Corrigendum: Effects of different sowing dates on biomass allocation of various organs and allometric growth of Fagopyrum esculentum
- 1State Key Laboratory of Black Soils Conservation and Utilization, Northeast Institute of Geography and Agroecology, Chinese Academy of Sciences, Changchun, China
- 2Jilin Provincial Key Laboratory of Grassland Animal Husbandry, Northeast Institute of Geography and Agroecology, Chinese Academy of Sciences, Changchun, China
- 3University of Chinese Academy of Sciences, Beijing, China
- 4State key Laboratory of Vegetation and Environmental Change, Institute of Botany, Chinese Academy of Sciences (CAS), Beijing, China
- 5College of Life Sciences, Jilin Agricultural University, Changchun, China
Introduction: The sowing date plays a crucial role in influencing the growth and reproduction of plants, with its specific impact on biomass allocation and allometric growth remaining unclear. Understanding these effects is essential for optimizing agricultural practices and enhancing crop productivity.
Methods: To investigate the effects of sowing dates on biomass allocation and allometric growth, a field experiment was conducted with sequential sowings of Fagopyrum esculentum from April 12th to August 11th in 2018. Biomass measurements were taken across various plant organs, and corresponding allocation calculations were made. A detailed analysis of the allometric growth relationship involving organ biomass variations was performed.
Results: The study revealed that the accumulation and allocation of organ biomass in buckwheat were significantly impacted by the sowing dates. Delayed planting led to reduced vegetative growth and increased biomass allocation towards reproduction. Allometric parameters such as exponent, constant, and individual size of buckwheat were notably affected by delayed planting. Interestingly, the allometric exponents governing the relationships between reproductive vs. vegetative biomass and belowground vs. aboveground biomass exhibited varying trends across different sowing dates.
Discussion: Notably, late sowings resulted in significantly higher reproductive biomass compared to early and middle sowings. These findings highlight the nuanced relationship between plant size and reproductive biomass under different sowing dates, emphasizing the critical role of planting timing in shaping mature plant sizes and reproductive outcomes. The study underscores the importance of considering sowing dates in agricultural practices to optimize plant growth and productivity.
1 Introduction
Plants undergo growth and reproduce throughout their life cycle. As plants experience grow, they need to adapt the dynamic environmental conditions. Previous research indicates that the increasing impact of climate change has repercussions on plant phenology, consequently influencing their growth and reproductive processes (Piao et al., 2019). Consequently, plants need to harmonize resource allocation among different organs and adjust their traits to adapt to the changing environment (Poorter et al., 2012; Heilmeier, 2019; Myers-Smith et al., 2019).
Biomass allocation significantly influences plant growth and productivity (McCarthy and Enquist, 2007). Plants continually allocate acquired resources, such as carbon and nutrients, to different tissues during growth (Shipley and Meziane, 2002). How plants respond to resource availability is a central question in plant ecology (McConnaughay and Coleman, 1999). The applications of biomass allocation in agriculture are extensive, as allocation patterns and their degree of variation between species constrain biomass production and utilization (Poorter et al., 2012). In essence, biomass allocation may vary over time, across environments, and among species (Niklas, 1994; Reich, 2002). In agroecosystems, crop productivity depends not only on the accumulation of dry matter, but also on the efficient distribution of dry matter to economically important plant parts (Kumar et al., 2006). Nutrition, temperature, solar radiation, and water conditions also affect biomass allocation (Kage et al., 2004; Poorter et al., 2012; Steinfort et al., 2017; Lizaso et al., 2018). Thus, partitioning patterns between the shoots and roots change with the sowing date and environmental conditions (Justes et al., 2002; Bonelli et al., 2016). In durum wheat (Triticum turgidum), late sowing resulted in a higher grain protein concentration than normal sowing, partially compensating for the reduction in dry matter accumulation (Ferrise et al., 2010). For example, the delayed planting of maize (Zea mays) and soybean (Glycine max) limited their photosynthetic source capacities, resulting in low-level light interception, reduced translocation of photo-assimilates to different organs, and decreased yields (Bonelli et al., 2016). Small differences in arrival time influence composition and productivity of plant communities (Koerner et al., 2008).
Various plant organs indicate different physiological and ecological functions. Throughout the process of plant growth and reproduction, all the organs exhibit conspicuous allometric growth, and this is an inherent characteristic determined by species inheritance (Weiner, 2004; Huang et al., 2009). Viewed from an allometric perspective, allocation is regarded as a size-dependent process; allometry constitutes the quantitative relationship between growth and allocation. Therefore, most studies about allocation should be posed allometrically, rather than as mere ratios or proportions. Plants evolve allometric patterns in response to numerous selection pressures and constraints, elucidating various behaviors among plant populations (Weiner, 2004). As an important aspect of plant ecology research, allometric growth analysis serves as a crucial method for studying plant adaptation strategies. The inherent relationship between plant density, individual size, and traits can be systematically elucidated through allometric growth analysis. This approach facilitates the analysis of individual size and reveals the strategic adjustments that plants make in response to abiotic factors. Several studies have demonstrated that the allometric growth of plants is significantly influenced by their reproductive period, particularly in relation to the relationship between the reproductive organs and individual size (Gomez-Fernandez and Milla, 2022). Therefore, it is necessary to take into account the changes in reproductive periods. Furthermore, the results derived from the allometric growth analysis of a single sample lack rigor; multiple rounds of sampling should be conducted throughout the entire plant life cycle to reveal the allometric growth patterns of different plant organs (Weiner, 2004).
The modulation of plant plasticity in natural environments is significantly influenced by abiotic factors and growth stages (Wang and Zhou, 2022). The emergence time, a mechanism intricately linked to environmental conditions, is considered a precise determinant of habitat selection or niche construction, shaping the plant’s interaction with the environment (Donohue, 2003, 2005). Consequently, it plays a crucial role in seedling establishment and subsequent growth (Donohue, 2005; Wilczek et al., 2009; Burghardt et al., 2015), particularly in competitive environments (Kelly and Levin, 1997; Dyer et al., 2000; Seiwa, 2000).
Fagopyrum esculentum, an annual herb within the Polygonaceae family, exhibits distinctive features. Buckwheat is widely cultivated worldwide, including in countries such as China, Russia, and the United States, and can be biannual sown in some region and boasts rapid grow. Characterized by white flowers and an infinite inflorescence, it undergoes a 30 to 45-day transition from flowering to maturity. Seed harvesting occurs when the plant’s seeds reach 75% to 80% maturity, marked by a brown hue resulting from uneven maturation. The plant is sensitive to unfavorable environmental factors, especially to frost, extreme temperatures, and droughts (Cogoni et al., 2013). Due to its inherent tolerance to various abiotic stresses and a short life cycle, buckwheat has garnered attention as a model crop plant (Zargar et al., 2023). It is primarily cultivated for its seeds’ advantageous chemical composition, which includes high levels of starch, minerals, vitamins, rutin, antioxidants, and dietary fiber. Additionally, its unique amino acid composition distinguishes it as particularly abundant in lysine and arginine while being gluten-free (Plazek et al., 2023). Meanwhile, it has economic value because of its seed yield. For example, The demand for buckwheat, particularly organic buckwheat, has witnessed a rapid surge in recent years and is predominantly fulfilled through imports from non-EU countries, notably China. Buckwheat generally exhibits a modest grain yield. However, it has demonstrated promising potential for commercial cultivation in Europe with grain yields ranging from 1.2 to 3.0 t ha−1 (Domingos and Bilsborrow, 2021).
However, there remains a dearth of research on the effects of different sowing dates on the biomass allocation of various organs and the allometric growth of F. esculentum. We conducted a field experiment involving the sowing of F. esculentum on nine distinct dates, intentionally inducing delayed planting. Our objective was to examine the biomass allocation of different organs and allometric growth within natural environments. Specifically, we aimed to address the following questions: (1) What impact does delayed planting affect biomass allocation and accumulation? (2) How does the planting time influence the allometric growth of F. esculentum?
2 Materials and methods
2.1 Experimental design
This experiment was conducted in 2018 at the Changling Ecological Research Station for Grassland Farming (ERSGF), Chinese Academy of Sciences (44°33′N, 123°31′E, 145 m alt), in the southern part of the Songnen grassland of northeast China. This region is characterized by a dry and windy spring and a hot and wet summer, with an average annual air temperature of 4.6–6.4°C, and average annual precipitation and evaporation totals of 410 mm and 1000–2000 mm, respectively. The average air temperature peaks in July (>20°C) during the primary growth season (between June and August), and precipitation during this period contributes 70%– 80% of the total annual precipitation. The annal average sunshine duration is 2505.53 hours (Supplementary Table S1). The original soil in the experimental field (aeolian sandy soil; pH = 8.61 ± 0.05; EC (soil electric conductivity) = 0.26 ± 0.02) was low in nutrients (SOM (soil organic matter) = 3.04 ± 0.46 g kg−1; available N = 31.58 ± 3.08 mg kg-1; available P = 30.69 ± 1.51 mg kg-1; available K = 162.86 ± 11.49 mg kg-1) at the 0–10 cm soil depth during the growth season (Supplementary Table S2).
