- 1Department of Natural Resource Management, South Dakota State University, Brookings, SD, United States
- 2Department of Biological Sciences, University of Maryland, College Park, MD, United States
- 3Department of Botany and Laboratories of Analytical Biology, National Museum of Natural History, Smithsonian Institution, Washington, DC, United States
The mating system of non-native plant populations plays a role in determining the colonizing success following introduction into locations outside of the native distribution. For plant species capable of mixed-mating, both selfing and outcrossing can be advantageous and promote the establishment, persistence, and spread of newly arrived populations. To investigate how mating systems may contribute to the invasion process we estimated mating system parameters in perennial populations of the model plant species, Mimulus guttatus from its native range (West coast USA), non-native populations that are established but have not become invasive (East coast USA, >50 years), and populations in invasive regions (UK >200 years). Studies that include mating system data across the continuum of the invasion process are rare, thus here we utilize molecular markers to estimate outcrossing rates (t), inbreeding coefficients (F), and inbreeding depression in native, naturalized, and invasive populations. Overall, we found support for the persistence of mixed-mating across populations, variability in the relationship between outcrossing rates and inbreeding depression across populations, and evidence for the bridgehead process, where non-native populations may be the sources for the further establishment or reinforcement of additional non-native populations. The methodology we deployed had its own assumptions and sampling design constraints, that contributed to the variation in the parameter estimates. All M. guttatus populations likely rely on selfing through both within clone, and within flower and plant mating in addition to vegetative propagation. The results underscore the importance of introduction history in determining the role of both sexual and asexual reproduction in the successful establishment of a plant species outside its native range.
Introduction
In hermaphroditic plants, self-compatibility is common, and populations of the same species can vary substantially in the degree to which they rely on outcrossing (Goodwillie et al., 2005; Whitehead et al., 2018). In native early-successional species (i.e. “weedy” species) or non-native plant species, outcrossing can provide a selective advantage by increasing the standing genetic variation required by populations to adapt to novel environmental conditions through admixture and recombination (Sax et al., 2007; Catford et al., 2009) which can also be beneficial in many management plans to address a lack of genetic variation in species of concern (Ralls et al., 2018; Rodger et al., 2024). However, we know much less about the mating system or how non-native naturalized or invasive populations persist outside their range and the factors that impact their expansion. From the literature we expect non-native plant species to begin as small populations during colonization owing to limited habitat, lack of pollinators and dispersal limitations inherent to sedentary life forms (Baker, 1967; Kolar and Lodge, 2001). In cases like these, a uniparental mode of reproduction, such as clonal propagation or self-fertilization (both within flower and geitonogamy within ramets), may be more selectively advantageous for population persistence (Vallejo-Marin and O'Brien, 2007; Pannell et al., 2015).
Given the adaptive advantages of both selfing and outcrossing are dependent on demographic and stochastic factors, many empirical and theoretical studies have led to the development of this field over time. One scenario is where complete outcrossing (t = 1) or complete selfing (t = 0) are alternative stable states (Lande and Schemske, 1985; Schemske and Lande, 1985) and propose that when intermediate outcrossing rates (0.2 < t < 0.8) are found, this “mixed mating” strategy, is simply a transitional state toward complete selfing or outcrossing. However, another scenario is also supported through theoretical (Latta and Ritland, 1994) and empirical studies and suggest that mixed-mating is an alternative stable state (Goodwillie et al., 2005; Jarne and Auld, 2006; Winn et al., 2011; Dart et al., 2012; Zhang et al., 2024). Both scenarios are based on the 3/2 transmission advantage following selfing compared to outcrossing (Fisher, 1941) and suggest that relaxation of certain selective forces can result in a stable mixed-mating strategy or complete selfing depending on the magnitude of inbreeding depression. It is during the early stages of colonization and establishment in part owing to lag effects in population expansion (Morgan et al., 2024) that the trajectory towards one of these three mating system strategies initially emerges although it could change over time following establishment.
The strongest selective force facilitating the transition from selfing to outcrossing is inbreeding depression, the decrease in fitness of progeny produced by matings between related individuals, i.e., selfing, relative to progeny produced from matings between unrelated individuals, i.e., outcrossing (e.g., Lande and Schemske, 1985; Charlesworth and Charlesworth, 1987; Dudash, 1990; Zhang et al., 2024; Rodger et al., 2024). Fitness is defined as both the quantity and quality of the progeny produced and can include various life history stages (ex., seed set, seed viability, germination, vegetative and floral size) depending on the study conducted. While inbreeding depression is likely the primary selective force, studies have suggested that the effects of inbreeding depression can be mitigated over time as the deleterious recessive alleles responsible for the decrease in fitness may be purged from the population (e.g., Hedrick, 1994; Dudash and Carr, 1998; Byers and Waller, 1999; Dussex et al., 2023).
Colonization success and its relationship to mating system has long been suggested and coined as “Baker’s Law” (Baker, 1955), where self-compatible species should be more successful colonizers following long-distance dispersal compared to obligate outcrossing species, in part because the former would need only one individual to establish a naturalized population (Baker, 1967; Stebbins, 1957; Kolar and Lodge, 2001). However, empirical evidence has both supported and contradicted this theory (Pannell and Barrett, 1998; Randle et al., 2009; Cheptou, 2012; Pannell et al., 2015) and have further highlighted a role for unisexual reproduction in colonization success (Pannell et al., 2015; Pantoja et al., 2017), thus there are likely multiple paths for establishment of plant populations outside of their native range. Furthermore, naturalization of a non-native plant species is often considered an intermediate critical stage prior to a population becoming invasive, representing a lag phase of slow population growth as it deals with deficiencies inherent to a novel population’s demographics or to maladaptation (Aikio et al., 2010; Murren et al., 2009; Richardson and Pyšek, 2006). Thus, various factors ultimately determine whether a non-native population goes extinct, remains cryptic and benign, or alternatively adapts and spreads into new locations (Catford et al., 2009; Richardson and Rejmánek, 2011).
The mode of reproduction, asexual versus sexual with the latter dependent on the mating system is key to how it may affect invasion success by influencing population size, dispersal, and genetic structure of introduced plant populations (Kinlan and Hastings, 2005; Bazin et al., 2014; Zimmer et al., 2022). Invasive plant species are characterized by an ability to colonize and spread throughout recipient habitats following dispersal (Lockwood et al., 2013). In cases where only a single or few individuals have become established, Allee effects can threaten persistence in a new location (Allee, 1951; Allendorf and Lundquist, 2003; Prentis et al., 2008). However, these factors can be offset by high rates of selfing though both within flower and between flower visits within a plant and matings between related plants, clonal propagation, or a combination, with the potential for inbreeding depression and subsequent purging of genetic load (Baker, 1955; Charlesworth and Charlesworth, 1987; Rambuda and Johnson, 2004). Alternatively, multiple introductions of plants from the same or different source populations can result in an establishment pathway that ameliorates the demographic and genetic constraints associated with an initially small colonizing cohort (Facon et al., 2006; Lombaert et al., 2010). Multiple introductions have been found to lead to greater population size and genetic diversity that can mitigate novel environmental constraints (Genton et al., 2005; Dlugosch and Parker, 2008; Wilson et al., 2009; Estoup and Guillemaud, 2010). A recent study has also demonstrated the role of the bridgehead process (where non-native populations act as a seed source for further non-native species expansion) and admixture between populations in helping to explain the global invasion of Mimulus guttatus (Vallejo-Marin et al., 2021).
The aim of this study was to examine the mating system in situ in perennial populations of the self-compatible Mimulus guttatus that occur along the invasion spectrum: native populations that have been evolving in their environments for millennia; naturalized populations that have established outside their native range but have not spread; and invasive populations that have dispersed beyond the point of initial introduction (Richardson et al., 2000). Studies of plant invasions that include comparisons among native, naturalized, and invasive populations are uncommon because naturalized populations are often cryptic and benign in the environment, and thus difficult to identify in the field (but see Muth and Pigliucci, 2006). We tested the following hypotheses: (1) Outcrossing rates and mean inbreeding coefficients differ among populations in three regions: native, naturalized, and invasive. If non-native populations of M. guttatus adhere to Baker’s Law, we predict that outcrossing rates will be lower, and inbreeding coefficients higher, in naturalized and invasive populations compared to native populations; (2) Inbreeding depression differs among populations in the three regions. We predict that inbreeding depression will be lower in populations that have lower outcrossing rates because they may have been established a sufficient length of time to have purged their genetic load, leading to an equilibrium state.
Methods
Study species
Mimulus guttatus (Phrymaceae; 2n = 2x = 28), or common monkeyflower, is a self-compatible mixed-mating species native to the West coast of North America, found from Mexico to Alaska (Dudash et al., 1997; Carr et al., 1997; Kelly and Arathi, 2003; Wu et al., 2008; Lowry et al., 2008). In its native range, M. guttatus populations can be found exhibiting either an annual (ephemeral water availability) or perennial life history (persistent water availability) (van Kleunen and Fischer, 2008; Lowry et al., 2008; Popovich and Lowry, 2020; Zell et al., 2023). Mimulus guttatus is pollinated by bees and other insects who readily visit multiple flowers on an individual. i.e., geitonogamy, suggesting limited gene flow via pollen dispersal (van Kleunen and Johnson, 2007). The seeds produced per fruit are often in the 100s and are small and can potentially be dispersed long distances by water and possibly wind (Grant, 1924; van Kleunen and Fischer, 2008).
Native populations of M. guttatus exhibit wide variation in outcrossing rates (Ritland and Ganders, 1987; Dudash and Carr, 1998). Transitions between outcrossing and selfing within the Mimulus genus (Fenster and Ritland, 1994) are likely controlled by polygenic inheritance (Fenster et al., 1995). The mode of self-fertilization in M. guttatus is largely competing selfing, which occurs concurrently with outcrossing through both within flower and geitonogamous self-pollination, as opposed to prior or delayed selfing (Lloyd and Schoen, 1992; Leclerc-Potvin and Ritland, 1994).
