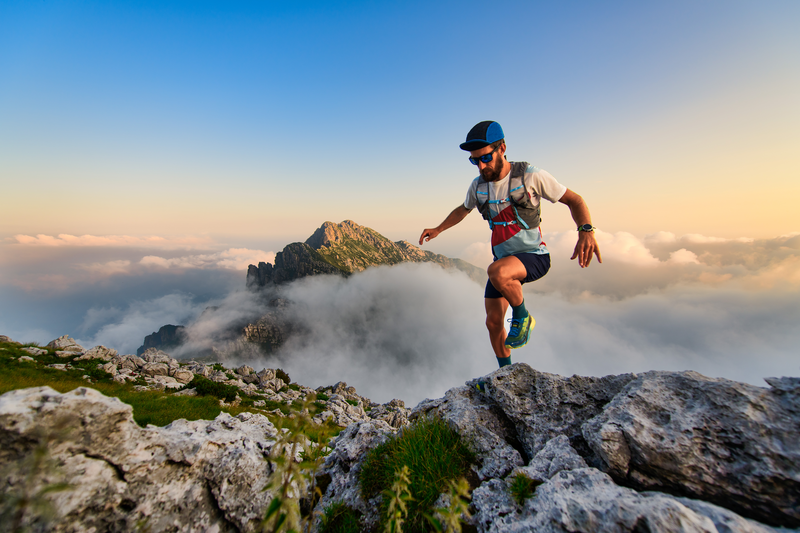
95% of researchers rate our articles as excellent or good
Learn more about the work of our research integrity team to safeguard the quality of each article we publish.
Find out more
SYSTEMATIC REVIEW article
Front. Plant Sci. , 04 July 2024
Sec. Crop and Product Physiology
Volume 15 - 2024 | https://doi.org/10.3389/fpls.2024.1428975
This article is part of the Research Topic Quality and Resistance Physiology and Regulation of Root and Tuber Crops View all 7 articles
Sweetpotato is an economically important crop, and it has various advantages over other crops in addressing global food security and climate change. Although substantial articles have been published on the research of various aspects of sweetpotato biology, there are no specific reports to systematically crystallize the research achievements. The current review takes the lead in conducting a keyword-centric spatiotemporal dimensional bibliometric analysis of articles on sweetpotato research using CiteSpace software to comprehensively clarify the development status, research hotspot, and development trend in the past 30 years (1993–2022). Quantitative analysis was carried out on the publishing countries, institutions, disciplines, and scholars to understand the basic status of sweetpotato research; then, visual analysis was conducted on high-frequency keywords, burst keywords, and keyword clustering; the evolution of major research hotspots and the development trend in different periods were summarized. Finally, the three main development stages—preliminary stage (1993–2005), rapid stage (2006–2013), and diversified mature stage (2014–2022)—were reviewed and analyzed in detail. Particularly, the development needs of sweetpotato production in improving breeding efficiency, enhancing stress tolerance, coordinating high yield with high quality and high resistance, and promoting demand were discussed, which will help to comprehensively understand the development dynamics of sweetpotato research from different aspects of biological exploration.
Declining agricultural land, increasing global population, and uncertain adverse environmental changes pose complex and daunting challenges to global food production. Although much progress has been made in crop yield improvement, most of the existing efforts have focused on aboveground traits (mainly cereal crops), which limits the further improvement of the yield potential, but exploring the root systems is expected to overcome the bottleneck. Consequently, it is imperative to stabilize and enhance crop yield by exploring the underground traits of root and tuber crops, which will enable a truly inclusive green revolution (Khan et al., 2016).
Root and tuber crops contain large amounts of carbohydrates and different levels of proteins and vitamins, making them an indispensable part of the human diet. Particularly, potato (tuber crops), sweetpotato, and cassava (tuberous root crops) are among the most important food crops globally, as well as core cereals and legumes. The storage organs of potato and sweetpotato are similar, but there are significant differences in botany: the former is formed from tubers, while the latter is developed from fibrous roots into expanded tuberous roots (also known as storage roots). The genus Ipomoea has the most species in the Convolvulaceae family, with approximately 800 species (Wood et al., 2020). These species are widely used in agriculture, industry, medicine, and ornamental plants, as well as evolutionary and molecular genetic studies (Meira et al., 2012; Yan et al., 2022). For instance, Ipomoea aquatica not only is one of the most abundant sources of carotenoids and chlorophylls but also contains a sufficient supply of the most essential amino acids and excellent bioelements, which can be comparable to conventional foodstuffs, and Ipomoea nil has a wide range of applications in garden purposes and genetic studies due to diverse flower colors and pigmentation patterns (Meira et al., 2012). Particularly, sweetpotato [Ipomoea batatas (L.) Lam.] is the only crop plant with starch storage roots among approximately 1,650 major tropical species in Convolvulaceae (Meira et al., 2012; Arisha et al., 2020).
Sweetpotato, a dicotyledonous hexaploid plant species (2n = 6x = 90, B1B1B2B2B2B2), has long been the seventh largest food crop worldwide among all food crops based on production, mainly produced by Asia and Africa, and China has consistently been the biggest sweetpotato producer (Liu, 2017; Yang et al., 2017). It offers many unique advantages such as strong stress resistance, tolerance to barrenness, high and stable yield, rich nutritional components, and diversified applications (Liu, 2017; Alam, 2021). Sweetpotato is cultivated in more than 120 countries and regions from the temperate zone south of 40°N to the tropics (Luo et al., 2023). It is primarily planted in arid, salinized, hilly regions with poor marginal conditions (Liu, 2017; Bach et al., 2021), which is of great significance for stabilizing and improving agricultural production. In terms of nutrition, sweetpotato is an excellent source of starches, proteins, vitamins, dietary fibers, flavonoids, carotenoids, minerals, and many other antioxidant compounds (Johnson and Pace, 2010; Alam, 2021). Additionally, sweetpotato leaves (greens, tops, or tips), as a fresh vegetable with rich nutrition and strong health promotion, can substantially improve the availability of foods (Islam, 2006). In 2006, the World Health Organization (WHO) voted sweetpotato as the champion of “the 10 best vegetables” (Ren et al., 2012), and it has also long been selected by the US National Aeronautics and Space Administration (NASA) as one of the primary food sources in controlled life-support systems (Hoff et al., 1982). Moreover, sweetpotato is used in traditional systems of medicine for preventing and treating various diseases, such as diabetes, hypertension, tumors, cancer, cardiovascular disease, inflammation, and aging (Behera et al., 2022). For the diversified applications, in addition to being a key functional food, sweetpotato is also mainly used as fodder, industrial raw materials, and energy source. Therefore, sweetpotato is indispensable for human nutrition and can help address the problems of food crisis, energy supply, and climate change.
Due to the biological importance of sweetpotato, its multi-dimensional research has attracted more and more attention from scholars, and the number of related articles has increased rapidly and greatly. Therefore, a summary analysis of the sweetpotato-related articles is necessary. Although many reviews on the research progress of sweetpotato have been published, they are mainly based on limited documents and summarized from different perspectives and directions in isolation. For example, the representative review topics include tuberous root development and starch (Lyu et al., 2021; Yang et al., 2023b), biotic and abiotic stress response (Yang et al., 2023a; Ahmed et al., 2024), anthocyanins, carotenoids, and the health-promoting functions (Li et al., 2019a; Alam, 2021; Tang et al., 2023), and genetics and genomics (Yan et al., 2022). However, these reviews cannot comprehensively and objectively reflect the whole picture of sweetpotato research; nor can they systematically exhibit the development process.
Bibliometrics can quickly draw and visualize the structures and dynamics of a research field by integrating numerous published articles, which can effectively reflect the current situation and future development of the subject. The comprehensive visual analysis software CiteSpace was found to be more suitable for statistical analysis than other commonly used software including HistCite, RefViz, SATI, and VOSviewer (Liu et al., 2022b). Herein, CiteSpace was employed to generate co-occurrence, co-citation, and timeline graphs to comprehensively visualize the structures and dynamics of sweetpotato research over the past 30 years (1993–2022), as only a little progress was made before 1993. The current review systemically summarizes the research progress and hotspots of sweetpotato, and identifies the key points and change trends through the econometric analysis, which will facilitate a comprehensive understanding of the development dynamics of sweetpotato research.
Web of Science (WoS) Core Collection database, as a globally recognized authoritative citation database, was searched for relevant articles published from January 1, 1993, to December 31, 2022. The search applied Boolean operator, Topic Search (including Title, Abstract, Author keywords, and Keywords Plus) = “sweet potato” OR sweetpotato OR “sweet potatoes” OR sweetpotatoes OR “Ipomoea batatas”. In total, 8,323 records, including 7,866 articles, 457 reviews, 63 highly cited articles, and one hot article, were exported with “Full Record and Cited References”. Then, the comprehensive retrospective analysis and summary were conducted using the CiteSpace software (Chen, 2006). Data processing adopted the theoretical framework of cluster analysis described in a previous report (Liu et al., 2022b). Briefly, the time partition was 1 year, the source included all items, Top = 50 for the threshold was selected, and others had default values. The keywords, authors, and references of the paper data units were selected as the node type. Critical path methods were applied to analyze the data collection element and draw the knowledge map, co-occurrence maps were employed to analyze the research hotspot, and time zone views were used to achieve development relationships.
The annual number of publications can intuitively reveal the rate of development and change in specific fields. The statistics of sweetpotato-related articles reveal that the annual volume was relatively small (all less than 200 articles) and growing slowly from 1993 to 2006; thus, sweetpotato research has experienced a long period of flat growth. Subsequently, the annual number of publications accelerated steadily from 224 articles in 2007 to 334 articles in 2016. Comparatively, the publication numbers increased sharply from 2017 to 2022, and over 600 articles were published after 2020. As of November 1, 2023, 483 articles have been published (Figure 1A). The increasing numbers firmly exhibit that sweetpotato research is receiving increasing attention.
Figure 1 Publication trend and cooperation network analysis of sweetpotato-related articles. (A) The annual number of papers on sweetpotato research in the past 30 years. (B) Top 20 countries in the publication volume of sweetpotato research. (C–E) Network diagram of national cooperation (C), institutional cooperation (D), and author’s cooperation (E) in the sweetpotato research. The larger the node, the higher the amount of articles. The more connections between the two nodes, the stronger their cooperative connections. The rings of different colors represent different years as shown in the color chrominance card, and the ring thickness is proportional to the number of articles for a given time. The outermost purple ring of the node represents the centrality value calculated by the computed node centrality function of CiteSpace tool.
Then, through a comparative analysis of the publications, the key countries and institutions with large publications on sweetpotato research were identified. From 1993 to 2022, a total of 147 countries/regions and 724 institutions published articles about sweetpotato. The top 10 were China, the USA, Japan, Brazil, South Korea, India, the UK, Australia, Nigeria, and Germany, which account for approximately 65.4% of the total number of articles, and Asia accounts for 56.2% of the top 10, illustrating the important contribution of Asian countries/regions to the sweetpotato research (Figure 1B). China was the leading country (2,258 articles), accounting for 20.1% and 56.5% of the world and Asia, respectively. Although the USA ranks only eighth in sweetpotato production (Bach et al., 2021), the USA (1695 articles) and China occupy the dominant position in the sweetpotato research.
Subsequently, the cooperative relationships between countries were analyzed using the CiteSpace software. The centrality degree is of great significance for the cooperative network analysis, and high centrality reflects that the node has a key influence on the relationship in the whole network. The results show that the USA had the highest centrality degree (0.32). Interestingly, although the co-occurrence frequencies of France and Australia were only 161 and 285 papers, respectively, their centrality degrees reached 0.18 and 0.17, respectively, even exceeding those of China (0.13). Furthermore, all countries with a high degree of centrality had a close cooperative relationship, and similar situations were observed in the subsequent analysis of institutional partnerships. Moreover, the early accumulation of sweetpotato research in the USA and Japan was more than that in China (before 2014) but was then quickly surpassed by China (Figures 1C, D).
