- College of Horticulture and Landscape Architecture, Henan Institute of Science and Technology, Xinxiang, Henan, China
The SBP-box gene significantly influences plant growth, development, and stress responses, yet its function in pepper plants during drought stress remains unexplored. Using virus-induced gene silencing and overexpression strategies, we examined the role of CaSBP13 during drought stress in plants. The results revealed that the expression of CaSBP13 can be induced by drought stress. Silencing of CaSBP13 in pepper notably boosted drought resistance, as evident by decreased active oxygen levels. Furthermore, the water loss rate, relative electrical conductivity, malondialdehyde content, and stomatal density were reduced in CaSBP13-silenced plants compared to controls. In contrast, CaSBP13 overexpression in Nicotiana benthamiana decreased drought tolerance with elevated reactive oxygen levels and stomatal density. Additionally, ABA signaling pathway genes (CaPP2C, CaAREB) exhibited reduced expression levels in CaSBP13-silenced plants post drought stress, as compared to control plants. On the contrary, CaPYL9 and CaSNRK2.4 showed heightened expression in CaSBP13-sienced plants under the same conditions. However, a converse trend for NbAREB, NbSNRK2.4, and NbPYL9 was observed post-four day drought in CaSBP13-overexpression plants. These findings suggest that CaSBP13 negatively regulates drought tolerance in pepper, potentially via ROS and ABA signaling pathways.
Introduction
With global climate shifts and expanding human activity, demand for water resources escalates, leading to widespread drought disasters globally. Droughts persist for lengthy periods and exert substantial influence on agricultural productivity (Vicente-Serrano et al., 2022). The absence of moisture impairs plant development across all stages. It diminishes seed germination, planting vigor, canopy development, root elongation, and subsequently triggers yield reduction or plant mortality (Abbas et al., 2023). Pepper, a highly valued vegetable crop, due to its shallowly rooted system with reduced regeneration capacity after root damage, making it especially prone to drought stress. Over time, vegetables have evolution multi-tiered defensive strategies against drought to sustain growth and metabolic processes (Abbas et al., 2023). Examples of physiological and biochemical alterations include the activation of stress responsive genes, which predominantly controlled by transcription factors (Thirumalaikumar et al., 2018; Zhang et al., 2023). For instance, the transcription factor AtJUB1 positively modulates stress response mechanisms in tomato under drought conditions. It has been demonstrated that AtJUB1 triggers DREB2A gene expression, a crucial transcriptional regulator of drought tolerance, and the DELLA genes GAI and RGL1 (Thirumalaikumar et al., 2018). OsERF71 enhances drought tolerance through augmenting the expression of ABA signaling and proline biosynthesis-correlated genes during drought conditions (Li et al., 2018). Lastly, in dicot DcPIF3 attenuates drought-driven ROS generation, upregulating ABA biosynthetic genes expression to augur increased cellular ABA concentration and thereby boosting drought resistance in carrot (Daucus carota L.) (Wang et al., 2022).
The SBP-box gene, a plant-specific transcription factor, possesses a well-conserved DNA-binding domain, the SBP domain (Klein et al., 1996; Cardon et al., 1999). Comprising approximately 76 amino acid residues, this domain participates in DNA binding and nuclear localization, inclusive of two zinc-binding sites (Yamasaki et al., 2004). Initially identified in Amirrhinum majus, the gene is composed of two subunits, AmSBP1 and AmSBP2, which interact with SQUAMOSA’s promoter to influence early floral development (Klein et al., 1996). Associated research indicates these genes could be pivotal in floral morphogenesis and developmental regulatory networks (Cui et al., 2020; Jeyakumar et al., 2020; Yang et al., 2023; Wei et al., 2024). Additionally, their potential role in abiotic stress adaptation has recently gained attention. In alfalfa, miR156’s pivotal role in enhanced drought resilience was observed via repression of SPL13 and up-regulation of WD40-1, similarly, stimulatory action of miR156 increased drought susceptibility (Arshad et al., 2017; Feyissa et al., 2019). Equally, OsSPL10 regulator rice’s response to salt stress, affecting both OsNAC2 expression and drought-induced ROS production (Lan et al., 2019; Li et al., 2023). The SBP-box gene (CnSBP7/9//10/14/17/19) of Chrysanthemum nankingense also reacts to drought stress (Lan et al., 2019). In Sweet Orange (Citrus sinensis), 15 genes showed response variations under low/high temperature and salt conditions (Song et al., 2021). Notably, the miR156/SPL module stimulates MdWRKY100 expression enhancing salt tolerance in apple (Hu et al., 2023). Furthermore, in pigeon pea (Cajanus cajan) under drought stress, increased levels of CcSPL2.1, 3, 13A were observed while prominent up-regulation of CcSPL14 and 15 was noted in salt-susceptible cultivars (Shaheen et al., 2024). Enhanced TaSPL6 expressions decrease drought tolerance, conversely, decreased TaSPL6 expressions improved drought resistance in wheat (Zhao et al., 2024). Enhanced drought resistance was observed in Arabidopsis overexpressing the ‘SiJiMi’ mango gene, MiSPL3a/b (Zhu et al., 2024).
However, according to our knowledge, no research exists elucidating the function of pepper SBP-box gene in drought stress tolerance. Hence, this study examined the contribution of CaSBP13 gene (Accession No. Capana10g002379) in pepper under drought stress. Results indicate that CaSBP13 suppresses pepper’s drought stress response, potentially via ROS and ABA signaling pathways.
Materials and methods
Plant materials and growth conditions
The plant materials used in this study consisted of the pepper cultivar AA3 and (Nicotiana benthamiana) N. benthamiana, sourced from the Horticultural Landscape Architecture faculty of Henan Institute of Science and Technology, Xinxiang 453003, China. Pepper was grown under controlled conditions (16/8 hour photoperiod, 22°C daytime/18°C nighttime temperature, and 80% humidity). N. benthamiana was cultivated at optimized conditions of 16/8 hour photoperiod, 25°C/18°C day/night temperature, and 60% humidity.
Virus-induced gene silencing of CaSBP13 in pepper
The CaSBP13 gene of pepper was silenced following the VIGS protocol described by Wang in 2013 (Wang, 2013). A 310bp fragment from the pepper CaSBP13 gene was amplified using specific primers, which were verified in both NCBI (http://www.ncbi.nlm.nih.gov/tools/primer-blast/index.cgi?LINK_LOC=BlastHome) and pepper genome databases (http://peppergenome.snu.ac.kr/) (Supplementary Table 1). The PCR product and TRV2 plasmid were cleaved with BamHI and KpnI restriction enzymes, followed by linkage using the T4-DNA ligase (Trans Gen Biotech, Beijing, China). The recombinant vector was sequenced at Sangon Biotech Company (Shanghai, China). The resultant vectors, i.e., TRV2:CaSBP13, TRV2: CaPDS (phytoenedesaturase, positive control), TRV2:00 (negative control), and TRV1, were transduced into Agrobacterium tumefaciens strain GV3101 via freeze-thaw technique.
A total of 400 pepper seedlings at the second true leaf phase (40 days post-germination) were utilized for CaSBP13 gene silencing as per the protocol outlined by Zhang et al. (2013). All seedlings exposed to infection were maintained in a controlled environmental growth chamber as per Wang’s specifications (Wang, 2013). Photo-bleaching noticed in TRV2:CaPDS positive subjects prompted collection of leaf samples from both the CaSBP13-silenced (TRV2:CaSBP13) and control (TRV2:00) plants for assessing efficiency of gene silencing.
Overexpression of CaSBP13 in N. benthamiana
The full-length sequence of CaSBP13 lacking its termination codon was amplified and cloned into a pVBG2307:GFP vector at the XbaI and KpnI restriction sites to form the pVBG2307:CaSBP13:GFP construct (Supplementary Table 1). Confirmation was achieved through sequence analysis by Sangon-Biotech Company (Shanghai, China). Subsequently, the recombinant pVBG2307:CaSBP13:GFP vector was utilized for CaSBP13 overexpression in N. benthamiana. Transgenic N. benthamiana overexpressing CaSBP13 were produced via Agrobacterium tumefaciens-mediated leaf disc transformation (Oh et al., 2005). We identified three kanamycin-resistant CaSBP13 transformants with RNA confirmation. T1 progeny were derived from T0 plants, and T2 progeny from T1 plants. Herein, we chose T3 progeny for further study.
Stress treatments and samples collection
To assess CaSBP13 transcript levels in peppers exposed to drought stress, a total of 200 seedlings with six to eight developed leaves were harvested from a substrate composed of matrix, vermiculite, and perlite (in a 3:1:1 ratio), subsequently reared in 1/2 Hoagland’s solution, and exposed to 20% Polyethylene glycol (PEG6000) after three days. A control group remained in 1/2 Hoagland’s solution only (Li et al., 2022). Leaves from 6-8 plants were collected at time 0h, 3 h, 6 h, 12 h, 24 h, and preserved at −80°C.
