas-Barbosa, D., Hassan, K., Poelman, E. H. (2020). Plant ontogeny determines strength and associated plant fitness consequences of plant-mediated interactions between herbivores and flower visitors. J. Ecol. 108, 1046–1060. doi: 10.1111/1365-2745.13370
Sargent, R. D., Goodwillie, C., Kalisz, S., Ree, R. H. (2007). Phylogenetic evidence for a flower size and number trade-off. Am. J. Bot. 94, 2059–2062. doi: 10.3732/ajb.94.12.2059
Shiba, M., Arihara, S., Harada, S., Fukuda, T. (2024a). Impact on the scape of Farfugium japonicum var. japonicum (Asteraceae) under strong wind conditions based on morphological and mechanical analyses. Front. Plant Sci. 15. doi: 10.3389/fpls.2024.1407127
Shiba, M., Fukuda, T. (2024). Rheophytic Osmunda lancea (Osmundaceae) exhibits large flexibility in the petiole. Sci. Rep. 14, 2866. doi: 10.1038/s41598-024-53406-4
Shiba, M., Mizuno, T., Fukuda, T. (2023). Effect of strong wind on laminas and petioles of Farfugium japonicum (L.) Kitam. var. japonicum (Asteraceae). Front. Plant Sci. 14. doi: 10.3389/fpls.2023.1182266
Shiba, M., Sato, R., Fukuda, T. (2024b). A comparison of mechanical characteristics among Setaria viridis var. minor, Setaria italica, and Setaria × Pycnocoma species of the family Poaceae. Plant Species Biol. 39, 51–58. doi: 10.1111/1442-1984.12435
Shiba, M., Tate, T., Fukuda, T. (2021). Rheophytic adaptation of Eurya japonica Thunb. (Ternstroemiaceae). Int. J. Biol. 13, 65–73. doi: 10.5539/ijb.v13n2p65
Shiba, M., Tate, T., Fukuda, T. (2022a). Adaptative leaf morphology of Eurya japonica Thunb. (Ternstroemiaceae) in serpentine areas. J. Plant Stud. 11, 10–18. doi: 10.5539/jps.v11n1p10
Shiba, M., Tate, T., Fukuda, T. (2022b). Serpentine adaptation of Ligustrum japonicum Thunb. (Oleaceae) based on morphological and anatomical approaches. Int. J. Biol. 14, 10–18. doi: 10.5539/ijb.v14n2p10
Shiba, M., Tate, T., Fukuda, T. (2022c). Leaf adaptation of Eurya japonica Thunb. (Pentaphylacaceae) in coastal area. J. Plant Stud. 11, 31–41. doi: 10.5539/jps.v11n1p31
Shochat, E., Lerman, S. B., Anderies, J. M., Warren, P. S., Faeth, S. H., Nilon, C. H. (2010). Invasion, competition, and biodiversity loss in urban ecosystems. J. Bio Sci. 60, 199–208. doi: 10.1525/bio.2010.60.3.6
Silveira, F. A. O., Negreiros, D., Araújo, L. M., Fernandes, G. W. (2012). Does seed germination contribute to ecological breadth and geographic range? A test with sympatric Diplusodon (Lythraceae) species from Rupestrian fields. Plant Species Biol. 27, 170–173. doi: 10.1111/j.1442-1984.2011.00342.x
Silvertown, J., Bullock, J. M. (2003). Do seedlings in gaps interact? A field test of assumptions in ESS seed size models. Oikos 101, 499–504. doi: 10.1034/j.1600-0706.2003.12247.x
Stephenson, A. G. (1981). Flower and fruit abortion: proximate causes and ultimate functions. Annu. Rev. Ecol. Syst. 12, 253–279. doi: 10.1146/annurev.es.12.110181.001345
Štofko, P., Kodrík, M. (2008). Comparison of the root system architecture between windthrown and undamaged spruces growing in poorly drained sites. J. For. Sci. 54, 150–160. doi: 10.17221/3101-JFS
Strauss, S. Y. (1997). Floral characters link herbivores, pollinators, and plant fitness. Ecology 78, 1640–1645. doi: 10.1890/0012-9658(1997)078[1640:FCLHPA]2.0.CO;2
Sun, S., Zhang, D. Y., Ives, A. R., Li, Q. J. (2011). Why do stigmas move in a flexistylous plant? J. Evol. Biol. 24, 497–504. doi: 10.1111/j.1420-9101.2010.02181.x
Sunami, T., Ohga, K., Muroi, M., Hayakawa, H., Yokoyama, J., Ito, K., et al. (2013). Comparative analyses of hairless-leaf and hairy-leaf type individuals in Aster hispidus var. insularis (Asteraceae). J. Plant Stud. 2, 1–6. doi: 10.5539/jps.v2n1p1
Sundararajan, P., Dey, A., Smith, A., Doss, A. G., Rajappan, M., Natarajan, S. (2006). Studies of anticancer and antipyretic activity of Bidens pilosa whole plant. Afr. Health Sci. 6, 27–30. doi: 10.5555/afhs.2006.6.1.27
Sutherland, S. (1986a). Floral sex ratios, fruit-set, and resource allocation in plants. Ecology 67, 991–1001. doi: 10.2307/1939822
Sutherland, S. (1986b). Patterns of fruit-set: what controls fruit-flower ratios in plants? Evolution 40, 117–128. doi: 10.1111/j.1558-5646.1986.tb05723.x
Sutherland, S., Delph, L. F. (1984). On the importance of male fitness in plants: patterns of fruit-set. Ecology 65, 1093–1104. doi: 10.2307/1938317
Takizawa, E., Shiba, M., Yoshizaki, S., Fukuda, T. (2023). Stomatal study of introduced species, Ligustrum lucidum Aiton (Oleaceae), in coastal areas of Japan. J. Plant Stud. 12, 24–36. doi: 10.5539/jps.v12n1p24