The top 20 institutions in sweetpotato research are shown in Supplementary Table S1, of which China and the USA had the largest number of institutions, with eight and six, respectively. Among them, the United States Department of Agriculture (USDA) ranked first (395), followed by the Consultative Group on International Agricultural Research (CGIAR; 391), Ministry of Agriculture and Rural Affairs (MARA; 298) of China, Chinese Academy of Agricultural Sciences (CAAS; 262), and International Center of Potato (CIP; 244). The USDA had the highest centrality degree (0.26), followed by CAAS (0.13), CGIAR (0.12), National Agriculture & Food Research Organization (NARO; 0.12) of Japan, and Chinese Academy of Sciences (0.11). Moreover, institutions such as MARA, CIP, North Carolina State University, and China Agricultural University are commensurate in their top ranking of publications, and all of their cooperative connections with other institutions are also close (Supplementary Table S1, Figures 1C, D).
Through the analysis of the author’s published articles and cooperation networks, over 1,300 authors involved in sweetpotato research were found. The top 10 prolific authors were all Chinese and South Korean scholars, with six and four, respectively. Among the top 20 scholars, except for two American scholars, all of them were from Asia (12 from China, five from South Korea, and one from Japan), strengthening that Asian countries/regions pay more attention and research on sweetpotato (Supplementary Table S2). The top 10 scholars have published 654 articles, and Kwak Sang-Soo from the Korea Research Institute of Bioscience and Biotechnology (KRIBB) has published 121 articles, ranking first among all scholars. Then, their cooperative relationships were explored, and both centralized and decentralized distribution patterns were found (Figure 1E). Due to the large number of authors and their significant dispersion, the author’s cooperation network is like a starry sky. The main cooperation links between Chinese scholars (such as Mu Tai-hua, Liu Qingchang, He Shaozhen, Ma Daifu, and Li Zongyun), South Korean scholars (such as Kwak Sang-Soo, Lee Haeng-Soon, Kim Yun-Hee, and Kim Ho Soo), and Chinese and South Korean scholars were detected, and many minority groups of scholars also have a close cooperative connection.
The co-occurrence analysis of the subject network can establish the evolution of mainstream and interdisciplinary subjects of the research field. The results clearly show that sweetpotato research is a multidisciplinary field, in which Food Science & Technology and Plant Sciences are the main disciplines, which is closely related to the fact that sweetpotato is not only one of the main food crops worldwide but also used as an excellent model crop in basic research, followed by Entomology, Agronomy, Applied Chemistry, Biochemistry & Molecular Biology, and Agriculture, highlighting the multidisciplinary nature of sweetpotato research, which is inseparable from sweetpotato as an all-around key crop. Despite this, many of them do not display centralization characteristics, such as Food Science & Technology, Entomology, and Agronomy. On the contrary, Environmental Sciences (0.21), Biotechnology & Applied Microbiology (0.19), Analytical Chemistry (0.18), and Biochemistry & Molecular Biology (0.16) have the highest degree of centrality (Figure 2A, Supplementary Table S3).
Figure 2 Analysis of disciplinary network, keyword co-occurrence, and timeline distribution of keywords. (A) Disciplinary network in the field of sweetpotato research from 1993 to 2022. (B) Keyword co-occurrence network in the field of sweetpotato research (1993–2022). The nodes represent the corresponding disciplines/keywords, and the node size is proportional to the number. The connection lines between nodes represent their relationships. The rings of different colors represent different years as shown in the color chrominance card, and the ring thickness is proportional to the number for a given time. The outermost purple ring of the node represents the centrality value calculated by the compute node centrality function of CiteSpace tool. (C) Co-occurrence clustering keyword network in the field of sweetpotato research. The serial number of clusters is inversely related to the number of their members; the smaller the number, the more members the cluster has. The keyword label with the largest value under the log-likelihood ratio (LLR) algorithm is used as the name of the cluster. (D) Timeline distribution of keywords for different topics. The vertical axis covers the same keywords of the same year, which shows the concerns of related fields in the same year. The horizontal axis concentrates the keywords of the same cluster, which displays the development result of the same cluster. The nodes and links in the graph display the inheritance and continuation of keywords, as well as the period between them.
Subsequently, the evolution of the top 20 discipline types emerging during 1993–2022 is listed in Table 1, which further revealed that sweetpotato research is a multidisciplinary cross. On average, there is a burst of discipline every 2 years, while the duration of different disciplines is different. Among them, Aerospace Engineering has the longest duration (15 years), followed by Cell Biology (11 years) and Biophysics (10 years), which are mainly concentrated in the early stages of sweetpotato research. Particularly, in the top 20 subject categories with the strongest citation bursts, there are multiple environment-related emerging disciplines, such as Environmental Sciences, Environmental Studies, and Environmental Engineering, suggesting that sweetpotato research is becoming more and more concerned in multiple disciplines.
Combined with the evolution of disciplines, the distribution of keywords was further analyzed. The co-occurrence network analysis of keywords can effectively reflect the development history and research hotspots. The intricate connection between approximately 1,000 keywords was found (Figure 2B), indicating the wide scopes and mature frameworks of sweetpotato research. In addition to the topic words sweetpotato and I. batatas (both have different spellings) having the largest nodes and complex network connections as expected, the top 10 keywords include Physicochemical property, Identification, Expression, Bemisia tabaci, Quality, Growth, Antioxidant activity, Plants, Resistance, and Cultivars, suggesting that they are the keywords with the most co-occurrences (Supplementary Table S4). Moreover, keyword clustering can reflect the main research clusters that have been formed in a certain field. The first six clusters represent the core of the sweetpotato research, including Sweetpotato, Antioxidant activity, B. tabaci, Antioxidant activity, Genetic diversity, Sweetpotato starch, Food security, and Health risk (Figure 2C), and the detailed keywords in each cluster are shown in Supplementary Table S5, illustrating their much broader relationships among the topics.
The refined keyword timeline diagram can reflect the evolution of research topics at different time stages. According to the node distribution and connecting lines, the diagram can be divided into three stages of development, which overlap with the stage division in publication trend analysis to a certain extent (Figures 1A, 2D). The first stage (before 2005) has a small number of nodes with a small area that contains few themes and connecting lines, reflecting that the early research mainly focuses on several topics such as sweetpotato leaf, anthocyanin, salt stress, and B. tabaci and is in the preliminary stage. In the second stage of rapid development (2006–2013), both the number and area of nodes proliferated, the interconnection lines between nodes were dense, and multiple new keywords began to appear; the topics covered were still concentrated on a few keywords, and some continue to be inherited and some begin to weaken, such as purple sweetpotato, drought tolerance, whitefly, and sweetpotato leaf. In the third stage (2014–2022), sweetpotato research has shifted to both diversification and centralization. The number and area of nodes were further improved, the connections between nodes were denser, and most of the topics were covered. Similarly, some topics continue to be inherited, and some begin to weaken, such as the emerging concern of Ipomoea trifida and sweetpotato starch (Figure 2D). Therefore, the sweetpotato research in the present stage was wider in dimensions, deeper in scales, and more diverse in themes, which was in line with the results of co-occurrence analysis of disciplines and keywords, underscoring the increasing popularity of sweetpotato research.
Subsequently, CiteSpace was used to further detect citation burst keywords with high frequency and centrality as indicators of research frontiers. Approximately 330 such keywords were found, and the top 10 keywords for duration include Homoptera, Purification, Bemisia argentifolii, Hemiptera, Aleyrodidae, Sweetpotato whitefly, Cotton, Impact, I. batatas, and Cells (Table 2), suggesting their research hotspots. Among them, Aleyrodidae has the longest duration (23 years), followed by Homoptera, Amino acids, Populations, Coleoptera, Hymenoptera, and Lepidoptera (17–20 years), which are mainly concentrated in the early stages of sweetpotato research. The emerging keywords with the strongest citation bursts include Resistant starch, Impact, Rheological property, Abiotic stress, and digestibility. Based on the above analysis of research contents, the keyword timeline diagram was found to be more suitable for analyzing the hotspot evolution of sweetpotato research. Subsequently, the research topics of the published papers were manually screened, classified, and counted mainly by reading their abstract contents, and the representative papers closely related to sweetpotato biology research and cited in the forefront were focused on.
During the preliminary accumulation stage, a total of 1,948 articles were published, including 51 review articles. During this period, the burst keywords related to whitefly were the highest, and the burst time was also the longest. Other keywords related to cells, DNA, sequence, virus, amylopectin, and caffeic acid were also found, indicating that related research had received significant attention in the early stage. For instance, biochemical, molecular, and whole-system approaches to study the potential diversity between reproductively isolated populations or biotypes of B. tabaci were systematically reviewed (Brown et al., 1995), and the migration, dispersal, and identification of sweetpotato whitefly B. tabaci have also been extensively explored (Byrne, 1999). Since sweetpotato whitefly is an effective vector for many viruses, sweetpotato virus disease (SPVD) and shoot tip culture of sweetpotato for virus elimination have also attracted corresponding attention (Gao et al., 2000; Tairo et al., 2005). Simultaneously, the beneficial effects of the antioxidant properties of sweetpotato chemical components on human health and nutrition have attracted much research (Cao et al., 1996; Philpott et al., 2004), and the efficacy of sweetpotato β-carotene has also made a small amount of progress (Van Jaarsveld et al., 2005). Sweetpotato starch has also received broad attention, but it mainly focuses on various physiological and biochemical characteristics (Zhang and Oates, 1999; Moorthy, 2002). Additionally, it is worth mentioning that the preface of sweetpotato genomics and molecular biology research has been opened at this stage, including the genetic diversity and relationship analysis of sweetpotato and its wild relatives, as well as their microsatellite sequence characterizations (Buteler et al., 1999; Huang and Sun, 2000), and several genes such as sweetpotato anionic POD gene swpa1 and trypsin inhibitor gene spTi-1 have been found to be associated with salt tolerance/oxidative stress tolerance and Spodoptera litura resistance, respectively (Yeh et al., 1997; Yun et al., 2000).
A total of 2,069 articles were published in the rapid stage, including 86 review articles and five highly cited articles were found. Compared with the preliminary accumulation stage, the continuous burst keywords related to whitefly, virus, β-carotene, flavonoids, and molecular cloning at this stage were found. Moreover, significant burst keywords such as insecticide resistance, ascorbate peroxidase, children, hydrogen peroxide, foods, rats/mice, mechanisms, and salt stress indicate the extensive depth of sweetpotato research. For example, host plants and natural enemies of the sweetpotato whitefly B. tabaci in China were investigated (Li et al., 2011), and the biotype-dependent secondary symbiont community in the sympatric population of sweetpotato whitefly B. tabaci and the global relationships of B. tabaci were studied (Boykin et al., 2007; Chiel et al., 2007). Moreover, the situation of sweetpotato whitefly in the UK and the prospects for developing eradication strategies were discussed (Cuthbertson et al., 2011). Correspondingly, research on sweetpotato viruses including their molecular detection was significantly improved (Kokkinos and Clark, 2006; Cuellar et al., 2009). For instance, the compositions of sweetpotato virus complexes, the effects of viruses on production, the biology of virus–plant interactions, and management approaches to viruses were summarized (Clark et al., 2012). Moreover, the comprehensive information on types of virus, yield loss mechanisms, increased yield, and benefit by cultivation of virus-free plants through meristem culture techniques and propagation system of virus-free seed tubers in China were systematically reviewed (Wang et al., 2010), and cryotherapy of shoot tips and the application in pathogen/virus eradication have also significantly served the preparation of healthy genetic resources (Wang and Valkonen, 2009; Feng et al., 2011).
