- 1Facultad de Ciencias Biológicas, P. Universidad Católica de Chile, Santiago, Chile
- 2Departamento de Ecología, Facultad de Ciencias, Universidad de Chile, Santiago, Chile
- 3Instituto de Investigaciones Agropecuarias (INIA), La Cruz, Chile
The Andean uplift and the concomitant aridification drove the rapid diversification of several plant lineages that were able to colonize warmer and drier habitats at low elevations and wetter and colder habitats at high elevations. These transitions may be facilitated by shifts in plant strategies to cope with drought and cold, which in turn can trigger episodes of accelerated species diversification. Here, we used four nuclear DNA markers to infer phylogenetic relationships of 80 Adesmia species of annuals, perennial herbs, shrubs and small shrubs that occur in Chile and Argentina. We reconstructed ancestral states for area, climatic niche and growth form to explore how Andean uplift and aridification promoted Adesmia diversification. We also performed logistic and linear regression analyses between different components of growth form (life span, woodiness and plant height) and climate. Finally, we estimated speciation rates across the phylogeny. Our results suggest that the ancestor of Chilean Adesmia was a perennial herb that probably originated in the high Andes of northern and central Chile. The low elevations of Central Chile were colonized in the late Miocene, whereas the high latitudes of Patagonia and the hyperarid coastal Atacama Desert were colonized repeatedly since Pliocene by lineages with different growth forms. Multiple and bidirectional transitions between annual and perennial habits and between herbaceous and woody habits were detected. These shifts were not correlated with climate, suggesting that the different growth forms are alternative and successful strategies to survive unfavorable seasons of both desert and high Andes. Net diversification analysis indicated a constant rate of diversification, suggesting that the high species diversity of Adesmia that occur in Chile is due to a uniform speciation process rather than to accelerated episodes of speciation.
Introduction
The central and southern Andes have a high diversity of plant species (Pérez-Escobar et al., 2022). The history of this diverse flora is linked to the orogeny of the Andes and the development of the South American Arid Diagonal (Simpson, 1983; Antonelli et al., 2009; Luebert and Weigend, 2014), a strip of arid climate that extends along the Andes from Peru to Argentinian Patagonia (Villagrán and Hinojosa, 1997; Abraham et al., 2020). Aridification occurred gradually during the Oligocene and Miocene as a consequence of the strong rain shadow produced by the uplift of the Andes (Dunai et al., 2005). The establishment of a phase of global climate cooling since the middle Miocene (Zachos et al., 2001) and the reinforcement of the cold Humboldt Current in the Pliocene intensified aridification (Hinojosa and Villagrán, 1997; Hartley et al., 2005; Garreaud et al., 2010).
The South American Arid Diagonal forms a biogeographic corridor that has allowed the expansion and diversification of plant genera of different biogeographic origins (Simpson, 1983; Antonelli et al., 2009; Luebert and Weigend, 2014; Glade-Vargas et al., 2021). In the high Andes, plant lineages that have migrated from high latitudes tracking cold-humid climates coexist with lineages that have shifted repeatedly between warmer and drier habitats at low elevations and colder and wetter habitats at high elevations (Antonelli, 2015). Transitions between habitats may be accompanied by shifts in the strategy of plants that allow surviving periods of extreme cold or drought (Ogburn and Edwards, 2015; Pérez et al., 2020); or alternatively, might be favored by the preexistence of “enabler” traits that allow species to cope with both conditions (Harvey and Pagel, 1991; Edwards and Donoghue, 2013; Zanne et al., 2014).
Plants have evolved different strategies that permitted them to survive unfavorable seasons (Grime, 1977; Stearns, 2000). The annual life strategy is part of a fast development strategy that allows plants to complete the life cycle within the favorable season of a single year (Evans et al., 2005; Kooyers, 2015; Friedman and Rubin, 2015; Pérez et al., 2020). Annuals are favored in hot and dry seasonal climates (Friedman, 2020; Boyko et al., 2023), but tend to be excluded from high elevation habitats, where short growing seasons and repeated frosts inhibit seedling establishment and reduce the probability of completing a life cycle in one season (Bliss, 1971; Korner, 2003; Givnish, 2015). Perennial plants also have adaptations that allow survival of unfavorable seasons. Perennial herbs can shed aboveground tissues during unfavorable seasons, leaving belowground buds in rhizomes (woody underground stems) or specialized reserve organs (such as bulbs, tubers or corms) that enable resprouting (Raunkiaer, 1934; Bliss, 1971) and allow plants to survive in cold and dry conditions (Howard et al., 2019). Woody plants can shed leaves and protect buds with scale-like modified leaves or become smaller, leaving buds close to the ground (Bliss, 1971; Korner, 2003; Zanne et al., 2014). According to these strategies, plants can be classified in those that persist as seeds or underground storage organs and those that have perennial buds at, near or above the soil surface (Raunkiaer, 1934; Taylor et al., 2023).
Accelerated rates of diversification can be triggered by shifts in traits related with plant life history strategies, including life span (Givnish, 2010; Friedman, 2020), woodiness (Nürk et al., 2019), plant size (Boucher et al., 2017) and reproductive strategy (de Vos et al., 2014). Annual and herbaceous clades often have more species than their sister woody clades (Givnish, 2010), indicating that the shorter generation time of herbs can accelerate speciation (Smith and Donoghue, 2008). Likewise, small plants have higher diversification rates than do larger plants (Boucher et al., 2017). Shifts from annual to perennial life history strategy have been proposed as key innovations that trigger accelerated species diversification in high elevation habitats (Drummond et al., 2012; Hughes and Atchison, 2015; Nevado et al., 2016; but see Givnish, 2015). Evolution of secondary woodiness from herbaceous ancestors also appears to act as a key innovation driving plant radiations on island systems (Nürk et al., 2019).
The Andean uplift and aridification drove the rapid diversification of several plant lineages, including some of the most species-rich genera of Fabaceae (Kenicer et al., 2005; Hughes and Eastwood, 2006; Scherson et al., 2008). In southern South America, the richest genus of Fabaceae is Adesmia DC. (Dalbergieae, Faboideae), which comprises around 230 species of annuals, perennial herbs, small cushions and shrubs (Figure 1) grouped in 43 series and two subgenera: Acanthadesmia (that includes all spiny shrubs) and Adesmia (that includes all unarmed forms) (Burkart, 1967). Species grow mainly along the Arid Diagonal from northern Peru to Patagonia, with some species extending to Southern Brazil. Adesmia has two centers of diversification, one in Chile and the other in Argentina (Burkart, 1967). Both areas have been proposed as centers of origin, with the Andes acting as a vicariant barrier (Mihoc, 2012) or as a biogeographic corridor from south to north (Burkart, 1967). A phylogenetic study has been conducted for Adesmia sect. psoraleoides, a monophyletic series endemic to southern Brazil (Iganci et al., 2013). This study, which included some species from other regions, found a north to south pattern of diversification, suggesting an expansion from arid regions of Bolivia and Chile to Patagonia, followed by colonization of southern Brazil.
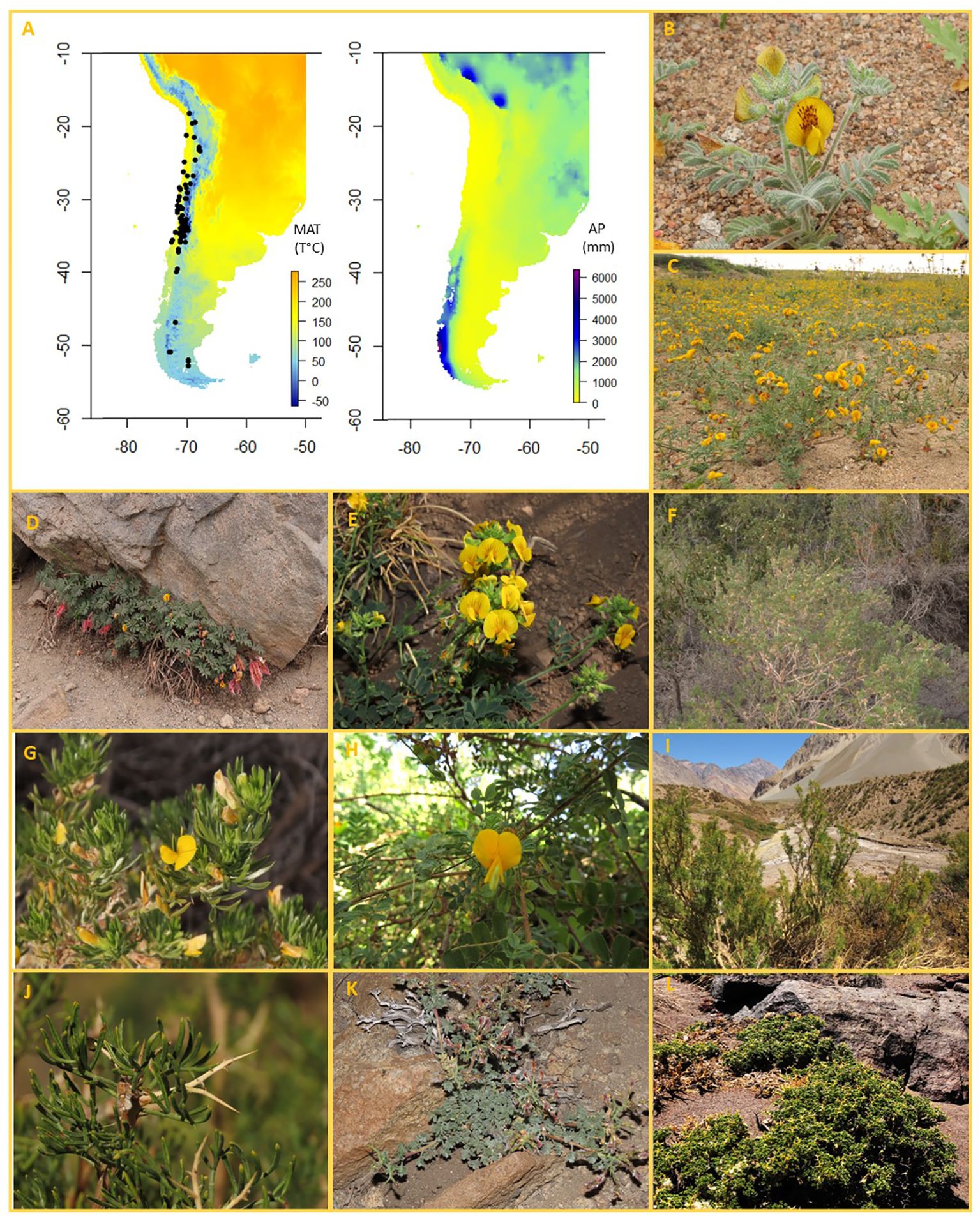
Figure 1. (A) Distribution of studied species of Adesmia in relation to mean annual precipitation (left) and mean annual temperature (right). (B-L) Images of 9 species of Adesmia showing the wide variety of growth forms found in the genus. (B, C) Annuals: (B) A. eremophila, (C) A tenella. (D, E) Perennial herbs: (D) A longipes, (E) A. coronilloides. (F-J) Shrubs (larger than 25 cm): (F, G) A. loudonia (unarmed shrub); (H). A. elegans (unarmed shrub); (I, J) A. pinifolia (spiny shrub). (K, L) Dwarf shrubs: (K) A. hirsuta; (L). A. hemisphaerica.
