- 1Department of Plant Biology and Ecology, Pharmacy Faculty, University of the Basque Country, Universidad del País Vasco/Euskal Herriko Unibertsitatea (UPV/EHU), Vitoria-Gasteiz, Spain
- 2Department of Crop, Soil, and Environmental Sciences, Auburn University, Auburn, AL, United States
- 3Department of Plant Biology, University of Illinois, Urbana, IL, United States
- 4Department of Biology, Loyola University Chicago, Chicago, IL, United States
- 5Department of Mathematics and Computer Science, University of La Rioja, Logroño, Spain
Introduction: Drought is one of the biggest problems for crop production and also affects the survival and persistence of soil rhizobia, which limits the establishment of efficient symbiosis and endangers the productivity of legumes, the main source of plant protein worldwide.
Aim: Since the biodiversity can be altered by several factors including abiotic stresses or cultural practices, the objective of this research was to evaluate the effect of water availability, plant genotype and agricultural management on the presence, nodulation capacity and genotypic diversity of rhizobia.
Method: A field experiment was conducted with twelve common bean genotypes under irrigation and rain-fed conditions, both in conventional and organic management. Estimation of the number of viable rhizobia present in soils was performed before the crop establishment, whereas the crop yield, nodule number and the strain diversity of bacteria present in nodules were determined at postharvest.
Results: Rainfed conditions reduced the number of nodules and of isolated bacteria and their genetic diversity, although to a lesser extent than the agrochemical inputs related to conventional management. In addition, the effect of water scarcity on the conventional management soil was greater than observed under organic conditions.
Conclusions: The preservation of diversity will be a key factor to maintain crop production in the future, as problems caused by drought will be exacerbated by climate change and organic management can help to maintain the biodiversity of soil microbiota, a fundamental aspect for soil health and quality.
1 Introduction
Nitrogen-fixing bacteria are a widely distributed phylogenetic group of prokaryotic microorganisms that play a crucial role in the functioning of ecosystems since they are involved in the entry of nitrogen into the soils (Borges et al., 2016). These microorganisms take atmospheric nitrogen (N2), the most abundant component of the atmosphere, and convert it to assimilable nitrogen for plants (NH4+), using the nitrogenase enzyme. Although most species can fix nitrogen in their free-living form, some of the microorganisms need to be associated with plants, thus symbiotic associations account for 50–70% of biological nitrogen fixation (BNF) in the world (Prasuna, 2014; Simon et al., 2014).
This symbiosis provides legumes a relevant ecological advantage as well as exceptional nutritional properties. Through BNF, legumes fulfil the N requirements needed for their growth (Masson-Boivin and Sachs, 2018; Song et al., 2024). This reduces the need of synthetic N fertilizers and improves the N content of the soils, increasing their fertility and, enabling crop development in N poor soils (Aserse et al., 2020; Lindström and Mousavi, 2020). Thereby, in farming systems, legumes are often used in crop rotation, as well as green fertilizers (Araújo et al., 2015; Aserse et al., 2020). Based on their ability to colonize low-N environments and represent an alternative for saving inputs and conserving resources, the Food and Agriculture Organization of the United Nations (FAO, 2013), considers legumes as one of the most promising components of the climate smart agriculture concept.
Drought is the most severe abiotic stress in agriculture, limiting crop growth and yields, and due to climate change, drought events are expected to increase in the early years, especially in southern Europe (IPCC, 2021). It is therefore essential to seek strategies to maintain food security in a sustainable way under water-limited conditions, and the selection of drought tolerant genotypes is one of the most important goals in breeding programs.
However, in the case of legumes, several authors have suggested that selecting drought tolerant rhizobia strains could be a more determining factor in drought tolerance than selecting a drought tolerant genotype (Mhadhbi et al., 2011; Sharaf et al., 2019). Thus, the establishment of symbiotic relationships with efficient rhizobia can alleviate the effects of stress in legumes (Igiehon et al., 2021; Omari et al., 2022; Oviya et al., 2023). This is the case of common bean, where it has been observed that symbiosis with drought-tolerant rhizobia improves plant tolerance to stress as well as legume yield and quality (Steiner et al., 2020; Rodiño et al., 2021; del-Canto et al., 2023), even under field conditions (Pastor-Bueis et al., 2019; Rodiño et al., 2021).
Rhizobia, are abundant in the soil of many ecosystems and show a great diversity at the species level, as well as great variability in their symbiotic efficiency (Borges et al., 2016; Lindström and Mousavi, 2020; Guanzon et al., 2023). Unfortunately, several abiotic factors such as drought can influence the survival, functioning and diversity of soil rhizobia, and thus, legume crop productivity (Benjelloun et al., 2019; Sharaf et al., 2019; Santillana Villanueva, 2021), because few strains of rhizobia show high tolerance to water stress (Sharaf et al., 2019; Sindhu et al., 2020). In addition, strain survival and competitiveness are not correlated with their N2 fixation efficiency (De Souza et al., 2016; Kibido et al., 2019; da Silva et al., 2024), reducing the possibilities of establishing efficient symbiotic relationships.
Therefore, a higher diversity of legume nodulating bacteria in the soil will maximize the biological nitrogen fixation under stress conditions (Borges et al., 2016; Lindström and Mousavi, 2020; Martins et al., 2024). In this sense, greater soil microbial diversity will favour the adaptation of microbial populations to different environments, increasing the likelihood of survival of stress-tolerant microbial species and the establishment of effective symbiotic relationships (Wang and Young, 2019), contributing to greater crop resilience to stress. In addition, a greater biodiversity improves soil structure, nutrient cycling, and nutrient and water uptake, especially under drought conditions (Prudent et al., 2020).
Unfortunately, conventional agriculture related practices such as the use of herbicides and fungicides have a negative effect on the soil microbiota survival and diversity, reducing the efficiency of symbiotic relationships (Silva Neto et al., 2013; Da-Silveira-Cardillo et al., 2019; Rao et al., 2019). Additionally, the continued use of inorganic N-fertilizers causes the evolution of less-mutualistic rhizobia (Heath and Tiffin, 2007; Weese et al., 2015; Rao et al., 2019), and the decrease of nodules production (Heath et al., 2010; Regus et al., 2016). Thereby, with domestication and breeding in high-soil-N environments, the natural legume defences against less-effective rhizobia strains have been altered favouring less-cooperative rhizobia and reducing the agricultural benefits of the symbiosis (Weese et al., 2015).
Organic or sustainable farming, contrary to conventional production, promotes the biodiversity of agrosystems based on the concept that the greater biodiversity of the system, the greater health and resilience of ecosystems (Pimentel et al., 2005; Kremen and Miles, 2012; Wang and Young, 2019). The diversity of crops and their rotation system prevents the total depletion of nutrients from soils (Wolff and Killebrew, 2010; Hossain and Bakhsh, 2020) increasing soil fertility, and the diversity and activity of soil micro and macrobiotic communities (Herencia et al., 2020; Prudent et al., 2020). In this regard, studies about the abundance and diversity of rhizobia in soils, as well as the factors affecting both parameters, are of special importance to study the responsiveness of agrosystems to stresses (Omari et al., 2022).
According to different authors, the conservation of soil rhizobial diversity in agrosystems is a sustainable strategy of great interest to improve crop tolerance to stress by favouring the establishment of efficient symbiotic relationships even under water scarcity conditions (Wolff and Killebrew, 2010; Szparaga et al., 2019; Hossain and Bakhsh, 2020). In addition to this, there is interest in the search for indigenous inocula that are better adapted to local growing conditions and therefore more efficient in responding to stress conditions (del-Canto et al., 2023).
This is especially important in common bean under drought conditions as it is a very drought-sensitive crop (Embrapa, 2018; Nawaz et al., 2021) with a high frequency of inefficient symbiotic relationships (Michiels et al., 1998; Mwenda et al., 2023) that affect productivity. Considering the high nutritional value of common bean and that it is the grain legume for human consumption with the highest production worldwide (Beebe et al., 2013; FAO, 2021), the search for strategies to improve its productivity under conditions of low water availability is a challenge of great interest.
With this in mind, our hypothesis was that the type of management would have an effect on bean response to water stress, as greater microbial diversity could favour greater crop resilience. To test this, we analysed the effect of management (organic, conventional) on the production of several common bean genotype under water scarcity and how they affect the abundance, nodulation capacity and genotypic diversity of common bean rhizobia.
2 Materials and methods
2.1 Plant material
Twelve bushy genotypes of Phaseolus vulgaris, most of them of great economic interest in the North of Spain, were selected for the evaluation of the effect of water scarcity and management system in nodulation and rhizobia diversity. Of the twelve genotypes, four correspond to commercial genotypes and eight to locally adapted genotypes from different rainfall areas (Online resource Supplementary Table SI.1). The eight local genotypes, not studied to date, are traditionally grown on small family farms typically under rainfed conditions. Five of them are from the Basque Country (Northern Spain): Arrocina de Álava (AA), Amarilla de Kuartango (AK), Morada de Usánsolo (MU), Pinta Alavesa (PA) and Verde de Orbiso (VO). One is originally from Navarra (Northern Spain), Negra de Basaburua (NB), and two from Castilla y León (Central Spain), Canela de León (CL) and Riñón de León (RL). The other four are commercial genotypes commonly grown all around Spain: Cocco Blanco (CB), Lingot (L), Negrita (N), also marketed as “Frijol Negro”, and Borlotto de Vigevano (B).
2.2 Location and soil characterization
The trials were performed in NEIKER experimental farm located in Arkaute, Álava (Spain), between May and August of 2015. Arkaute (WGS84: 42.850254, -2.621362) is located at 532 meters above sea level and has an oceanic climate, type Cfb (temperate oceanic climate or subtropical highland climate), according to the Köppen Geiger climate classification (1900), which is, a temperate and humid climate, in transition with the Mediterranean climate (information obtained from Euskalmet, Euskal meteorologia agentzia). The average temperature throughout the growing season was 17.8°C, with three days having a minimum temperature less than 5°C and 6 days having a maximum temperature that exceeded 35°C. The accumulated precipitation during the experiment was 116.5 mm.
The field experiment was conducted under conventional and organic management. Both types of soils were catalogued according to European standards and the current legislations of the Government of Spain (MAPA, 2023), which describe and classify the type of practices in each management system. The soil of the selected plots for the study presented close locations (one contiguous to the other), similar cropping histories (rotations between cereals, potatoes, vegetables and legumes), and generally were grown under irrigated conditions (Figure 1). The main differences in the management history of both soils were due to agrochemical supplies. In the conventional plots, different agrochemicals (herbicides, pesticides, and chemical fertilization) were frequently applied depending on the different types of crops and the requirements of each year. In the other hand, organic plots avoided the use of synthetic chemical products. The supply of nitrogen and nutrients was provided through organic amendments, according to the Council Regulation EC No 834/2007 of 28 June 2007. The conventionally managed soil has been worked under this type of management for more than ten years, while the organically managed soil has been worked in this way, without the use of synthetic chemicals, for five years. Although common beans were frequently grown in both soils, no plot had a history of commercial inoculation with rhizobia. Therefore, all the possible rhizobium inoculums were naturally occurring.For the soil characterization, eight soil samples 20 cm deep were randomly collected from each soil (conventional and organic) and analysed at the Fraisoro Agro-environmental Laboratory (Diputación Foral de Gipuzkoa) to study their physical-chemical characteristics, according to the official methods of analysis of the Ministry of Agriculture of Spain (MAPA, 1994). The Ph, electrical conductivity in calcium sulfate (EC, µS·cm-1) and effective cation exchange capacity (CEC, meq·100 ml-1) were determined by ADAS method; the organic matter content (OM, %) was determined by the Walkley-Black method without heat input; the nitrogen (N) content (%), by Kjeldahl method; the phosphorous (P) content (mg·L-1), by the Olsen-Watanabe method performing an extraction in sodium bicarbonate at pH 8.5; the content of potassium (K, mg·L-1), calcium (Ca, mg·L-1) and magnesium (Mg, mg·L-1), by ADAS method with extraction in ammonium nitrate and subsequent reading in ICP-OES. The C/N balance was calculated based on the following formula:
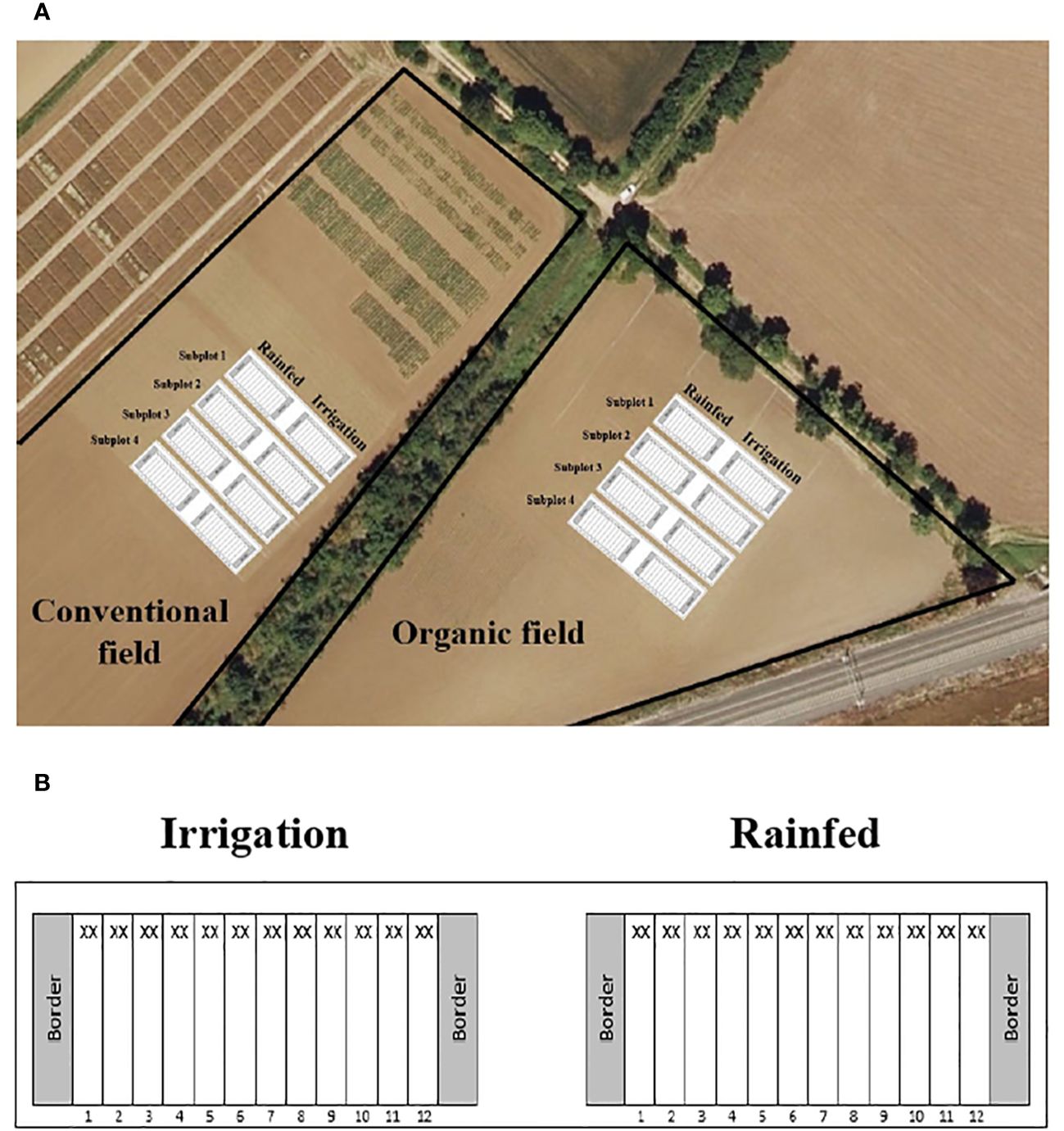
Figure 1 Location of the two crop fields of the study, including the experimental design scheme (A), and detail the subplots (B).