Takizawa, E., Tate, T., Shiba, M., Ishii, C., Yoshizaki, S., Fukuda, T. (2022). Coastal adaptation of Ligustrum japonicum Thunb. (Oleaceae) - a case study of stomatal adaptation pattern into coastal forests -. J. Jpn. Soc Coast. Forest. 21, 1–8. doi: 10.60398/kaiganrin.21.1_1
Tamasi, E., Stokes, A., Lasserre, B., Danjon, F., Berthier, S., Fourcaud, T., et al. (2005). Influence of wind loading on root system development and architecture in oak (Quercus robur L.) seedlings. Trees 19, 374–384. doi: 10.1007/s00468-004-0396-x
Thomson, C. E., Hadfield, J. D. (2017). Measuring selection when parents and offspring interact. Methods Ecol. Evol. 8, 678–687. doi: 10.1111/2041-210X.12795
Tobinaga, S., Sharma, M. K., Aalbersberg, W. G., Watanabe, K., Iguchi, K., Narui, K., et al. (2009). Isolation and identification of a potent antimalarial and antibacterial polyacetylene from Bidens pilosa. Planta Med. 75, 624–628. doi: 10.1055/s-0029-1185377
Turbill, C. (2008). Winter activity of Australian tree-roosting bats: influence of temperature and climatic patterns. J. Zool. 276, 285–290. doi: 10.1111/j.1469-7998.2008.00487.x
Ubillas, R. P., Mendez, C. D., Jolad, S. D., Luo, J., King, S. R., Carlson, T. J., et al. (2000). Antihyperglycemic acetylenic glucosides from Bidens pilosa. Planta Med. 66, 82–83. doi: 10.1055/s-0029-1243117
Ueda, R., Minamiya, Y., Hirata, A., Hayakawa, H., Muramatsu, Y., Saito, M., et al. (2012). Morphological and anatomical analyses of rheophytic Rhododendron ripense Makino (Ericaceae). Plant Species Biol. 27, 233–240. doi: 10.1111/j.1442-1984.2011.00345.x
Uraguchi, S., Watanabe, I., Kuno, K., Hoshino, Y., Fujii, Y. (2003). Allelopathy of floodplain vegetation species in the middlecourse of Tama River. J. Weed Sci. Technol. 48, 117–129. doi: 10.3719/weed.48.117
van Mölken, T., Jorritsma-Wienk, L. D., van Hoek, P. H., de Kroon, H. (2005). Only seed size matters for germination in different groups of the dimorphic Tragopogon pratensis subsp. pratensis (Asteraceae). Am. J. Bot. 92, 432–437. doi: 10.3732/ajb.92.3.432
Walker, M. D., Wahren, C. H., Hollister, R. D., Henry, G. H., Ahlquist, L. E., Alatalo, J. M., et al. (2006). Plant community responses to experimental warming across the tundra biome. Proc. Natl. Acad. Sci. U. S. A. 103, 1342–1346. doi: 10.1073/pnas.0503198103
Wania, A., Kühn, I., Klotz, S. (2006). Plant richness patterns in agricultural and urban landscapes in central Germany—spatial gradients of species richness. Landsc. Urban Plan. 75, 97–110. doi: 10.1016/j.landurbplan.2004.12.006
Wilcock, C., Neiland, R. (2002). Pollination failure in plants: why it happens and when it matters. Trends Plant Sci. 7, 270–277. doi: 10.1016/s1360-1385(02)02258-6
Worley, A. C., Barrett, S. C. H. (2000). Evolution of floral display in Eichhornia paniculata (Pontederiaceae): direct and correlated responses to selection on flower size and number. Evolution 54, 1533–1545. doi: 10.1111/j.0014-3820.2000.tb00699.x
Yamada, Y., Hayakawa, H., Minamiya, Y., Ito, K., Shibayama, Z., Arakawa, R., et al. (2011). Comparative morphology and anatomy of rheophytic Aster microcephalus (Miq.) Franch. et Sav. Var. Ripensis Makino (Asteraceae). J. Phytogeogr. Taxon. 59, 35–42. doi: 10.24517/00053453
Yokoyama, N., Hayakawa, H., Matsuyama, K., Muroi, M., Ohga, K., Ito, K., et al. (2012). Morphological and molecular analyses of rheophytic Rhododendron ripense and its allied dryland species R. macrosepalum (Ericaceae). Environ. Control Biol. 50, 305–312. doi: 10.2525/ecb.50.305
Keywords: alien species, Bidens pilosa var. pilosa, morphology, seed production, wind stress
Citation: Shiba M, Kobayashi N, Harada S and Fukuda T (2024) Decrease in wind stress leads to an increase in the above ground morphology and number of seeds of an invasive alien species, Bidens pilosa (Asteraceae). Front. Plant Sci. 15:1445437. doi: 10.3389/fpls.2024.1445437
Received: 07 June 2024; Accepted: 17 October 2024;
Published: 08 November 2024.
Edited by:
Yu-Long Feng, Shenyang Agricultural University, ChinaReviewed by:
Denny Kurniadie, Padjadjaran University, IndonesiaTong Qiu, The Pennsylvania State University (PSU), United States
Copyright © 2024 Shiba, Kobayashi, Harada and Fukuda. This is an open-access article distributed under the terms of the Creative Commons Attribution License (CC BY). The use, distribution or reproduction in other forums is permitted, provided the original author(s) and the copyright owner(s) are credited and that the original publication in this journal is cited, in accordance with accepted academic practice. No use, distribution or reproduction is permitted which does not comply with these terms.
*Correspondence: Masayuki Shiba, msykshiba48@gmail.com