- College of Horticulture and Landscape, Yangzhou University, Yangzhou, China
Ficus carica is an economically important horticultural plant. Due to its abundant secondary metabolites, F. carica has gained interest for its applications in medicine and as a nutritional supplement. Both external and internal factors affect the accumulation of secondary metabolites in F. carica. The assembly of the F. carica genome has facilitated functional analysis of key genes and transcription factors associated with the biosynthesis of secondary metabolites, particularly anthocyanin. In this review, we summarize the various types and functions of secondary metabolites, with a particular focus on flavonoids, coumarins, and terpenes. We also explore the factors influencing their biosynthesis and accumulation, including varieties, tissue, environmental factors (e.g., light), stresses (e.g., high temperature, low temperature, drought, nutrient deficiencies, salinity), hormonal treatments, and developmental factors. Furthermore, we discuss the involvement of structural genes and transcription factors in the biosynthesis of secondary metabolites, specifically anthocyanin and furanocoumarins, knowledge of which will promote the breeding and genetic engineering of novel F. carica varieties.
1 Introduction
Ficus carica, commonly known as the fig tree, is one of the oldest cultivated plants and is among the most important commercial fruit trees in the genus Ficus. F. carica is extensively cultivated in numerous regions worldwide, particularly in nations of the Mediterranean Basin such as Portugal and Turkey (Dueñas et al., 2008). Fresh and dried F. carica fruits are nutritious sources of secondary metabolites, making them popular ingredients in culinary preparations such as sauces, fruit wines, and dried fruit assortments. Extracts of F. carica leaves and fruits, as well as latex, have been used to treat gastrointestinal, respiratory, inflammatory, metabolic, and cardiovascular conditions (Mawa et al., 2013). The accumulation of secondary metabolites in F. carica is influenced by internal and external factors, such as light quality, high temperature, drought, salt stress, and stress-associated hormones. Development, storage, and processing techniques also affect the accumulation of secondary metabolites in F. carica fruit (Sandhu et al., 2023). The availability of the genome of F. carica and advancements in molecular biology and sequencing technologies have yielded insights into the biosynthetic mechanisms of important secondary metabolites in F. carica (Usai et al., 2020). A comprehensive understanding of secondary metabolites in F. carica is essential for its horticultural cultivation, commercial significance, and potential health benefits. Here, we review the types and functions of secondary metabolites in F. carica, as well as the factors influencing their biosynthesis and accumulation. We also summarize the regulatory mechanisms of important secondary metabolites, including anthocyanins and furanocoumarins, in F. carica, focusing on the related structural genes and transcription factors.
2 Types and functions of secondary metabolites in F. carica
More than 100 bioactive compounds have been identified in F. carica. Almost all parts of the F. carica tree are abundant in secondary metabolites, particularly flavonoids, terpenes, and coumarins (Hajam and Saleem, 2022) (Figure 1). In this section, we focus on these secondary metabolites and their effects on health.
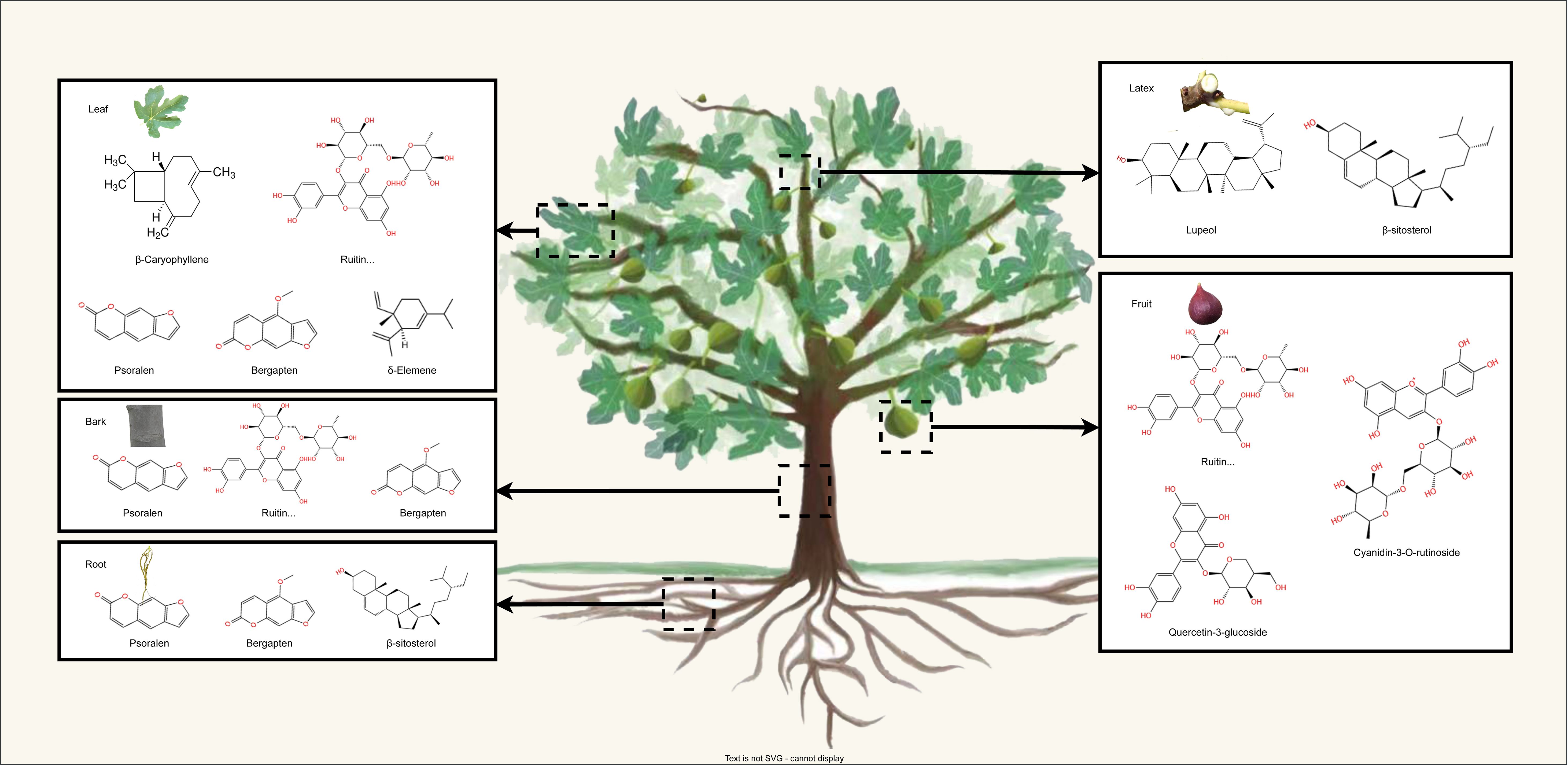