The variety we used is Jiqiao 10. It is a new buckwheat variety grown by mixed selection method. Its main advantages are high quality, high yield and stress resistance. Seeds of Jiqiao 10 were purchased from the ChunYu Seed Company (Jilin, China). The seeds were stored in dark at room temperature (18–20°C) before sowing.
A completely randomized design was implemented with four replicates, and the area of the individual plots totaled approximately 32 m2. (There were 7 ridges per plot; the ridge distance was 65 cm, and the ridge length was 7 m). In this experiment, we sowed the plants on the following nine dates: 12 April, 27 April, 12 May, 27 May, 11 June, 26 June, 11 July, 26 July, and 11 August (Table 1). Thus, there were 36 plots in total. In addition, the growing conditions, such as, accumulated temperature (°C), effective accumulated temperatures (°C), and the precipitation values (mm) were changed among the sowing treatments (Supplementary Table S3). In each plot, we sowed 941 g of seeds. The sowing dates of buckwheat is generally sown from late May to early June in Northern China. The amounts of actual NPK fertilizer applied in each plot were 22.5 kg ha-1, 3.86 kg ha-1, and 8.67 kg ha-1, respectively. All the plots were irrigated immediately after sowing, and also irrigated during the dry season in order to prevent drought stress. After seedling emergence, the seedlings were thinned by 1 million plants per hectare when they reached the four-leaf stage to ensure uniform initial density across all sowing treatments. Undesired weeds were controlled adequately through manual weeding at regular intervals.
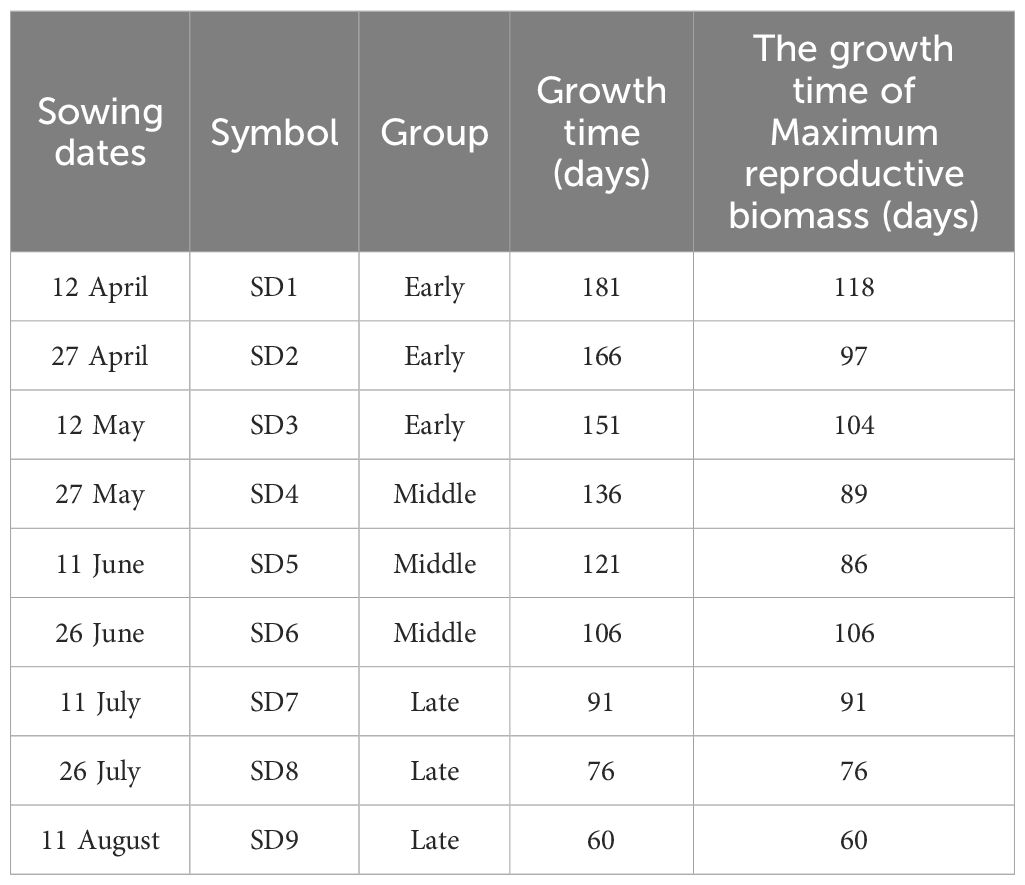
Table 1. Information about sowing dates, symbols, groups, growth times, and the growth of maximum reproductive biomass in F. esculentum.
2.2 Plant sampling and measurements
Plant biomass accumulation and allocation were measured at various sampling times. We selected eight plants in each plot with four replicates for each treatment from 11 June to 10 October. The plant organs were divided into five parts, including the root, stem, leaf, flower, and mature seed. The value of biomass per organ was obtained after oven drying the samples at 65°C for 48 h. The total biomass of the plant represents the sum of the biomass from five organs. The vegetative biomass represents the sum of the stem, leaf, and root biomass. The reproductive biomass represents the sum of the flower and mature seed biomass. The aboveground biomass represents the sum of stem, leaf, flower, and mature seed biomass. The belowground biomass represents the root biomass. The aboveground, belowground, reproductive, and vegetative biomass allocations were all obtained by dividing their respective biomass by the total biomass.
2.3 Data analysis
Firstly, the reproductive biomass accumulation was analyzed for all the sowing dates (SD1-SD9) throughout sampling (Supplementary Figure S1). Then, the maximum reproductive biomass of all the plants was selected to analyze the differences in biomass accumulation and allocation among various organs (vegetative organs and reproductive organs) of F. esculentum using one-way analysis of variance (ANOVA). We performed post hoc tests using LSD. According to the significant difference in the total biomasses when the maximum reproductive biomass was reached in each sowing period, the plants were divided into three groups (early, middle, and late sowing treatments) (Table 1 and Supplementary Table S4). Locally weighted regression was used to fit the variation in reproductive, vegetative, belowground, and aboveground biomass with the delay in the sowing date and growth time. Standardized major axis regression was used to analysis the dynamic and static allometric growth among the different biomass of the organs (reproductive biomass vs. vegetative biomass; belowground biomass vs. aboveground biomass) of F. esculentum using Smatr package in R Version 4.2.2 (R Core Team, 2022). Standardized major axis regression is widely used to estimate the relationship between two variables for line-fitting in allometry (Warton et al., 2006). The allometric relationship between X and Y was Y=bXa, where a was the allometric exponent (slope) and b was the allometric coefficient (constant) or “scaling factor” (Y intercept). After logarithmic transformation, the equation is logY = a+ blogX (Huang et al., 2009). The dynamic and static allometric exponents and constants of reproductive biomass vs. vegetative biomass and belowground biomass vs. aboveground biomass from the early to late sowing dates were analyzed. All the statistical analyses and plots were performed and drawn in R Version 4.2.2 (R Core Team, 2022).
3 Results
3.1 The effects of sowing dates on biomass accumulation and allocation
Firstly, the impact of the sowing date on the biomass accumulation of F. esculentum was examined. The sowing dates had a significant effect on the reproductive, vegetative, aboveground, and belowground biomass of F. esculentum, thereby impacting the maximum reproductive biomass (p < 0.001). Specifically, with the delay in the sowing date, the reproductive biomass of F. esculentum significantly decreased from early to middle sowing treatments. However, the reproductive biomass did not exhibit any statistically significant variation among late sowing, early sowing, and middle sowing (Figure 1A). The vegetative biomass of F. esculentum increased gradually with a delayed germination time (Figure 1B). Considering the reproductive and vegetative biomass allocation of F. esculentum on the maximum reproductive biomass, our findings demonstrate that the reproductive biomass allocation value remained 14%~16% for the early and middle sowing treatments, and then increased to 32% for the late sowing treatment (Figure 1C). When we compared the vegetative biomass allocation, we found that they remained at 70%-72% for the early and middle sowing treatments, and then decreased to 56% (SD7-SD9) (Figure 1D). Similarly, the allocation and accumulation of reproductive and vegetative biomass in all the sowing treatments (SD1-SD9) were in line with the above findings (Supplementary Tables S4, S5).
