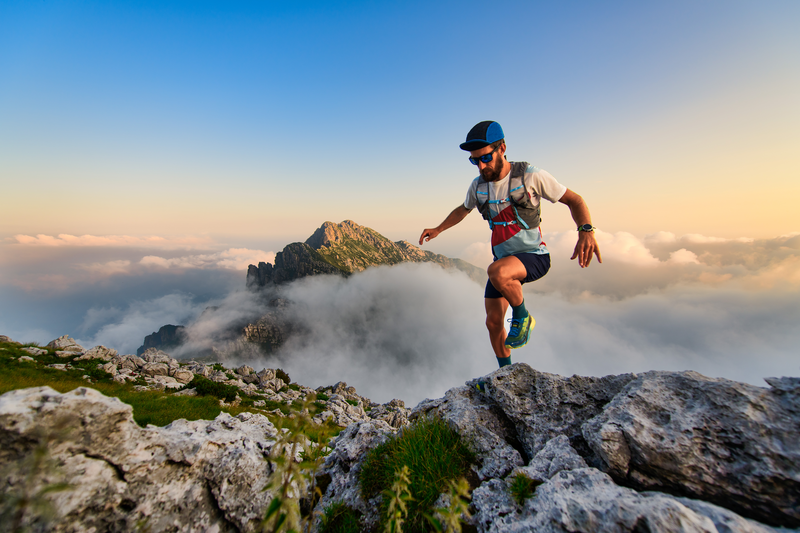
94% of researchers rate our articles as excellent or good
Learn more about the work of our research integrity team to safeguard the quality of each article we publish.
Find out more
ORIGINAL RESEARCH article
Front. Plant Sci. , 12 June 2024
Sec. Plant Metabolism and Chemodiversity
Volume 15 - 2024 | https://doi.org/10.3389/fpls.2024.1428234
Cotton, a crucial economic crop, is also the preferred host plant of the mirid bug Apolygus lucorum. In our previous field experiments, we found that cotton cultivars Kelin 08–15 and BR-S-10 (healthy and herbivore-damaged plants) exhibit distinct attraction and repellence to A. lucorum, respectively. However, the key plant volatiles determining attraction or repulsion effects remain unknown. Here, we investigated the volatiles emitted by these two cotton cultivars before and after herbivore infestation. We found that susceptible Kelin 08–15 emitted a greater diversity and quantity of volatiles than those of BR-S-10, with herbivore-damaged cottons releasing more volatile substances. Electroantennogram (EAG) recordings further revealed that 15 representative volatiles identified above could elicited electrophysiological responses in female and male A. lucorum antennae. Among them, behavioral assays showed that two compounds, 1,3-Diethylbenzene and 4-Ethylbenzaldehyde, exhibited attractive properties, whereas six volatiles including Hexyl Acrylate, Cumene, 2,4-Dimethylstyrene, Eucalyptol, Linalool and Butyl Acrylate demonstrated repellent effects on A. lucorum. Taken together, our findings suggest the critical role of volatile compounds in mediating bug-plant interactions and provide a foundation for the development of strategies to prevent and control of A. lucorum in cotton fields.
The growth and development of cotton plants are threatened by a multitude of pests, resulting in significant yield losses and economic damages worldwide (Wu and Guo, 2005; Luttrell et al., 2015). The commercialization of Bacillus thuringiensis (Bt) transgenic cotton has led to effective control of lepidopteran insects, including cotton bollworm (Malaquias et al., 2021). However, the dramatic increase of Apolygus lucorum populations in cotton fields has resulted in substantial economic losses annually (Jiang et al., 2015). In response to the resurgence of this notorious pest, researchers have refocused their attention on developing strategies for A. lucorum management (Lu et al., 2010). As a polyphagous pest, A. lucorum can thrive on diverse hosts, exploiting its highly developed chemosensory system to switch host plants in the field, thereby causing significant damage to multiple crops, including cotton (Pan et al., 2013). The excessive use of insecticides to control A. lucorum has been associated with rapid development of insect resistance, pesticide residues, and non-target organism mortality (Zhang et al., 2020; Hua et al., 2023). Therefore, it is crucial to prioritize sustainable and environmentally friendly approaches to efficiently manage A. lucorum populations and mitigate the associated ecological and economic impacts.
