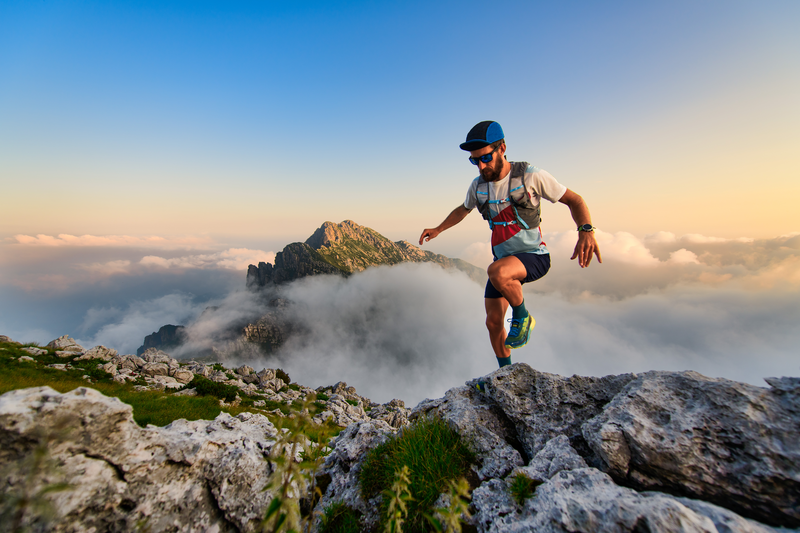
94% of researchers rate our articles as excellent or good
Learn more about the work of our research integrity team to safeguard the quality of each article we publish.
Find out more
ORIGINAL RESEARCH article
Front. Plant Sci. , 08 May 2024
Sec. Plant Pathogen Interactions
Volume 15 - 2024 | https://doi.org/10.3389/fpls.2024.1398014
This article is part of the Research Topic The Use of Microbial Volatile Compounds for Controlling Plant Pathogens View all 8 articles
Volatile organic compounds (VOCs) are responsible for the antagonistic activity exerted by different biological control agents (BCAs). In this study, VOCs produced by Pseudomonas synxantha strain 117-2b were tested against two kiwifruit fungal postharvest pathogens: Cadophora luteo-olivacea and Botrytis cinerea, through in vitro and in vivo assays. In vitro results demonstrated that P. synxantha 117-2b VOCs inhibit mycelial growth of C. luteo-olivacea and B. cinerea by 56% and 42.8% after 14 and 5 days of exposition, respectively. In vivo assay demonstrated significant inhibitory effects. VOCs used as a biofumigant treatment reduced skin-pitting symptoms disease severity by 28.5% and gray mold incidence by 66.6%, with respect to the untreated control. BCA volatiles were analyzed by solid-phase microextraction coupled with gas chromatography–mass spectrometry (SPME–GC/MS), and among the detected compounds, 1-butanol, 3-methyl and 1-nonene resulted as the most produced. Their efficacy as pure synthetic compounds was assayed against mycelial growth of fungal pathogens by different concentrations (0.34, 0.56, and 1.12 µL mL−1 headspace). The effect of the application of VOCs as a biofumigant was also investigated as the expression level of seven defense-related genes of kiwifruit at different exposition times. The results indicated an enhancement of the expression of almost all the genes starting from 3 h of treatment. These results described P. synxantha VOCs characteristics and their potential as a promising method to adopt for protecting kiwifruit from postharvest diseases caused by C. luteo-olivacea and B. cinerea.
In recent years, new strategies have been considered for postharvest fruit management in line with the new European Plant Health Regulation (EU 2016/2031) and jointly with the European Green Deal policy that aim to accelerate the transition to more sustainable systems of food production, reduce by 50% the use of pesticides, and increase by 25% the land areas used for organic farming by 2030. Because of all these health concerns, biopesticides based on antagonistic microorganisms have been discovered and firstly tested under controlled conditions, and subsequently in commercial and semi-commercial settings (Droby et al., 2016; Droby and Wisniewski, 2018; Teixidó et al., 2022).
Numerous biological control agents (BCAs), particularly bacteria belonging to Pseudomonas spp. and Bacillus spp., are able to produce volatile metabolites known for their antifungal efficacy (Gotor-Vila et al., 2017; Toffano et al., 2017). These metabolites, commonly known as volatile organic compounds (VOCs), can act directly or indirectly against fungal pathogen development (Toral et al., 2021).
The strain P. synxantha 117-2b has already shown a great ability to produce secondary metabolites (Di Francesco et al., 2023) and, for this reason, has been studied and tested in the present work as an alternative method to control kiwifruit postharvest diseases. Usually, microbial VOCs are alcohols, alkanes, acids, or ketones, characterized by their low molecular weight (Calvo et al., 2020). Volatile metabolites are produced by microorganism biosynthetic pathways as integral components of secondary metabolism (Che et al., 2017). VOCs could be potentially used with success as gaseous fruit postharvest treatment in a process defined as “biofumigation” (Di Francesco et al., 2015).
Kiwifruit can be stored up to 5 months at 0°C and a relative humidity (RH) of 92%–95% at normal refrigeration (NR) or for an extended period in controlled atmosphere (CA) (Di Francesco et al., 2018). During the storage period, fungal infections are a major problem for kiwifruit, causing serious economic losses (Niu et al., 2023). Botrytis cinerea is the causal agent of kiwifruit gray mold (Li Z. X. et al., 2023), identified as one of the primary causes of fruit losses (almost up to 30%) mainly during the export phase (Hyun et al., 2022). Conversely, Cadophora luteo-olivacea, a re-emerging fungal pathogen associated with kiwifruit skin-pitting symptom (Di Francesco et al., 2023) or side rot of pears (Wenneker et al., 2016) and apples (Köhl et al., 2018), is considered less harmful because of its latent behavior and sporadic emergence. However, the pathogen can infect fruits during the developmental stage, remaining latent during harvest, and appearing after 3–4 months of cold storage causing important economic repercussions for fruit postharvest industry (Di Francesco et al., 2022).