Where 1.72 is the factor of Van Bemmelen for the conversion of organic matter into Carbon. Finally, the granulometric characteristics of the soil (percentages of fine sand, coarse sand, silt, and clay) were determined according to the ISSS soil particle size fraction system.
2.3 Estimation of the number of viable rhizobia present in soils
The number of viable rhizobia present in the soils was estimated according to the methodology described by Hungría and Araujo (1994) with little modifications. The most probable number (MPN) of viable rhizobia is an indirect method for counting rhizobia present in soils. This method assumes that an infectious or viable rhizobia is capable of developing a nodule. While a negative result, absence of nodules, indicates the absence of infectious rhizobia. For that, before the crop establishment, soil samples were taken from both conventional and organic management. The soil samples were passed through a 4 mm sieve. Then, 10 g of processed soil was diluted in 95 mL of diluent solution (phosphate buffer, pH 7.3) in a beaker with glass pearls on a horizontal shaker at slow speed at a temperature of 25°C for 30 minutes. From this soil solution, five serial dilutions were made (1 mL of previous solution and 9 mL of diluent solution), making four replications of each one. In addition, a small aliquot of the sieved soil sample was weighed (fresh weight, FW), and after drying in the oven at 80°C for two days, the sample was reweighed (dry weight, DW) in order to calculate the humidity factor (HF) and to compare the different soils regardless of its water content with the following formula:
Previously, sterilized common bean seeds of Arrocina de Álava genotype (10 min in sodium hypochlorite 1%) were germinated in opaque cultivation jars containing 500 mL of nitrogen-free Fahraeus solution (Fahraeus, 1957). The seeds were held in a funnel, connected to the nutrient solution by a filter paper wick. Then, each of the seedlings were inoculated with one of the serial solutions of soil and grown in a growth chamber (Ibercex SA, Alcalá de Henares) under controlled conditions (12 h photoperiod, light intensity 500 µmoles of photons·m-2·s-1, 20/25°C temperature and 70/60% relative humidity, night/day respectively). After three weeks, the number of plants that developed nodules (positives) and those that did not (negatives) were counted. From these values, using a mathematical formula or a table of results (Hungría and Araujo, 1994), and considering the soil humidity factor (HF), the MPN of viable rhizobia was estimated, as well as the occurrence probabilities and lower and upper limits of the 95% confidence interval.
2.4 Experimental design and growth conditions
Irrigated and rain-fed treatments were performed in two subplots from each selected management plot. One subplot for conventional and the other for organic management. The 12 genotypes were sown by hand at a depth of 2 cm using a randomized block design with 4 biological replicates per water regime. A distance of 5 m separated each block, which consisted of two 10 m long rows with a 0.5 m between rows and 0.2 m separation between plants within the row, achieving a stand density of 100,000 plants ha-1.
The water inputs were estimated controlling the irrigation time of the irrigation system (6 mm·h-1 flow), while the rainfall data were taken from the weather station Arkaute I (Euskalmet, 2023) located close to the experimental fields. Under rain-fed conditions, the seeds from common bean genotypes described above only received a minimum amount of water after sowing to assure the emergence and seedlings survival (12 mm). Afterwards the seedlings only received rainwater (116.5 mm). For the irrigated trials, three supplemental irrigations were supplied to plants during their growth (12 mm each). Therefore, the irrigated plots received a total contribution of 164.5 mm, while those under rainfed conditions received 128.5 mm, which is, a 22% reduction of water availability.
Under organic management, weeding control was mechanical and manual. In fields under conventional management, various herbicides were applied: preemergence herbicide Linuron 500 g·L-1 (Linurex ® 50 SC, Adama Chile SA), at a minimum recommended dose of 0.8 L·ha-1; and post-emergence herbicide, pendimethalin 45.5% (Stomp® Aqua, BASF), at a minimum recommended dose of 2 L·ha-1, at the V3 development stage, plants with the first trifoliate leaf (Fernández et al., 1986).
2.5 Nodule sampling
In the pre-flowering stage, R5 (Fernández et al., 1986), the nodules from three randomly selected plants from each experimental plots were collected, with four replicates of each, i.e. 12 nodules were harvested from each experimental condition (three plants of 12 genotypes, grown under two different water regimes in two different agricultural managements, with four replications) a total of 576 plants. Using a shovel, and measuring 20 cm from the center of the plat, the root system was excavated until a depth of 26 cm totalling a soil volume of 10.4 L per plant, similarly to Somasegaran and Hoben (1994), to ensure the harvest of practically the entire root system. Once in the laboratory, whole plants were carefully removed from the soil to obtain roots and nodules, and adhering soil was removed from roots by careful shaking. Then, the removed soil was carefully examined to recover any nodules left in the soil. Harvested nodules were washed in water and gently dried with paper. The nodules were counted and desiccated in bottles with silica gel at 4°C to preserve and use them in future research.
2.6 Yield quantification
At harvest, all plants from each block were counted, collected individually, dried, threshed, and cleaned separately to quantify the yield (Kg·ha-1). Four biological replicates of each experimental conditions.
2.7 Endosymbiont isolation
The use of common bean “trap plants” grown in local field under rainfed conditions, could guarantee that isolated rhizobia have a certain competitiveness and possibly tolerance to water stress. Therefore, once the four most productive genotypes under rainfed conditions and a less productive one were selected, the bacteria present in their nodules were extracted from eight nodules randomly picked from each plot according to Sanz-Sáez et al. (2015). That is, 8 nodules per experimental condition, a total of 160 nodules (8 nodules of 5 genotypes grown under rainfed and irrigation conditions in two agricultural management systems). The nodules were first rehydrated by immersion in sterile distilled water for 2-4 h and surface-sterilized by immersing them in 70% ethanol (5 sec), and then in 50% sodium hypochlorite (5 min). Later, they were rinsed several times with sterile distilled water to remove the bleach residues. The nodules were crushed in a petri dish and 10-20 μL of autoclaved distilled water was added on the medulla of the nodule, aspirating, and expiring several times with the micro pipette to collect the bacteria (Somasegaran and Hoben, 1994; Howieson and Dilworth, 2016) and placing on a plate with solid TY medium (Beringer, 1974) to be grown at a constant temperature of 29°C.
Once the bacteria had grown, a single colony was chosen randomly and replated to a new solid TY plate for purification of rhizobia isolates. This operation was repeated for all observed colonies of different morphology and appearance from each plate. Using this method, 368 total isolates were obtained. These pure cultures were preserved at 4°C and were then transferred to 50% glycerol in TY, to preserve them at -80°C, in order to use them in future trials.
2.8 Isolated strain diversity by BOX+REP polymerase chain reaction genomic fingerprinting
Consensus sequences such as repetitive extragenetic palindromic sequences (REP), enterobacterial repetitive intergenic consensus (ERIC) and BOX elements, related to repetitive and conservative elements diffused in DNA, have been extensively used for rhizobial strain identification due to its ease, quickness, and high discriminatory power at infraspecific level (Kaschuk et al., 2006; Borges et al., 2016; Benjelloun et al., 2019). In our study, we used BOX and REP-PCR since the application of both PCR methods increases the accuracy when compared with only one PCR (Olive and Bean, 1999). The DNA extraction was performed according to Heath and Tiffin (2009) with modifications: 104 μL of liquid medium TY (Beringer, 1974) incubated with bacteria during 24-48 h at 28°C was centrifuged for 5 min at 16000 g and the pellet containing the cells was washed 3 times with 1 M NaCl and once with 100% ethanol and dried before extracting DNA. Then, the cells were re-suspended in 104 µL of sterile milli-Q water and homogenizing gently with the micropipette. The suspension was centrifuged 10 min at 16000 g and the aqueous phase was removed. For the DNA extraction, 30 µL of 10 mM tris-HCL (pH 8) and 1 µL of 20 mg·mL-1 protein kinase K enzyme (Invitrogen, Carisbad, CA) was added to the pellet and homogenized again with the micropipette and vortex. Then, 30 µL were transferred to a 0.2 mL PCR tube, and 55°C was applied for 4 h in order to let the protein kinase K to perform its function. After that it was deactivated by heating the solution at 95°C for 10 min. DNA samples were quantified using a NanoDrop spectrophotometer (Thermo scientific, Wilmington, DE, USA) and diluted to 25 ng μL-1. The DNA extraction was stored at 4°C.
BOX-PCR was performed according to the method described by Kaschuk et al., 2006 with modifications, using the BOX A1R primer (5′-CTACGG CAAGGCGACGCTGACG-3′; Versalovic et al., 1991). REP-PCR was performed according to the method described by Versalovic et al., 1991 with modifications, using the primers REP-1 (5´-IIIICGICGICATCIGGC-3´) and REP-2 (5´-ICGICTTATCIGGCCT AC-3´). Each PCR reaction was performed in a final volume of 10 μL containing: dNTPs 0.3 μL (10 mM); reaction buffer 1μL (10x BioLabs, New England); primer 0.5 μL (10 mM), for two primers in REP; Taq DNA polymerase 0.08 μL (5 Um·L-1); Betaine 0.5 μL (5 mM), DNA 1.5 μL; sterile milli-Q water to complete the volume. The amplification program was performed in a thermocycler S1000™ (Bio-Rad Laboratories, Inc.), applying an initial denaturing step (95°C, 7 min) with 30 cycles of denaturation (95°C, 1 min), annealing (53°C, 8 min for BOX-PCR and 40°C; 8 min for REP-PCR) and extension (65°C, 8 min); and a final extension cycle (65°C, 16 min). The PCR products were separated by horizontal electrophoresis on a 1.5% agarose gel (EEO-Mr<0.15) in TBE buffer (0.5x) at 90 V for 5 h, using a 1 Kb DNA marker (BioLabs, New England). The gels were stained with ethidium bromide, and visualized under UV light using a benchtop UV transilluminator (Bio-Doc-It™ UVP Imaging System).
2.9 Statistical analysis
The diversity of strains was analysed from the images of the gels (PCR fingerprints) using the free software GelJ v.2.0 (Heras et al., 2015) and transformed into a binary matrix. After analysing a large number of samples, the most consistent bands were selected, with 70% or more percentage of appearance. BOX and REP-PCR data were combined for each isolate.
From this BOX+REP binary matrix, Python software was used to build similarity matrices. Using the Jaccard coefficient and applying the UPGMA algorithm unweighted pair-group method with arithmetic mean (Sokal, 1973), the dendrogram and diversity indexes (Shannon and Weaver, 1949), richness (Margalef, 1958) and evenness (Pielou, 1977) were obtained. The graphic representations of the dendrograms were made with the free software iTOL (Interactive tree of Life, Leunic and Bork, 2019). Through these analyses, the different isolates were grouped according to the degree of similarity of their PCR fingerprints in different clusters. The number of clusters also indicates the strain diversity or diversity at infraspecific level. The number of clusters and their bootstrapping was calculated at 70% similarity. Due to the observed high variability, to obtain larger clusters, 35% similarity was also used (Grange and Hungria, 2004).