In addition to its sexual mating strategies of reproduction, M. guttatus is capable of forming clones via asexual reproduction, i.e., fragmentation and stolon production when water is persistent across years (Grant, 1924; Vickery, 1959; Truscott et al., 2006; Zimmer et al., 2022). Mimulus guttatus has become a model system in studies of evolutionary, developmental, and population genetics because of its broad phenotypic and genetic diversity (e.g., Dudash et al., 2005; Wu et al., 2008; Yuan, 2019).
Study populations
For this study, we only sampled from perennial native populations because all known non-native populations are perennial. The eight native populations sampled, and their coordinates (Table 1) include three from Alaska (AKS1, AKS2, AKA), one from Washington (WA), three from Oregon (OR02, OR04, and OR06), and one from California (BB1). In prior work, we identified a few isolated populations in Eastern North America that have become naturalized and shown no detectable spread beyond their current locations (Murren et al., 2009 and Dudash pers. obs.). Little is known of the evolutionary history of these naturalized populations, but the two included in this study, Fly Creek, NY (FC) and Springfield, New Brunswick, Canada (NBS), are thought to have established over 50 years ago (Murren et al., 2009). In the United Kingdom (UK), M. guttatus is considered an invasive that was intentionally introduced as a horticultural species over 200 years ago (Truscott et al., 2008; van Kleunen and Fischer, 2008; Vallejo-Marin et al., 2021).
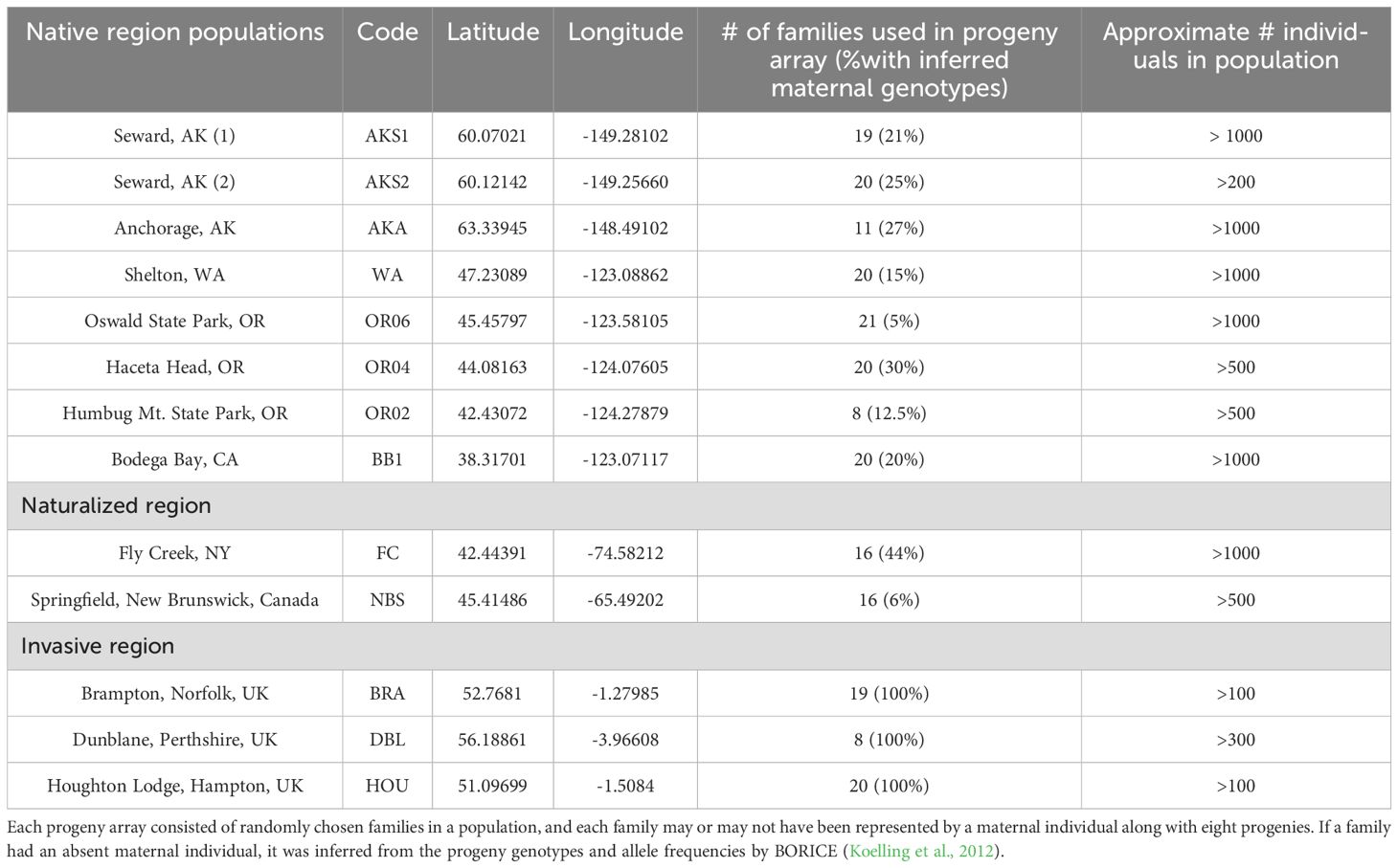
Table 1. Location and approximate population size of 8 Mimulus guttatus populations from the native region, two from the naturalized region, and three from the invasive region used for progeny array analyses.
Sampling and genotyping for progeny array analysis
We conducted a primarily field-based progeny array analysis to estimate outcrossing rates (t), inbreeding coefficients (F), and relative fitness (inbreeding depression) of progeny for M. guttatus populations in the native, naturalized, and invasive ranges. This was completed in one season along the West coast of North America and the East coast as noted above (Table 1). The field route to survey the eight West coast native perennial populations was based on records obtained from colleagues (B. Blackman and D. Lowry, pers. comm.) and local contacts. For each of the 10 native and 2 naturalized populations visited, we randomly sampled seed and leaf tissue from 30-50 maternal families that were > 1 m apart to increase the chances of sampling multiple genotypes. Leaf tissue was immediately stored in silica gel until DNA extraction. For the three M. guttatus populations in the invasive region in the UK, we did not collect leaf tissue, but instead obtained field-collected seed from a colleague (M. Vallejo-Marin).
Seed representing progeny for all maternal families was grown in the University of Maryland (UMD) greenhouse and when seedlings were ~ 6 cm tall, leaf tissue was collected from eight progeny/maternal family and stored in silica gel. Up to thirty maternal families per population were randomly sown but fewer families were used in the progeny array analysis due to variable germination success and poor-quality DNA in some families (Table 1). This approach only captures the very early life history stages of the progeny while field collected tissue from maternal plants reflect selective pressures up to the adult stage. Furthermore, sampling was limited in the non-native regions (5 versus 10 native).
We extracted DNA from field-collected maternal leaf tissue (all populations except the three from the invasive region) and from leaf tissue collected from greenhouse-grown progeny originating from field-collected seed (all populations including the invasive populations). We used a modified CTAB protocol and an automated Autogen® robotic DNA extractor (Doyle and Doyle, 1990). Genotyping methods and amplification protocol is provided in Appendix 1.
Bayesian estimation of t and F using BORICE
We chose to use the software BORICE (Bayesian Outcrossing Rate and Inbreeding Coefficient Estimation; Koelling et al., 2012; http://www.python.org/) because of its ability to statistically infer maternal genotype when unknown and the Bayesian based program can calculate both outcrossing rates (t) and inbreeding coefficients (F) when sampling numerous maternal families with as few as 8 progeny per maternal plant. Maternal genotypes for the three UK populations had to be inferred along with some families from the native and naturalized populations when missing maternal genotype data (Table 2). This approach has been shown to be preferable to Ritland (1990) MLTR approach because of its ability to estimate mating system parameters with fewer progeny allowing larger maternal family sampling (Koelling et al., 2012). However, this approach also assumes that populations are in equilibrium, which can impact its reliability owing to its sampling design preferring more families and fewer progeny per maternal family to infer population level information. Details on BORICE joint estimation procedure can be found in Appendix 2.