Concerning all these considerations, the present study has the aim to (i) evaluate Pseudomonas synxantha strain 117-2b VOCs’ efficacy against kiwifruit postharvest fungal pathogens, B. cinerea and C. luteo-olivacea, by in vitro and in vivo assays; (ii) biochemically characterize bacteria VOCs by using headspace solid-phase microextraction coupled with gas chromatography–mass spectrometry (SPME–GC/MS) and verify the effect of single synthetic pure compounds on the mycelial growth of the target pathogens; and (iii) check the effect of fruit biofumigation with P. synxantha VOCs, combined with curing, on the expression of kiwifruit defense-related genes.
Kiwifruits cv “Hayward” [Actinidia deliciosa (A. Chev.) C.F. Liang & A. R. Ferguson] were harvested at commercial maturity (7.2° Brix) from an orchard under integrated pest management (IPM) production system located in the Friuli Venezia Giulia (FVG) region (46°02′19.08″N, 12°57′33.66″E), Italy. Fruits were selected apparently healthy, homogeneous in size, and free of lesions and stored at 0°C for 5 days with 92% RH until use.
Botrytis cinerea (Bc13), C. luteo-olivacea (Cad21), and P. synxantha (117-2b) belonged to the mycological collection of University of Udine—Di4A. Cadophora luteo-olivacea Cad21 was isolated from the tissue of symptomatic kiwifruit and molecularly characterized (Di Francesco et al., 2023). Pseudomonas synxantha 117-2b was originally isolated from the surface of kiwifruits and subsequently molecularly characterized (Di Lenarda et al., 2010). Botrytis cinerea Bc13 was isolated from infected kiwifruit, purified, and morphologically characterized. The fungal pathogens were cultivated on potato dextrose agar (PDA, 39 g L−1) (Oxoid, UK) at 25°C, 1 and 2 weeks before the experiments, for B. cinerea and C. luteo-olivacea, respectively. Bacillus pumilus QST2808, the active component of the commercial product Sonata®, was purchased from the NRRL (Northern Regional Research Laboratory, IL, USA) since its effectiveness against the target pathogens was tested in our previous study (Di Francesco et al., 2023) and it was used as a positive control in the present work. For the assays, both bacterial strains were grown on nutrient agar (NA, 13 g L−1) (Oxoid, UK) at 25°C and the cells from 2-day-old NA plates were suspended in potassium phosphate buffer (PPB, 70 mL of 0.2 M KH2PO4, 30 mL of 0.2 M K2HPO4, and 300 mL of deionized water v/v/v, pH 6.5) and adjusted to the final concentration of 1×108 cells mL−1.
To verify P. synxantha 117-2b (Di Francesco et al., 2023) VOCs’ efficacy against C. luteo-olivacea (Cad21) and B. cinerea (Bc13) mycelial growth, a double Petri dish assay was performed as reported by Di Francesco et al. (2019). Plates were incubated at 25°C under dark conditions for 14 and 5 days for C. luteo-olivacea and B. cinerea, respectively. Bacillus pumilus QST2808 (1×108 cells mL−1) was inoculated as reported above and used as a positive control. NA plates inoculated with 100 μL of sterile distilled water (SDW) represented the negative control. The sample unit of each treatment was represented by five replicates and the experiment was performed twice. The inhibition rate of mycelial growth was calculated using the equation (Dennis and Webster, 1971):
where d1 and d2 are the control colony and the treated colony diameters, respectively.
To identify VOCs produced by P. synxantha (117-2b) (Di Francesco et al., 2023), sterile glass vials containing 10 mL of NA were inoculated with 50 μL of P. synxantha suspension (1×108 cells mL−1). Vials were incubated at 25°C for 2 days and then the VOCs were extracted and analyzed by a gas chromatograph (7890B Agilent Technologies, Santa Clara, USA) coupled with a mass spectrometer detector (5977A, Agilent Technologies, Santa Clara, USA) equipped with an autosampler for SPME automatic injections (PAL RSI 85, CTC). An SPME fiber with 50/30 μm DVB/CAR/PDMS coating was selected and conditioned before use. The method used for the extraction and absorption of volatiles provided an incubation of the vial at 40°C for 5 min, followed by fiber exposure of 30 min at the same temperature. For the desorption of the volatiles, the fiber was introduced into the injector at 250°C for 5 min. The separation of analytes was performed on a 60-m-long fused silica capillary column, with an internal diameter of 0.50 mm and a polar stationary phase (DB-WAX, Agilent Technologies, Santa Clara, USA) with a film thickness of 0.5 µm. The GC oven temperature program was as follows: 40°C for 3 min, raised from 40°C to 220°C by a constant ramp of 4°C min−1; 220°C for 1 min, raised from 220°C to 250°C at 10°C min−1; and 250°C for 1 min. Helium was used as carrier gas with a constant column flow rate of 1.5 mL min−1. The transfer line temperature was maintained constant at 250°C. Ion source and quadrupole were set at 230°C and 150°C, respectively. Upon exiting the column, compounds were ionized via electron impact at 70 eV and detected with a quadrupole mass spectrometer in the range of a mass/charge ratio (m/z) from 35 to 500. Chromatograms were processed and analyzed by Mass Hunter (Agilent, USA). Compound identification was achieved by comparing the spectra with the NIST Standard Reference Database (NIST2014) and by linear retention indices (LRI) calculated under the same chromatographic conditions, injecting C7–C30 n‐alkane series (Supelco, Milan, Italy). VOC content was reported as absolute peak area (Aprea et al., 2012). The sample unit consisted of four replicates.