The nodule number and yield was analysed using the statistical package SPSS Statistics 24.0 (IBM Corporation, Armonk, NY, USA). The normality of the non-standardized residuals of the data was studied using the Shapiro-Wilk test and the homoscedasticity of the variance was studied with the Levenne test. As the water availability treatment was not randomized and organic and conventional management soils were separated, the behaviour of the genotypes was studied in the four experimental conditions: irrigated conventional management; rainfed conventional management; irrigated organic management; and rainfed organic management as it has been performed previously by Sanz-Sáez et al. (2019). For this, a one-way analysis of variance (ANOVA) was performed with genotypes as factor and replicates as random effect. When the genotype effect was significant, least square means post-hoc test was performed to compare means (Tukey or Kruskal Wallis). The management effect was also analysed separating the data according to the water regime through one-way analysis of variance (ANOVA), with agricultural management as factor and replicates as random effect. The graphic representations of the nodule number were made with SigmaPlot 15 (Systat Software, Inc.).
As one of the objectives of this research was to investigate the effect of water scarcity over the nodulation, the two management systems were treated as two different locations (environments) and the effect of water scarcity was analysed for each location separately, despite not being randomized. One-way ANOVA with water availability was treated as factor and replicates as random effect.
3 Results
3.1 Soil characterization
The properties and characteristics of soils cultivated under organic and conventional management were similar (Table 1). Both were clay-loam soils with a very light salinity, had a basic pH typical of limestone soils, contained an optimal carbon balance, and contained adequate nitrogen and phosphorus content with a medium magnesium content. However, both soils differed in their organic matter, magnesium and potassium content all being higher in the organic management soil, while higher calcium content occurred in the conventional management field.
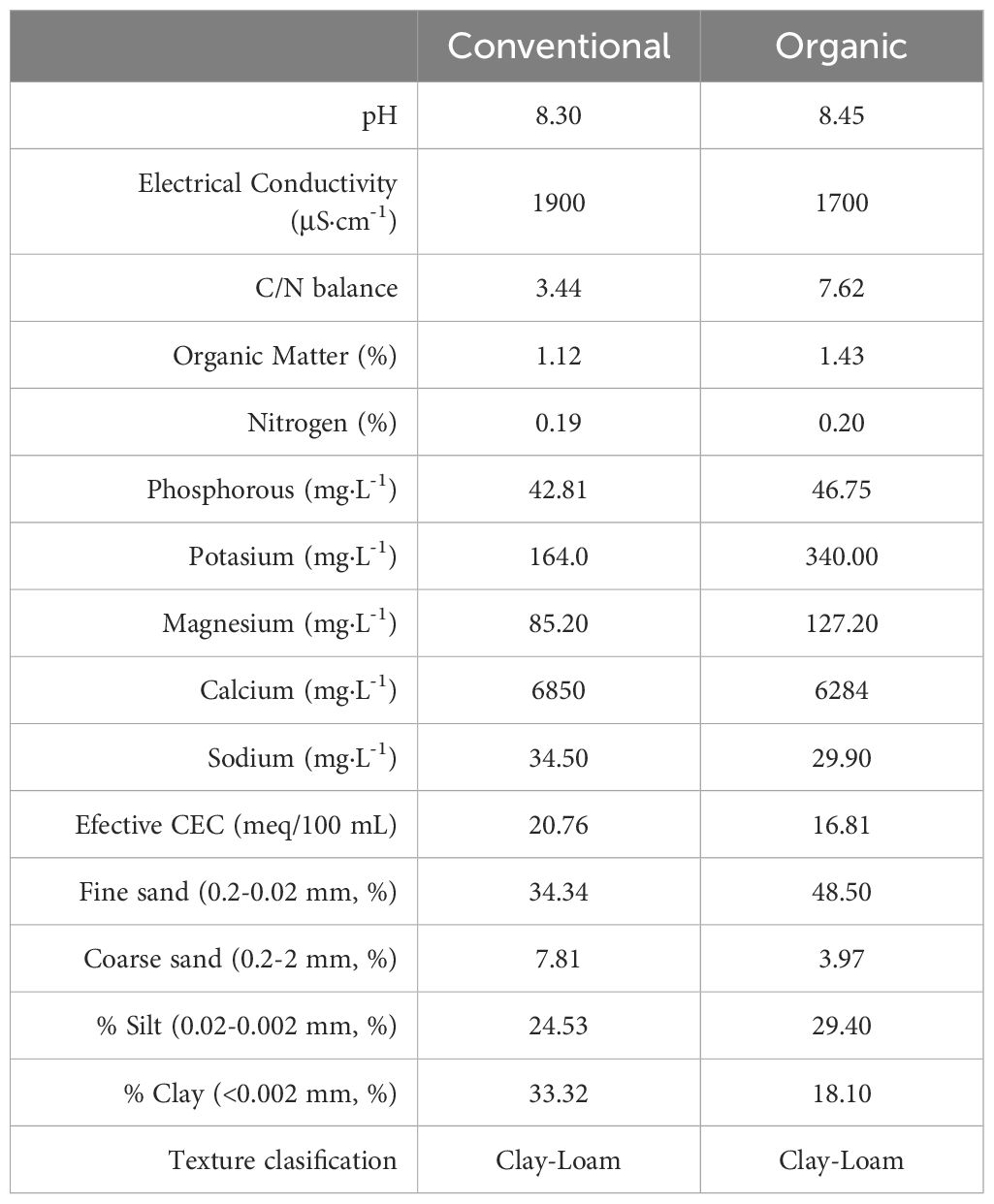
Table 1 Physical-chemical characteristics of conventional and organic agricultural soils of the experimental fields.
3.2 Estimation of the number of viable rhizobia present in soils
The estimation of rhizobia cells existing in the sampled soils before sowing and the establishment of water treatment are shown in Table 2. The MPN shows the number of live rhizobia cells present per unit of volume in the matrix solution taking into consideration the soil humidity correction factor which allows for comparisons of the different soils regardless of water content. The MPN values were more than 20-fold higher in the organic (21.102) than in the conventional (0.609) management soil (Table 2).
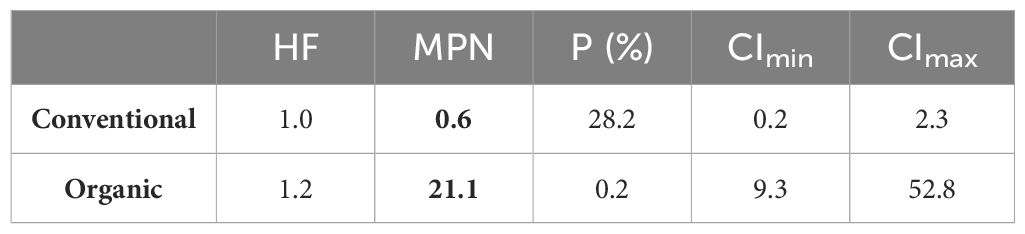
Table 2 Most probable number of viable rhizobia in organic and conventional plots before sowing: HF, soil humidity factor; MPN, most probable number of viable rhizobia cells present in the soil matrix solution corrected with HF; P (%), probability of the combination occurrence if the experiment is repeated an infinite number of times with the same matrix solution; CImin and CImax, lower and upper limits of the 95% confidence interval.
3.3 Effect of water stress, agricultural management and legume genotypes on nodulation
The infection capacity of rhizobia, showed as nodule number per plant, was almost 58.25% lower, on average, in conventional management than in organic management (Table 3). The number of nodules was also reduced by low water availability in both management systems although its effect was less evident than that of the management itself. Although there was not management by water availability interaction, rainfed conditions seemed to decrease the rhizobia infection capacity more in conventional (24.56%) than in organic management (20.30%) (Table 3).
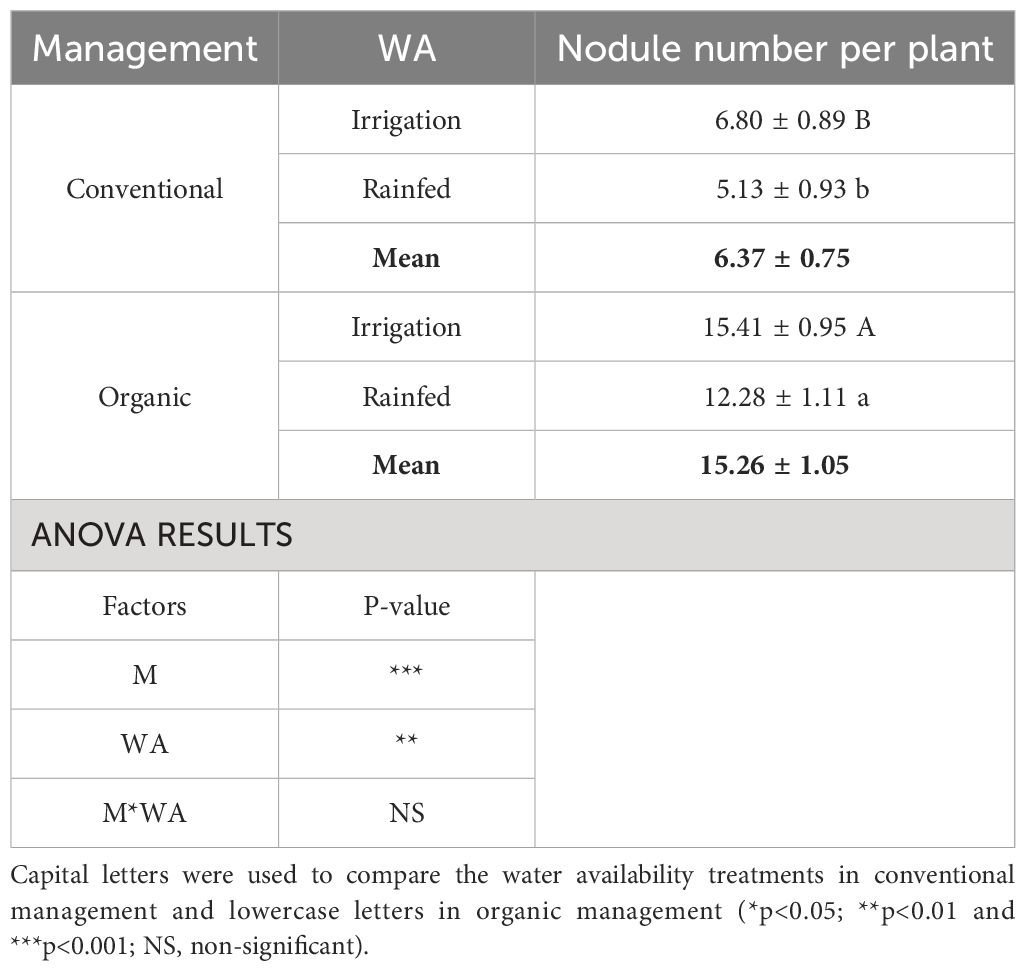
Table 3 Mean values ( ± SE) and ANOVA results (p-value) of nodule number per plant of different common bean plants grown under different water availability (WA) conditions: irrigated and rainfed and in different management systems (M), conventional and organic.
The nodulation capacity also varied depending on the genotypes although all of them showed higher nodulation capacity in organic than in conventional management (Figure 2). The genotypes RL and MU showed a greater number of nodules under irrigation in both management systems, while CB, PA and CL showed the least in the conventional management, and B showed the least in the organic management field. Reduced water availability only decreased nodule number in RL and NB genotypes in the organic plots, whereas in conventional plots no significant effect of rainfed conditions was observed in any of the genotypes tested. RL was by far the genotype that developed a greater number of nodules under rainfed conditions in conventional management (Figure 2) but was more affected by water availability in organic management. Under organic management, the MU and L cultivars were those that showed greater nodulation under rainfed conditions, unlike CB, B and NB that were the less nodulated genotypes. The B and NB genotypes also showed less nodules in conventional management under rainfed conditions. Therefore, the genotypes that most times showed the highest nodulation capacity were the RL and MU, while the B and NB were the ones showing the lowest nodulation capacity.