Figure 1 The schematic representation illustrates the distribution of main secondary metabolite in the leaves, fruits barks, roots, and latex of F. carica.
2.1 Flavonoids
Flavonoids, a class of polyphenolic secondary metabolites, are formed through the combination of two six-carbon phenyl rings connected by an oxygen heterocycle-containing ring. F. carica produces flavonoids in various organs, and the latex secreted from the vascular bundles of the petioles and peduncles also contains flavonoids and phenolic acids (Abdel-Aty et al., 2019). Flavonoids encompass several types, including anthocyanins, flavonols, and isoflavones, with significant research focusing on anthocyanins in F. carica fruit (Mao et al., 2023). The content and composition of these anthocyanins play a crucial role in determining the fruit’s color (Castañeda-Ovando et al., 2009). Anthocyanin pigments such as cyanidin, delphinidin, peonidin, petudinin, and malvidin are found in red, purple, and other dark-colored fruits.
Cyanidin is the predominant anthocyanin found in F. carica. During the ripening process of purple peel, cyanidin O-malonylhexoside levels increase dramatically. Other cyanidins, including cyanidin 3-O-glucoside and cyanidin-3, 5-O-diglucoside, also experience a significant increase (Wang et al., 2019). Among five different varieties of F. carica with distinct peel colors, cyanidin 3-rutinoside was the predominant anthocyanin, accounting for 48–81% of peel content and 68–79% of pulp content, while cyanidin 3-glucoside constituted 5–18% of peel content and 10–15% of pulp content (Dueñas et al., 2008). Notably, the dark-purple peel of the ‘Soltani’ variety contains nine identified anthocyanins, primarily cyanidin 3-rutinoside and cyanidin 3,5-diglucoside. The peel of ‘Soltani’ also revealed the presence of a novel rutinoside and petunidin dimer, yet its function remains unclear (Ammar et al., 2015).
Quercetin, an abundant flavonol in nature, has been extensively studied for its antioxidative and anti-inflammatory properties. In vitro, animal, and clinical studies have shown that quercetin can effectively reduce oxidative stress and exhibit anti-inflammatory activity (Ginwala et al., 2019; Jucá et al., 2020). Notably, quercetin has been found to reduce blood glucose levels in diabetic mice (Deepa et al., 2018; Dureshahwar et al., 2019). It is hypothesized that quercetin, along with other compounds, contributes to the observed hypoglycemic effect of the extract. Rutin, a glycoside form of quercetin, is predominantly present in F. carica fruit and leaves. Rutin has significant antioxidant activity, anticancer activity, and anti-inflammatory and antibacterial effects (Yang et al., 2008; Hao et al., 2016). It is a popular dietary supplement without any significant side effects. According to the available reports, the highest extraction rate of rutin from F. carica pulp was 0.44% (Ammar et al., 2015). In addition to flavonols like quercetin and rutin, F. carica also contains isoflavones. Isoflavones, which are 3-phenyl derivatives of benzone rings, are synthesized during the phenylalanine metabolism in plants. F. carica fruit has been found to contain 16 types of isoflavones, while the leaf contains 2 types. Interestingly, the isoflavones present in F. carica fruit have been shown to possess anti-inflammatory effects by inhibiting the production of nitric oxide (NO) (Liu et al., 2019). Several specific isoflavones, including ficucaricone A, ficucaricone B, and indicanine A, have been reported to exhibit potent anti-inflammatory activities (Magalhães et al., 2006).