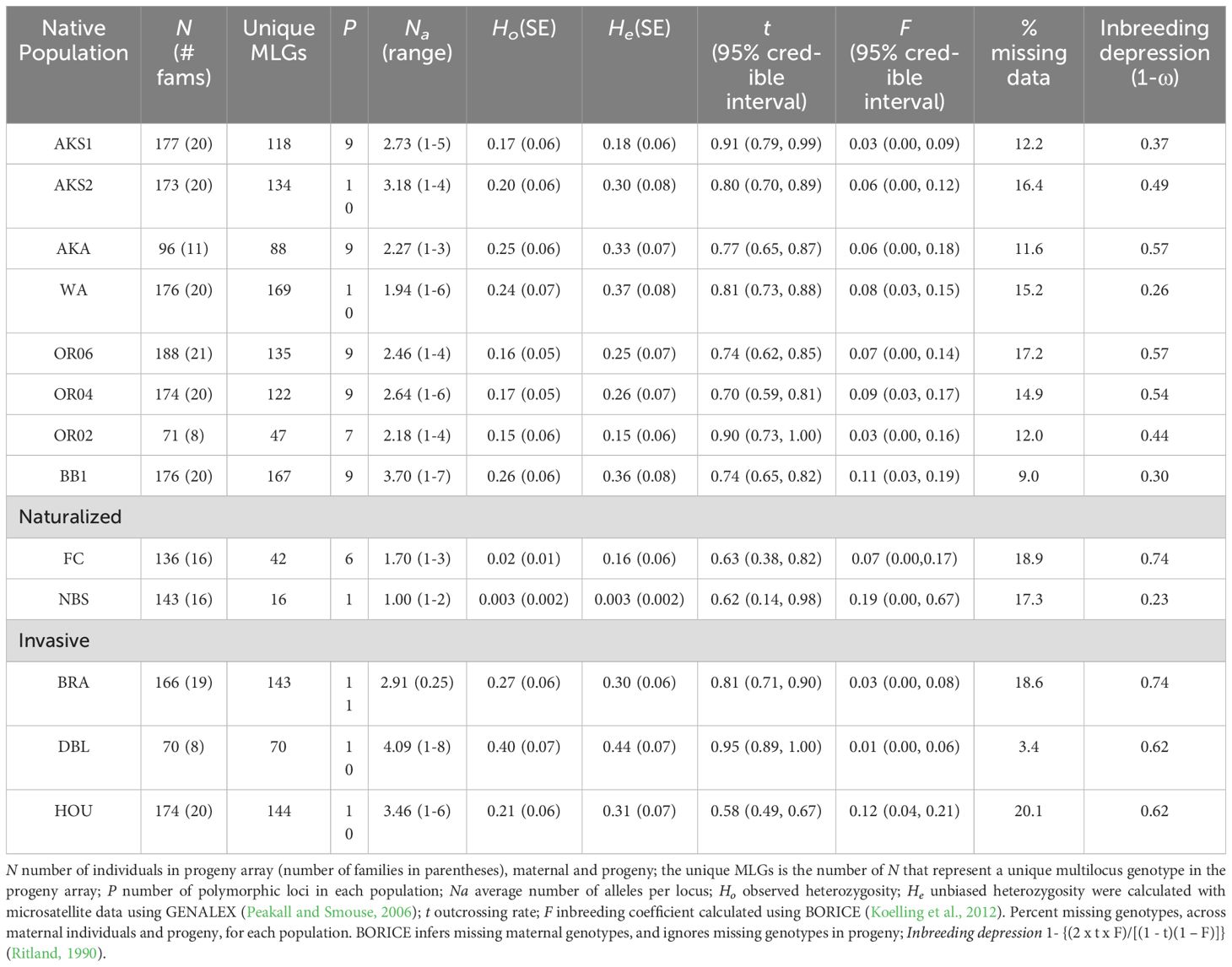
Table 2. Outcrossing rates (t), inbreeding coefficients (F), and inbreeding depression (1-ω) in native and non-native populations of M. guttatus.
We used a chain of 100,000 steps with a burn-in of 10,000 steps. Once the posterior t and F were estimated, we calculated Ritland (1990) estimator for relative fitness of selfed progeny (ω) to characterize inbreeding depression (1- ω) in each population. Assuming F is constant across generations, relative fitness is found using:
and inbreeding depression is 1-ω. Because inbreeding depression is dependent only on t and F, understanding different outcomes regarding the selection against selfed individuals is straightforward. For example, if both t and F in a population are low, inbreeding depression could be relatively high. This occurs because while many selfed progeny are produced (low t), few survive to adulthood (low F among maternal individuals). Alternatively, if t is low and F is high, the measure of inbreeding depression could be low because the high number of offspring produced by selfing survive and are represented in the next maternal individuals, as evidenced by the high F. Additionally, when outcrossing rates are high, the estimate of inbreeding depression will generally be low regardless of the magnitude of F, because when selfed progeny are produced they result in an opportunity to purge genetic load through selection eliminating deleterious recessive alleles. Finally, if there is high inbreeding depression in a selfing population this implies that purging is still ongoing and following many generations of selfing purging should further eliminate deleterious alleles and then we would expect lower inbreeding depression and higher inbreeding coefficients in the population.
Statistical analysis
We used Welch’s t-tests (R Core Team, 2016), which do not require the assumption of equal variances between unpaired samples, to examine pairwise differences between M. guttatus regions (native, naturalized, and invasive) regarding t, F, and inbreeding depression (1- ω). All mating system and genetic diversity parameters comparisons were based on family averages for each study population’s estimates from one season.
Results
For the 13 populations in the progeny arrays, we utilized 11 codominant markers (Table 3). These included five microsatellite loci previously used to genotype North American and British M. guttatus populations (Kelly and Willis, 1998; Vallejo-Marin and Lye, 2013), and six markers revealing length polymorphisms in introns of single-copy nuclear genes in M. guttatus (Fishman and Willis, 2005; Lowry et al., 2008; Vallejo-Marin and Lye, 2013; Zimmer et al., 2022). These intron length polymorphisms, or MgSTS (M. guttatus sequence-tagged sites) were found to be suitable for genotyping based on a selection strategy of Vallejo-Marin et al. (2011). These markers are variable in samples of M. guttatus, its close relative, the tetraploid M. luteus, and the triploid hybrid produced by them, M. x robertsii Silverside, and are suitable for multiplexing (Vallejo-Marin and Lye, 2013).
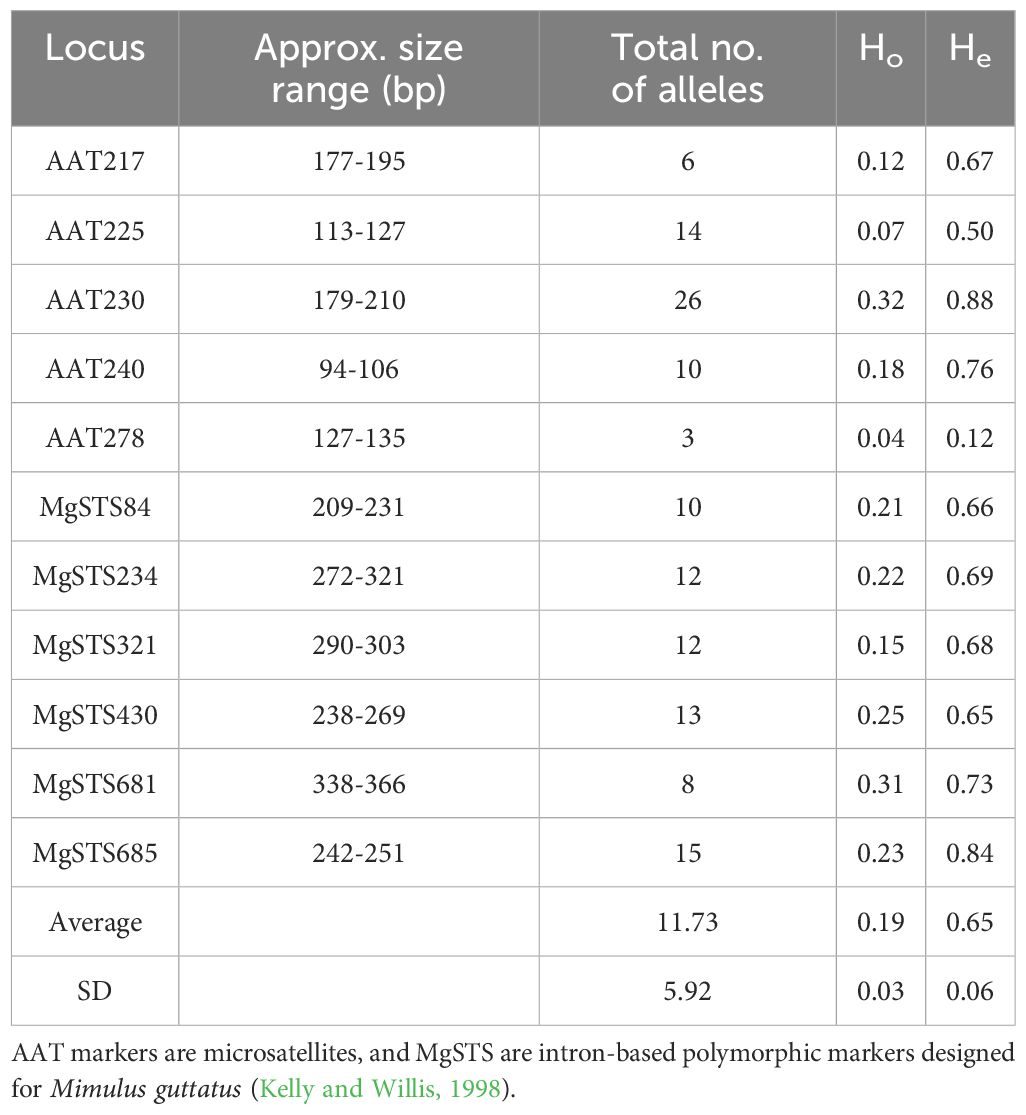
Table 3. Diversity of eleven molecular markers used in the study, across 13 native and non-native populations of M. guttatus.
All markers utilized were polymorphic, with between three and 26 alleles (Table 3). There was evidence for the presence of null alleles in the form of ‘impossible genotypes’ reported by BORICE. A total of 10 impossible genotypes for loci AAT230, AAT278, and MgSTS84 were reported in five populations (AKA, OR02, BB1, FC, and HOU) (Zimmer et al., 2022). Therefore, while null alleles were allowed in the final analysis of all populations, a random sample of three populations (AKA, OR04, and HOU) was run without allowing for null alleles and the results for t and F were not statistically different from the models that allowed for nulls (t-test, P > 0.05). The naturalized East coast FC population had the greatest number of private alleles (Zimmer et al., 2022).and the lowest number of unique MLGs (Table 2). Additionally, the percent of missing data across this study ranged from 3-20% (Table 2).
Family level averages of outcrossing rates (t), inbreeding coefficients (F), and inbreeding depression (1-ω) estimates were calculated to provide population level estimates for the native and non-native populations of M. guttatus in Table 2. Please note that there is much overlap among population mating system estimates when examining the 95% credible intervals, thus we will be discussing trends along with significant differences below.