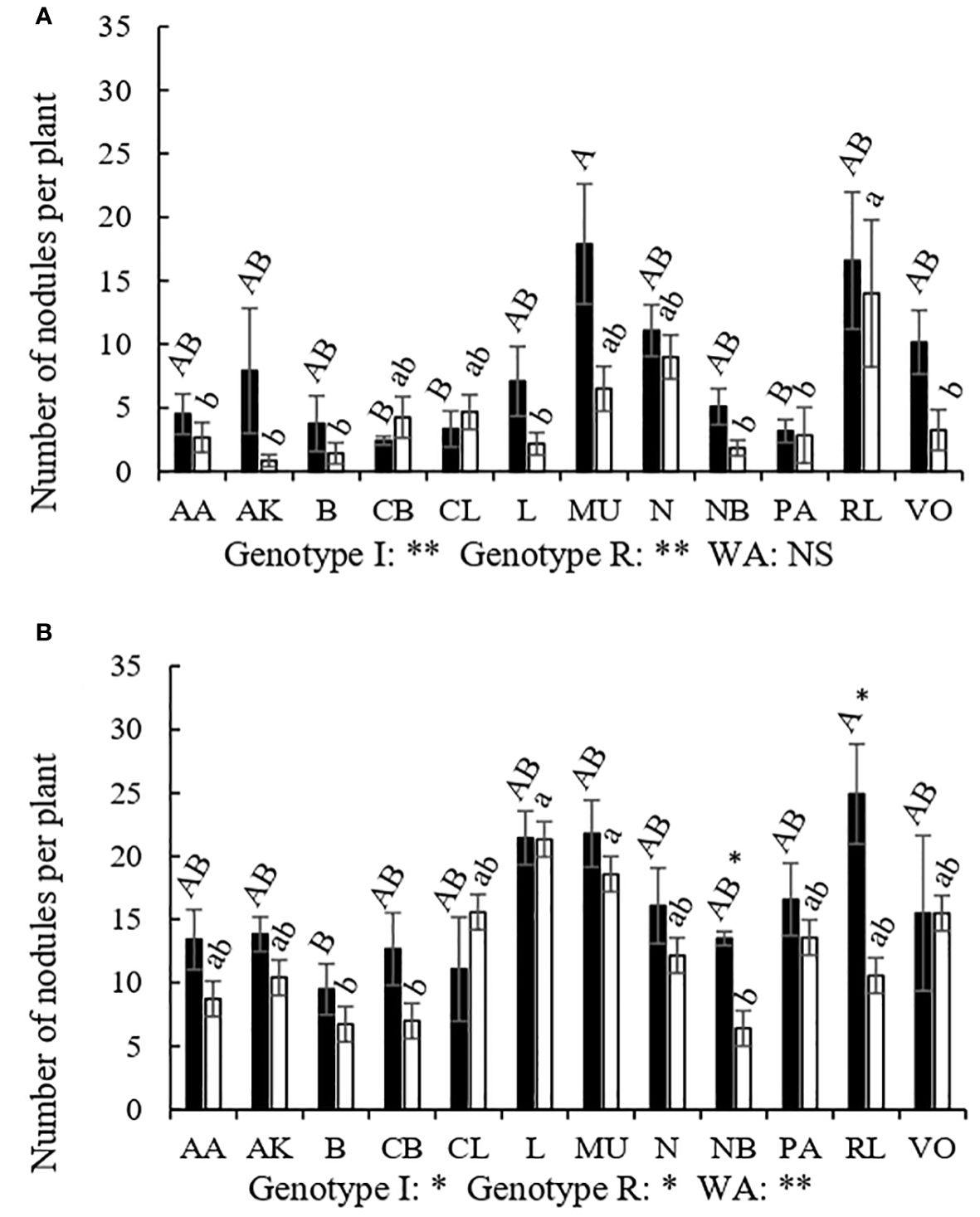
Figure 2 Number of nodules per plant of different genotypes of Phaseolus vulgaris (AA, Arrocina de Álava; AK, Amarilla de Kuartango; B, Borlotto; CB, Coco blanco; CL, Canela de León; L, Lingot; MU, Morada de Usansolo; N, Negrita; NB, Negra de Basaburua; PA, Pinta alavesa; RL, Riñón de León; y VO, Verde de Orbiso), under different water availability (WA) treatments in the field: irrigation (dark) and rainfed (light); both in conventional (A) and organic management (B). Bars indicate the mean of each genotype for number of nodules. Different capital letters designate significantly different genotype means under irrigated conditions while lower case letters designate significant different genotype means for the rainfed treatment according to LSD post-hoc analysis. Asterisks withing the figures were used to show the effect of water in each genotype separately. Genotype I illustrate the p-value of the genotype effect under irrigated conditions; Genotype R illustrates the p-value of the genotype effect under rainfed conditions; WA, indicated the effect of water availability factor in each management (*p<0.05; **p<0.01 and ***p<0.001; NS, non-significant).
3.4 Yield quantification
The yields obtained in conventional management were higher than those obtained with organic management. However, while rainfed conditions considerably reduced common bean production under conventional management, water scarcity did not significantly reduce organic bean production (Table 4).
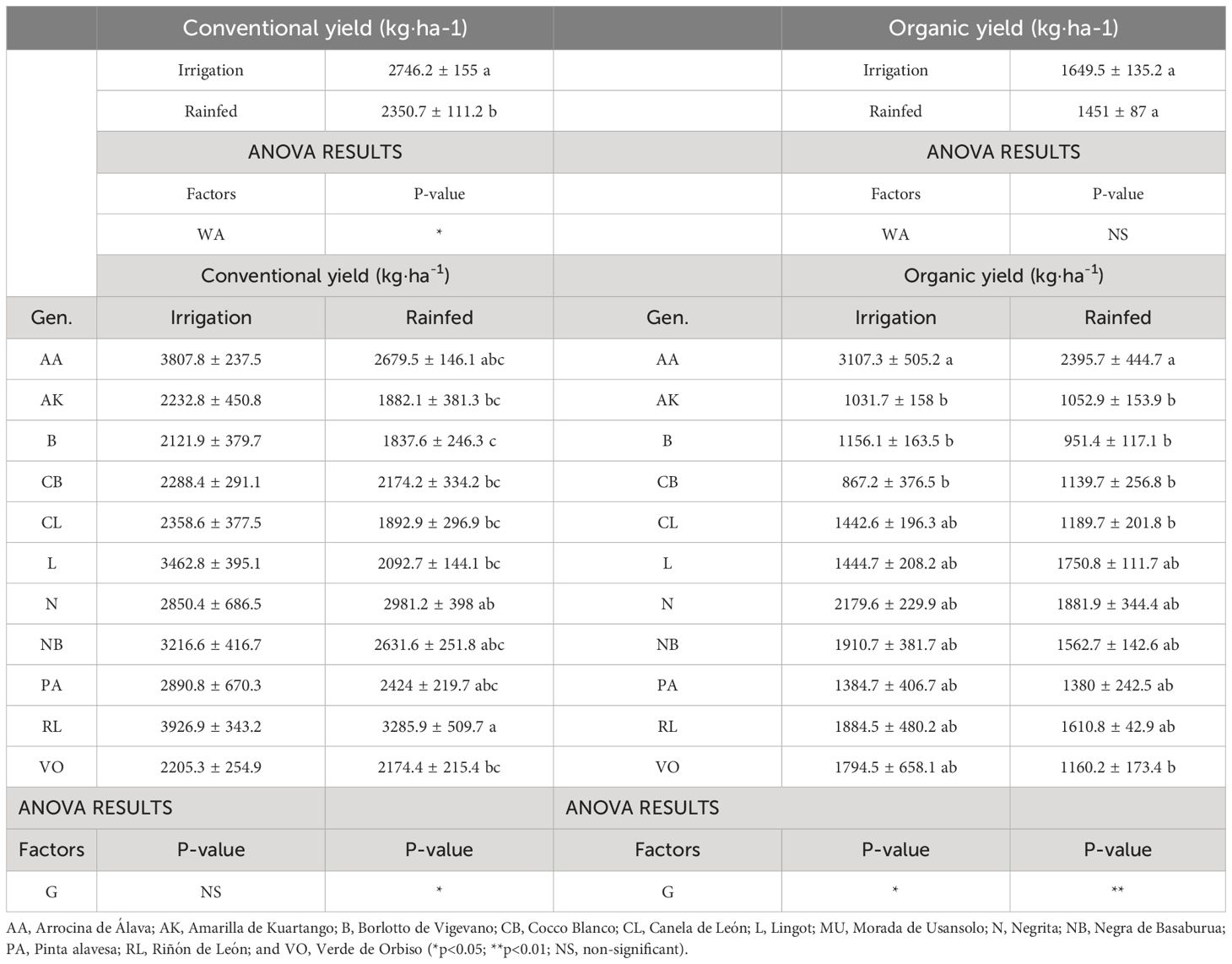
Table 4 Mean values ( ± SE) and ANOVA results (p-value) of the effect of water availability (WA) and genotype (G) on common bean yield (kg·ha-1). both in conventional and organic management.
On the other hand, it was observed that AA, N, NB and RL were the genotypes that ranked as most productive under different experimental conditions, also under rainfed conditions, while AK was one of the least productive (Table 4). Thus, those were the genotypes selected to study the diversity of the bacteria strains inside their nodules.
3.5 Isolated strain diversity by BOX+REP PCR genomic fingerprinting
From the 368 bacteria isolated from nodules of plants grown under different water availability in both organic and conventional management, BOX+REP PCR fingerprinting were obtained on 320 isolates, since some strains did not amplify with the primers used similarly to other literature (Judd et al., 1993; Mostasso et al., 2002; Kaschuk et al., 2006).
The genomic fingerprinting showed a high level of genetic diversity among the strains, considering a similarity of 70% in the clustering analysis, confirmed by the low final levels of similarity (Online Resource, Supplementary Table SI.2). With this level of similarity (70%) the vast majority of the generated clusters in the different experimental conditions were composed of a single strain and the values obtained from bootstrapping were generally greater than 0.60, thus, can be considered stable groups (Jain and Moreau, 1987; Kerr and Churchill, 2001). To obtain larger clusters, formed by a greater number of strains, the isolated strains were also grouped at a 35% similarity level (Figure 3, Tables 5, 6). Thus, larger clusters were obtained and formed by several strains, although bootstrap values remained below 0.60.
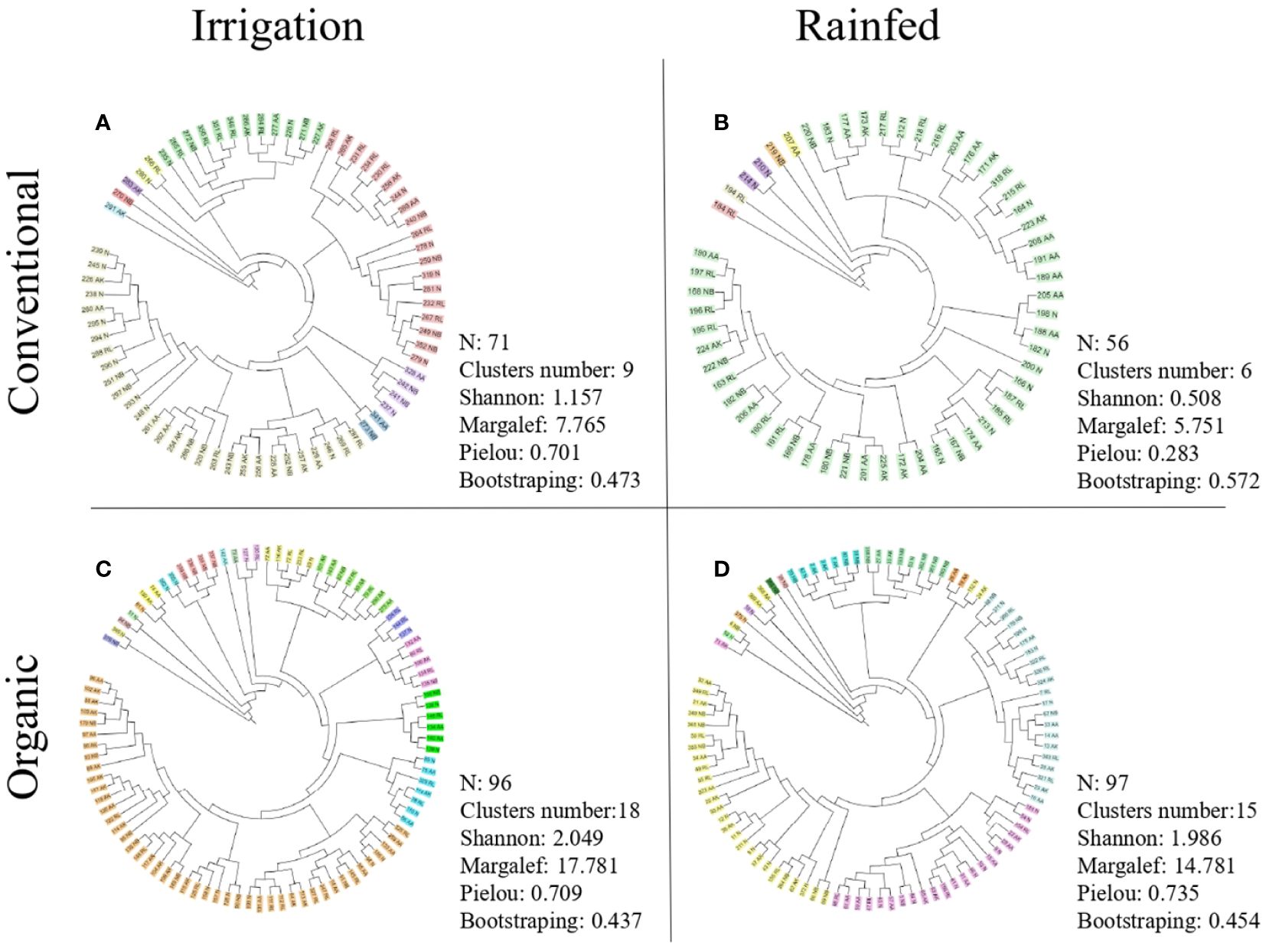
Figure 3 Dendrogram constructed from the BOX+REP PCR genomic fingerprinting. Number of isolated bacteria (N), number of clusters (represented graphically with different colours), diversity indices (Shannon, Margalef and Pielou) and cluster bootstraping obtained at a 35% similarity level in: conventional management under irrigation conditions (A); conventional management under rainfed conditions (B); organic management under irrigation conditions (C); and organic management under rainfed conditions (D).