2.2 Coumarins
Coumarins account for 51.46% of the volatile oils in F. carica leaves (Shahrajabian et al., 2021). Psoralen, bergapten, angelicin, rutaretin, pimpinellin, and seselin are all members of the coumarin family, serving as important secondary metabolites in F. carica (Marrelli et al., 2012). Among them, psoralen and bergapten are two of the most prominent coumarins due to their medicinal importance. They have been identified as anticancer agents with the ability to suppress the survival and migration of triple-negative breast cancer MDA-MB-231 cells (Zhang et al., 2018). Psoralens have shown promise in clinical studies, upregulating the level of ERα protein in osteoclasts and inhibiting the growth of breast cancer cells (Zhang et al., 2017). Additionally, psoralens and bergaptens can inhibit acetylcholinesterase (AChE) activity and play a role in the treatment of Alzheimer’s disease (Parhiz et al., 2015). Due to their ability to interact with DNA when exposed to ultraviolet A radiation (UV-A), these compounds, especially psoralens, are often used in the treatment of skin diseases such as vitiligo, alopecia areata, psoriasis, and neodermal diseases (Son et al., 2017). Psoralens and bergaptens have also demonstrated significant insecticidal activity properties (Chung et al., 2011; Siyadatpanah et al., 2022).
2.3 Terpenes
F. carica is rich in terpenes. Carotenoids, which belong to the group of tetraterpenoids, are a large group of phytochemicals with powerful antioxidant activities (Kaulmann and Bohn, 2014). F. carica contains the carotenoids typically present in human plasma, including β-carotene, lutein, and lycopene (Su et al., 2002). Similar to anthocyanins, carotenoids contribute to the coloration of F. carica fruits, giving them an orange or yellow color. The carotenoid contents of fresh and dried fruits differ. B-carotene, zeaxanthin, and lutein are predominant in fresh F. carica and β-cryptoxanthin and lutein in dried F. carica (Arvaniti et al., 2019). These carotenoids can prevent cardiovascular disease by reducing blood pressure, preventing oxidative stress, and decreasing the secretion of pro-inflammatory factors (Mordente et al., 2011).
Triterpenoids and sesquiterpenes are abundant in the extracts of F. carica root, leaf, and latex. Dried and young F. carica fruits contain the triterpenoid 9,19-cyclopropyl-24,25-ethylene-5ene-3β-spirosterol, which has anticancer activity (Yin et al., 1997). The main sesquiterpenes in F. carica leaf are genanthene, β-caryophyllene, and τ-elemiene (Oliveira et al., 2010c). β-Caryophyllene is used in cosmetics for its spicy aroma (Reinsvold, 2010). Sesquiterpenes are also the most abundant terpenes in F. carica latex, accounting for 91% of all identified compounds.
Fresh latex from F. carica has been used to treat a variety of types of cancers (Oliveira et al., 2010b; Arvaniti et al., 2019).
3 Accumulation and distribution of secondary metabolites in F. carica
3.1 Varieties (cultivars)
The accumulation and distribution of secondary metabolites vary markedly among F. carica cultivars (Table 1). In general, dark F. carica varieties exhibit higher polyphenol content and total anthocyanin content. Compared with those with light-colored peels, such as green and yellowish-green peels, cultivars with black and purple peels have higher levels of phenolics, flavonoids, and anthocyanins (Çalişkan and Polat, 2011; Ercişli et al., 2012; Hssaini et al., 2020). ‘Bursa siyahi’ (purple) F. carica shows a much higher total proanthocyanidin content than ‘Sarilop’ (yellow) F. carica (yellow) (Kamiloglu and Çapanoğlu, 2015). However, some light-colored F. carica varieties exhibit slightly higher proanthocyanidin content (Hssaini et al., 2020). Additionally, varieties of different colors reportedly share some similar anthocyanin profiles, principally cyanidin-3-O-rutinoside (> 90% of total anthocyanins). Moreover, varieties with black peels have high levels of total phenolic compounds, and those with green, greenish-yellow, and brown fruit peels have high levels of chlorogenic acid, vitamin C, and total tannins (Solomon et al., 2006; Pereira et al., 2017).