Exploiting host plant resistance to insects has been reported as an eco-friendly and effective method for pest control, serving as a viable alternative to insecticides (Broekgaarden et al., 2011). Cotton varieties exhibit varying levels of resistance to insect feeding, with cotton Kelin 08–15 being a susceptible variety and cotton BR-S-10 being a resistant variety that can avoid bug feeding (Cao et al., 2012). As part of their biotic stress response, resistant plants possess diverse defense strategies to perceive and endure stress from insects or pathogens by producing a wide range of chemical constituents, including allelochemicals (Tosh et al., 2003; Dixit et al., 2020). Among these constituents, volatile secondary metabolites play a crucial role in host finding, feeding, mating, and oviposition for herbivorous insects (Dicke, 2016). For instance, a transgenic rice line expressing the terpenoid synthase gene OsTPS46 showed increased resistance to Rhopalosiphum padi due to the emission of specific volatile compounds, including lemonene, (E)-β-farnesene, and linalool (Sun et al., 2017). Similarly, a resistant Arabidopsis line with high emissions of (3E)-4,8-dimethyl-1,3,7-nonatriene (DMNT) repelled Plutella xylostella and reduced the survival rate of larvae feeding on the resistant line (Chen et al., 2021a). Herbivore-induced stress can stimulate significant changes in plant volatile production, which tend to possess insect-resistant functions (Wani et al., 2022). For example, DMNT emitted by Spodoptera littoralis-infested cotton affected its tendency choice, thereby influencing mating and oviposition behavior (Hatano et al., 2015). Similarly, Spodoptera exigua-injured corn released (Z)-3-hexenyl acetate, which triggered plant defense against herbivorous insects (Engelberth et al., 2004). However, it remains unclear whether there are differences in the volatile profiles of susceptible cotton Kelin 08–15 and resistant cotton BR-S-10 before and after herbivore-damaged stress. Are these differential volatiles related to insect resistance?
In the present study, we employed a dynamic headspace sampling system coupled with gas chromatography-mass spectrometry (GC-MS) to collect and analyze plant volatiles from resistant and susceptible cotton varieties. Moreover, the significant increase in sap-sucking insects’ population has had a profound impact on cotton yields, such as A. lucorum. Therefore, we also collected volatiles from cotton plants damaged by A. lucorum. The volatile profiles of different treatments, including resistant and susceptible cotton varieties, herbivore-damaged and undamaged cotton plants, were determined and compared. We identified differential volatiles and further investigated the electrophysiological and behavioral responses of A. lucorum adults to these volatiles using electroantennogram (EAG) recordings and Y-tube olfactometer assays, respectively. The outcomes of this study will contribute to the identification of cotton volatile compounds with potential ecological functions, which can inform the development of promising and sustainable approaches to enhance biological control strategies.
Cotton cultivars BR-S-10 (resistant) and Kelin 08–15 (susceptible) were obtained from the Xinxiang Plant Protection Scientific Observation and Experiment Station of the Chinese Academy of Agricultural Sciences, Henan Province, China (35°09′ N, 113°48′ E). The cotton plants were grown in plastic pots (15 cm in height and diameter) in a greenhouse with a temperature of 27 ± 2°C, relative humidity of 50 ± 10%, and a 16:8 light/dark cycle. Water was supplied every two days for irrigation. Cotton plants with 6~7 fully expanded leaves were used in next experiment.
Three days old adults of A. lucorum were kindly provided by the National Plant Protection Scientific Observation and Experiment Station Langfang, Hebei Province, China (39°08′ N, 116°23′ E). The laboratory colony was maintained at a temperature of 28 ± 1°C, relative humidity of 60 ± 10%, and a 14:10 h light/dark photoperiod.