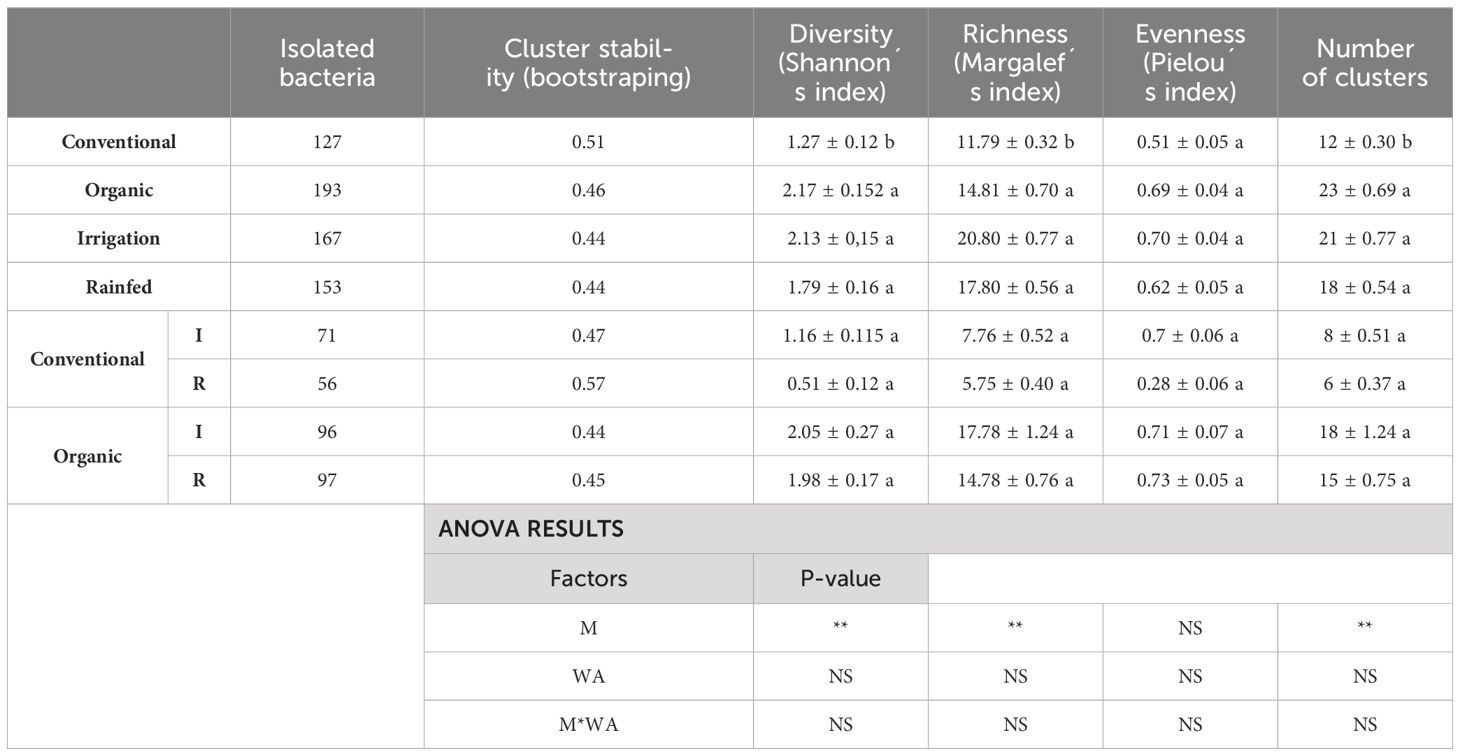
Table 5 Number of isolated bacteria, average stability of clusters and mean values ( ± SE) and ANOVA results (p-value) of strain diversity indices (Shanon, Margalef and Pielou) and number of obtained clusters at a similarity level of 35% according to agricultural management (conventional and organic) and water availability (I, irrigated; and R, rainfed) (*p<0.05; **p<0.01 and ***p<0.001; NS, non-significant).
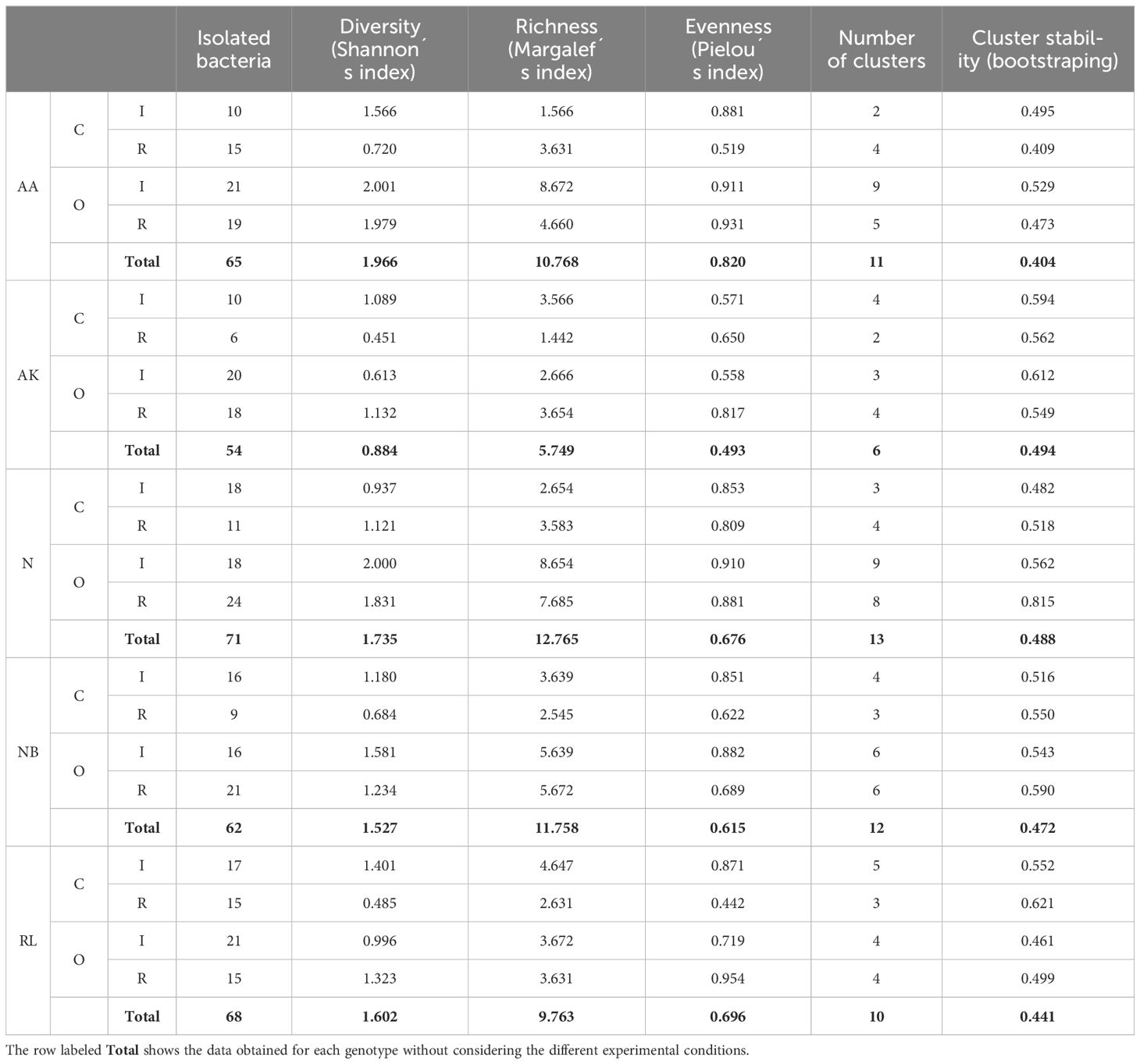
Table 6 Number of isolated bacteria, strain diversity indices (Shanon, Margalef and Pielou) and number, structure and average stability of clusters obtained at a similarity level of 35% according to agricultural management (C, conventional; O, organic), water availability (I, irrigated; R, rainfed), and genotype (AA, Arrocina de Álava; AK, Amarilla de Kuartango; N, Negrita; NB, Negrita de Basaburua; RL, Riñon de Leon).
The number of isolated bacteria (Online resource, Supplementary Table SI.2, Supplementary Table SI.3), clusters, diversity (Shannon, H0), richness (Margalef, R1) and evenness (Pielou, E1) of strains were greater in the nodules of the plants grown under organic management than under conventional management, regardless of the considered similarity level (Tables 5, 6, for 35% of similarity and Online Resource, Supplementary Table SI.2, Supplementary SI.3, for 70% of similarity). Rainfed conditions also caused a drop, although not statistically significant, in the diversity indices (Shannon, Margalef and Pielou), number of isolates and obtained clusters, at both levels of similarity (Table 5 and Online resource, Supplementary Table SI.2). This can be observed when analysing both managements separately based on water availability (Figure 3). Nodules of plants grown in organic soils always showed higher strain genetic diversity (diversity indices and number of clusters), even under rainfed conditions, with respect to the most favourable conditions (irrigation) of conventional management (Figure 3, Table 5 and Online resource Supplementary Table SI.2).
In conventional management under irrigated conditions, 71 bacteria were isolated and grouped into 9 clusters, two of which represent 67.6% of the strains. Under rainfed conventional management, the isolated bacteria were reduced to 56 bacteria and 89.3% of them were grouped into one large cluster (Figure 3). Nevertheless, under organic management and irrigation conditions, 96 bacteria were isolated and grouped into 18 clusters, with the largest cluster representing 46.9% of the isolated bacteria. Under rainfed organic conditions, 97 strains were isolated and grouped into 15 clusters, three of which represented 71.1% of the isolated bacteria. Rainfed conditions reduced the number of isolated bacteria strains by 21.1% under conventional management but not in organic management soil. The genetic diversity of strains (number of clusters) was reduced by 33.3% in conventional management and by 16.7% in organic management due to low water availability (Figure 3).
Finally, when analysing the different genotypes (Table 6), AK showed the lowest number of isolated bacteria (54), the lowest indexes of genetic diversity and the lowest number of clusters. On the other hand, N and NB, showed the highest indexes of genetic diversity and a greater number of clusters (13 and 12 respectively). N was the genotype from which the greatest number of bacteria were isolated (71).
4 Discussion
The great diversity that rhizobia show at the species level is essential for Phaseolus vulgaris to stablish efficient symbiosis and maintain crop productivity in a sustainable way, especially under drought conditions (Lindström and Mousavi, 2020; Guanzon et al., 2023; da Silva et al., 2024). Rhizobia can play a more important role in the resistance to stress of the symbiosis relationship than the genotype of plant (Mhadhbi et al., 2011; Sharaf et al., 2019). Unfortunately, the symbiotic efficiency of rhizobia is highly variable and, on many occasions, establish inefficient symbiotic relationships with common bean having a negative impact on productivity (Michiels et al., 1998; Armenta-Borjóquez et al., 2016; Mwenda et al., 2023). Thus, greater microbial diversity in soils and the better the response of crops to stress will increase the probability of appearance of efficient stress-tolerant microbial species (Wang and Young, 2019) that can maximize biological nitrogen fixation under such conditions (Borges et al., 2016; Lindström and Mousavi, 2020; Liyanage et al., 2023).
The BOX and REP-PCR fingerprinting analysis showed a high level of genetic diversity among the bacteria isolated from nodules of all the sampled plants grown under the different agronomic conditions. These data confirm the great promiscuity of the common bean plants which are capable of nodulating with many different bacteria. Our results are comparable to those of other studies using similar techniques, in which most of the groups, clustered at 70% similarity, were formed just by one or two strains (Grange and Hungria, 2004; Chibeba et al., 2020; Odori et al., 2020).
In most clusters, the values obtained from bootstrapping were close to 0.60 at 70% similarity and even lower values were obtained at 35% of. These low bootstrap values are usually obtained in these type of dendrograms (Aulakh et al., 2020) due to both, the type of data (they are not related sequences), and to the large number of operational taxonomic units (OTUs, bacterial strains), which contribute to lower the value of the bootstraps (Efron and Tibshirani, 1993). However, despite the low bootstrap values, this technique is considered a powerful tool to detect strain diversity (Alberton et al., 2006; Kaschuk et al., 2006; Costa et al., 2018).
Water scarcity provokes reduction of nodule number per common bean plant (Berny-Mier et al., 2019; Aserse et al., 2020; Prudent et al., 2020). In this study, a reduction of only 22% in water availability affected the nodulation efficiency, reducing the number of nodules per plant and the number of isolated bacteria per nodule. However, it did not significantly affect the strain genetic diversity, although there was a tendency to decrease under water scarcity conditions.
Water scarcity is one of the factors that most affect the rhizobia survival in their free-life phase (Hungria et al., 2000; Sindhu et al., 2020; Santillana Villanueva, 2021) and the nodulation process, affecting chemotaxis, initiation, formation and development of nodules (Sindhu et al., 2020; Viti et al., 2021; Omari et al., 2022) thus reducing the number of nodules. In fact, under sufficiently prolonged or severe drought conditions the nodule formation can be completely inhibited (Gerosa-Ramos et al., 2003). In drought-affected soils, the decrease of bacterial diversity and nodulation capacity also translates into a lower diversity of the nodule bacteriome, reducing the number of isolated bacteria and the genetic diversity of detected strains. Similar effects were described in soybean (Sharaf et al., 2019) and common bean (Cytryn et al., 2007).