Two synthetic compounds (1-butanol, 3-methyl and 1-nonene) (Merck, Germany) identified as the main VOCs produced by P. synxantha 117-2b were selected and singularly used. For each pure compound, different concentrations (15, 25, and 50 µL, corresponding to 0.34, 0.56, and 1.12 µL mL−1 headspace, respectively) were settled on sterile filter paper (Whatman®, 90 mm Ø), previously placed inside the Petri dishes (90 mm Ø) containing PDA inoculated with fungal plugs (6 mm Ø) of each pathogen. The dishes were immediately double-sealed with Parafilm® and incubated at 25°C for 14 and 5 days, respectively, for C. luteo-olivacea and B. cinerea. The effect of each compound was detected by measuring the diameters of each fungal colony (Youssef and Hashim, 2019). The control was represented by plates containing un-wet sterile filter papers. Each VOC concentration was represented by three plates per pathogen. The experiment was performed twice.
Biofumigation assay was conducted to verify the effect of volatile compounds produced by the BCAs in increasing the fruit resistance to gray mold and skin-pitting during the cold storage. In vivo assay was conducted by using sterile plastic boxes (29 × 18 × 10 cm, L × W × H) containing 150 mL of NA at the bottom. Six hundred microliters of P. synxantha 117-2b suspension (1×108 cells mL−1) was spread on the medium. The boxes were immediately double-sealed with Parafilm® and incubated at 25°C for 2 days. Kiwifruits were surface-sterilized for 1 min with sodium hypochlorite (0.1% v/v), rinsed with distilled water for 1 min, and air-dried at room temperature. Fruits were artificially wounded (2 × 2 × 2 mm) at the equatorial part with a sterile nail and inoculated with 20 μL of conidial suspension of C. luteo-olivacea (Cad21) (1×105 conidia mL−1) or B. cinerea (Bc13) (1×105 conidia mL−1). Once dried, fruits were placed on sterile grills inside the boxes to be separated from the medium. Boxes, incubated at 15°C and 85% RH, were exposed to bacterial VOCs for 96 h. After the biofumigation, treated fruits were removed from the boxes and stored at 0°C for 3 months and 1 month for C. luteo-olivacea and B. cinerea, respectively. As a positive control, B. pumilus QST2808 was used as a biofumigant treatment. Boxes containing just NA were considered the negative control. Each sample unit was represented by three boxes (containing eight kiwifruit) for each pathogen and treatment.
Kiwifruit peel was sampled from unwounded fruits treated with P. synxantha 117-2b VOCs (as described in Section 2.6), and collected at 3, 6, 12, 24, 48, and 96 h of biofumigation treatment using a sterile peeler. Fruit peel samples were immediately frozen in liquid nitrogen, lyophilized by freeze-drying (FD-10 Freezing Dryer, Lab kits, H.K.) under vacuum (<20 Pa) at a temperature of −36°C and freeze-dried for 7 days, and stored at −80°C until use. Fruits not exposed to bacterial VOCs were considered as control. Three boxes containing eight fruits were used for each VOC exposition time.
Total RNA was extracted from the collected fruit peel samples using the Spectrum™ Plant Total RNA Kit (Sigma Aldrich®, DE, USA), according to the manufacturer’s protocol. The total RNA concentration and RNA quality were determined using a NanoDrop™ 2000 spectrophotometer (Thermo Scientific, DE, USA). The integrity of RNA was further confirmed with an agarose gel stained with GelRed™ Nucleic Acid Gel Stain (Biotium, Hayward, CA, USA). The cDNA synthesis was performed using 1 μg of total RNA and the SuperScript™ IV cDNA synthesis kit (Invitrogen, Thermo Fisher Scientific).
The transcription levels of seven selected fruit defense genes (Supplementary Table S1) were assessed by qPCR using the 7500 Real-Time PCR System (Applied Biosystems, Foster City, CA). The reaction mixture consisted of the KAPA SYBR® Fast qPCR Master Mix (Kapa Biosystems, Inc., Wilmington, USA), 100 nM of each primer, and 9 ng of cDNA. Thermal cycling conditions were as follows: (i) 10 min at 95°C for initial denaturation, (ii) followed by 40 cycles of 15 s at 95°C, and (iii) annealing at 60°C for 1 min. A final amplification cycle at 95°C for 15 s, 60°C for 1 min, 95°C for 30 s, and 60°C for 15 s was applied to determine the melting curve. A non-template control (NTC) was included in each run using DNase-free water instead of cDNA. The sequence of each targeted gene was identified in the kiwifruit genome database (https://kiwifruitgenome.org/), and specific primers were designed de novo (Supplementary Table S1) using the NCBI primer blast tool (https://www.ncbi.nlm.nih.gov/tools/primer-blast/). Primer efficiency was determined by the serial dilution method, using a mix of all cDNA samples as a template. Genes encoding for ACTIN and 18S RNA were checked as independent reference genes, and ACTIN was selected for its constant expression among sample conditions. Relative gene expression was expressed as mean normalized expression (MNE) according to Muller et al. (2002), using ACTIN as a reference gene. Results were obtained from the average of three biological replicates.
Data were statistically handled by one-way analysis of variance (ANOVA) using MiniTab 17. Mean statistical comparison of the effect of VOCs on the fungal colony diameter and on the incidence and disease severity was carried out by using Tukey’s HSD test (α = 0.05), as reported by Abo-Elyousr et al. (2020). For gene expression analysis, comparison of the means within the control and treated samples was performed using the Student’s t-test (α ≤ 0.05), and that between different time points for control and treated samples was carried out using Tukey’s HSD test (*α ≤ 0.05, ** α ≤ 0.01).
The double Petri dish assay displayed the potential in vitro efficacy of VOCs produced by P. synxantha 117-2b against C. luteo-olivacea and B. cinerea. Figure 1 reports the results of the experiment that showed a significant inhibitory effect of P. synxantha 117-2b and B. pumilus QST2808 VOCs against pathogens’ mycelial diameter. Pseudomonas synxantha 117-2b showed an inhibition of 56% and 42.8%, with respect to the relative control, against C. luteo-olivacea and B. cinerea, respectively. Likewise, B. pumilus QST2808 showed a significant inhibition, with respect to the relative control, against both fungal pathogens, of 52% and 44.9%, respectively.
