- Research Center on Plant Health Modelling (PHeM), Department of Sustainable Crop Production (DI.PRO.VE.S.), Università Cattolica del Sacro Cuore, Piacenza, Italy
Sour rot (SR) is one of the major diseases affecting grapevine berries, causing severe yield losses and deterioration of wine quality. SR is caused by an etiologic complex of microorganisms, including yeasts, bacteria, and filamentous fungi. This systematic review focuses on the etiology, epidemiology, and control of SR. A total of 74 papers published between 1986 and 2023 were assessed in this review. Description of disease symptoms was quite consistent across the papers, including oxidation of the grape skin, disaggregation of the internal tissues, and detachment of the rotten berries from the pedicel. The affected bunches are characterized by the smell of acetic acid and ethyl acetate that attracts fruit flies (Drosophila spp.). However, several knowledge gaps and/or inconsistencies were identified with respect to SR etiology, epidemiology, and control. Overall, 146 microorganisms were isolated from the affected berries (44.5% yeasts, 34.3% bacteria, and 21.2% filamentous fungi); however, the selected papers could not definitively clarify which species are primarily involved in the etiology of the disease. A general inconsistency was also observed in the methods used to assess the incidence and severity of SR in vineyards, making inter-study comparisons extremely challenging. Inconsistencies were also found in the methods used for pathogenicity assessment in artificial inoculation studies. Furthermore, gaps were detected in terms of SR epidemiology, with a focus on environmental conditions affecting the disease development. The SR management options are limited, and efficacy trials often result in poor, variable, and inconsistent levels of control, which might be attributed to the lack of knowledge on disease epidemiology. These knowledge gaps and inconsistencies were analyzed in this review to inform future research activities.
1 Introduction
Sour rot (SR) is one of the late-season, non-Botrytis rots affecting grapevines (Hewitt, 1974; Stapleton and Grant, 1992; Duncan et al., 1995). These rots include Alternaria rot (caused by Alternaria spp.), Aspergillus rot (or black sooty mold, caused by black Aspergilla and particularly by Aspergillus carbonarius, a toxigenic species producing Ochratoxin A), bitter rot (caused by Greeneria uvicola), and others caused by Penicillium, Cladosporium, and Rhizopus spp (Kassemeyer and Berkelmann-Löhnertz, 2009; Loureiro et al., 2012; Steel et al., 2013; Barata, 2011; Hewstone et al., 2007).
Previously, SR was regarded as the final stage of Botrytis bunch rot (BBR) (Bisiach et al., 1986). However, today, it is considered a separate disease (Rooney-Latham et al., 2008; McFadden-Smith et al., 2015; Haviland et al., 2017). Hall et al. (2018a), while investigating the potential competition between the development of BBR and SR on grape clusters, showed that the progression of Botrytis infection and the rate of its colonization halted in the presence of SR.
The etiology and epidemiology of SR are not completely understood (Guerzoni and Marchetti, 1987; Nigro et al., 2006). In the last two decades, however, the disease has gained attention due to its potential to reduce crop yield and wine quality (Nigro et al., 2006; Oriolani et al., 2009; Huber et al., 2011; Barata et al., 2011a, b; Wei et al., 2015). SR is caused by a complex of microorganisms, including yeasts, bacteria, and filamentous fungi (Barata, 2011; Hewstone et al., 2007; Steel et al., 2013). The role of each of these microorganisms in the development of the disease is unclear, making its management challenging and often ineffective (Hall et al., 2018a).
The current systematic review aims at performing a comprehensive analysis of SR, focusing on its etiology, epidemiology, and control. It also intends to identify the knowledge gaps in the research to date to inform future studies.
2 Materials and methods
A systematic literature review is a scientific method to identify available literature on a particular topic, which is robust, rigorous, objective, and transparent (Randall and James, 2012). A systematic literature search was undertaken on October 17, 2023, using the following three relevant bibliographic databases: i) Scopus (https://www.scopus.com/); ii) Web of Science Core Collection (http://webofknowledge.com/WOS); and iii) Google Scholar (https://scholar.google.it/).
Searches were conducted in English, and the search terms “sour rot,” “grape,” and “vitis vinifera” were combined into search strings using wildcards and connectors. The wildcard (*) enables the search to detect multiple word endings. For example, “grape*” would detect grape, grapes, and grapevine. Search terms were combined using the operators AND (both terms must be present somewhere in the search field) and OR (at least one of the terms must be present in the search field). Specific queries were formulated to search academic papers, reviews, papers in press, conference papers, and Ph.D. dissertations. The search was restricted to titles, abstracts, and keywords in Scopus and Web of Science, and titles in Google Scholar.
The following search strings were used: Scopus: (TITLE-ABS-KEY ((“sour rot”) AND (grape* OR “vitis vinifera”))) – 79 hits; Web of Science: (TS= ((“sour rot”) AND (grape* OR “vitis vinifera”))) – 121 hits; Google Scholar: (allintitle: “sour rot” grape OR “vitis vinifera”) – 32 hits.
The papers identified through the search were merged, and duplicates were removed to obtain a refined list comprising 135 papers (Figure 1). These papers were then screened based on the following criteria: i) The name of the disease appears in the title, abstract, and keywords; ii) The disease affects vitis vinifera; and iii) The paper concerns the epidemiology, etiology, or control of SR. Abstracts of these papers were read using the RefWorks software (ProQuest, MI, USA), and papers not fulfilling the inclusion criteria were discarded. Through this approach, 70 papers were shortlisted. After examining the bibliography of the shortlisted papers, we identified and included four more papers to the list of shortlisted papers.
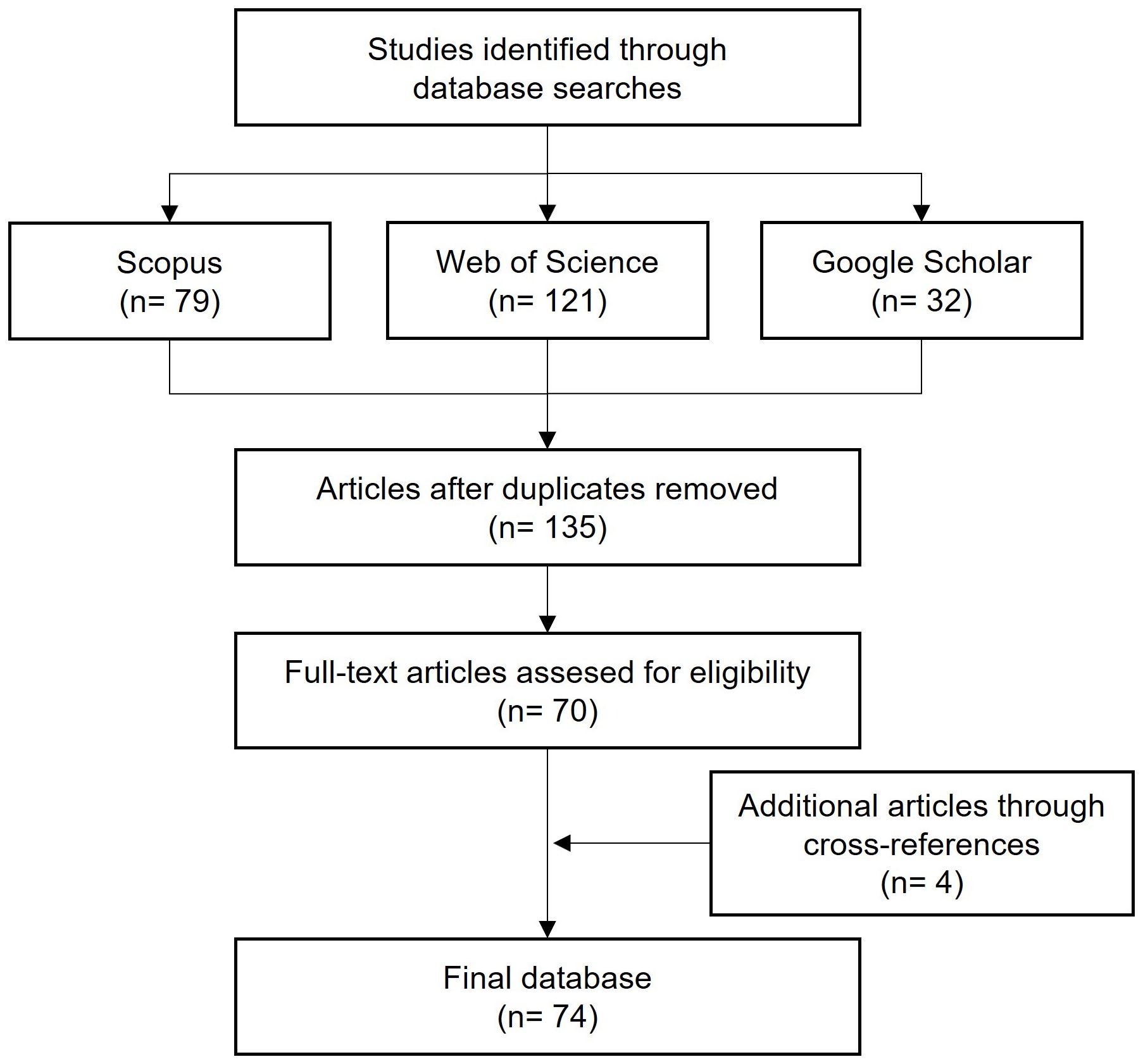
Figure 1 Schematic representation of the systematic review process [based on Biesbroek et al. (2013) and Mckenzie and Joy (2020)].
Next, we extracted relevant data from these 74 papers based on a structured scheme comprising the following items: (i) Bibliographic information: Author(s), title, and year of publication; (ii) Location of the study (country); (iii) Grapevine varieties included in the study; iv) SR symptoms and methods for disease assessment (e.g., visual observations or chemical analysis); (v) Microorganisms associated with SR in vineyards (i.e., microorganisms isolated from symptomatic berries in field studies); vi) Microorganisms used for artificial inoculation studies; vii) Epidemiological information (e.g., vectors, environmental conditions, etc.); and vi) SR management approaches adopted and their efficacy.
3 Results
3.1 Bibliographic information
Figure 2 shows the number of shortlisted papers published per year. The earliest paper was published in 1986 by Bisiach et al. (1986). From 1995 to 2007, only one or two papers were published each year. Thereafter, the number of publications increased each year. The highest number of publications per year (n = 6) were obtained from 2017 and 2020. The trend shows the increasing interest in SR in the last two decades.
3.2 Location of the studies
Figure 3 shows the worldwide distribution of the papers. The papers spread across a total of 16 countries. The highest number of papers were conducted in the US (n = 24), followed by Nord and South Italy (n = 13), Canada (n = 7), China (n = 4), Portugal (n = 4), Spain (n = 4), and South America (n = 3). Eight papers were conducted in France, Greece, Australia, and Israel (each n = 2). Eight papers were conducted in Germany, Poland, and South Africa (each n = 1). Furthermore, more papers were conducted in the northern than in the southern hemisphere (n = 65 vs. 9, respectively).
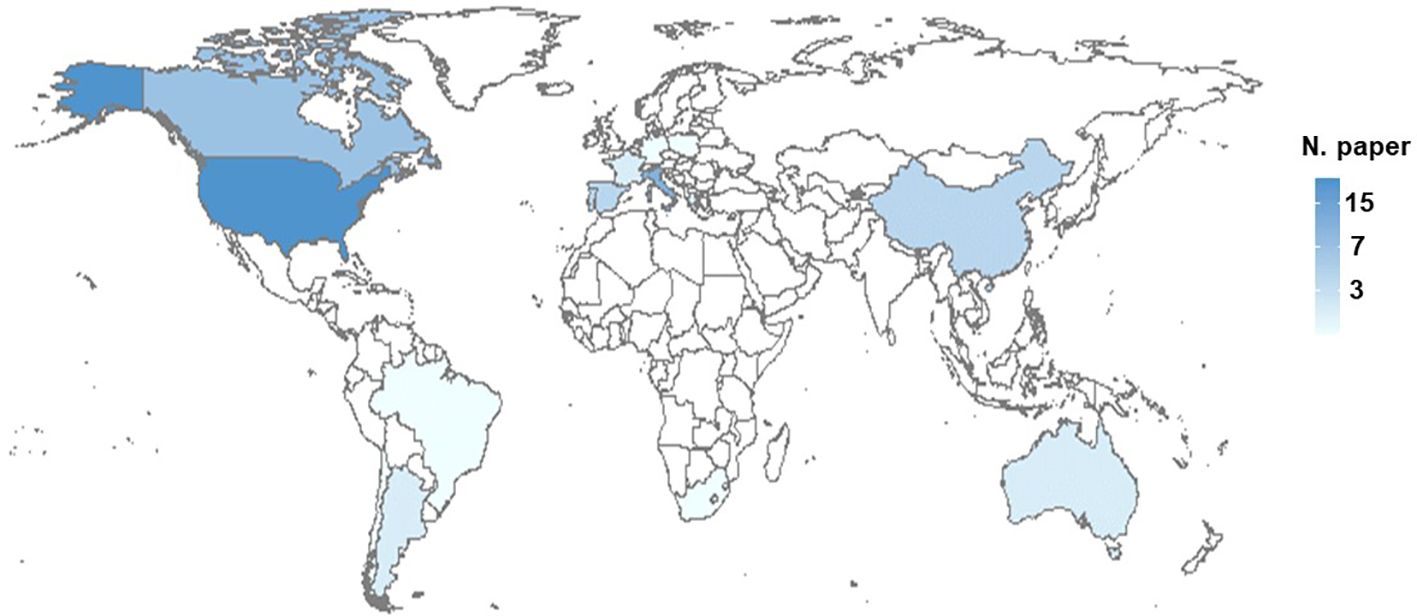
Figure 3 Distribution of sour rot studies worldwide. The total number of papers per country is shown as a color gradient, from white (no papers) to dark blue (high number of papers).