The reduction of the bacterial diversity parameters due to lower water availability was observed in both managements, however, the effect was slightly higher under conventional management than that observed under organic conditions. In the latter, water scarcity had no effect on yield and higher nodulation, higher number of isolated strains and greater genetic diversity of strains were recorded even under rainfed conditions. These results would demonstrate that organic soil´s microbiota reflect wider environmental adaptation and superior competitive ability (Yan et al., 2017; Costa et al., 2018) and would confirm that these soils have greater resilience to adverse conditions such as water stress via greater microbial abundance and diversity (Borges et al., 2016; Lindström and Mousavi, 2020). As some authors suggest, the maintenance of the soil microbial genetic biodiversity is of great importance because it provides a major buffering capacity of the soil (Loreau et al., 2001) and it is related to soil health and quality as well as agricultural sustainability (Kaschuk et al., 2006).
Conventional plots showed lower abundance and diversity in the soil microbiome even before sowing and under irrigated conditions. This would be related to the practices and agrochemicals used in conventional agriculture (Rao et al., 2019), since the two sampled agricultural soils, conventional and organic, had similar edapho-climatic conditions and both presented similar cropping histories and soil characteristics.
The soil of conventional management plots was subjected to years of agrochemicals and N-fertilization inputs. Mineral fertilization reduces plant nodulation (Heath et al., 2010; Regus et al., 2016) and symbiotic efficiency (Heath and Tiffin, 2007; Weese et al., 2015; Gordon et al., 2016; Rao et al., 2019) because it is energetically cheaper for plants to reduce ammonium and nitrate than to fix N2. This leads to a reduction on the presence and diversity of rhizobia over time (Moreira et al., 2006; Da-Silveira-Cardillo et al., 2019). In addition, during the experiment, two doses of herbicides, Pedimethalin and one of Linuron, were applied to conventional plots. There is quite a consensus on the negative effect of herbicides on bacterial diversity. Coinciding with our observations, García-Garijo et al (2014) detected that Imazamox drastically affected the nodulation and biological nitrogen fixation of common beans, and Darine et al. (2015) reported that Fusilade herbicide causes a decline in richness and structure of soil bacterial communities, mainly at the rhizosphere level. Furthermore, although the literature contains little information on the effects of pesticides on legume-rhizobia signal exchange, some in vitro work with 30 different pesticides showed that Sinorhizobium meliloti NodD was affected resulting in delayed nodulation and reduced biological nitrogen fixation in Medicago sativa (Fox et al., 2001).
Consequently, the use of agrochemicals leads to a loss of abundance and diversity in the soil microbiome (Rao et al., 2019), as well as of rhizobia (Silva Neto et al., 2013; Weese et al., 2015), reducing plant nodulation and genetic diversity of strains. On the contrary, organic amendments, such as manure, a common practice in organic management, usually increase the abundance and diversity of microorganisms and rhizobia (Rao et al., 2019; Li et al., 2022), promoting a greater plant nodulation (Herencia et al., 2020), as in our results.
This negative effect of agrochemicals on common bean symbiosis was even more drastic than that produced by a reduction of 22% in water availability. In fact, the use of agrochemicals reduced nodulation, the number of isolated bacteria and the genetic diversity of nodule bacteriome strains, while water availability only reduced nodulation, and no significant effect on strain diversity was observed.
Although in organic management no effect of water scarcity was observed in yield, confirming a greater resilience to water stress (Borges et al., 2016; Lindström and Mousavi, 2020), the yields obtained in this management were lower than in conventional management, probably due to the difficulty of weed control, since the physical and chemical conditions were not very different in both fields. Therefore, it is evident the need to study different strategies to improve crop productivity in organic management to avoid yield losses, such us a better weed control, one of the prime causes of yield losses in organic management (Entz et al., 2001; Posner et al., 2008).
Finally, we also detected an effect of plant genotype on nodule number, as it has been observed in several publications (Berny-Mier et al., 2019; Goyal et al., 2021; Omari et al., 2022), although without interaction with the rest of the studied factors. In addition, we detected differences in the diversity of isolated strains across genotypes, as it was observed in soybeans (Sharaf et al., 2019) and in common bean (Shamseldin and Velázquez, 2020). Although some authors have not found effect of common bean genotype on rhizobial diversity (Grange and Hungria, 2004), other researchers have reported that the legume plants control the nodulation process (Ferguson et al., 2019), and the involvement of several Phaseolus vulgaris genes in strain-specific selection (Rípodas et al., 2019).
RL and MU were the genotypes that showed more nodules in both management practices, also under rainfed conditions. RL was also the second genotype with a higher number of strains, after the N genotype. On the contrary, NB, was the least nodulated genotype, but showed great strain diversity and was one of the most productive genotypes under limited water conditions. On the other hand, AK, a genotype selected as the least productive, showed few nodules and low number of isolated bacteria, with less strain diversity under rainfed conditions. These results agree with the findings of other authors (Aserse et al., 2020) that suggest that the number of nodules is not always related to biological nitrogen fixation efficiency (Zurdo-Piñeiro et al., 2009; Flores-Félix et al., 2019; Shamseldin and Velázquez, 2020). However, the number of nodules gives an idea of the abundance and/or the viability of the rhizobia present in soils, and it has also been stated that more drought-tolerant strains show higher nodulation capacity under drought conditions (Aulakh et al., 2020; Omari et al., 2022; del-Canto et al., 2023).
The results show that the rhizobia isolated in this work, are efficient inocula able to nodulate under rainfed conditions as was demonstrated with some of them (del-Canto et al., 2023) and it would be interesting to test their efficiency under field conditions, following successful formulations such as those described by Pastor-Bueis et al. (2019).
5 Conclusion
Rainfed conditions reduced the number of nodules per plant and the number of isolated bacteria, however, the use of agrochemicals products related to conventional management had a greater negative effect than that observed by a reduction of 22% in water availability, and also affected the strain genetic diversity of the nodule bacteriome. In addition, while water limitation did not have an effect on the organic management yield, it was reduced in conventional management. Consequently, the effect of rainfed conditions on the conventional management soil was greater than observed under organic conditions.
These results would confirm that the use of agrochemicals leads to a loss of rhizobia abundance and diversity while organic management practices maintains higher values of rhizobia abundance, nodulation and diversity, even under rainfed conditions. This maintenance of diversity will be a key factor in the future, as problems caused by drought will be exacerbated by climate change. Maintaining microbial diversity implies broader environmental adaptation, superior competitive ability and greater resilience to adverse conditions. Therefore, it is necessary to develop sustainable and environmentally friendly agricultural systems, free of agrochemicals that allows for maintaining or even increasing the biodiversity of soil microbiota, a fundamental aspect for soil health and quality.
Data availability statement
The raw data supporting the conclusions of this article will be made available by the authors, without undue reservation.
Author contributions
AD: Data curation, Formal analysis, Investigation, Methodology, Visualization, Writing – original draft, Writing – review & editing. AS: Conceptualization, Data curation, Formal analysis, Supervision, Writing – review & editing. KH: Conceptualization, Resources, Supervision, Writing – review & editing. MG: Investigation, Methodology, Writing – review & editing. JH: Software, Visualization, Writing – review & editing. ML: Conceptualization, Data curation, Funding acquisition, Investigation, Project administration, Resources, Supervision, Writing – review & editing.
Funding
The author(s) declare financial support was received for the research, authorship, and/or publication of this article. This work was founded by research projects from the Basque Government: Grupo de Investigación del Sistema Universitario Vasco IT1022-16 and T1682-22; and projects from the Basque Country Government: Dpto. de Desarrollo Económico e Infraestructuras (32-2016-00043) and Dpto. de Desarrollo Económico, Sostenibilidad y Medioambiente (37-2017-00047, 00049-IDA2019-38 and 0039-IDA2021-45). A. Del-Canto was the recipient of a predoctoral fellowship granted by the Education Department of the Basque Country Government, Spain.
Acknowledgments
We thank Mr. Gary Wayne Morgan for his contribution reviewing the manuscript for English Grammatical correctness. We also thank Agustín Miranda del Ama for collaborating in the nodule sampling.
Conflict of interest
The authors declare that the research was conducted in the absence of any commercial or financial relationships that could be construed as a potential conflict of interest.
The author(s) declared that they were an editorial board member of Frontiers, at the time of submission. This had no impact on the peer review process and the final decision.
Publisher’s note
All claims expressed in this article are solely those of the authors and do not necessarily represent those of their affiliated organizations, or those of the publisher, the editors and the reviewers. Any product that may be evaluated in this article, or claim that may be made by its manufacturer, is not guaranteed or endorsed by the publisher.
Supplementary material
The Supplementary Material for this article can be found online at: https://www.frontiersin.org/articles/10.3389/fpls.2024.1408125/full#supplementary-material
Abbreviations
AA, Arrocina de Álava; AK, Amarilla de Kuartango; BNF, biological nitrogen fixation; B, Borlotto de Vigevano; CL, Canela de León; CB, Cocco Blanco; DW, dry weight; FW, fresh weight; HF, humidity factor; L, Lingot; MPN, the most probable number; MU, Morada de Usánsolo; N, Negrita; NB, Negra de Basaburua; OM, organic matter content; PA, Pinta Alavesa; RL, Riñón de León; OV, Verde de Orbiso.
References
Alberton, O., Kaschuk, G., Hungria, M. (2006). Sampling effects on the assessment of genetic diversity of rhizobia associated with soybean and common bean. Soil Biol. Biochem. 38, 1298–1307. doi: 10.1016/j.soilbio.2005.08.018
Araújo, S. S., Beebe, S., Crespi, M., Delbreil, B., González, E. M., Gruber, V., et al. (2015). Abiotic stress responses in legumes: Strategies used to cope with environmental challenges. Crit. Rev. Plant Sci. 34, 237–280. doi: 10.1080/07352689.2014.898450
Armenta-Borjóquez, A. D., Robledo-Ramirez, H. R., Camacho-Báez, J. R., Mundo-Ocampo, M., Gaarcía.Gutierrez, C., Armenta-Medina, A. (2016). Organic versus synthetic fertilization of beans (Phaseolus vulgaris L.) in Mexico. Exp. Agric. 52, 154–162. doi: 10.1017/S0014479715000010
Aserse, A. A., Markos, D., Getachew, G., Yli-Halla, M., Lindström, K. (2020). Rhizobial inoculation improves drought tolerance, biomass and grain yields of common bean (Phaseolus vulgaris L.) and soybean (Glycine max L.) at Halaba and Boricha in southern Ethiopia. Arch. Agron. Soil Sci. 66, 488–501. doi: 10.1080/03650340.2019.1624724
Aulakh, A. M., Qadir, G., Hassan, F. U., Hayat, R., Sultan, T., Billah, M., et al. (2020). Desert soil microbes as a mineral nutrient acquisition tool for chickpea (Cicer arietinum L.) productivity at different moisture regimes. Plants (Basel) 9, 1629. doi: 10.3390/plants9121629
Beebe, S. E., Rao, I. M., Blair, M. W., Acosta-Gallegos, J. A. (2013). Phenotyping common beans for adaptation to drought. Front. Physiol. 4. doi: 10.3389/fphys.2013.00035
Benjelloun, I., Thami Alami, I., Douira, A., Udupa, S. M. (2019). Phenotypic and genotypic diversity among symbiotic and non-symbiotic bacteria present in chickpea nodules in Morocco. Front. Microbiol. 10. doi: 10.3389/fmicb.2019.01885
Beringer, J. E. (1974). R factor transfer in Rhizobium leguminosarum. J. Gen. Microbiol. 84, 188–198. doi: 10.1099/00221287-84-1-188
Berny-Mier, J. C., Konzen, E. R., Medina, V., Palkovic, A., Ariani, A., Tsai, S. M., et al. (2019). Root and shoot variation in relation to potential intermittent drought adaptation of Mesoamerican wild common bean (Phaseolus vulgaris L.). Ann. Bot. 124, 917–932. doi: 10.1093/aob/mcy221
Borges, W. L., Prin, Y., Ducousso, M., Le Roux, C., de Faria, S. M. (2016). Rhizobial characterization in revegetated areas after bauxite mining. Braz. J. Microbiol. 47, 314–321. doi: 10.1016/j.bjm.2016.01.009
Chibeba, A. M., Pereira, C. S., Antunes, J. E. L., Ribeiro, R. A., Angela, C., Gomes, R. L. F., et al. (2020). Polyphasic characterization of nitrogen-fixing and co-resident bacteria in nodules of Phaseolus lunatus inoculated with soils from Piauí State, Northeast Brazil. Symbiosis 80, 279–292. doi: 10.1007/s13199-020-00672-1
Costa, R. M., Amaral, A., Chibeba, M., Fábio, A., Mercante, M. (2018). Polyphasic characterization of rhizobia microsymbionts of common bean (Phaseolus vulgaris L.) Isolated in Mato Grosso do Sul, a hotspot of Brazilian biodiversity. Symbiosis 16, 163–176. doi: 10.1007/s13199-018-0543-6
Cytryn, E. J., Sangurdekar, D. P., Streeter, J. G., Franck, W. L., Chang, W., Stacey, G., et al. (2007). Transcriptional and physiological responses of Bradyrhizobium japonicum to desiccation-induced stress. J. Bacteriol. 189, 6751–6762. doi: 10.1128/JB.00533-07
Darine, T., Alaeddine, C., Fethi, B., Ridha, M. (2015). Fluazifop-P-butyl (herbicide) affects richness and structure of soil bacterial communities. Soil Biol. Biochem. 81, 89–97. doi: 10.1016/j.soilbio.2014.10.030
da Silva, H. A. P., Caetano, V. S., Pessôa, D. D. V., Pacheco, R. S., Meneses, C. H. S. G., Simões-Araújo, J. L. (2024). Unraveling the drought-responsive transcriptomes in nodules of two common bean genotypes during biological nitrogen fixation. Front. Plant Sci. 15. doi: 10.3389/fpls.2024.1345379
Da-Silveira-Cardillo, B. E., Pádua-Oliveira, D., Lima-Soares, B., Dias-Martins, F. A., Rufini, M., da Silva, J. S., et al. (2019). Nodulation and yields of common bean are not affected either by fungicides or by the method of inoculation. Agron. J. 111, 694–701. doi: 10.2134/agronj2018.06.0389
del-Canto, A., Sanz-Saez, A., Sillero-Martínez, A., Mintegi, E., Lacuesta, M. (2023). Selected indigenous drought tolerant rhizobium strains as promising biostimulants for common bean in Northern Spain. Front. Plant Sci. 14. doi: 10.3389/fpls.2023.1046397
De Souza, E. M., Bassani, V. L., Sperotto, R. A., Granada, C. E. (2016). Inoculation of new rhizobial isolates improve nutrient uptake and growth of bean (Phaseolus vulgaris) and arugula (Eruca sativa). J. Sci. Food Agric. 96, 3446–3453. doi: 10.1002/jsfa.7527
Efron, B., Tibshirani, R. (1993). An introduction to the bootstrap. 1. publ. ed (New York: Chapman & Hall), 436. doi: 10.1007/978-1-4899-4541-9
Embrapa. (2018). “Conhecendo a fenologia do feijoeiro e seus aspectos fitotécnicos,” in Embrapa Arroz e Feijão-Livro técnico (INFOTECA-E) (Brasília D.F). Available at: https://ainfo.cnptia.embrapa.br/digital/bitstream/item/173690/1/CNPAF-2018-lvfeijoeiro.pdf.