Additionally, research on the benefits of sweetpotato to human health and nutrition, especially in antioxidant relevance, disease prevention, and treatment, has been further strengthened (Kurata et al., 2007; Teow et al., 2007; Padda and Picha, 2008). For instance, the beneficial effects on the health and nutrition of sweetpotato leaves were systematically reviewed and studied (Islam, 2006; Johnson and Pace, 2010). Ulteriorly, the characterization of anthocyanins and anthocyanidins in purple-fleshed sweetpotato was identified (Truong et al., 2010). In addition, more and more attention has been paid to research on carotenoids, such as their identification, content, and stability detection (Van Jaarsveld et al., 2006; Kimura et al., 2007). Moreover, changing the expression of carotenoid-related genes by genetic engineering not only can promote carotenoid accumulation but also can enhance the salt tolerance of transgenic sweetpotato (Kim et al., 2012, 2013). Therefore, this significantly promoted the application of orange-fleshed sweetpotato as a biofortified crop to prevent vitamin A deficiency.
Sweetpotato transgenic research has made great progress in the rapid stage, especially in the study of stress resistance. For instance, the overexpression of CuZnSOD, IbLEA14, LOS5, or SPCP2 in sweetpotato/tobacco/Arabidopsis enhanced tolerance to various abiotic stresses, including salt, drought, and oxidative stress (Lee et al., 2007; Chen et al., 2010; Gao et al., 2011; Park et al., 2011). Moreover, molecular biology studies related to starch and tuberous root development have also made breakthroughs, and the functions of multiple related genes have been analyzed, including IbEXP1, IbMADS1, SRD1, GBSSI, and SBEII (Kitahara et al., 2007; Ku et al., 2008; Noh et al., 2010, 2013). With the advancement and popularization of omics technology, the era of multi-omics analysis of sweetpotato is also coming. During this period, various tissues/conditions underwent omics analysis including root transcriptomic/proteomic study (Lee et al., 2012; Firon et al., 2013), flower transcriptome (Tao et al., 2013), various tissues, and developmental stages (Tao et al., 2012), and transcriptomic and proteomic responses to whitefly (Yang et al., 2013).
In the diversified maturity stage, a total of 4,306 articles (51.7% of the total) were published, including 320 review articles, 55 highly cited articles, and one hot article. There are many kinds of burst keywords such as Abiotic stress, Digestibility, Purple-fleshed sweetpotato, Resistant starch, Antioxidant, Health, Microstructure, Drought, Technology, Transcription factors, Carotenoids, Tuberous roots, Iron, Salt, Pigments, Amylose content, Biofortification, Overexpression, Reactive oxygen species, Model, and Molecular characterization, denoting that the biological research of sweetpotato is carried out comprehensively and in-depth at the current stage. Through reading and summarizing the research contents of published articles, it was found that studies on various aspects of sweetpotato have been involved in more and more in-depth mechanism explorations, which is inseparable from the divine assistance of its genomic information being decrypted. Due to the complexity of the genome of hexaploid sweetpotato, although the next-generation genome sequencing technology has been developed for more than a decade, its genome information has not made breakthrough progress until this period. Presently, the genome sequences of hexaploid cultivated species ‘Taizhong 6’ (a half haplotype-resolved hexaploid genome) (Yang et al., 2017) (https://sweetpotao.com/) and ‘Xushu 18’ (Yoon et al., 2022) (https://plantgarden.jp/ja/list/t4120/genome/t4120.G001), and two probable diploid wild relatives, I. trifida and Ipomoea triloba (http://sweetpotato.uga.edu/), are available (Hirakawa et al., 2015; Wu et al., 2018). Additionally, the complete chloroplast genomes of hexaploid and wild sweetpotato have also been sequenced (Yan et al., 2015; Wang et al., 2019b). This provides the most fundamental basis for the application of CRISPR/Cas technology, gene function analysis, molecular evolution resolution, and transcriptomic detection. Benefiting from this, research on the origin and diversity of sweetpotato from the molecular level is increasing in-depth, such as the genetic diversity, origin and evolution, allopolyploid discussion, and genome composition analysis (Muñoz-Rodríguez et al., 2018; Wadl et al., 2018). Of course, the current explorations based on specific locus amplified fragment sequencing (SLAF-seq), restriction-site associated DNA sequencing (RAD-seq), and simple sequence repeat (SSR) markers are also widely focused (Yang et al., 2015; Su et al., 2017; Feng et al., 2020). It is worth mentioning that the review article ‘Exploring and exploiting genetics and genomics for sweetpotato improvement: Status and perspectives’ is the present hot paper on sweetpotato research (Yan et al., 2022). Moreover, the acquisition of genomic information also provides the possibility for genome-wide gene family identification. Presently, approximately 35 different types of gene family papers have been published in hexaploid and its wild species, such as NAC (Guo et al., 2022), bZIP (Liu et al., 2022a), MADS-box (Shao et al., 2021) and SWEET (Dai et al., 2022), which lays a solid foundation for the standardized naming, evolutionary relationship, and functional analysis of genes.
During this period, the application of multi-omics in sweetpotato research, especially transcriptomic analysis, has made great progress. Approximately 85 relevant papers were published, mainly focusing on the response to abiotic stresses such as salt, drought, and low temperature (Ji et al., 2019; Zhu et al., 2019; Arisha et al., 2020; Meng et al., 2020), and anthocyanin biosynthesis and regulation (Qin et al., 2020; Li et al., 2021b; Zhang et al., 2022b), with approximately 25 and 15 papers, respectively. Moreover, many advances have also been made in biotic stress response (Lin et al., 2017; Lee et al., 2019), tuberous root formation and development (Wang et al., 2015; Ponniah et al., 2017; Dong et al., 2019), carotenoid biosynthesis (Li et al., 2015; Jia et al., 2022), and starch biosynthesis (Kou et al., 2020; Qin et al., 2021).
Moreover, many aspects of the rapid stage of research, such as whiteflies, viruses, flavonoids, and gene function identification, have made unprecedented significant progress. For example, the genome sequencing of the sweetpotato whitefly was completed (Xie et al., 2017), the insecticide resistance and management and control strategy of B. tabaci species were reviewed (Horowitz et al., 2020; Saurabh et al., 2021), and the invasion biology and management of sweetpotato whitefly in China were investigated (Guo et al., 2021). Correspondingly, research on SPVD and its prevention and control has also been continuously a hot topic, especially through molecular biology methods. Species and genetic variability of sweetpotato viruses in China (Liu et al., 2017; Wang et al., 2021) and virus incidence in Korea (Kim et al., 2017) were investigated, and the degeneration, decreased storage root yield and quality due to virus diseases, and their spread, transmission, and control were discussed (Gibson and Kreuze, 2015; Loebenstein, 2015; Hou et al., 2020). In detail, targeting SPCSV-RNase3 by CRISPR-Cas13, inhibition of miR397 by short tandem target mimic (STTM) technology, and gene silencing induced by coat protein gene segments of various sweetpotato viruses could confer resistance against SPVD (Sivparsad and Gubba, 2014; Yu et al., 2022; Li et al., 2022a). In addition, new extensive progress has been made in the exploration and control of many other types of sweetpotato pests and bacterial pathogens, and multiple biotic stress resistance genes have been identified in this period, including chit42 for white rot (Ojaghian et al., 2020), IbBBX24 for Fusarium wilt (Zhang et al., 2020a), IbMPK3/IbMPK6-mediated IbSPF1 for Pseudomonas syringae (Kim et al., 2019), IbSWEET10 for Fusarium oxysporum (Li et al., 2017), and IbNAC1 for pest S. litura (Chen et al., 2016). Furthermore, the exploration and control of sweetpotato weevil, parasitic nematodes, and root-knot nematodes have also achieved fruitful results (Kim and Yang, 2019; Okada et al., 2019; Nokihara et al., 2021).
More attention has been focused on research on the health-promoting functions of sweetpotato storage roots and leaves. The functionalities of various components such as carotenoids, anthocyanins, and caffeoylquinic acids were further determined through plenty of in vitro and in vivo assays. For example, the functional components of sweetpotato and their health effects, medicinal applications, and genetic improvements were comprehensively summarized in many review papers (Mohanraj and Sivasankar, 2014; De Albuquerque et al., 2019; Li et al., 2019a; Alam, 2021; Nguyen et al., 2021). Correspondingly, the chemical characterizations, nutritional functions, and antioxidant properties of roots and leaves were systematically studied (Sun et al., 2014b; Fu et al., 2016; Vishnu et al., 2019). In particular, the efficacy of anthocyanins in purple-fleshed sweetpotato in a series of diseases, including colon, bladder, and breast cancers (Li et al., 2018; Mazewski et al., 2018; Xu et al., 2018), inflammation (Sugata et al., 2015), hyperuricemia (Zhang et al., 2015), kidney and liver damage (Shan et al., 2014; Sun et al., 2014a), cognitive deficit (Zhuang et al., 2019), diabetes (Luo et al., 2021), and skin aging (Zhi et al., 2020) has attracted increasing attention and recognition from scholars. At the same time, many genes involved in their biosynthesis pathways and genetic modification/improvement have been identified, for instance, the IbMYB340-bHLH2-NAC56 complex (Wei et al., 2020), IbERF71 (Ning et al., 2021), and IbMYB44 (Li et al., 2021a) for anthocyanin biosynthesis and IbOr (Goo et al., 2015), IbLCYB2 (Kang et al., 2018), and IbGGPS (Li et al., 2022b) for carotenoid biosynthesis.
Furthermore, the development and popularization of sweetpotato transgenic technology have significantly improved the functional elucidation of related genes. The process of sweetpotato in response to environmental stresses has always been the focus and hotspot of research worldwide as described above. Among various abiotic stresses, salt and drought are the two main constraints for sweetpotato production, as most of them are produced in semi-arid regions (Solis Sarmiento, 2012). In this period, many successful stories about enhancing its drought and salt tolerance via genetic engineering have been reported. The encoding products of these genes are involved in a variety of pathways to confer sweetpotato resistance to salt and drought stress, mainly focusing on osmotic adjustments; for example, IbPYL8, IbMIPS1, and IbPSS1 all confer sweetpotato salt and/or drought tolerance (Zhai et al., 2016; Yu et al., 2020; Xue et al., 2022); ion homeostasis, such as IbNHX2, could enhance salt and drought tolerance (Wang et al., 2016a); antioxidation, such as CuZnSOD, APX, IbCAT2, and IbLCYB2, improve stress tolerance through the enzymatic or non-enzymatic system (Yan et al., 2016; Yong et al., 2017; Kang et al., 2018); transcriptional regulation and signal transduction, such as IbBBX24, IbbHLH66, IbNAC7, IbC3H18, IbSnRK1, and IbSIZ1a/b/c, all positively regulate the stress tolerance (Zhang et al., 2019; Meng et al., 2020; Ren et al., 2020; Xue et al., 2022; Zhang et al., 2022a).
Furthermore, because sweetpotato is susceptible to chilling damage (4°C–10°C), the identification of cold stress tolerance genes began to receive particular attention at this stage, such as IbMPK3 (Jin et al., 2022), IbbHLH116 (Pan et al., 2022), and IbCAD1 (Lee et al., 2021). Other stress resistance genes, such as those involved in the response to oxidative stress (Seo et al., 2015), heat stress (Ji et al., 2017), cadmium stress (Huo et al., 2018), and iron deficiency (Zhu et al., 2022), have also progressed. Moreover, functional explorations of genes related to tuberous root formation and development continue to show its hotspots, and the functions of multiple related genes have been revealed. For instance, the progress in molecular studies on storage root formation and physicochemical properties and molecular structures of starch in sweetpotato were summarized (Zhu and Wang, 2014; Tanaka, 2016), and genome-wide identification of candidate genes involved in storage root development was explored (Li et al., 2019b; Bararyenya et al., 2020). The roles of multiple genes in tuberous root development, such as IbNAC083 (He et al., 2021), IbRAP2.4 (Bian et al., 2022), IbCAD1 (Lee et al., 2021), and IbPAL1 (Yu et al., 2021), and starch biosynthesis, such as IbSnRK1 (Ren et al., 2019), IbVP1 (Fan et al., 2021), and IbAATP (Wang et al., 2016b), were assessed. Moreover, the knockout of starch biosynthesis genes IbGBSSI and IbSBEII via CRISPR/Cas9 to improve starch quality has been attempted (Wang et al., 2019a). This is the first application of CRISPR/Cas technology in sweetpotato breeding, which provides new opportunities for polyploid crops to accelerate breeding efficiency and increase yield and starch properties.