In this study, we established phylogenetic relationships using four nuclear DNA markers of 80 Adesmia species that occur in Chile and Argentina from latitude 18° to 52°S and from sea level to 4500 m elevation (Figure 2). We reconstructed ancestral states for area, climatic niche and growth form, to understand how the Andean uplift and the onset of arid and semiarid conditions promoted diversification of Adesmia. We also included the presence/absence of spines to explore whether the two subgenera (Acanthadesmia and Adesmia) are monophyletic. In addition, we examined whether shifts in traits related to plant life history strategy (life span, woodiness and plant height) evolved in relation to climate. Finally, we determined whether the high species diversity of Chilean Adesmia is due to uniform speciation, or to events of accelerated speciation triggered by shifts in growth form.
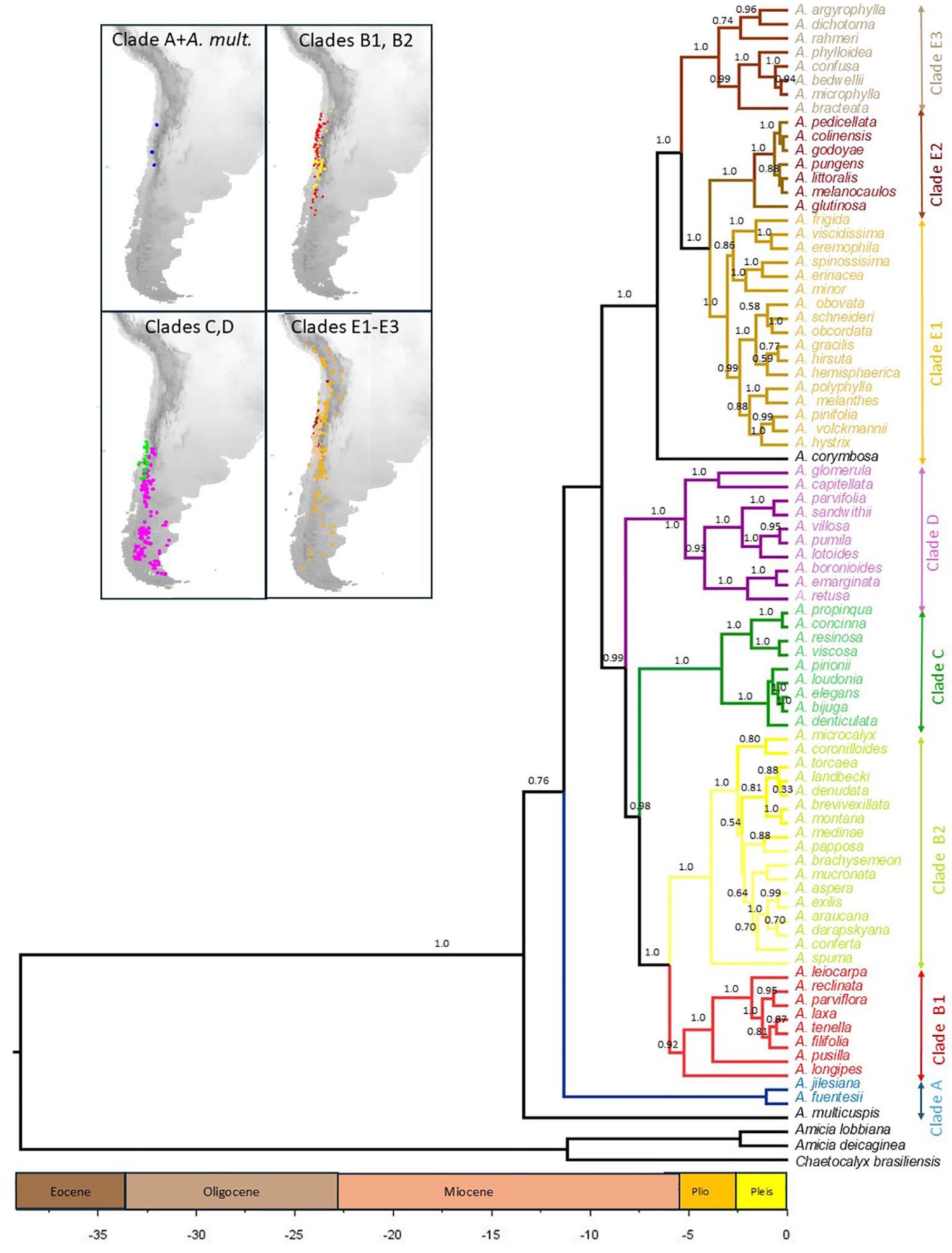
Figure 2. Time-calibrated phylogeny of 80 species of Adesmia that grow in Chile based on four nuclear DNA sequences obtained in BEAST. Three species of the related genera Amicia and Chaetocalyx were included as outgroups. Divergence time is given in million years before present. The posterior probabilities of nodes are shown above branches (only values higher than 0.5). Distribution of species of the main lineages (denoted with different colors) are shown in maps.
Materials and methods
Taxon sampling
We obtained DNA from 80 of the 132 species described for Chile (Rodríguez et al., 2018; Table 1). DNA was extracted from leaf material of individuals collected in the field and from herbarium specimens stored at CONC (Herbarium of the Department of Botany, University of Concepción) and SGO (Herbarium of the National Museum of Natural History). As outgroup species, we included Chaetocalyx brasiliensis (Vogel) Benth., Amicia medicaginea Griseb., and Amicia lobbiana Benth.
DNA extraction, amplification, and sequencing
Genomic DNA was extracted using the DNeasy Plant Kit (Qiagen, Valencia, CA, United States). PCR amplification was performed for the internal transcribed spacer region (ITS), the external transcribed spacer region (ETS), and the auxin-independent growth (AIGP) gene using previously published primers (Ariati et al., 2006; Choi et al., 2004; Table 2). Additionally, we searched for nuclear single-copy gene using the transcriptome sequences of two species of Adesmia obtained by Eshel et al. (2021). We designed primers for ten candidate regions and then we conducted PCR amplification in ten species to test for amplification consistency and specificity. We selected the two most polymorphic regions, one corresponding to U5 small nuclear ribonucleoprotein component (CLO) and the other to the “vacuolar-sorting receptor 1” gene (exons 2 to 7). Sanger sequencing was performed in the Plataforma de Secuenciación y Tecnologías Omicas, Pontificia Universidad Católica de Chile, using the ABI PRISM 3500 xl Genetic Analyzer (Applied Biosystems). All new sequences were deposited in GenBank (Supplementary Table 1). Sequences were aligned using the ClustalW algorithm in BioEdit 7.0 (Hall, 1999) and concatenated into a single dataset consisting of a total of 2949 positions. Alignments were manually inspected. Previously, we performed a homogeneity test in PAUP 4.0 (Swofford, 2003) to assess whether multi-copy ribosomal markers (ITS, ETS) and single-copy nuclear genes are congruent. We found that the two datasets are congruent (p=0.17). Given that this test can yield false negatives (Pirie, 2015), we also analyzed the ribosomal and single-copy nuclear genes separately. Then, the resulting phylogenetic trees were examined manually.
Phylogenetic analyses
Phylogeny was reconstructed using Bayesian Inference (BI) as implemented in MrBayes 3.1.2 (Ronquist et al., 2012) on the combined dataset. Analyses were conducted using four partitions corresponding to each DNA region and a GTR + γ model of evolution, with two independent runs for 10 million generations, sampling every 5,000 generations. We discarded the first 25% of generations as burn-in to construct a 50% majority-rule consensus tree and to obtain posterior probabilities of each node (PP). To estimate species divergence times, we conducted a Bayesian relaxed-clock analysis in BEAST (BE) program (version 1.4.8; Drummond and Rambaut, 2007). We used the age of the most recent common ancestor of Adesmia and Chaetocalyx (40.9 ± 4 Mya) as a calibration point according to Simon et al. (2009). MCMC chains were run for 50 million generations, sampling every 5,000 generations. The time-scaled maximum clade credibility (MCC) tree was then identified using TreeAnnotator. We discarded the first 25% of generations as burn-in to obtain posteriori probabilities of each node.