For ABA stress, a total of 200 seedlings were treated with 20μM ABA employing the method outlined by Yin et al. (2014). Aqueous solution consisting of 0.5% tween and 0.1% ethanol served as the control for ABA exposure. Leaves from 6-8 plants were harvested at 0, 3, 6, 12, 24, and 48 hours, promptly frozen with liquid nitrogen and preserved at -80°C.
The drought experiments of CaSBP13-silenced and CaSBP13-overexpressing plants were adapted from Liu’s methodology, with minor modifications (Liu et al., 2017). For CaSBP13-silenced plant’s drought treatment required consistent exposure of all peppers in identical environmental conditions. A single deep watering occurred one month prior to the drought treatment, followed by equal watering every three days. After the last watering three days was considered the initiation of drought stress and was denoted as day 0. Samples from 6-8 plants were collected and preserved at −80°C at time intervals of 0, 1, 2, 3, 4 days post-drought.
For CaSBP13-overexpressing plants under drought stress, the same procedure was employed with minor alterations (672 seedlings were used for this experiment): A single deep watering was provided one month before drought, followed by equal watering every four days. The fourth day post the last watering marked day 0 of drought stress. Samples from 6-8 plants were collected and stored at −80°C after post-drought at 0, 2, 4 days intervals.
The detection of seed germination rate and root length for CaSBP13-overexpression plants is according to the method described by Ma et al. (2011). The seeds are immersed in ABA solutions at 0g/L, 0.1g/L, 0.5g/L, and 1g/L concentration gradients for 24 hours, and then placed on double-layered filter paper moistened with deionized water in a culture dish. The dish is maintained in a 25°C light incubator with 16 hours of day and 8 hours of night, with regular ventilation for 10 minutes and added fresh water to maintain moisture daily. Germination counts are recorded on the third, fifth, and tenth days. The seeds of CaSBP13-overexpression plants that germinated without any treatment during the same period undergo identical ABA treatment and cultivation methods. Root length is measured after 10 days treatment.
RNA extraction and real-time quantitative PCR
Total RNA was isolated using the Takara MiniBEST Plant RNA Extraction Kit per manufacturer’s guidelines (Takara, Dalian, China). The first strand synthesis was accomplished with the Prime Script Kit (Takara, Dalian, China). The resultant cDNA solution was standardized to 50 ng/L and utilized for real-time quantitative (qPCR) analysis.
The Bio-Rad iCycler thermocycler (Hercules, CA, USA) performed real-time qPCR as per Zhang et al. (2020) methodology (Zhang et al., 2020). This included a pre-denaturation phase at 95°C for 1 minute, followed by 40 cycles of denaturation (95°C, 10 seconds), annealing (56°C, 30 seconds), and elongation (72°C, 30s). Fluorescence quantification was conducted at each cycle’s completion via post-PCR melting curve analysis ranging from 56 to 95°C for assessing primer specificity. Pepper ubiquitin-binding protein gene (CaUBI3) served as a reference while Nicotiana benthamiana actin gene, Nbactin-97, functioned as a control in Nicotiana benthamiana experiments (Du et al., 2015; Zhang et al., 2016). All primer pairs were verified through NCBI Primer BLAST. The specificities of these primers are provided in Supplementary Table 1. Gene expression was quantified utilizing the 2-△△CT method (Schmittgen and Livak, 2008).
Physiological indicators measurement
The determination of malondialdehyde (MDA), relative electrical conductivity, and relative water content (RWC) was performed per Zhang et al. (2018) and Pan et al. (2012). Water loss calculations followed Ma et al. (2021) protocol. Chlorophyll content quantification used an approach detailed by Arkus et al. (2005). The activities of peroxidase (POD), superoxide dismutase (SOD), and catalase (CAT) were examined per Zhang et al. (2018) and Stewart and Bewley (1980).
The methods of DAB and NBT staining for hydrogen peroxide (H2O2) and oxygen (O2-) radical analysis were adapted from Kim et al. (2012) and Thordal-Christensen et al. (1997). Their stain areas were quantified based on Sekulska-nalewajko et al. (2016). H2O2 content was determined using Liu et al. (2010). Superoxide anion (O2-) detection is executed as per Solarbio Superoxide Anion kit instructions (Solarbio, Beijing, China).
Scanning electron microscopy (SEM), FEI Quanta 200 (USA), allowed a high resolution investigation of stomata morphologies. The imaging was quantified using Image J software from the National Institutes of Health. The stomatal apertures was also detected using SEM. The stomatal aperture size includes the length and width of pores, with the pore length determined in this test as the dumbbell-shape aperturae. Pore width denotes the maximum value perpendicular to the dumbbell-shape aperturae.
Statistical analysis
Statistical evaluation was executed using SPSS 22.0 software. One-way ANOVA evaluated treatment variations. Significant distinctions were established at P ≤ 0.05 and P ≤0.01 utilizing Tukey’s post hoc test. Data are showcased as mean ± SD (standard deviation). All experiments were independently conducted, with a minimum of three biological replicates.
Results
Expression of the CaSBP13 gene in pepper under drought and ABA stress
To explore the role of CaSBP13 in drought stress, we evaluated its transcriptional profile post-stressor. As revealed in Supplementary Figure 1, the CaSBP13 gene displays significant increases post-drought stress, peaking at 6 hours post-stress. Further, the expression of CaSBP13 fluctuates, notably attenuating at 12h after ABA treatment. These data suggest a plausible involvement of CaSBP13 in drought stress and ABA treatment response.
Silencing the CaSBP13 gene improved pepper plant resilience to drought stress.
To further investigate the function of CaSBP13 under drought stress, it was silenced utilizing the virus-induced gene silencing method (Wang, 2013). Here, a positive control vector, TRV2:CaPDS, silenced the CaPDS gene, resulting in photo-bleached leaves. Meanwhile, TRV2:00 served as a negative control. At the appearance of photo-bleached leaves in TRV2:CaPDS plants, the silencing efficacy of TRV2:CaSBP13 and TRV2:00 was examined (see Supplementary Figure 2). It shows morphologically identical states between CaSBP13-silenced (TRV2:CaSBP13) and control (TRV2:00) plants under normal conditions, attesting to over 94% silencing efficiency. Consequently, both CaSBP13-silenced and control plants were selected for further analysis.
After three days of drought stress, a wilt was observed in lower leaves of CaSBP13-silenced plants. In contrast, the leaves of control plants displayed almost complete wilt (Figure 1A). Upon drought stress, both CaSBP13-silenced and control plants exhibited a decrease in chlorophyll content; however, the former had significantly greater amounts than the latter (Figure 1B). Additionally, the water loss rate of CaSBP13-silenced plants was distinctly lower than control plants at two days of drought, exhibited a complex pattern (Figure 1C). Similarly, the relative electrical conductivity of CaSBP13-silenced and control plants trended upward, yet control plants consistently surpassed CaSBP13-silenced plants, especially on Days 1 and 2 (Figure 1C). The MDA content of both plant types showed an ascending-descending trajectory, with control plants exhibiting higher levels on Days 1 and 2 (Figure 1C). POD, CAT, and SOD activity in CaSBP13-silenced and control plants initially rose, then declined, with CAT activity being significantly higher in CaSBP13-silenced plants than control ones. POD activity was notably higher in CaSBP13-silenced plants than control plants at day 1, while SOD activity followed the opposite trend compared to POD activity (Figure 1C). These findings indicate enhanced drought tolerance in CaSBP13-silenced plants.