Figure 1 Effect of volatile compounds produced by Pseudomonas synxantha 117-2b and Bacillus pumilus QST2808 on the mycelial growth (mm) of Cadophora luteo-olivacea (Cad21) and Botrytis cinerea (Bc13). Fungal colony diameters (mm) were measured after 14 and 5 days at 25°C, respectively. Each value is the mean of five plates (replicates) ± standard error. Different letters represent significant differences among the treatments within each pathogen according to Tukey’s HSD test (α = 0.05).
The volatile profile of P. synxantha 117-2b was investigated on NA medium through SPME–GC/MS (Supplementary Table S2). VOCs emitted by the bacterial antagonist exhibited a miscellaneous range of chemical compound classes, particularly alcohols (1-butanol, 3-methyl and phenylethyl alcohol), alkenes (1-nonene), and ketones (2-pentanone) (Table 1). VOCs that showed a higher absolute area (AA) were 1-nonene and 1-butanol, 3-methyl.
Table 1 Volatile organic compounds (VOCs) produced by Pseudomonas synxantha 117-2b at 25 °C after 48 h on Nutrient Agar (NA).
Among the detected VOCs, those with a higher AA such as 1-butanol, 3-methyl and 1-nonene were selected for assessing their effect on C. luteo-olivacea (Figure 2A) and B. cinerea (Figure 2B) colony growth. The compound 1-butanol, 3-methyl became more effective at 1.12 µL mL−1 headspace, showing an inhibition by 55.3% and 100% of C. luteo-olivacea and B. cinerea colony growth compared to the control, respectively (Figure 2C). The alkene 1-nonene did not exhibit any significant inhibitory effect against both fungal pathogens.
Figure 2 Effect of different concentrations (15, 25, and 50 µL, corresponding to 0.34, 0.56, and 1.12 µL mL−1 headspace, respectively) of synthetic volatile compounds mainly produced by Pseudomonas synxantha 117-2b on the mycelial growth (mm) of Cadophora luteo-olicea (Cad21) (A, C) and Botrytis cinerea (Bc13) (B, C). Fungal colony diameters (mm) were measured after 14 and 5 days at 25°C, respectively. Each value is the mean of three plates (replicates) ± standard error. Different letters represent significant differences among the concentrations within each volatile compound according to Tukey’s HSD test (α = 0.05).
VOCs produced by P. synxantha 117-2b showed a significant inhibitory effect against the targeted pathogens (Supplementary Figures 1A, B). Regarding C. luteo-olivacea, results were reported as severity (mm) of the disease (Figure 3A), and for B. cinerea, results were reported as percentage (%) of the disease incidence (Figure 3B), after 3 months and 1 month, respectively, of cold storage after 96 h of bacterial VOC biofumigation. The symptoms caused by the two pathogens were differently detected. The diameter of gray mold lesions was not easily detectable on kiwifruit, and conversely for the skin-pitting. Moreover, as regards C. luteo-olivacea, the incidence inhibition of the symptoms on fruits was not reported as not statistically different from the control. Regarding the skin-pitting symptoms, P. synxantha VOCs reduced the disease severity by 28.5% with respect to the control. For B. cinerea, the displayed inhibition of the disease incidence was 66.6% with respect to the control. VOCs produced by the reference strain B. pumilus QST2808, showed, in the case of skin-pitting, the same efficacy of the tested BCA, and conversely significantly higher in the case of gray mold incidence (90.3%).
Figure 3 Effect of 96 h of exposition to VOCs produced by Pseudomonas synxantha 117-2b and Bacillus pumilus QST2808 on Cadophora luteo-olivacea severity (mm) (A) and Botrytis cinerea (B) disease incidence (%) on kiwifruit after 3 months and 1 month of cold storage (0°C), respectively. The symptoms caused by the two pathogens were differently detected for their different appearance on kiwifruit. The diameter of gray mold lesions was not detectable on kiwifruit, conversely for the skin-pitting. Moreover, C. luteo-olivacea incidence was not reported as not statistically different from the control.
With the aim of determining the expression pattern of defense-related genes activated in kiwifruit as a response to P. synxantha 117-2b VOC exposition, the expression levels of several genes (Supplementary Table S1) at six different time points (3, 6, 12, 24, 48, and 96 h) after biofumigation were analyzed. Results revealed that the bacterial VOCs, in general, promoted the upregulation of the target genes (Figure 4). Specifically, the results for CAT expression levels (Figure 4A) revealed a significant increase in its expression at 3, 24, 48, and 96 h from the treatment with bacterial VOCs, compared to the control. Notably, the highest expression level was observed at 48 h post-treatment, which was 1.2-fold higher than the expression in the treated sample compared to the control. However, no significant differences in CAT gene expression compared to the control were observed after 6 and 12 h from the treatment. Moreover, a significant increase in the expression level of the CAT gene was also observed in the control samples from 24 to 96 h. Similarly, the expression of CHI gene (Figure 4B) started to increase significantly at 3 h from the treatment and continued to rise for 12 h. However, after the 12-h time point, the expression levels of the CHI gene were sustained, although a significantly higher expression occurred at 24 and 96 h, compared to the control. In the control fruit, the CHI gene showed a higher expression after 48 and 96 h compared to the earlier time points. In the case of GLU (Figure 4C), a significant increase in the expression was observed at 6 h from the treatment compared to the untreated fruit, increasing its expression for 96 h. No significant increase was detected in the control samples over time. The results of the NPR gene expression level (Figure 4D) revealed a significant increase along with the treatment time points and the maximum induction took place after 96 h from the treatment compared to the control. Conversely, no significant differences in NPR gene expression were observed at 6 and 12 h with respect to the control. The exposition of fruits to bacterial VOCs caused a significant increase in POD gene expression (Figure 4E) at 6 h, 24 h, 48 h, and 96 h compared to the control. Specifically, a notable upregulation occurred at 96 h, being 2.3-fold higher compared to the control. However, if compared to the control, no significant differences in POD expression at 3 and 12 h were detected. Regarding the SOD gene (Figure 4F), the treatment induced its expression levels after 12 h, and this pattern was sustained for 96 h. SOD gene expression increased in the control samples at 96 h compared to 0-h time point. The fruit PAL gene (Figure 4G) was notably upregulated after bacterial VOC exposition at 24, 48, and 96 h compared to the earlier time points. In contrast, no significant differences in PAL expression were observed in control samples, except for 96 h, in which gene expression levels were upregulated compared to the other time points.