As an economically important crop, sweetpotato has played a pivotal role in many aspects of its long history, such as food, energy, health, and environmental adaptation. In this review, the development history of sweetpotato research over the past 30 years is visually and comprehensively analyzed using the CiteSpace metrological analysis software, including the basic context, research priority, and development trend through the WoS database. Quantitative analysis and visual review show that Asia, especially China, has an important position in sweetpotato research. Although the USA has a small share of sweetpotato production, it is dominant in sweetpotato research and has a key influence in the global cooperation network. Contrarily, high sweetpotato yields in African countries do not match their research outputs. Generally, the research subjects of sweetpotato in China and abroad are roughly the same, but the contents and focus are different. Sweetpotato research in China involves more diverse research disciplines, covering a wide range of morphological, physiological, and biochemical responses and molecular dynamics studies, while the studies abroad pay more attention to its microscopic processes.
Keyword analysis shows primary research points and development directions in three main periods: preliminary stage (1993–2005), rapid stage (2006–2013), and diversified maturity stage (2014–2022). In recent years, sweetpotato research has developed rapidly, with unprecedented volume, diversity, and depth. Continuous development and innovation are in full swing in a multitude of fields of sweetpotato biology research, from genome sequencing to genetic improvement and application of genes related to tuberous root development, starch and pigment synthesis, and stress resistance; from B. tabaci and virus investigation to their prevention and control; and from anthocyanin and carotenoid identification to their antioxidant properties and health-promoting functions. Collectively, the general trend is gradually shifting from macro-scale to micro-scale and detailed exploration. Increasing studies have focused on revealing the relationships between morphological and physiological characteristics of sweetpotato and genetic information.
Although sweetpotato has incomparable advantages over other staple crops in solving the food crisis and climate change worldwide, its basic research is still seriously lagging compared with other staple crops such as rice, wheat, and maize. Through retrospective analysis, we can conclude that sweetpotato production still faces many thorny challenges and difficulties that require scholars’ continuous innovation and collaborative efforts to solve. 1) Accelerate the breeding efficiency of sweetpotato. The application of traditional breeding methods is obviously lagging behind due to the complicated polyploidy genome, limited flowering ability, and self- and cross-incompatibility of sweetpotato. As summarized above, in the past 15 years, with the continuous development of sweetpotato transgenic technology, sweetpotato genetic engineering has made remarkable progress, while more efficient and practical genetic transformation technology remains to be further developed, e.g., the cut-dip-budding delivery system (Cao et al., 2023). Furthermore, precise editing of starch biosynthesis genes via CRISPR/Cas9 and SPCSV-RNase3 by CRISPR/Cas13 was shown to improve the starch quality and SPVD resistance in sweetpotato, respectively (Wang et al., 2019a; Yu et al., 2022), which will promote its breeding efficiency of genetic improvement. However, studies using the existing and subsequently improved CRISPR/Cas system to improve the related traits of sweetpotato are warranted. 2) Enhance the resistance to biotic and abiotic stresses. The yield and quality of sweetpotato are still observably affected by various adverse environmental conditions and virus diseases. The current study mainly focused on single stress or single genes, which will significantly limit the application of laboratory achievements in actual production. Therefore, more efforts should be made to research sweetpotato resistance to simultaneous abiotic stresses, crosstalk between abiotic and biotic stress signals, and co-expression of multiple genes. Additionally, the economical and applicable methods for large-scale production of disease-free sweetpotato materials through different types of tissue culture need to be continuously improved and explored. 3) Coordinate high yield with high quality and high resistance. Understanding the reciprocal regulation between stress-response and growth-control pathways is critical for resetting their balance and thus engineering stress-resistant and high-yield crops (Zhang et al., 2020b). With the significant development of agricultural production, although the yield should not be the primary goal of evaluating the quality of sweetpotato varieties (including edible quality, processing quality, and storage quality), no matter what type of sweetpotato, the yield traits must be considered. The current research isolated high yield, high quality, and high resistance, such as focusing only on the improvement of stress resistance while ignoring whether it affects yield or quality, or their opposite concerns. 4) Develop in-depth the health functions and added values. Promoting sweetpotato consumption is the original impetus for increasing its investment in multiple dimensions. Continuous and in-depth exploration of the health benefits and demands of sweetpotato is particularly critical, for instance, orange- and purple-fleshed sweetpotato in nutritional security and medicinal value; the development of new products including sweetpotato fries, wedges, bread, baked slices, and frozen products can enhance the industrial demand. Therefore, in order to cope with these challenges, long-term efforts and exhaustive research are needed under the synergistic mode of traditional and modern methods to cultivate elite sweetpotato varieties that reconcile high resistance and high quality with high yield.
XM: Funding acquisition, Investigation, Software, Validation, Writing – original draft. TD: Funding acquisition, Writing – review & editing. ZL: Funding acquisition, Writing – review & editing. MZ: Conceptualization, Formal analysis, Funding acquisition, Investigation, Software, Supervision, Writing – original draft, Writing – review & editing.
The author(s) declare financial support was received for the research, authorship, and/or publication of this article. This work was supported by grants from the National Natural Science Foundation of China (32171936, 32201703, and 32072117), the earmarked fund for CARS-10-Sweetpotato, and the Natural Science Foundation of Jiangsu Province (BK20231174). The authors apologize to those whose work is not cited due to space limitations.
The authors are grateful to Lili Tan for assistance in visual analysis using the CiteSpace software.
The authors declare that the research was conducted in the absence of any commercial or financial relationships that could be construed as a potential conflict of interest.
All claims expressed in this article are solely those of the authors and do not necessarily represent those of their affiliated organizations, or those of the publisher, the editors and the reviewers. Any product that may be evaluated in this article, or claim that may be made by its manufacturer, is not guaranteed or endorsed by the publisher.
The Supplementary Material for this article can be found online at: https://www.frontiersin.org/articles/10.3389/fpls.2024.1428975/full#supplementary-material
Ahmed, S., Khan, M. S. S., Xue, S., Islam, F., Ikram, A. U., Abdullah, M., et al. (2024). A comprehensive overview of omics-based approaches to enhance biotic and abiotic stress tolerance in sweet potato. Horticulture Res. 11, uhae014. doi: 10.1093/hr/uhae014
Alam, M. K. (2021). A comprehensive review of sweet potato (Ipomoea batatas [L.] Lam): Revisiting the associated health benefits. Trends Food Sci. Technol. 115, 512–529. doi: 10.1016/j.tifs.2021.07.001
Arisha, M. H., Aboelnasr, H., Ahmad, M. Q., Liu, Y., Tang, W., Gao, R., et al. (2020). Transcriptome sequencing and whole genome expression profiling of hexaploid sweetpotato under salt stress. BMC Genomics 21, 197. doi: 10.1186/s12864-020-6524-1
Bach, D., Bedin, A. C., Lacerda, L. G., Nogueira, A., Demiate, I. M. (2021). Sweet potato (Ipomoea batatas L.): a versatile raw material for the food industry. Braz. Arch. Biol. Technol. 64, e21200568. doi: 10.1590/1678-4324-2021200568
Bararyenya, A., Olukolu, B. A., Tukamuhabwa, P., Grüneberg, W. J., Ekaya, W., Low, J., et al. (2020). Genome-wide association study identified candidate genes controlling continuous storage root formation and bulking in hexaploid sweetpotato. BMC Plant Biol. 20, 1–16. doi: 10.1186/s12870-019-2217-9
Behera, S., Chauhan, V. B. S., Pati, K., Bansode, V., Nedunchezhiyan, M., Verma, A. K., et al. (2022). Biology and biotechnological aspect of sweet potato (Ipomoea batatas L.): A commercially important tuber crop. Planta 256, 40. doi: 10.1007/s00425-022-03938-8
Bian, X., Kim, H. S., Kwak, S.-S., Zhang, Q., Liu, S., Ma, P., et al. (2022). Different functions of ibRAP2.4, a drought-responsive AP2/ERF transcription factor, in regulating root development between arabidopsis and sweetpotato. Front. Plant Sci. 13, 820450. doi: 10.3389/fpls.2022.820450
Boykin, L. M., Shatters, R. G., Jr., Rosell, R. C., Mckenzie, C. L., Bagnall, R. A., De Barro, P., et al. (2007). Global relationships of Bemisia tabaci (Hemiptera: Aleyrodidae) revealed using Bayesian analysis of mitochondrial COI DNA sequences. Mol. Phylogenet. Evol. 44, 1306–1319. doi: 10.1016/j.ympev.2007.04.020
Brown, J. K., Frohlich, D. R., Rosell, R. C. (1995). The sweetpotato or silverleaf whiteflies: Biotypes of Bemisia tabaci or a species complex? Annu. Rev. Entomology 40, 511–534. doi: 10.1146/annurev.en.40.010195.002455