3.3 Grapevine varieties included in the studies
The selected papers mentioned a total of 118 grape varieties, with 59 white (n = 22 table grape; n = 37 wine grape) and 59 red grape varieties (n = 19 table grape; n = 40 wine grape) (Table 1). The most frequent cultivars were Sauvignon Blanc and Vignoles (n = 9), followed by Riesling, Pinot noir, Red Globe, Thompson Seedless (n = 7), and Chardonnay (n = 6). It is not clear whether these cultivars were included in the studies due to their relevance in the study area or their level of susceptibility to SR. However, it is commonly accepted that late-ripening cultivars with thin-skinned and tightly packed clusters (e.g., Vignoles, Riesling, Pinot Noir, Pinot Gris, Seyval, etc.) are particularly susceptible to rots close to harvest (Barata et al., 2012a; McFadden-Smith et al., 2015; Bordelon, 2016) because they are prone to berry splitting, and microclimatic conditions within the cluster are more conducive to SR (Steel et al., 2016; Lisek and Lisek, 2021; Cornelissen et al., 2023). Fidelibus et al. (2006, 2007, and 2009) evaluated different varieties of Chardonnay, Merlot, and Barbera and observed that the varieties with larger berries were more susceptible to SR, as large berries often contribute to the development of tight clusters. Lisek and Lisek (2021) assessed the susceptibility to SR of 28 wine and 25 table grape cultivars with diverse geographic and genetic origins. These cultivars were characterized by a significant variation in the density of bunches (loose to very dense), skin thickness (thin to thick), and the time of veraison. They also showed that wine cultivars such as Riesling, Pinot Noir, and Seyval had the highest susceptibility to SR due to their dense clusters and thin skin. Among table grape cultivars, Rusven, Piesnia, Krasotka, Galbena Nou, and Argo, which originated from Russia and Ukraine, exhibited the highest susceptibility to SR (Lisek and Lisek, 2021).
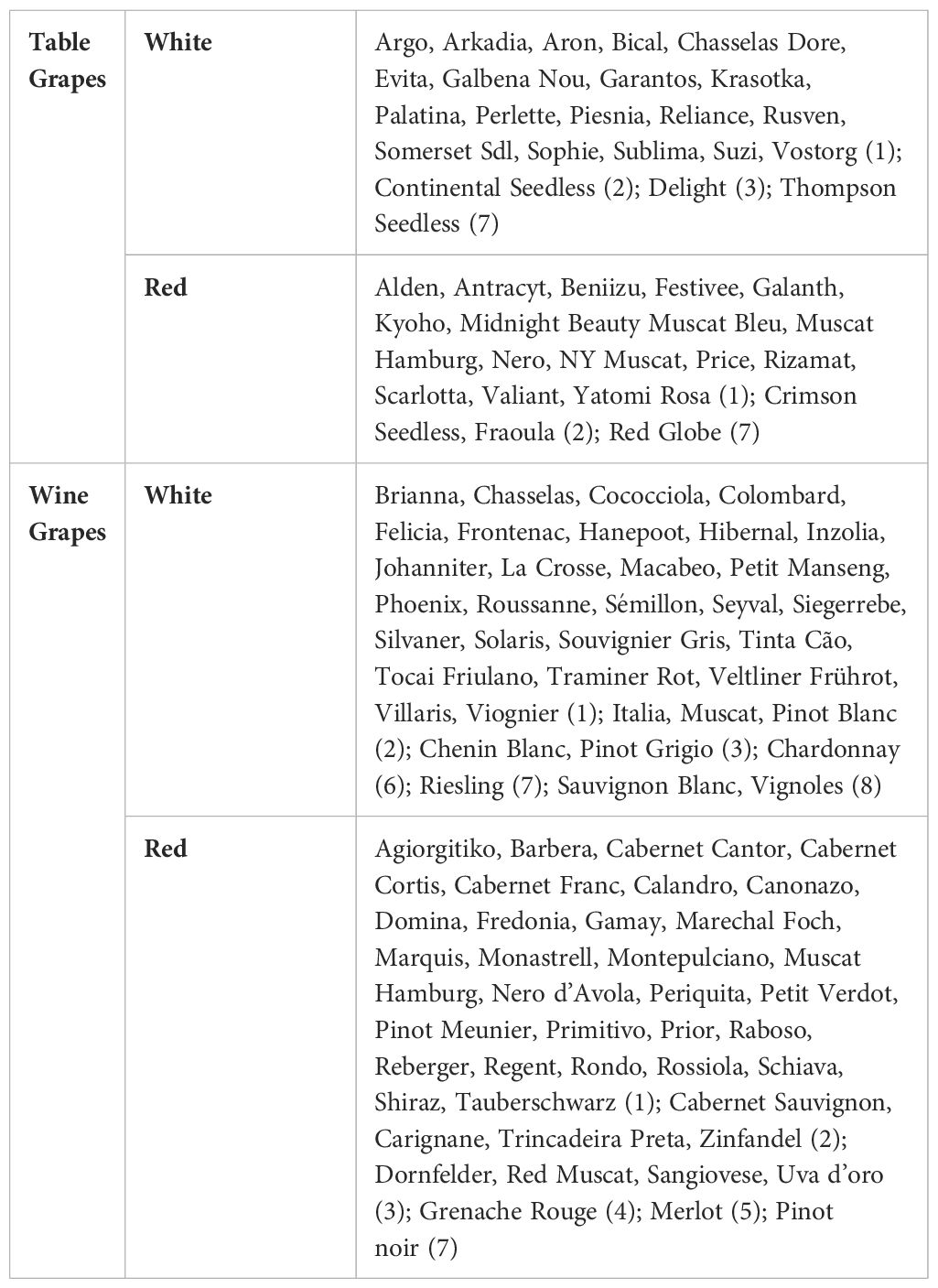
Table 1 Grape varieties discussed in the selected papers, with the numbers in parentheses depicting the numbers of cultivars.
3.4 SR symptoms and assessment methods
The description of SR symptoms was consistent across all the selected papers. The symptoms usually start from an injury site or where the berry attaches to the pedicel. In both red and white grape varieties, the skin of the affected berries exhibits oxidation, turning brown (Gravot et al., 2001; Hall et al., 2018b), cracking and becoming extremely fragile. Then, the internal berry tissue disaggregates, softens, and exudes from the skin cracks. In addition, rotten berries might detach from the pedicel (Bisiach et al., 1986; Guerzoni and Marchetti, 1987). The name “sour rot” originates from the strong and pungent smell emanating from the rotten berries as a result of the production of several chemical compounds, such as acetic acid, glycerol, ethyl acetate, ethanol, galacturonic acid, acetaldehyde, and gluconic acid. These compounds form during the fermentation of the berry pulp sugars, resulting in the production of ethanol (mainly by yeasts) that is oxidized into acetic acid (mainly by bacteria) (Pinto et al., 2019), or can be directly produced by SR-related microbes (Marchetti et al., 1984; Zoecklein et al., 2000). Yeasts producing ethanol did not seem to induce SR alone and required the activity of bacteria that produced high levels of acetic and gluconic acid and initiated berry fermentation (Barata et al., 2012b).
Among the selected papers, 28 papers discussed an assessment of the SR symptoms. In the majority of these papers (n = 22), the disease was assessed by visual observations in terms of disease incidence or severity. Disease incidence was expressed as the percentage of clusters showing SR symptoms (Duncan et al., 1995; Tjamos et al., 2004; Dimakopoulou et al., 2008; Calvo-Garrido et al., 2013; Calzarano et al., 2019, 2020; Carbó et al., 2019; Vogel et al., 2020; Kenney and Hall, 2021; Owoyemi et al., 2022), as the proportion of clusters having four or more adjoining berries with SR symptoms (Fidelibus et al., 2005, 2006, 2007, 2009), or as the percentage of affected berries over the total number of detached berries (Gao et al., 2020). Disease severity was expressed as the percentage of affected berries (i.e., berries with SR symptoms) over the total berries in a cluster (Tjamos et al., 2004; Calvo-Garrido et al., 2013; Calzarano et al., 2019, 2020; VanderWeide et al., 2020; Vogel et al., 2020; Kenney and Hall, 2021; Carbó et al., 2019), as the percentage of the cluster surface with SR symptoms (Tjamos et al., 2004; Hall et al., 2018b; Vogel et al., 2020; Lisek and Lisek, 2021; Cornelissen et al., 2022), or as an empirical rating (Schena et al., 2005; Nigro et al., 2006; Huber, 2016; Madden et al., 2017; Pinto et al., 2017; Hall et al., 2018b; Calderone et al., 2022).
However, the scales for rating disease severity were not uniform. Schena et al. (2005) used the following scale: 0 = bunch without rots and 1 = 1–5%, 2 = 6–10%, 3 = 11–25%, and 4 = >25% of rotted berries in a cluster. Huber (2016) used the following scale: 0 = 0%, 1 = <10%, 2 = 10–25%, 3 = 25–75%, and 4 = >75% infected tissue. Calderone et al. (2022) used the following scale: 0 = 0%, 1 = 1–25%, 2 = 25.1–50%, 3 = 50.1–75%, and 4 = 75.1–100% of affected berries in a cluster. Nigro et al. (2006) used an empirical scale as follows: 0 = bunch without rots and 1 = 1–5%, 2 = 6–10%, 3 = 11–25%, 4 = 26–50%, 5 = 51–75%, 6 = >76% of rotted berries. Different from other studies, Hall et al. (2018a) rated disease severity through the following scale: 0 = berry is symptomless and completely intact; 1 = berry is completely intact, with some discoloration of the skin only around the wound site; 2 = berry is entirely intact but with obvious discoloration of the skin extending beyond the wound site; 3 = majority of the berry skin is discolored but berry is still intact; and 4 = berry is no longer intact, the inner pulp is liquefied and leaking, and the skin is completely discolored (characteristic SR symptoms). Pinto et al. (2017) evaluated disease severity using an empirical scale with five degrees: 0 = sound berries and 1 = 1–10%, 2 = 11–20%, 3 = 21–50%, and 3 = >50% spoilage of berry surface. Madden et al. (2017) used a qualitative scale based on the images of the affected berries to assess the severity of berry decomposition. The lack of uniformity in the methods for disease severity assessment makes the inter-study comparison difficult, warranting a need for a common standard scale.
In nine papers, the disease was assessed indirectly, based on the changes in the chemical composition and properties of the juice of affected berries in comparison to the juice of healthy ones, including volatile acidity, pH, and levels of acetic acid, ethanol, glycerol, and gluconic acid (Zoecklein et al., 2000; Barata et al., 2012b; Mateo et al., 2014; Hall et al., 2015; Madden et al., 2017; Pinto et al., 2017; Hall et al., 2018a; Pinto et al., 2019; Cornelissen et al., 2022). Glycerol, acetic acid, and gluconic acid levels are considered SR markers (Barata et al., 2012b; Cornelissen et al., 2022). The most commonly used technique for chemical analysis was high-performance liquid chromatography (HPLC) (Zoecklein et al., 2000; Hall et al., 2015; Hall et al., 2018a; Mateo et al., 2014; Pinto et al., 2017, 2019) using a column packed with hydrogen sulfonated divinyl benzene-styrene (Castellari et al., 2000). The advantages of this method include speed, simplicity, accuracy, precision, and sensitivity (Kupina, 1984). Some authors also used respective commercial assay kits (Barata et al., 2012b; Madden et al., 2017; Cornelissen et al., 2022). Notably, the results of visual rating were not consistent with those of chemical analysis (Madden et al., 2017; Hall et al., 2018b). According to Madden et al. (2017), this discrepancy might be attributed to SR-associated filamentous fungi that metabolize organic acids in affected berries. Hall et al. (2018b) observed that the relationship between SR symptoms and acetic acid levels in berries depends on the combination of yeasts and acetic acid bacteria (AAB) they harbor. Hence, it is difficult to compare the results of studies that assessed disease severity solely on the basis of symptoms or the chemical composition of berries.
3.5 SR-associated microbes in vineyards
The surface of grape berries harbors a complex microbial community comprising filamentous fungi, yeasts, and bacteria (Barata et al., 2012c; Rousseaux et al., 2014). Among the selected papers, 22 papers focused on isolating and identifying the microbes present on the berry surface and 10 of these papers compared the surface microbial diversity between SR-affected and -unaffected berries.
In these studies, the microorganisms were isolated by plating a suspension obtained by washing the berry surface or berry juice collected in the vineyards onto the following artificial media: i) General yeast peptone (GYP) agar (Barata et al., 2011b) for yeasts; ii) selective/differential media for specific microorganisms (Schuller et al., 2000); iii) glucose yeast extract (GY) and De Man, Rogosa, and Sharpe (MRS) agar (De Man et al., 1960) for enumeration of AAB and lactic acid bacteria (LAB), respectively; and iv) Czapeck-Agar medium for fungi. After isolation, the microorganisms were identified based on the morphology of colonies and/or the sequencing of standard rDNA regions, i.e., the 5.8S-internal transcribed spacer (ITS) for yeast and fungi, and the 16s ribosomal RNA (rRNA) for bacteria.
Overall, 146 microorganisms were isolated from the affected berries, with 44.5% yeasts, 34.3% bacteria, and 21.2% filamentous fungi (Figure 4). The most frequently isolated genera were Pichia (29.0%), Candida (21.3%), and Hanseniaspora (17.4%) among yeasts (Figure 5A); Gluconobacter (24.1%), Acetobacter (22.9%), and Bacillus (12.0%) (Figure 5B) among bacteria; and Aspergillus (31.7%) and Aureobasidium (17.1%) among filamentous fungi (Figure 5C).