Figure 4 Effect of volatile organic compounds (VOCs) produced by Pseudomonas synxantha 117-2b on the expression level of CAT (A), CHI (B), GLU (C), NPR (D), POD (E), SOD (F), and PAL (G) genes in kiwifruit sampled after different time points (3, 6, 12, 24, 48, and 96 h) from bacterial VOC exposition. Data are expressed as the mean of three biological replicates ± standard error. For each time point (lowercase—control − uppercase—treatment), different letters indicate significant differences according to Student’s t-test (α ≤ 0.05). Asterisks denote significant differences between control and VOC-treated samples at the same time point (*α ≤ 0.05, ** α ≤ 0.01).
The present study aimed to find a sustainable solution to use during kiwifruit storage, for its preservation from skin-pitting and gray mold diseases by using a bacterial BCA instead of a synthetic fungicide. Many microorganisms including bacteria, yeasts, and fungi are VOC producers, and their biocontrol activity has been studied (Gotor-Vila et al., 2017; Di Francesco et al., 2020; Guo et al., 2023). Among bacteria, Pseudomonas has been studied as a potential BCA against a wide range of fungal pathogens, mainly for its ability to produce antifungal compounds (Sang and Kim, 2014; Aiello et al., 2019; Rojas-Solis et al., 2020; Di Francesco et al., 2023). Mukherjee et al. (2014) reported P. synxantha as a producer of bioactive compounds, including volatile compounds, effective against several microorganisms. The antagonistic activity through the production of secondary metabolites was already verified for P. synxantha strain 117-2b by Di Francesco et al. (2023). In the last few years, many studies on the different modes of action exerted by BCAs in the inhibition of pathogenic fungi have been conducted. Recently, the essential role of VOCs has been given more consideration (Toffano et al., 2017), specially for the potential use during the fruit postharvest phase as biofumigants. The present study has displayed the efficacy of the strain P. synxantha 117-2b against skin-pitting and gray mold of kiwifruit by producing VOCs, as it was already reported as an effective antagonist against C. luteo-olivacea through other modes of action (Di Francesco et al., 2023). In fact, using the double Petri dish assay, P. synxantha 117-2b showed a significant inhibition of the mycelial growth of the targeted pathogens C. luteo-olivacea (Cad21) and B. cinerea (Bc13) after the exposition to VOCs, reaching an inhibition of 56% and 42.8%, respectively. Its effectiveness was compared to B. pumilus QST2808, a strain selected for its high efficacy against C. luteo-olivacea in a previous study (Di Francesco et al., 2023). In in vitro assays, both treatments showed the same efficacy against the two target pathogens, making P. synxantha 117-2b a promising candidate for further investigation and potential application. The outcomes of our study are partially in agreement with the antifungal effect of VOCs produced by P. synxantha strain DLS65 applied against Monilinia fructicola and Monilinia fructigena in stone fruits (Aiello et al., 2019). In fact, Aiello et al. (2019) reported that the best performances of P. synxantha DLS65 were determined mainly by the mechanisms of action that involved the presence of bacterial living cells. In our case, VOCs produced by P. synxantha 117-2b also became effective in in vivo assays where an incidence reduction of almost 70% was registered for B. cinerea. In fact, the experiment combined two different strategies, curing and biofumigation, that can also contribute to an enhancement of kiwifruit disease resistance. Firstly, it was necessary to determine the P. synxantha 117-2b VOC profile by SPME–GC/MS analysis. Results revealed an array of volatile compounds belonging mainly to alcohols, aldehydes, and alkane classes.
Usually, the principal group of microorganism metabolites was formed by alcohols as reported in previous studies (Stoppacher et al., 2010; Rouissi et al., 2013). However, the applied methodology to collect and detect VOCs, as well as the growth medium, can strongly influence the results and often hinder the comparison between studies (Martins et al., 2010; Di Francesco et al., 2015). Comprehensively, P. synxantha VOC application as a biofumigant treatment during the curing phase slightly reduced C. luteo-olivacea symptoms without controlling the infection; thus, in this case, the treatment could be part of an integrated strategy to control skin-pitting. Instead, for B. cinerea, P. synxantha VOCs notably reduced the pathogen incidence (66.6%) compared to the untreated control. Perhaps, this effectiveness can be partly attributed to the overexpression of different fruit defense-related genes, such as CAT, CHI, GLU, NPR, POD, SOD, and PAL. In particular, the antioxidant role of genes such as CAT, POD, and SOD has been proven to be crucial for fruit disease resistance, also contributing to the response to biotic and abiotic stresses (Pan et al., 2020). Recent lines of evidence suggest that plants treated with resistance-inducing substances (e.g., chitosan) exhibit faster and stronger defense responses when infected by pathogens (Maya and Matsubara, 2013; Zhang et al., 2018). Our results showed that the bacterial VOCs’ exposition slightly increased the expression level of all targeted genes in treated kiwifruits. It is also known at the molecular level as bacterial VOCs could show up- and downregulation of gene expression (Wheatley, 2002).