Buteler, M. I., Jarret, R. L., Labonte, D. R. (1999). Sequence characterization of microsatellites in diploid and polyploid Ipomoea. Theor. Appl. Genet. 99, 123–132. doi: 10.1007/s001220051216
Byrne, D. N. (1999). Migration and dispersal by the sweet potato whitefly, Bemisia tabaci. Agric. For. Meteorology 97, 309–316. doi: 10.1016/S0168-1923(99)00074-X
Cao, G. H., Sofic, E., Prior, R. L. (1996). Antioxidant capacity of tea and common vegetables. J. Agric. Food Chem. 44, 3426–3431. doi: 10.1021/jf9602535
Cao, X., Xie, H., Song, M., Lu, J., Ma, P., Huang, B., et al. (2023). Cut–dip–budding delivery system enables genetic modifications in plants without tissue culture. Innovation 4, 100345. doi: 10.1016/j.xinn.2022.100345
Chen, C. (2006). CiteSpace II: Detecting and visualizing emerging trends and transient patterns in scientific literature. J. Am. Soc. Inf. Sci. Technol. 57, 359–377. doi: 10.1002/asi.20317
Chen, H. J., Su, C. T., Lin, C. H., Huang, G. J., Lin, Y. H. (2010). Expression of sweet potato cysteine protease SPCP2 altered developmental characteristics and stress responses in transgenic Arabidopsis plants. J. Plant Physiol. 167, 838–847. doi: 10.1016/j.jplph.2010.01.005
Chen, S.-P., Lin, I. W., Chen, X., Huang, Y.-H., Chang, S.-C., Lo, H.-S., et al. (2016). Sweet potato NAC transcription factor, IbNAC1, upregulates sporamin gene expression by binding the SWRE motif against mechanical wounding and herbivore attack. Plant J. 86, 234–248. doi: 10.1111/tpj.13171
Chiel, E., Gottlieb, Y., Zchori-Fein, E., Mozes-Daube, N., Katzir, N., Inbar, M., et al. (2007). Biotype-dependent secondary symbiont communities in sympatric populations of Bemisia tabaci. Bull. Entomological Res. 97, 407–413. doi: 10.1017/S0007485307005159
Clark, C. A., Davis, J. A., Mukasa, S. B., Abad, J. A., Tugume, A. K., Cuellar, W. J., et al. (2012). Sweetpotato viruses: 15 years of progress on understanding and managing complex diseases. Plant Dis. 96, 168–185. doi: 10.1094/PDIS-07-11-0550
Cuellar, W. J., Kreuze, J. F., Rajamaki, M.-L., Cruzado, K. R., Untiveros, M., Valkonen, J. P. T. (2009). Elimination of antiviral defense by viral RNase III. Proc. Natl. Acad. Sci. United States America 106, 10354–10358. doi: 10.1073/pnas.0806042106
Cuthbertson, A. G. S., Blackburn, L. F., Eyre, D. P., Cannon, R. J. C., Miller, J., Northing, P. (2011). Bemisia tabaci: The current situation in the UK and the prospect of developing strategies for eradication using entomopathogens. Insect Sci. 18, 1–10. doi: 10.1111/ins.2011.18.issue-1
Dai, Z., Yan, P., He, S., Jia, L., Wang, Y., Liu, Q., et al. (2022). Genome-wide identification and expression analysis of SWEET family genes in sweet potato and its two diploid relatives. Int. J. Mol. Sci. 23, 15848. doi: 10.3390/ijms232415848
De Albuquerque, T. M. R., Sampaio, K. B., De Souza, E. L. (2019). Sweet potato roots: Unrevealing an old food as a source of health promoting bioactive compounds–A review. Trends Food Sci. Technol. 85, 277–286. doi: 10.1016/j.tifs.2018.11.006
Dong, T., Zhu, M., Yu, J., Han, R., Tang, C., Xu, T., et al. (2019). RNA-Seq and iTRAQ reveal multiple pathways involved in storage root formation and development in sweet potato (Ipomoea batatas L.). BMC Plant Biol. 19, 1–16. doi: 10.1186/s12870-019-1731-0
Fan, W., Zhang, Y., Wu, Y., Zhou, W., Yang, J., Yuan, L., et al. (2021). The H+-pyrophosphatase IbVP1 regulates carbon flux to influence the starch metabolism and yield of sweet potato. Horticulture Res. 8, 20. doi: 10.1038/s41438-020-00454-2
Feng, C., Yin, Z., Ma, Y., Zhang, Z., Chen, L., Wang, B., et al. (2011). Cryopreservation of sweetpotato (Ipomoea batatas) and its pathogen eradication by cryotherapy. Biotechnol. Adv. 29, 84–93. doi: 10.1016/j.biotechadv.2010.09.002
Feng, J., Zhao, S., Li, M., Zhang, C., Qu, H., Li, Q., et al. (2020). Genome-wide genetic diversity detection and population structure analysis in sweetpotato (Ipomoea batatas) using RAD-seq. Genomics 112, 1978–1987. doi: 10.1016/j.ygeno.2019.11.010
Firon, N., Labonte, D., Villordon, A., Kfir, Y., Solis, J., Lapis, E., et al. (2013). Transcriptional profiling of sweetpotato (Ipomoea batatas) roots indicates down-regulation of lignin biosynthesis and up-regulation of starch biosynthesis at an early stage of storage root formation. BMC Genomics 14, 1–25. doi: 10.1186/1471-2164-14-460
Fu, Z.-F., Tu, Z.-C., Zhang, L., Wang, H., Wen, Q.-H., Huang, T. (2016). Antioxidant activities and polyphenols of sweet potato (Ipomoea batatas L.) leaves extracted with solvents of various polarities. Food bioscience 15, 11–18. doi: 10.1016/j.fbio.2016.04.004
Gao, F., Gong, Y. F., Zhang, P. B. (2000). Production and deployment of virus-free sweetpotato in China. Crop Prot. 19, 105–111. doi: 10.1016/S0261-2194(99)00085-X
Gao, S., Yuan, L., Zhai, H., Liu, C. L., He, S. Z., Liu, Q. C. (2011). Transgenic sweetpotato plants expressing an LOS5 gene are tolerant to salt stress. Plant Cell Tissue Organ Culture 107, 205–213. doi: 10.1007/s11240-011-9971-1
Gibson, R. W., Kreuze, J. F. (2015). Degeneration in sweetpotato due to viruses, virus-cleaned planting material and reversion: a review. Plant Pathol. 64, 1–15. doi: 10.1111/ppa.12273
Goo, Y.-M., Han, E.-H., Jeong, J. C., Kwak, S.-S., Yu, J., Kim, Y.-H., et al. (2015). Overexpression of the sweet potato IbOr gene results in the increased accumulation of carotenoid and confers tolerance to environmental stresses in transgenic potato. Comptes rendus biologies 338, 12–20. doi: 10.1016/j.crvi.2014.10.006
Guo, F., Liu, S., Zhang, C., Dong, T., Meng, X., Zhu, M. (2022). Genome-wide systematic survey and analysis of NAC transcription factor family and their response to abiotic stress in sweetpotato. Scientia Hortic. 299, 111048. doi: 10.1016/j.scienta.2022.111048
Guo, C.-L., Zhu, Y.-Z., Zhang, Y.-J., Keller, M. A., Liu, T.-X., Chu, D. (2021). Invasion biology and management of sweetpotato whitefly (Hemiptera: aleyrodidae) in China. J. Integrated Pest Manage. 12, 2. doi: 10.1093/jipm/pmaa024
He, S., Wang, H., Hao, X., Wu, Y., Bian, X., Yin, M., et al. (2021). Dynamic network biomarker analysis discovers IbNAC083 in the initiation and regulation of sweet potato root tuberization. Plant J. 108, 793–813. doi: 10.1111/tpj.15478
Hirakawa, H., Okada, Y., Tabuchi, H., Shirasawa, K., Watanabe, A., Tsuruoka, H., et al. (2015). Survey of genome sequences in a wild sweet potato, Ipomoea trifida (H. B. K.) G. Don. DNA Res. 22, 171–179. doi: 10.1093/dnares/dsv002
Hoff, J., Howe, J., Mitchell, C. A. (1982). Nutritional and cultural aspects of plant species selection for a controlled ecological life support system (No. NASA-CR-166324).
Horowitz, A. R., Ghanim, M., Roditakis, E., Nauen, R., Ishaaya, I. (2020). Insecticide resistance and its management in Bemisia tabaci species. J. Pest Sci. 93, 893–910. doi: 10.1007/s10340-020-01210-0
Hou, F., Xie, B., Qin, Z., Li, A., Dong, S., Zhang, H., et al. (2020). Sweetpotato leaf curl virus decreased storage root yield and quality of edible sweetpotato in China. Agron. J. 112, 3948–3962. doi: 10.1002/agj2.20025
Huang, J. C., Sun, M. (2000). Genetic diversity and relationships of sweetpotato and its wild relatives in Ipomoea series Batatas (Convolvulaceae) as revealed by inter-simple sequence repeat (ISSR) and restriction analysis of chloroplast DNA. Theor. Appl. Genet. 100, 1050–1060. doi: 10.1007/s001220051386
Huo, J., Du, B., Sun, S., He, S., Zhao, N., Liu, Q., et al. (2018). A novel aldo-keto reductase gene, IbAKR, from sweet potato confers higher tolerance to cadmium stress in tobacco. Front. Agric. Sci. Eng. 5, 206–213. doi: 10.15302/J-FASE-2018225
Islam, S. (2006). Sweetpotato (Ipomoea batatas L.) leaf: its potential effect on human health and nutrition. J. Food Sci. 71, R13–R121. doi: 10.1111/j.1365-2621.2006.tb08912.x
Ji, C. Y., Bian, X., Lee, C.-J., Kim, H. S., Kim, S.-E., Park, S.-C., et al. (2019). De novo transcriptome sequencing and gene expression profiling of sweet potato leaves during low temperature stress and recovery. Gene 700, 23–30. doi: 10.1016/j.gene.2019.02.097
Ji, C. Y., Jin, R., Xu, Z., Kim, H. S., Lee, C.-J., Kang, L., et al. (2017). Overexpression of Arabidopsis P3B increases heat and low temperature stress tolerance in transgenic sweetpotato. BMC Plant Biol. 17, 1–11. doi: 10.1186/s12870-017-1087-2
Jia, R., Zhang, R., Gangurde, S. S., Tang, C., Jiang, B., Li, G., et al. (2022). Integrated analysis of carotenoid metabolites and transcriptome identifies key genes controlling carotenoid compositions and content in sweetpotato tuberous roots (Ipomoea batatas L.). Front. Plant Sci. 13, 993682. doi: 10.3389/fpls.2022.993682
Jin, R., Kim, H. S., Yu, T., Liu, M., Yu, W., Zhao, P., et al. (2022). Overexpression of IbMPK3 increases low-temperature tolerance in transgenic sweetpotato. Plant Biotechnol. Rep. 16, 91–100. doi: 10.1007/s11816-021-00730-0
Johnson, M., Pace, R. D. (2010). Sweet potato leaves: properties and synergistic interactions that promote health and prevent disease. Nutr. Rev. 68, 604–615. doi: 10.1111/nure.2010.68.issue-10
Kang, C., Zhai, H., Xue, L., Zhao, N., He, S., Liu, Q. (2018). A lycopene β-cyclase gene, IbLCYB2, enhances carotenoid contents and abiotic stress tolerance in transgenic sweetpotato. Plant Sci. 272, 243–254. doi: 10.1016/j.plantsci.2018.05.005
Khan, M. A., Gemenet, D. C., Villordon, A. (2016). Root system architecture and abiotic stress tolerance: current knowledge in root and tuber crops. Front. Plant Sci. 7, 1584. doi: 10.3389/fpls.2016.01584
Kim, S. H., Ahn, Y. O., Ahn, M.-J., Jeong, J. C., Lee, H.-S., Kwak, S.-S. (2013). Cloning and characterization of an Orange gene that increases carotenoid accumulation and salt stress tolerance in transgenic sweetpotato cultures. Plant Physiol. Biochem. 70, 445–454. doi: 10.1016/j.plaphy.2013.06.011
Kim, S. H., Ahn, Y. O., Ahn, M.-J., Lee, H.-S., Kwak, S.-S. (2012). Down-regulation of β-carotene hydroxylase increases β-carotene and total carotenoids enhancing salt stress tolerance in transgenic cultured cells of sweetpotato. Phytochemistry 74, 69–78. doi: 10.1016/j.phytochem.2011.11.003