Climatic niche
We estimated the realized climatic niche of each species using the maximum entropy approach with MAXENT (Phillips et al., 2006), incorporating 19 bioclimatic and topographic variables from the World Climate database (Hijmans et al., 2005) at 30 arc seconds of resolution one km (30s) resolution (~1 Km at the equator). We compiled distribution information for each species from GBIF and newly collected field data. These occurrences were verified with each species distribution reported in Rodriguez et al. (2018). Occurrences outside the known distribution range were eliminated. Background points were randomly selected within the area enclosed by a minimum convex polygon comprising all species records. Occurrence data were partitioned 100 times into training and test datasets (80% and 20%, respectively) for model evaluation using the operating characteristic curve (AUC). We focused on mean annual temperature (MAT) and annual precipitation (AP) because these variables describe better the diversity of habitats occurring along the latitudinal and elevational gradient where Adesmia is distributed. Probability distributions derived from MAXENT were used to obtain predicted niche occupancy profiles of each species with respect to mean annual temperature (MAT) and mean annual precipitation (AP). We estimated the weighted mean of each climatic variable (w-MAT, w-AP). All analyses were conducted using the R-package phyloclim (Heibl, 2011). The ancestral states of w-AP and w-MAT were reconstructed using the MCC tree recovered from BEAST analysis and the maximum likelihood method of Schluter et al. (1997) under a Brownian model of evolution implemented in the R package phytools (Revell, 2012).
Evolutionary shifts in growth forms
Growth forms of Adesmia were classified as annuals (persist as seed), perennial herbs (shed aboveground tissues during unfavorable seasons, leaving resistant buds in rhizomes) and shrubs (Supplementary Table 2). The ancestral states of growth form were reconstructed using the Markov Chain Monte Carlo (MCMC) approach implemented in BayesTraits v4 (Meade and Pagel, 2023), which takes into account phylogenetic uncertainty (Pagel and Meade, 2006). These analyses were conducted with 1000 trees randomly sampled from the posterior of the BEAST analysis. We performed a likelihood ratio test (LR) to compare the goodness of fit of an equal rates model with an unequal rates model. We selected the first model because it did not lead to a significant reduction in likelihood (Log marginal likelihood: unequal rates model = -43.2; equal rates model = -44.8; LR=3.2; Chi5 = 3.6, p=0.39). We used this model to estimate the average probability of each possible growth form (annual herb, perennial herb, or shrub) for each node based on 1,000 sampled trees. Additionally, we analyzed the presence of spines, a key trait that has been used to separate the currently recognized subgenera Adesmia and Acanthadesmia.
We also examined whether shifts in different components of the growth form (life span, woodiness, and plant height) evolved in relation to climate, using two approaches. First, we reconstructed the ancestral states for w-MAT and w-AP using the lambda model that incorporates a scaling parameter (λ) to account for the effects of phylogenetic relationships on trait evolution. Previously, we compared the lambda model with a Brownian (BM), early burst (EB) an Ornstein-Uhlenbec (OU) model using the Akaike Information Criterion (AIC). We selected the first model for both climatic variables because it had the lowest AIC values (w-MAT: AIC lambda=175, AIC BM=186, AIC OU 186, AIC EB=187; w-AP: AIC lambda=1100; AIC BM=1127, AIC OU= 1129, AIC EB=1129). Second, for discrete traits, life span (annual/perennial) or woodiness (herb/shrub), we fitted a phylogenetic logistic regression model using w-MAT and w-AP as continuous predictor variables in the R package phylolm (Ho and Ane, 2014). y. For continuous traits (plant height), we conducted a phylogenetic linear regression using w-MAT and w-AP as predictor variables.
Ancestral area reconstruction
We reconstructed the ancestral distributions of Adesmia using several evolutionary process models implemented in the BioGeoBEARS R package (Matzke, 2014) and the consensus tree recovered from BEAST analysis. BioGeoBEARS implemented several anagenetic and cladogenetic process models, including the LaGrange Dispersal-Extinction Cladogenesis Model (DEC) (Ree and Smith, 2008), a likelihood version of DIVA (DIVALIKE), and BAYAREA (Yu et al., 2015). A jump parameter (j) can also be added to any of these models to account for speciation processes mediated by founder events (Matzke, 2014). Models were compared using the Akaike Information Criterion (AIC). We considered five areas: (D) Desert, corresponding to low to mid elevations of northern Chile (18 to 30°S); (N) Northern Chilean High Andes (up to 2000 m, 18 to 30°S); (M) Mediterranean region, corresponding to low to mid elevations of central and southern Chile (30 to 40°S); (C) Central Chilean High Andes: high elevations of central Chile (30 to 40°S) and (P) Patagonia, cold steppes at high-latitudes in Patagonia (southern 40°S). A maximum of two areas was allowed for reconstructions.
Diversification analyses
To estimate whether the high species diversity of Adesmia is due to uniform divergence or events of accelerated speciation, we estimated speciation rates across the Adesmia phylogeny using a time-dependent model implemented in BAMM v.2.5 with expected number of shifts set to one and sampling probability set to 0.64 (corresponding to the proportion of Chilean species sampled). Four Markov Chains Monte Carlo (MCMC) runs were performed with 50 million generations, sampling parameters every 5,000 generations. Diversification rates and rate shift configurations were plotted using the BAMMtools R-package (Rabosky et al., 2014).
Results
Molecular phylogeny
Similar topologies were obtained by BEAST (BE) and MrBayes (BI) analyses. In both approaches A. multicuspis, an annual herb that grows in northern Chile, was positioned as the sister to the rest of the genus. Five clades (named Clades A to E; see Figure 2) were revealed in both analyses. Clade A includes the perennial herbs A. fuentesi and A. jilesiana, which occur in northern and central Chile at high elevations (up to 4000 m). Clade B (PP: BI=1.0; BE=1.0) is formed by annual and perennial herbs, which are grouped into two subclades: B1 (BI=0.87; BE=0.92) and B2 (BI=1.0; BE=1.0). Except for A. longipes, all species of subclade B1 grow in warm and arid habitats at low and middle elevations of northern and central Chile, whereas species of subclade B2 primarily grow in middle and high elevations of central Chile under colder and wetter conditions (Figure 3). Clade B is positioned sister to Clade C (BI=0.96; BE=1.0), which is formed by unarmed shrubs restricted to low and middle elevations of central Chile. Group D (BI=1.0; BE=1.0is formed mostly by herbs that grow in cold steppes at high elevations or latitudes. Clade E (Support: BI=0.92; BE=1.0) includes the perennial herb A. corymbosa, which is positioned as sister to three lineages (E1-E3). Subclade E1 (BI=0.98; BE=1.0) is formed by spiny shrubs that grow mainly in cold habitats at high elevations or latitudes, except for two herbaceous species that grow in the coastal Atacama Desert (A. viscidissima and A. eremophila). Subclades E2 (BI=1.0; BE=1.0) and E3 (BI=1.0; BE=1.0) are formed principally by unarmed and spiny shrubs that occur in warm and arid habitats at low and middle elevations of northern and central Chile. Similar topologies were obtained when ribosomal and single-copy nuclear markers were analyzed separately (see Supporting Information), although some differences emerged that could result from their varying levels of resolution. Clades A to D were well supported by the ribosomal data (BI > 0.94), while the single-copy nuclear data provided weaker support. The combined dataset offered greater support and resolution for the main clades, except for clade C, which was not supported by the single-copy data. Additionally, the combined dataset provided greater support for clades E and its subclades E1, E2, and E3 than the separate datasets.
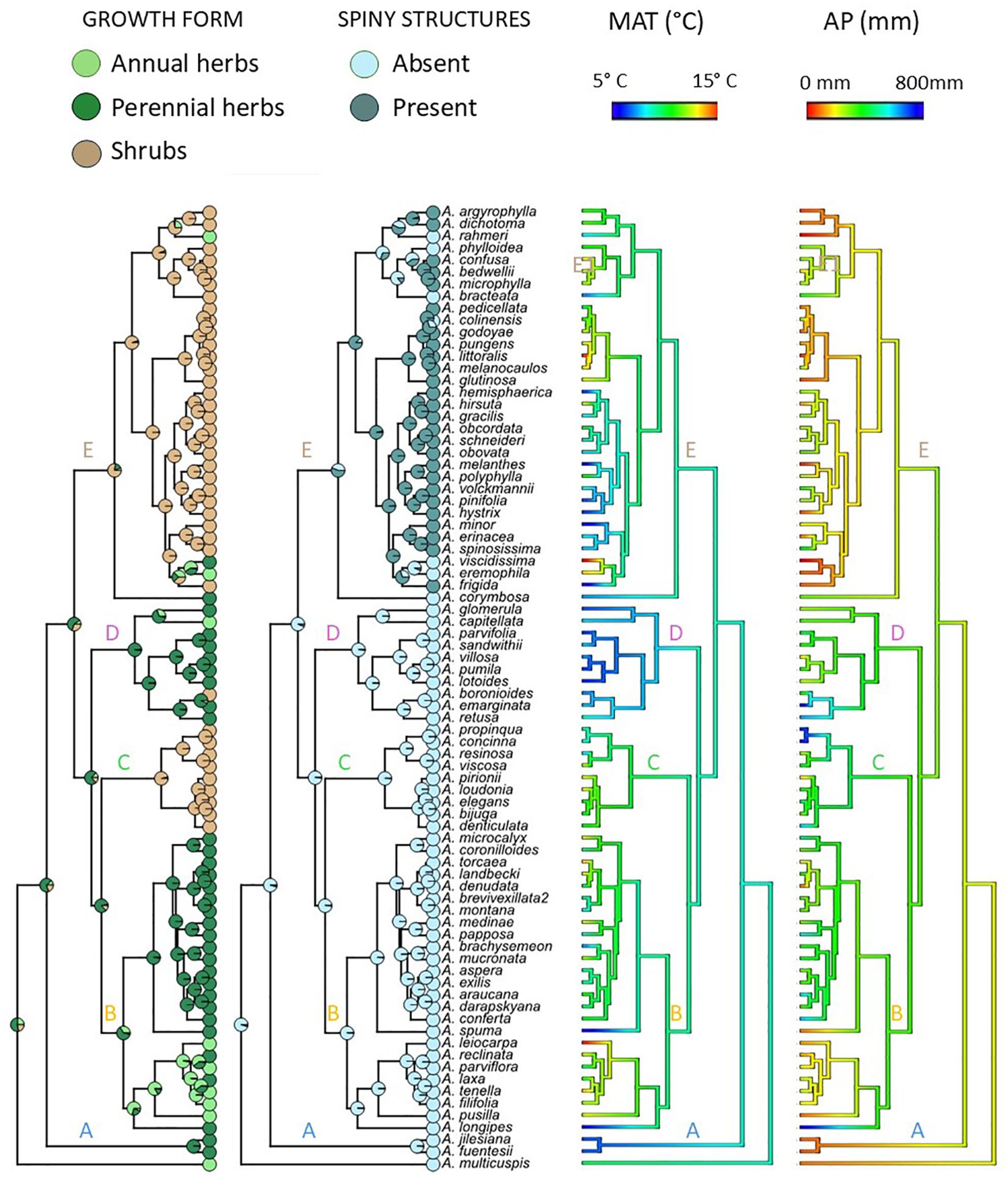
Figure 3. Reconstruction of ancestral states of growth forms, presence/absence of spiny structures and climatic niche. Ancestral reconstruction of growth form and presence/absence of spiny structures were performed using the Markov Chain Monte Carlo (MCMC) approach implemented in BayesTraits v4. Pie diagrams at nodes show the average probabilities of the possible states of growth form (annual, perennial herb and shrub) estimated from the 1,000 sampled traits to take into account phylogenetic uncertainty. Ancestral reconstruction of weighted mean of mean annual temperature (MAT) and mean annual precipitation (MAP) obtained from were performed PNO using a maximum likelihood method for continuous traits under a lambda model.