To investigate the herbivore-induced plant volatiles (HIPVs) emitted from resistant cotton cultivar BR-S-10 and susceptible cotton cultivar Kelin 08–15, ten A. lucorum adults were placed on each cotton plant. After 48 hours of exposure, the bugs were carefully removed, and the cotton plants were immediately subjected to subsequent HIPVs collection. Cotton plants without herbivore damage maintained under identical conditions were served as controls. The experiment was conducted with three biological replicates for each treatment.
Plant volatiles emitted from herbivore-damaged and healthy cotton plants were collected using a headspace sampling system (Huang et al., 2015). Briefly, pots containing one A. lucorum-damaged or control plant were placed within a glass jar (30 cm in height and diameter) equipped with inlet and outlet ports. A vacuum pump was used to draw air through the jar at a flow rate of 1 L/min. The volatiles were then trapped on 50 mg of 60/80 mesh Tenax-TA (Shanghai ANPEL Scientific Instrument Company, Shanghai, China) in an 8-mm-diameter glass cartridge located at the outlet. Volatile sampling was conducted over a 4-hour period. Following collection, the volatiles were extracted with 200 μl of dichloromethane, to which n-octane (Sigma-Aldrich) was added as an internal standard for quantitative analysis. This procedure was repeated three times for each treatment.
The extracted volatile samples were then analyzed using a Shimadzu GC-MS system (GC6890-MSD5973, Agilent Technologies, CA) equipped with a HP-5MS column (30 m × 0.25 mm × 0.25 μm, Agilent Technologies, CA). The GC oven temperature was programmed as follows: initial temperature of 40°C for 1 min, increasing to 100°C at a rate of 4°C/min (held for 1 min), then to 150°C at a rate of 6°C/min (held for 1 min), and finally to 250°C at a rate of 10°C/min (held for 5 min). Peak identification was performed by comparing retention times and mass spectra with those of authentic standards analyzed under the same conditions.
Based on the GC-MS results, 15 volatile compounds were selected for EAG recordings to evaluate their effects on the antennal responses of A. lucorum adults. These compounds included Hexyl Acrylate, Cumene, 1,3-Diethylbenzene, 1,4-Diethylbenzene, 2,4-Dimethylstyrene, 4-Ethylbenzaldehyde, Naphthalene, 4-Ethylacetophenone, 2,2,4,4,6,8,8-Heptamethylnonane, P-Xylene, (1R)-(+)-α-Pinene, Camphene, Eucalyptol, Linalool, and Butyl Acrylate. In EAG recordings, standard compounds were dissolved in liquid paraffin at a concentration of 100 μg/μL. Pure liquid paraffin and methyl phenylacetate were used as blank control and reference compound, respectively. A piece of folded filter paper (5 mm × 20 mm) with 50 μL of each compound was placed into a glass Pasteur pipet. The antenna of 3-day-old adult bug was carefully removed at the base and tip, and then instantly connected to electrode holders using electrode gel. The stimulus was delivered to the antennal sample through a constant flow of clean air (activated charcoal-filtered and humidified) at a rate of 300 mL/min for 0.3 s. EAG signals were recorded and analyzed using a Syntech IDAC-2 (Intelligent Data Acquisition Controller) and EAGPro V. 2 (Syntech, Kirchzarten, Germany). Each compound was tested on 15 antennal samples.
Based on the results of volatile analysis and EAG recordings, compounds identified as eliciting strong EAG responses in A. lucorum adults were used in subsequent behavioral assays. The behavioral responses of A. lucorum to volatile standards were evaluated in a Y-tube olfactometer. The olfactometer consisted of a 30 cm main stem, two 30 cm lateral arms, and a 60° angle between arms, all made of glass. The two branch tubes were connected to separate odor-source flasks. The Y-tube was placed into a steel chamber (1 m × 0.8 m × 0.8 m) which was equipped with two 40-W fluorescent lamps providing uniform lighting (~2000 lux) as illumination sources. Each compound was individually formulated in liquid paraffin to a concentration of 100 μg/μL, and a 50 µL sample was applied to a filter paper strip (50 mm × 5 mm) before being placed inside the treatment flask. Pure liquid paraffin was served as the control. The stimuli were delivered to the olfactometer arms at a constant air flow rate of 500 mL/min. Three-day-old adult bugs were released individually at the base of the central arm. A bug was considered to have made a choice if it reached the midpoint of the lateral arm and remained there for at least 5 s. If a bug failed to make a choice within 5 min, it was recorded as no choice. Each insect was used only once. After testing five bugs, the position of the treatment and control arms were switched. The Y-tube olfactometer was replaced with a clean one after testing ten individuals. A total of 60 adult bugs were tested for each compound.