Outcrossing rates
Population level outcrossing rate estimates (t) in the native region on average were similar to those in the invasive region of M. guttatus, (native mean = 0.80 (SE ± 0.03), n = 8 populations; invasive mean = 0.78 (SE ± 0.11), n = 3 populations; t score = 0.15, P = 0.90). However, one population from the invasive region (HOU) had the lowest outcrossing rate recorded among all populations sampled, t = 0.58 while another had the highest t recorded (DBL, t = 0.95). Contrastingly, the mean t for the two naturalized populations was significantly lower than that in the native region (naturalized mean = 0.63 (SE ± 0.01), n = 2 populations; native mean = 0.80 (SE ± 0.03), n = 8 populations, t score = 3.63, P < 0.01). The mean estimate of t in the naturalized region (t = 0.63, SE ± 0.01) was also lower than the invasive region (t = 0.78, SE ± 0.11), but not significant (t score = 1.11, P = 0.35; Table 2).
Inbreeding coefficients
The mean estimate of inbreeding coefficients (F) was also similar among the native and invasive regions (native mean = 0.07 (SE ± 0.01); invasive mean = 0.05 (SE ± 0.03); t score = 0.52, P = 0.62). The mean F in the two naturalized populations trended higher than F estimates in the native and invasive regions (naturalized mean = 0.13 (SE ± 0.06); vs. native, t score = 2.03, P = 0.08; vs. invasive, t score = 1.23, P = 0.31) but were not significant (Table 2).
Inbreeding depression
Inbreeding depression (1 – ω, where ω = the relative fitness of progeny produced by selfing) was greatest in the invasive region, and significantly greater than that found in the native region (native mean = 0.44 (SE ± 0.04); invasive mean = 0.66 (SE ± 0.04); t score = 2.87, P = 0.02). The mean level of inbreeding depression in the naturalized populations was intermediate between native and invasive levels (naturalized mean = 0.49 (SE ± 0.26); vs. native mean = 0.44 (SE ± 0.04), t score = 0.31, P = 0.76; vs. invasive, t score = 0.88, P = 0.44, but there was a large amount of variation between estimates of the two naturalized populations, FC (0.74) and NBS (0.23), which was not significantly different Table 2).
Discussion
The evolution of mating systems in colonizing plants has been a primary focus in ecology for decades and is likely to be case dependent, with demographic and stochastic variables playing consequential roles in determining the extent of selfing versus outcrossing in incipient populations (Baker, 1967; Cheptou, 2012; Pannell et al., 2015). This is the first study that we aware of to utilize BORICE to compare in situ outcrossing rates (t) and mean inbreeding coefficients (F) of maternal individuals between native populations of M. guttatus and two separate categories of non-native populations, naturalized and invasive. Our data revealed that overall native populations exhibited a relatively high outcrossing rates and lower inbreeding coefficient across the 8 populations as would be expected. However, inbreeding depression was quite variable with four native populations above or equal to 0.5 and the other four native populations below 0.5 suggesting different suites of factors likely influenced their mating system evolution. In contrast the three UK non-native populations differed in their reliance on selfing to persist in their respective locations demonstrated by their variable outcrossing rates. Only one naturalized populations (NBS) in Eastern North America, demonstrated an outcrossing rate greater than 0.5 and inbreeding depression below 0.5 as predicted from transmission bias theory (e.g., Fisher, 1941) compared to four populations in the native region that also demonstrated this pattern. The remaining two populations in the invasive UK region and the one naturalized population on the east coast had relatively high outcrossing rates, low adult inbreeding depression coefficients, and high inbreeding depression suggesting ongoing purging in these populations along with some populations in the native region. Below we discuss probable scenarios and limitations of our study including the limited sampling design in the non-native compared to the native region that may help explain the variable estimates of t, F and inbreeding depression found in these populations. We also explore how different mating system approaches and parameters may be limited in their ability to predict the maintenance of current and future mating systems in these populations
Outcrossing rate variation in both native and non-native M. guttatus populations suggested the persistence of mixed-mating system in these populations (Table 2). The prediction that we would see a positive relationship between outcrossing rate and inbreeding depression was weakly supported in three of the eight native populations, which are most likely to be in genetic equilibrium owing to their long-term persistence in the native range. We found little support for our prediction that non-native populations of M. guttatus would adhere to Baker’s Law, such that outcrossing rates would be lower and inbreeding coefficients higher in naturalized and invasive populations compared to native populations with only the naturalized NBS populations exhibiting relatively low t and high F (Table 2). In addition, the averages for t and F in the two UK populations (BRA, DBL) in the invasive region were nearly identical to that in the native region and differed from the UK HOU, which appeared to support Baker’s Law. The population estimate outcrossing rates among the eight native M. guttatus populations sampled of, 0.70-0.91 (even with their large 95% credible interval; Table 2), was approximately within the range of outcrossing rates found in past studies using MLTR (0.41-0.76, Ritland and Ganders, 1987; 0.68-0.80, Dudash and Ritland, 1991). This general agreement in outcrossing rates supports the population estimates found by BORICE (Koelling et al., 2012) here compared to MTLR prior estimates of Dudash and Ritland (1991) even with their contrasting approaches, sampling, and inherent assumptions.
Inbreeding coefficients in the native region were generally low, as would be expected with the outcrossing rates detected (Table 2). The estimates of t and F for two of the three populations from the UK, where M. guttatus is considered an invasive, fell within the ranges of t and F for the native region (Table 2). Our results support the idea that there can be variation in outcrossing rates among populations of the same species independent of how they are categorized (native, naturalized, or invasive) and likely in part because each population has its own unique past. An extensive survey of outcrossing rates of 105 plant flowering species represented by a minimum of 3 populations each, demonstrated great variation in outcrossing rates and warned about extrapolating the results of one population to the whole species (Whitehead et al., 2018). Dart et al. (2012) also documented three modal stable mixed mating systems among 40 populations across the range of Camissoniopsis cheiranthifolia that varied from predominately outcrossing with self-incompatible individuals with large flowers to moderate selfing populations with individuals with large self-compatible flowers to higher selfing populations associated of individuals with smaller autogamous flowers. Overall, our work and these studies suggest that the variation in mating systems observed is likely context dependent and a result of both environmental and genetic factors along with where a particular population lies along a continuum within its native range, at its edge, if and how long it has been naturalized, and its likelihood of becoming invasive.
It has been widely documented that invasive M. guttatus populations in the UK are the unintended consequence of the horticulture trade between the US and Europe over 200 years ago (Preston et al., 2002; Truscott et al., 2008; van Kleunen and Fischer, 2008; Puzey and Vallejo-Marín, 2014; Vallejo-Marin et al., 2021). By repeatedly introducing propagules to non-native regions (intentionally as a horticultural species, in the case of M. guttatus), the likelihood of forming natural populations with greater densities and genotypic diversity increases (Kalisz et al., 2004; Eppley and Pannell, 2007; Friedman and Barrett, 2008). A positive correlation between outcrossing rates and plant density has frequently been shown in herbaceous plant species (Karron et al., 1995; Herlihy and Eckert, 2004; Brunet and Sweet, 2006). For this study, we estimated population size by the number of adult plants present at each of the 13 locations when we collected maternal family seed and found no clear relationship between estimated population size and outcrossing rate, inbreeding coefficients and inbreeding depression perhaps in part because of the overall size similarities of the populations sampled (i.e., readily detected) for this study (Table 1).
Many ornamentals were introduced into Europe (Reichard and White, 2001; Mack, 2003; Thuiller et al., 2005); because of traits associated with reproductive fitness, such as floral size and biomass. These same traits are also thought to increase the likelihood that escaped plants will successfully establish outside of their native range (Mack, 2000; Kowarik, 2003). It is an open question as to whether M. guttatus populations in introduced regions display differences in various fitness traits that may facilitate establishment and invasion. For example, one study compared floral traits, along with sexual and vegetative reproduction, between invasive versus native populations of M. guttatus and found no significant differences (van Kleunen and Fischer, 2008). However, a companion greenhouse study using these same populations, found evidence for a significant increase in floral size (floral width x floral length) in invasive populations compared to native populations (Berg, 2018) and a significant reduction in floral size of the naturalized populations. Interestingly, there was no difference in stigma-anther separation among the populations across native, naturalized, and invasive populations in this study even though floral size varied (Berg, 2018) suggesting uniform selection against within floral self-pollination. Because increasing floral size traits have been associated with higher outcrossing rates in M. guttatus (Ritland and Ritland, 1989), more research is needed to evaluate the role of enhanced sex allocation to increasing floral size in the mating systems of non-native populations.
In one UK population (HOU), and in one naturalized population on the East coast (NBS), outcrossing rates were lower and the mean inbreeding coefficient among maternal individuals tended to be greater compared to native populations. In the naturalized populations, the lower outcrossing rates can be explained by the lack of genotypic diversity in each population for total number of alleles per loci and average number of alleles per loci such that outcrossing events could not be readily detected even when they occurred (Zimmer et al., 2022). The observed heterozygosity from limited microsatellite data in the naturalized FC and NBS populations was much lower compared to the 11 other populations (Table 2) suggesting that asexual clonal propagation, geitonogamy, and fixation of alleles were important characteristics of these naturalized East coast M. guttatus populations (Zimmer et al., 2022). When examining the expected heterozygosity (He) versus observed heterozygosity (Ho) of the naturalized populations the FC population He is much higher than the Ho while both Ho and He are low in NBS population. This suggests there are more alleles in FC than NBS and the reason for the low Ho is different (confirmed in Zimmer et al., 2022). In NBS an outcrossing event cannot be as easily detected owing to a lack of genetic variation while this is not necessarily the case for the FC population. Furthermore, the number of private alleles detected for FC (n=5) compared to NBS (n=0) supports this line of reasoning (Zimmer et al., 2022). Another parameter that provided further insight was the proportion of unique multilocus genotypes (MLGs) determined with poppr (Zimmer et al., 2022) which produced outcrossing estimates lower in the naturalized east coast populations compared to the native and invasive UK populations. On the East coast FC and NBS, the proportion of unique MLGs of the total number of individuals sampled, maternal and progeny, was only 31% and 11%, respectively. These proportions are low compared to an average 82% (SD = 0.13) across the remaining 11 populations in the study. Pantoja et al. (2017) provides further evidence for great differences in genetic variation and clonal reproduction among 14 populations of M. guttatus in the non-native invasive UK range when compared to 10 native populations. Their results demonstrate a great breadth in how non-native populations (invasive and naturalized) establish through sexual versus asexual reproduction as well as hints to historical routes of introduction into the UK. Together these studies highlight the potential role of uniparental (asexual through clonality) reproduction in non-native population persistence and their ability to spread outside their native range.