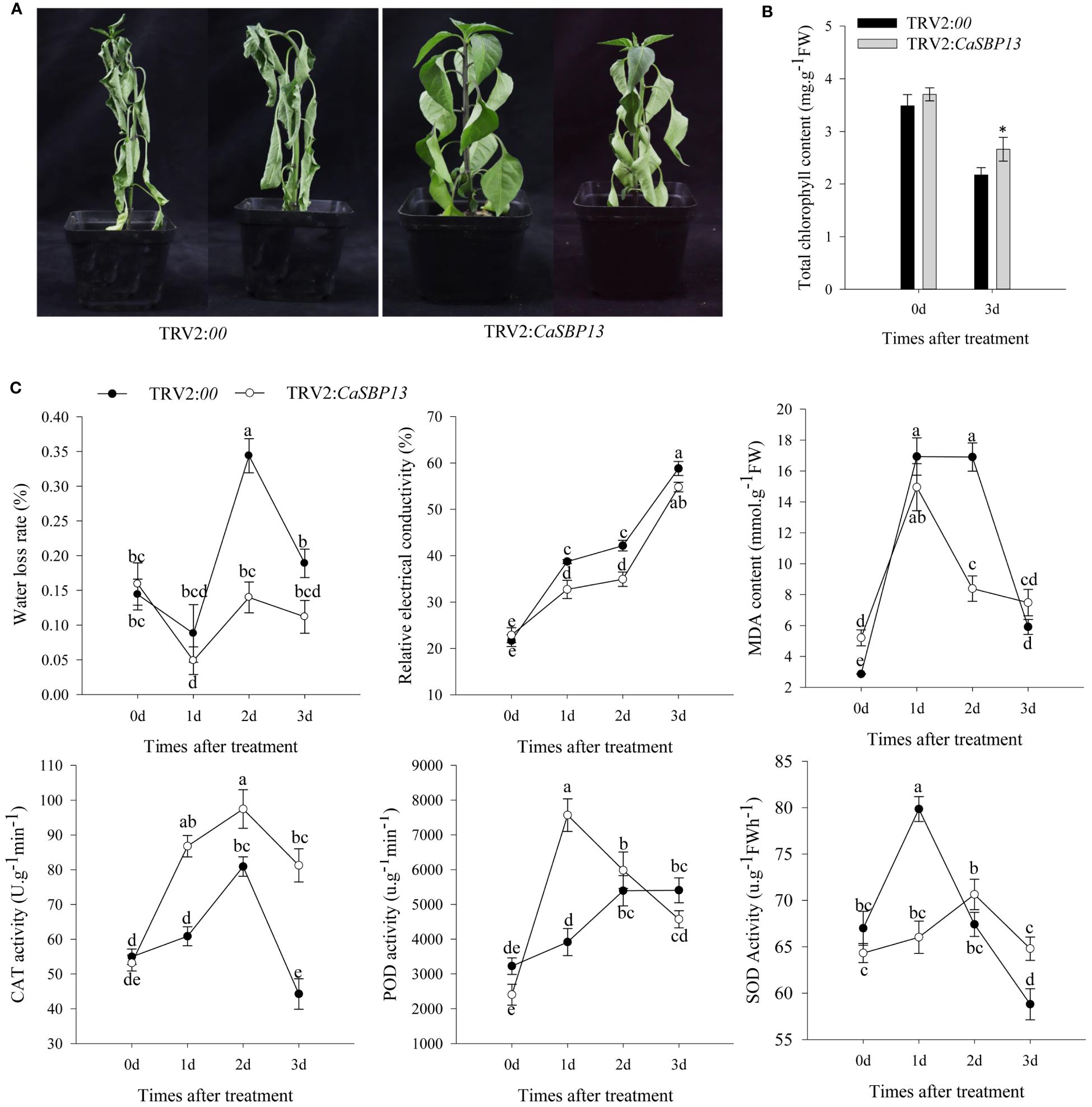
Figure 1 Identifying drought tolerance of CaSBP13-silenced plants. (A) Phenotypes of the CaSBP13-silenced and control plants after three days of drought stress. (B) The total chlorophyll content of CaSBP13-silenced and control plants after three days of drought stress. (C) The water loss rate, relative electrical conductivity, malondialdehyde (MDA) content, peroxidase (POD), catalase (CAT) and superoxide dismutase (SOD) activities of CaSBP13-silenced and control plants after drought stress. d: day. One-way ANOVA was employed to examine the differences between treatments, significant variances were identified via Tukey’s post hoc test. *represent significant differences at P ≤ 0.05. Bars with different letters indicate significant differences at P ≤ 0.05. Mean values and SDs for three replicates are shown.
Furthermore, to evaluate the increase of reactive oxygen species (ROS) in both CaSBP13-silenced and control plants under drought stress, hydrogen peroxide (H2O2) and superoxide anion (O2-) levels were measured using DAB and NBT staining (Figures 2A–D). After four days of drought, significant differences were observed between control and CaSBP13-silenced plants for leaf area displaying DAB and NBT staining (Figures 2A–D), suggesting higher H2O2 and O2- in controls (Figures 2E, F). Also, considering relative stomatal density, control plants had a higher number than CaSBP13-silenced plants (Figures 2G, H). Besides, post drought stress four days, stomatal length and width decrease. However, these parameters in the CaSBP13-silenced plants are notably larger than those of the control plants (Figures 2I, J). This indicated lower ROS accumulation in CaSBP13-silenced leaves compared to controls.
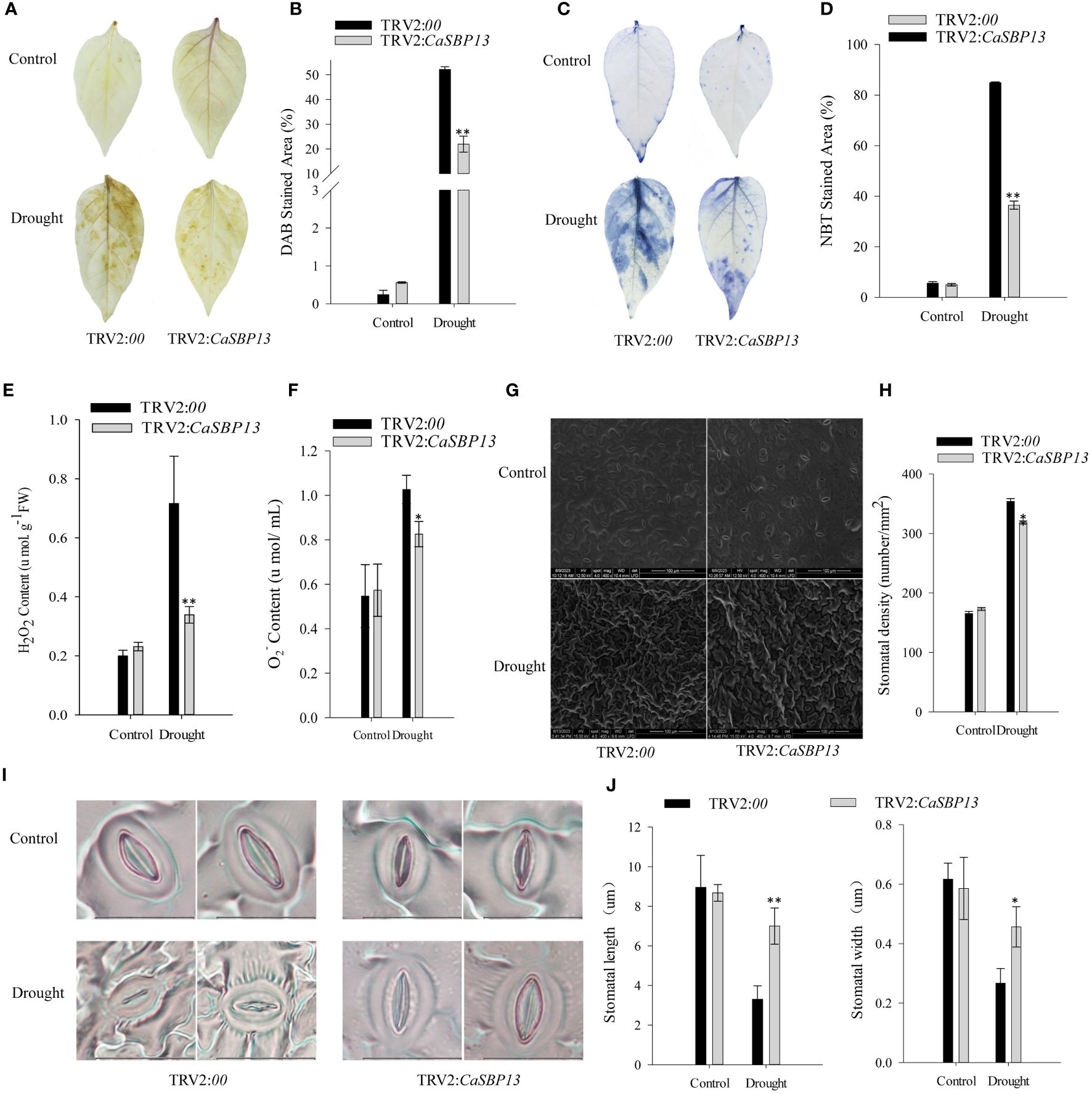
Figure 2 DAB and NBT staining of CaSBP13-silenced and control plants, coupled with quantification of stomatal density and apertures. (A) DAB staining in leaves of CaSBP13-silenced and control plants after four days of drought stress. (B) DAB stained area of CaSBP13-silenced and control plants after four days of drought stress. (C) NBT staining in leaves of CaSBP13-silenced and control plants after four days of drought stress. (D) NBT stained area of CaSBP13-silenced and control plants after four days of drought stress. (E) The H2O2 content of CaSBP13-silenced and control plants after four days of drought stress. (F) The O2- content of CaSBP13-silenced and control plants after four days of drought stress. (G, H) stomatal density assessment. Scale bar, 100 µm. (I, J) The morphological features and length/width of stomata. Scale bar, 20 µm. One-way ANOVA was employed to examine the differences between treatments, significant variances were identified via Tukey’s post hoc test. * and ** represent significant differences at P ≤ 0.05 and P ≤0.01 respectively. Mean values and SDs for three replicates are shown.
To further analyzes, gene expressions of ROS scavenging enzymes (including CaAPX1, CaCAT2, CaSOD, and CaPOD) and key genes in ABA signaling pathway modeling (such as CaPYL9, CaPP2C, CaAREB, and CaSNRK2.4) were examined, as Figure 3 illustrates. At Day 4 of drought stress, the expression of CaPOD in CaSBP13-silenced plants was significantly lower compared to control, while others (CaAPX1, CaCAT2, CaSOD) were notably higher (Figure 3). Moreover, we assessed the expression dynamics of these key genes in the ABA signaling pathway. As suggested in Figure 3, following 4 days of drought stress, CaPYL9 and CaSNRK2.4 manifested higher expression in CaSBP13-silenced plants, while CaPP2C and CaAREB displayed lower expression in these plants compared to the control. Significantly, even without treatment, the expression of CaPOD, CaAPX1, CaPP2C, and CaAREB expression was higher in the CaSBP13-silenced plants compared to the control plants (Figure 3).