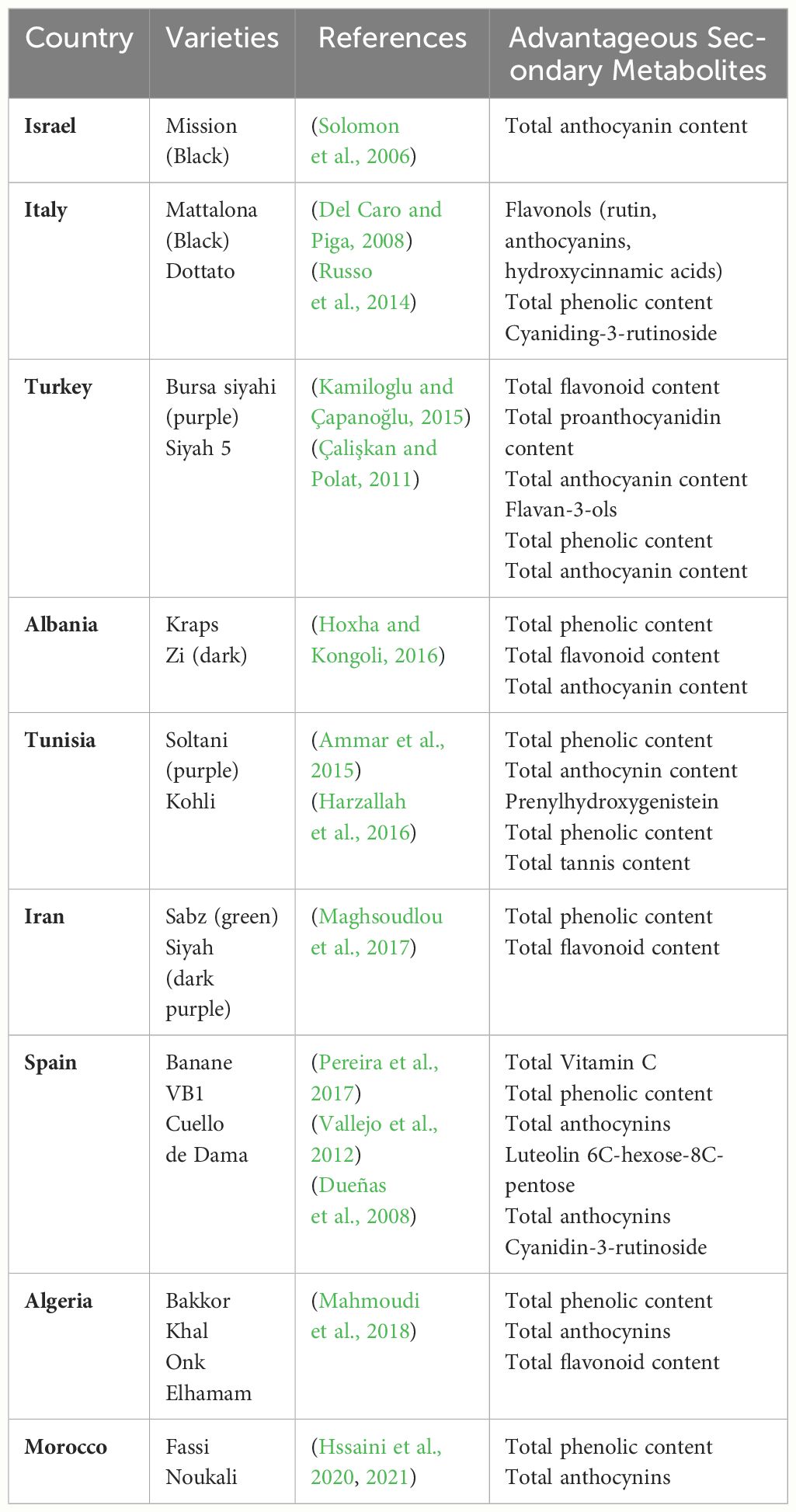
Table 1 Advantageous secondary metabolites in the main cultivated F. carica varieties in fruit peel.
Among the diverse array of F. carica varieties, certain varieties have shown exceptional potential. Notably, in the ‘Dottato’ and ‘Cuello de Dama’ varieties from Italy, cyanidin-3-rutinoside, a type of anthocyanin, was identified within the fruit’s pulp (Russo et al., 2014). Additionally, the ‘Boughandjo’, a high-yielding variety, and the ‘Bither’, an early-ripening variety from Algeria, although not the richest in secondary metabolites, have gained consumer favor for their taste (Mahmoudi et al., 2018). Moreover, cluster analysis of 37 F. carica varieties has identified ‘Grise de Saint Jean’ and ‘Grise de Tarascon’ as having minimal levels of furanocoumarins (which cause skin inflammation), making them promising candidates for the development of functional foods and pharmaceutical products (Takahashi et al., 2017).