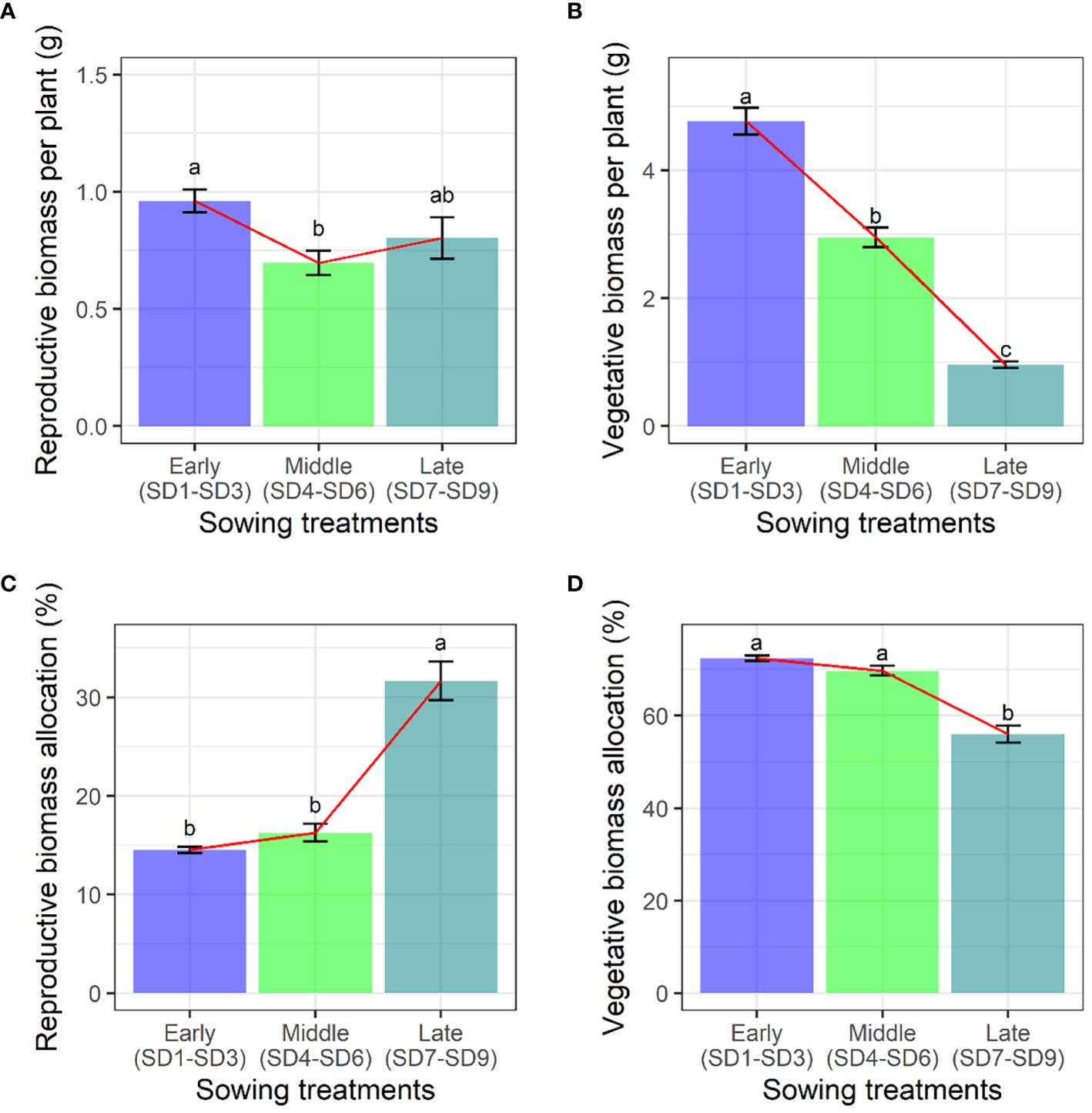
Figure 1. Effects of sowing treatments (early, middle, and late) on (A) reproductive biomass, (B) vegetative biomass, (C) reproductive biomass allocation, and (D) vegetative biomass allocation when F. esculentum reached the maximum reproductive biomass. Different lowercase letters represent significant differences among three sowing treatments at p<0.05.
When we compared the aboveground and belowground biomass of F. esculentum, we found that they decreased gradually with the delay in the sowing date (Figures 2A, B). However, the sowing treatments had no significant effect on biomass allocation (Figures 2C, D). With the delay of planting time (SD1-SD9), The aboveground biomass and belowground biomass were gradually decreased. However, the aboveground biomass and belowground biomass allocation were not significantly different among sowing treatments (SD1-SD9) (Supplementary Tables S4, S5).
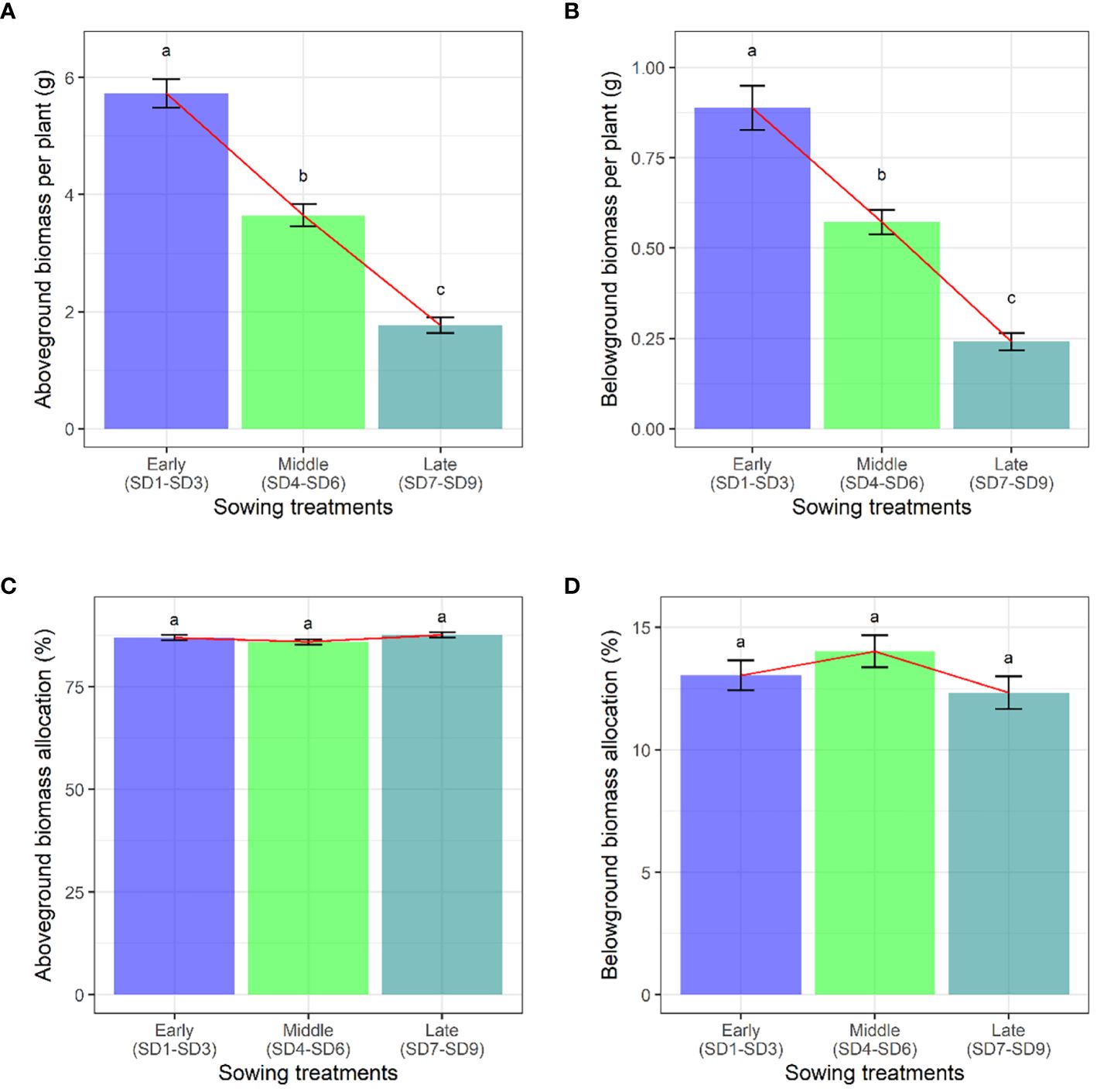
Figure 2. Effects of sowing treatments (early, middle, and late) on (A) aboveground biomass, (B) belowground biomass, (C) aboveground biomass allocation, and (D) belowground biomass allocation when F. esculentum reached the maximum reproductive biomass. Different lowercase letters represent significant differences among three sowing treatments at p<0.05.
We compared the reproductive, vegetative, aboveground, and belowground biomasses with the growth time among the early, middle, and late sowing groups. The early and middle sowing treatments caused an increased accumulation of vegetative and reproductive biomasses compared to that of the late sowing treatment (Figure 3), but the latter caused the plants to reach the maximum reproductive biomass in a shorter period of time, which was significantly higher than those of the middle and late sowing treatments (Figure 3A). In terms of the aboveground and belowground biomass, the early and middle sowing treatments caused an increased accumulation of biomass compared to that of the late sowing treatment (Figures 3B–D).