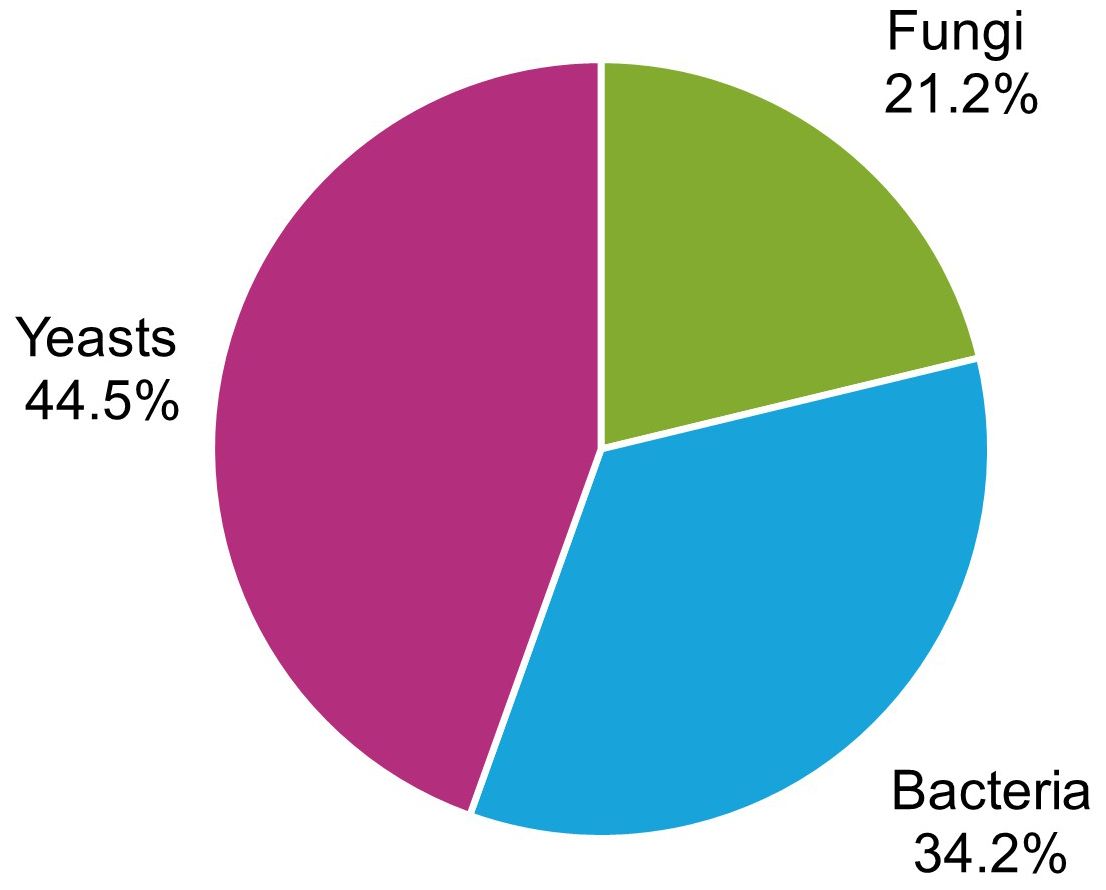
Figure 4 Frequency of the microorganisms isolated from grape bunches affected by sour rot, grouped as yeasts, bacteria, and filamentous fungi.
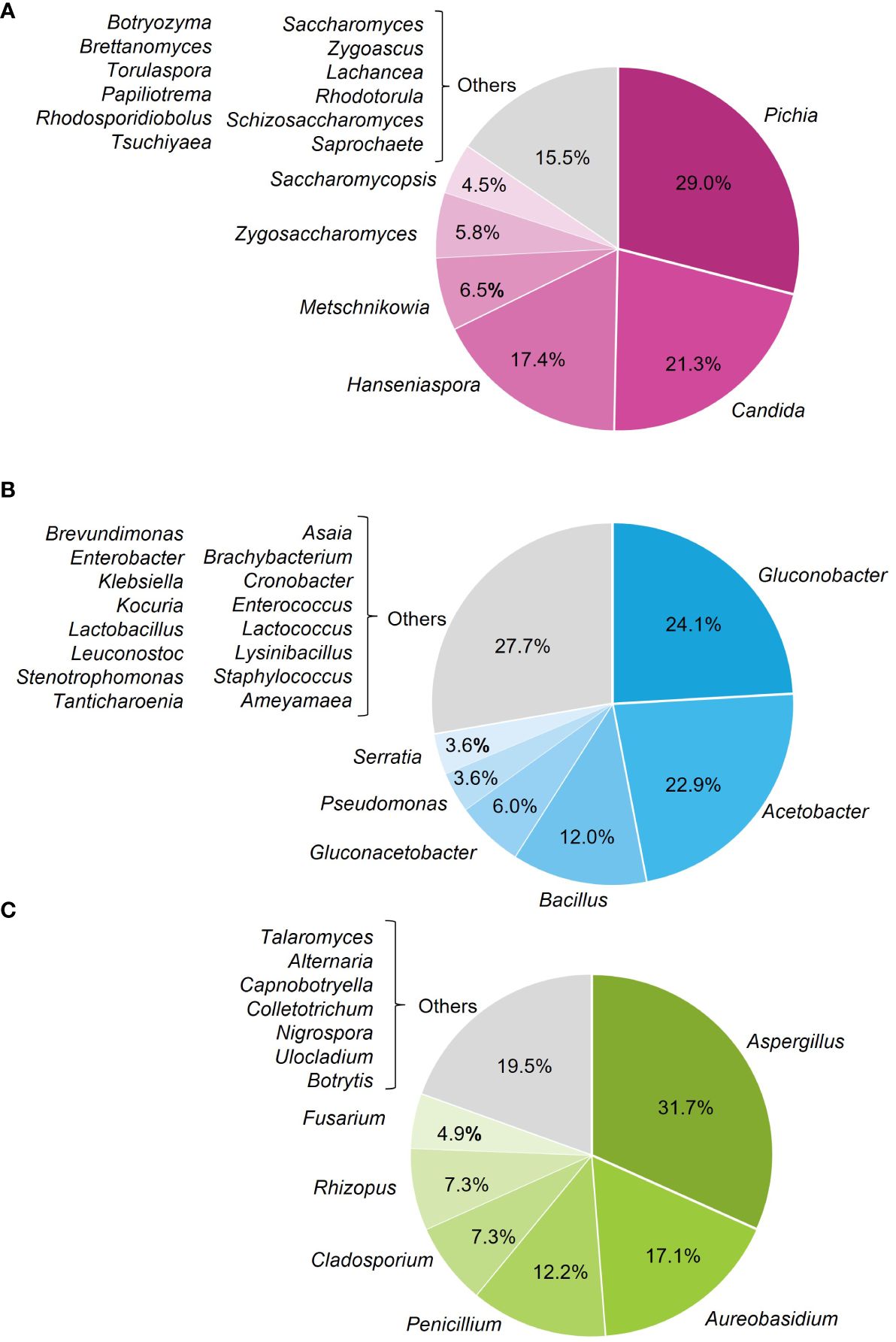
Figure 5 Frequency of the microorganisms isolated from grape bunches affected by sour rot, based on genera of yeasts (A), bacteria (B), and filamentous fungi (C).
While AAB were commonly associated with SR (Barata et al., 2012a, b), there is some debate about some yeast species. For example, Barata et al. (2012b) showed that some yeast species (i.e., Pichia terricola, Hanseniaspora uvarum, and Candida zemplinina) were not able to produce the metabolites characteristic of SR (gluconic and acetic acids) in inoculated berries. In contrast, Huber (2016) showed that H. uvarum and C. zemplinina contributed to the development of SR symptoms, in accordance with other literature (Gravot et al., 2001).
Some authors reported the presence of Penicillium, Cladosporium, Alternaria, and Rhizopus members in SR-affected berries (Bisiach et al., 1986; Hewstone et al., 2007). However, Bisiach et al. (1986) and Oriolani et al. (2007) stated that these fungi were not capable of initiating an SR infection and considered these fungi as endophytes, secondary invaders, or producing quiescent infection in berries (Duncan et al., 1995; Mostert et al., 2000; Dugan et al., 2002; Barata et al., 2008; González and Tello, 2011; Steel et al., 2013). In contrast, Latorre et al. (2002) reported that Aspergillus spp., Penicillium spp., Rhizopus spp., and Cladosporium spp. were primarily responsible for SR in Chile, with yeasts and bacteria being secondary invaders. In addition, Rooney-Latham et al. (2008) found that A. niger and A. carbonarius were the first microbial species to colonize the wounded berries and initiate their rot. Following Wilcox et al. (2015), these fungi and namely Aspergilla, do not cause true SR even though AAB can produce the vinegar smell in rotten berries; it would be desirable to have a common disease definition of the (true) SR, as a disease characterized by the rapid decomposition of ripening grape berries which exude the vinegarlike odor of acetic acid and ethyl acetate, which is caused by yeasts and AAB.
When the microorganisms from SR affected and un-affected berries were compared in the same vineyard, there were 69 microorganisms isolated from un-affected berries and 128 from affected ones. Among the filamentous fungi, no significant difference was observed in the frequencies of Aureobasidium and Rhizopus, while Aspergillus, Cladosporium, and Taloromyces were more frequently isolated from the affected berries (Figure 6A). Among the bacteria, Gluconobacter, Acetobacter, Gluconoacetobacter, Bacillus, and Pseudomonas were more prevalent in the affected berries (Figure 6B). Among the yeasts, Saccharomyces was frequently isolated from both unaffected and affected berries, while Pichia and Candida were more prevalent in the affected berries (Figure 6C). These comparisons revealed that some microbes were more prevalent in the affected berries. However, the metadata from these studies did not allow a statistical comparison between the affected and unaffected berries. Thus, these studies could not determine which species are primarily involved in SR etiology.
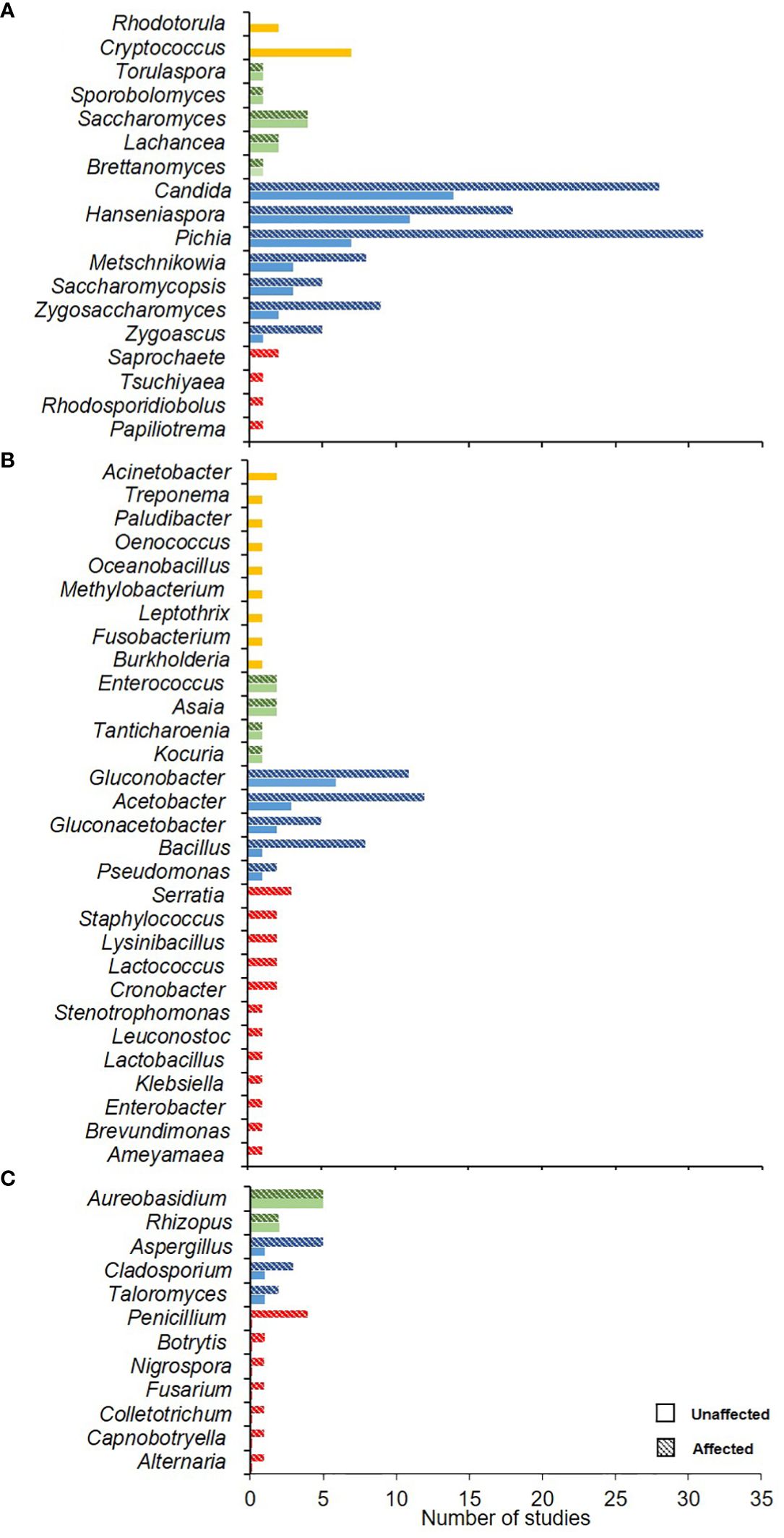
Figure 6 Number of studies wherein each microorganism (yeasts, A; bacteria, B; and filamentous fungi, C) was isolated from grape bunches unaffected and affected by sour rot. Yellow and red colors represent the microorganisms found only on unaffected and affected bunches, respectively. Green and blue colors represent the genera found in unaffected (full bars) and affected (dotted bars) bunches, in equal or different numbers, respectively.
The abundance and diversity of microbial species found in the different studies vary based on grape varieties, grape ripening stages, environmental conditions, soil types, cultivation practices, and the general grape health status (Barnett et al., 1972; Davenport, 1974; Rosini et al., 1982; Longo et al., 1991; Yanagida et al., 1992; Schütz and Gafner, 1994; Martini et al., 1996; De La Torre et al., 1999; Guerra et al., 1999; Mortimer and Polsinelli, 1999; Pretorius et al., 1999; Fleet et al., 2002; Combina et al., 2005; Valero et al., 2005; Raspor et al., 2006; Nisiotou and Nychas, 2007; Valero et al., 2007; Chavan et al., 2009; Li et al., 2010; Rousseaux et al., 2014). These factors significantly influence the composition of the grape microbiome (Raspor et al., 2006) and, therefore, the prevalence of some species over others. For example, Aureobasidium pullulans was isolated more frequently from red than white grapevine cultivars (Raspor et al., 2006). Moreover, a greater yeast population was found in Merlot than in Cabernet Sauvignon berries (Renouf et al., 2005). In China, the yeast population density and diversity of three grape varieties varied across different vine-growing regions (Li et al., 2010), with H. uvarum being rarely found in regions with cool and dry climates.
The availability of nutrients in grape berries may also influence the composition and size of epiphytic microbial populations (Shiraishi et al., 2010). The presence of (micro)cracks or wounds on the berry skin increases by about 100 times the content of sugars, organic acids, and amino acids on the surface of berries in comparison to intact berries, enabling a massive growth of opportunistic microorganisms (Fonseca and Inácio, 2006; Loureiro et al., 2012). In addition, the interactions between the microorganisms present on the surface of affected berries can influence the composition of the microflora. For instance, the presence of acetic acid due to AAB can inhibit the growth of the yeast population and slow down the accumulation of ethanol deriving from soluble sugar metabolism (Pinto et al., 2019).