Entz, M. H., Guilford, R., Gulden, R. (2001). Crop yield and soil nutrient status on 14 organic farms in the eastern portion of the northern Great Plains. Can. J. Plant Sci. 81, 351–354. doi: 10.4141/P00-089
Euskalmet. (2023). Agencia Vasca de Meteorología. Datos de estaciones. Available at: https://www.euskalmet.euskadi.eus/observacion/datos-de-estaciones/.
Fahraeus, G. (1957). The infection of clover root hairs by nodule bacteria studied by a simple glass slide technique. J. Gen. Microbiol. 16, 374–381. doi: 10.1099/00221287-16-2-374
FAO. (2013). “Climate-smart agriculture,” in Sourcebook (Rome Italy: Food and agriculture organization of the united nations). Available at: https://www.cac.int/sites/default/files/FAO.%202013.%20CSA%20Sourcebook.pdf.
FAO. (2021). Databases & software: crop information, bean. Available at: http://www.fao.org/land-water/databases-and-software/crop-information/bean/en/.
Ferguson, B., Mens, C., Hastwell, A., Zhang, M., Su, H., Jones, C., et al. (2019). Legume nodulation: The host controls the party. Plant Cell Environ. 42, 41–51. doi: 10.1111/pce.13348
Fernández, F., Gepts, F., López, M. (1986). Etapas de desarrollo de la planta de frijol común (Phaseolus vulgaris L.) (Colombia: CIAT, centro internacional de agricultura tropical), ISBN: ISBN 84-89206-54-6. 34p.
Flores-Félix, J. D., Sánchez-Juanes, F., García-Fraile, P., Valverde, A., Mateos, P. F., Gónzalez-Buitrago, J. M., et al. (2019). Phaseolus vulgaris is nodulated by the symbiovar viciae of several genospecies of Rhizobium laguerreae complex in a spanish region where Lens culinaris is the traditionally cultivated legume. System. Appl. Microbiol. 42, 240–247. doi: 10.1016/j.syapm.2018.10.009
Fox, J. E., Starcevic, M., Kow, K. Y., Burrow, M. Y., Mcalachlan, J. A. (2001). Nitrogen fixation endocrine disrupters and flavonoid signalling. Nat. (London) 413, 128–129. doi: 10.1038/35093163
García-Garijo, A., Tejera, N. A., Lluch, C., Palma, F. (2014). Metabolic responses in root nodules of Phaseolus vulgaris and Vicia sativa exposed to the imazamox herbicide. Pesticide Biochem. Physiol. 111, 19–23. doi: 10.1016/j.pestbp.2014.04.005
Gerosa-Ramos, M., Parsons, R., Sprent, J. I., James, E. K. (2003). Effect of water stress on nitrogen fixtion and nodule structure of common bean. Pesquisa. Agropecuaria. Brasileira 38, 339–347. doi: 10.1590/S0100-204X2003000300002
Gordon, B. R., Klinger, C. R., Weese, D. J., Lau, J. A., Burke, P. V., Dentinger, B. T. M., et al. (2016). Decoupled genomic elements and the evolution of partner quality in nitrogen-fixing rhizobia. Ecol. Evol. 6, 1317–1327. doi: 10.1002/ece3.1953
Goyal, R. K., Mattoo, A. K., Schmidt, M. A. (2021). Rhizobial–host interactions and symbiotic nitrogen fixation in legume crops toward agriculture sustainability. Front. Media SA. 12. doi: 10.3389/fmicb.2021.669404
Grange, L., Hungria, M. (2004). Genetic diversity of indigenous common bean (Phaseolus vulgaris) rhizobia in two Brazilian ecosystems. Soil Biol. Biochem. 36, 1389–1398. doi: 10.1016/j.soilbio.2004.03.005
Guanzon, I. M., Mason, M. L. T., Juico, P. P., Fiegalan, F. T. (2023). Isolation of three genera of microorganisms in lahar-laden soils of Sta. Rita, Pampanga, Philippines through the 16s rRNA gene sequence analysis, Acta Agriculturae Scandinavica, Section B. Soil Plant Sci. 73, 1, 1–1,12. doi: 10.1080/09064710.2022.2163281
Heath, K. D., Stock, A. J., Stinchcombe, J. R. (2010). Mutualism variation in the nodulation response to nitrate. J. Evol. Biol. 23, 2494–2500. doi: 10.1111/j.1420-9101.2010.02092.x
Heath, K. D., Tiffin, P. (2007). Context dependence in the coevolution of plant and rhizobial mutualists. Proc. R. Soc. B: Biol. Sci. 274, 1905–1912. doi: 10.1098/rspb.2007.0495
Heath, K. D., Tiffin, P. (2009). Stabilizing mechanisms in a legume-rhizobium mutualism. Evolution 63, 652–662. doi: 10.1111/evo.2009.63.issue-3
Heras, J., Domínguez, C., Mata, E., Pascual, V., Lozano, C., Torres, C., et al. (2015). GelJ–a tool for analyzing DNA fingerprint gel images. BMC Bioinf. 16, 270. doi: 10.1186/s12859-015-0703-0
Herencia, J. F., Pérez-Romero, L. F., Daza, A., Arroyo, F. T. (2020). Chemical and biological indicators of soil quality in organic and conventional Japanese plum orchards. Biol. Agric. Horticult. 1-20, 71–90. doi: 10.1080/01448765.2020.1842243
Hossain, M. J., Bakhsh, A. (2020). “Development and applications of transplastomic plants; A way towards eco-friendly agriculture,” in Environment, climate, plant and vegetation growth (Springer International Publishing, Cham), 285–322.
Howieson, J. G., Dilworth, M. J. (Eds.) (2016). Working with rhizobia (Canberra: Australian Centre for International Agricultural Research), ISBN: ISBN 978 1 925436 18 1.
Hungria, M., Andrade, D., de O Chueire, L. M., Probanza, A., Guttierrez-Mañero, F. J., Megías, M. (2000). Isolation and characterization of new effcient and competitive bean (Phaseolus vulgaris L.) rhizobia from Brazil. Soil Biol. Biochem. 32, 1515–1528. doi: 10.1016/S0038-0717(00)00063-8
Hungría, M., Araujo, R. S. (1994). Manual de métodos empregados em estudos de microbiologia agrícola (Barasília, DF: EMBRAPA Serviço de Produçao de Informaçao).
Igiehon, N. O., Babalola, O. O., Cheseto, X., Torto, B. (2021). Effects of rhizobia and arbuscular mycorrhizal fungi on yield, size distribution and fatty acid of soybean seeds grown under drought stress. Microbiol. Res. 242, 126640. doi: 10.1016/j.micres.2020.126640
IPCC (2021). “Summary for policymarkers,” in Masson-Delmotte, V., Zhai, P., Pirani, A., Connors, S. L., Péan, C., Berger, S., et al editors. Climate change 2021: The physical science basis contribution of wroking group I to the sixth assessment report of the intergovernmental panel on climate change. Cambridge University Press, Cambridge, United Kingdom and New York, NY, USA, pp. 3–32. doi: 10.1017/9781009157896.001
Jain, A. K., Moreau, J. V. (1987). Bootstrap technique in cluster analysis. Pattern Recogn. 20, 547–568. doi: 10.1016/0031-3203(87)90081-1
Judd, A., Schneider, M., Sadowsky, M. J., de Bruijn, F. (1993). Use of repetitive sequences and the polymerase chain reaction technique to classify genetically related Bradyrhizobium japonicum serocluster 123 strains. Appl. Environ. Microbiol. 59, 1702–1708. doi: 10.1128/aem.59.6.1702-1708.1993
Kaschuk, G., Hungria, M., Andrade, D. S., Campo, R. J. (2006). Genetic diversity of rhizobia associated with common bean (Phaseolus vulgaris L.) grown under no-tillage and conventional systems in southern Brazil. Appl. Soil Ecol. 32, 210–220. doi: 10.1016/j.apsoil.2005.06.008
Kerr, M. K., Churchill, G. A. (2001). Bootstrapping cluster analysis: Assessing the reliability of conclusions from microarray experiments. Proc. Natl. Acad. Sci. - PNAS 98, 8961–8965. doi: 10.1073/pnas.161273698
Kibido, T., Kunert, K., Makgopa, M., Greve, M., Vorster, J. (2019). Improvement of rhizobium-soybean symbiosis and nitrogen fixation under drought. Food Energy Secur. 9, 177. doi: 10.1002/fes3.177
Kremen, C., Miles, A. (2012). Ecosystem services in biologically diversified versus conventional farming systems. Ecol. Soc. 17, 40. doi: 10.5751/ES-05035-170440
Leunic, I., Bork, P. (2019). Interactive tree of life (iTOL) v4: Recent updates and new developments. Nucleic Acids Res. 47 (W1), W256–W259. doi: 10.1093/nar/gkz239
Li, X., Chu, Y., Jia, Y., Yue, H., Han, Z., Wang, Y. (2022). Changes to bacterial communities and soil metabolites in an apple orchard as a legacy effect of different intercropping plants and soil management practices. Front. Microbiol. 13. doi: 10.3389/fmicb.2022.956840
Lindström, K., Mousavi, S. A. (2020). Effectiveness of nitrogen fixation in rhizobia. Microbial. Biotechnol. 13, 1314–1335. doi: 10.1111/1751-7915.13517
Liyanage, D. K., Torkamaneh, D., Belzile, F., Balasubramanian, P., Hill, B., Thilakarathna, M. S. (2023). The Genotypic Variability among Short-Season Soybean Cultivars for Nitrogen Fixation under Drought Stress. Plants 12, 1004. doi: 10.3390/plants12051004
Loreau, M., Naeem, S., Inchausti, P., Bengtsoom, J., Grime, J. P., Tilman, D., et al. (2001). Biodiversity and functioning: current knowledge and future challenges. Science 294, 804–808. doi: 10.1126/science.1064088
MAPA. (1994). “Métodos oficiales de análisis,” in Tomo III: Métodos oficiales de análisis de suelos y aguas para el riego (Ministerio de Agricultura, Pesca y Alimentación. Servicio de Publicaciones, Madrid), 205–285.
MAPA. (2023). Ministerio de Agricultura, Pesca y Alimentación (Madrid, Spain: Agriculture. Legislation). Available at: https://www.mapa.gob.es/en/agricultura/legislacion/.
Margalef, D. R. (1958). Information theory in ecology. Memorias la Real Academica Cienc. y artes Barcelona 32, 374–559.