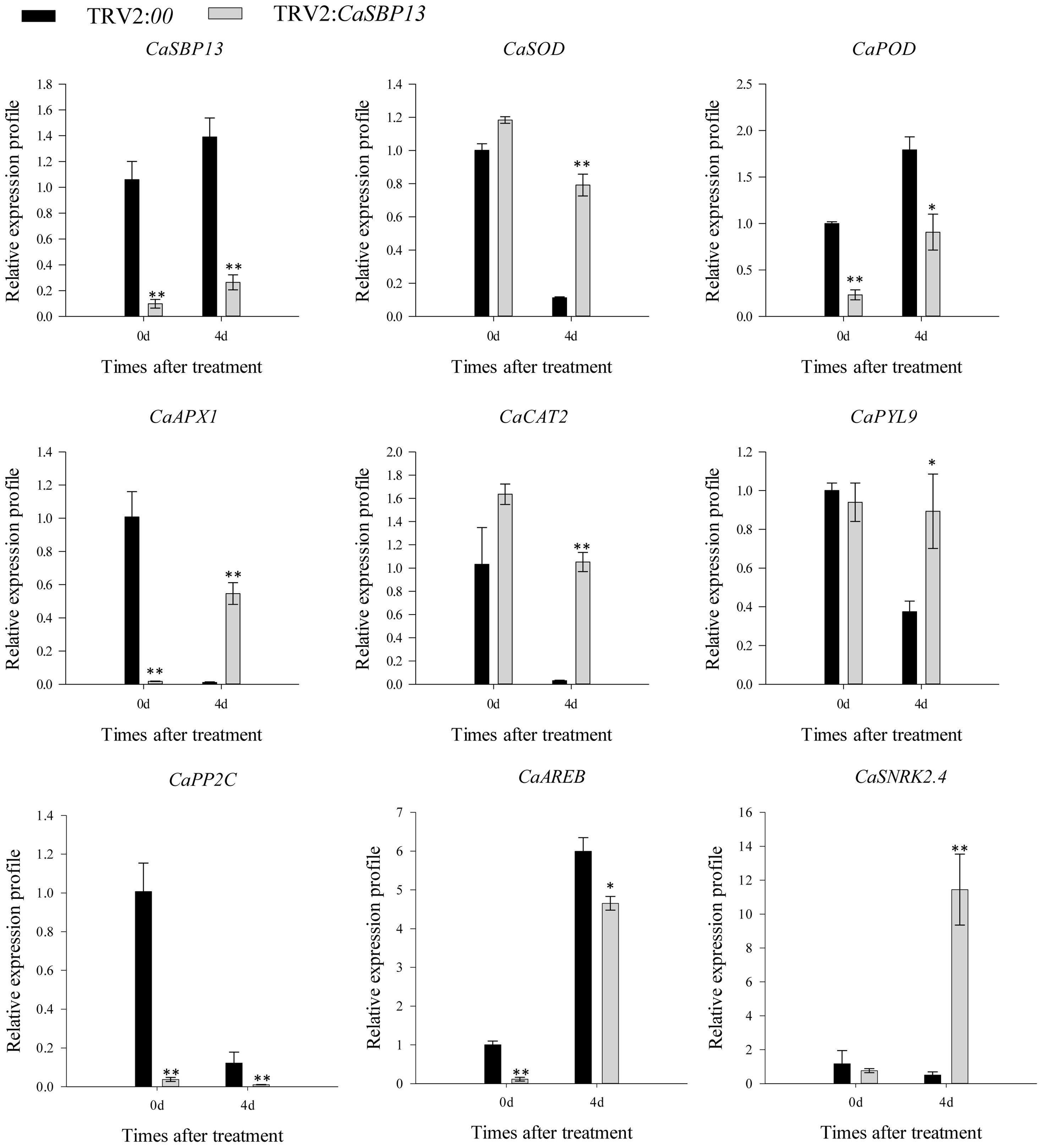
Figure 3 The expression of ROS-scavenging enzymes related genes and the key genes of ABA signaling pathway after drought stress in CaSBP13-silenced and control plants. One-way ANOVA was employed to examine the differences between treatments, significant variances were identified via Tukey’s post hoc test. * and ** represent significant differences at P ≤ 0.05 and P ≤0.01 respectively. Mean values and SDs for three replicates are shown.
Overexpression of CaSBP13 notably compromises drought tolerance in Nicotiana Benthamiana
To further study the effects of CaSBP13 on drought resistance, it was overexpressed in Nicotiana Benthamiana. Transgenic lines 1, 10, and 16 overexpressing CaSBP13 were chosen for subsequent analysis. Their expression levels are shown in Supplementary Figure 3. Following 7 days of drought, both transgenics and wild-types exhibited wilting (Figure 4A). Notably, the lower leaves of the transgenics display severe wilting and yellowing compared to the wild-type (Figure 4A). Similarly, at day 4 of drought, the chlorophyll content of the transgenics was markedly diminished compared to the wild-type (Figure 4B). Moreover, their relative electrical conductivity and MDA content were higher than the wild-type plants (Figure 4C). Over time during drought, the relative water content of the transgenics decreased significantly, reaching a notable decline by day 9 of drought stress (Figure 4C). Furthermore, at day 4 of drought, the DAB and NBT staining areas of the transgenics were notably larger than the wild-type plant (Figures 5A–C). Correspondingly, the H2O2 and O2- content in the transgenics was drastically higher than the wild-type (Figure 5C). Additionally, the stomatal density of the transgenics was significantly elevated compared to the wild-type (Figures 5D, E). Besides, both the length and width of stomata in both transgenics plants and wild-type plants decreased, but these variables in the wild-type plants significantly surpassed those in the transgenics plants (Figures 5F, G).
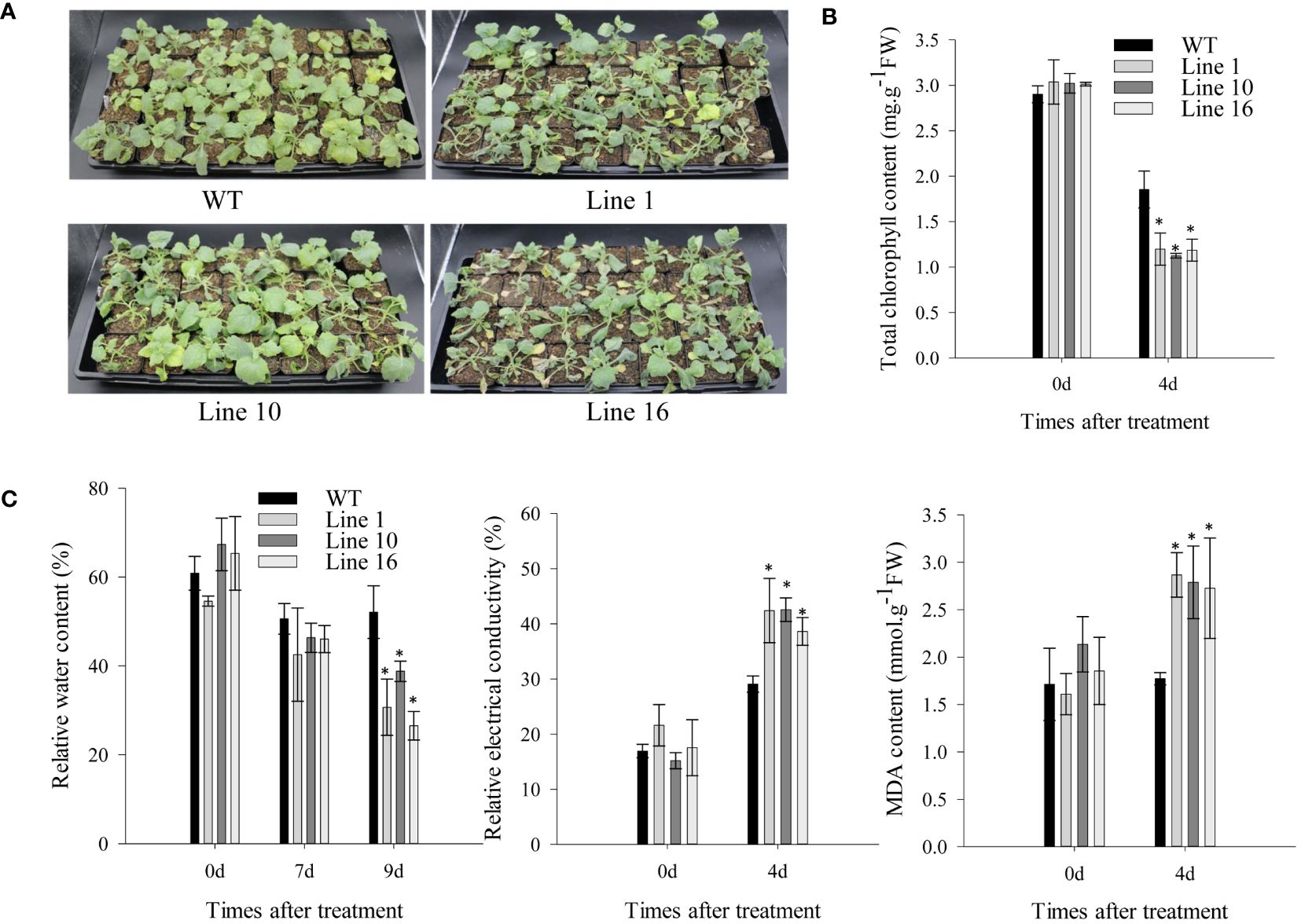
Figure 4 Overexpression of CaSBP13 in Nicotiana Benthamiana enhanced susceptibility to drought stress. (A) The phenotype of transgenic and wild-type lines under seven days of drought stress. (B) The total chlorophyll content of transgenic and wild-type lines after four days of drought stress. (C) The relative water content, relative electrical conductivity, malondialdehyde (MDA) content of transgenic and wild-type lines after four days of drought stress. One-way ANOVA was employed to examine the differences between treatments, significant variances were identified via Tukey’s post hoc test. *represent significant differences at P ≤ 0.05. Mean values and SDs for three replicates are shown.