Kim, H. S., Bian, X., Lee, C.-J., Kim, S.-E., Park, S.-C., Xie, Y., et al. (2019). IbMPK3/IbMPK6-mediated IbSPF1 phosphorylation promotes tolerance to bacterial pathogen in sweetpotato. Plant Cell Rep. 38, 1403–1415. doi: 10.1007/s00299-019-02451-9
Kim, Y.-H., Yang, J.-W. (2019). Recent research on enhanced resistance to parasitic nematodes in sweetpotato. Plant Biotechnol. Rep. 13, 559–566. doi: 10.1007/s11816-019-00557-w
Kim, J., Yang, J. W., Kwak, H.-R., Kim, M.-K., Seo, J.-K., Chung, M.-N., et al. (2017). Virus incidence of sweet potato in korea from 2011 to 2014. Plant Pathol. J. 33, 467–477. doi: 10.5423/PPJ.OA.08.2016.0167
Kimura, M., Kobori, C. N., Rodriguez-Amaya, D. B., Nestel, P. (2007). Screening and HPLC methods for carotenoids in sweetpotato, cassava and maize for plant breeding trials. Food Chem. 100, 1734–1746. doi: 10.1016/j.foodchem.2005.10.020
Kitahara, K., Hamasuna, K., Nozuma, K., Otani, M., Hamada, T., Shimada, T., et al. (2007). Physicochemical properties of amylose-free and high-amylose starches from transgenic sweetpotatoes modified by RNA interference. Carbohydr. Polymers 69, 233–240. doi: 10.1016/j.carbpol.2006.09.025
Kokkinos, C. D., Clark, C. A. (2006). Real-time PCR assays for detection and quantification of sweetpotato viruses. Plant Dis. 90, 783–788. doi: 10.1094/PD-90-0783
Kou, M., Su, Z.-X., Zhang, Y.-G., Li, Q. (2020). Comparative transcriptome analysis of Sweetpotato (Ipomoea batatas L.) and discovery of genes involved in starch biosynthesis. Plant Biotechnol. Rep. 14, 713–723. doi: 10.1007/s11816-020-00647-0
Ku, A. T., Huang, Y.-S., Wang, Y.-S., Ma, D., Yeh, K.-W. (2008). IbMADS1 (Ipomoea batatas MADS-box 1 gene) is involved in tuberous root initiation in sweet potato (Ipomoea batatas). Ann. Bot. 102, 57–67. doi: 10.1093/aob/mcn067
Kurata, R., Adachi, M., Yamakawa, O., Yoshimoto, M. (2007). Growth suppression of human cancer cells by polyphenolics from sweetpotato (Ipomoea batatas L.) leaves. J. Agric. Food Chem. 55, 185–190. doi: 10.1021/jf0620259
Lee, C.-J., Kim, S.-E., Park, S.-U., Lim, Y.-H., Choi, H.-Y., Kim, W.-G., et al. (2021). Tuberous roots of transgenic sweetpotato overexpressing IbCAD1 have enhanced low-temperature storage phenotypes. Plant Physiol. Biochem. 166, 549–557. doi: 10.1016/j.plaphy.2021.06.024
Lee, I. H., Shim, D., Jeong, J. C., Sung, Y. W., Nam, K. J., Yang, J.-W., et al. (2019). Transcriptome analysis of root-knot nematode (Meloidogyne incognita)-resistant and susceptible sweetpotato cultivars. Planta 249, 431–444. doi: 10.1007/s00425-018-3001-z
Lee, J. J., Park, K. W., Kwak, Y. S., Ahn, J. Y., Jung, Y. H., Lee, B. H., et al. (2012). Comparative proteomic study between tuberous roots of light orange- and purple-fleshed sweetpotato cultivars. Plant Sci. 193, 120–129. doi: 10.1016/j.plantsci.2012.06.003
Lee, Y.-P., Kim, S.-H., Bang, J.-W., Lee, H.-S., Kwak, S.-S., Kwon, S.-Y. (2007). Enhanced tolerance to oxidative stress in transgenic tobacco plants expressing three antioxidant enzymes in chloroplasts. Plant Cell Rep. 26, 591–598. doi: 10.1007/s00299-006-0253-z
Li, A., Xiao, R., He, S., An, X., He, Y., Wang, C., et al. (2019a). Research advances of purple sweet potato anthocyanins: extraction, identification, stability, bioactivity, application, and biotransformation. Molecules 24, 3816. doi: 10.3390/molecules24213816
Li, C., Liu, X.-X., Abouelnasr, H., Mohamed Hamed, A., Kou, M., Tang, W., et al. (2022a). Inhibition of miR397 by STTM technology to increase sweetpotato resistance to SPVD. J. Integr. Agric. 21, 2865–2875. doi: 10.1016/j.jia.2022.07.054
Li, L.-X., Wei, Z.-Z., Zhou, Z.-L., Zhao, D.-L., Tang, J., Yang, F., et al. (2021a). A single amino acid mutant in the EAR motif of IbMYB44.2 reduced the inhibition of anthocyanin accumulation in the purple-fleshed sweetpotato. Plant Physiol. Biochem. 167, 410–419. doi: 10.1016/j.plaphy.2021.08.012
Li, M., Yang, S., Xu, W., Pu, Z., Feng, J., Wang, Z., et al. (2019b). The wild sweetpotato (Ipomoea trifida) genome provides insights into storage root development. BMC Plant Biol. 19, 1–17. doi: 10.1186/s12870-019-1708-z
Li, Q., Kou, M., Li, C., Zhang, Y.-G. (2021b). Comparative transcriptome analysis reveals candidate genes involved in anthocyanin biosynthesis in sweetpotato (Ipomoea batatas L.). Plant Physiol. Biochem. 158, 508–517. doi: 10.1016/j.plaphy.2020.11.035
Li, R.-J., Zhai, H., He, S.-Z., Zhang, H., Zhao, N., Liu, Q.-C. (2022b). A geranylgeranyl pyrophosphate synthase gene, IbGGPS, increases carotenoid contents in transgenic sweetpotato. J. Integr. Agric. 21, 2538–2546. doi: 10.1016/j.jia.2022.07.029
Li, R., Zhai, H., Kang, C., Liu, D., He, S., Liu, Q. (2015). De novo transcriptome sequencing of the orange-fleshed sweet potato and analysis of differentially expressed genes related to carotenoid biosynthesis. Int. J. Genomics 2015, 843802. doi: 10.1155/2015/843802
Li, S. J., Xue, X., Ahmed, M. Z., Ren, S. X., Du, Y. Z., Wu, J. H., et al. (2011). Host plants and natural enemies of Bemisia tabaci (Hemiptera: Aleyrodidae) in China. Insect Sci. 18, 101–120. doi: 10.1111/ins.2011.18.issue-1
Li, W.-L., Yu, H.-Y., Zhang, X.-J., Ke, M., Hong, T. (2018). Purple sweet potato anthocyanin exerts antitumor effect in bladder cancer. Oncol. Rep. 40, 73–82. doi: 10.3892/or
Li, Y., Wang, Y., Zhang, H., Zhang, Q., Zhai, H., Liu, Q., et al. (2017). The plasma membrane-localized sucrose transporter ibSWEET10 contributes to the resistance of sweet potato to fusarium oxysporum. Front. Plant Sci. 8, 198. doi: 10.3389/fpls.2017.00197
Lin, Y., Zou, W., Lin, S., Onofua, D., Yang, Z., Chen, H., et al. (2017). Transcriptome profiling and digital gene expression analysis of sweet potato for the identification of putative genes involved in the defense response against Fusarium oxysporum f. sp batatas. PloS One 12, e0187838. doi: 10.1371/journal.pone.0187838
Liu, Q. (2017). Improvement for agronomically important traits by gene engineering in sweetpotato. Breed. Sci. 67, 15–26. doi: 10.1270/jsbbs.16126
Liu, Q., Wang, Y., Zhang, Z., Lv, H., Qiao, Q., Qin, Y., et al. (2017). Diversity of sweepoviruses infecting sweet potato in China. Plant Dis. 101, 2098–2103. doi: 10.1094/PDIS-04-17-0524-RE
Liu, S., Zhang, C., Zhu, Q., Guo, F., Chai, R., Wang, M., et al. (2022a). Genome-and transcriptome-wide systematic characterization of bZIP transcription factor family identifies promising members involved in abiotic stress response in sweetpotato. Scientia Hortic. 303, 111185. doi: 10.1016/j.scienta.2022.111185
Liu, X., Zhao, S., Tan, L., Tan, Y., Wang, Y., Ye, Z., et al. (2022b). Frontier and hot topics in electrochemiluminescence sensing technology based on CiteSpace bibliometric analysis. Biosensors Bioelectronics 201, 113932. doi: 10.1016/j.bios.2021.113932
Loebenstein, G. (2015). “Control of Sweet Potato Virus Diseases,” in Advances in Virus Research, Vol 91: Control of Plant Virus Diseases Vegetatively-Propagated Crops. Eds. Loebenstein, G., Katis, N. I. ELSEVIER, 33–45.
Luo, D., Mu, T., Sun, H. (2021). Sweet potato (Ipomoea batatas L.) leaf polyphenols ameliorate hyperglycemia in type 2 diabetes mellitus mice. Food Funct. 12, 4117–4131. doi: 10.1039/D0FO02733B
Luo, Z., Yao, Z., Yang, Y., Wang, Z., Zou, H., Zhang, X., et al. (2023). Genetic fingerprint construction and genetic diversity analysis of sweet potato (Ipomoea batatas) germplasm resources. BMC Plant Biol. 23, 355. doi: 10.1186/s12870-023-04329-1
Lyu, R., Ahmed, S., Fan, W., Yang, J., Wu, X., Zhou, W., et al. (2021). Engineering properties of sweet potato starch for industrial applications by biotechnological techniques including genome editing. Int. J. Mol. Sci. 22, 9533. doi: 10.3390/ijms22179533
Mazewski, C., Liang, K., De Mejia, E. G. (2018). Comparison of the effect of chemical composition of anthocyanin-rich plant extracts on colon cancer cell proliferation and their potential mechanism of action using in vitro, in silico, and biochemical assays. Food Chem. 242, 378–388. doi: 10.1016/j.foodchem.2017.09.086
Meira, M., Silva, E. P. D., David, J. M., David, J. P. (2012). Review of the genus Ipomoea: traditional uses, chemistry and biological activities. Rev. Bras. Farmacognosia 22, 682–713. doi: 10.1590/S0102-695X2012005000025
Meng, X., Liu, S., Dong, T., Xu, T., Ma, D., Pan, S., et al. (2020). Comparative transcriptome and proteome analysis of salt-tolerant and salt-sensitive sweet potato and overexpression of IbNAC7 confers salt tolerance in Arabidopsis. Front. Plant Sci. 11, 572540. doi: 10.3389/fpls.2020.572540
Mohanraj, R., Sivasankar, S. (2014). Sweet Potato (Ipomoea batatas [L.] Lam)-A valuable medicinal food: A review. J. medicinal Food 17, 733–741. doi: 10.1089/jmf.2013.2818
Moorthy, S. N. (2002). Physicochemical and functional properties of tropical tuber starches: A review. Starch-Starke 54, 559–592. doi: 10.1002/(ISSN)1521-379X
Muñoz-Rodríguez, P., Carruthers, T., Wood, J. R., Williams, B. R., Weitemier, K., Kronmiller, B., et al. (2018). Reconciling conflicting phylogenies in the origin of sweet potato and dispersal to Polynesia. Curr. Biol. 28, 1246–1256. doi: 10.1016/j.cub.2018.03.020
Nguyen, H. C., Chen, C.-C., Lin, K.-H., Chao, P.-Y., Lin, H.-H., Huang, M.-Y. (2021). Bioactive compounds, antioxidants, and health benefits of sweet potato leaves. Molecules 26, 1820. doi: 10.3390/molecules26071820
Ning, Z., Hu, K., Zhou, Z., Zhao, D., Tang, J., Wang, H., et al. (2021). IbERF71, with IbMYB340 and IbbHLH2, coregulates anthocyanin accumulation by binding to the IbANS1 promoter in purple-fleshed sweet potato (Ipomoea batatas L.). Plant Cell Rep. 40, 157–169. doi: 10.1007/s00299-020-02621-0
Noh, S. A., Lee, H.-S., Huh, E. J., Huh, G. H., Paek, K.-H., Shin, J. S., et al. (2010). SRD1 is involved in the auxin-mediated initial thickening growth of storage root by enhancing proliferation of metaxylem and cambium cells in sweetpotato (Ipomoea batatas). J. Exp. Bot. 61, 1337–1349. doi: 10.1093/jxb/erp399