3.2 Tissues
Different tissues of F. carica have different secondary metabolite profiles (Table 2). Previous studies have focused more on the secondary metabolites found in the fruits, pulp, peel, leaves, and latex of F. carica, with only a limited number of studies exploring the roots and bark.
F. carica fruits serve as a rich reservoir of flavonoids, which notably include quercetin 3-O-rutinoside, quercetin-3-glucosides, polymeric procyanidins, and cyanidin-3-O-rutinoside among their key constituents (Mopuri and Islam, 2016). Quercetin-3-O-rutinoside (rutin) is the predominant flavonoid found in light-colored F. carica peel, whereas cyanidin-3-O-rutinoside is the primary flavonoid present in dark-colored F. carica peel (Palmeira et al., 2019; Calani et al., 2022). Within fruits, the peel is richer in secondary metabolites than the pulp. For example, the anthocyanin content of the peel of the dark-colored ‘Mission’ variety was 100-fold that of the pulp (Solomon et al., 2006). Portuguese F. carica variety had higher levels of nutritional and phenolic components 158 (Palmeira et al., 2019). Interestingly, an exception is observed in the green F. carica varieties ‘Bidhi’ and ‘Kadota’, where the concentration of total phenolics in the edible pulp significantly surpasses that found in the peel (Mahmoudi et al., 2018). However, in the F. carica processing industry, peels are frequently discarded as waste, highlighting the importance of studying the bioactivity of F. carica peels and the need to develop and utilize by-products.
Notably, the fruit pulp is a significantly richer source of prenylated flavonoids compared to the fruit peel (Ammar et al., 2015; Liu et al., 2019). A study analyzed the phenolic composition of leaves, fruit, peel, and pulp, identifying an isomer of prenylhydroxygenistein as the primary compound in the pulps (Ammar et al., 2015). This compound, a type of prenylated flavonoids, is known for its anti-inflammatory and cancer-preventive activities, making it a valuable constituent for the development of functional foods (Chang et al., 2021).
The bioactive compounds present in F. carica leaves were extensively summarized in a previous review (Li et al., 2021). Leaves are found to be more abundant in secondary metabolites compared to their fruit counterparts. Overall, F. carica leaves are a good source of flavonoids, coumarins, and terpenes. Rutin is the main flavonoid in F. carica leaves, and psoralen is the main furanocoumarin compound (Ammar et al., 2015). An experiment measuring coumarin content in leaves, bark, and the woody part of F. carica showed that the leaves contain higher levels of psoralen and bergapten (Conforti et al., 2012). Psoralen and bergapten are also major coumarin compounds in the root heartwood (Jaina et al., 2013). Recent research on the phenolic composition of F. carica bark identified rutin as the predominant compound (Yahiaoui et al., 2024). In addition, compared with pulp and peel, F. carica leaves contain a notably higher content of sesquiterpenes, with β-Caryophyllene and δ-Elemene as the main components, but monoterpenes are rare (Oliveira et al., 2010c).
Latex is rich in coumarins and has a large amount of sterols. A hexane extract of Tunisian common ‘Jrani caprifig’ latex identified 36 compounds, 14 of which were coumarins (Lazreg-Aref et al., 2012). A total of 7 phytosterols were identified, among which β-sitosterol and lupeol were the compounds with higher concentrations (Oliveira et al., 2010a). Notably, a mixture of 6-O-acyl-beta-D-glucosyl-beta-sitosterols, which has a significant inhibitory effect on cancer cells, was only isolated from latex (Rubnov et al., 2001).
4 Factors that affect the secondary metabolite biosynthesis and accumulation
4.1 Abiotic stresses
Environmental factors and stressors affect secondary metabolite accumulation in F. carica. Light influences the biosynthesis of anthocyanins in plants (Mao et al., 2023). Although light deprivation did not affect the coloration or anthocyanin content of F. carica female flower tissues, it significantly inhibited the biosynthesis of anthocyanins in fruit peel (Wang et al., 2019). In the bagged fruit (female flower tissue), 12 flavonoids were detected, of which 11 increased, and apigenin increased by 11 times (Wang et al., 2021). In addition, pulse light treatment using a high-energy xenon flashlight after harvesting resulted in a 20-fold increase in the anthocyanin content of F. carica fruit peel. Moreover, pulse light treatment increased the total phenolic content of fruit. Therefore, pulse light markedly promotes the accumulation of anthocyanins and other phenolic compounds in F. carica (Rodov et al., 2012).