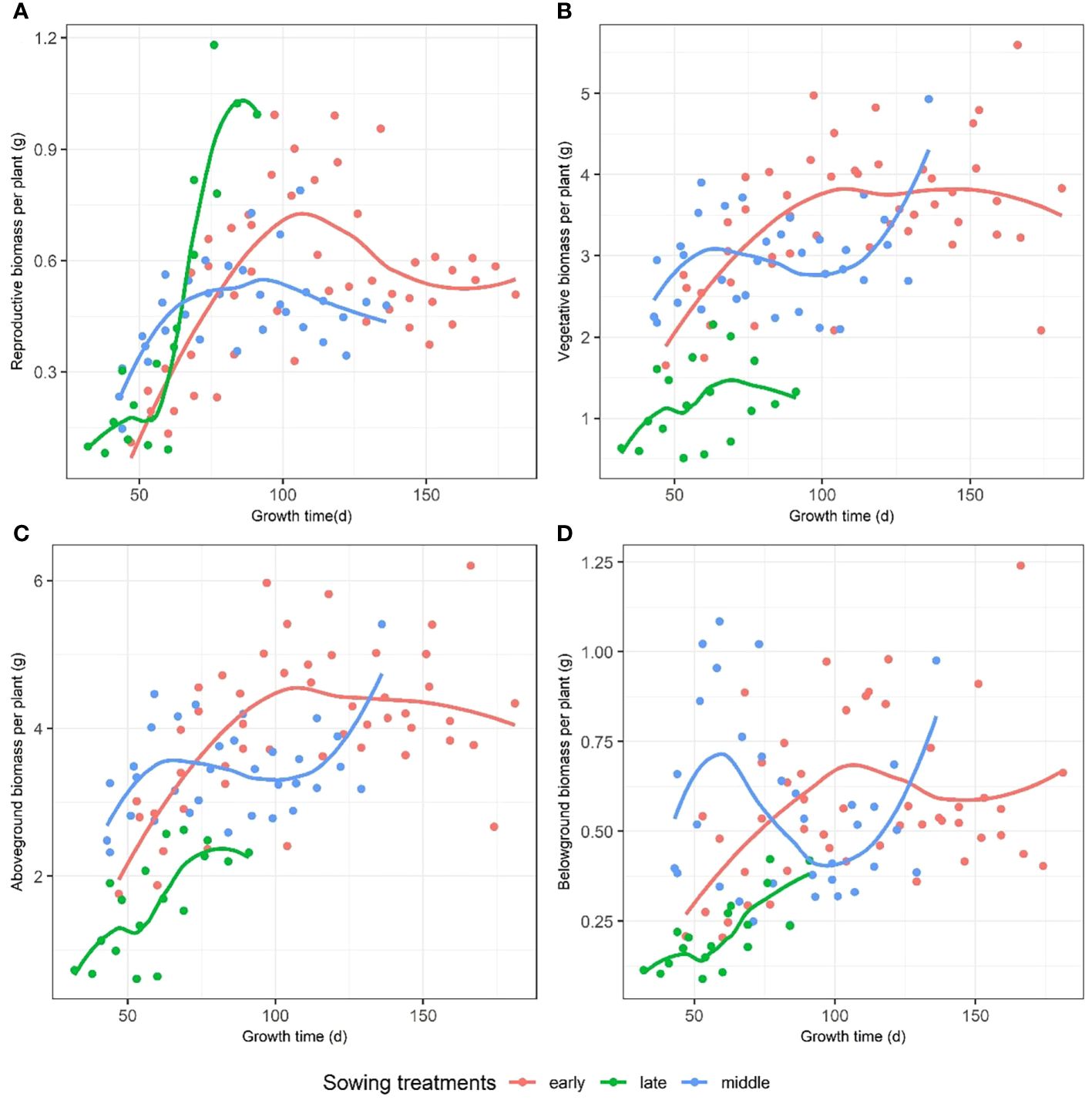
Figure 3. The dynamic changes in (A) reproductive, (B) vegetative, (C) aboveground, and (D) belowground biomasses with growth time among early, middle, and late sowing treatments on F. esculentum.
3.2 Allometric growth of different organs
By analyzing the allometric growth of the organ biomass of F. esculentum, we found a power function growth pattern among the different sowing dates. When the data transformed by log10, we can figure out the allometric relationship between reproductive biomass vs. vegetative biomass, and belowground biomass vs. aboveground biomass among the early, middle, and late sowing treatments (Figure 4). The allometric growth of the belowground vs. aboveground biomass and reproductive vs. vegetative biomass changed over time (p< 0.05). We found that the allometric exponent and constant were significantly changed by the sowing treatments (p < 0.05), of which the homogeneity of the slope and shifts in the intercept and along the common slope were significantly different among the sowing treatments (early, middle and late) (Supplementary Table S3). Sowing on different dates had a significant effect on the allometric exponent and constant and individual size of F. esculentum (p < 0.05) (Table 2).
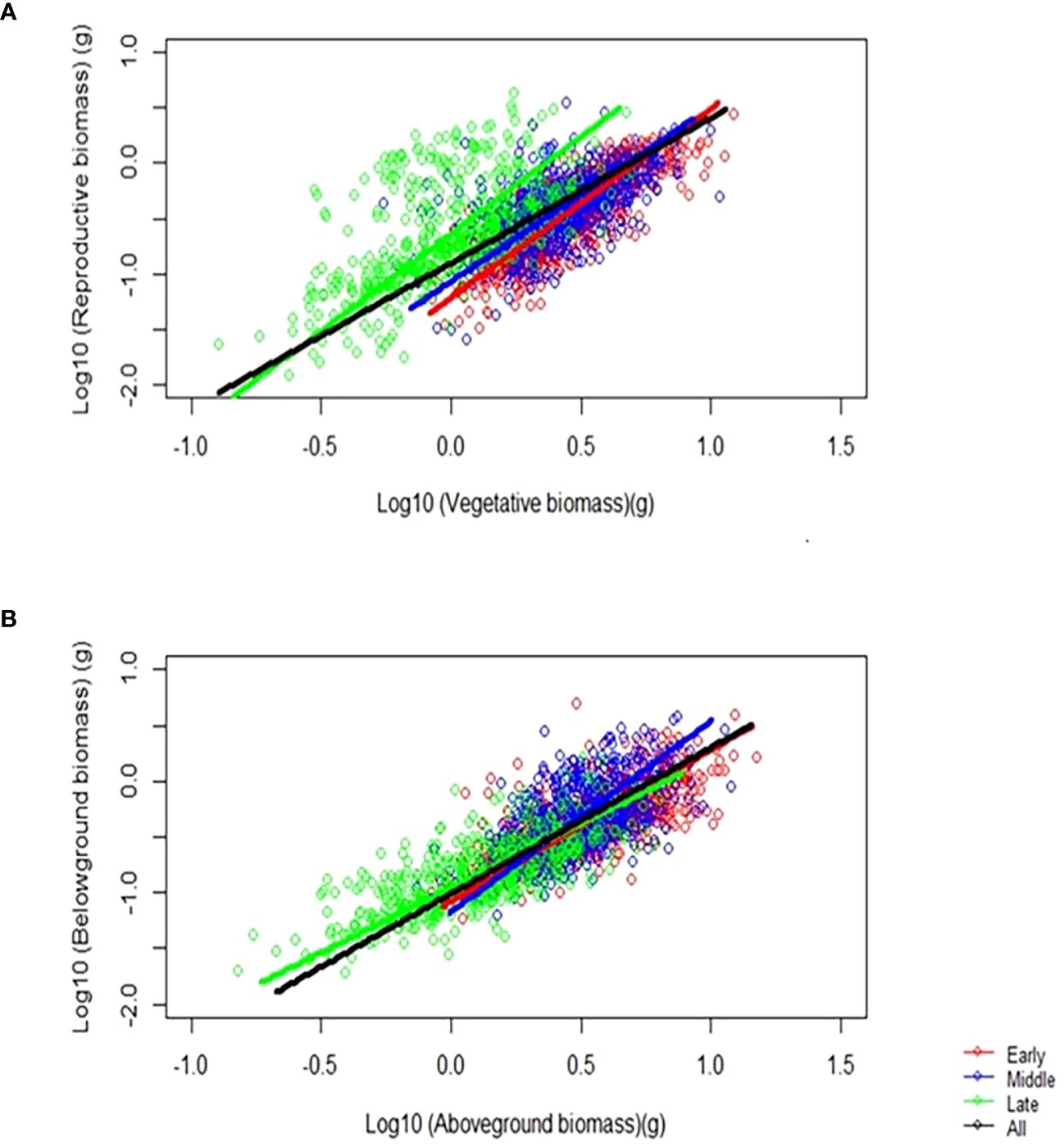
Figure 4. The effect of sowing dates on the (A) allometric relationship between reproductive and vegetative biomass, and (B) belowground and aboveground biomass among the early, middle, and late sowing treatments when the maximum reproductive biomass of F. esculentum was reached.