3.6 Artificial inoculation studies
In eight of the selected papers, unaffected and intact berries from the vineyard were artificially inoculated with single or multiple microorganisms. The berries were first rinsed in water, then surface disinfected, washed again to remove disinfectants, and finally wounded with a sterile needle. The inoculation methods varied across the studies, including immersion of wounded berries in an inoculum suspension (containing 106–107 cells/mL), spraying of the inoculum suspensions (containing 105–107 cells/mL) on the surface of wounded berries, or pipetting a microbial suspension (containing 104–108 cells/mL) into the wound. Following inoculation, berries were kept at temperatures ranging between 22 and 27°C, in the dark or with a 12-h photoperiod, for 5–14 days, depending on the study.
Furthermore, variability in the methods used for artificial inoculation might have influenced the results of different studies, making them difficult to compare. For instance, Barata et al. (2012b) did not obtain any symptoms of SR even after 12 days of incubation post-yeast inoculation. However, Huber (2016) observed a mean SR incidence of 60% after 14 days of incubation. The two studies used similar concentrations of inoculum (106 and 107 cells/mL) and incubation temperatures (22 and 25°C), but the former study inoculated wounded berries through immersion in the inoculum suspension, while the latter inoculated through pipetting the microbial suspension into the wound.
Figure 7 shows the frequency with which different yeast, fungal, and bacterial genera were used to inoculate wounded berries. The most frequently used genera were Aspergillus (n = 13), followed by Hanseniaspora (n = 8), Acetobacter (n = 6), Gluconobacter (n = 5). Candida (n = 4), and Zygoascus (n = 4). The most commonly used species were H. uvarum (n = 6), followed by C. zemplinina (n = 3), Zygoascus hellenicus (n = 3), A. carbonarius (n = 3), Acetobacter aceti (n = 3), and Gluconobacter oxydans (n = 5). Figure 8 shows the mean SR incidence after inoculation of wounded berries with a single species. Moreover, the berries inoculated with A. aculeatus, A. carbonarius, and A. niger showed an SR incidence of 90%. Inoculation with A. malorum and H. uvarum resulted in 50–60% disease incidence, while inoculation with C. zemplinina, Z. hellenicus, and G. saccharivorans resulted in a lower SR incidence (24 to 28%).
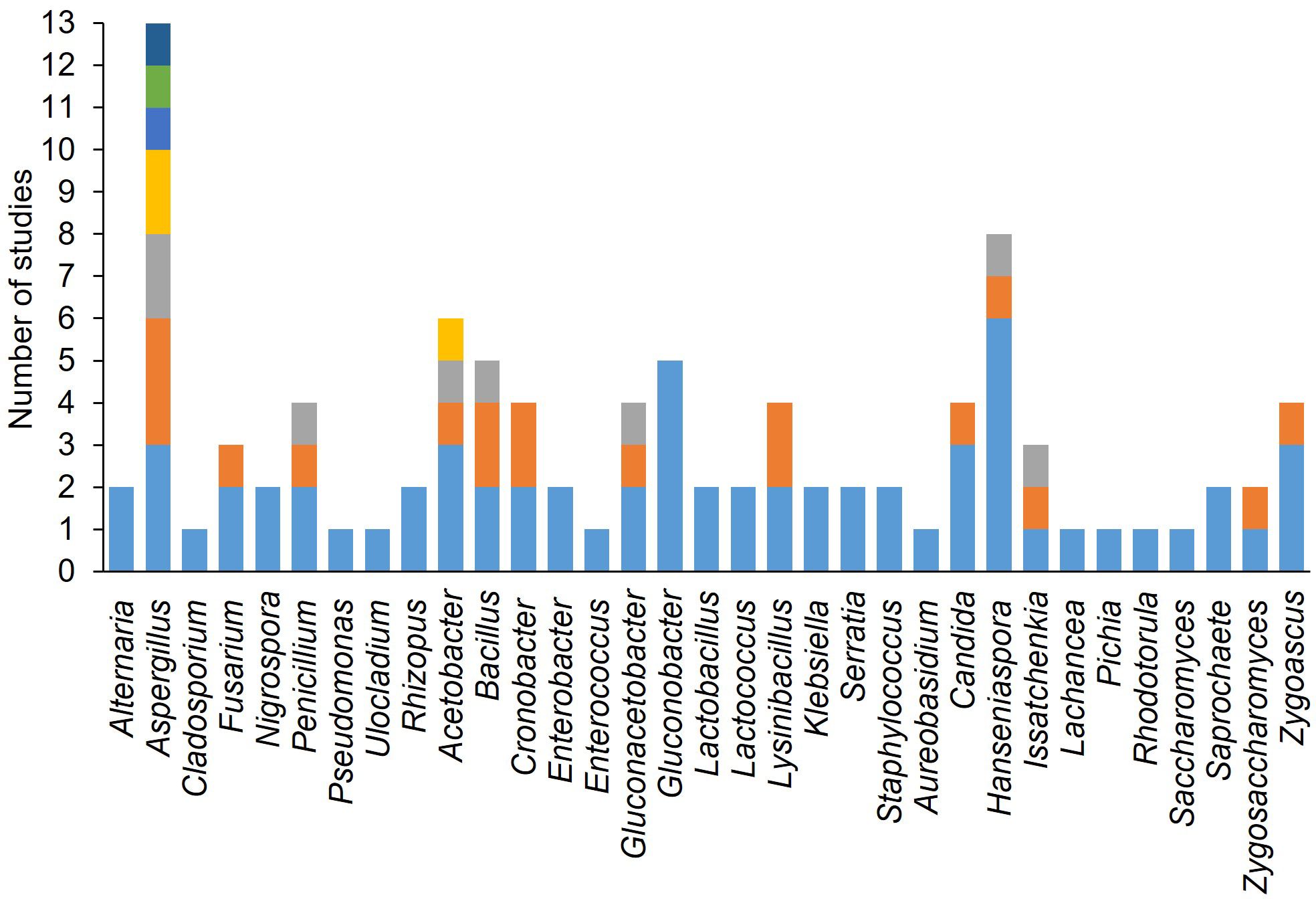
Figure 7 Number of studies wherein each microorganism was used in artificial inoculation studies on berries. The colors of the bars indicate different species within a genus.
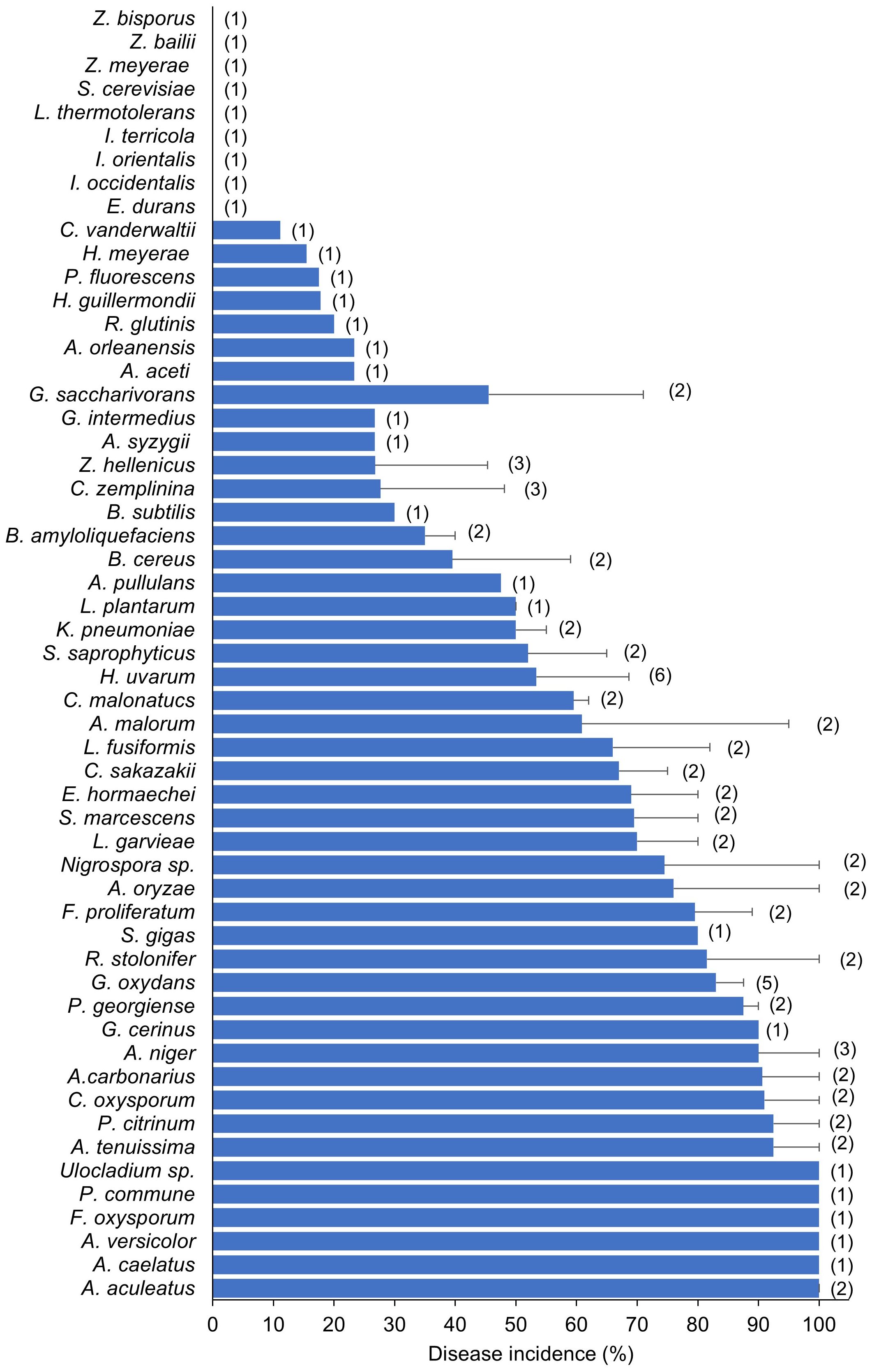
Figure 8 Sour rot incidence in artificial inoculation studies on berries. Bars represent average disease incidence with standard errors; numbers in parentheses indicate the number of studies (that corresponds to the number of entries as disease incidence values used to calculate averages and standard errors).
The above mentioned studies involving artificial inoculation were mainly conducted using single microbial species. Pinto et al. (2017) evaluated the development of SR in wounded berries inoculated with different yeasts, bacteria, and their combinations. Figure 9 shows the difference between disease incidences following inoculation with single bacterial species and with combined inoculation of bacteria and yeasts. The results showed that the SR incidence obtained after the inoculation of multiple species was different from that obtained after the inoculation of a single species. In some cases, the inoculation of single species, including G. oxidans, A. aceti, A. malorum, and A. orleanensis, resulted in a higher disease incidence than after the inoculation of the consortia of species.
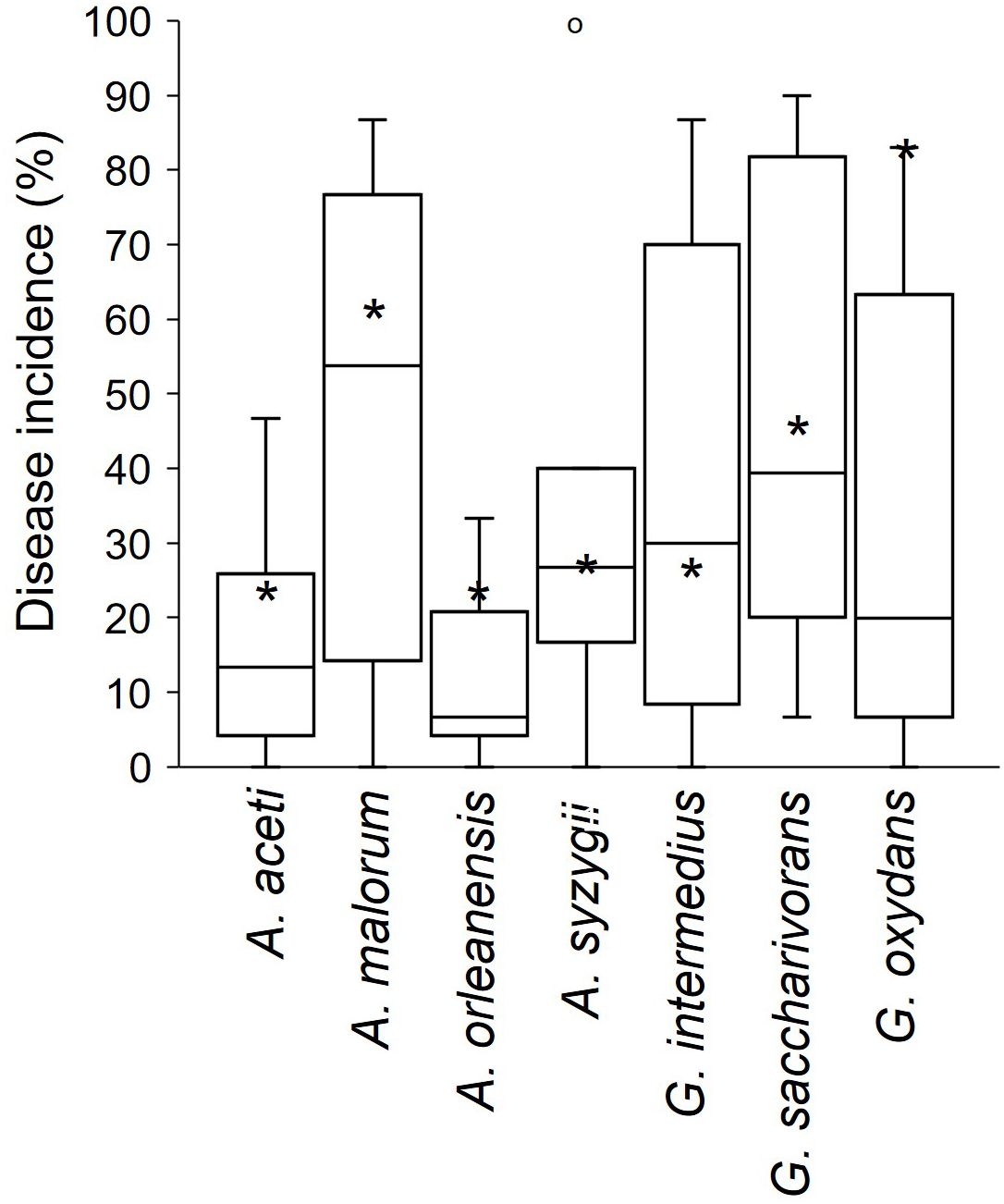
Figure 9 Incidence (%) of sour rot in grapevine berries artificially inoculated with single bacterial species or with consortia of yeasts. (*) indicates the average values of disease incidence in berries artificially inoculated with single bacterial species (data extracted from the whole literature); boxplots represent the artificial inoculation of each single bacterial species with the consortia composed by the following species: Candida vanderwalti, C. zemplinina, Hanseniaspora guilliermondii, H. meyerae, H. uvarum, Zygoascus hellenicus, and Z. meyerae [data provided by Pinto et al. (2017)]. The boxes extend from the 25th to the 75th quartile of the data distribution, the lines crossing the boxes represent the average values, and the whiskers extend to the maximum and minimum values. The dots represent outliers.