Evolution of growth form and climatic niche
Ancestral state reconstruction of growth form based on the 1,000 sampled trees suggests that the ancestor of Adesmia was a perennial herb (Figure 3). In 82% of them, the most likely growth form for the ancestor was a perennial herb. On average, there is a 53% chance that the ancestor of Adesmia was a perennial herb, a 26% chance that it was an annual herb, and a 21% chance that it was a shrub.
The annual habit originated at least five times independently, with one reversion. Only three of the five transitions to annuality matched with shifts to warmer and drier habitats (Figure 3), corresponding to species of subclade B1, A. eremophila of clade E1, and A. multicuspis (basal species). The other two transitions occurred at high elevations, involving the origin of A. rahmeri and A. capitellata. Shrub habit evolved independently three times: in A. boronioides, Clade C, and Clade E (Figure 3). One reversion from shrub habit to herbaceous habit was detected, which matched the colonization of Coastal Atacama Desert by A. viscidissima and A. eremophila. Ancestral reconstruction for spine presence/absence indicated that spiny structures were acquired by the ancestor of Clade E. These structures would have been lost at least once in each subclade (E1, E2, and E3) and reacquired two times in subclade E1. These results indicate that the subgenus Acanthadesmia is not monophyletic. Phylogenetic logistic regression analyses revealed a non-statistically significant association between life span and MAT (estimate= 0.013, p=0.23) or AP (estimate<0.001, p=0.39). Likewise, no association between woodiness and MAT (estimate = -0.009, p = 0.34) nor AP (estimate <0.001, p = 0.43) was detected. Plant height did not correlate with MAT (estimate=0.006, p=0.85) or AP (estimate=-0.004, p=0.56). Phylogenetic signal lambda values for longevity (Λ= 0.76), woodiness (Λ=1.0), plant height (Λ=0.80), wMAT (Λ=0.68) and wAP (Λ=0.65) were significantly higher than zero, indicating a moderate to strong phylogenetic signal.
Ancestral reconstruction of area
BEAST analyses indicated that the early cladogenetic events of Adesmia occurred approximately 10 Myr. in the Late Miocene. Ancestral range estimations under the best fit model (DEC + J; Table 3) suggested that the most probable ancestral area for extant Chilean species of Adesmia was the high Andes of northern and central Chile (Figure 4). Low and middle elevations of central Chile were colonized during the Late Miocene by the ancestor of the unarmed shrubs of Clade C, and later during the Pliocene and Pleistocene by several lineages of spiny shrubs and perennial herbs. The ancestor of Clade D colonized high latitudes of Patagonia during the Pliocene, followed by several lineages of spiny shrubs that extended their ranges during Pleistocene. The coastal Atacama Desert was colonized at least twice during the Pliocene, once by herbs of subclade B1 and again by spiny shrubs of subclade E2. Spiny shrubs of subclade E1 and herbs of subclade E3 colonized coastal Atacama Desert later during Pleistocene. The inclusion of the parameter “J” in the model DEC suggests that the colonization of new areas (Mediterranean region and Atacama Desert) involved multiple founder events from High Andes in northern and central Chile.
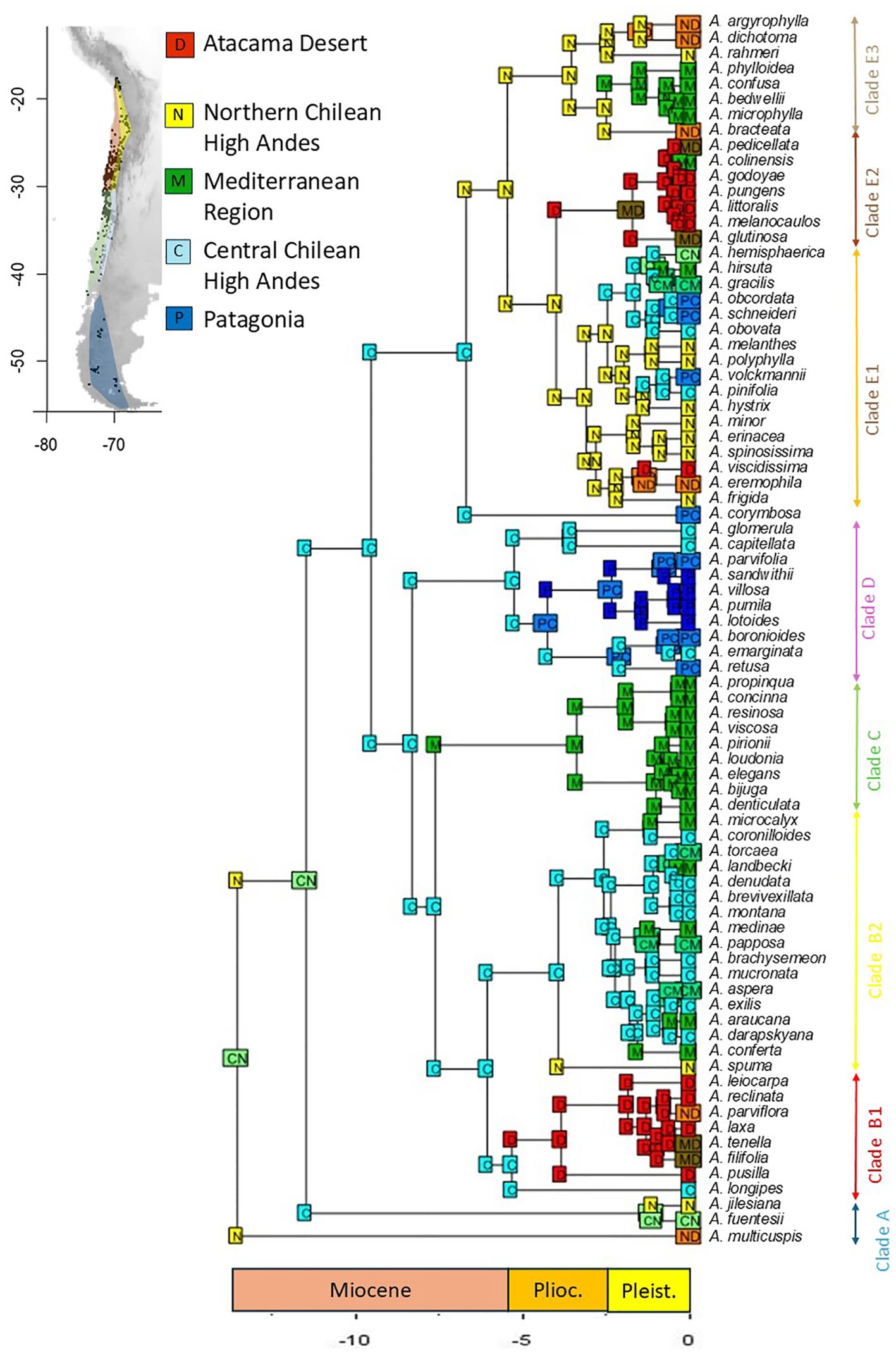
Figure 4. Reconstruction of ancestral distributions of Adesmia using the best model DEC+J (a maximum of three areas was allowed). Five areas were considered: (D) Desert, corresponding to low to mid elevations of northern Chile (18 to 30°S); (N) Northern Chilean High Andes (up to 2000 m, 18 to 30°S), (M) Mediterranean region, corresponding to low to mid elevations of central and southern Chile (30 to 40°S); (C) Central Chilean High Andes, corresponding to high elevations of central Chile (30 to 40°S); (P) Patagonia, cold steppes at high-latitudes in Patagonia (southern 40°S).
Diversification analyses
The rate of diversification tends to decrease slightly over time, from a mean net diversification rate of 0.57 lineages per Myr at the root to 0.42 at the tips. However, this tendency was not statistically significant, as revealed by overlapping 95% confidence intervals (Figure 5). BAMM detected no significant shifts in the rate of diversification across Adesmia, suggesting that the high species diversity of Adesmia that occur in Chile is due to a uniform speciation process rather than to accelerated events of speciation.