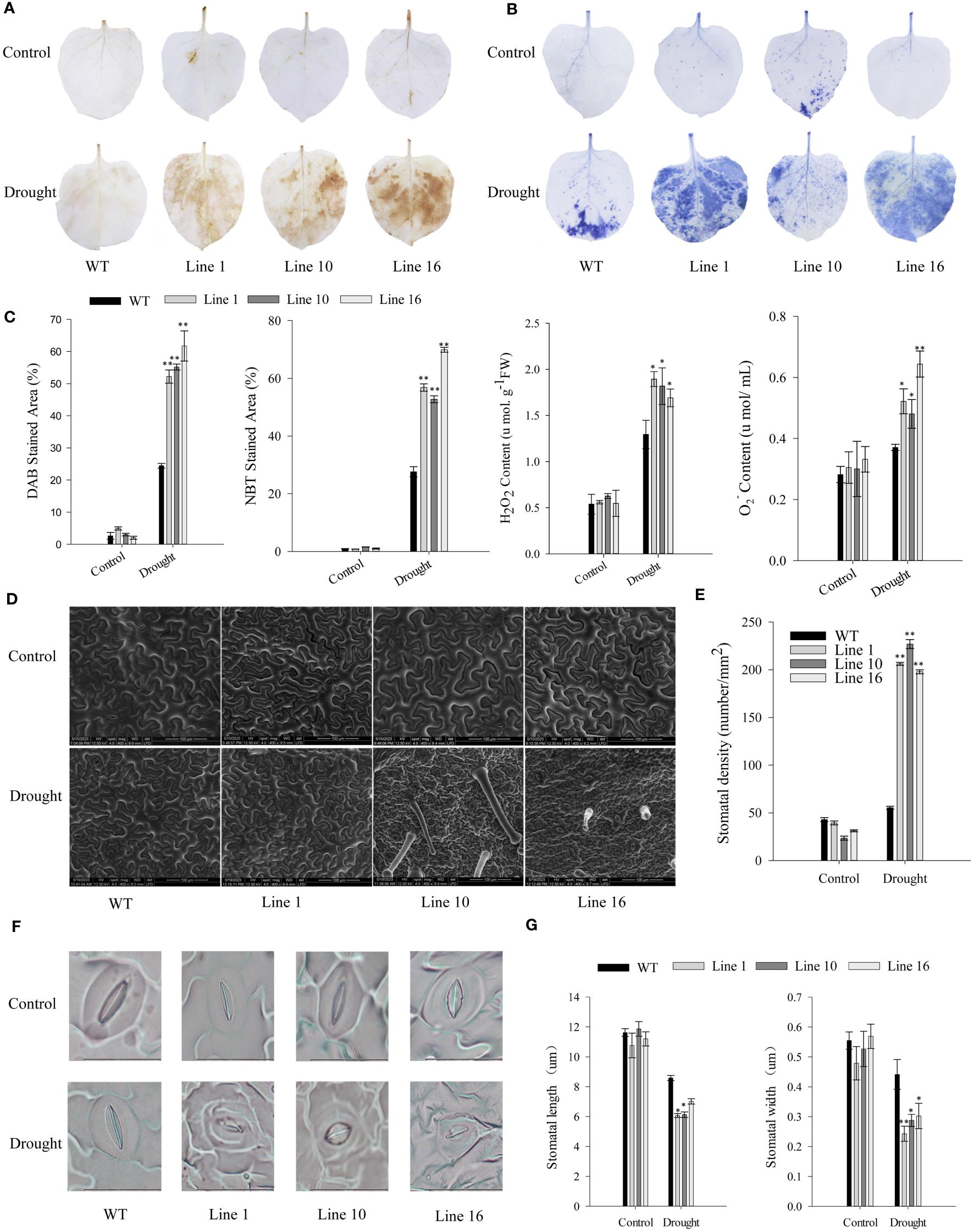
Figure 5 DAB and NBT staining of transgenic and wild-type lines, coupled with quantification of stomatal density. (A) DAB staining in leaves of transgenic and wild-type lines after four days of drought stress. (B) NBT staining in leaves of transgenic and wild-type lines after four days of drought stress. (C) DAB and NBT stained area of transgenic and wild-type lines after four days of drought stress. The H2O2 and O2- content of transgenic and wild-type lines after four days of drought stress. (D, E) stomatal density assessment. Scale bar, 100 µm. (F, G) The morphological features and length/width of stomata. Scale bar, 20 µm. One-way ANOVA was employed to examine the differences between treatments, significant variances were identified via Tukey’s post hoc test. * and ** represent significant differences at P ≤ 0.05 and P ≤0.01 respectively. Mean values and SDs for three replicates are shown.
Based on the above results, we suggest that CaSBP13’s function under drought stress may be related to active oxygen and ABA signaling pathways. Therefore, we evaluated the expression of genes involved in active oxygen metabolism and the ABA core pathway, and assessed germination and root growth of the transgenic plants under varying ABA concentration gradients. When subjected to drought stress for four days, the expression of NbSOD, NbAPX, and NbCAT1 was significantly lower in the transgenics than in the wild-type (Figure 6). Conversely, the expression of NbPOD was augmented compared to the wild-type. Of particular note, the expression of critical genes in the ABA signaling pathway model such as NbPYL9, NbSRK2E, NbPP2C, and NbSNRK2.4 was significantly reduced in the transgenics compared to the wild-type (Figure 6). However, the expression of NbAREB in the transgenics was notably higher than in the wild-type. Without external intervention, the expression of NbSOD, NbAPX, NbPOD, NbCAT1, NbPYL9, and NbAREB genes was significantly lower in the transgenics than in the wild-type (Figure 6). Furthermore, the germination rate of trangenics and wild-type plants declined with increasing ABA concentration. Under 0g/LABA treatment, both types germinated rapidly, achieving full germination by day 5. At 0.1g/LABA, the germination rate of trangenics plants was significantly higher than that of wild-type plants on day 3, achieving full germination by day 5. Similarly, at 0.5g/LABA, the germination rate of trangenics plants was significantly higher than that of wild-type plants on day 3, achieving full germination by day 10. Lastly, under 1g/LABA, the germination rate of trangenics plants was significantly higher than that of wild-type plants on day 5, achieving full germination by day 10 (Supplementary Table 2). Additionally, the root length of trangenics and wild plants decreased with increasing treatment concentration in different concentration gradients, but the root length of trangenics plants was significantly higher than that of wild-type plants under 0.1g/LABA, 0.5g/LABA, and 1g/LABA treatments (Supplementary Figures 4A, B). Overall, these findings suggest that overexpression of CaSBP13 in Nicotiana Benthamiana exacerbates the vulnerability of the transgenic plants to drought stress.