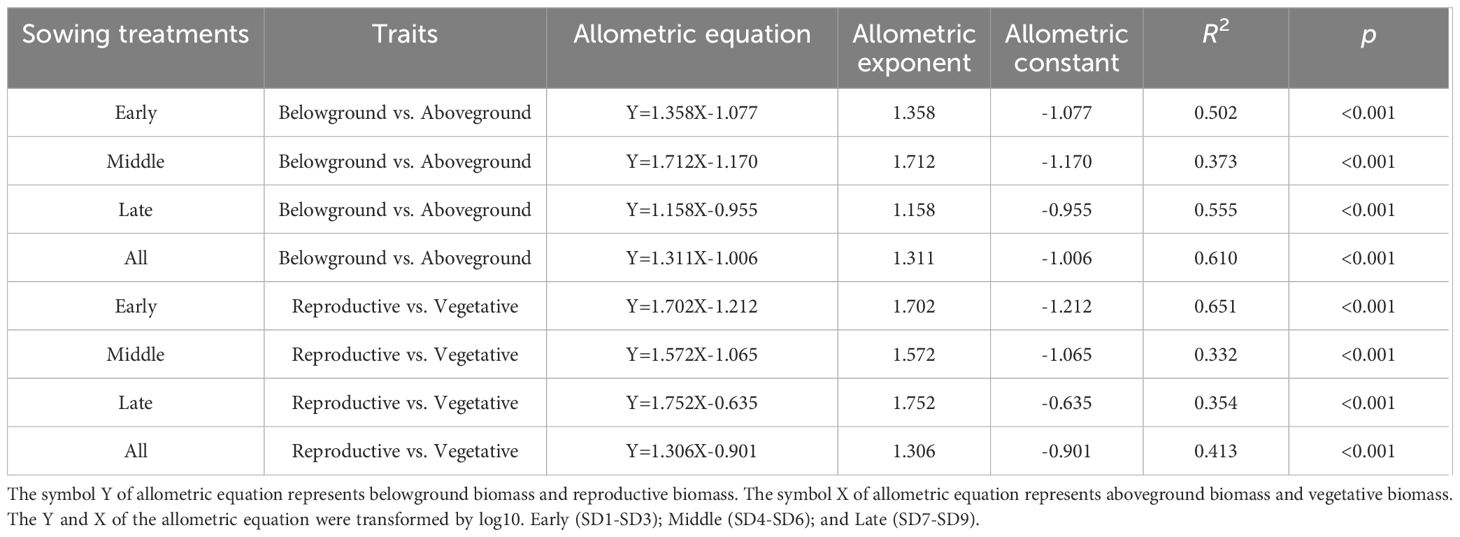
Table 2. The allometric exponent and constant of reproductive vs. vegetative biomass and below-ground vs. aboveground biomass of F. esculentum among the sowing dates.
We analyzed the allometric exponent and constant (dynamic and static) of the reproductive vs. vegetative biomass and belowground vs. aboveground biomass among the early, middle, and late sowing treatments. The dynamic allometric exponent and constant reflect the slope and intercept of the allometric growth equation between the sampling initiation and the period when plants reach their maximum reproductive biomass (reproductive biomass vs. vegetative biomass, belowground biomass vs. aboveground biomass).Static allometric exponent and constant reflect the slope and intercept of the allometric growth equation at the point when plants reach their maximum reproductive biomass (reproductive biomass vs. vegetative biomass, belowground biomass vs. aboveground biomass). With the delay in planting time, the dynamic allometric exponent of the reproductive vs. vegetative biomass first decreased from the early to middle sowing treatments, and then increased or the late sowing treatment (Figure 5A). However, the static allometric exponent of the reproductive vs. vegetative biomass increased gradually from the early to late sowing treatments (Figure 5B). As for the dynamic and static allometric exponents of the belowground vs. aboveground biomass, we found the former first increased, and then gradually decreased from the early to late sowing treatments; on the contrary, the static allometric exponent decreased gradually with the delay in planting time (Figures 6A, B). In general, the allometric exponents (dynamic and static) between the reproductive vs. vegetative biomass and belowground vs. aboveground biomass had an opposite tendency. It suggested that the trade-off between reproductive vs. vegetative and belowground vs. aboveground growth. The dynamic and static allometric constants decreased gradually with the delay in sowing time between the reproductive vs. vegetative biomass and belowground vs. aboveground biomass (Figures 5C, D, 6C, D).
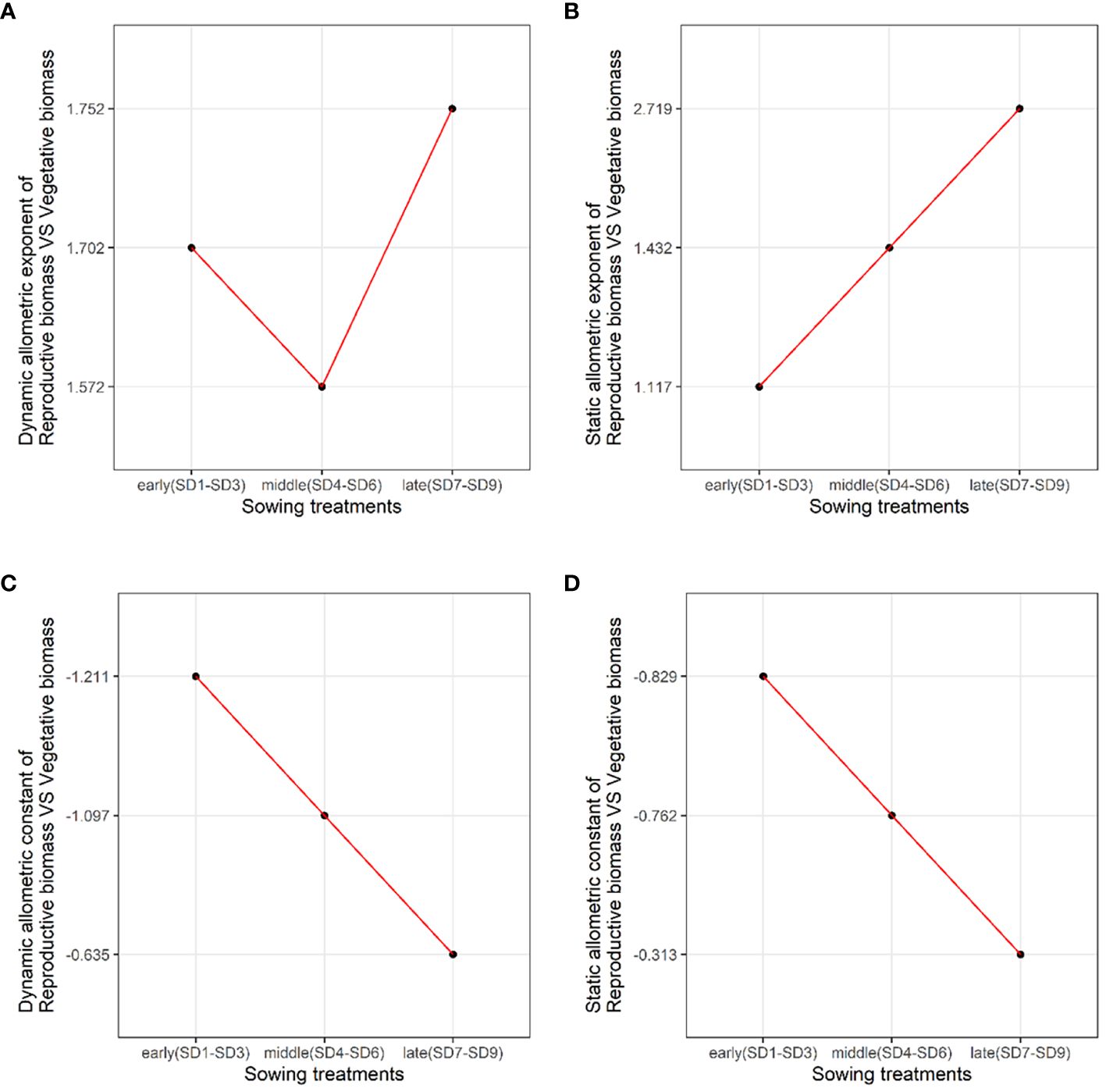
Figure 5. The changes in the dynamic (A) allometric exponent, (B) static allometric exponent, (C) dynamic allometric constant, and (D) static allometric constant of reproductive vs. vegetative biomass among the early, middle, and late sowing dates when the maximum reproductive biomass of F. esculentum was reached.