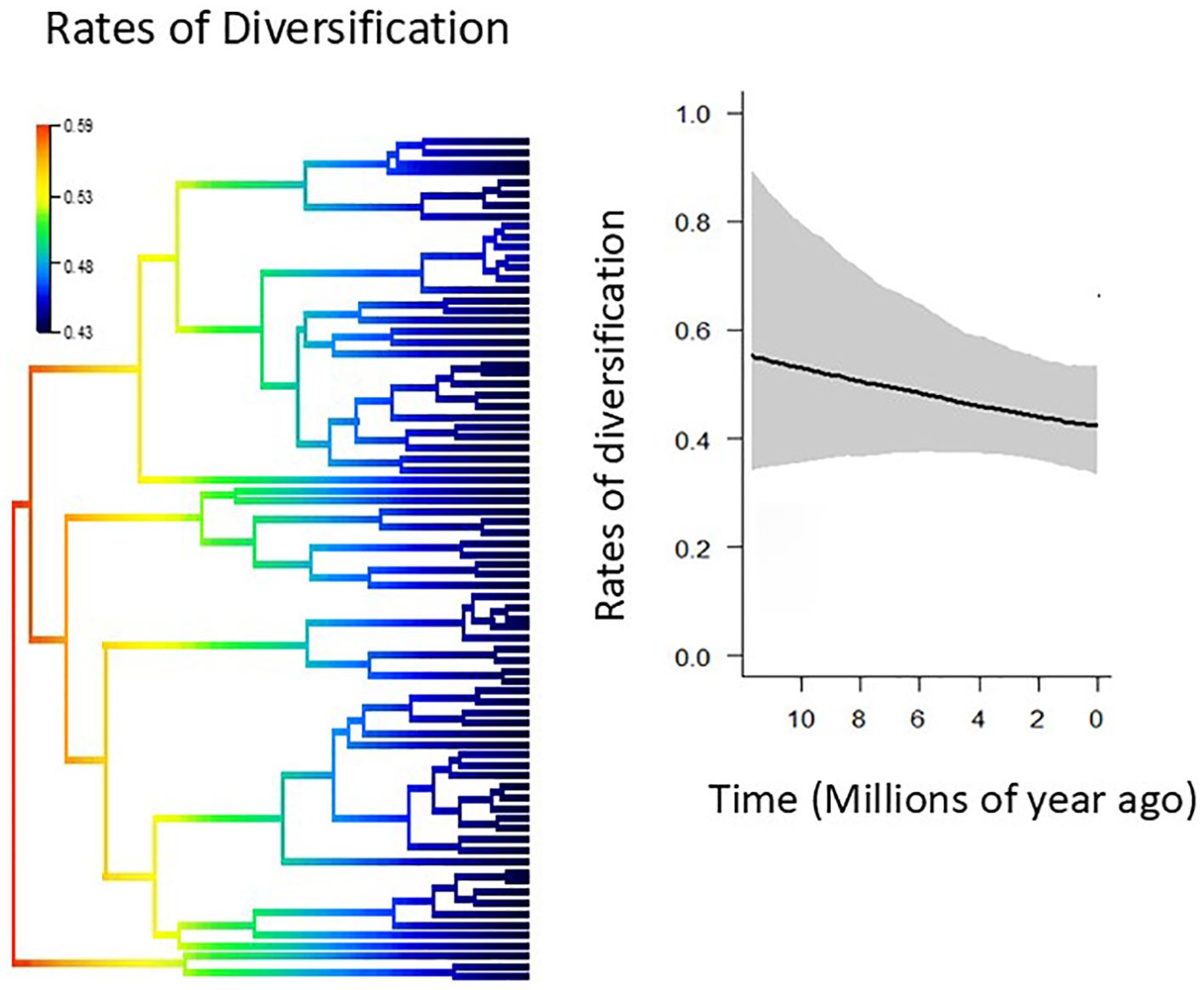
Figure 5. Comparison of net diversification rates through phylogeny (left) and time (right) obtained from BAMM analyses. The most probable scenario indicated that diversification rates were constant over time with no shifts (P=1.0).
Discussion
Our findings suggest that the most likely growth form of the ancestor of Chilean Adesmia was a perennial herb, which is also the predominant growth form in its sister genera Chaetocalyx, Zornia, and Amicia. However, incorporating phylogenetic uncertainty makes this conclusion less certain. In 82% of the 1,000 sampled trees, the most probable ancestor of Adesmia was identified as a perennial herb. On average, the likelihood that the ancestor was a perennial herb (53%) was twice as high as the probability that it was an annual (26%) or a shrub (21%). Our results also suggest that the ancestor of Chilean Adesmia likely originated in the high Andes of northern and central Chile. The low and middle elevations of central Chile were colonized in the late Miocene. These events correlate with the last pulses of Andean uplift in northern and central Chile (Ramos and Giglione, 2008; Giambiagi et al., 2016; Garzione et al., 2017) and the intensification of aridification produced by the establishment of a phase of global climate cooling (Hinojosa and Villagrán, 1997; Hartley et al., 2005; Zachos et al., 2001).
Patagonia was colonized by perennial herbs in the Pliocene (Clade D), and later by spiny shrubs. Shifts between the high Andes and the Patagonian steppe are common (Heibl and Renner, 2012), but diversification patterns are generally consistent with south-to-north migration (Nicolas and Plunkett, 2012; Simpson et al., 2009). We found that the climatic niche associated with temperature is highly conserved in the groups that reached Patagonia (clade D and subclade E1), supporting the hypothesis that Andes acted as a corridor facilitating the dispersion of lineages that track cold conditions (Luebert and Weigend, 2014).
Atacama Desert was colonized repeatedly since the middle Pliocene, first by lineages that acquired an annual habit, and later by several lineages of perennial herbs and spiny shrubs. Multiples plant genera colonized the coastal Atacama Desert in the last 10 Mya, including Heliotropium sect. Cochranea (Luebert and Wen, 2008), Malesherbia (Gengler-Nowak, 2002), Nolana (Tu et al., 2008), Cristaria (Bohnert et al., 2022) and Argylia (Glade-Vargas et al., 2021). Different scenarios have been proposed to explain these diversification patterns. Glade-Vargas et al. (2021) suggested that intensification of aridification during the late Miocene and Pliocene, together with the elevation of coastal range during Pleistocene, generated a biogeographic corridor along the Pacific Coast. According to this hypothesis, we observed a close relationship between coastal Atacama and Mediterranean species from subclades E2 and E3. However, we also identified a close relationship between species from different elevations, indicating that east-west migrations also occurred. Activation of east-west oriented canyons during the brief pluvial phases that interrupted hyperarid conditions in the Pliocene and Pleistocene of northern Chile (Jordan et al., 2014) might have facilitated species migration. Recently Bohnert et al. (2022) showed that Cristaria lineages colonized different habitats in the Atacama Desert during these pluvial phases.
Adesmia showed multiple and bidirectional transitions between growth forms that are non-significantly associated with climate. Interestingly, annuals, perennial herbs, and shrubs can coexist under the same climatic conditions. This pattern has also been described by Hjertaas et al. (2023), who suggest that similar environments can select for different life history strategies, or alternatively, that the evolution of life strategies can be constrained by historical and developmental factors. Cousins-Westerberg et al. (2023) also failed to detect a relationship between cold tolerance and growth form in Salicornieae. These results also parallel findings in Aeonium (dos Santos et al., 2022), but in this case, the authors demonstrated that growth forms modulate the response of leaf size, height, and other plant traits to climate rather than being adaptations per se. Our results contrast to other comparative studies indicating that climate strongly influences the evolution of annuality (Friedman, 2020; Boyko et al., 2023), plant height (Bliss, 1971; Korner, 2003; Zanne et al., 2014), and woodiness (Howard et al., 2019; Klimes et al., 2022).
The shrub habit evolved at least three times, with two reversions to the herbaceous form. Across angiosperms, multiple transitions between herbaceous and woody habits have occurred (Klimes et al., 2022), encompassing varying degrees of woodiness, from extensive wood production (in shrubs or trees) to reduced secondary growth limited to the basis of stems (typically seen in perennial herbs) or to fascicular areas (Rowe and Paul-Victor, 2012; Trueba et al., 2015). Woodiness has been observed in island (Dulin and Kirchoff, 2010; Zizka et al., 2022) and continental lineages (Kidner et al., 2016), where it evolved in response to multiple factors such as drought resistance (Frankiewicz et al., 2021; Klimes et al., 2022), extended plant longevity resulting from a stable climate or reduced herbivory (Zizka et al., 2022), and adaptation to toxic soils (Kidner et al., 2016). Conversely, the herbaceous habit is linked to tolerance to frost and shade (Klimes et al., 2022).
The annual habit originated five times independently from a perennial herbaceous ancestor. Three transitions matched events of desert colonization, in agreement with other comparative studies that show than annual form is favored in warm and arid environments (Evans et al., 2005; Friedman and Rubin, 2015; Pérez et al., 2020). However, it also has been suggested that annual habit might evolve under other seasonally stressful conditions, such as flooding and erosion (Hjertaas et al., 2023) or frosty seasons (Kadereit et al., 2007) as an escape strategy. Accordingly, the annual habit in Adesmia also evolved twice in the High Andes, where short growing seasons and repeated frosts are thought to inhibit seedling establishment (Bliss, 1971; Korner, 2003; Givnish, 2015). Warm and moist soils under the snowpack is also expected to difficult the persistence of seed banks in high elevations (Hjertaas et al., 2023). The evolution of the annual habit in A. capitellata is accompanied by the acquisition of autonomous selfing and reduced allocation to floral structures (Arroyo and Uslar, 1993), suggesting that the annual strategy might have evolved as part of a rapid development strategy that copes with the impoverished and variable conditions for animal pollination of high Andes (Arroyo et al., 2006).