However, the different genes showed different behavior patterns and a higher expression level at different time points. Kiwifruit CHI, NPR, GLU, and CAT genes showed an early response to bacterial VOC exposition starting their induction at 3 h from the treatment. Among the selected defense genes, the CHI gene represents one of the main pathogenesis-related (PR) members (Zhang et al., 2018) responsible for plant defense against pathogenic fungi by breaking down their cell walls (Li et al., 2013). Similar to the CHI gene, VOC exposition also triggered the expression of the GLU gene in kiwifruit. β-1,3-Glucanase plays a direct role in fungal defense by hydrolyzing fungal cell walls and promoting the formation of elicitors (Ebrahim et al., 2011). Our study reported that the increased expression of the kiwifruit GLU gene by VOC treatment was principally detected after 6 h compared to the control.
Regarding the NPR gene, it has been identified as a positive controller of defense response mediated by salicylic acid (SA) (Hong et al., 2016). In certain plants like Arabidopsis and grapes, the activation of NPR genes can occur within a day when exposed to SA or analogs, or to specific pathogens (Le Henanff et al., 2009). In our case, results showed a significant increase in NPR1 expression levels after 3 h from treatment exposition, such as occurred for CHI, GLU, and CAT genes. In fact, a significant upregulation of key defense-related genes like CAT, CHI, GLU, NPR, POD, SOD, and PAL supports the hypothesis that P. synxantha 117-2b VOCs induced defense mechanisms in kiwifruit. However, the findings of our study demonstrated that the activation of each defense-related genes is strictly dependent on the different treatment times. Moreover, we also observed a gradual increase in the expression of CAT, CHI, POD, and PAL genes in the untreated samples over time. This phenomenon is likely due to the possible kiwifruit endogenous processes such as the natural senescence process that triggers the upregulation of defense-related genes, probably stimulated by the curing period (Wurms et al., 1997). Overall, our results demonstrated that VOCs produced by P. synxantha strain 117-2b can elicit a defense response in kiwifruit during the curing phase, supporting the obtained in vivo results, where the treatment reduced only gray mold incidence. Further studies are needed to better investigate the molecular mechanisms involved in the activation of defense response in kiwifruit and to implement the practical application of biocontrol agents and volatile metabolites in the postharvest phase to manage fruits through a sustainable strategy.
The findings of the present study provide new insights into the effects of VOCs produced by the strain P. synxantha 117-2b against C. luteo-olivacea and B. cinerea, both fungal postharvest pathogens of kiwifruit. The results showed how the production of VOCs plays an essential role in the antagonistic activity of a BCA and how their production can be strongly influenced by growth substrates. In addition, the biofumigant treatment of kiwifruits by P. synxantha 117-2b notably promoted the expression levels of fruit defense genes. For this reason, this BCA may be applied with the purpose of inhibiting the pathogen’s growth and, at the same time, increasing fruit defense responses. However, further investigation is necessary to understand the exact contribution of P. synxantha 117-2b in the control of kiwifruit postharvest pathogens and to improve its efficacy, which depended on the pathogen and the experimental conditions.
Data will be made available on request. Requests to access the datasets should be directed to YWxlc3NhbmRyYS5kaWZyYW5jZXNjb0B1bml1ZC5pdA==.
AD: Conceptualization, Data curation, Methodology, Writing – original draft, Writing – review & editing. FJ: Methodology, Writing – original draft, Writing – review & editing. NV-l: Data curation, Methodology, Supervision, Writing – review & editing. EM: Methodology, Writing – review & editing. MM: Supervision, Writing – review & editing. RT: Supervision, Writing – review & editing. PE: Project administration, Writing – review & editing. NT: Conceptualization, Methodology, Supervision, Writing – review & editing.
The author(s) declare financial support was received for the research, authorship, and/or publication of this article. This work was supported by Convenzione ERSA per “Sviluppo e adattamento del kiwi al cambiamento climatico e alle sindromi emergent” CUP: F23C23000300002.
The authors want to thank the kiwifruit producers of the “Friulkiwi” cooperative (Rauscedo, Italy) and Giovanni Fontanel and Iolanda Nicolau for the technical support.
The authors declare that the research was conducted in the absence of any commercial or financial relationships that could be construed as a potential conflict of interest.
The author(s) declared that they were an editorial board member of Frontiers, at the time of submission. This had no impact on the peer review process and the final decision.
All claims expressed in this article are solely those of the authors and do not necessarily represent those of their affiliated organizations, or those of the publisher, the editors and the reviewers. Any product that may be evaluated in this article, or claim that may be made by its manufacturer, is not guaranteed or endorsed by the publisher.