Increased production of secondary metabolites can ameliorate stress-induced damage in plants (Figure 2). The total phenolic level of F. carica is increased by phosphorus and calcium deficiency (Garza-Alonso et al., 2020). Salt stress increased the total phenolic content of F. carica by 5.6%, with a particularly pronounced increase observed in the levels of epicatechin (Francini et al., 2021). Additionally, high temperatures increase the phenolic content of the leaves of F. carica seedlings (Guo et al., 2017), and drought stress increases the α-tocopherol content (Gholami et al., 2012). In a study of fresh, frozen, and processed fruit, storage of F. carica at −18°for 4 months resulted in significant decreases in the total phenolic, total flavonoid, and total anthocyanin contents (Petkova et al., 2019).
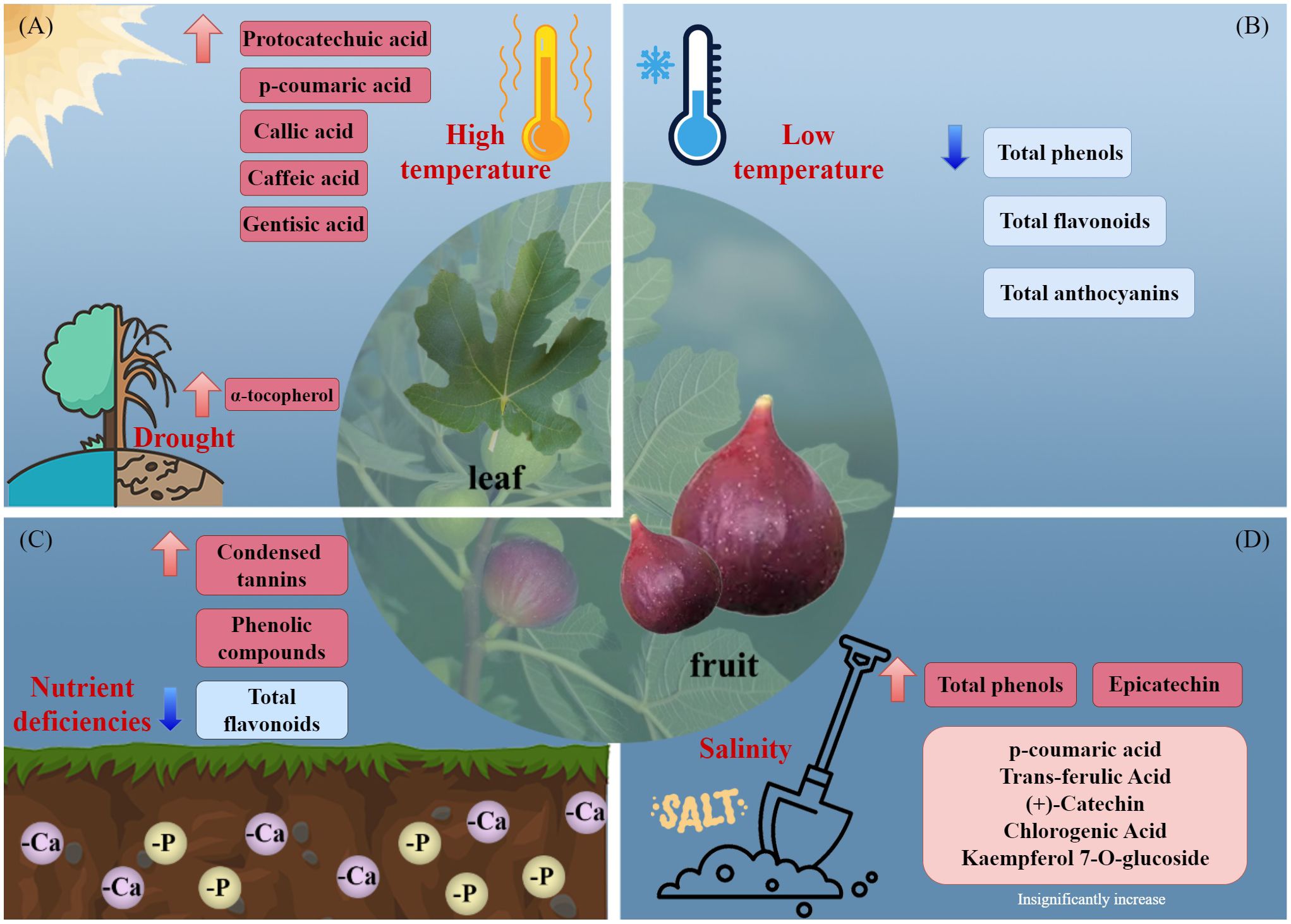
Figure 2 Effects of environmental stresses affect the accumulation of secondary metabolites. (A) high temperature and drought, (B) low temperature, (C) nutrient deficiencies, and (D) salinity.