We also detected at least one reversion from the annual form to the perennial form. Transitions from perennial to annual habitat have occurred thousands of times in flowering plants, but reversions are less frequent (Friedman, 2020; Boyko et al., 2023; Hjertaas et al., 2023). Derived perennial habits have been described, for example, in Orobanchaceae and Montiaceae (Tank and Olmstead, 2008; Ogburn and Edwards, 2015).
BAMM analyses indicated a constant rate of diversification, suggesting that the high species diversity of Chilean Adesmia is due to uniform speciation rather than to accelerated events of speciation. No evidence of radiations was also found in Oxalis (Heibl and Renner, 2012), which colonized Andean and desert habitats several times. Accelerated speciation events have been detected in lineages that acquired a perennial habit, which acted as a key innovation in high elevation habitats (Drummond et al., 2012; Hughes and Atchison, 2015; Nevado et al., 2016). In contrast, our data indicates that shifts in growth form in Adesmia did not lead to an increase in speciation. Furthermore, perennial herbs and spiny shrubs shifted repeatedly between low and high elevations habitats, suggesting that these are successful adaptations to survive both drought and unfavorable cold seasons. However, it is important to highlight that our study sampled only the 64% of Chilean Adesmia species. According to Cusimano and Renner (2010), sampling less than 80% of the species can introduce biases toward downturns. These biases are particularly pronounced when deeper nodes are oversampled. Nevertheless, we believe this issue does not significantly affect our study because we made a deliberate effort to include species representing the full range of morphological and taxonomic diversity within Chilean Adesmia.
It is also important to note that our study only sampled the 34% of the species in the genus. Little is known about phylogenetical relationships of the remaining species of Adesmia, and accordingly, expanding the sample size could potentially alter the outcomes of this study, particularly the ancestral reconstruction of area and growth form. A phylogenetic study has been conducted for Brazilian species of sect. psoraleoides (Iganci et al., 2013). This study, which included some species from other regions, found a north to south pattern of diversification, suggesting an expansion from arid regions to Patagonia, followed by colonization of southern Brazil. These results indicate that Chilean species might be paraphyletic.
Our phylogenetic analysis based on five nuclear regions of Chilean Adesmia does not match Burkart (1967) classification of Adesmia into two subgenera: Acanthadesmia, which groups spiny shrubs, and Adesmia, which groups unarmed forms. Ancestral reconstruction for spine presence/absence suggest that spiny structures were acquired by the ancestor of Clade E. These structures would have lost at least once in each subclade (E1, E2 and E3) is not monophyletic. Discrepancy between morphological and molecular data was also detected by Iganci et al. 2013. Most series described for Adesmia by Burkart (1967) were also not recovered in the molecular phylogeny of Chilean Adesmia, indicating that the systematics of the genus should be revisited.
Overall, our results showed that growth forms are evolutionarily labile in Adesmia. However, contrary to expectations, transitions were not significantly associated with climate, suggesting that annual, perennial herbaceous, and woody habits are alternative and successful strategies to survive unfavorable seasons in both the Atacama Desert and the high Andes. The high species diversity in these regions resulted from repeated events of colonization facilitated by adaptations that allowed plants to cope with both drought and cold.
Data availability statement
The datasets presented in this study can be found in online repositories. The names of the repository/repositories and accession number(s) can be found below: https://www.ncbi.nlm.nih.gov/genbank/, Numbers in Table 1.
Author contributions
FP: Conceptualization, Formal analysis, Funding acquisition, Investigation, Project administration, Writing – original draft, Writing – review & editing, Methodology. NL: Data curation, Formal analysis, Methodology, Writing – review & editing, Investigation. LH: Conceptualization, Formal analysis, Investigation, Writing – review & editing. MC: Writing – review & editing, Investigation, Methodology. DA: Data curation, Methodology, Writing – review & editing. NS: Investigation, Methodology, Writing – review & editing. VM: Methodology, Writing – review & editing.
Funding
The author(s) declare that financial support was received for the research, authorship, and/or publication of this article. This study was supported by Fondecyt-Chile grant 1211765 to FP and Fondecyt-Chile grant 1221214 to FH.
Acknowledgments
We are grateful to the curators and staff of CONC (Alicia Marticorena) and SGO (Gloria Rojas and Victor Ardiles) herbaria for providing access to material under their care. We thank Gioconda Peralta of the Plataforma de Secuenciación y Tecnologías Ómicas, Pontificia Universidad Católica de Chile for laboratory support and expert capillary electrophoresis analysis (CONICYT–FONDEQUIP EQM1500777). We would like to thank Claire de Schrevel, Phillipe Dandois, Macarena Villalobos, Ludovica Santilli and Mauricio Rebolledo for providing essential herbarium material of Adesmia for the present work.
Conflict of interest
The authors declare that the research was conducted in the absence of any commercial or financial relationships that could be construed as a potential conflict of interest.
Publisher’s note
All claims expressed in this article are solely those of the authors and do not necessarily represent those of their affiliated organizations, or those of the publisher, the editors and the reviewers. Any product that may be evaluated in this article, or claim that may be made by its manufacturer, is not guaranteed or endorsed by the publisher.
Supplementary material
The Supplementary Material for this article can be found online at: https://www.frontiersin.org/articles/10.3389/fpls.2024.1403273/full#supplementary-material
References
Abraham, E., Rodríguez, M., Rubio, C., Guida–Johnson, B., Gómez, M., Rubio, C. (2020). Disentangling the concept of “South american arid diagonal. J. Arid Env 175. doi: 10.1016/j.jaridenv.2019.104089
Antonelli, A. (2015). Biodiversity: multiple origins of mountain life. Nature 524, 300–301. doi: 10.1038/nature14645
Antonelli, A., Nylander, J., Persson, C., Sanmartín, I. (2009). Tracing the impact of the Andean uplift on Neotropical plant evolution. PNAS 106, 9749–9754. doi: 10.1073/pnas.0811421106
Ariati, S. R., Murphy, D. J., Udovicic, F., Ladiges, P. Y. (2006). Molecular phylogeny of three groups of acacias (Acacia subgenus Phyllodineae) in arid Australia based on the internal and external transcribed spacer regions of nrDNA. Syst. Biodivers 4, 417–426. doi: 10.1017/S1477200006001952
Arroyo, M. T. K., Muñoz, M. S., Henríquez, C., Till-Bottraud, I., Pérez, F. (2006). Erratic pollination, high selfing levels and their correlates and consequences in an altitudinally widespread above-tree-line species in the high Andes of Chile. Acta Oecol 30, 248–257. doi: 10.1016/j.actao.2006.05.006
Arroyo, M. T. K., Uslar, P. (1993). Breeding systems in a temperate mediterranean-type climate montane sclerophyllous forest in central Chile. Bot. J. Linn. Soc 111, 83–102. doi: 10.1111/j.1095-8339.1993.tb01892.x
Bliss, L. C. (1971). Arctic and alpine plant life cycles. Annu. Rev. Ecol. Syst. 2, 405–438. doi: 10.1146/annurev.es.02.110171.002201
Böhnert, T., Luebert, F., Merklinger, F. F., Harpke, D., Stoll, A., Schneider, J. V., et al. (2022). Plant migration under long-lasting hyperaridity–phylogenomics unravels recent biogeographic history in one of the oldest deserts on Earth. New Phytol. 234, 1863–1875. doi: 10.1111/nph.18082
Boucher, F. C., Verboom, G. A., Musker, S., Ellis, A. G. (2017). Plant size: a key determinant of diversification? New Phyto 216, 24–31. doi: 10.1111/nph.14697
Boyko, J. D., Hagen, E. R., Beaulieu, J. M., Vasconcelos, T. (2023). The evolutionary responses of life-history strategies to climatic variability in flowering plants. New Phytol. 240, 1587–1600. doi: 10.1111/nph.18971
Burkart, A. (1967). Sinopsis del género sudamericano de Leguminosas Adesmia DC.(Contribución al estudio del género Adesmia, VII). Darwinianat. 14, 463–568.