The microbes used in artificial inoculation studies have been commonly associated with SR in vineyards (Figures 6A–C) and were isolated more frequently from the affected than the unaffected berries (Figure 7). Notably, some relevant microbes, more frequently isolated from the affected than the unaffected berries, have been poorly considered for artificial inoculation studies, such as Bacillus and Pichia, or never considered, such as Metschnikowia. Future studies should consider involving these microorganisms in artificial inoculation.
3.7 SR epidemiology
The studies suggest that SR-associated microorganisms penetrate the berries through any kind of wound, whether caused by abiotic (e.g., rain, hail, berry abrasion, etc.) or biotic factors (e.g., insects, birds, etc.), including fungal pathogens such as B. cinerea (Marchetti et al., 1984) and Erisiphe necator (Gadoury et al., 2007). Physiological microcracks of the berry skin, especially those close to the pedicel favor pathogen penetration (Considine, 1982; Gravot et al., 2001). Microcracks occur naturally on ripening berries due to different causes that are not completely understood (Becker and Knoche, 2012; Ramteke et al., 2017). The number of microcracks also depend on the orientation of the berry in the cluster and berry region (stylar scar, cheek, or pedicel end) (Becker and Knoche, 2012).
It is also commonly accepted that the insects belonging to the genus Drosophila (Diptera: Tephritidae) are the main vectors of SR-inducing microbes. Among the 74 selected papers in this review, 14.9% of the papers focused on SR vectors. Of these, 72.7% of papers focused on Drosophila spp. (both the fruit fly D. melanogaster and the spotted wing D. suzukii) while 27.3% of studies investigated other possible insect vectors, namely Lobesia botrana (Lepidoptera: Torticiae), Anastrepha fraterculus (Diptera: Tephritidae), and Polistes dominulus (Vespidae).
Both D. melanogaster and D. suzukii (Entling and Hoffmann, 2020) are attracted by damaged and rotting fruit (Bisiach et al., 1986; Fermaud et al., 2002; Barata et al., 2012b; Huber, 2016; Hall et al., 2018a). They collect and transport yeasts and AAB on their body and deposit them on the berries by direct contact while feeding (Ioriatti et al., 2018). D. melanogaster does not have a sharp ovipositor to cause lesions on the grape epidermis, and thus, they can lay eggs only in wounded or rotten grapes (Atallah et al., 2014; Zhu et al., 2023). In contrast, D. suzukii infests on healthy berries through their zig-zag ovipositor, creating wounds on fruits and creating oviposition sites for both species. Once eggs are laid, the larvae develop and trigger the decomposition of berries, thereby inducing SR development (Rombaut et al., 2017; Hall et al., 2018a; Entling and Hoffmann, 2020; Zhu et al., 2023).
Barata et al. (2012b) demonstrated the crucial role of Drosophila in SR development, inoculating both intact and wounded berries with different microorganisms, including C. zemplinina, H. uvarum, Issatchenkia occidentalis (current name Pichia occidentalis), I. orientalis (current name Pichia kudriavzevii), I. terricola (current name P. terricola), Lachancea thermotolerans, Saccharomyces cerevisiae, Zygoascus hellenicus, Zygosaccharomyces bailii, Z. bisporus, G. saccharivorans, and Enterococcus durans. The study showed that in the presence of adult flies, all these microbes induced SR in berries. In contrast, in the absence of a vector, only the wounded berries inoculated with Gluconobacter develop SR. Hall et al. (2018a) showed that only the berries inoculated with the combination of Pichia kluyveri with G. oxydans, S. cerevisiae with A. aceti, and S. cerevisiae with G. oxydans, in the presence of D. melanogaster, induced SR symptoms, confirming the results of Barata et al. (2012b). Furthermore, the authors postulated that the role of Drosophila goes beyond vectoring because artificial inoculation studies with axenic flies (lacking gut or surface microbiota) showed that Drosophila spp. also has a non-microbial contribution in SR development, presumably through their role in promoting the loss of berry integrity. This is caused by at least in part, enzymes released by the larval stage in order to facilitate consumption of the pulp (Gregg et al., 1990; Sakaguchi and Suzuki, 2013).
In other studies, however, artificial inoculation of wounded berries resulted in SR symptoms even in the absence of flies (Pinto et al., 2017).
Machota et al. (2016) showed that South American fruit fly, A. fraterculus (Diptera: Tephritidae), might also facilitate the penetration of SR-inducing microorganisms through the wounds caused by their ovipositor or other body parts. Madden et al. (2017) showed that the European paper wasp, P. dominulus (Vespidae), harbors the SR-inducing polymicrobial community and is capable of dispersing living microorganisms when foraging, spreading the disease in the absence of other insect vectors. However, Pavan et al. (2014) concluded that the European grapevine moth L. botrana (Lepidoptera: Torticiae) does not play a role in the development of SR in vineyards.
Within the affected cluster, SR can also spread via the fermented pulp oozing from the affected berries and dropping onto the underlying berries (Bisiach et al., 1986; Guerzoni and Marchetti, 1987; Barata et al., 2008; Hall et al., 2018a). Splash dispersal or rain falling on the pulp oozing from rotten berries is also a potential dispersal mechanism, even though it has not yet been documented.
The environmental factors influencing SR development have not been fully investigated. Wounded berries inoculated with H. uvarum and G. oxydans and incubated at different temperatures (5–10, 10–15, 15–20, and 20–25°C) for 7 days showed increasing disease severity at increasing temperatures, with the most severe symptoms at 20–25°C and least severe symptoms at <10°C (Huber, 2016). These results were in agreement with the cardinal temperatures of H. uvarum and G. oxydans previously reported by Du Toit and Pretorius (2002) and Salvadó et al. (2011). Other studies reported that warm and rainy conditions between veraison and harvest favored SR epidemic under natural conditions (Zoecklein et al., 2000; Calvo-Garrido et al., 2013; Steel et al., 2013; Huber, 2016).
Climate change potentially elevates SR incidence in many of the major grape-growing regions of the world (Steel et al., 2013). In the past few years, overall SR disease severity, especially in Europe, increased with increasing rainfall events during the period between veraison and harvest (Hausinger et al., 2015; VanderWeide et al., 2020; Lisek and Lisek, 2021; Cornelissen et al., 2023). Additionally, climate warming can accelerate SR spread by directly increasing the vector population that transmits the associated pathogens (Canto et al., 2009; Zhu et al., 2023). Notably, the D. suzukii population might decrease at temperatures of >25°C, influencing both adult reproduction and larval survival (Zerulla et al., 2017; Krüger et al., 2021; Zhu et al., 2023). However, a temperature range of 25–29°C does not affect the fecundity of adults or viability of larvae in D. melanogaster (Schnebel and Grossfield, 1986; Dillon et al., 2007; Min et al., 2021; Zhu et al., 2023), reproducing six times faster at 25°C than at 12°C (Galet, 1999).
Similarly, the development of B. cinerea and non-Botrytis rots can be influenced by high temperatures. B. cinerea has an optimum temperature range of 20–25°C for growth (Ciliberti et al., 2015) but does not grow and infect grape berries at temperatures over 30°C (Latorre et al., 2002), providing opportunities for the development of SR-inducing yeasts (Calvo-Garrido et al., 2013). For non-Botrytis rots, the optimum temperatures for the growth of the fungi C. acutatum and G. uvicola, responsible for ripe rot and bitter rot, respectively, are 25 and 35°C, respectively (Steel et al., 2007). Furthermore, the temperature ranges for optimal growth of P. expansum, A. carbonarius, and R. stolonifer are 20–25, 10–40, and 20–30°C, respectively (Esteban et al., 2004; Judet-Correia et al., 2010; Amiri et al., 2011).
3.8 SR control
SR management via chemical means is generally considered poorly effective given the low efficacy of pre-harvest fungicide applications (Gravot et al., 2001; Nigro et al., 2006), with a few exceptions. For instance, fludioxinil (but not cyprodinil and carbendazim) application was highly effective when applied to manage Aspergillus spp (Tjamos et al., 2004). Similarly, SR was significantly and consistently reduced by approximately 70% after applying combinations of insecticides (such as the spinetoram and zeta-cypermethrin combination to control Drosophila spp.) and antimicrobial agents, such as potassium metabisulfite, copper hydroxide, BLAD (banda de Lupinus albus doce), and/or a mixture of hydrogen dioxide and peroxyacetic acid (Hall et al., 2018b).
Because of the poor efficacy of chemicals, agronomical practices are recommended for SR management. These practices aim at creating a less conducive environment by reducing fruit fly infestation, preventing berry damage by biotic or abiotic factors, and managing the canopy to facilitate air movement and reduce the humidity (Bisiach et al., 1986; Stapleton and Grant, 1992; Zoecklein et al., 1992; McFadden-Smith, 2009; Hall et al., 2018b).
Among the papers selected in the current review, 23% of the papers focused on SR control. In these papers, SR incidence in the NT plots ranged from 0.3 to 97.5%, indicating that the control methods had been tested in a wide range of conditions. Of these, 76% of the papers were conducted in the field and 29% in the laboratory. In particular, three papers involved testing for different biological control agents (BCAs). Four papers compared the application of a single BCA with combinations of BCAs with natural substances or chemicals. Three papers employed only agronomical practices, and in only one paper, the agronomical techniques were combined with chemicals. One paper focused on managing SR with only chemicals, comparing the efficacies of fludioxonil, cyprodinil, and carbendazim. Two papers used natural substances, comparing their efficacies with chemicals or with their combinations with the chemicals. The details of the studies are summarized in Supplementary Material.
Among the agronomical practices, leaf removal at the fruit zone decreased the incidence and severity of SR through an elevated air movement and penetration of solar radiation through the canopy, reducing the humidity within the fruiting zone. Leaf removal also allows greater penetration of fungicides into the fruit zone (Gubler et al., 1987; Bettiga et al., 1989).
The efficacy of BCAs in managing SR was assessed under laboratory conditions against various species of Aspergillus (A. caelatus, A. carbonarius, A. niger, and A. terreus). Twenty-one different BCA species primarily belonging to the following genera were used: Candida, Cyberlindnera, Debaryomyces, Dekkera, Issatchenkia, Lachancea, Kloeckera, Kluyveromyces, Pichia, Saccharomyces, and Torulospora, for a total of 178 cases (that is, one BCA against one pathogen in one experiment). In 91%, 2.8%, and 7.3% of these cases, BCAs showed low, intermediate, and high efficacies (<30%, 30–60%, and >60% SR reduction compared to untreated control), respectively.
Some BCAs, however, showed very high SR-reducing activity. For instance, I. orientalis substantially inhibited A. terreus, C. famata, D. vanrijiae, and K. marxianus, reducing their growth by 100%, 76%, 77%, and 82%, respectively. Moreover, S. chevalieri and C. rugosa affected A. caelatus growth (both by 65%) (Nally et al., 2013). In some cases, the level of SR control mediated by BCAs was comparable to that mediated by fungicides. For instance, SR control mediated by A. pullulans (73%) was similar to that mediated by the fungicide mixture fludioxonil + cyprodinil (69%) (Dimakopoulou et al., 2008).
Previous studies have reported different mechanisms of SR biocontrol, including the (i) inhibition of spore germination, germ tube, and hyphal growth through the production of bioactive metabolites, enzymes (e.g., laminarinases produced by S. cerevisiae, P. membranifaciens, C. catenulata, and others), and volatile organic compounds, and (ii) competition for nutrients (carbon sources and/or iron) (Nally et al., 2013). For instance, S. cerevisiae, T. delbrueckii, C. sake, D. vanrijiae, C. catenulata, and C. famata had high Niche Overlap Index (NOI) scores, suggesting their ability to successfully assimilate a wide variety of carbon sources, making these nutrients unavailable for fungi and allowing a rapid proliferation of yeasts (that is, competitive exclusion) (Spadaro et al., 2010; Bautista-Rosales et al., 2014). In addition, S. cerevisiae, S. chevalieri, C. catenulata, C. famata, C. sake, and others produce siderophores that seize ferric iron, which is biologically important as a co-factor in various fungal enzymes (Meziane et al., 2005; Macagnan et al., 2008), making this ion unavailable to filamentous fungi.
Furthermore, natural compounds were tested in the field, and their SR-reducing efficacy was evaluated in a total of six cases. The natural compounds included plant resistance inducers (specifically, COS-OGA, a complex of oligochitosans and oligopectates), zeolite, and a complex Cu-S. All these compounds exhibited high SR-controlling efficacy. For instance, Calzarano et al (2019 and 2020) showed that zeolitite-based products provided >74% SR control, similar to the fungicides cyprodinil and fludioxonil. Nigro et al. (2006) also evaluated the efficacy of some carbonates and bicarbonates when applied 21 and 5 days before harvest. They reported an SR incidence reduction of 59%, which is higher than that obtained by chemical treatments with cyprodinil and fludioxonil. In addition, calcium might show both direct and indirect SR-reducing activities. The direct activity has been demonstrated for P. expansum and B. cinerea, where in calcium inhibits the polygalacturonase activity that mediates pathogenicity (Droby et al., 1997; Conway et al., 1999). The indirect activity is related to increased resistance of plant cell walls to hydrolytic enzymes produced by the decay microbes via the formation of calcium cross-linkage between pectin polymers (Tobias et al., 1993).