4.2 Phytohormone treatments
Phytohormones related to stress resistance, such as abscisic acid (ABA), jasmonic acid, and ethylene, can modulate the biosynthesis of anthocyanins in F. carica (Figure 3) (Guo et al., 2017; Lama et al., 2019; Cui et al., 2021). ABA promotes the accumulation of anthocyanins during fruit ripening, thereby initiating color development (Lama et al., 2019). F. carica treated with ABA had higher levels of cyanidin 3-O-glucoside and cyanidin 3-O-rucoside and developed a dark-purple color earlier compared with those treated with an ABA inhibitor (Lama et al., 2020). Transcriptomic analysis indicated that ABA upregulated the expression levels of genes related to anthocyanin biosynthesis, whereas an ABA inhibitor reversed this effect, confirming the importance of ABA for the biosynthesis of anthocyanins. Ethylene is a phytohormone associated with plant maturation and is implicated in fruit development and stress responses. Ethylene has bidirectional regulatory effects on female flower and peel coloration in F. carica. Treatment of F. carica with exogenous ethylene upregulated the expression levels of genes related to flavonoid biosynthesis, thereby accelerating the coloration of the receptacle, whereas downregulation of these genes delayed the coloration of female tissues (Cui et al., 2019). In addition, F. carica seedlings treated with methyl jasmonate under high-temperature stress had elevated total phenolic contents (Guo et al., 2017).
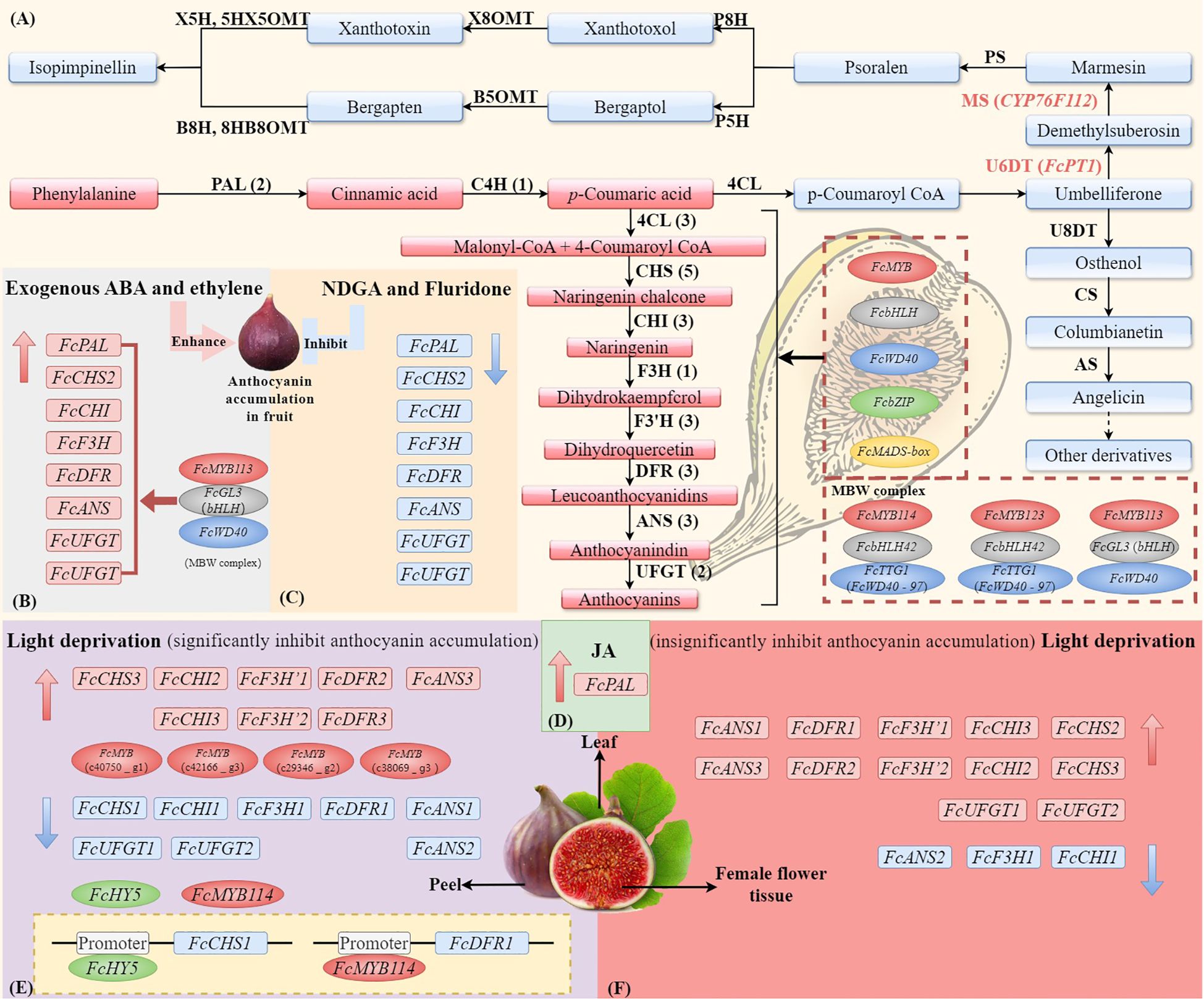
Figure 3 The synthesis pathways of anthocyanin and coumarin in F. carica and the external factors (ABA, light, and JA) affect the expression of genes and TFs. (A) The biosynthesis of anthocyanins in F. carica is regulated by MYB, bHLH, WD40, bZIP, and MADS-box transcription factors, as well as the MBW (MYB-bHLH-WD40) complex. Effects of exogenous abscisic acid (ABA) or ethylene (B), ABA inhibitors nordihydroguaiaretic acid (NDGA) or fluridone (C), and jasmonic acids (JAs) (D) on the expression of anthocyanin biosynthesis genes in fruit. Effects of light deprivation on anthocyanin biosynthesis genes in fig peel (E) and female flower tissue (F). The structural genes involved in the biosynthesis of anthocyanins include phenylalanine ammonium lyase (PAL), cinnamate-4-hydroxylase (C4H), 4-coumarate: coenzyme A ligase (4CL), chalcone synthase (CHS), chalcone isomerase (CHI), flavanone 3-hydroxylase (F3H), flavanone 3’-hydroxylase (F3’H), dihydroflavonol 4-reductase (DFR), anthocyanin synthetase (ANS), and UDP glucose: flavonoid 3-O-glucosyltransferase (UFGT). The simplified biosynthetic pathways of coumarin and furanocoumarin (FC). The core skeleton structures of linear and angular FCs are psoralen and angelicin. The key enzymes involved in the biosynthesis of FCs include umbelliferone 6-dimethylallyltransferase (U6DT), umbelliferone 8-dimethylallyltransferase (U8DT), marmesin synthase (MS), columbianetin synthase (CS), psoralen synthase (PS), angelicin synthase (AS), psoralen 5-hydroxylase (P5H), psoralen 8-hydroxylase (P8H), bergaptol 5-O-methyltransferase (B5OMT), xanthotoxol 8-O-methyltransferase (X8OMT), bergapten 8-hydroxylase (B8H), xanthotoxin 5-hydroxlyase (X5H), 8-hydroxybergapten 8-O-methyltransferase (8HB8OMT), and 5-hydroxyxanthotoxin 5-O-methyltransferase (5HX5OMT).