Choi, H. K., Kim, D., Uhm, T., Limpens, E., Lim, H., Mun, J. H., et al. (2004). A sequence-based genetic map of Medicago truncatula and comparison of marker colinearity with M. sativa. Genetics 166, 1463–1502. doi: 10.1534/genetics.166.3.1463
Cousins-Westerberg, R., Dakin, N., Schat, L., Kadereit, G., Humphreys, A. M. (2023). Evolution of cold tolerance in the highly stress-tolerant samphires and relatives (Salicornieae: Amaranthaceae). Bot. J. Linn. Soc. 203, 20–36. doi: 10.1093/botlinnean/boad009
Cusimano, N., Renner, S. S. (2010). Slowdowns in diversification rates from real phylogenies may not be real. Syst- Biol. 59, 458–464. doi: 10.1093/sysbio/syq032
de Vos, J. M., Hughes, C. E., Schneeweiss, G. M., Moore, B. R., Conti, E. (2014). Heterostyly accelerates diversification via reduced extinction in primroses. Proc. R. Soc Biol. Sc 281. doi: 10.1098/rspb.2014.0075
dos Santos, P., Brilhante, M. A., Messerschmid, T., Thibaud, F. E., Serrano, H. C., Branquinho, C., et al. (2022). Plant growth forms dictate adaptations to the local climateFront. Plant Sci. 13. doi: 10.3389/fpls.2022.1023595
Drummond, C. S., Eastwood, R. J., Miotto, S. T., Hughes, C. E. (2012). Multiple continental radiations and correlates of diversification in Lupinus (Leguminosae): testing for key innovation with incomplete taxon sampling. Syst. Biol. 61, 443–460. doi: 10.1093/sysbio/syr126
Drummond, A. J., Rambaut, A. (2007). BEAST: Bayesian evolutionary analysis by sampling tress. Evol. Biol. 7, 214–221. doi: 10.1186/1471–2148-7-214
Dulin, M. W., Kirchoff, B. K. (2010). Paedomorphosis, secondary woodiness, and insular woodiness in plants. Bot. Rev. 76, 405–490. doi: 10.1111%2Fnph.15797
Dunai, T. J., López, G. A. G., Juez-Larré, J. (2005). Oligocene–Miocene age of aridity in the Atacama Desert revealed by exposure dating of erosion-sensitive landforms. Geology 33, 321–324. doi: 10.1130/g21184.1
Edwards, E. J., Donoghue, M. J. (2013). Is it easy to move and easy to evolve? Evolutionary accessibility and adaptation. J. Exp. Bot. 64, 4047–4052. doi: 10.1093/jxb/ert220
Eshel, G., Araus, V., Undurraga, S., Soto, D. C., Moraga, C., Montecinos, A., et al. (2021). Plant ecological genomics at the limits of life in the Atacama Desert. PNAS 118, e2101177118. doi: 10.1073/pnas.2101177118
Evans, M. E. K., Hearn, D., Hahn, W., Spangle, J., Venable, D. L. (2005). Climate and life-history evolution in evening primroses (Oenothera, Onagraceae): a phylogenetic comparative analysis. Evolution 59, 1914–1927. doi: 10.1111/j.0014-3820.2005.tb01061.x
Frankiewicz, K. E., Banasiak, Ł., Oskolski, A. A., Magee, A. R., Alsarraf, M., Trzeciak, P., et al. (2021). Derived woodiness and annual habit evolved in african umbellifers as alternative solutions for coping with drought. BMC Plant Biol. 21, 1–18. doi: 10.1186/s12870-021-03151-x
Friedman, J. (2020). The evolution of annual and perennial plant life histories: ecological correlates and genetic mechanisms. Annu. Rev. Ecol. Evol. Syst. 51, 461–481. doi: 10.1146/annurev-ecolsys-110218-024638
Friedman, J., Rubin, M. J. (2015). All in good time: understanding annual and perennial strategies in plants. Am. J. Bot. 102, 497–499. doi: 10.3732/ajb.1500062
Garreaud, R. D., Molina, A., Farias, M. (2010). Andean uplift, ocean cooling and Atacama hyperaridity: a climate modeling perspective. Earth Planet Sci. Lett. 292, 39–50. doi: 10.1016/j.epsl.2010.01.017
Garzione, C. N., McQuarrie, N., Perez, N. D., Ehlers, T. A., Beck, S. L., Kar, N., et al. (2017). Tectonic evolution of the Central Andean plateau and implications for the growth of plateaus. Ann. Rev. Earth Planet Sci. 45, 529–559. doi: 10.1146/annurev-earth-063016-020612
Gengler-Nowak, K. (2002). Reconstruction of the biogeographical history of Malesherbiaceae. Bot. Rev. 68, 171–188. doi: 10.1663/0006-8101(2002)068[0171:ROTBHO]2.0.CO;2
Giambiagi, L., Mescua, J., Bechis, F., Hoke, G., Suriano, J., Spagnotto, S., et al. (2016). “Cenozoic orogenic evolution of the southern central Andes (32–36°S),”. Ib: Growth of the Southern Andes. Eds. Folguera, A., Naipauer, M., Sagripanty, L., Ghiglione, M. C., Orts, D., Giambiagi, L. (Buenos Aires: Springer), 63–98. doi: 10.1007/978-3-319-23060-3
Givnish, T. J. (2015). Adaptive radiation versus ‘radiation’ and ‘explosive diversification’: why conceptual distinctions are fundamental to understanding evolution. New Phytol. 207, 297–303. doi: 10.1111/nph.13482
Glade-Vargas, N. S., Rojas, C., Jara-Arancio, P., Vidal, P., Arroyo, M. T. K., Hinojosa, L. F. (2021). Biogeography of Argylia D. Don (Bignoniaceae): diversification, Andean uplift and niche conservatism. Front. Plant Sci. 12. doi: 10.3389/fpls.2021.724057
Grime, J. P. (1977). Evidence for the existence of three primary strategies in plants and its relevance to ecological and evolutionary theory. Am. Nat. 111, 1169–1194. doi: 10.1086/283244
Hall, T. A. (1999). BioEdit: a user-friendly biological sequence alignment editor and analysis program for windows 95/98/NT. Nucleic Acids Symp. 41, 95–98.
Hartley, A., Chong, G., Houston, J., Mather, A. E. (2005). 150 million years of climatic stability: evidence from the Atacama Desert, Northern Chile. J. Geol. Soc 162, 421–424. doi: 10.1144/0016-764904-071
Harvey, P. H., Pagel, M. D. (1991). The Comparative Method in Evolutionary Biology (Oxford:Oxford University Press).
Heibl, C. (2011). PHYLOCLIM: Integrating Phylogenetics and Climatic Niche Modelling. R Package Version 0.9.5. Available online at: https://cran.rproject.org/web/packages/phyloclim/phyloclim.pdf (Accessed Jun 2022).
Heibl, C., Renner, S. S. (2012). Distribution models and a dated phylogeny for Chilean Oxalis species reveal occupation of new habitats by different lineages, not rapid adaptive radiation. Syst. Biol. 61, 823–834. doi: 10.1093/sysbio/sys034
Hijmans, R. J., Cameron, S. E., Parra, J. L., Jones, P. G., Jarvis, A. (2005). Very high resolution interpolated climate surfaces for global land areas. Int. J. Climatol. 25, 1965–1978. doi: 10.1002/joc.1276
Hinojosa, L. F., Villagrán, C. (1997). Historia de los bosques del sur de Sudamérica, I: antecedentes paleobotánicos, geológicos y climáticos del Terciario del cono sur de América. Rev. Chil Hist Nat. 70, 225–239.
Hjertaas, A. C., Preston, J. C., Kainulainen, K., Humphreys, A. M., Fjellheim, S. (2023). Convergent evolution of the annual life history syndrome from perennial ancestors. Front. Plant Sci. 13. doi: 10.3389/fpls.2022.1048656
Ho, L. S. T., Ane, C. (2014). A linear-time algorithm for Gaussian and non-Gaussian trait evolution models. Syst. Biol. 63, 397–408.
Howard, C. C., Folk, R. A., Beaulieu, J. M., Cellinese, N. (2019). The monocotyledonous underground: global climatic and phylogenetic patterns of geophyte diversity. Am. J. Bot. 106, 850–863. doi: 10.1002/ajb2.1289
Hughes, C. E., Atchison, G. W. (2015). The ubiquity of alpine plant radiations: from the Andes to the Hengduan Mountains. New Phytol. 207, 275–282. doi: 10.1111/nph.13230
Hughes, C., Eastwood, R. (2006). Island radiation on a continental scale: exceptional rates of plant diversification after uplift of the Andes. PNAS 103, 10334–10339. doi: 10.1073/pnas.0601928103
Iganci, J. R. V., Miotto, S. T. S., Souza-Chies, T. T., Särkinen, T. E., Simpson, B. B., Simon, M. F., et al. (2013). Diversification history of Adesmia ser. psoraleoides (Leguminosae).: evolutionary processes and the colonization of the southern Brazilian highland grasslands. S Afr J. Bot. 89, 257–264. doi: 10.1016/j.sajb.2013.06.016
Jordan, T. E., Kirk-Lawlor, N. E., Blanco, N. P., Rech, J. A., Cosentino, N. J. (2014). Landscape modification in response to repeated onset of hyperarid paleoclimate states since 14 Ma, Atacama Desert, Chile. Bulletin 126, 1016–1046. doi: 10.1130/B30978.1
Kadereit, G., Ball, P., Beer, S., Mucina, L., Sokoloff, D., Teege, P., et al. (2007). A taxonomic nightmare comes true: phylogeny and biogeography of glassworts (Salicornia L., Chenopodiaceae). Taxon 56, 1143–1170. doi: 10.2307/25065909
Kenicer, G. J., Kajita, T., Pennington, R. T., Murata, J. (2005). Systematics and biogeography of Lathyrus (Leguminosae) based on internal transcribed spacer and cpDNA sequence data. Am. J. Bot. 92, 1199–1209. doi: 10.3732/ajb.92.7.1199
Kidner, C., Groover, A., Thomas, D. C., Emelianova, K., Soliz-Gamboa, C., Lens, F. (2016). First steps in studying the origins of secondary woodiness in Begonia (Begoniaceae): combining anatomy, phylogenetics, and stem transcriptomics. Biol. J. Linn. Soc 117, 121–138. doi: 10.1111/bij.12492
Klimeš, A., Šímová, I., Zizka, A., Antonelli, A., Herben, T. (2022). The ecological drivers of growth form evolution in flowering plants. J. Ecol. 110, 1525–1536. doi: 10.1111/1365-2745.13888
Kooyers, N. J. (2015). The evolution of drought escape and avoidance in natural herbaceous populations. Plant Sci. 234, 155–162. doi: 10.1016/j.plantsci.2015.02.012
Korner, C. (2003). Alpine Plant Life: Functional Plant Ecology of High Mountain Ecosystems (Berlin: Springer).
Luebert, F., Weigend, M. (2014). Phylogenetic insights into Andean plant diversification. Front. Ecol. Evol. 2. doi: 10.3389/fevo.2014.00027
Luebert, F., Wen, J. (2008). Phylogenetic analysis and evolutionary diversification of Heliotropium sect. cochranea (Heliotropiaceae) in the Atacama Desert. Syst. Bot. 33, 390–402. doi: 10.1600/036364408784571635
Matzke, N. J. (2014). Model selection in historical biogeography reveals that founder-event speciation is a crucial process in island clades. Syst. Biol. 63, 951–970. doi: 10.1093/sysbio/syu056
Meade, A., Pagel, M. (2023). BayesTraits V4.0.0. Manual updated October 2023. Retrieved May 1, 2024. Available online at: https://www.evolution.reading.ac.uk/BayesTraitsV4.0.0/Files/BayesTraitsV4.0.0-Manual.pdf.