A few of the selected papers assessed the effects of the combined use of BCA and natural substances. Calvo-Garrido et al. (2013) evaluated the effect of BCAs (that is, the yeast C. sake and the fungus Ulocladium oudemansii), a natural coating product (Fungicover) able to improve C. sake survival on grape host tissues, and a resistance inducer (chitosan). They reported that the application of C. sake plus Fungicover reduced SR severity with 58.3% efficacy. The application of U. oudemansii in the early season and chitosan or C. sake plus Fungicover from veraison to harvest led to SR control with 41.7% and 50% efficacies, respectively.
Finally, Gadoury et al. (2023) showed that nighttime application of germicidal ultraviolet at 200 J/m2 significantly reduced the SR severity by 80.2% compared to the untreated test, while the fungicide Oxidate 2.0 (27% hydrogen peroxide plus 2% peroxyacetic acid, BioSafe Systems LLC) had an efficacy of 60.2%.
4 Discussion
This review focused on 74 papers published between 1986 and 2023, with a higher number of publications in the last two decades. Despite the increased interest in SR, especially in the northern hemisphere, the present review highlighted several knowledge gaps in SR etiology, epidemiology, and control.
4.1 Knowledge gaps in SR etiology
Given the complexity of grape microflora (composed of filamentous fungi, yeasts, and bacteria), the selected papers focused on isolating and identifying microorganisms from the affected berries. However, they could not definitively clarify which species are primarily involved in SR etiology. Pichia, Candida, Hanseniaspora, Gluconobacter, and Acetobacter were the most frequently isolated yeasts and bacteria from the affected berries and were considered the most important SR-inducing agents. However, a wide variability in the SR-related microbial species was observed across the different studies. This variability was attributed to the varying geographic areas and climatic conditions, grape varieties, grape maturity stages, and berry damage status. This variability could also be originated from when the microbial analysis has been conducted in relation to the succession of the different functional groups during the berry rotting processes, the initial presence of yeast producing ethanol from sugars followed by acetic bacteria able to oxidize the ethanol in acetic acid) (Barata et al., 2012b). To better understand the role of microorganisms isolated from the affected grape bunches in the different studies, the isolated microorganisms must be artificially inoculated into healthy to verify the Koch’s postulates. However, such inoculation studies have rarely been conducted. Even more rarely, studies were conducted considering not only the inoculation of single microbial species but their consociation and/or succession, given that the order/succession of microorganisms and their trophic levels have shown a relevant effect in some studies (Dutt et al., 2022).
4.2 Inconsistency among artificial inoculation studies
A general inconsistency was observed in the methods used to assess the incidence and severity of SR in vineyards, making inter-study comparisons challenging. Inconsistencies were also found in the methods used for pathogenicity assessment in artificial inoculation studies, which were also related to advancements in research and scientific methods, e.g., from classical microbial analysis to DNA-based microbiome analysis. This observation was significant with respect to extracting robust information on disease etiology. Efforts should then be devoted by researchers to devise standard inoculation methods (taking into account healthy or wounded berries, type of wounding, etc.) with uniform inoculum concentrations. Such studies should be conducted with a common method or scale for assessing disease symptoms, such as discoloration and rotting severity, complemented with the evaluation of chemical changes in the juice of the inoculated berries, such as total and volatile acidity, pH, glycerol, acetic and gluconic acid levels, and alcohol levels, which are essential factors impacting SR progression. Two additional aspects should be considered when planning such studies: The temperature at which inoculated berries are incubated and the time lag between inoculation and assessment of disease and chemical composition. These aspects significantly impact the results. The present review revealed high variability for both these aspects, making inter-study comparisons difficult.
4.3 Knowledge gaps in SR epidemiology
The variability in the temperatures employed in the selected artificial inoculation studies revealed another knowledge gap, which is the ecology and epidemiology of the causal agents. We found only one study on the temperature response patterns of SR-inducing microorganisms (Huber, 2016), and none of the studies considered water response as either relative humidity, surface water, or water activity in berry tissue. Furthermore, none of the studies reported the survival rates, inoculum sources, and changes in berry susceptibility depending on growth stages, with the exception of Hall et al. (2018b), who observed that SR symptoms do not appear before the sugar content of berries is 15°Brix. However, some studies thoroughly evaluated the role of insect vectors in the inoculum dispersal, which was different from other possible microbe dispersal mechanisms. Our epidemiological knowledge on SR is less than that on other important grape diseases. This gap markedly impacts the efficacy of SR control methods (Hall et al., 2018b), and further research is warranted to fill this gap.
4.4 Inconsistency among SR control studies
The studies selected in this review also provided information on SR management strategies. The management options for SR are limited, and the efficacy trials often result in poor, variable, and inconsistent levels of control, which might be attributed to the lack of knowledge on disease epidemiology. Because of the ongoing increase in restrictions on pesticide use (Pertot et al., 2017), reliable, low-impact alternatives for disease control, such as BCAs, natural substances, and agronomical practices, are urgently required. The different strategies for SR control adopted in the studies selected in this review could be integrated through a network meta-analysis that allows direct comparisons of all the strategies and takes into account all the correlations (Madden et al., 2017).
Data availability statement
The raw data supporting the conclusions of this article will be made available by the authors, without undue reservation.
Author contributions
CB: Formal analysis, Methodology, Writing – original draft. VR: Conceptualization, Methodology, Writing – review & editing. GF: Conceptualization, Methodology, Writing – review & editing.
Funding
The author(s) declare that no financial support was received for the research, authorship, and/or publication of this article.
Acknowledgments
CB conducted this study within the Doctoral School on the Agro-Food System (AgriSystem) at the Università Cattolica del Sacro Cuore, Piacenza, Italy.
Conflict of interest
The authors declare that the research was conducted in the absence of any commercial or financial relationships that could be construed as a potential conflict of interest.
Publisher’s note
All claims expressed in this article are solely those of the authors and do not necessarily represent those of their affiliated organizations, or those of the publisher, the editors and the reviewers. Any product that may be evaluated in this article, or claim that may be made by its manufacturer, is not guaranteed or endorsed by the publisher.
Supplementary material
The Supplementary Material for this article can be found online at: https://www.frontiersin.org/articles/10.3389/fpls.2024.1415379/full#supplementary-material
References
Amiri, A., Chai, W., Schnabel, G. (2011). Effect of nutrient status, pH, temperature and water potential on germination and growth of Rhizopus stolonifer and Gilbertella persicaria. J. Plant Pathol. 3, 603–612. Available at: https://www.jstor.org/stable/41999037.
Atallah, J., Teixeira, L., Salazar, R., Zaragoza, G., Kopp, A. (2014). The making of a pest: the evolution of a fruit-penetrating ovipositor in Drosophila suzukii and related species. Proc. R. Soc Lond. B. 281, 20132840. doi: 10.1098/rspb.2013.2840
Barata, A. (2011). Microbial ecology of sour rotten grapes and their influence onchemical and sensorial wine quality. Tese apresentada para obtenc¸ ão do grau deDoutor em Engenharia Alimentar. Portugal: Instituto Superior de Agronomía, UniversidadTécnica de Lisboa, 6.
Barata, A., Campo, E., Malfeito-Ferreira, M., Loureiro, V., Cacho, J., Ferreira, V. (2011a). Analytical and sensorial characterization of the aroma of wines produced with sour rotten grapes using GC-O and GC-MS: identification of key aroma compounds. J. Agric. Food Chem. 59, 2543–2553. doi: 10.1021/jf104141f
Barata, A., González, S., Malfeito-Ferreira, M., Querol, A., Loureiro, V. (2008). Sour rot-damaged grapes are sources of wine spoilage yeasts. FEMS Yeast. Res. 8, 1008–1017. doi: 10.1111/j.1567–1364.2008.00399.x
Barata, A., Malfeito-Ferreira, M., Loureiro, V. (2012a). Changes in sour rotten grape berry microbiota during ripening and wine fermentation. Int. J. Food Microbiol. 154, 152–161. doi: 10.1016/j.ijfoodmicro.2011.12.029
Barata, A., Malfeito-Ferreira, M., Loureiro, V. (2012c). The microbial ecology of wine grape berries. Int. J. Food Microbiol. 153, 243–259. doi: 10.1016/j.ijfoodmicro.2011.11.025
Barata, A., Pais, A., Malfeito-Ferreira, M., Loureiro, V. (2011b). Influence of sour rotten grapes on the chemical composition and quality of grape must and wine. Eur. Food Res. Technol. 233, 183–194. doi: 10.1007/s00217–011-1505-x
Barata, A., Santos, S. C., Malfeito-Ferreira, M., Loureiro, V. (2012b). New insights into the ecological interaction between grape berry microorganisms and Drosophila flies during the development of sour rot. Microb. Ecol 64, 416–430. doi: 10.1007/s00248–012-0041-y
Barnett, J. A., Delaney, M. A., Jones, E., Magson, A. B., Winch, B. (1972). The numbers of yeasts associated with wine grapes of Bordeaux. Archiv. Mikrobiol. 83, 52–55. doi: 10.1007/BF00425044
Bautista-Rosales, P. U., Calderon-Santoyo, M., Servín-Villegas, R., Ochoa-Álvarez, N. A., Vázquez-Juárez, R., Ragazzo-Sánchez, J. A. (2014). Biocontrol action mechanisms of Cryptococcus laurentii on Colletotrichum gloeosporioides of mango. Crop Prot. 65, 194–201. doi: 10.1016/j.cropro.2014.07.019
Becker, T., Knoche, M. (2012). Water induces microcracks in the grape berry cuticle. Vitis J. Grapevine. Res. 51, 141–142.
Bettiga, L., Gubler, W., Marois, J., Bledsoe, A. (1989). Integrated control of Botrytis bunch rot of grape. Calif. Agric. 43, 9–11.
Biesbroek, G. R., Klostermann, J. E., Termeer, C. J., Kabat, P. (2013). On the nature of barriers to climate change adaptation. Reg. Environ. Chang. 13, 1119–1129. doi: 10.1007/s10113-013-0421-y
Bisiach, M., Minervini, G., Zerbetto, F. (1986). Possible integrated control of grapevine sour rot. Vitis 25, 118–128.
Bordelon, B. (2016). Grapes: the Sour Rot Situation (Facts for Fancy Fruit 25). West Lafayette, IN, USA: Purdue University, 1–4.
Calderone, F., Vitale, A., Panebianco, S., Lombardo, M. F., Cirvilleri, G. (2022). COS-OGA applications in Organic Vineyard manage major airborne diseases and maintain postharvest quality of wine grapes. Plants (Basel). 11, 1763. doi: 10.3390/plants11131763
Calvo-Garrido, C., Viñas, I., Elmer, P. A. G., Usall, J., Teixidó, N. (2013). Candida sake CPA-1 and other biologically based products as potential control strategies to reduce sour rot of grapes. Lett. Appl. Microbiol. 57, 356–361. doi: 10.1111/lam.12121
Calzarano, F., Seghetti, L., Pagnani, G., Di Marco, S. (2020). Italian zeolitites in the control of grey mould and sour rot and their effect on leaf reflectance, grape and wine. Agriculture 10, 580. doi: 10.3390/agriculture10120580
Calzarano, F., Valentini, G., Arfelli, G., Seghetti, L., Manetta, A. C., Metruccio, E. G., et al. (2019). Activity of Italian natural chabasite-rich zeolitites against grey mould, sour rot and grapevine moth, and effects on grape and wine composition. Phytopathol. Mediterr. 58, 307–322.
Canto, T., Aranda, M. A., Fereres, A. (2009). Climate change effects on physiology and population processes of hosts and vectors that influence the spread of hemipteran-borne plant viruses. Glob. Change Biol. 15, 1884–1894. doi: 10.1111/j
Carbó, A., Torres, R., Usall, J., Marín, A., Chiralt, A., Teixidó, N. (2019). Novel film-forming formulations of the biocontrol agent Candida sake CPA-1: biocontrol efficacy and performance at field conditions in organic wine grapes. Pest Manage. Sci. 75, 959–968. doi: 10.1002/ps.5200
Castellari, M., Versari, A., Spinabelli, U., Galassi, S., Amati, A. (2000). An improved HPLC method for the analysis of organic acids, carbohydrates, and alcohols in grape musts and wines. J. Liq. Chromatogr. Relat. Technol. 23, 2047–2056. doi: 10.1081/JLC-100100472
Chavan, P., Mane, S., Kulkarni, G., Shaikh, S., Ghormade, V., Nerkar, D. P., et al. (2009). Natural yeast flora of different varieties of grapes used for wine making in India. Food Microbiol. 26, 801–808. doi: 10.1016/j.fm.2009.05.005
Ciliberti, N., Fermaud, M., Roudet, J., Rossi, V. (2015). Environmental conditions affect Botrytis cinerea infection of mature grape berries more than the strain or transposon genotype. Phytopathology 105, 1090–1096. doi: 10.1094/PHYTO-10–14-0264-R
Combina, M., Mercado, L., Borgo, P., Elia, A., Jofré, V., Ganga, A., et al. (2005). Yeasts associated to Malbec grape berries from Mendoza, Argentina. J. Appl. Microbiol. 98, 1055–1061. doi: 10.1111/j.1365–2672.2005.02540.x
Considine, J. A. (1982). Physical aspects of fruit growth: Cuticular fracture and fracture patterns in relation to fruit structure in vitis vinifera. J. Hortic. Sci. 57, 79–91. doi: 10.1080/00221589.1982.11515027
Conway, W. S., Janisiewicz, W. J., Klein, J. D., Sams, C. E. (1999). Strategy for combining heat treatment, calcium infiltration, and biological control to reduce postharvest decay of “Gala” apples. HortSci 34, 700–704. doi: 10.21273/HORTSCI.34.4.700
Cornelissen, R. J., Aleixandre Tudo, J. L., Nieuwoudt, H. H. (2023). Winery on-site assessment of grapevine bunch rot: in pursuit of sustainable practices. S. Afr. J. Enol. Vitic. 44, 81–95. doi: 10.21548/44-1-5701
Cornelissen, R. J., Kidd, M., Aleixandre-Tudo, J. L., Nieuwoudt, H. H. (2022). Data-driven determination of disease markers’ threshold values in rot-affected wine grapes. S. Afr. J. Enol. Vitic. 43, 85–95. doi: 10.21548/43–1-4960
Davenport, R. R. (1974). Microecology of yeasts and yeast-like organisms associated with an English vineyard. Vitis 13, 123.