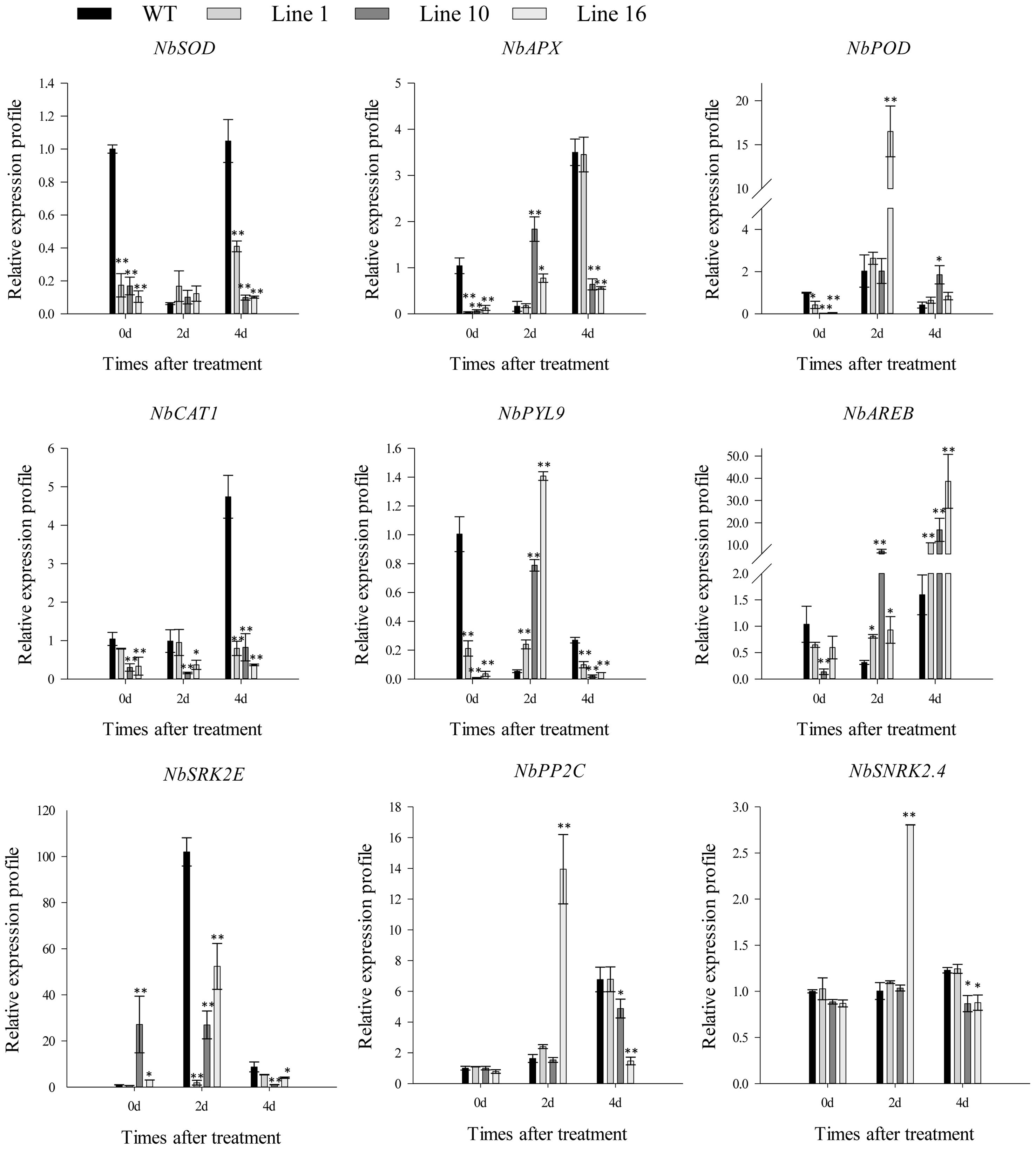
Figure 6 The expression of ROS-scavenging enzymes related genes and the key genes of ABA signaling pathway after drought stress in transgenic and wild-type lines. One-way ANOVA was employed to examine the differences between treatments, significant variances were identified via Tukey’s post hoc test. * and ** represent significant differences at P ≤ 0.05 and P ≤ 0.01 respectively. Mean values and SDs for three replicates are shown.
Discussion
The SBP-box gene, a specific transcription factor in plants, influences plant growth, signaling, and stress responses. However, its function under drought stress in pepper remains elusive. Here, we demonstrate that one SBP-box gene, CaSBP13, suppresses plant defense against drought stress.
The CaSBP13 amino acid sequence contains all the features of typical SBP-box proteins including two zinc finger-like structures (C3H, C2HC) and a putative nuclear localization signal (Zhang et al., 2016). Furthermore, prior experiments demonstrate that CaSBP13 localizes to the nucleus (Zhang, 2020). Besides, it can be induced by drought stress and suppressed by ABA treatment at 12h (Supplementary Figure 1). Additionally, silencing CaSBP13 enhanced plant tolerance to drought stress (Figure 1). It has been reported that under drought conditions, elevated levels of reactive oxygen species (ROS) accumulate in plants, causing oxidative damage to proteins, carbohydrates, lipids and DNA. This initiates an antioxidant defense mechanism, generating enzymes such as catalase (CAT), superoxide dismutase (SOD), and peroxidase (POD) to counteract ROS damage (Gill and Tuteja, 2010). Moderate drought induces stomatal opening, but prolonged drought leads to closure due to ABA stimulation, reducing transpiration (Ashraf, 2010). In this study, it was observed that CAT activities in CaSBP13-silenced plants surpassed those in controls. Additionally, the peak of SOD activity appeared earlier in controls than in CaSBP13-silenced plants (Figure 1C). However, the peak of POD activity appeared later and lower in control than in CaSBP13-silenced plants (Figure 1C). Remarkably, CaSBP13-silenced plants accumulation of H2O2 and O2- was lower than controls (Figure 2). Increased stomatal density was observed in both silenced and control plants, though controls had a higher count (Figures 2G, H). Furthermore, post-drought stress, changes in ROS-scavenging enzymes including CaAPX1, CaPOD, CaCAT2, and CaSOD were detected in CaSBP13-silenced plants. After 4 days of drought stress, the expression of CaAPX1, CaSOD, CaCAT2 was elevated compared to the control, except for CaPOD (Figure 3). Escalation of BpSPL9 immune response supported ROS scavenging via peroxidase (POD) and superoxide dismutase (SOD) enzymes, augmenting plant resilience to drought and salt stress (Ning et al., 2017). Overexpression of OsSPL10 appeared pivotal in drought resilience by controlling ROS generation and stomatal functions (Li et al., 2023). Over-expression of TaSPL6-A decreased wheat drought resilience, signifying unrestrained ROS elevation (Zhao et al., 2024). Augmentation of the ‘SiJiMi’ mango gene MiSPL3a/b in Arabidopsis enhanced drought resistance in transgenics (Zhu et al., 2024). Identifying higher stomatal densities in the CaSBP13-silenced plants, we suggest the role of drought tolerance by CaSBP13 may encompass the ABA signaling pathway. Consequently, expression elevations of CaPYL9, and CaSNRK2.4 were observed in CaSBP13-silenced plants post drought stress (Figure 3). Conversely, expressions of CaPP2C, and CaAREB were reduced in CaSBP13-silenced plants compared to control plants. Notably, the expression of CaPOD, CaAPX1, CaPP2C, and CaAREB was markedly lessened in CaSBP13-silenced plants under normal conditions, suggesting a regulatory role for CaSBP13 during drought stress. In the ABA signal pathway, there are three critical components: PYL, an ABA receptor, PP2C, a key negative regulator, and SnRK2, a significant positive mediator. These components establish a complex interplay to manipulate ABA signal transduction and responses, forming a dual negative regulatory system (PYL-PP2C-SnRK2) (Sun et al., 2020). Under non-stressful conditions, where ABA levels are restricted, PYL does not bind to ABA. PP2C suppresses the activation of SnRK2 by dephosphorylation, halting the activation of transcription factors ABF/AREB upon ABA perception, resulting in pathway inactivation. However, under severe conditions such as drought, salinity or elevated temperature, the fast accumulation of plant ABA incites the formation of a PYL-ABA complex, initiating intermolecular interactions between PYL and PP2C. This impedes dephosphorylation of SnRK2 by PP2C, thereby releasing the suppression of PP2C on SnRK2. Phosphorylated SnRK2 subsequently stimulates or suppresses the expression of various downstream transcriptional regulators or effectors, initiating ABA signal transduction and regulating plant growth, development, or stress response (Cutler et al., 2010; Zhao et al., 2013). Besides, it has been reported that phosphorylation of CaNAC035 by CaSnRK2.4 modulates abscisic acid synthesis in pepper under cold stress (Zhang et al., 2023). To validate CaSBP13’s role in plant drought stress response, N. benthamiana overexpressing CaSBP13 was engineered. These transgenic plants exhibited heightened susceptibility to drought stress, exhibiting increased relative electrical conductivity, MDA concentration, and active oxygen accumulation (Figures 4, 5). Notably, the transgenic plants had significantly reduced expression of NbSOD, NbAPX, and NbCAT1 compared to wild-type plants post-4 days of drought stress, mirroring previous pepper research findings (Figures 3, 6). Correspondingly, the levels of NbPYL9 and NbSNRK2.4 in the transgenic plants were diminished at day 4 compared to wild type plants, along with a marked upregulation of NbAREB, corroborating predictions from pepper studies (Figures 3, 6). In contrast, without any treatments conditions, NbSOD, NbAPX, NbPOD, NbCAT1, NbAREB, and NbPYL9 gene expressions in the transgenic plants were notably lower than wild type plants (Figure 6). Moreover, both the germination rate and root length of the transgenic and wild-type plants were suppressed under varying ABA concentrations. However, the wild-type plants exhibited a greater response to ABA treatment than the transgenic plants(Supplementary Table 2, Supplementary Figure 4). Therefore, we propose that the CaSBP13 gene enhances plant drought resistance via ROS and ABA signaling pathways. Nevertheless, further empirical validation is necessary to confirm these proposals.