All data were analyzed using SPSS STATISTICS 18.0 software (SPSS Inc., Chicago, IL, USA). The results are presented as the mean ± standard error of the mean (SEM). To compare volatile emissions between different treatments, a one-way analysis of variance (ANOVA) was performed, followed by the least-significant difference (LSD) test. The significance level was set at P < 0.05. For EAG experiments, the relative EAG value was calculated using the following formula: EAG relative value = [(EAG value of compound - EAG value of control)/(EAG value of reference - EAG value of control)] × 100. Student’s t-test was used to compare recorded EAG values between males and females, with a significance level of P < 0.05. In the Y-tube behavioral trial, a Chi-Square test with a 50:50 distribution was performed to determine the preference of bugs between plant volatile standards and controls.
GC-MS analysis showed significant differences in the volatile profiles of BR-S-10 and Kelin 08–15. Notably, six compounds including Hexyl Acrylate, 2,4-Dimethylstyrene, 4-Ethylbenzaldehyde, m-Ethylacetophenone, 1-(4-Ethylphenyl)ethanone, and 2,2,4,4,6,8,8-Heptamethylnonane, were unique to the volatile emissions of susceptible cotton Kelin 08–15, whereas 1-Methylethylbenzene1) was exclusively released by resistant cotton BR-S-10. The quantitative analysis of each compound was further performed by comparing the peak area ratio to an internal standard (Table 1). However, the emissions of 1,3-Diethylbenzene (P = 0.0012), 1,4-Diethylbenzene (P = 0.0001), and Naphthalene (P = 0.0425) were significantly lower in resistant cotton BR-S-10 compared to susceptible cotton Kelin 08–15.
The composition and content of volatiles emitted from healthy and herbivore-damaged cotton plants also exhibited significant differences. Nine differential volatiles were identified in A. lucorum-damaged BR-S-10 plants, with five compounds showing slight up-regulation (Ethylbenzene, p-Xylene, 2-Propenoic acid butyl ester, Camphene and 1,4-Dichlorobenzene) and four compounds showing slight down-regulation (n-Butyl ether, (1R)-(+)-α-Pinene, 1,3-Diethylbenzene, and 1,4-Diethylbenzene). Two compounds including 1-Methylethylbenzene, and Eucalyptol were not detected (Table 2).
In A. lucorum-damaged cotton cultivar Kelin 08–15, p-Xylene and 2,2,4,4,6,8,8-Heptamethylnonane were not detected, whereas the emissions of (1R)-(+)-α-Pinene, 1,3-Diethylbenzene, 1,4-Diethylbenzene, 2,4-Dimethylstyrene, 4-Ethylbenzaldehyde, Naphthalene, m-Ethylacetophenone, and 1-(4-Ethylphenyl) ethanone were increased. Only 1,4-Dichlorobenzene showed decreased emission Table 3.
Fifteen selected cotton volatile compounds were used to investigate the EAG responses of A. lucorum (Table 4). All 15 compounds elicited intense EAG responses in both female and male A. lucorum adults. Notably, 2,4-Dimethylstyrene exhibited significant sexual dimorphism, with stronger EAG responses in females compared to males. No significant differences in EAG responses to the other compounds were observed between males and females (Figure 1).
Figure 1 EAG recordings of male and female A. lucorum adults to 15 plant volatile compounds. Error bars represent standard error of the mean (SEM). Significant differences between males and females are denoted by asterisks at P < 0.05.