De La Torre, M. J., Millan, M. C., Perez-Juan, P., Morales, J., Ortega, J. M. (1999). Indigenous yeasts associated with two vitis vinifera grape varieties cultured in southern Spain. Microbios 100, 27–40.
De Man, J. C., Rogosa, M., Sharpe, M. E. (1960). A medium for the cultivation of lactobacilli. J. Appl. Bacteriol. 23, 130–135. doi: 10.1111/j.1365–2672.1960.tb00188.x
Dillon, M. E., Cahn, L. R., Huey, R. B. (2007). Life history consequences of temperature transients in Drosophila melanogaster. J. Exp. Biol. 210, 2897–2904. doi: 10.1242/jeb.00755
Dimakopoulou, M., Tjamos, S. E., Antoniou, P. P., Pietri, A., Battilani, P., Avramidis, N., et al. (2008). Phyllosphere grapevine yeast Aureobasidium pullulans reduces Aspergillus carbonarius (sour rot) incidence in wine-producing vineyards in Greece. Biol. Control. 46, 158–165. doi: 10.1016/j.biocontrol.2008.04.015
Droby, S., Wisniewski, M. E., Cohen, L., Weiss, B., Touitou, D., Eilam, Y., et al. (1997). Influence of CaCl2 on Penicillium digitatum, grapefruit peel tissue, and biocontrol activity of Pichia guilliermondii. Phytopathology 87, 310–315. doi: 10.1094/PHYTO.1997.87.3.310
Dugan, F. M., Lupien, S. L., Grove, G. G. (2002). Incidence, aggressiveness and in planta interactions of Botrytis cinerea and other filamentous fungi quiescent in grape berries and dormant buds in Central Washington State. J. Phytopathol. Phytopathol. Z. 150, 375–381. doi: 10.1046/j.1439–0434.2002.00774.x
Duncan, R. A., Stapleton, J. J., Leavitt, G. M. (1995). Population dynamics of epiphytic mycoflora and occurrence of bunch rots of wine grapes as influenced by leaf removal. Plant Pathol. 44, 956–965. doi: 10.1111/j.1365–3059.1995.tb02653.x
Du Toit, W. J., Pretorius, I. S. (2002). The occurrence, control and esoteric effect of acetic acid bacteria in winemaking. Ann. Microbiol. 52, 155–179.
Dutt, A., Andrivon, D., Le May, C. (2022). Multi-infections, competitive interactions, and pathogen coexistence. Plant Pathol. 71, 5–22. doi: 10.1111/ppa.13469
Entling, W., Hoffmann, C. (2020). Single and combined effects of Drosophila suzukii and Drosophila melanogaster on sour rot development in viticulture. J. Appl. Entomol. 144, 153–160. doi: 10.1111/jen.12721
Esteban, A., Abarca, M. L., Bragulat, M. R., Cabañes, F. J. (2004). Effects of temperature and incubation time on production of ochratoxin A by black aspergilli. Res. Microbiol. 155, 861–866. doi: 10.1016/j.resmic.2004.07.002
Fermaud, M., Gravot, E., Blancard, D. (2002). Sour rot in the vineyards of Bordeaux [France]. 2. Vection of pathogenic micro-organisms by fruit flies. Phytoma 547, 41–44. La défense des végétaux (France).
Fidelibus, M. W., Christensen, L. P., Golino, D. A., Sweet, N. L., Cathline, K. A. (2009). Yield components and fruit composition of five Barbera grapevine selections in the San Joaquin valley, California. Am. J. Enol. Vitic. 60, 533–536. doi: 10.5344/ajev.2009.60.4.533
Fidelibus, M. W., Christensen, L. P., Katayama, D. G., Verdenal, P. T. (2005). Performance of Zinfandel and Primitivo grapevine selections in the central San Joaquin valley, California. Am. J. Enol. Vitic. 56, 284–286. doi: 10.5344/ajev.2005.56.3.284
Fidelibus, M. W., Christensen, L. P., Katayama, D. G., Verdenal, P. T. (2006). Yield components and fruit composition of six Chardonnay grapevine clones in the Central San Joaquin valley, California. Am. J. Enol. Vitic. 57, 503–506. doi: 10.5344/ajev.2006.57.4.503
Fidelibus, M. W., Christensen, L. P., Katayama, D. G., Verdenal, P. T., Cathline, K. (2007). Fruit characteristics of six Merlot grapevine selections in the Central San Joaquin valley, California. Am. J. Enol. Vitic. 58, 259–261. doi: 10.5344/ajev.2007.58.2.259
Fleet, G. H., Prakitchaiwattana, C., Beh, A. L., Heard, G. (2002). The Yeast Ecology of Wine Grapes. Biodiversity and Biotechnology of Wine Yeasts. Trivandrum: Research Signpost, 1–17.
Fonseca, Á., Inácio, J. (2006). “Phylloplane yeasts,” in Biodiversity and Ecophysiology of Yeasts. Eds. Rosa, C. A., Péter, G. (Springer-Verlag, Berlin, Heidelberg), 263–301. doi: 10.1007/3–540-30985–3_13
Gadoury, D. M., Sapkota, S., Cadle-Davidson, L., Underhill, A., McCann, T., Gold, K. M., et al. (2023). Effects of nighttime applications of germicidal ultraviolet light upon powdery mildew (Erysiphe necator), downy mildew (Plasmopara viticola), and sour rot of grapevine. Plant Dis. 107, 1452–1462. doi: 10.1094/PDIS-04–22-0984-RE
Gadoury, D. M., Seem, R. C., Wilcox, W. F., Henick-Kling, T., Conterno, L., Day, A., et al. (2007). Effects of diffuse colonization of grape berries by Uncinula necator on bunch rots, berry microflora, and juice and wine quality. Phytopathology 97, 1356–1365. doi: 10.1094/PHYTO-97–10-1356
Gao, H., Yin, X., Jiang, X., Shi, H., Yang, Y., Wang, C., et al. (2020). Diversity and spoilage potential of microbial communities associated with grape sour rot in eastern coastal areas of China. PeerJ 8, e9376. doi: 10.7717/peerj.9376
González, V., Tello, M. L. (2011). The endophytic mycota associated with vitis vinifera in central Spain. Fungal Divers. 47, 29–42. doi: 10.1007/s13225–010-0073-x
Gravot, E., Blancard, D., Fermaud, M., Lonvaud, A., Joyeux, A. (2001). Sour rot. I: etiology. Research into the causes of this form of rot of grapes in Bordeaux vineyards. Phytoma 543, 36–39.
Gregg, T. G., McCrate, A., Reveal, G., Hall, S., Rypstra, A. L. (1990). Insectivory and social digestion in Drosophila. Biochem. Genet. 28, 197–207. doi: 10.1007/BF00561337
Gubler, W. D., Marois, J. J., Bledsoe, A. M., Bettiga, L. J. (1987). Control of Botrytis bunch rot of grape with canopy management. Plant Dis. 71, 599–601. doi: 10.1094/PD-71–0599
Guerra, E., Sordi, G., Mannazzu, I., Clementi, F., Fatichenti, F. (1999). Occurrence of wine yeasts on grapes subjected to different pesticide treatments. Ital. J. Food Sci. 3, 221–230.
Guerzoni, E., Marchetti, R. (1987). Analysis of yeast flora associated with grape sour rot and of the chemical disease markers. Appl. Environ. Microbiol. 53, 571–576. doi: 10.1128/aem.53.3.571–576.1987
Hall, M. E., Loeb, G. M., Cadle-Davidson, L., Evans, K. J., Wilcox, W. F. (2018a). Grape sour rot: a four-way interaction involving the host, yeast, acetic acid bacteria, and insects. Phytopathology 108, 1429–1442. doi: 10.1094/PHYTO-03–18-0098-R
Hall, M. E., Loeb, G. M., Wilcox, W. F. (2015). Etiology and management strategies for sour rot on grapes. Phytopathology 105, S4.
Hall, M. E., Loeb, G. M., Wilcox, W. F. (2018b). Control of sour rot using chemical and canopy management techniques. Am. J. Enol. Vitic. 69, 342–350. doi: 10.5344/ajev.2018.17091
Hausinger, K., Lipps, M., Raddatz, H., Rosch, A., Scholten, G., Schrenk, D. (2015). Automated optical grape-sorting of rotten grapes: effects of rot infections on gluconic acid concentration and glycerol/gluconic acid ratios in must and wine. J. Wine Res. 26, 18–28. doi: 10.1080/09571264.2014.993950
Haviland, D. R., Bettiga, L. J., Varela, L. G., Roncoroni, J. A., Smith, R. J., Westerdahl, B. B., et al. (2017). UC IPM Pest Management Guidelines Grape. UC ANR Publ (Oakland, CA: Online Publication), 3448. Available at: http://ipm.ucanr.edu/PMG/pmgauthors.html?grapes.
Hewitt, W. B. (1974). Rots and Bunch Rots of Grapes Vol. 868 (California Agricultural Experiment Station, University of California), 52.
Hewstone, N., Valenzuela, J., Muñoz, C. (2007). Nueva variedad de uva de mesa. Agric. Cult. Tec. 67, 201–204. doi: 10.4067/S0365-28072007000200011
Huber, C. (2016). Etiology and Management of Grape Sour Rot (St. Catharines, ON, Canada: Dept of Biological Sciences, Brock University), 2016.
Huber, C., McFadden-Smith, W., Inglis, D. (2011). Management and etiology of grape sour rot in the Niagara region. Phytopathology 101, S259–S259.
Ioriatti, C., Guzzon, R., Anfora, G., Ghidoni, F., Mazzoni, V., Villegas, T. R., et al. (2018). Drosophila suzukii (Diptera: Drosophilidae) contributes to the development of sour rot in grape. J. Econ. Entomol. 111, 283–292. doi: 10.1093/jee/tox292
Judet-Correia, D., Bollaert, S., Duquenne, A., Charpentier, C., Bensoussan, M., Dantigny, P. (2010). Validation of a predictive model for the growth of Botrytis cinerea and Penicillium expansum on grape berries. Int. J. Food Microbiol. 142, 106–113. doi: 10.1016/j.ijfoodmicro.2010.06.009
Kassemeyer, H. H., Berkelmann-Löhnertz, B. (2009). “Fungi of grapes,” in Biology of Microorganisms on Grapes, in Must and in Wine. Eds. König, H., Unden, G., Fröhlich, J. (Springer-Verlag, Berlin, Heidelberg), 61.e87.
Kenney, P., Hall, M. (2021). Reducing sour rot spray applications initiated after symptom development does not impact disease control. Am. J. Enol. Vitic. 5, 22–28. doi: 10.5344/catalyst.2021.20008
Krüger, A. P., Vieira, J. G., Scheunemann, T., Nava, D. E., Garcia, F. R. (2021). Effects of temperature and relative humidity on mating and survival of sterile Drosophila suzukii. J. Appl. Entomol. 145, 789–799. doi: 10.1111/jen.12894
Kupina, S. A. (1984). Simultaneous quantitation of glycerol, acetic acid and ethanol in grape juice by high performance liquid chromatography. Am. J. Enol. Vitic. 35, 59–62. doi: 10.5344/ajev.1984.35.2.59
Latorre, B., Franck, J., Zoffoli, J. P., Viertel, S. (2002). Pudrición ácida de la vid. Rev. Frutícola. (Chile). 23, 53–58.
Li, S. S., Cheng, C., Li, Z., Chen, J. Y., Yan, B., Han, B. Z., et al. (2010). Yeast species associated with wine grapes in China. Int. J. Food Microbiol. 138, 85–90. doi: 10.1016/j.ijfoodmicro.2010.01.009
Lisek, J., Lisek, A. (2021). Varietal response to sour bunch rot in polish grapevine genetic resources. Agronomy 11, 1537. doi: 10.3390/agronomy11081537
Longo, E., Cansado, J., Agrelo, D., Villa, T. G. (1991). Effect of climatic conditions on yeast diversity in grape musts from northwest Spain. Am. J. Enol. Vitic. 42, 141–144. doi: 10.5344/ajev.1991.42.2.141
Loureiro, V., Ferreira, M. M., Monteiro, S., Ferreira, R. B. (2012). The Microbial Community of Grape Berry. The Biochemistry of the Grape Berry. Sharjah: Bentham Science Publishers, 241–268.
Macagnan, D., Romeiro, R. D. S., Pomella, A. W. V., deSouza, J. T. (2008). Production of lytic enzymes and siderophores, and inhibition of germination of basidiospores of Moniliophthora (ex Crinipellis) perniciosa by phylloplane actinomycetes. Biol. Control. 47, 309–314. doi: 10.1016/j.biocontrol.2008.08.016
Machota, R., Bortoli, L. C., Cavalcanti, F. R., Botton, M., Grützmacher, A. D. (2016). Assessment of injuries caused by Anastrepha fraterculus (Wied.)(Diptera: Tephritidae) on the incidence of bunch rot diseases in table grape. Neotrop. Entomol. 45, 361–368. doi: 10.1007/s13744–016-0377-y
Madden, A. A., Boyden, S. D., Soriano, J. N., Corey, T. B., Leff, J. W., Fierer, N., et al. (2017). The emerging contribution of social wasps to grape rot disease ecology. PeerJ 5, e3223. doi: 10.7717/peerj.3223
Marchetti, R., Guerzoni, M. E., Gentile, M. (1984). Research on the etiology of a new disease of grapes: sour rot. Vitis 23, 55–65.
Martini, A., Ciani, M., Scorzetti, G. (1996). Direct enumeration and isolation of wine yeasts from grape surfaces. Am. J. Enol. Vitic. 47, 435–440. doi: 10.5344/ajev.1996.47.4.435
Mateo, E., Torija, M. J., Mas, A., Bartowsky, E. J. (2014). Acetic acid bacteria isolated from grapes of South Australian vineyards. Int. J. Food Microbiol. 178, 98–106. doi: 10.1016/j.ijfoodmicro.2014.03.010
McFadden-Smith, W. (2009). Efficacy of treatments for control of Botrytis bunch rot and sour rot of grapevine 2008. Plant Disease Management Rep. 3: SMF003.