The Supplementary Material for this article can be found online at: https://www.frontiersin.org/articles/10.3389/fpls.2024.1398014/full#supplementary-material
Abo-Elyousr, K. A. M., Almasoudi, N. M., Abdelmagid, A. W. M., Roberto, S. R., Youssef, K. (2020). Plant extract treatments induce resistance to bacterial spot by tomato plants for a sustainable system. Horticulturae 6, 36. doi: 10.3390/horticulturae6020036
Aiello, D., Restuccia, C., Stefani, E., Vitale, A., Cirvilleri, G. (2019). Postharvest biocontrol ability of Pseudomonas synxantha against Monilinia fructicola and Monilinia fructigena on stone fruit. Postharvest Biol. Technol. 149, 83–89. doi: 10.1016/j.postharvbio.2018.11.020
Aprea, E., Corollaro, M. L., Betta, E., Endrizzi, I., Demattè, M. L., Biasioli, F., et al. (2012). Sensory and instrumental profiling of 18 apple cultivars to investigate the relation between perceived quality and odour and flavour. Food Res. Int. 49, 677–686. doi: 10.1016/j.foodres.2012.09.023
Calvo, H., Mendiara, I., Arias, E., Gracia, A. P., Blanco, D., Venturini, M. E. (2020). Antifungal activity of the volatile organic compounds produced by Bacillus velezensis strains against postharvest fungal pathogens. Postharvest Biol. Technol. 166, 111208. doi: 10.1016/j.postharvbio.2020.111208
Che, J., Liu, B., Liu, G., Chen, Q., Lan, J. (2017). Volatile organic compounds produced by Lysinibacillus sp. FJAT-4748 possess antifungal activity against Colletotrichum acutatum. Biocontrol Sci. Technol. 27, 1349–1362. doi: 10.1080/09583157.2017.1397600
Dennis, C., Webster, J. (1971). Antagonistic properties ofspecies-groups of Trichoderma: II. production of volatileantibiotics. Transact. Br. Mycol. Soc 57, 363–369. doi: 10.1016/S0007-1536(71)80077-3
Di Francesco, A., Di Foggia, M., Baldo, D., Ratti, C., Baraldi, E. (2022). Preliminary results on Cadophora luteo-olivacea pathogenicity aspects on kiwifruit. Eur. J. Plant Pathol. 163, 997–1001. doi: 10.1007/s10658-022-02518-6
Di Francesco, A., Di Foggia, M., Baraldi, E. (2020). Aureobasidium pullulans volatile organic compounds as alternative postharvest method to control brown rot of stone fruits. Food Microbiol. 87, 103395. doi: 10.1016/j.fm.2019.103395
Di Francesco, A., Jabeen, F., Di Foggia, M., Zanon, C., Cignola, R., Sadallah, A., et al. (2023). Study of the efficacy of bacterial antagonists against Cadophora luteo-olivacea of kiwifruit. Biol. Control 180, 105199. doi: 10.1016/j.biocontrol.2023.105199
Di Francesco, A., Mari, M., Ugolini, L., Baraldi, E. (2018). Effect of Aureobasidium pullulans strains against Botrytis cinerea on kiwifruit during storage and on fruit nutritional composition. Food Microbiol. 72, 67–72. doi: 10.1016/j.fm.2017.11.010
Di Francesco, A., Ugolini, L., Lazzeri, L., Mari, M. (2015). Production of volatile organic compounds by Aureobasidium pullulans as a potential mechanism of action against postharvest fruit pathogens. Biol. Control 81, 8–14. doi: 10.1016/j.biocontrol.2014.10.004
Di Lenarda, S., Martini, M., Osler, R., Vizzotto, G., Prete, G., Frausin, C. (2010). Biological and agronomical strategies for controlling skin-pitting of kiwifruit. VII International Symposium on Kiwifruit. Acta Hortic. 913, 501–508.
Droby, S., Wisniewski, M. (2018). The fruit microbiome: A new frontier for postharvest biocontrol and postharvest biology. Post. Biol. Technol. 140, 107–112. doi: 10.1016/j.postharvbio.2018.03.004
Droby, S., Wisniewski, M., Teixidó, N., Spadaro, D., Jijakli, M. H. (2016). The science, development, and commercialization of postharvest biocontrol products. Postharvest Biol. Technol. 122, 22–29. doi: 10.1016/j.postharvbio.2016.04.006
Ebrahim, S., Usha, K., Singh, B. (2011). Pathogenesis-related (PR)-proteins: Chitinase and β-1, 3-glucanase in defense mechanism against malformation in mango (Mangifera indica L.). Scientia Hortic. 130, 847–852. doi: 10.1016/j.scienta.2011.09.014
Gotor-Vila, A., Teixidó, N., Di Francesco, A., Usall, J., Ugolini, L., Torres, R., et al. (2017). Antifungal effect of volatile organic compounds produced by Bacillus amyloliquefaciens CPA-8 against fruit pathogen decays of cherry. Food Microbiol. 64, 219–225. doi: 10.1016/j.fm.2017.01.006
Guo, J., Xu, Y., Liang, S., Zhou, Z., Zhang, C., Li, K., et al. (2023). Antifungal activity of volatile compounds from Bacillus tequilensis XK29 against Botrytis cinerea causing gray mold on cherry tomatoes. Postharvest Biol. Technol. 198, 112239. doi: 10.1016/j.postharvbio.2022.112239
Hong, K., Gong, D., Zhang, L., Hu, H., Jia, Z., Gu, H., et al. (2016). Transcriptome characterization and expression profiles of the related defense genes in postharvest mango fruit against Colletotrichum gloeosporioides. Gene 576, 275–283. doi: 10.1016/j.gene.2015.10.041
Hyun, J., Lee, J. G., Yang, K. Y., Lim, S., Lee, E. J. (2022). Postharvest fumigation of (E)-2-hexenal on kiwifruit (Actinidia chinensis cv.‘Haegeum’) enhances resistance to Botrytis cinerea. Postharvest Biol. Technol. 187, 111854. doi: 10.1016/j.postharvbio.2022.111854
Köhl, J., Wenneker, M., Groenenboom-de Haas, B. H., Anbergen, R., Goossen-van de Geijn, H. M., Lombaers-van der Plas, C. H., et al. (2018). Dynamics of post-harvest pathogens Neofabraea spp. and Cadophora spp. in plant residues in Dutch apple and pear orchards. Plant Pathol. 67, 1264–1277. doi: 10.1111/ppa.12854
Le Henanff, G., Heitz, T., Mestre, P., Mutterer, J., Walter, B., Chong, J. (2009). Characterization of Vitis vinifera NPR1 homologs involved in the regulation of pathogenesis-related gene expression. BMC Plant Biol. 9, 1–14. doi: 10.1186/1471-2229-9-54
Martins, M., Henriques, M., Azeredo, J., Rocha, S. M., Coimbra, M. A., Oliveira, R. (2010). Candida species extracellular alcohols: production and effect in sessile cells. J. Basic Microbiol. 50, S89–S97. doi: 10.1002/jobm.200900442
Maya, M. A., Matsubara, Y. I. (2013). Tolerance to Fusarium wilt and anthracnose diseases and changes of antioxidative activity in mycorrhizal cyclamen. Crop Prot. 47, 41–48. doi: 10.1016/j.cropro.2013.01.007
Mukherjee, K., Mandal, S., Mukhopadhyay, B., Mandal, N. C., Sil, A. K. (2014). Bioactive compound from Pseudomonas synxantha inhibits the growth of mycobacteria. Microbiol. Res. 169, 794–802. doi: 10.1016/j.micres.2013.12.005
Muller, P. Y., Miserez, A. R., Dobbie, Z. (2002). Short technical report processing of gene expression data generated. Gene Exp. 33, 514.