To further investigate the behavioral preference of A. lucorum to the 15 selected compounds which could elicit EAG responses in both female and male adults, we performed Y-tube olfactometer assays. Our results indicated that two compounds, 1,3-Diethylbenzene and 4-Ethylbenzaldehyde acting as attractants could induce significant positive behavioral preference of A. lucorum. In contrast, six compounds including Hexyl Acrylate, Cumene, 2,4-Dimethylstyrene, Eucalyptol, Linalool and Butyl Acrylate showed significant repellent effects on A. lucorum. The remaining seven compounds had no obvious behavioral influence (Figure 2).
Figure 2 Behavioral responses of A. lucorum adults to 15 plant volatile compounds. The proportion of individuals choosing mineral oil (white bars) versus compound stimuli (grey bars) is presented. Asterisks indicate significant differences between test and control groups at 0.05 level.
Cotton plants is an important summer host for the mirid bug, A. lucorum. Our previous observation found that A. lucorum exhibited differential selection and adaptation to resistant cotton BR-S-10 and susceptible cotton Kelin 08–15. Plants interact with external environment such as other plants, insects and abiotic factors through releasing a diverse array of volatile compounds (Dudareva et al., 2013). For instance, silence of the linalool synthase gene OsLIS in rice plants fails to release linalool, consequently, the attractiveness of transegenic rice plants to the brown planthopper, Nilaparvata lugens, were increased (Xiao et al., 2012). To investigate the volatile compounds emitted by resistant and sensitive cotton varieties, we performed GC-MS analysis on BR-S-10 and Kelin 08–15. Here, our results revealed significant differences in the volatile profiles between the BR-S-10 and kelin 08–15. Notably, several volatile compounds including Hexyl Acrylate, 2,4-Dimethylstyrene, 4-Ethylbenzaldehyde, m-Ethylacetophenone, 1-(4-Ethylphenyl) ethanone, and 2,2,4,4,6,8,8-Heptamethylnonan were exclusively detected in susceptible cotton Kelin 08–15. Meanwhile, three volatile compounds, 1,3-Diethylbenzene, 1,4-Diethylbenzene and Naphthalene, exhibited higher emission in BR-S-10. These volatile compounds described above are also found in other plant species (Zhang et al., 2019; Koner et al., 2022; de Paula et al., 2023; Qu et al., 2023). For example, 2,4-Dimethylstyrene has been identified as an aroma compound in mango and a key odorant responsible for the chestnut-like aroma of green tea (Zhang et al., 2019; Qu et al., 2023). It is well established that cotton plants damaged by piercing-sucking hemipterans, such as A. lucorum, undergo both quantitative and qualitative changes in their volatile emissions (Williams et al., 2005; Huang et al., 2015). Similarly, the emissions of Camphene, (1R)-(+)-α-Pinene, 1,3-Diethylbenzene, Eucalyptol, and other volatiles are altered following insect damage. However, in this study, A. lucorum-damaged cotton plants emitted fewer types of volatiles than healthy cotton plants. We hypothesize that the response mechanisms of plants to stress may vary across different plant-herbivore interaction systems. Similarly, Isopropyl myristate, (E,E)-2,4-heptadienal and (E,E) 2,4-hexadienal emitted in healthy rice, and can’t emitted after being induced by rice planthopper (Chen et al., 2021b). In any case, insect-induced plant volatiles (HIPVs) widespread in plants often play crucial roles in both direct defense and indirect defense (Santos and Rao, 2000; Kessler et al., 2006; Salehi et al., 2019).