The lack of genotypic and genetic diversity in the progeny arrays of the East coast naturalized populations (observed heterozygosity in FC was 0.02, and effectively zero in NBS; Table 2) results in an inability to distinguish whether any particular offspring was the result of selfing, because the progeny genotypes are identical to the maternal genotype, or rather of outcrossing within a genet (geitonogamy), because many of the maternal genotypes are identical to one another. In these cases, the Bayesian posterior probability of t will approach 0.5 because half of the offspring will be deemed the product of a selfing event, and the other half of the product of outcrossing between parents that share the same genotype. Evidence for lack of confidence in determining whether progenies were produced via selfing or outcrossing in the two naturalized populations can be seen in the high variability surrounding the mean posterior estimate of t in FC and NBS (Table 2). This lack of genotypic and genetic diversity in the East coast populations could be the result of limited opportunities for colonization, a recent bottleneck (Tsutsui et al., 2000; Frankham, 2005; Prentis et al., 2008), a dominating role of genetic drift over new mutations (Crooks et al., 1999; Eckert et al., 1996), or their dependence on both sexual and asexual reproduction (Pantoja et al., 2017). Why these two naturalized populations with similar outcrossing rates, low observed heterozygosity, but contrasted markedly in inbreeding depression have not expanded is unclear and alludes to how one year’s data can be inadequate to understand the history of any population over time. Both populations were discovered in the early 2000s (Dudash pers obs.) and their ongoing persistence could be in part due to their dependence on water availability owing to their perennial growth form. Another explanation is that we did not search in a wide enough area to detect the establishment of other populations in their respective regions of the east coast.
In the invasive UK populations, the resulting inability to distinguish selfing events from outcrossing within clones and the lower outcrossing rate in the HOU population can be attributed with more confidence to actual selfing events. This is because at least in part to nearly all maternal individuals (93%) exhibiting a unique MLG, making exclusion of paternity via an outcrossing event more straightforward compared to the naturalized east coast populations. Additionally, the UK HOU population appears to be on a different evolutionary trajectory compared to the UK DBL population because it exhibited a lower outcrossing rate and higher inbreeding coefficient than the DBL population with the highest outcrossing rate and lowest inbreeding coefficient of the invasive populations even though they were found to be most similar using Nei’s genetic distance (Zimmer et al., 2022; see Figure 3) suggesting they may be at different places along the continuum of invasion although no relative establishment dates are known.
The contrast in outcrossing rates in the UK invasive region between the lower t found in the HOU population and the other two populations with relatively higher t values (BRA and DBL) is interesting and could be associated with any number of factors, including smaller effective population size in the HOU population or differences in environmental factors (Schemske and Lande, 1985; Ellstrand and Elam, 1993; van Treuren et al., 1993). Another possibility is that HOU is the result of a bridgehead effect, a phenomenon in which the source of a non-native population or invasive population is not from the native region, but rather from a distant non-native population (Lombaert et al., 2010). Recently Vallejo-Marin et al. (2021) have demonstrated the high likelihood of non-native populations in the UK contributing to the establishment of M. guttatus populations globally. Taking this idea one step further, we can imagine a scenario where one of the naturalized east coast populations may have contributed propagules to the establishment of the invasive UK HOU population. A genetic comparison based on Nei’s genetic distance (Nei, 1978) from a companion study of the same populations studied here (Zimmer et al., 2022) showed that the UK HOU and DBL populations are closely related but more distantly related to the UK BRA population and could be evidence of a UK population providing the source propagules for another UK population. Additionally, the naturalized NBS population could also be the result of introductions from the invasive UK populations (Zimmer et al., 2022). Collectively these M. guttatus studies provide evidence for potential bridgehead effects as a pathway to invasion for non-native plant populations and could serve as an interesting model to investigate this phenomenon in other invasive plant species in the future.
Now turning to inbreeding depression, six (five native and one non-native naturalized) of the thirteen M. guttatus populations demonstrated levels of inbreeding depression below 0.5, the theoretical threshold where an allele causing selfing should potentially be able to spread through an outcrossing population and there has been a sufficient length of time to purge the major deleterious alleles from the population. (Fisher, 1941; Lande and Schemske, 1985; Ritland, 1990) In agreement with our prediction, inbreeding depression levels did appear to depend on a population’s geographic region with inbreeding depression levels greater in four out of the five non-native populations compared to the native populations suggesting the maintenance of an outcrossing mating system. In four native populations we found t ≥ 0.8, F was relatively lower, and all inbreeding depression estimates were below 0.5, supporting the potential evolution of selfing by the 3/2 transmission bias over outcrossing (e.g., Fisher, 1941). Subsequently, there are various scenarios that could explain this one year’s parameter estimates and their potential implications to whether selfing is expected to evolve or is still actively being purged from a population promoting outcrossing.
Recall that we calculated an in-situ level of inbreeding depression for each population from field collected seed that germinated in a greenhouse and collected as seedlings such that both very early and later life history stages were not captured likely leading to underestimates (e.g., Husband and Schemske, 1996; Byers and Waller, 1999). Additionally, the progeny collected at the seedling stage in the greenhouse likely minimizes the impact of inbreeding depression compared to the tissue collected from surviving maternal parents in the field. Furthermore, M. guttatus is capable of producing 100s of seeds per flower and numerous flowers per plant (e.g., Dudash and Ritland, 1991) thus 8 progeny per maternal family is not ideal to capture a robust estimate of individual adult plant fitness in the field. Finally, we examined only one generation to calculate inbreeding depression that was measured by using t as an estimate of the proportion of selfed progeny produced and mean maternal F as an estimate of the number of those selfed individuals that survive to adulthood with the assumption that all populations were in equilibrium, which was not tested and could also be contributing to the large variation in our estimates (Ritland, 1990).
It has been repeatedly shown that inbreeding depression can be intense in early life history stages (e.g., Husband and Schemske, 1996), and later life history stages (e.g., Dudash et al., 1997), thus deleterious alleles can be purged throughout a plants life history and inbreeding depression is likely dependent on variation in the maternal family mating history (Dudash and Carr, 1998), particularly when negative density-dependent interactions occur in populations (Mitchell-Olds and Waller, 1985). Results from studies focusing on M. guttatus have concluded that inbreeding depression impacts pollen viability (Willis, 1993), and pollen and ovule production (Carr and Dudash, 1995; Carr et al., 1997; Dudash and Carr, 1998) and is expressed throughout the later life history stages as well (e.g., total flower production, adult above-ground biomass; Dudash et al., 1997). There is also evidence of differential purging of genetic load in M. guttatus, among maternal families, when quantifying later life history traits (Dudash et al., 1997; Dudash and Carr, 1998). A companion greenhouse study investigated the magnitude of inbreeding depression across early and later life history stages among these same populations and the results supports prior work in M. guttatus with once again finding that self-progeny accumulated significantly less above-ground biomass than outcrossed progeny (Berg, 2018).
Another explanation of why we observed inconsistency among outcrossing rates, inbreeding depression, and the inbreeding coefficient may be because some populations had not met equilibrium assumptions (Ritland, 1990). This is more likely in our study because we sampled each population only once, thus we did not have multiple generations of inbreeding coefficients that would have added rigor when calculating these mating system parameters. Ritland (1990) demonstrated that when equilibrium assumptions were not met large variances in inbreeding depression were detected. Furthermore, the inbreeding depression estimates often did not correlate with selfing rate and there was often a positive bias in the estimate leading to false negative correlations between inbreeding depression and selfing rates. Our study supports Ritland (1990) findings because we did not find a consistent relationship between inbreeding depression and outcrossing rate estimates using the one-year snapshot approach, warranting further investigation.