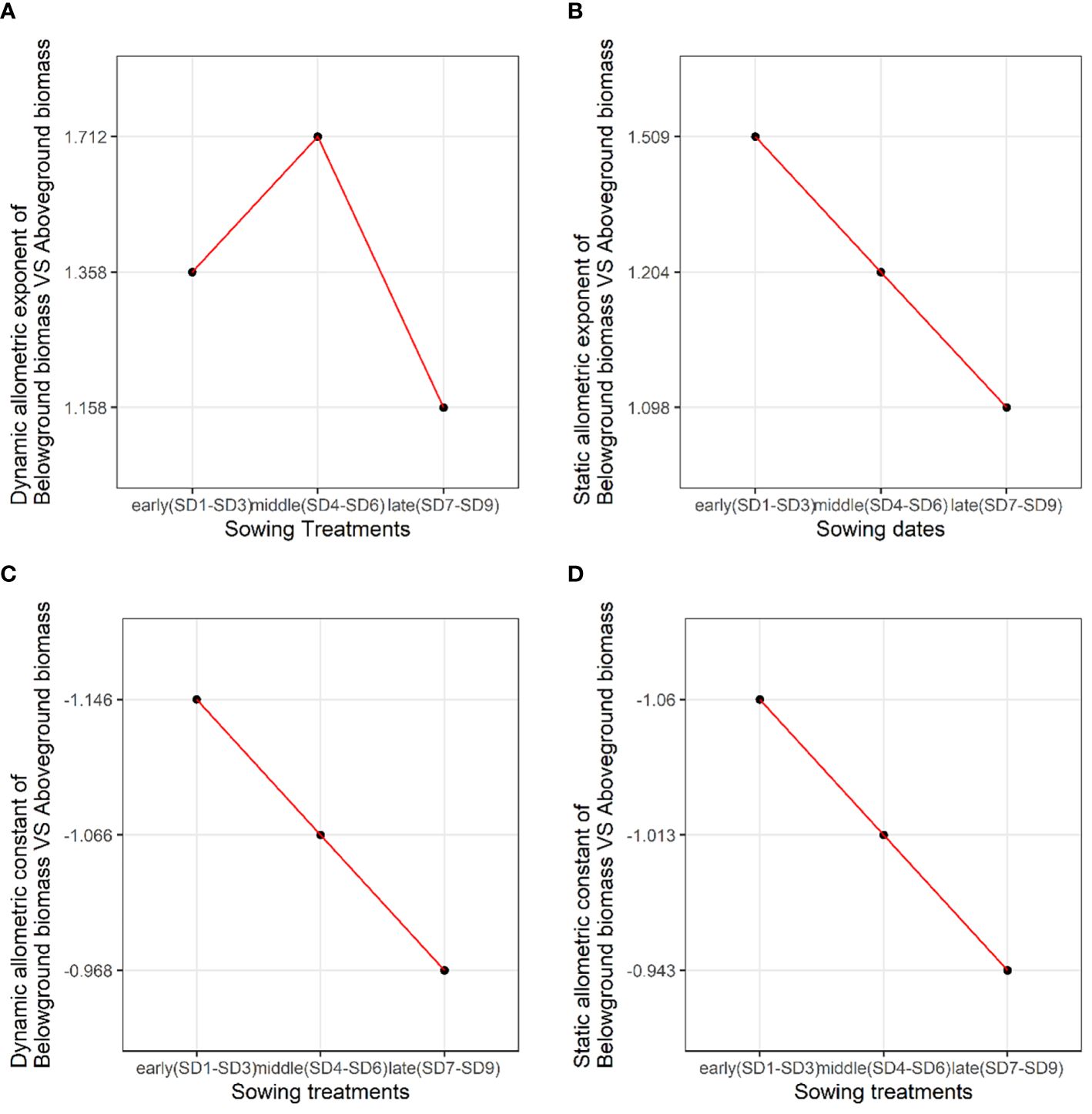
Figure 6. The changes in the (A) dynamic allometric exponent, (B) static allometric exponent, (C) dynamic allometric constant, and (D) static allometric constant of belowground vs. aboveground biomass among the early, middle, and late sowing dates when the maximum reproductive biomass of F. esculentum was reached.
4 Discussion
4.1 The effects of sowing dates on biomass accumulation and allocation
Buckwheat is an important economic crop with rich nutritional content in its seeds, possessing extremely high economic value. Understanding the pattern of resource allocation in buckwheat is the primary motivation for studying the changes in plant growth patterns. Environmental changes exert varying degrees of influence on the biomass allocation of different organs. Sowing on different dates produced a plastic phenological response, impacting the key life cycle stages, including the onset of reproduction (Zhou et al., 2005). Our findings reveal that early sowing tends to allocate more biomass to the vegetative organs, such as the leaves and stems (Figures 1, 2). This can be attributed to the survival strategy of the plant (Zhou et al., 2005). Conversely, late sowing of F. esculentum leads to a higher allocation of biomass to reproductive organs rather than vegetative ones, facilitating rapid growth. As the sowing date is delayed, the size (vegetative or aboveground biomass) of F. esculentum is gradually decreases. Biomass accumulation and allocation to different components also showed plastic variation based on sowing dates (Supplementary Tables S4, S5). Earlier sowing resulted in heavier root, stem, leaf, and reproductive tissues, providing ample time and resources for both vegetative and reproductive growth. Earlier-sown plants allocated more biomass to the roots and stems, while less was directed to the reproductive tissues and leaves compared with the later-germinating plants. Accumulation and partitioning of biomass in reproductive organs play crucial roles in plants’ fitness across diverse environments (Weiner, 1988; Hartnett, 1990; Sadras et al., 1997; Vega et al., 2000). The contrasting reproductive patterns between the early and late germinating plants indicate varied fitness priorities in the different environments. In unfavorable conditions, a shorter life cycle, flowering earlier, and enhanced reproductive allocation confer advantages, while in favorable conditions, plants tend to have a longer lifespan, delayed flowering, and extended vegetative growth with a great reproductive output to enhance fitness (Sultan, 2000). The greater investment of resources in reproduction during a short life cycle is characteristic of annual weeds, where fewer resources are allocated to the supporting structures like roots and stems. This flexible partitioning appears to be typical behavior observed in annual plants (Hermanutz and Weaver, 1996; Cheplick, 2001). Wang and Zhou (2022) conducted an experiment on the effect of emergence time and population density on the dynamic reproductive plasticity of Abutilon theophrasti, and found that both advanced and delayed emergence resulted in less vegetative growth and earlier reproduction (Wang and Zhou, 2022). Moreover, environmental factors may affect the optimal relative allocation for survival, growth, and reproduction, shaping cost functions for individual fitness components (Sletvold and Agren, 2015). F. esculentum, being a short-day plants, exhibits photoperiodic control as the primary environmental cue for the flowering in short-lived herbs (Rathcke and Lacey, 1985; Klinkhamer et al., 1987; Kudoh et al., 1995). Vegetative growth switches to reproductive growth with decreasing day-length (Huang et al., 2000). With the delay in sowing, the day length gradually became shorter, so F. esculentum reproduced earlier. This plant demonstrates the ability to regulate its life cycle in response to environmental changes. A plant’s ability to reproduce early can be considered as an opportunity to successfully complete its life history in unfavorable conditions, as is the case with agricultural weeds that can set seeds before crop harvesting.
However, our study revealed that the reproductive biomass does not exhibit a negative linear relationship with the size of individuals, challenging previous findings suggesting a decrease in reproductive biomass with size (Weiner et al., 2009). Previous study has shown that the populations of F. esculentum sown at higher densities supported significantly more biomass per unit volume for a given canopy height (Li et al., 2013). Unlike prior research indicating size-dependent reproductive biomass reduction, our results demonstrated that reproductive biomass is not size-independent. As sowing date is delayed, total biomass gradually decreased, however, initially decreases from early to mid-sowing dates and subsequently increases from mid to late sowing dates. Research has shown that the relationship between plant size and reproductive output is pivotal for a plant’s strategy in converting growth into fitness (Weiner et al., 2009). They propose that a plant, with specific resource amounts at any given time, allocates these resources to different tissues. Reproduction is commonly gauged by the reproductive biomass divided by total biomass, assuming the reproductive effort ratio is size-independent, but our findings indicate a size-dependent reproductive allocation. Furthermore, the plant size is determined by numerous interacting factors (Li et al., 2015). Several studies assert that the growth duration influences plant development, and the growth dynamics are highly functionally coordinated with the plant size (Gomez-Fernandez and Milla, 2022). Our results suggest that the sowing treatments influence the maximum reproductive biomass. Reproductive, vegetative, aboveground, and belowground biomasses changed over time. However, the impact of sowing treatments on F. esculentum biomass varied over time (Figure 3). When categorizing sowing dates into early, middle, and late treatments, the reproductive biomass of F. esculentum initially increased, and then gradually decreased over time for early and mid-stage seeding. Conversely, for the plants sown late, reproductive biomass initially increased, and then decreased over time, with their maximum reproductive biomass substantially higher than that of the plants sown at the early and middle (Figure 3).