To investigate the biological roles of differential volatiles emitted from resistant and susceptible cultivars, we used 15 identified volatile compounds to assess their impacts on the electrophysiological and behavioral response of A. lucorum. EAG recordings confirmed that the 15 candidate volatile compounds could elicit strong EAG responses in A. lucorum. Additionally, Y-tube assays revealed that A. lucorum responded positively to 1,3-Diethylbenzene and 4-Ethylbenzaldehyde. Notably, the emission of 1,3-Diethylbenzene was significantly higher in susceptible cotton Kelin 08–15 than in resistant cotton BR-S-10, while 4-Ethylbenzaldehyde was exclusively released from Kelin 08–15. Interestingly, 1,3-Diethylbenzene has been reported to predominate in the volatile organic compounds of Rumex dentatus and is attractive to Galerucella placida females (Koner et al., 2022). In contrast, six compounds including 2,4-Dimethylstyrene, Eucalyptol, Linalool and so on exhibited significant repellency against A. lucorum. Eucalyptol, a component of essential oil isolated from Eucalyptus globulus, Cinnamomum longepaniculatum, Rosmarinus officinalis, and Salvia japonica, has been shown to have repellent activities against Aedes albopictus (Huang et al., 2010; Cai et al., 2021). These certain volatiles with attraction or repellence to A. lucorum may be used in developing novel pest management strategy. Moreover, resistant cotton BR-S-10 can be used as insect-resistant germplasm resources for biological breeding. These findings provide valuable insights in understanding sustainable and environmentally friendly pest management.
The raw data supporting the conclusions of this article will be made available by the authors, without undue reservation.
JW: Conceptualization, Data curation, Formal analysis, Investigation, Methodology, Writing – original draft, Writing – review & editing. YC: Investigation, Methodology, Writing – original draft. DT: Conceptualization, Investigation, Methodology, Writing – original draft, Writing – review & editing. SS: Data curation, Formal analysis, Supervision, Validation, Writing – review & editing. TG: Data curation, Formal analysis, Writing – review & editing. XH: Software, Visualization, Writing – review & editing. YZ: Conceptualization, Funding acquisition, Project administration, Supervision, Writing – review & editing.
The author(s) declare financial support was received for the research, authorship, and/or publication of this article. This work was supported by the National Natural Science Foundation of China (32372639 and 31972338), the China Agriculture Research System (CARS-02-26), the National Key Research and Development Program of China (2021YFD1400700).
The authors declare that the research was conducted in the absence of any commercial or financial relationships that could be construed as a potential conflict of interest.
All claims expressed in this article are solely those of the authors and do not necessarily represent those of their affiliated organizations, or those of the publisher, the editors and the reviewers. Any product that may be evaluated in this article, or claim that may be made by its manufacturer, is not guaranteed or endorsed by the publisher.
Broekgaarden, C., Snoeren, T. A., Dicke, M., Vosman, B. (2011). Exploiting natural variation to identify insect-resistance genes. Plant Biotechnol. J. 9, 819–825. doi: 10.1111/j.1467-7652.2011.00635.x
Cai, Z., Peng, J., Chen, Y., Tao, L., Zhang, Y., Fu, L., et al. (2021). 1,8-Cineole: a review of source, biological activities, and application. J. Asian. Nat. Prod. Res. 23, 938–954. doi: 10.1080/10286020.2020.1839432