Conclusions
In conclusion, the expression of CaSBP13 gene rises during drought stress in pepper. Silencing CaSBP13 improves plants drought resistance witch lowering ROS production compared to control plants. However, CaSBP13 overexpression in N. benthamiana intensifies drought sensitivity and ROS production in comparison to wild-type plants. Four days post drought stress, CaAPX1, CaCAT2, and CaSOD expression is heightened in CaSBP13-silenced plants compared to controls, while CaPOD expression is reduced. Also, NbCAT1, NbSOD and NbCAT1 transcripts decrease while NbPOD transcription increases in CaSBP13-overexpressiing plants. Remarkably, under nonstressed conditions, CaPP2C, and CaAREB transcripts of the ABA signaling pathway are significantly reduced in CaSBP13-silenced plants. Similarly, NbAREB, and NbPYL9 transcripts of the ABA signaling pathway are lower in transgenics than wild-types plants under similar conditions. These findings suggest a negative effect of CaSBP13 on plant drought tolerance possibly linked to its role in the ROS- and ABA-signaling pathways. Additional experimentation is needed to elucidate these mechanisms.
Data availability statement
The original contributions presented in the study are included in the article/Supplementary Material. Further inquiries can be directed to the corresponding author.
Author contributions
H-XZ: Conceptualization, Data curation, Formal analysis, Funding acquisition, Investigation, Methodology, Project administration, Resources, Supervision, Validation, Visualization, Writing – original draft, Writing – review & editing. YZ: Data curation, Formal analysis, Methodology, Writing – review & editing. B-WZ: Data curation, Methodology, Software, Writing – review & editing.
Funding
The author(s) declare financial support was received for the research, authorship, and/or publication of this article. This work was supported through funding from the National Natural Science Foundation of China (No.32202492) and Henan Province Science and Technology Research Projects (No.242102111159) and Introduction of leading talents and construction of high-level talent teams (No.103020221002/014) and K Teacher Team Doctoral Program Construction Project (No.103020222001/050)and High level Talent Introduction Program Project 2 (No.103020224001/009).
Conflict of interest
The authors declare that the research was conducted in the absence of any commercial or financial relationships that could be construed as a potential conflict of interest.
Publisher’s note
All claims expressed in this article are solely those of the authors and do not necessarily represent those of their affiliated organizations, or those of the publisher, the editors and the reviewers. Any product that may be evaluated in this article, or claim that may be made by its manufacturer, is not guaranteed or endorsed by the publisher.
Supplementary material
The Supplementary Material for this article can be found online at: https://www.frontiersin.org/articles/10.3389/fpls.2024.1412685/full#supplementary-material
References
Abbas, K., Li, J., Gong, B., Lu, Y., Wu, X., Lü, G., et al. (2023). Drought stress tolerance in vegetables: The functional role of structural features, key gene pathways, and exogenous hormones. Int. J. Mol. Sci. 24, 13876. doi: 10.3390/ijms241813876
Arkus, K. A. J., Cahoon, E. B., Jez, J. M. (2005). Mechanistic analysis of wheat chlorophyllase. Arch. Biochem. Biophys. 438, 146–155. doi: 10.1016/j.abb.2005.04.019
Arshad, M., Feyissa, B. A., Amyot, L., Aung, B., Hannoufa, A. (2017). MicroRNA156 improves drought stress tolerance in alfalfa (Medicago sativa) by silencing SPL13. Plant Sci. 258, 122–136. doi: 10.1016/j.plantsci.2017.01.018
Ashraf, M. (2010). Inducing drought tolerance in plants: Recent advances. Biotechnol. Adv. 28, 169–183. doi: 10.1016/j.biotechadv.2009.11.005
Cardon, G., Höhmann, S., Klein, J., Nettesheim, K., Saedler, H., Huijser, P. (1999). Molecular characterisation of the Arabidopsis SBP-box genes. Gene 237, 91–104. doi: 10.1016/S0378-1119(99)00308-X
Cui, L., Zheng, F., Wang, J., Zhang, C., Xiao, F., Ye, J., et al. (2020). miR156a-targeted SBP-Box transcription factor SlSPL13 regulates inflorescence morphogenesis by directly activating SFT in tomato. Plant Biotechnol. J. 18, 1670–1682. doi: 10.1111/pbi.13331
Cutler, S. R., Rodriguez, P. L., Finkelstein, R. R., Abrams, S. R. (2010). Abscisic acid: Emergence of a core signaling network. Annu. Rev. Plant Biol. 61, 651–679. doi: 10.1146/annurev-arplant-042809-112122
Du, Y., Berg, J., Govers, F., Bouwmeester, K. (2015). Immune activation mediated by the late blight resistance protein R1 requires nuclear localization of R1 and the effector AVR1. New Phytol. 207, 735–747. doi: 10.1111/nph.13355
Feyissa, B. A., Arshad, M., Gruber, M. Y., Kohalmi, S. E., Hannoufa, A. (2019). The interplay between miR156/SPL13 and DFR/WD40-1 regulate drought tolerance in alfalfa. BMC Plant Biol. 19, 1–19. doi: 10.1186/s12870-019-2059-5
Gill, S. S., Tuteja, N. (2010). Reactive oxygen species and antioxidant machinery in abiotic stress tolerance in crop plants. Plant Physiol. Biochem. 48, 909–930. doi: 10.1016/j.plaphy.2010.08.016
Hu, L., Qi, P., Peper, A., Kong, F., Yao, Y., Yang, L. (2023). Distinct function of SPL genes in age-related resistance in Arabidopsis. PLoS Pathog. 19, 1–21. doi: 10.1371/journal.ppat.1011218
Jeyakumar, J. M. J., Ali, A., Wang, W. M., Thiruvengadam, M. (2020). Characterizing the role of the miR156-SPL network in plant development and stress response. Plants 9, 1–15. doi: 10.3390/plants9091206
Kim, J. M., Woo, D. H., Kim, S. H., Lee, S. Y., Park, H. Y., Seok, H. Y., et al. (2012). Arabidopsis MKKK20 is involved in osmotic stress response via regulation of MPK6 activity. Plant Cell Rep. 31, 217–224. doi: 10.1007/s00299-011-1157-0
Klein, J., Saedler, H., Huijser, P. (1996). A new family of DNA binding proteins includes putative transcriptional regulators of the Antirrhinum majus floral meristem identity gene SQUAMOSA. Mol. Gen. Genet. 250, 7–16. doi: 10.1007/BF02191820
Lan, T., Zheng, Y., Su, Z., Yu, S., Song, H., Zheng, X., et al. (2019). OsSPL10, a SBP-Box gene, plays a dual role in salt tolerance and trichome formation in rice (Oryza sativa L.). G3 Genes Genomes Genet. 9, 4107–4114. doi: 10.1534/g3.119.400700
Li, J., Guo, X., Zhang, M., Wang, X., Zhao, Y., Yin, Z., et al. (2018). OsERF71 confers drought tolerance via modulating ABA signaling and proline biosynthesis. Plant Sci. 270, 131–139. doi: 10.1016/j.plantsci.2018.01.017
Li, J.-Q., Shi-Lei, L., Shuai-Lei, Z., Wen-Yuan, Z., Guo-Bin, Z. (2022). Genome-wide identification of pepper OSCA gene family and expression analysis under different stress conditions. Plant Sci. J. 40, 187–196. doi: 10.1193/PSJ.2095-0837.2022.20187
Li, Y., Han, S., Sun, X., Khan, N. U., Zhong, Q., Zhang, Z., et al. (2023). Variations in OsSPL10 confer drought tolerance by directly regulating OsNAC2 expression and ROS production in rice. J. Integr. Plant Biol. . 65, 918–933. doi: 10.1111/jipb.13414
Liu, X. W., Chen, Z. L., Shen, J. M., Ye, M. M., Chen, W. H. (2010). Spectrophotometric determination of low concentration of hydrogen peroxide in O3/H2O2 system using titanium sulfate. China Water Wastewater 26, 126–129. doi: 10.19853/j.zgjsps.1000-4602.2010.16.035
Liu, Y., Huang, W., Xian, Z., Hu, N., Lin, D., Ren, H., et al. (2017). Overexpression of slGRAS40 in tomato enhances tolerance to abiotic stresses and influences auxin and gibberellin signaling. Front. Plant Sci. 8. doi: 10.3389/fpls.2017.01659
Ma, W., Cui, H., Li, Y., Zheng, Y., Hu, J. (2011). Effects of Seed Soaking with Different Agents on Seed Germination and Seedling Growth in Tobacco (Nicotiana tabacum L .) under Low Temperature Stress. Bull. Sci. andTechnology 27, 873–880. doi: 10.13774/j.cnki.kjtb.2011.06.008