4.2 The effects of sowing dates on allometric growth
Allometric growth analysis, as a crucial method in plant ecology research, facilitates the systematic elucidation of the inherent relationship between plant density, individual size, and traits. It enables the analysis of individual size and reveals the strategic adjustments made by plants make in response to biotic and abiotic factors. Our study revealed that sowing F. esculentum at different times significant influenced the allometric trend, altering the allometric exponent and constant (Figures 5, 6). Similarly, previous study has found that the allometric analysis of reproductive and vegetative biomass across treatments showed an increase in reproductive effort with varying planting densities, but decrease when the plant were sown on different dates. The allometric exponent between the treatments varied significantly with the sowing date (Wang et al., 2006). However, our study has demonstrated opposite tendencies in the dynamic and static allometric exponents between the reproductive vs. vegetative biomass and belowground vs. aboveground biomass (Figures 5, 6). This suggests that the sowing treatments experience trade-offs between reproductive vs. vegetative biomass and belowground vs. aboveground biomass. Although the dynamic allometric exponent of reproductive vs. vegetative biomass was the highest among the sowing treatments, that of the belowground vs. aboveground biomass was the lowest. In summary, analyzing the allometric relationship allows us to discern resource partitioning in a plant sown late.
The size and yield of plants are intricately linked to their ontogeny, particularly the growth dynamics (Gomez-Fernandez and Milla, 2022). Our data collection spanned from the reproduction to the end of growth stages, overlooking the periods from emergence to reproduction. To comprehensively explore the effects of sowing on different dates, future studies should consider the growth dynamics throughout the plant’s entire life history. Meanwhile, we designed a field experiment to determine the suitable sowing dates in the northeast area of China. However, our study still has some limitations; we did not study F. esculentum throughout its whole life history.
5 Conclusions
F. esculentum, an annual herb within the Polygonaceae family, exhibits distinctive features. Buckwheat is widely cultivated worldwide, including in countries such as China, Russia, and the United States, and can be biannual sown and boasts rapid grow. Our results demonstrate that the biomass allocation and allometric growth (reproductive biomass vs. vegetative biomass, belowground biomass vs. aboveground biomass) of F. esculentum are significantly affected by the sowing date. Delayed sowing leads to an increase in the reproductive biomass allocation of F. esculentum. Furthermore, delayed planting had significant effects on the allometric exponent, constant, and individual size of F. esculentum. In general, the allometric exponents (dynamic and static) between the reproductive vs. vegetative biomass and belowground vs. aboveground biomass exhibit an opposite tendency. Our study reveals that the reproductive biomass does not exhibit a negative linear relationship with the size of the individuals. In summary, our results contribute to a more comprehensive understanding of the effect of sowing dates on plants’ reproductive biomass, and highlight the role of emergence time among mature plants with diverse sizes and reproductive biomass. It is crucial to extend study on this plants’ adaptations from emergence to maturity across various environments.
Data availability statement
The original contributions presented in the study are included in the article/Supplementary Material. Further inquiries can be directed to the corresponding author.
Author contributions
HW: Data curation, Formal analysis, Investigation, Writing – original draft, Writing – review & editing. CW: Formal analysis, Methodology, Writing – review & editing. GF: Investigation, Methodology, Writing – review & editing. CF: Conceptualization, Methodology, Writing – review & editing. YH: Conceptualization, Funding acquisition, Resources, Writing – review & editing. XL: Writing – review & editing. SW: Writing – review & editing. KW: Writing – review & editing.
Funding
The author(s) declare financial support was received for the research, authorship, and/or publication of this article. This study was funded by the Strategic Priority Research Program of the Chinese Academy of Sciences (XDA28110201) and Major Science and Technology Programs in Jilin Province (20230303008SF).
Conflict of interest
The authors declare that the research was conducted in the absence of any commercial or financial relationships that could be construed as a potential conflict of interest.
Publisher’s note
All claims expressed in this article are solely those of the authors and do not necessarily represent those of their affiliated organizations, or those of the publisher, the editors and the reviewers. Any product that may be evaluated in this article, or claim that may be made by its manufacturer, is not guaranteed or endorsed by the publisher.
Supplementary material
The Supplementary Material for this article can be found online at: https://www.frontiersin.org/articles/10.3389/fpls.2024.1399155/full#supplementary-material
References
Bonelli, L. E., Monzon, J. P., Cerrudo, A., Rizzalli, R. H., Andrade, F. H. (2016). Maize grain yield components and source-sink relationship as affected by the delay in sowing date. Field Crops Res. 198, 215–225. doi: 10.1016/j.fcr.2016.09.003
Burghardt, L. T., Metcalf, C. J. E., Wilczek, A. M., Schmitt, J., Donohue, K. (2015). Modeling the influence of genetic and environmental variation on the expression of plant life cycles across landscapes. Am. Nat. 185, 212–227. doi: 10.1086/679439
Cheplick, G. P. (2001). Quantitative genetics of mass allocation and the allometry of reproduction in Amaranthus albus:: Relation to soil nutrients. Int. J. Plant Sci. 162, 807–816. doi: 10.1086/320778
Cogoni, D., Fenu, G., Bacchetta, G. (2013). Effects of timing of emergence and microhabitat conditions on the seedling performance of a coastal Mediterranean plant. Ecoscience 20, 131–136. doi: 10.2980/20-2-3583
Domingos, I. F. N., Bilsborrow, P. E. (2021). The effect of variety and sowing date on the growth, development, yield and quality of common buckwheat (Fagopyrum esculentum Moench). Eur. J. Agron. 126, 126264. doi: 10.1016/j.eja.2021.126264
Donohue, K. (2003). Setting the stage: Phenotypic plasticity as habitat selection. Int. J. Plant Sci. 164, S79–S92. doi: 10.1086/368397
Donohue, K. (2005). Niche construction through phenological plasticity: life history dynamics and ecological consequences. New Phytol. 166, 83–92. doi: 10.1111/j.1469-8137.2005.01357.x
Dyer, A. R., Fenech, A., Rice, K. J. (2000). Accelerated seedling emergence in interspecific competitive neighbourhoods. Ecol. Lett. 3, 523–529. doi: 10.1111/j.1461-0248.2000.00187.x
Ferrise, R., Triossi, A., Stratonovitch, P., Bindi, M., Martre, P. (2010). Sowing date and nitrogen fertilisation effects on dry matter and nitrogen dynamics for durum wheat: An experimental and simulation study. Field Crops Res. 117, 245–257. doi: 10.1016/j.fcr.2010.03.010
Gomez-Fernandez, A., Milla, R. (2022). How seeds and growth dynamics influence plant size and yield: Integrating trait relationships into ontogeny. J. Ecol. 110, 2684–2700. doi: 10.1111/1365-2745.13979
Hartnett, D. C. (1990). Size-dependent allocation to sexual and vegetative reproduction in 4 clonal composites. Oecologia 84, 254–259. doi: 10.1007/BF00318281
Heilmeier, H. (2019). Functional traits explaining plant responses to past and future climate changes. Flora 254, 1–11. doi: 10.1016/j.flora.2019.04.004
Hermanutz, L. A., Weaver, S. E. (1996). Agroecotypes or phenotypic plasticity? Comparison of agrestal and ruderal populations of the weed Solanum ptycanthum. Oecologia 105, 271–280. doi: 10.1007/BF00328557
Huang, J. Z., Shrestha, A., Tollenaar, M., Deen, W., Rahimian, H., Swanton, C. J. (2000). Effects of photoperiod on the phenological development of redroot pigweed (Amaranthus retroflexus L.). Can. J. Plant Sci. 80, 929–938. doi: 10.4141/P99-134
Huang, Y., Zhao, X., Zhang, H., Japhet, W., Zuo, X., Luo, Y., et al. (2009). Allometric effects of agriophyllum squarrosum in response to soil nutrients, water, and population density in the horqin sandy land of China. J. Plant Biol. 52, 210–219. doi: 10.1007/s12374-009-9027-9