Cao, Y., Wu, D., Zhao, Q., Guo, Y., Zhang, Y. (2012). Field evaluation of the resistance of different cotton varieties to mirid bugs. Entomological Knowledge 49, 917–922.
Chen, C., Chen, H., Huang, S., Jiang, T., Wang, C., Tao, Z., et al. (2021a). Volatile DMNT directly protects plants against Plutella xylostella by disrupting peritrophic matrix barrier in midgut. eLife 10, e63938. doi: 10.7554/eLife.63938
Chen, C., Zhang, X. L., Liu, B. L., Qin, X. Y., Guo, H., Feng, R. (2021b). Rice volatile components induced by rice planthopper. J. South. Agric. 51, 37–44. doi: 10.3969/j.issn.2095-1191.2021.01.005
de Paula, L. L., Campos, V. P., Terra, W. C., Pedroso, M. P., Barros, A. F., Pacheco, P. V. M., et al. (2023). Effect of 4-Ethylbenzaldehyde from the volatilome of Annona muricata on Meloidogyne incognita. Plant Dis. 107, 2352–2358. doi: 10.1094/PDIS-05-22-1075-RE
Dicke, M. (2016). Induced plant volatiles: plant body odours structuring ecological networks. New Phytol. 210, 10–12. doi: 10.1111/nph.13896
Dixit, G., Srivastava, A., Rai, K. M., Dubey, R. S., Srivastava, R., Verma, P. C. (2020). Distinct defensive activity of phenolics and phenylpropanoid pathway genes in different cotton varieties toward chewing pests. Plant Signal Behav. 15, 1747689. doi: 10.1080/15592324.2020.1747689
Dudareva, N., Klempien, A., Muhlemann, J. K., Kaplan, I. (2013). Biosynthesis, function and metabolic engineering of plant volatile organic compounds. New Phytol. 198, 16–32. doi: 10.1111/nph.12145
Engelberth, J., Alborn, H. T., Schmelz, E. A., Tumlinson, J. H. (2004). Airborne signals prime plants against insect herbivore attack. Proc. Natl. Acad. Sci. U. S. A. 101, 1781–1785. doi: 10.1073/pnas.0308037100
Hatano, E., Saveer, A. M., Borrero-Echeverry, F., Strauch, M., Zakir, A., Bengtsson, M., et al. (2015). A herbivore-induced plant volatile interferes with host plant and mate location in moths through suppression of olfactory signalling pathways. BMC Biol. 13, 75. doi: 10.1186/s12915-015-0188-3
Hua, L., Zhao, D., Wang, H., Wei, T. (2023). Residues and bioavailability of neonicotinoid pesticide in shaanxi agricultural soil. Water Air Soil pollut. 234, 129. doi: 10.1007/s11270-023-06159-1
Huang, X., Chen, J., Xiao, H., Xiao, Y., Wu, J., Wu, J., et al. (2015). Dynamic transcriptome analysis and volatile profiling of Gossypium hirsutum in response to the cotton bollworm Helicoverpa armigera. Sci. Rep. 5, 11867. doi: 10.1038/srep11867
Huang, C., Xia, L., Yang, X., He, Y., Xia, H., Huang, H. (2010). Gas chromatography and Aedes albopictus repellent activity of eucalyptus oil patch. Chin. J. Hyg. Insect Equip. 27, 210–212. doi: 10.19821/j.1671-2781.2021.03.005
Jiang, Y., Lu, Y., Zeng, J. (2015). Forecast and management of mirid bugs in multiple agroecosystems of China. China Agric. Press. 12–15.
Kessler, A., Halitschke, R., Diezel, C., Baldwin, I. T. (2006). Priming of plant defense responses in nature by airborne signaling between Artemisia tridentata and Nicotiana attenuate. Oecologia. 148, 280–292. doi: 10.1007/s00442-006-0365-8
Koner, A., Das, S., Karmakar, A., Barik, A. (2022). Attraction of the biocontrol agent, galerucella placida towards volatile blends of two polygonaceae weeds, rumex dentatus and polygonum glabrum. J. Chem. Ecol. 48, 165–178. doi: 10.1007/s10886-021-01332-4
Luttrell, R. G., Teague, T. G., Brewer, M. J. (2015). ““Cotton insect pest management,”,” in the Cotton Production, vol. 85–107 . Eds. Khawar, D. J., Bhagirath, S. C. (John Wiley & Sons Ltd) 85–107.