The only East coast naturalized non-native population that fell below the inbreeding depression threshold of 0.5 was the clonal NBS population, which consisted of only a few unique multilocus genotypes (MLGs; Table 2). All loci were also monomorphic for a single allele except for locus AAT225, which had a second, rare allele (frequency of allele “97” = 0.99, frequency of allele “103” = 0.01; Zimmer et al., 2022 see Table 3). It is difficult to know whether the genetic diversity described in NBS is the result of an origin in which a single or few genotypes were introduced and through subsequent selfing and asexual vegetative propagation resulted in a genotypically homogenized monoculture at this location. It is also possible that multiple introductions occurred at this location, and through selection, drift, or a combination of the two, and concurrent high rates of selfing resulted in the genetic pattern observed. There has been much discussion about the ability of invasive plant populations to purge their genetic load and escape the detrimental effects of inbreeding depression resulting in populations where selfing is evident and outcrossing less pronounced (Byers and Waller, 1999; Dudash and Fenster, 2000; Frankham, 2005) and whether in particular instances some genetic load may be beneficial for establishment under novel environmental conditions for long term population persistence (Bazin et al., 2014). Whether repeated introductions of novel individuals into a population slows down the purging process through selfing or mating between relatives, could perhaps explain the divergent results in the two East coast naturalized populations that had similar outcrossing rates and divergent inbreeding depression estimates for the one year of data presented in this study
Conclusion
In this one-year study we have shown that mating system estimates in native, naturalized, and invasive plant populations of M. guttatus overall support the existence of mixed-mating populations in both the native and non-native regions. There appears to be a relationship between outcrossing rate, population location and persistence history in a particular region. Additionally, we observed variation in estimates of outcrossing rate, inbreeding coefficient, and inbreeding depression. This is in part because of the methodology utilized to estimate the mating system parameters and their inherent sampling design and assumptions. Nevertheless, we have provided evidence for trends supporting the predicted weak relationship between higher outcrossing rates and lower inbreeding depression in the native range and both high rates of outcrossing (with high inbreeding depression) and lower rates of outcrossing (with lower inbreeding depression) in non-native populations of a single species. These results might be due at least in part to the mid-range heterozygosity levels found across the native and invasive study populations but not the naturalized populations. We have also found support for the bridgehead process, which can be thought of as a stepping stone, where one established invasive population provides seed for the establishment of another non-native population. Where a specific population at any point in time we investigate falls along a continuum of their historical persistence in a location is likely variable and dependent on many factors including both sexual and asexual reproduction for these perennial M. guttatus populations.
Data availability statement
The datasets presented in this study can be found in online repositories. The names of the repository/repositories and accession number(s) can be found below: https://doi.org/10.5061/dryad.cvdncjt7c, 0000.
Author contributions
MD: Conceptualization, Funding acquisition, Investigation, Methodology, Project administration, Resources, Supervision, Writing – review & editing. JB: Conceptualization, Data curation, Formal analysis, Investigation, Methodology, Software, Validation, Visualization, Writing – original draft. EZ: Conceptualization, Data curation, Funding acquisition, Project administration, Resources, Supervision, Writing – review & editing.
Funding
The author(s) declare that no financial support was received for the research, authorship, and/or publication of this article.
Acknowledgments
We thank Mario Vallejo-Marin for providing sequences of primers useful for Mimulus guttatus profiling, and Nicole Volmer, Gabriel Johnson, and Juannan Zhou for advice on working with microsatellite screening and analyses. The authors also thank the reviewers for their thoughtful comments that have greatly enhanced the manuscript. The research was funded through discretionary funds provided by both MD and EZ. This paper is part of a dissertation to the graduate school at University of Maryland in partial fulfillment of the requirements for the PhD degree under the mentoring of MD and EZ.
Conflict of interest
The authors declare that the research was conducted in the absence of any commercial or financial relationships that could be construed as a potential conflict of interest.
Publisher’s note
All claims expressed in this article are solely those of the authors and do not necessarily represent those of their affiliated organizations, or those of the publisher, the editors and the reviewers. Any product that may be evaluated in this article, or claim that may be made by its manufacturer, is not guaranteed or endorsed by the publisher.
Supplementary material
The Supplementary Material for this article can be found online at: https://www.frontiersin.org/articles/10.3389/fpls.2024.1411868/full#supplementary-material
References
Aikio, S., Duncan, R. P., Hulme, P. E. (2010). Lag-phases in alien plant invasions: Separating the facts from the artefacts. Oikos 119, 370–378. doi: 10.1111/j.1600-0706.2009.17963.x
Allendorf, F. W., Lundquist, L. L. (2003). Introduction: population biology, evolution, and control of invasive species. Conserv. Biol. 17, 24–30. doi: 10.1046/j.1523-1739.2003.02365.x
Baker, H. G. (1955). Self-compatibility and establishment after ‘long-distance’ dispersal. Evolution 9, 347–349.
Baker, H. G. (1967). Support for baker’s law - as a rule. Evolution 21, 853–856. doi: 10.2307/2406780
Bazin, E., Mathe’-Hubert, H., Facon, B., Carlier, J., Ravigne, V. (2014). The effect of mating system on invasiveness: some genetic load may be advantageous when invading new environments. Biol. Invasions. 16 (4), 875–886. doi: 10.1007/s10530-013-0544-6
Berg, J. A. (2018). Factors that influence the mating system in native and non-native populations of the potentially invasive plant, Mimulus guttatus. Dissertation (College Park, USA: University of Maryland).
Brunet, J., Sweet, H. R. (2006). Impact of insect pollinator group and floral display size on outcrossing rate. Evolution 60, 234–246. doi: 10.1111/j.0014-3820.2006.tb01102.x
Byers, D. L., Waller, D. M. (1999). Do plant populations purge their genetic load? Effects of population size and mating history on inbreeding depression. Annu. Rev. Ecology Evol. Systematics 30, 479–513. doi: 10.1146/annurev.ecolsys.30.1.479
Carr, D. E., Dudash, M. R. (1995). Inbreeding depression under a competitive regime in Mimulus guttatus: Consequences for potential male and female function. Heredity 75, 437–445. doi: 10.1038/hdy.1995.159
Carr, D. E., Fenster, C. B., Dudash, M. R. (1997). The relationship between mating-system characters and inbreeding depression in Mimulus guttatus. Evolution 51, 363–372. doi: 10.1111/j.1558-5646.1997.tb02423.x
Catford, J. A., Jansson, R., Nilsson, C. (2009). Reducing redundancy in invasion ecology by integrating hypotheses into a single theoretical framework. Diversity Distributions 15, 22–40. doi: 10.1111/j.1472-4642.2008.00521.x
Charlesworth, D., Charlesworth, B. (1987). Inbreeding depression and its evolutionary consequences. Annu. Rev. Ecology Evol. Systematics 18, 237–268. doi: 10.1146/annurev.es.18.110187.001321
Crooks, J. A., Soulé, M. E., Sandlund, O. T. (1999). “Lag times in population explosions of invasive species: causes and implications,” in Invasive species and biodiversity management. Eds. Schei, P. J., Viken, Ä. (The Netherlands: Kluwer Academic Publishers), 103–125.
Dart, S. R., Samis, K. E., Austen, E., Eckert, C. G. (2012). Broad geographic variation between floral traits and the mating system in Camissoniopsis cheiranthifolia (Onagraceae): multiple stable mixed mating systems across a species’ range? Ann. Bot. 109, 599–611. doi: 10.1093/aob/mcr266
Dlugosch, K. M., Parker, I. M. (2008). Founding events in species invasions: genetic variation, adaptive evolution, and the role of multiple introductions. Mol. Ecol. 17, 431–449. doi: 10.1111/j.1365-294X.2007.03538.x
Dudash, M. R. (1990). Relative fitness of selfed and outcrossed progeny in a self-compatible, protandrous species, Sabatia angularis L. (Gentianaceae): A comparison in three environments. Evolution 44, 1129–1139. doi: 10.2307/2409277
Dudash, M. R., Carr, D. E. (1998). Genetics underlying inbreeding depression in Mimulus with contrasting mating systems. Nature 393, 682–684. doi: 10.1038/31468
Dudash, M. R., Carr, D. E., Fenster, C. B. (1997). Five generations of enforced selfing and outcrossing in Mimulus guttatus: Inbreeding depression variation at the population and family level. Evolution 51, 54–65. doi: 10.1111/j.1558-5646.1997.tb02388.x
Dudash, M. R., Fenster, C. B. (2000). Inbreeding and outbreeding depression in fragmented populations (UK: Cambridge University Press), 35–54.
Dudash, M. R., Murren, C. J., Carr, D. E. (2005). Using Mimulus as a model system to understand the role of inbreeding depression in conservation: Genetic and ecological approaches. Ann. Missouri Botanic Garden 92, 36–51.