Noh, S. A., Lee, H.-S., Kim, Y.-S., Paek, K.-H., Shin, J. S., Bae, J. M. (2013). Down-regulation of the IbEXP1 gene enhanced storage root development in sweetpotato. J. Exp. Bot. 64, 129–142. doi: 10.1093/jxb/ers236
Nokihara, K., Okada, Y., Ohata, S., Monden, Y. (2021). Transcriptome analysis reveals key genes involved in weevil resistance in the hexaploid sweetpotato. Plants-Basel 10, 1535. doi: 10.3390/plants10081535
Ojaghian, S., Wang, L., Xie, G.-L. (2020). Enhanced resistance to white rot in Ipomoea batatas expressing a Trichoderma harzianum chitinase gene. J. Gen. Plant Pathol. 86, 412–418. doi: 10.1007/s10327-020-00942-2
Okada, Y., Monden, Y., Nokihara, K., Shirasawa, K., Isobe, S., Tahara, M. (2019). Genome-wide association studies (GWAS) for yield and weevil resistance in sweet potato (Ipomoea batatas (L.) Lam). Plant Cell Rep. 38, 1383–1392. doi: 10.1007/s00299-019-02445-7
Padda, M. S., Picha, D. H. (2008). Quantification of phenolic acids and antioxidant activity in sweetpotato genotypes. Scientia Hortic. 119, 17–20. doi: 10.1016/j.scienta.2008.07.008
Pan, R., Buitrago, S., Peng, Y., Abou-Elwafa, S. F., Wan, K., Liu, Y., et al. (2022). Genome-wide identification of cold-tolerance genes and functional analysis of IbbHLH116 gene in sweet potato. Gene 837, 146690. doi: 10.1016/j.gene.2022.146690
Park, S.-C., Kim, Y.-H., Jeong, J. C., Kim, C. Y., Lee, H.-S., Bang, J.-W., et al. (2011). Sweetpotato late embryogenesis abundant 14 (IbLEA14) gene influences lignification and increases osmotic-and salt stress-tolerance of transgenic calli. Planta 233, 621–634. doi: 10.1007/s00425-010-1326-3
Philpott, M., Gould, K. S., Lim, C., Ferguson, L. R. (2004). In situ and in vitro antioxidant activity of sweetpotato anthocyanins. J. Agric. Food Chem. 52, 1511–1513. doi: 10.1021/jf034593j
Ponniah, S. K., Thimmapuram, J., Bhide, K., Kalavacharla, V., Manoharan, M. (2017). Comparative analysis of the root transcriptomes of cultivated sweetpotato (Ipomoea batatas [L.] Lam) and its wild ancestor (Ipomoea trifida [Kunth] G. Don). BMC Plant Biol. 17, 1–14. doi: 10.1186/s12870-016-0950-x
Qin, Z., Hou, F., Li, A., Dong, S., Huang, C., Wang, Q., et al. (2020). Comparative analysis of full-length transcriptomes based on hybrid population reveals regulatory mechanisms of anthocyanin biosynthesis in sweet potato (Ipomoea batatas (L.) Lam). BMC Plant Biol. 20, 1–12. doi: 10.1186/s12870-020-02513-1
Qin, Z., Li, A., Dong, S., Wang, Q., Hou, F., Zhang, H. (2021). Comparative transcriptome analysis of hybrid population provides insights into starch content in sweet potato (Ipomoea batatas L.) storage root. Plant Mol. Biol. Rep. 39, 673–684. doi: 10.1007/s11105-021-01282-x
Ren, K., Qiu, J., Wang, X., Niu, F., Jiang, T. (2012). The effect of a sweet potato, footbath, and acupressure intervention in preventing constipation in hospitalized patients with acute coronary syndromes. Gastroenterol. Nurs. 35, 271–277. doi: 10.1097/SGA.0b013e31825ed7bc
Ren, Z., He, S., Zhao, N., Zhai, H., Liu, Q. (2019). A sucrose non-fermenting-1-related protein kinase-1 gene, IbSnRK1, improves starch content, composition, granule size, degree of crystallinity and gelatinization in transgenic sweet potato. Plant Biotechnol. J. 17, 21–32. doi: 10.1111/pbi.12944
Ren, Z., He, S., Zhou, Y., Zhao, N., Jiang, T., Zhai, H., et al. (2020). A sucrose non-fermenting-1-related protein kinase-1 gene, IbSnRK1, confers salt, drought and cold tolerance in sweet potato. Crop J. 8, 905–917. doi: 10.1016/j.cj.2020.04.010
Saurabh, S., Mishra, M., Rai, P., Pandey, R., Singh, J., Khare, A., et al. (2021). Tiny flies: a mighty pest that threatens agricultural productivity—a case for next-generation control strategies of whiteflies. Insects 12, 585. doi: 10.3390/insects12070585
Seo, S.-G., Jang, H.-R., Shin, J.-M., Jun, B. K., Shim, I.-S., Kim, S.-H. (2015). Ectopic expression of ibPDS gene enhanced tolerance to oxidative stress in transgenic tobacco plants. Plant Growth Regul. 77, 245–253. doi: 10.1007/s10725-015-0041-2
Shan, Q., Zheng, Y., Lu, J., Zhang, Z., Wu, D., Fan, S., et al. (2014). Purple sweet potato color ameliorates kidney damage via inhibiting oxidative stress mediated NLRP3 inflammasome activation in high fat diet mice. Food Chem. Toxicol. 69, 339–346. doi: 10.1016/j.fct.2014.04.033
Shao, Z., He, M., Zeng, Z., Chen, Y., Hanna, A.-D., Zhu, H. (2021). Genome-wide identification and expression analysis of the MADS-box gene family in sweet potato ipomoea batatas (L.) lam. Front. Genet. 12, 750137. doi: 10.3389/fgene.2021.750137
Sivparsad, B. J., Gubba, A. (2014). Development of transgenic sweet potato with multiple virus resistance in South Africa (SA). Transgenic Res. 23, 377–388. doi: 10.1007/s11248-013-9759-7
Solis Sarmiento, J. (2012). Genomic approaches to understand sweetpotato root development in relation to abiotic factors (Louisiana State University: Doctoral dissertation).
Su, W., Wang, L., Lei, J., Chai, S., Liu, Y., Yang, Y., et al. (2017). Genome-wide assessment of population structure and genetic diversity and development of a core germplasm set for sweet potato based on specific length amplified fragment (SLAF) sequencing. PloS One 12, e0172066. doi: 10.1371/journal.pone.0172066
Sugata, M., Lin, C.-Y., Shih, Y.-C. (2015). Anti-inflammatory and anticancer activities of Taiwanese purple-fleshed sweet potatoes (Ipomoea batatas L. Lam) extracts. BioMed. Res. Int. 2015, 768093. doi: 10.1155/2015/768093
Sun, H., Mu, T., Liu, X., Zhang, M., Chen, J. (2014a). Purple sweet potato (Ipomoea batatas L.) anthocyanins: Preventive effect on acute and subacute alcoholic liver damage and dealcoholic effect. J. Agric. Food Chem. 62, 2364–2373. doi: 10.1021/jf405032f
Sun, H., Mu, T., Xi, L., Zhang, M., Chen, J. (2014b). Sweet potato (Ipomoea batatas L.) leaves as nutritional and functional foods. Food Chem. 156, 380–389. doi: 10.1016/j.foodchem.2014.01.079
Tairo, F., Mukasa, S. B., Jones, R. C., Kullaya, A., Rubaihayo, P. R., Valkonen, J. P. T. (2005). Unravelling the genetic diversity of the three main viruses involved in Sweet Potato Virus Disease (SPVD), and its practical implications. Mol. Plant Pathol. 6, 199–211. doi: 10.1111/j.1364-3703.2005.00267.x
Tanaka, M. (2016). Recent progress in molecular studies on storage root formation in sweetpotato (Ipomoea batatas). Japan Agric. Res. Q. 50, 293–299. doi: 10.6090/jarq.50.293
Tang, C., Han, J., Chen, D., Zong, S., Liu, J., Kan, J., et al. (2023). Recent advances on the biological activities of purple sweet potato anthocyanins. Food Bioscience 53, 102670. doi: 10.1016/j.fbio.2023.102670
Tao, X., Gu, Y.-H., Jiang, Y.-S., Zhang, Y.-Z., Wang, H.-Y. (2013). Transcriptome analysis to identify putative floral-specific genes and flowering regulatory-related genes of sweet potato. Bioscience Biotechnol. Biochem. 77, 2169–2174. doi: 10.1271/bbb.130218
Tao, X., Gu, Y.-H., Wang, H.-Y., Zheng, W., Li, X., Zhao, C.-W., et al. (2012). Digital gene expression analysis based on integrated De Novo transcriptome assembly of sweet potato Ipomoea batatas (L.) Lam. PloS One 7, e36234. doi: 10.1371/journal.pone.0036234
Teow, C. C., Truong, V.-D., Mcfeeters, R. F., Thompson, R. L., Pecota, K. V., Yencho, G. C. (2007). Antioxidant activities, phenolic and beta-carotene contents of sweet potato genotypes with varying flesh colours. Food Chem. 103, 829–838. doi: 10.1016/j.foodchem.2006.09.033
Truong, V.-D., Deighton, N., Thompson, R. T., Mcfeeters, R. F., Dean, L. O., Pecota, K. V., et al. (2010). Characterization of anthocyanins and anthocyanidins in purple-fleshed sweetpotatoes by HPLC-DAD/ESI-MS/MS. J. Agric. Food Chem. 58, 404–410. doi: 10.1021/jf902799a
Van Jaarsveld, P. J., Faber, M., Tanumihardjo, S. A., Nestel, P., Lombard, C. J., Benadé, A. J. S. (2005). β-Carotene–rich orange-fleshed sweet potato improves the vitamin A status of primary school children assessed with the modified-relative-dose-response test. Am. J. Clin. Nutr. 81, 1080–1087. doi: 10.1093/ajcn/81.5.1080
Van Jaarsveld, P. J., Marais, D. W., Harmse, E., Nestel, P., Rodriguez-Amaya, D. B. (2006). Retention of beta-carotene in boiled, mashed orange-fleshed sweet potato. J. Food Composition Anal. 19, 321–329. doi: 10.1016/j.jfca.2004.10.007
Vishnu, V. R., Renjith, R. S., Mukherjee, A., Anil, S. R., Sreekumar, J., Jyothi, A. N. (2019). Comparative study on the chemical structure and in vitro antiproliferative activity of anthocyanins in purple root tubers and leaves of sweet potato (Ipomoea batatas). J. Agric. Food Chem. 67, 2467–2475. doi: 10.1021/acs.jafc.8b05473
Wadl, P. A., Olukolu, B. A., Branham, S. E., Jarret, R. L., Yencho, G. C., Jackson, D. M. (2018). Genetic diversity and population structure of the USDA sweetpotato (Ipomoea batatas) germplasm collections using GBSpoly. Front. Plant Sci. 9, 1166. doi: 10.3389/fpls.2018.01166
Wang, B., Zhai, H., He, S., Zhang, H., Ren, Z., Zhang, D., et al. (2016a). A vacuolar Na+/H+ antiporter gene, IbNHX2, enhances salt and drought tolerance in transgenic sweetpotato. Scientia Hortic. 201, 153–166. doi: 10.1016/j.scienta.2016.01.027
Wang, H., Wu, Y., Zhang, Y., Yang, J., Fan, W., Zhang, H., et al. (2019a). CRISPR/Cas9-based mutagenesis of starch biosynthetic genes in sweet potato (Ipomoea Batatas) for the improvement of starch quality. Int. J. Mol. Sci. 20, 4702. doi: 10.3390/ijms20194702
Wang, Q., Valkonen, J. P. T. (2009). Cryotherapy of shoot tips: novel pathogen eradication method. Trends Plant Sci. 14, 119–122. doi: 10.1016/j.tplants.2008.11.010
Wang, Q., Zhang, L., Wang, B., Yin, Z., Feng, C., Wang, Q. (2010). Sweetpotato viruses in China. Crop Prot. 29, 110–114. doi: 10.1016/j.cropro.2009.11.002
Wang, X.-P., Cheng, X., Zhang, Y.-J. (2019b). The complete chloroplast genome of a wild sweet potato, Ipomoea trifida (Kunth) G. Don. Mitochondrial DNA Part B 4, 2063–2064. doi: 10.1080/23802359.2019.1574672
Wang, Y.-N., Li, Y., Zhang, H., Zhai, H., Liu, Q.-C., He, S.-Z. (2016b). A plastidic ATP/ADP transporter gene, lbAATP, increases starch and amylose contents and alters starch structure in transgenic sweetpotato. J. Integr. Agric. 15, 1968–1982. doi: 10.1016/S2095-3119(15)61192-3