Malaquias, J. B., Santana, D. R. S., Degrande, P. E., Ferreira, C. P., de Melo, E. P., Godoy, W. A. C., et al. (2021). Shifts in ecological dominance between two lepidopteran species in refuge areas of Bt cotton. Insects. 12, 157. doi: 10.3390/insects12020157
Pan, H., Lu, Y., Wyckhuys, K. A., Wu, K. (2013). Preference of a polyphagous mirid bug, Apolygus lucorum (Meyer-Dür) for flowering host plants. PloS One 8, e68980. doi: 10.1371/journal.pone.0068980
Qu, F., Li, X., Wang, P., Han, Y., Wu, Y., Hu, J., et al. (2023). Effect of thermal process on the key aroma components of green tea with chestnut-like aroma. J. Sci. Food Agric. 103, 657–665. doi: 10.1002/jsfa.12177
Salehi, B., Upadhyay, S., Erdogan Orhan, I., Kumar Jugran A., L. D., Jayaweera, S., A. Dias, D., et al. (2019). Therapeutic potential of α- and β-pinene: a miracle gift of nature. Biomolecules. 9, 738. doi: 10.3390/biom9110738
Santos, F. A., Rao, V. S. (2000). Antiinflammatory and antinociceptive effects of 1,8-cineole a terpenoid oxide present in many plant essential oils. Phytother. Res. 14, 240–244. doi: 10.1002/(ISSN)1099-1573
Sun, Y., Huang, X. Z., Ning, Y. S., Jing, W. X., Bruce, T. J. A., Qi, F. J., et al. (2017). TPS46, a rice terpene synthase conferring natural resistance to bird cherry-oat aphid, Rhopalosiphum padi (Linnaeus). Front. Plant Sci. 8, 110. doi: 10.3389/fpls.2017.00110
Tosh, C. R., Powell, G., Hardie, J. (2003). Decision making by generalist and specialist aphids with the same genotype. J. Insect Physiol. 49, 659–669. doi: 10.1016/S0022-1910(03)00066-0
Wani, S. H., Choudhary, M., Barmukh, R., Bagaria, P. K., Samantara, K., Razzaq, A., et al. (2022). Molecular mechanisms, genetic mapping, and genome editing for insect pest resistance in field crops. Theor. Appl. Genet. 135, 3875–3895. doi: 10.1007/s00122-022-04060-9
Williams, L., 3rd, Rodriguez-Saona, C., Paré, P. W., Crafts-Brandner, S. J. (2005). The piercing-sucking herbivores Lygus hesperus and Nezara viridula induce volatile emissions in plants. Arch. Insect Biochem. Physiol. 58, 84–96. doi: 10.1002/arch.20035
Wu, K. M., Guo, Y. Y. (2005). The evolution of cotton pest management practices in China. Annu. Rev. Entomol. 50, 31–52. doi: 10.1146/annurev.ento.50.071803.130349
Xiao, Y., Wang, Q., Erb, M., Turlings, T. C., Ge, L., Hu, L., et al. (2012). Specific herbivore-induced volatiles defend plants and determine insect community composition in the field. Ecol. Lett. 15, 1130–1139. doi: 10.1111/j.1461-0248.2012.01835.x
Zhang, H., Chen, A., Shan, T., Dong, W., Shi, X., Gao, X. (2020). Cross-resistance and fitness cost analysis of resistance to thiamethoxam in melon and cotton Aphid (Hemiptera: Aphididae). J. Econ. Entomol. 113, 1946–1954. doi: 10.1093/jee/toaa090
Keywords: cotton, mirid bug, plant volatiles, electrophysiological responses, behavioral preference
Citation: Wu J, Cao Y, Teng D, Shan S, Geng T, Huang X and Zhang Y (2024) Volatiles of different resistant cotton varieties mediate the host preference of Mirid bug Apolygus lucorum. Front. Plant Sci. 15:1428234. doi: 10.3389/fpls.2024.1428234
Received: 06 May 2024; Accepted: 31 May 2024;
Published: 12 June 2024.
Edited by:
Mariam Gaid, Independent researcher, Braunschweig, GermanyReviewed by:
Xingyuan Men, Shandong Academy of Agricultural Sciences, ChinaCopyright © 2024 Wu, Cao, Teng, Shan, Geng, Huang and Zhang. This is an open-access article distributed under the terms of the Creative Commons Attribution License (CC BY). The use, distribution or reproduction in other forums is permitted, provided the original author(s) and the copyright owner(s) are credited and that the original publication in this journal is cited, in accordance with accepted academic practice. No use, distribution or reproduction is permitted which does not comply with these terms.
*Correspondence: Yongjun Zhang, eWp6aGFuZ0BpcHBjYWFzLmNu; emhhbmd5b25nanVuQGNhYXMuY24=
†These authors have contributed equally to this work
Disclaimer: All claims expressed in this article are solely those of the authors and do not necessarily represent those of their affiliated organizations, or those of the publisher, the editors and the reviewers. Any product that may be evaluated in this article or claim that may be made by its manufacturer is not guaranteed or endorsed by the publisher.
Research integrity at Frontiers
Learn more about the work of our research integrity team to safeguard the quality of each article we publish.