Niu, Y., Wang, D., Ye, L., Wang, Y., Liang, J., Huang, T., et al. (2023). Nondestructive detection of kiwifruit infected with Penicillium expansum based on electrical properties. Postharvest Biol. Technol. 195, 112150. doi: 10.1016/j.postharvbio.2022.112150
Pan, L., Zhao, X., Chen, M., Fu, Y., Xiang, M., Chen, J. (2020). Effect of exogenous methyl jasmonate treatment on disease resistance of postharvest kiwifruit. Food Chem. 305, 125483. doi: 10.1016/j.foodchem.2019.125483
Rojas-Solis, D., Vences-Guzmán, M. A., Sohlenkamp, C., Santoyo, G. (2020). Bacillus toyonensis COPE52 modifies lipid and fatty acid composition, exhibits antifungal activity, and stimulates growth of tomato plants under saline conditions. Curr. Microbiol. 77, 2735–2744. doi: 10.1007/s00284-020-02069-1
Rouissi, W., Ugolini, L., Martini, C., Lazzeri, L., Mari, M. (2013). Control of postharvest fungal pathogens by antifungal compounds from Penicillium expansum. J. Food Prot. 76, 1879–1886. doi: 10.4315/0362-028X.JFP-13-072
Sang, M. K., Kim, K. D. (2014). Biocontrol activity and root colonization by Pseudomonas corrugata strains CCR04 and CCR80 against Phytophthora blight of pepper. Biocontrol 59, 437–448. doi: 10.1007/s10526-014-9584-9
Stoppacher, N., Kluger, B., Zeilinger, S., Krska, R., Schuhmacher, R. (2010). Identification and profiling of volatile metabolites of the biocontrol fungus Trichoderma atroviride by HS-SPME-GC-MS. J. Microbiol. Method. 81, 187–193. doi: 10.1016/j.mimet.2010.03.011
Teixidó, N., Usall, J., Torres, R. (2022). Insight into a successful development of biocontrol agents: production, formulation, packaging, and shelf life as key aspects. Horticulturae 8, 305. doi: 10.3390/horticulturae8040305
Toffano, L., Fialho, M. B., Pascholati, S. F. (2017). Potential of fumigation of orange fruits with volatile organic compounds produced by Saccharomyces cerevisiae to control citrus black spot disease at postharvest. Biol. Control 108, 77–82. doi: 10.1016/j.biocontrol.2017.02.009
Toral, L., Rodríguez, M., Martínez-Checa, F., Montaño, A., Cortés-Delgado, A., Smolinska, A., et al. (2021). Identification of volatile organic compounds in extremophilic bacteria and their effective use in biocontrol of postharvest fungal phytopathogens. Front. Microbiol. 12. doi: 10.3389/fmicb.2021.773092
Wenneker, M., Pham, K. T. K., Lemmers, M. E. C., de Boer, F. A., van Leeuwen, P. J., Hollinger, T. C., et al. (2016). First report of Cadophora luteo-olivacea causing side rot on ‘Conference’pears in the Netherlands. Plant Dis. 100, 2162–2162. doi: 10.1094/PDIS-02-16-0212-PDN
Wheatley, R. E. (2002). The consequences of volatile organic compound mediated bacterial and fungal interactions. Ant. van Leeuw. 81, 357–364. doi: 10.1023/a:1020592802234
Wurms, K., Long, P., Greenwood, D., Sharrock, K., Ganesh, S. (1997). Endo-and exochitinase activity in kiwifruit infected with Botrytis cinerea. J. Phytopathol. 145, 145–151. doi: 10.1111/j.1439-0434.1997.tb00377.x
Youssef, K., Hashim, A. H. (2019). Inhibitory effect of clay/chitosan nanocomposite against Penicillium digitatum on citrus and its possible mode of action. Jordan J. Biol.l Scie. 13, 349–355.
Keywords: biocontrol, biofumigation, defense genes, grey mold, skin pitting, volatile metabolites
Citation: Di Francesco A, Jabeen F, Vall-llaura N, Moret E, Martini M, Torres R, Ermacora P and Teixidó N (2024) Pseudomonas synxantha volatile organic compounds: efficacy against Cadophora luteo-olivacea and Botrytis cinerea of kiwifruit. Front. Plant Sci. 15:1398014. doi: 10.3389/fpls.2024.1398014
Received: 08 March 2024; Accepted: 17 April 2024;
Published: 08 May 2024.
Edited by:
Franco Nigro, University of Bari Aldo Moro, ItalyReviewed by:
Shixiang Yao, Southwest University, ChinaCopyright © 2024 Di Francesco, Jabeen, Vall-llaura, Moret, Martini, Torres, Ermacora and Teixidó. This is an open-access article distributed under the terms of the Creative Commons Attribution License (CC BY). The use, distribution or reproduction in other forums is permitted, provided the original author(s) and the copyright owner(s) are credited and that the original publication in this journal is cited, in accordance with accepted academic practice. No use, distribution or reproduction is permitted which does not comply with these terms.
*Correspondence: Núria Vall-llaura, bnVyaWEudmFsbC1sbGF1cmFAaXJ0YS5jYXQ=; Paolo Ermacora, cGFvbG8uZXJtYWNvcmFAdW5pdWQuaXQ=
†These authors have contributed equally to this work
Disclaimer: All claims expressed in this article are solely those of the authors and do not necessarily represent those of their affiliated organizations, or those of the publisher, the editors and the reviewers. Any product that may be evaluated in this article or claim that may be made by its manufacturer is not guaranteed or endorsed by the publisher.
Research integrity at Frontiers
Learn more about the work of our research integrity team to safeguard the quality of each article we publish.