Martins, J. T., Buzo, F. de S., Garé, L. M., Garcia, N. F. S., Sales, L.Z., Do Nascimento, M. V. L., et al. (2024). Diazotrophic bacteria increase yield and profitability in organic cultivation of common bean. Rev. Bras. Engenharia Agricola e Ambiental 28. doi: 10.1590/1807-1929/agriambi.v28n2e272029
Masson-Boivin, C., Sachs, J. L. (2018). Symbiotic nitrogen fixation by rhizobia — the roots of a success story. Curr. Opin. Plant Biol. 44, 7–15). doi: 10.1016/j.pbi.2017.12.001
Mhadhbi, H., Chihaoui, S., Mhamdi, R., Bacem, M., Jebara, M., Mhandi, R. (2011). A highly osmotolerant rhizobial strain confers a better tolerance of nitrogen fixation and enhances protective activities to nodules of Phaseolus vulgaris under drought stress. Afr. J. Biotechnol. 10, 4555–4563. doi: 10.5897/AJB10.1991
Michiels, J., Dombrecht, B., Vermeiren, N., Xi, C., Luyten, E., Vanderleyden, J. (1998). Phaseolus vulgaris is a non-selective host for nodulation. FEMS Microbiol. Ecol. 26 (3), 193–205. doi: 10.1111/fem.1998.26.issue-3
Moreira, F. M. S., Siqueira, J. O., Brussaard, L. (2006). Soil biodiversity in Amazonian and other Brazilian ecosystems (Cambridge, England: Cabi), ISBN: ISBN-10: 1-84593-032-0. doi: 10.1079/9781845930325.0001
Mostasso, L., Mostasso, F. L., Dias, B. G., Vargas, M. A. T., Hungria, M. (2002). Selection of bean (Phaseolus vulgaris L.) rhizobial strains for the Brazilian Cerrados. Field Crops Res. 73, 121–132. doi: 10.1016/S0378-4290(01)00186-1
Mwenda, G. M., Hill, Y. J., O’hara, G. W., Reeve, W. G., Howieson, J. G., Terpolilli, J. J. (2023). Competition in the Phaseolus vulgaris-Rhizobium symbiosis and the role of resident soil rhizobia in determining the outcomes of inoculation. Plant Soil 487, 61–77. doi: 10.1007/s11104-023-05903-0
Nawaz, H., Hussain, N., Ahmed, N., Haseeb-ur-Rehman, Alam, J. (2021). Efficiency of seed bio-priming technique for healthy mungbean productivity under terminal drought stress. J. Integr. Agric. 20, 87–99. doi: 10.1016/S2095-3119(20)63184-7
Odori, C., Ngaira, J., Kinyua, J., Nyaboga, E. N. (2020). Morphological, genetic diversity and symbiotic functioning of rhizobia isolates nodulating cowpea (Vigna unguiculata L.) in soils of western Kenya and their tolerance to abiotic stress. Cogent Food & Agriculture 6 (1). doi: 10.1080/23311932.2020.1853009
Olive, D. M., Bean, P. (1999). Principles and applications of methods for DNA-based typing of microbial organisms. J. Clin. Microbiol. 37, 1661–1669. doi: 10.1128/JCM.37.6.1661-1669.1999
Omari, R. A., Yuan, K., Anh, K. T., Reckling, M., Halwani, M., Egamberdieva, D., et al. (2022). Enhanced soybean productivity by inoculation with indigenous bradyrhizobium strains in agroecological conditions of northeast Germany. Front. Media SA. 12. doi: 10.3389/fpls.2021.707080
Oviya, G., Rangasamy, A., Ariyan, M., Krishnamoorthy, R. R., Senthilkumar, M. M., Gopal, N. O., et al. (2023). Halotolerant nodule rhizobial and passenger endophytes alleviates salinity stress in groundnut (Arachis hypogaea L.). J. Plant Growth Regul. 42, 6620–6635. doi: 10.1007/s00344-023-10919-y
Pastor-Bueis, R., Sánchez-Cañizares, C., James, E. K., González-Andrés, F. (2019). Formulation of a highly effective inoculant for common bean based on an autochthonous elite strain of Rhizobium leguminosarum bv. phaseoli, and genomic-based insights into its agronomic performance. Front. Microbiol. 10. doi: 10.3389/fmicb.2019.02724
Pielou, E. C. (1977). Mathematical ecology. 2nd ed (New York: Wiley), ISBN: ISBN: 0 677 03580 2. 385p.
Pimentel, D., Hepperly, P., Hanson, J., Douds, D., Seidel, R. (2005). Environmental, energetic, and economic comparisons of organic and conventional farming systems. BioScience 55, 573–582. doi: 10.1641/0006-3568(2005)055[0573:EEAECO]2.0.CO;2
Posner, J. L., Baldock, J. O., Hedtcke, J. L. (2008). Organic and conventional production systems in the Wisconsin integrated cropping systems trials: I. productivity 1990-2002. Agron. J. 100, 253–260. doi: 10.2134/agrojnl2007.0058
Prasuna, M. L. (2014). Biological studies on the effect of agrochemicals on nodulation of some cultivated legumes. J. Ind. pollut. Control 30, 317–319.
Prudent, M., Dequiedt, S., Sorin, C., Girodet, S., Nowak, V., Duc, G., et al. (2020). The diversity of soil microbial communities matters when legumes face drought. Plant Cell Environ. 43, 1023–1035. doi: 10.1111/pce.13712
Rao, D., Adhya, T. K., Saxena, A. K. (2019). Agricultural microbiology research progress in India in the new millennium. Proc. Indian Natl. Sci. Academy Part A: Phys. Sci. 85, 925–947. doi: 10.16943/ptinsa/2019/49718
Regus, J. U., Wendlandt, C. E., Bantay, R. M., Gano-Cohen, K. A., Gleason, N. J., Hollowell, A. C., et al. (2016). Nitrogen deposition decreases the benefits of symbiosis in a native legume. Plant Soil 414, 159–170. doi: 10.1007/s11104-016-3114-8
Rípodas, C., Castaingts, M., Clúa, J., Villafañe, J., Blanco, F. A., Zanetti, M. E. (2019). The PvNF-YA1 and PvNF-YB7 subunits of the heterotrimeric NF-Y transcription factor influence strain preference in the Phaseolus vulgaris-Rhizobium etli symbiosis. Front. Plant Sci. 10. doi: 10.3389/fpls.2019.00221
Rodiño, A. P., Riveiro, M., De Ron, A. M. (2021). Implications of the symbiotic nitrogen fixation in common bean under seasonal water stress. Agronomy 11 (1), 70. doi: 10.3390/agronomy11010070
Santillana Villanueva, N. (2021). Mecanismos de inducción de rizobios para reducir el estrés por sequía en las leguminosas (Ayacucho, Perú: Universidad Nacional Del Altiplano Puno Peru). doi: 10.18271/ria.2021.263
Sanz-Sáez, A., Heath, K. D., Burke, P. V., Ainsworth, E. A. (2015). Inoculation with an enhanced N2-fixing Bradyrhizobium japonicum strain response to elevated CO2. Plant Cell Environ. 38, 2589. doi: 10.1111/pce.12577
Sanz-Sáez, A., Maw, M. J. W., Polania, J. A., Rao, I. M., Beebe, S. E., Fritschi, F. B. (2019). Using carbon isotope discrimination to assess genotypic differences in drought resistance of parental lines of common bean. Crop Sci. 59, 2153–2166. doi: 10.2135/cropsci2019.02.0085
Shamseldin, A., Velázquez, E. (2020). The promiscuity of Phaseolus vulgaris L. (common bean) for nodulation with rhizobia: A review. World J. Microbiol. Biotechnol. 36, 36–63. doi: 10.1007/s11274-020-02839-w
Shannon, C. E., Weaver, W. (1949). The mathematical theory of communication (Urbana: University Illinois Press). 117p.
Sharaf, H., Rodrigues, R. R., Moon, J., Zhang, B., Mills, K., Williams, M. A. (2019). Unprecedented bacterial community richness in soybean nodules vary with cultivar and water status. Microbiome 7, 1–18. doi: 10.1186/s40168-019-0676-8
Silva Neto, M. L., Smiderle, O. J., Silva, K., Fernandes Junior, P. I., Xabier, G. R., Zilli, J. E. (2013). Compatibility of the cowpea seed Treatment with fungicides and inoculation with fungicides and inoculation with Bradyrhizobium strains. Pesq. Agropec. Bras. 24, 56–68. doi: 10.1590/S0100-06832008000200040
Simon, Z., Mtei, K., Gessesse, A., Ndakidemi, P. A. (2014). Isolation and characterization of nitrogen fixing rhizobia from cultivated and uncultivated soils of northern Tanzania. Am. J. Plant Sci. 5, 4050–4067. doi: 10.4236/ajps.2014.526423
Sindhu, S., Dahiya, A., Gera, R., Sindhu, S. S. (2020). Mitigation of abiotic stress in legume-nodulating rhizobia for sustainable crop production. Agric. Res. (India: Online) 9, 444–459. doi: 10.1007/s40003-020-00474-3
Sokal, R. R. (1973). “Numerical taxonomy,” in A statistical method for evaluating systematic relationships, vol. 38 . Ed. Felsein, J. (San Francisco: Springer-Verlag), 1409–1438.
Somasegaran, P., Hoben, H. J. (1994). Hand Book for Rhizobia: Methods in legume rhizobium technology (New York: Springer-Verlag). doi: 10.1007/978-1-4613-8375-8
Song, X., Yuan, Z. Q., Fang, C., Hu, Z. H., Li, F. M., Sardans, J., et al. (2024). The formation of humic acid and micro-aggregates facilitated long-time soil organic carbon sequestration after Medicago sativa L. introduction on abandoned farmlands. Geoderma 445, 116889. doi: 10.1016/j.geoderma.2024.116889
Steiner, F., da Silva Oliveira, C. E., Zoz, T., Zuffo, A. M., de Freitas, R. S. (2020). Co-inoculation of common bean with Rhizobium and Azospirillum enhance the drought tolerance. Russian J. Plant Physiol. 67, 923–932. doi: 10.1134/S1021443720050167
Szparaga, A., Maciej Kubo, M., Kocira, S., Czerwinska, E., Pawlowska, A., Hara, P. (2019). Towards a sustainable agriculture, agronomic and economic effects of biostimulants use in common bean cultivation. Sustainability 11, 4575. doi: 10.3390/su11174575
Versalovic, J., Koeuth, T., Lupski, J. R. (1991). Distribution of repetitive DNA sequences in eubacteria and application to fingerprinting of bacterial genomes. Nucleic Acids Res. 19, 6823–6831. doi: 10.1093/nar/19.24.6823
Viti, C., Bellabarba, A., Daghio, M., Mengoni, A., Mele, M., Buccioni, A., et al. (2021). Alfalfa for a sustainable ovine farming system: proposed research for a new feeding strategy based on alfalfa and ecological leftovers in drought conditions. MDPI AG 13 (7), 3880. doi: 10.3390/su13073880
Wang, E. T., Young, J. P. W. (2019). History of rhizobial taxonomy. In. Ecol. Evol. rhizobia, 23–39. doi: 10.1007/978-981-32-9555-1
Weese, D. J., Heath, K. D., Dentinger, B. T. M., Lau, J. A. (2015). Long-term nitrogen addition causes the evolution of less-cooperative mutualists. Evolution 69, 631–642. doi: 10.1111/evo.2015.69.issue-3
Wolff, H., Killebrew, K. (2010). Environmental impacts of agricultural technologies (No. UWEC-2011-01). (University of Washington, Department of Economics: Working Papers).
Yan, J., Yan, H., Liu, L., Chen, W.--., Zhang, X. X., Verástegui-Valdés, M., et al. (2017). Rhizobium hidalgonense sp. nov., a nodule endophytic bacterium of Phaseolus vulgaris in acid soil. Arch. Microbiol. 199, 97–104. doi: 10.1007/s00203-016-1281-x
Zurdo-Piñeiro, J. L., García-Fraile, P., Rivas, R., Peix, Á., León-Barrios, M., Willems, A., et al. (2009). Rhizobia from Lanzarote, the Canary Islands, that nodulate Phaseolus vulgaris have characteristics in common with Sinorhizobium meliloti isolates from mainland Spain. Appl. Environ. Microbiol. 75, 2354–2359. doi: 10.1128/AEM.02811-08
Keywords: genomic fingerprinting, nodulation, organic management, common bean, strain diversity, yield
Citation: del-Canto A, Sanz-Saez A, Heath KD, Grillo MA, Heras J and Lacuesta M (2024) Conventional management has a greater negative impact on Phaseolus vulgaris L. rhizobia diversity and abundance than water scarcity. Front. Plant Sci. 15:1408125. doi: 10.3389/fpls.2024.1408125
Received: 27 March 2024; Accepted: 22 May 2024;
Published: 01 July 2024.
Edited by:
Dinesh Yadav, Deen Dayal Upadhyay Gorakhpur University, IndiaReviewed by:
Abhishek Joshi, Mohanlal Sukhadia University, IndiaClarisse Brígido, University of Evora, Portugal
Copyright © 2024 del-Canto, Sanz-Saez, Heath, Grillo, Heras and Lacuesta. This is an open-access article distributed under the terms of the Creative Commons Attribution License (CC BY). The use, distribution or reproduction in other forums is permitted, provided the original author(s) and the copyright owner(s) are credited and that the original publication in this journal is cited, in accordance with accepted academic practice. No use, distribution or reproduction is permitted which does not comply with these terms.
*Correspondence: Arantza del-Canto, YXJhbnR6YS5kZWxjYW50b0BlaHUuZXVz; Maite Lacuesta, bWFpdGUubGFjdWVzdGFAZWh1LmV1cw==