Justes, E., Thiébeau, P., Avice, J. C., Lemaire, G., Volenec, J. J., Ourry, A. (2002). Influence of summer sowing dates, N fertilization and irrigation on autumn VSP accumulation and dynamics of spring regrowth in alfalfa (Medicago sativa L.). J. Exp. Bot. 53, 111–121. doi: 10.1093/jexbot/53.366.111
Kage, H., Kochler, M., Stützel, H. (2004). Root growth and dry matter partitioning of cauliflower under drought stress conditions: measurement and simulation. Eur J Agron. 20, 379–394. doi: 10.1016/s1161-0301(03)00061-3
Kelly, M. G., Levin, D. A. (1997). Fitness consequences and heritability aspects of emergence date in Phlox drummondii. J. Ecol. 85, 755–766. doi: 10.2307/2960599
Klinkhamer, P. G. L., Dejong, T. J., Meelis, E. (1987). Life-history variation and the control of flowering in short-lived monocarps. Oikos 49, 309–314. doi: 10.2307/3565766
Koerner, C., Stoecklin, J., Reuther-Thiebaud, L., Pelaez-Riedl, S. (2008). Small differences in arrival time influence composition and productivity of plant communities. New Phytologist 177, 698–705. doi: 10.1111/j.1469-8137.2007.02287.x
Kudoh, H., Ishiguri, Y., Kawano, S. (1995). Phenotypic plasticity in Cardamine flexuosa - variation among populations in plastic response to chilling treatments and photoperiods. Oecologia 103, 148–156. doi: 10.1007/BF00329074
Kumar, R., Sarawgi, A. K., Ramos, C., Amarante, S. T., Ismail, A. M., Wade, L. J. (2006). Partitioning of dry matter during drought stress in rainfed lowland rice. Field Crops Res. 98, 1–11. doi: 10.1016/j.fcr.2005.09.015
Li, H. Y., Lindquist, J. L., Yang, Y. F. (2015). Effects of sowing date on phenotypic plasticity of fitness-related traits in two annual weeds on the Songnen plain of China. PloS One 10, e0127795. doi: 10.1371/journal.pone.0127795
Li, L., Weiner, J., Zhou, D., Huang, Y., Sheng, L. (2013). Initial density affects biomass-density and allometric relationships in self-thinning populations of Fagopyrum esculentum. J. Ecol. 101, 475–483. doi: 10.1111/1365-2745.12039
Lizaso, J. I., Ruiz-Rarnos, M., Rodríguez, L., Gabaldon-Leal, C., Oliveira, J. A., Lorite, I. J., et al. (2018). Impact of high temperatures in maize: Phenology and yield components. Field Crops Res. 216, 129–140. doi: 10.1016/j.fcr.2017.11.013
McCarthy, M. C., Enquist, B. J. (2007). Consistency between an allometric approach and optimal partitioning theory in global patterns of plant biomass allocation. Funct. Ecol. 21, 713–720. doi: 10.1111/j.1365-2435.2007.01276.x
McConnaughay, K. D. M., Coleman, J. S. (1999). Biomass allocation in plants: Ontogeny or optimality? A test along three resource gradients. Ecology 80, 2581–2593. doi: 10.1890/0012-9658(1999)080[2581:BAIPOO]2.0.CO;2
Myers-Smith, I. H., Thomas, H. J. D., Bjorkman, A. D. (2019). Plant traits inform predictions of tundra responses to global change. New Phytol. 221, 1742–1748. doi: 10.1111/nph.15592
Niklas, K. J. (1994). Plant allometry. The scaling of form and process (Chicago, IL, USA: The University of Chicago Press).
Piao, S. L., Liu, Q., Chen, A. P., Janssens, I. A., Fu, Y. S., Dai, J. H., et al. (2019). Plant phenology and global climate change: Current progresses and challenges. Global Change Biol. 25, 1922–1940. doi: 10.1111/gcb.14619
Plazek, A., Kopec, P., Dziurka, M., Slomka, A. (2023). The yield of common buckwheat (Fagopyrum esculentum Moench) depends on the genotype but not on the Pin-to-Thrum ratio. Sci. Rep. 13, 16022. doi: 10.1038/s41598–023-43059–0
Poorter, H., Niklas, K. J., Reich, P. B., Oleksyn, J., Poot, P., Mommer, L. (2012). Biomass allocation to leaves, stems and roots: meta-analyses of interspecific variation and environmental control. New Phytol. 193, 30–50. doi: 10.1111/j.1469-8137.2011.03952.x
Rathcke, B., Lacey, E. P. (1985). Phenological patterns of terrestrial plants. Annu. Rev. Ecol. Systematics 16, 179–214. doi: 10.1146/annurev.es.16.110185.001143
R Core Team. (2022). R: A language and environment for statistical computing (Vienna: R Foundation for Statistical Computing). Available at: https://www.R-project.org/.
Reich, P. (2002). “Root–shoot relations: optimality in acclimation and adaptation or the ‘‘Emperor’s new clothes’’?,” in Plant roots, the hidden half, 3rd edn. Eds. Waisel, Y., Eshel, A., Kafkafi, U. (Marcel Dekker, New York, NY, USA).
Sadras, V. O., Bange, M. P., Milroy, S. P. (1997). Reproductive allocation of cotton in response to plant and environmental factors. Ann. Bot. 80, 75–81. doi: 10.1006/anbo.1997.0402
Seiwa, K. (2000). Effects of seed size and emergence time on tree seedling establishment: importance of developmental constraints. Oecologia 123, 208–215. doi: 10.1007/s004420051007
Shipley, B., Meziane, D. (2002). The balanced-growth hypothesis and the allometry of leaf and root biomass allocation. Funct. Ecol. 16, 326–331. doi: 10.1046/j.1365-2435.2002.00626.x
Sletvold, N., Agren, J. (2015). Nonlinear costs of reproduction in a long-lived plant. J. Ecol. 103, 1205–1213. doi: 10.1111/1365-2745.12430
Steinfort, U., Trevaskis, B., Shu, F. K., Bell, K. L., Dreccer, M. F. (2017). Vernalisation and photoperiod sensitivity in wheat: Impact on canopy development and yield components. Field Crops Res. 201, 108–121. doi: 10.1016/j.fcr.2016.10.012
Sultan, S. E. (2000). Phenotypic plasticity for plant development, function and life history. Trends Plant Sci. 5, 537–542. doi: 10.1016/S1360-1385(00)01797-0
Vega, C. R. C., Sadras, V. O., Andrade, F. H., Uhart, S. A. (2000). Reproductive allometry in soybean, maize and sunflower. Ann. Bot. 85, 461–468. doi: 10.1006/anbo.1999.1084
Wang, S., Zhou, D. W. (2022). Dynamic reproductive plasticity in response to emergence time and population density in Abutilon theophrasti (Malvaceae). Braz. J. Bot. 45, 1057–1066. doi: 10.1007/s40415-022-00814-3
Wang, T. H., Zhou, D. W., Wang, P., Zhang, H. X. (2006). Size-dependent reproductive effort in Amaranthus retroflexus:: the influence of planting density and sowing date. Can. J. Botany-Revue Can. Botanique 84, 485–492. doi: 10.1139/b06-011
Warton, D. I., Wright, I. J., Falster, D. S., Westoby, M. (2006). Bivariate line-fitting methods for allometry. Biol. Rev. 81, 259–291. doi: 10.1017/S1464793106007007
Weiner, J. (1988). “The influence of competition on plant reproduction,” in Plant reproductive ecology: patterns and strategies (Oxford University Press, New York).
Weiner, J. (2004). Allocation, plasticity and allometry in plants. Perspect. Plant Ecol. Evol. Systematics 6, 207–215. doi: 10.1078/1433-8319-00083
Weiner, J., Campbell, L. G., Pino, J., Echarte, L. (2009). The allometry of reproduction within plant populations. J. Ecol. 97, 1220–1233. doi: 10.1111/j.1365-2745.2009.01559.x
Wilczek, A. M., Roe, J. L., Knapp, M. C., Cooper, M. D., Lopez-Gallego, C., Martin, L. J., et al. (2009). Effects of genetic perturbation on seasonal life history plasticity. Science 323, 930–934. doi: 10.1126/science.1165826
Zargar, S. M., Hami, A., Manzoor, M., Mir, R. A., Mahajan, R., Bhat, K. A., et al. (2023). Buckwheat OMICS: present status and future prospects. Crit. Rev. Biotechnol, 1–18. doi: 10.1080/07388551.2023.2229511
Keywords: sowing dates, reproduction, biomass allocation, allometric growth, Fagopyrum esculentum
Citation: Wang H, Wang C, Fan G, Fu C, Huang Y, Liu X, Wang S and Wang K (2024) Effects of different sowing dates on biomass allocation of various organs and allometric growth of Fagopyrum esculentum. Front. Plant Sci. 15:1399155. doi: 10.3389/fpls.2024.1399155
Received: 12 March 2024; Accepted: 24 May 2024;
Published: 07 June 2024.
Edited by:
Alexander Betekhtin, University of Silesia in Katowice, PolandReviewed by:
Aneta Słomka, Jagiellonian University, PolandAgnieszka Płażek, University of Agriculture in Krakow, Poland
Copyright © 2024 Wang, Wang, Fan, Fu, Huang, Liu, Wang and Wang. This is an open-access article distributed under the terms of the Creative Commons Attribution License (CC BY). The use, distribution or reproduction in other forums is permitted, provided the original author(s) and the copyright owner(s) are credited and that the original publication in this journal is cited, in accordance with accepted academic practice. No use, distribution or reproduction is permitted which does not comply with these terms.
*Correspondence: Yingxin Huang, aHVhbmd5eEBpZ2EuYWMuY24=