Ma, Y., Xue, H., Zhang, F., Jiang, Q., Yang, S., Yue, P., et al. (2021). The miR156/SPL module regulates apple salt stress tolerance by activating MdWRKY100 expression. Plant Biotechnol. J. 19, 311–323. doi: 10.1111/pbi.13464
Ning, K., Chen, S., Huang, H., Jiang, J., Yuan, H., Li, H. (2017). Molecular characterization and expression analysis of the SPL gene family with BpSPL9 transgenic lines found to confer tolerance to abiotic stress in Betula platyphylla Suk. Plant Cell. Tissue Organ Cult. 130, 469–481. doi: 10.1007/s11240-017-1226-3
Oh, S. K., Jeong, M. P., Young, H. J., Lee, S., Chung, E., Kim, S. Y., et al. (2005). A plant EPF-type zinc-finger protein, CaPIF1, involved in defence against pathogens. Mol. Plant Pathol. 6, 269–285. doi: 10.1111/j.1364-3703.2005.00284.x
Pan, Y., Seymour, G. B., Lu, C., Hu, Z., Chen, X., Chen, G. (2012). An ethylene response factor (ERF5) promoting adaptation to drought and salt tolerance in tomato. Plant Cell Rep. 31, 349–360. doi: 10.1007/s00299-011-1170-3
Schmittgen, T. D., Livak, K. J. (2008). Analyzing real-time PCR data by the comparative C-T method. Nat. Protoc. 3, 1101–1108. doi: 10.1038/nprot.2008.73
Sekulska-nalewajko, J., Gocławski, J., Chojak-koz, J. (2016). Automated image analysis for quantification of reactive oxygen species in plant leaves. Methods 109, 114–122. doi: 10.1016/j.ymeth.2016.05.018
Shaheen, T., Rehman, A., Abeed, A. H. A., Waqas, M., Aslam, A., Azeem, F., et al. (2024). Identification and expression analysis of SBP-Box-like (SPL) gene family disclose their contribution to abiotic stress and flower budding in pigeon pea (Cajanus cajan). Funct. Plant Biol. . 51, FP23237. doi: 10.1071/FP23237
Song, N., Cheng, Y., Peng, W., Peng, E., Zhao, Z., Liu, T., et al. (2021). Genome-wide characterization and expression analysis of the sbp-box gene family in sweet orange (Citrus sinensis). Int. J. Mol. Sci. 22, 8918. doi: 10.3390/ijms22168918
Stewart, R. R. C., Bewley, J. D. (1980). Lipid peroxidation associated with accelerated aging of soybean axes. Plant Physiol. 65, 245–248. doi: 10.1104/pp.65.2.245
Sun, Y., Pri-Tal, O., Michaeli, D., Mosquna, A. (2020). Evolution of abscisic acid signaling module and its perception. Front. Plant Sci. 11. doi: 10.3389/fpls.2020.00934
Thirumalaikumar, V. P., Devkar, V., Mehterov, N., Ali, S., Ozgur, R., Turkan, I., et al. (2018). NAC transcription factor JUNGBRUNNEN1 enhances drought tolerance in tomato. Plant Biotechnol. J. . 16, 354–366. doi: 10.1111/pbi.12776
Thordal-Christensen, H., Zhang, Z., Wei, Y., Collinge, D. B. (1997). Subcellular localization of H2O2 in plants: H2O2 accumulation in papillae and hypersensitive response during the barley-powdery mildew interaction. Plant J. 11, 1187–1194. doi: 10.1046/j.1365-313X.1997.11061187.x
Vicente-Serrano, S. M., Peña-Angulo, D., Beguería, S., Domínguez-Castro, F., Tomás-Burguera, M., Noguera, I., et al. (2022). Global drought trends and future projections. Philos. Trans. R. Soc A Math. Phys. Eng. Sci. 380, 20210285. doi: 10.1098/rsta.2021.0285
Wang, J. E. (2013). Expression analysis and functional identification of CaRGA1and CaPOD genes induced by phytophthora capsici in pepper (Yangling, China: Institute of Horticulture, Northwest A&F University), 2013.
Wang, X.-R., Wang, Y.-H., Jia, M., Zhang, R.-R., Liu, H., Xu, Z.-S., et al. (2022). The phytochrome-interacting factor DcPIF3 of carrot plays a positive role in drought stress by increasing endogenous ABA level in Arabidopsis. Plant Sci. 322, 111367. doi: 10.1016/j.plantsci.2022.111367
Wei, H., Luo, M., Deng, J., Xiao, Y., Yan, H., Liu, H., et al. (2024). SPL16 and SPL23 mediate photoperiodic control of seasonal growth in Populus trees. New Phytol. . 241, 1646–1661. doi: 10.1111/nph.19485
Yamasaki, K., Kigawa, T., Inoue, M., Tateno, M., Yamasaki, T., Yabuki, T., et al. (2004). A novel zinc-binding motif revealed by solution structures of DNA-binding domains of Arabidopsis SBP-family transcription factors. J. Mol. Biol. 337, 49–63. doi: 10.1016/j.jmb.2004.01.015
Yang, Q., Wang, J., Zhang, S., Zhan, Y., Shen, J., Chang, F. (2023). ARF3-mediated regulation of SPL in early anther morphogenesis: maintaining precise spatial distribution and expression level. Int. J. Mol. Sci. 24, 11740. doi: 10.3390/ijms241411740
Yin, Y. X., Guo, W. L., Zhang, Y. L., Ji, J. J., Xiao, H. J., Yan, F., et al. (2014). Cloning and characterisation of a pepper aquaporin, CaAQP, which reduces chilling stress in transgenic tobacco plants. Plant Cell. Tissue Organ Cult. 118, 431–444. doi: 10.1007/s11240-014-0495-3
Zhang, H.-X. (2020). Functional identification and Regulation Mechanism of Pepper SBP-box Gene in Response to Phytophthora Capsici Infection Vol. 2020 (Yangling, China: Institute of Horticulture, Northwest A&F University).
Zhang, H., Ali, M., Feng, X., Jin, J., Huang, L., Khan, A., et al. (2018). A Novel Transcription Factor CaSBP12 Gene Negatively Regulates the Defense Response against Phytophthora capsici in Pepper ( Capsicum annuum L .). Int. J. Mol. Sci. 20, 1–20. doi: 10.3390/ijms20010048
Zhang, H. X., Feng, X. H., Jin, J. H., Khan, A., Guo, W. L., Du, X. H., et al. (2020). CaSBP11 participates in the defense response of pepper to phytophthora capsici through regulating the expression of defense-related genes. Int. J. Mol. Sci. 21, 1–22. doi: 10.3390/ijms21239065
Zhang, Y. L., Jia, Q. L., Li, D. W., Wang, J. E., Yin, Y. X., Gong, Z. H. (2013). Characteristic of the pepper CaRGA2 gene indefense responses against phytophthora capsici leonian. Int. J. Mol. Sci. 14, 8985–9004. doi: 10.3390/ijms14058985
Zhang, H.-X., Jin, J.-H., He, Y.-M., Lu, B.-Y., Li, D.-W., Chai, W.-G., et al. (2016). Genome-Wide Identification and Analysis of the SBP-Box Family Genes under Phytophthora capsici Stress in Pepper (Capsicum annuum L.). Front. Plant Sci. 7. doi: 10.3389/fpls.2016.00504
Zhang, H., Pei, Y., Zhu, F., He, Q., Zhou, Y., Ma, B., et al. (2023). CaSnRK2.4-mediated phosphorylation of CaNAC035 regulates abscisic acid synthesis in pepper (Capsicum annuum L.) responding to cold stress. Plant J. 117, 1–15. doi: 10.1111/tpj.16568
Zhao, Y., Chan, Z., Xing, L., Liu, X., Hou, Y. J., Chinnusamy, V., et al. (2013). The unique mode of action of a divergent member of the ABA-receptor protein family in ABA and stress signaling. Cell Res. 23, 1380–1395. doi: 10.1038/cr.2013.149
Zhao, Y., He, J., Liu, M., Miao, J., Ma, C., Feng, Y., et al. (2024). The SPL transcription factor TaSPL6 negatively regulates drought stress response in wheat. Plant Physiol. Biochem. . 206, 108264. doi: 10.1016/j.plaphy.2023.108264
Keywords: pepper, CaSBP13, drought, Nicotiana benthamiana, ABA signaling pathway
Citation: Zhang H-X, Zhang Y and Zhang B-W (2024) Pepper SBP-box transcription factor, CaSBP13, plays a negatively role in drought response. Front. Plant Sci. 15:1412685. doi: 10.3389/fpls.2024.1412685
Received: 05 April 2024; Accepted: 01 July 2024;
Published: 12 July 2024.
Edited by:
Byeong-ha Lee, Sogang University, Republic of KoreaReviewed by:
Sung Chul Lee, Chung-Ang University, Republic of KoreaRugang Chen, Northwest A&F University, Shaanxi, Xianyang, Yangling District, China
Copyright © 2024 Zhang, Zhang and Zhang. This is an open-access article distributed under the terms of the Creative Commons Attribution License (CC BY). The use, distribution or reproduction in other forums is permitted, provided the original author(s) and the copyright owner(s) are credited and that the original publication in this journal is cited, in accordance with accepted academic practice. No use, distribution or reproduction is permitted which does not comply with these terms.
*Correspondence: Huai-Xia Zhang, zhang1142154371@163.com