Mihoc, M. (2012). Sistemática y Biogeografía del Subgénero Acanthadesmia Burkart (Adesmia, Fabaceae). Universidad de Concepción, Concepción.
Nevado, B., Atchison, G. W., Hughes, C. E., Filatov, D. A. (2016). Widespread adaptive evolution during repeated evolutionary radiations in New World lupins. Nat. Commun. 7, 12384. doi: 10.1038/ncomms12384
Nicolas, A. N., Plunkett, G. M. (2012). Untangling generic limits in Azorella, Laretia, and Mulinum (Apiaceae: Azorelloideae): insights from phylogenetics and biogeography. Bot. J. Linn. Soc 170, 49–79. doi: 10.1002/tax.614008
Nürk, N. M., Atchison, G. W., Hughes, C. E. (2019). Island woodiness underpins accelerated disparification in plant radiations. New Phytol. 224, 518–531. doi: 10.1111/nph.15797
Ogburn, R. M., Edwards, E. J. (2015). Life history lability underlies rapid climate niche evolution in the angiosperm clade Montiaceae. Mol. Phylogenet. Evol. 92, 181–192. doi: 10.1016/j.ympev.2015.06.006
Pagel, M., Meade, A. (2006). Bayesian analysis of correlated evolution of discrete characters by reversible-jump Markov chain Monte Carlo. Am. Nat. 167, 808–825. doi: 10.1086/503444
Pérez, F., Lavandero, N., Ossa, C. G., Hinojosa, L. F., Jara-Arancio, P., Arroyo, M. T. K. (2020). Divergence in plant traits and increased modularity underlie repeated transitions between low and high elevations in the Andean Genus Leucheria. Front. Plant Sci. 11. doi: 10.3389/fpls.2020.00714
Pérez-Escobar, O. A., Zizka, A., Bermúdez, M. A., Meseguer, A. S., Condamine, F. L., Hoorn, C., et al. (2022). The Andes through time: evolution and distribution of Andean floras. Trends Plant Sci. doi: 10.1016/j.tplants.2021.09.010
Phillips, S. J., Anderson, R. P., Schapire, R. E. (2006). Maximum entropy modeling of species geographic distributions. Ecol. Model. 190, 231–259. doi: 10.1016/j.ecolmodel.2005.03.026
Pirie, M. D. (2015). Phylogenies from concatenated data: Is the end nigh? Taxon 64, 421–423. doi: 10.12705/643.1
Rabosky, D. L., Grundler, M., Anderson, C., Title, P., Shi, J. J., Brown, J. W., et al. (2014). BAMM tools: an R package for the analysis of evolutionary dynamics on phylogenetic trees. Methods Ecol. Evol. 5, 701–707. doi: 10.1111/2041-210X.12199
Ramos, V. A., Giglione, M. C. (2008). Tectonic evolution of the patagonian andes. Dev. Quat. Sci. 11, 57–71. doi: 10.1016/S1571-0866(07)10004-X
Raunkiaer, C. (1934). The life forms of plants and statistical plant geography (London, UK: Oxford University Press).
Ree, R. H., Smith, S. A. (2008). Maximum likelihood inference of geographic range evolution by dispersal, local extinction, and cladogenesis. Syst. Biol. 57, 4–14. doi: 10.1080/10635150701883881
Revell, L. J. (2012). Phytools: an R package for phylogenetic comparative biology (and other things). Methods Ecol. Evol. 3, 217–223. doi: 10.1111/j.2041-210X.2011.00169.x
Rodríguez, R., Marticorena, C., Alarcón, D., Baeza, C., Cavieres, L., Finot, V. L., et al. (2018). Catálogo de las plantas vasculares de Chile. Gayana Bot. 75, 1–430. doi: 10.4067/S0717-66432018000100001
Ronquist, F., Teslenko, M., van der Mark, P., Ayres, D. L., Darling, A., Höhna, S., et al. (2012). MrBayes 3.2: efficient Bayesian phylogenetic inference and model choice across a large model space. Syst. Biol. 61, 539–542. doi: 10.1093/sysbio/sys029
Rowe, N., Paul-Victor, C. (2012). Herbs and secondary woodiness–keeping up the cambial habit. New Phytol. 193, 3–5. doi: 10.1111/j.1469-8137.2011.03980.x
Scherson, R. A., Vidal, R., Sanderson, M. J. (2008). Phylogeny, biogeography, and rates of diversification of New World Astragalus (Leguminosae). with an emphasis on South American radiations. Am. J. Bot. 95, 1030–1039. doi: 10.3732/ajb.0800017
Schluter, D., Price, T., Mooers, A. Ø., Ludwig, D. (1997). Likelihood of ancestor states in adaptive radiation. Evolution 51, 1699–1711. doi: 10.1111/j.1558-5646.1997.tb05095.x
Simon, M. F., Grether, R., de Queiroz, L. P., Skema, C., Pennington, R. T., Hughes, C. E. (2009). Recent assembly of the cerrado, a neotropical plant diversity hotspot, by in situ evolution of adaptations to fire. Proc. Natl. Acad. Sci. 106, 20359–20364. doi: 10.1073/pnas.0903410106
Simpson, B. B. (1983). An historical phytogeography of the high Andean flora. Rev.Chil Hist Nat. 56, 109–122.
Simpson, B. B., Arroyo, M. T., Sipe, S., Dias de Moraes, M., McDill, J. (2009). Phylogeny and evolution of perezia (Asteraceae, mutisieae, nassauviinae). J. Syst. Evol. 47, 431–443. doi: 10.1111/j.1759-6831.2009.00039.x
Smith, S. A., Donoghue, M. J. (2008). Rates of molecular evolution are linked to life history in flowering plants. Science 322, 86–89. doi: 10.1126/science.1163197
Stearns, S. C. (2000). Life history evolution: successes, limitations, and prospects. Naturwiss 87, 476–486. doi: 10.1007/s001140050763
Swofford, D. L. (2003). PAUP* (Phylogenetic Analysis Using Parsimony and Other Methods), Version 4.0b10 (Sinauer Associates, Sunderland, Massachusetts). doi: 10.1111/j.0014-3820.2002.tb00191.x
Tank, D. C., Olmstead, R. G. (2008). From annuals to perennials: phylogeny of subtribe Castillejinae (Orobanchaceae). Am. J. Bot. 95, 608–625. doi: 10.3732/ajb.2007346
Taylor, A., Weigelt, P., Denelle, P., Cai, L., Kreft, H. (2023). The contribution of plant life and growth forms to global gradients of vascular plant diversity. New Phytol. doi: 10.1111/nph.19011
Trueba, S., Rowe, N. P., Neinhuis, C., Wanke, S., Wagner, S. T., Isnard, S. (2015). Stem anatomy and the evolution of woodiness in Piperales. Int. J. Pl Sc 176, 468–485. doi: 10.1086/680595
Tu, T., Dillon, M. O., Sun, H., Wen, J. (2008). Phylogeny of Nolana (Solanaceae) of the Atacama and Peruvian deserts inferred from sequences of four plastid markers and the nuclear LEAFY second intron. Mol. Phylogenet. Evol. 49, 561–573. doi: 10.1016/j.ympev.2008.07.018
Villagrán, C., Hinojosa, L. F. (1997). Historia de los bosques del sur de Sudamérica, II: Análisis fitogeográfico. Rev. Chil Hist Nat. 70, 241–267.
Yu, Y., Harris, A. J., Blair, C., He, X. J. (2015). RASP (Reconstruct Ancestral State in Phylogenies): a tool for historical biogeography. Mol. Phylogenet. Evol. 87, 46–49. doi: 10.1016/j.ympev.2015.03.008
Zachos, J., Pagani, M., Sloan, L., Thomas, E., Billups, K. (2001). Trends, rhythms, and aberrations in global climate 65 Ma to present. Science 292, 686–693. doi: 10.1126/science.1059412
Zanne, A. E., Tank, D. C., Cornwell, W. K., Eastman, J. M., Smith, S. A., FitzJohn, R. G., et al. (2014). Three keys to the radiation of angiosperms into freezing environments. Nature 506, 89–92. doi: 10.1038/nature12872
Keywords: Andes, Atacama Desert, biogeography, climatic niche, diversification, life history strategy, South American Arid Diagonal
Citation: Pérez F, Lavandero N, Hinojosa FL, Cisternas M, Araneda D, Pinilla N and Moraga V (2024) Speciation and evolution of growth form in Adesmia D. C. (Dalbergieae, Fabaceae): the relevance of Andean uplift and aridification. Front. Plant Sci. 15:1403273. doi: 10.3389/fpls.2024.1403273
Received: 19 March 2024; Accepted: 27 September 2024;
Published: 15 October 2024.
Edited by:
Aelys Muriel Humphreys, Stockholm University, SwedenReviewed by:
Jurriaan Michiel de Vos, University of Basel, SwitzerlandDanilo T. Amaral, Universidade Federal do ABC, Brazil
Copyright © 2024 Pérez, Lavandero, Hinojosa, Cisternas, Araneda, Pinilla and Moraga. This is an open-access article distributed under the terms of the Creative Commons Attribution License (CC BY). The use, distribution or reproduction in other forums is permitted, provided the original author(s) and the copyright owner(s) are credited and that the original publication in this journal is cited, in accordance with accepted academic practice. No use, distribution or reproduction is permitted which does not comply with these terms.
*Correspondence: Fernanda Pérez, bXBlcmV6dEBiaW8ucHVjLmNs