Dudash, M. R., Ritland, K. (1991). Multiple paternity and self-fertilization in relation to floral age in Mimulus guttatus (Scrophulariaceae). Am. J. Bot. 78, 1746–1753. doi: 10.1002/j.1537-2197.1991.tb14539.x
Dussex, N., Morales, H. E., Grossen, C., Dalen, L., van Osterhout, C. (2023). Purging and accumulation of genetic load in conservation. Trends Ecol. Evol. 38, 961–969. doi: 10.1016/j.tree.2023.05.008
Eckert, C. G., Manicacci, D., Barrett, S. C. H. (1996). Genetic drift and founder effect in native versus introduced populations of an invading plant, Lythrum salicaria (Lythraceae). Evolution 4, 1512–1519. doi: 10.2307/2410888
Ellstrand, N. C., Elam, D. R. (1993). Population genetic consequences of small population size: Implications for plant conservation. Annu. Rev. Ecology Evol. Systematics 24, 217–242. doi: 10.1146/annurev.es.24.110193.001245
Eppley, S. M., Pannell, J. R. (2007). Density-dependent self-fertilization and male versus hermaphrodite siring success in an androdioecious plant. Evolution 61, 2349–2359. doi: 10.1111/j.1558-5646.2007.00195.x
Estoup, A., Guillemaud, T. (2010). Reconstructing routes of invasion using genetic data: Why, how and so what? Mol. Ecol. 19, 4113–4130. doi: 10.1111/j.1365-294X.2010.04773.x
Facon, B., Genton, B. J., Shykoff, J., Jarne, P., Estoup, A., David, P. (2006). A general eco-evolutionary framework for understanding bioinvasions. Trends Ecol. Evol. 21, 130–135. doi: 10.1016/j.tree.2005.10.012
Fenster, C. B., Diggle, P. K., Barrett, S. C., Ritland, K. (1995). The genetics of floral development differentiating two species of Mimulus (Scrophulariaceae). Heredity 74, 258–266. doi: 10.1038/hdy.1995.39
Fenster, C. B., Ritland, K. (1994). Evidence for natural selection on mating system in Mimulus (Scrophulariaceae). Int. J. Plant Sci. 155, 588–596. doi: 10.1086/297197
Fisher, R. (1941). Average excess and average effect of a gene substitution. Ann. Eugen 63, 53–63. doi: 10.1111/j.1469-1809.1941.tb02272.x
Fishman, L., Willis, J. H. (2005). A novel meiotic drive locus almost completely distorts segregation in Mimulus (monkeyflower) hybrids. Genetics 169, 347–353. doi: 10.1534/genetics.104.032789
Frankham, R. (2005). Resolving the genetic paradox in invasive species. Heredity 94, 385. doi: 10.1038/sj.hdy.6800634
Friedman, J., Barrett, S. C. H. (2008). A phylogenetic analysis of the evolution of wind pollination in the angiosperms. Int. J. Plant Sci. 169, 49–58. doi: 10.1086/523365
Genton, B. J., Shykoff, J. A., Giraud, T. (2005). High genetic diversity in French invasive populations of common ragweed, Ambrosia artemisiifolia, as a result of multiple sources of introduction. Mol. Ecol. 14, 4275–4285. doi: 10.1111/j.1365-294X.2005.02750.x
Goodwillie, C., Kalisz, S., Eckert, C. G. (2005). The evolutionary enigma of mixed mating systems in plants: Occurrence, theoretical explanations, and empirical evidence. Annu. Rev. Ecology Evol. Systematics 36, 47–79. doi: 10.1146/annurev.ecolsys.36.091704.175539
Grant, A. L. (1924). A monograph of the genus mimulus. Ann. Missouri Botanic Garden 11, 99–388. doi: 10.2307/2394024
Hedrick, P. W. (1994). Purging inbreeding depression and the probability of extinction: full-sib mating. Heredity 73, 363–372. doi: 10.1038/hdy.1994.183
Herlihy, C. R., Eckert, C. G. (2004). Experimental dissection of inbreeding and its adaptive significance in a flowering plant, Aquilegia canadensis (Ranunculaceae). Evolution 58, 2693. doi: 10.1111/j.0014-3820.2004.tb01622.x
Husband, B. C., Schemske, D. W. (1996). Evolution of the magnitude and timing of inbreeding depression in plants. Evolution 50, 54–70. doi: 10.1111/j.1558-5646.1996.tb04472.x
Jarne, P., Auld, J. R. (2006). Animals mix it up, too: The distribution of self-fertilization among hermaphroditic animals. Evolution 60, 1816–1824. doi: 10.1554/06-246.1
Kalisz, S., Vogler, D. W., Hanley, K. M. (2004). Context-dependent autonomous self-fertilization yields reproductive assurance and mixed mating. Nature 430, 884–887. doi: 10.1038/nature02776
Karron, J. D., Thumser, N. N., Tucker, R., Hessenauer, A. J. (1995). The influence of population density on outcrossing rates in Mimulus ringens. Heredity 75, 175–180. doi: 10.1038/hdy.1995.121
Kelly, A. J., Willis, J. H. (1998). Polymorphic microsatellite loci in Mimulus guttatus and related species. Mol. Ecol. 7, 769–774. doi: 10.1046/j.1365-294x.1998.00328.x
Kelly, J. K., Arathi, H. S. (2003). Inbreeding and the genetic variance in floral traits of Mimulus guttatus. Heredity 90, 77–83. doi: 10.1038/sj.hdy.6800181
Kinlan, B. P., Hastings, A. (2005). “What exotic species tell us about rates of population spread and geographic range expansion,” in Species invasions: insights to ecology, evolution and biogeography (Sunderland, Mass: Sinauer Associates), 381–419.
Koelling, V. A., Monnahan, P. J., Kelly, J. K. (2012). A Bayesian method for the joint estimation of outcrossing rate and inbreeding depression. Heredity 109, 393–400. Available at: http://www.python.org/.
Kolar, C. S., Lodge, D. M. (2001). Progress in invasion biology: Predicting invaders. Trends Ecol. Evol. 16, 199–204. doi: 10.1016/S0169-5347(01)02101-2
Kowarik, I. (2003). Human agency in biological invasions: secondary releases foster naturalisation and population expansion of alien plant species. Biol. Invasions 5, 293–312. doi: 10.1023/B:BINV.0000005574.15074.66
Lande, R., Schemske, D. W. (1985). The evolution of self-fertilization and inbreeding depression in plants. I. Genetic Models. Evolution 39, 24–40. doi: 10.1111/j.1558-5646.1985.tb04077.x
Latta, R., Ritland, K. (1994). Conditions favoring stable mixed mating systems with jointly evolving inbreeding depression. J. Theor. Biol. 170, 15–23. doi: 10.1006/jtbi.1994.1165
Leclerc-Potvin, C., Ritland, K. (1994). Modes of self-fertilization in Mimulus guttatus (Scrophulariaceae): A field experiment. Am. J. Bot. 81, 199–205. doi: 10.2307/2445634
Lloyd, D. G., Schoen, D. J. (1992). Self-and cross-fertilization in plants. I. Functional dimensions. Int. J. Plant Sci. 153, 358–369. doi: 10.1086/297040
Lockwood, J. L., Hoopes, M. F., Marchetti, M. P. (2013). Invasion Ecology (Wiley-Blackwell: John Wiley and Sons).
Lombaert, E., Guillemaud, T., Cornuet, J.-M., Malausa, T., Facon, B., Estoup, A. (2010). Bridgehead effect in the worldwide invasion of the biocontrol Harlequin ladybird. PLoS One 5, e9743. doi: 10.1371/journal.pone.0009743
Lowry, D. B., Rockwood, R. C., Willis, J. H. (2008). Ecological reproductive isolation of coast and inland races of Mimulus guttatus. Evolution 62, 2196–2214. doi: 10.1111/j.1558-5646.2008.00457.x
Mack, R. N. (2000). Cultivation fosters plant naturalization by reducing environmental stochasticity. Biol. Invasions 2, 111–122. doi: 10.1023/A:1010088422771
Mack, R. N. (2003). “Global plant dispersal, naturalization, and invasion: pathways, modes, and circumstances,” in Invasive species: vectors and management strategies. Eds. Ruiz, G. M., Carlton, J. T. (Island Press, USA).
Mitchell-Olds, T., Waller, D. M. (1985). Relative performance of selfed and outcrossed progeny in Impatiens capensis. Evolution 39, 533–544. doi: 10.2307/2408651
Morgan, W. H., Palmer, S. C. F., Lambin, X. (2024). Mating system induced lags in rates of range expansion for different simulated mating systems and dispersal strategies: a modelling study. Oecologia. 204, 119–132. doi: 10.1007/s00442-023-05492-w
Murren, C. J., Chang, C. C., Dudash, M. R. (2009). Patterns of selection of two North American native and nonnative populations of monkeyflower (Phrymaceae). New Phytol. 183, 691–701. doi: 10.1111/j.1469-8137.2009.02928.x
Muth, N. Z., Pigliucci, M. (2006). Traits of invasives reconsidered: Phenotypic comparisons of introduced invasive and introduced noninvasive plant species within two closely related clades. Am. J. Bot. 93, 188–196. doi: 10.3732/ajb.93.2.188
Nei, M. (1978). Estimation of average heterozygosity and genetic distance from a small number of individuals. Genetics 89, 583–590. doi: 10.1093/genetics/89.3.583
Pannell, J. R., Auld, J. R., Brandvain, Y., Burd, M., Busch, J. W., Cheptou, P. O., et al. (2015). The scope of Baker’s law. New Phytol. 208, 656–667. doi: 10.1111/nph.2015.208.issue-3
Pannell, J. R., Barrett, S. C. H. (1998). Baker’s law revisited: Reproductive assurance in a metapopulation. Evolution 52, 657–668. doi: 10.2307/2411261
Pantoja, P. O., Simón-Porcar, V. I., Puzey, J. R., Vallejo-Marín, M. (2017). Genetic variation and clonal diversity in introduced populations of Mimulus guttatus assessed by genotyping at 62 single nucleotide polymorphism loci. Plant Ecol. Diversity 10, 5–15. doi: 10.1080/17550874.2017.1287785
Peakall, R., Smouse, P. (2006). GENALEX 6: genetic analysis in Excel. Population genetic software for teaching and research. Molecular Ecology Notes 6, 288–295. doi: 10.1111/j.1471-8286.2005.01155.x
Popovich, D., Lowry, D. B. (2020). Contrasting environmental factors drive local adaptation at opposite end of an environmental gradient in the yellow monkeyflower (Mimulus guttatus). Am. J. Bot. 107, 298–307. doi: 10.1002/ajb2.1419
Prentis, P. J., Wilson, J. R., Dormontt, E. E., Richardson, D. M., Lowe, A. J. (2008). Adaptive evolution in invasive species. Trends Plant Sci. 13, 288–294. doi: 10.1016/j.tplants.2008.03.004
Preston, C. D., Pearman, D. A., Dines, T. D. (2002). New Atlas of the British and Irish Flora (Oxford: Oxford University Press).
Puzey, J., Vallejo-Marín, M. (2014). Genomics of invasion: Diversity and selection in introduced populations of monkeyflowers (Mimulus guttatus). Mol. Ecol. 23, 4472–4485. doi: 10.1111/mec.2014.23.issue-18
R Core Team. (2016). R: A Language and Environment for Statistical Computing. Vienna, Austria. Retrieved from https://www.R-project.org/.