Wang, Y., Qin, Y., Wang, S., Zhang, D., Tian, Y., Zhao, F., et al. (2021). Species and genetic variability of sweet potato viruses in China. Phytopathol. Res. 3, 1–12. doi: 10.1186/s42483-021-00097-8
Wang, Z., Fang, B., Chen, X., Liao, M., Chen, J., Zhang, X., et al. (2015). Temporal patterns of gene expression associated with tuberous root formation and development in sweetpotato (Ipomoea batatas). BMC Plant Biol. 15, 1–13. doi: 10.1186/s12870-015-0567-5
Wei, Z.-Z., Hu, K.-D., Zhao, D.-L., Tang, J., Huang, Z.-Q., Jin, P., et al. (2020). MYB44 competitively inhibits the formation of the MYB340-bHLH2-NAC56 complex to regulate anthocyanin biosynthesis in purple-fleshed sweet potato. BMC Plant Biol. 20, 1–15. doi: 10.1186/s12870-020-02451-y
Wood, J., Muñoz-Rodríguez, P., Williams, B., Scotland, R. (2020). A foundation monograph of Ipomoea (Convolvulaceae) in the New World. PhytoKeys 143, 1–823. doi: 10.3897/phytokeys.143.32821
Wu, S., Lau, K. H., Cao, Q., Hamilton, J. P., Sun, H., Zhou, C., et al. (2018). Genome sequences of two diploid wild relatives of cultivated sweetpotato reveal targets for genetic improvement. Nat. Commun. 9, 1–12. doi: 10.1038/s41467-018-06983-8
Xie, W., Chen, C., Yang, Z., Guo, L., Yang, X., Wang, D., et al. (2017). Genome sequencing of the sweetpotato whitefly Bemisia tabaci MED/Q. GigaScience 6, gix018. doi: 10.1093/gigascience/gix018
Xu, H., Li, Y., Han, B., Li, Z., Wang, B., Jiang, P., et al. (2018). Anti-breast-cancer activity exerted by β-sitosterol-D-glucoside from sweet potato via upregulation of microRNA-10a and via the PI3K–Akt signaling pathway. J. Agric. Food Chem. 66, 9704–9718. doi: 10.1021/acs.jafc.8b03305
Xue, L., Wei, Z., Zhai, H., Xing, S., Wang, Y., He, S., et al. (2022). The IbPYL8-IbbHLH66-IbbHLH118 complex mediates the abscisic acid-dependent drought response in sweet potato. New Phytol. 236, 2151–2171. doi: 10.1111/nph.18502
Yan, H., Li, Q., Park, S.-C., Wang, X., Liu, Y.-J., Zhang, Y.-G., et al. (2016). Overexpression of CuZnSOD and APX enhance salt stress tolerance in sweet potato. Plant Physiol. Biochem. 109, 20–27. doi: 10.1016/j.plaphy.2016.09.003
Yan, L., Lai, X., Li, X., Wei, C., Tan, X., Zhang, Y. (2015). Analyses of the complete genome and gene expression of chloroplast of sweet potato ipomoea batata. PloS One 10, e0124083. doi: 10.1371/journal.pone.0124083
Yan, M., Nie, H., Wang, Y., Wang, X., Jarret, R., Zhao, J., et al. (2022). Exploring and exploiting genetics and genomics for sweetpotato improvement: Status and perspectives. Plant Commun. 3, 100332. doi: 10.1016/j.xplc.2022.100332
Yang, J., Moeinzadeh, M.-H., Kuhl, H., Helmuth, J., Xiao, P., Haas, S., et al. (2017). Haplotype-resolved sweet potato genome traces back its hexaploidization history. Nat. Plants 3, 696. doi: 10.1038/s41477-017-0002-z
Yang, N., Xie, W., Yang, X., Wang, S. L., Wu, Q. J., Li, R. M., et al. (2013). Transcriptomic and proteomic responses of sweetpotato whitefly, bemisia tabaci, to thiamethoxam. PloS One 8, e61820. doi: 10.1371/journal.pone.0061820
Yang, X.-S., Su, W.-J., Wang, L.-J., Lei, J., Chai, S.-S., Liu, Q.-C. (2015). Molecular diversity and genetic structure of 380 sweetpotato accessions as revealed by SSR markers. J. Integr. Agric. 14, 633–641. doi: 10.1016/S2095-3119(14)60794-2
Yang, Y., Chen, Y., Bo, Y., Liu, Q., Zhai, H. (2023a). Research progress in the mechanisms of resistance to biotic stress in sweet potato. Genes 14, 2106. doi: 10.3390/genes14112106
Yang, Y., Zhu, J., Sun, L., Kong, Y., Chen, J., Zhu, M., et al. (2023b). Progress on physiological and molecular mechanisms of storage root formation and development in sweetpotato. Scientia Hortic. 308, 111588. doi: 10.1016/j.scienta.2022.111588
Yeh, K. W., Lin, M. I., Tuan, S. J., Chen, Y. M., Lin, C. Y., Kao, S. S. (1997). Sweet potato (Ipomoea batatas) trypsin inhibitors expressed in transgenic tobacco plants confer resistance against Spodoptera litura. Plant Cell Rep. 16, 696–699. doi: 10.1007/s002990050304
Yong, B., Wang, X., Xu, P., Zheng, H., Fei, X., Hong, Z., et al. (2017). Isolation and abiotic stress resistance analyses of a catalase gene from Ipomoea batatas (L.) Lam. BioMed. Res. Int. 2017, 6847532. doi: 10.1155/2017/6847532
Yoon, U.-H., Cao, Q., Shirasawa, K., Zhai, H., Lee, T.-H., Tanaka, M., et al. (2022). Haploid-resolved and chromosome-scale genome assembly in hexa-autoploid sweetpotato (Ipomoea batatas (L.) Lam). bioRxiv. doi: 10.1101/2022.12.25.521700
Yu, Y., Pan, Z., Wang, X., Bian, X., Wang, W., Liang, Q., et al. (2022). Targeting of SPCSV-RNase3 via CRISPR-Cas13 confers resistance against sweet potato virus disease. Mol. Plant Pathol. 23, 104–117. doi: 10.1111/mpp.13146
Yu, Y., Wang, Y., Yu, Y., Ma, P., Jia, Z., Guo, X., et al. (2021). Overexpression of IbPAL1 promotes chlorogenic acid biosynthesis in sweetpotato. Crop J. 9, 204–215. doi: 10.1016/j.cj.2020.06.003
Yu, Y., Xuan, Y., Bian, X., Zhang, L., Pan, Z., Kou, M., et al. (2020). Overexpression of phosphatidylserine synthase IbPSS1 affords cellular Na+ homeostasis and salt tolerance by activating plasma membrane Na+/H+ antiport activity in sweet potato roots. Horticulture Res. 7, 1–12. doi: 10.1038/s41438-020-00358-1
Yun, B. W., Huh, G. H., Lee, H. S., Kwon, S. Y., Jo, J. K., Kim, J. S., et al. (2000). Differential resistance to methyl viologen in transgenic tobacco plants that express sweet potato peroxidases. J. Plant Physiol. 156, 504–509. doi: 10.1016/S0176-1617(00)80165-0
Zhai, H., Wang, F., Si, Z., Huo, J., Xing, L., An, Y., et al. (2016). A myo-inositol-1-phosphate synthase gene, IbMIPS1, enhances salt and drought tolerance and stem nematode resistance in transgenic sweet potato. Plant Biotechnol. J. 14, 592–602. doi: 10.1111/pbi.12402
Zhang, H., Gao, X., Zhi, Y., Li, X., Zhang, Q., Niu, J., et al. (2019). A non-tandem CCCH-type zinc-finger protein, IbC3H18, functions as a nuclear transcriptional activator and enhances abiotic stress tolerance in sweet potato. New Phytol. 223, 1918–1936. doi: 10.1111/nph.15925
Zhang, H., Wang, Z., Li, X., Gao, X., Dai, Z., Cui, Y., et al. (2022a). The IbBBX24-IbTOE3-IbPRX17 module enhances abiotic stress tolerance by scavenging reactive oxygen species in sweet potato. New Phytol. 233, 1133–1152. doi: 10.1111/nph.17860
Zhang, H., Zhang, Q., Zhai, H., Gao, S., Yang, L., Wang, Z., et al. (2020a). IbBBX24 promotes the jasmonic acid pathway and enhances fusarium wilt resistance in sweet potato. Plant Cell 32, 1102–1123. doi: 10.1105/tpc.19.00641
Zhang, H., Zhao, Y., Zhu, J.-K. (2020b). Thriving under stress: how plants balance growth and the stress response. Dev. Cell 55, 529–543. doi: 10.1016/j.devcel.2020.10.012
Zhang, R., Li, M., Tang, C., Jiang, B., Yao, Z., Mo, X., et al. (2022b). Combining metabolomics and transcriptomics to reveal the mechanism of coloration in purple and cream mutant of sweet potato (Ipomoea batatas L.). Front. Plant Sci. 13, 877695. doi: 10.3389/fpls.2022.877695
Zhang, T., Oates, C. G. (1999). Relationship between alpha-amylase degradation and physico-chemical properties of sweet potato starches. Food Chem. 65, 157–163. doi: 10.1016/S0308-8146(98)00024-7
Zhang, Z.-C., Su, G.-H., Luo, C.-L., Pang, Y.-L., Wang, L., Li, X., et al. (2015). Effects of anthocyanins from purple sweet potato (Ipomoea batatas L. cultivar Eshu No. 8) on the serum uric acid level and xanthine oxidase activity in hyperuricemic mice. Food Funct. 6, 3045–3055. doi: 10.1039/C5FO00499C
Zhi, Q., Lei, L., Li, F., Zhao, J., Yin, R., Ming, J. (2020). The anthocyanin extracts from purple-fleshed sweet potato exhibited anti-photoaging effects on ultraviolent B-irradiated BALB/c-nu mouse skin. J. Funct. Foods 64, 103640. doi: 10.1016/j.jff.2019.103640
Zhu, F., Wang, S. (2014). Physicochemical properties, molecular structure, and uses of sweetpotato starch. Trends Food Sci. Technol. 36, 68–78. doi: 10.1016/j.tifs.2014.01.008
Zhu, H., Yang, X., Li, Q., Guo, J., Ma, T., Liu, S., et al. (2022). The sweetpotato voltage-gated K+ channel β subunit, KIbB1, positively regulates low-K+ and high-salinity tolerance by maintaining ion homeostasis. Genes 13, 1100. doi: 10.3390/genes13061100
Zhu, H., Zhou, Y.-Y., Zhai, H., He, S.-Z., Zhao, N., Liu, Q.-C. (2019). Transcriptome profiling reveals insights into the molecular mechanism of drought tolerance in sweetpotato. J. Integr. Agric. 18, 9–23. doi: 10.1016/S2095-3119(18)61934-3
Keywords: bibliometrics, CiteSpace, research trend, research hotspot, sweetpotato, visualization analysis
Citation: Meng X, Dong T, Li Z and Zhu M (2024) First systematic review of the last 30 years of research on sweetpotato: elucidating the frontiers and hotspots. Front. Plant Sci. 15:1428975. doi: 10.3389/fpls.2024.1428975
Received: 07 May 2024; Accepted: 17 June 2024;
Published: 04 July 2024.
Edited by:
Kai Zhang, Southwest University, ChinaReviewed by:
Guo-Fei Tan, Guizhou Academy of Agricultural Sciences, ChinaCopyright © 2024 Meng, Dong, Li and Zhu. This is an open-access article distributed under the terms of the Creative Commons Attribution License (CC BY). The use, distribution or reproduction in other forums is permitted, provided the original author(s) and the copyright owner(s) are credited and that the original publication in this journal is cited, in accordance with accepted academic practice. No use, distribution or reproduction is permitted which does not comply with these terms.
*Correspondence: Mingku Zhu, bWluZ2t1emh1MDA3QDEyNi5jb20=
Disclaimer: All claims expressed in this article are solely those of the authors and do not necessarily represent those of their affiliated organizations, or those of the publisher, the editors and the reviewers. Any product that may be evaluated in this article or claim that may be made by its manufacturer is not guaranteed or endorsed by the publisher.
Research integrity at Frontiers
Learn more about the work of our research integrity team to safeguard the quality of each article we publish.