McFadden-Smith, W., Gubler, W. D., Rot, S. (2015). Compendium of Grape Diseases, Pests, and Disorders. 2nd ed.Eds. Wilcox, W. F., Gubler, W. D., Uyemoto, J. K. (St. Paul, MN: APS Publishing), 87–90.
McKenzie, J., Joy, A. (2020). Family intervention improves outcomes for patients with delirium: Systematic review and meta-analysis. Australas. J. Ageing. 39 (1), 21–30.
Meziane, H., van der Sluis, I., Van Loon, L. C., Höfte, M., Bakker, P. A. (2005). Determinants of Pseudomonas putida WCS358 involved in inducing systemic resistance in plants. Mol. Plant Pathol. 6, 177–185. doi: 10.1111/j.1364–3703.2005.00276.x
Min, K. W., Jang, T., Lee, K. P. (2021). Thermal and nutritional environments during development exert different effects on adult reproductive success in Drosophila melanogaster. Ecol. Evol. 11, 443–457. doi: 10.1002/ece3
Mortimer, R., Polsinelli, M. (1999). On the origins of wine yeast. Res. Microbiol. 150, 199–204. doi: 10.1016/s0923–2508(99)80036–9
Mostert, L., Crous, P. W., Petrini, O. (2000). Endophytic fungi associated with shoots and leaves of vitis vinifera, with specific reference to the Phomopsis viticola complex. Sydowia 52, 46–58.
Nally, M. C., Pesce, V. M., Maturano, Y. P., Toro, M. E., Combina, M., Castellanos de Figueroa, L. I., et al. (2013). Biocontrol of fungi isolated from sour rot infected table grapes by Saccharomyces and other yeast species. Postharvest. Biol. Technol. 86, 456–462. doi: 10.1016/j.postharvbio.2013.07.022
Nigro, F., Schena, L., Ligorio, A., Pentimone, I., Ippolito, A., Salerno, M. G. (2006). Control of table grape storage rots by pre-harvest applications of salts. Postharvest Biol. Technol 42, 142–149. doi: 10.1016/j.postharvbio.2006.06.005
Nisiotou, A. A., Nychas, G. J. E. (2007). Yeast populations residing on healthy or Botrytis-infected grapes from a vineyard in Attica, Greece. Appl. Environ. Microbiol. 73, 2765–2768. doi: 10.1128/AEM.01864–06
Oriolani, E. J. A., Rodríguez, M. C., Combina, M. (2007). Complejo parasitario de la podredumbre ácida de los racimos de la vid, en Mendoza y San Juan Argentina. XI Congreso Latinoamericano de Viticultura y Enología. Anales. Mendoza.
Oriolani, E. J., Rodriguez-Romera, M., Combina, M. (2009). Parasitic complex of acid rot bunches of grapes, in, in Mendoza and San Juan Abstract Book of the XV Latin American Congress and XVIII Chilean Congress of Plant Pathology (Santiago, Chile: Asociación Latinoamericana de Fitopatología and the Sociedad Chilena de Fitopatología. Argentina), 204.
Owoyemi, A., Lapidot, O., Kochanek, B., Zahavi, T., Salzer, Y., Porat, R., et al. (2022). Sour rot in the vineyard is an indicator of Botrytis rot in grapes after storage. Postharvest. Biol. Technol. 191, 111980. doi: 10.1016/j.postharvbio.2022.111980
Pavan, F., Bigot, G., Cargnus, E., Zandigiacomo, P. (2014). Influence of the carpophagous generations of the European grapevine moth Lobesia botrana on grape bunch rots. Phytoparasitica 42, 61–69. doi: 10.1007/s12600–013-0338–5
Pertot, I., Caffi, T., Rossi, V., Mugnai, L., Hoffmann, C., Grando, M. S., et al. (2017). A critical review of plant protection tools for reducing pesticide use on grapevine and new perspectives for the implementation of IPM in viticulture. Crop Prot. 97, 70–84. doi: 10.1016/j.cropro.2016.11.025
Pinto, L., Caputo, L., Quintieri, L., De Candia, S., Baruzzi, F. (2017). Efficacy of gaseous ozone to counteract postharvest table grape sour rot. Food Microbiol. 66, 190–198. doi: 10.1016/j.fm.2017.05.001
Pinto, L., Malfeito-Ferreira, M., Quintieri, L., Silva, A. C., Baruzzi, F. (2019). Growth and metabolite production of a grape sour rot yeast-bacterium consortium on different carbon sources. Int. J. Food Microbiol. 296, 65–74. doi: 10.1016/j.ijfoodmicro.2019.02.022
Pretorius, I. S., van der Westhuizen, T. J., Augustyn, O. P. H. (1999). Yeast biodiversity in vineyards and wineries and its importance to the South African wine industry: a review. SAJEV 20, 61–70. doi: 10.21548/20–2-2234
Ramteke, S. D., Urkude, V., Parhe, S. D., Bhagwat, S. R. (2017). Berry cracking; its causes and remedies in grapes-a review. Trends Biosci. 10, 549–556.
Randall, N. P., James, K. L. (2012). The effectiveness of integrated farm management, organic farming and agri-environment schemes for conserving biodiversity in temperate Europe-a systematic map. Environ. Evid. 1, 1–21. doi: 10.1186/2047–2382-1–4
Raspor, P., Milek, D. M., Polanc, J., Možina, S. S., Čadež, N. (2006). Yeasts isolated from three varieties of grapes cultivated in different locations of the Dolenjska vine-growing region, Slovenia. Int. J. Food Microbiol. 109, 97–102. doi: 10.1016/j.ijfoodmicro.2006.01.017
Renouf, V., Claisse, O., Lonvaud-Funel, A. (2005). Understanding the microbial ecosystem on the grape berry surface through numeration and identification of yeast and bacteria. Aust. J. Grape Wine Res. 11, 316–327. doi: 10.1111/j.1755–0238.2005.tb00031.x
Rombaut, A., Guilhot, R., Xuéreb, A., Benoit, L., Chapuis, M. P., Gibert, P., et al. (2017). Invasive Drosophila suzukii facilitates Drosophila melanogaster infestation and sour rot outbreaks in the vineyards. R. Soc Open Sci. 4, 170117. doi: 10.1098/rsos.170117
Rooney-Latham, S., Janousek, C. N., Eskalen, A., Gubler, W. D. (2008). First report of Aspergillus carbonarius causing sour rot of table grapes (vitis vinifera) in California. Plant Dis. 92, 651–651. doi: 10.1094/PDIS-92–4-0651A
Rosini, G., Federici, F., Martini, A. (1982). Yeast flora of grape berries during ripening. Microb. Ecol. 8, 83–89. doi: 10.1007/BF02011464
Rousseaux, S., Diguta, C. F., Radoï-Matei, F., Alexandre, H., Guilloux-Bénatier, M. (2014). Non-Botrytis grape-rotting fungi responsible for earthy and moldy off-flavors and mycotoxins. Food Microbiol. 38, 104–121. doi: 10.1016/j.fm.2013.08.013
Sakaguchi, H., Suzuki, M. G. (2013). Drosophila melanogaster larvae control amylase secretion according to the hardness of food. Front. Physiol. 4. doi: 10.3389/fphys.2013.00200
Salvadó, Z., Arroyo-López, F. N., Guillamón, J. M., Salazar, G., Querol, A., Barrio, E. (2011). Temperature adaptation markedly determines evolution within the genus Saccharomyces. Appl. Environ. Microbiol. 77, 2292–2302. doi: 10.1128/AEM.01861–10
Schena, L., Nigro, F., Soleti Ligorio, V., Yaseen, T., Ippolito, A., El Ghaouth, A. (2005). Biocontrol activity of bio-coat and biocure against postharvest rots of table grapes and sweet cherries. Acta Hortic. 682, 2115–2120. doi: 10.17660/ActaHortic.2005.682.288
Schnebel, E. M., Grossfield, J. (1986). Oviposition temperature range in four drosophila species triads from different ecological backgrounds. Am. Midl. Nat. 1, 25–35. doi: 10.2307/24259
Schuller, D., Côrte-Real, M., Leão, C. (2000). A differential medium for the enumeration of the spoilage yeast Zygosaccharomyces bailii in wine. J. Food Prot. 63, 1570–1575. doi: 10.4315/0362–028x-63.11.1570
Schütz, M., Gafner, J. (1994). Dynamics of the yeast strain population during spontaneous alcoholic fermentation determined by CHEF gel electrophoresis. Lett. Appl. Microbiol. 19, 253–257. doi: 10.1111/j.1472–765X.1994.tb00957.x
Shiraishi, M., Fujishima, H., Chijiwa, H. (2010). Evaluation of table grape genetic resources for sugar, organic acid, and amino acid composition of berries. Euphytica 174, 1–13. doi: 10.1007/s10681–009-0084–4
Spadaro, D., Ciavorella, A., Dianpeng, Z., Garibaldi, A., Gullino, M. L. (2010). Effect of culture media and pH on the biomass production and biocontrol efficacy of a Metschnikowia pulcherrima strain to be used as a biofungicide for postharvest disease control. Can. J. Microbiol. 56, 128–137. doi: 10.1139/w09–117
Stapleton, J. J., Grant, R. S. (1992). Leaf removal for nonchemical control of the summer bunch rot complex of wine grapes in the San Joaquin valley. Plant Dis. 76, 205–208. doi: 10.1094/PD-76–0205
Steel, C. C., Blackman, J. W., Schmidtke, L. M. (2013). Grapevine bunch rots: impacts on wine composition, quality, and potential procedures for the removal of wine faults. J. Agric. Food Chem. 61, 5189–5206. doi: 10.1021/jf400641r
Steel, C. C., Greer, L. A., Savocchia, S. (2007). Studies on Colletotrichum acutatum and Greeneria uvicola: two fungi associated with bunch rot of grapes in sub-tropical Australia. Aust. J. Grape Wine Res. 13, 23–29. doi: 10.1111/j.1755–0238.2007.tb00068.x
Steel, C. C., Savocchia, S., Greer, L. A. (2016). Management of bunch rot diseases of grapes in subtropical vineyards in Australia. Acta Hortic. 1115, 265–271.
Tjamos, S. E., Antoniou, P. P., Kazantzidou, A., Antonopoulos, D. F., Papageorgiou, I., Tjamos, E. C. (2004). Aspergillus Niger and Aspergillus carbonarius in Corinth raisin and wine-producing vineyards in Greece: population composition, ochratoxin A production and chemical control. J. Phytopathol. 152, 250–255. doi: 10.1111/j.1439–0434.2004.00838.x
Tobias, R. B., Conway, W. S., Sams, C. F., Gross, K. C., Whitaker, B. E. (1993). Cell wall composition of calcium-treated apples inoculated with Botrytis cinerea. Phytochemistry 32, 35–39. doi: 10.1016/0031-9422(92)80102-K
Valero, E., Cambon, B., Schuller, D., Casal, M., Dequin, S. (2007). Biodiversity of Saccharomyces yeast strains from grape berries of wine-producing areas using starter commercial yeasts. FEMS Yeast. Res. 7, 317–329. doi: 10.1111/j.1567–1364.2006.00161.x
Valero, E., Schuller, D., Cambon, B., Casal, M., Dequin, S. (2005). Dissemination and survival of commercial wine yeast in the vineyard: a large-scale, three-years study. FEMS Yeast. Res. 5, 959–969. doi: 10.1016/j.femsyr.2005.04.007
VanderWeide, J., Frioni, T., Ma, Z., Stoll, M., Poni, S., Sabbatini, P. (2020). Early leaf removal as a strategy to improve ripening and lower cluster rot in cool climate (vitis vinifera L.) Pinot Grigio. Am. J. Enol. Vitic. 71, 70–79. doi: 10.5344/ajev.2019.19042
Vogel, A., Breeden, S., Brannen, P., Blaauw, B., Hickey, C. (2020). Grape sour rot. UGA cooperative extension circular. 1212.
Wei, Y., Wang, C., Zhao, X., Shang, Q., Liu, Z. (2015). Identification and biological characteristics of the pathogenic sf-19 strain of grape sour rot from Beijing. Phytopathology 105, 147–147.
Wilcox, W. F., Gubler, W. D., Uyemoto, J. K. (2015). Compendium of grape diseases, disorders, and pests (St. Paul, MN, USA: APS Press, The American Phytopathological Society), 39–45.
Yanagida, F., Ichinose, F., Shinohara, T., Goto, S. (1992). Distribution of wild yeasts in the white grape varieties at central Japan. J. Gen. Appl. Microbiol. 38, 501–504. doi: 10.2323/jgam.38.501
Zerulla, F. N., Augel, C., Zebitz, C. P. W. (2017). ). Oviposition activity of Drosophila suzukii as mediated by ambient and fruit temperature. PloS One 12, e0187682. doi: 10.1371/journal.pone.0187682
Zhu, L., Xue, Q., Ma, G., Ma, C. S. (2023). Climate warming exacerbates plant disease through enhancing commensal interaction of co-infested insect vectors. J. Pest Sci. 96, 945–959. doi: 10.1007/s10340–022-01574–5
Zoecklein, B. W., Williams, J. M., Duncan, S. E. (2000). Effect of sour rot on the composition of white Riesling (vitis vinifera L.) grapes. Small. Fruits. Rev. 1, 63–77. doi: 10.1300/J301v01n01_08
Keywords: vitis vinifera, bunch microflora, causal agents, pathogenicity studies, integrated disease control
Citation: Brischetto C, Rossi V and Fedele G (2024) Knowledge gaps on grape sour rot inferred from a systematic literature review. Front. Plant Sci. 15:1415379. doi: 10.3389/fpls.2024.1415379
Received: 10 April 2024; Accepted: 17 June 2024;
Published: 03 July 2024.
Edited by:
David Gramaje, Spanish National Research Council (CSIC), SpainReviewed by:
Amnon Lichter, Agricultural Research Organization (ARO), IsraelMegan Hall, Terroir Consulting Group, United States
Copyright © 2024 Brischetto, Rossi and Fedele. This is an open-access article distributed under the terms of the Creative Commons Attribution License (CC BY). The use, distribution or reproduction in other forums is permitted, provided the original author(s) and the copyright owner(s) are credited and that the original publication in this journal is cited, in accordance with accepted academic practice. No use, distribution or reproduction is permitted which does not comply with these terms.
*Correspondence: Giorgia Fedele, giorgia.fedele@unicatt.it