Ralls, K., Ballou, J. D., Dudash, M. R., Eldridge, M. D. B., Fenster, C. B., Lacy, R. C., et al. (2018). Call for a paradigm shift in the genetic management of fragmented populations. Conserv. Lett. 11, 1–6. doi: 10.1111/conl.12412
Rambuda, T. D., Johnson, S. D. (2004). Breeding systems of invasive alien plants in South Africa: Does Baker’s rule apply? Diversity Distributions 10, 409–416. doi: 10.1111/j.1366-9516.2004.00100.x
Randle, A. M., Slyder, J. B., Kalisz, S. (2009). Can differences in autonomous selfing ability explain differences in range size among sister-taxa pairs of Collinsia (Plantaginaceae)? An extension of Baker’s Law. New Phytol. 183, 618–629. doi: 10.1111/j.1469-8137.2009.02946.x
Reichard, S. H., White, P. (2001). Horticulture as pathways of plant introductions in the United States. Am. Institute Biol. Sci. 51, 103–113. doi: 10.1641/0006-3568(2001)051[0103:HAAPOI]2.0.CO;2
Richardson, D. M., Pysek, P., Rejmanek, M., Barbour, M. G., West, C. J., Panetta, F. D. (2000). Naturalization and invasion of alien plants: concepts and definitions. Diversity Distributions 6, 93–107. doi: 10.1046/j.1472-4642.2000.00083.x
Richardson, D. M., Pysek, P. (2006). Plant invasions: merging the concepts of species invasiveness and community invasibility. Progress in Physical Geography 30, 409–431. doi: 10.1191/0309133306pp490pr
Richardson, D. M., Rejmánek, M. (2011). Trees and shrubs as invasive alien species–a global review. Diversity and Distributions 17, 788–809. doi: 10.1111/j.1472-4642.2011.00782.x
Ritland, K. (1990). Inferences about inbreeding depression based on changes of the inbreeding coefficient. Evolution 44, 1230–1241. doi: 10.2307/2409284
Ritland, K., Ganders, F. (1987). Covariation of selfing rates with parental gene fixation indices within populations of Mimulus guttatus. Evolution 41, 760–771. doi: 10.2307/2408886
Ritland, C., Ritland, K. (1989). Variation of sex allocation among eight taxa of the Mimulus guttatus species complex (Scrophulariaceae). Am. J. Bot. 76, 1731–1739. doi: 10.1002/j.1537-2197.1989.tb15163.x
Rodger, Y. S., Dillon, R., Monro, K., Pavlova, A., Coates, D. J., Byrne, M., et al. (2024). Benefits of outcrossing and their implications for genetic management of an endangered species with mixed-mating system. Restor. Ecol. 32, e14057. doi: 10.1111/rec.14057
Sax, D. F., Stachowicz, J. J., Brown, J. H., Bruno, J. F., Dawson, J. F., Gaines, S. D., et al. (2007). Ecological and evolutionary insights from species invasions Ecological and evolutionary insights from species invasions. Trends Ecol. Evol. 22, 465–471. doi: 10.1016/j.tree.2007.06.009
Schemske, D. W., Lande, R. (1985). The evolution of self-fertilization and inbreeding depression in plants. II. Empirical observations. Evolution 39, 41–52. doi: 10.1111/j.1558-5646.1985.tb04077.x
Stebbins, G. L. (1957). Self-fertilization and population variability in the higher plants. Am. Nat. 91, 337–354. doi: 10.1086/281999
Thuiller, W., Lavorel, S., Araújo, M. B., Sykes, M. T., Prentice, I. C. (2005). Climate change threats to plant diversity in Europe. Proc. Natl. Acad. Sci. 102, 8245–8250. doi: 10.1073/pnas.0409902102
Truscott, A.-M., Palmer, S. C. F., Soulsby, C., Hulme, P. E. (2008). Assessing the vulnerability of riparian vegetation to invasion by Mimulus guttatus: relative importance of biotic and abiotic variables in determining species occurrence and abundance. Diversity Distributions 14, 412–421. doi: 10.1111/j.1472-4642.2007.00449.x
Truscott, A.-M., Soulsby, C., Palmer, S. C. F., Newell, L., Hulme, P. E. (2006). The dispersal characteristics of the invasive plant Mimulus guttatus and the ecological significance of increased occurrence of high-flow events. J. Ecol. 94, 1080–1091. doi: 10.1111/j.1365-2745.2006.01171.x
Tsutsui, N. D., Suarez, A. V., Holway, D. A., Case, T. J. (2000). Reduced genetic variation and the success of an invasive species. Proc. Natl. Acad. Sci. 97, 5948–5953. doi: 10.1073/pnas.100110397
Vallejo-Marin, M., Friedman, J., Twyford, A. D., Lepais, O., Ickert-Bond, S. M., Streisfeld, M. A., et al. (2021). Population genomic and historical analysis suggests a global invasion by bridgehead processes in Mimulus guttatus. Commun. Biol. 4, 327. doi: 10.1038/s42003-021-01795-x
Vallejo-Marin, M., Lye, G. C. (2013). Hybridisation and genetic diversity in introduced Mimulus (Phrymaceae). Heredity 110, 111–122. doi: 10.1038/hdy.2012.91
Vallejo-Marín, M., Solis-Montero, L., Bacles, C. F. E., Lepais, O. (2011). Thirteen microsatellites developed by SSR-enriched pyrosequencing for Solanum rostratum (Solanaceae) and related species. American Journal of Botany 98, e296–e299. doi: 10.3732/ajb.1100159
Vallejo-Marin, M., O'Brien, H. E. (2007). Correlated evolution of self-incompatibility and clonal reproduction in Solanum (Solanaceae). New Phytol. 173, 415–421. doi: 10.1111/j.1469-8137.2006.01924.x
van Kleunen, M., Fischer, M. (2008). Adaptive rather than non-adaptive evolution of Mimulus guttatus in its invasive range. Basic Appl. Ecol. 9, 213–223. doi: 10.1016/j.baae.2007.03.006
van Kleunen, M., Johnson, S. D. (2007). Effects of self-compatibility on the distribution range of invasive European plants in North America. Conserv. Biol. 21, 1537–1544. doi: 10.1111/j.1523-1739.2007.00765.x
van Treuren, R., Bijlsma, R., Ouborg, N. J., van Delden, W. (1993). The effects of population size and plant density on outcrossing rates in locally endangered Salvia pratensis. Evolution 47, 1094–1104. doi: 10.2307/2409977
Vickery, R. K. (1959). Barriers to gene exchange within Mimulus guttatus (Scrophulariaceae). Evolution 13, 300–310. doi: 10.2307/2406107
Whitehead, M. R., Lanfear, R., Mitchell, R. J., Karron, J. D. (2018). Plant mating systems often vary widely among populations. Front. Ecol. Evol. 6. doi: 10.3389/fevo.2018.00038
Willis, J. H. (1993). Effects of different levels of inbreeding on fitness components in Mimulus guttatus. Evolution 47, 864–876. doi: 10.2307/2410190
Wilson, J. R. U., Dormontt, E. E., Prentis, P. J., Lowe, A. J., Richardson, D. M. (2009). Something in the way you move: Dispersal pathways affect invasion success. Trends Ecol. Evol. 24, 136–144. doi: 10.1016/j.tree.2008.10.007
Winn, A. A., Elle, E., Kalisz, S., Cheptou, P.-O., Eckert, C. G., Goodwillie, C., et al. (2011). Analysis of inbreeding depression in mixed-mating plants provides evidence for selective interference and stable mixed mating. Evolution 65, 3339–3359. doi: 10.1111/j.1558-5646.2011.01462.x
Wu, C. A., Lowry, D. B., Cooley, A. M., Wright, K. M., Lee, Y. W., Willis, J. H. (2008). Mimulus is an emerging model system for the integration of ecological and genomic studies. Heredity 100, 220–230. doi: 10.1038/sj.hdy.6801018
Yuan, Y.-W. (2019). Monkeyflowers (Mimulus): new model system for plant developmental genetics and evo-devo. New Phytol. 222, 694–700. doi: 10.1111/nph.2019.222.issue-2
Zell, A., Yost, J., Ritter, M., Grossenbacher, D. (2023). Phenotypic variation in seep monkeyflowers along a fine-scale soil moisture gradient. Madrono 70, 138–150. doi: 10.3120/0024-9637-70.3.138
Zhang, Q., Zhang, J., Sun, X., Feng, W., Wang, R., Wang, H., et al. (2024). Mating strategies of Vitex negundo L. Var. heterophylla (Franch.) Rehder (Lamiaceae): a mixed mating system with inbreeding depression. Ecol. Evol. 14, e11165. doi: 10.1002/ece3.11165
Keywords: colonization, mating system, sexual reproduction, asexual reproduction, non-native populations
Citation: Dudash MR, Berg JA and Zimmer EA (2024) Progeny array analysis to estimate outcrossing rates, inbreeding coefficients, and inbreeding depression among native, naturalized, and invasive populations of Mimulus guttatus (Phrymaceae). Front. Plant Sci. 15:1411868. doi: 10.3389/fpls.2024.1411868
Received: 03 April 2024; Accepted: 02 September 2024;
Published: 21 November 2024.
Edited by:
Johanne Brunet, Brunet Research, United StatesReviewed by:
Adrian Christopher Brennan, Durham University, United KingdomJeremie Benjamin Fant, Chicago Botanic Garden, United States
Copyright © 2024 Dudash, Berg and Zimmer. This is an open-access article distributed under the terms of the Creative Commons Attribution License (CC BY). The use, distribution or reproduction in other forums is permitted, provided the original author(s) and the copyright owner(s) are credited and that the original publication in this journal is cited, in accordance with accepted academic practice. No use, distribution or reproduction is permitted which does not comply with these terms.
*Correspondence: Michele R. Dudash, bWljaGVsZS5kdWRhc2hAc2RzdGF0ZS5lZHU=
†ORCID: Michele R. Dudash, orcid.org/0000-0002-8634-3527
Elizabeth A. Zimmer, orcid.org/0000-0003-3949-4290