4.3 Developmental factors
Developmental and ripening stages affect the accumulation of secondary metabolites in F. carica fruit (Veberic et al., 2008). Some species of F. carica can ripen twice and be harvested twice annually: first from late May until the end of June (‘Breba’) and subsequently from mid-July to early September (main crop). In the F. carica cultivars ‘Zuccherina’, ‘Crna Petrovka’, and ‘Miljska’, fruits from the second ripening had higher levels of phenolic than those from the first ripening (Veberic et al., 2008). Similarly, the main crops of two ‘Albanian’ cultivars had higher phenolics contents compared with the ‘Brebas’ from the second ripening (Hoxha and Kongoli, 2016). However, in the cultivars ‘VB1’, ‘Antonio’, and ‘Santado’, the levels of phenolic compounds were higher in fruits from the first ripening than in those from the second ripening, suggesting that secondary metabolite accumulation varies among developmental and ripening stages (Vallejo et al., 2012). In addition, the contents of furanocoumarins and pyranocoumarins are higher in the first ripening in June, whereas those of polyphenolics are highest in the second ripening in September (Marrelli et al., 2012).
The types and levels of secondary metabolites also vary according to the maturity of F. carica fruit within a ripening stage. Among fruits at the immature, mature, and fully mature stages, the total phenolic content increased during maturation and peaked at the fully mature stage (Pereira et al., 2017). However, others have reported that the total phenolic content decreases with fruit development (Gündeli et al., 2021; Hoxha et al., 2022). In addition, the polyphenolic contents of dark-colored F. carica cultivars change more during ripening compared with light-colored cultivars (Wang et al., 2019). Overall, the accumulation of secondary metabolites in F. carica fruit is influenced by the developmental time, stage of maturity, and also depends on the cultivars.
4.4 Post-harvest processing techniques
As a result of its susceptibility to physical damage and post-harvest infection, fresh F. carica fruit is typically processed into dried fruits, jams, jellies, and syrups, as examples. These processes affect the secondary metabolite profiles of F. carica fruit (Liu et al., 2020). Drying, including sun- and oven-drying, is the most common method for preserving fresh F. carica fruit. Sun drying markedly reduces the levels of polyphenols in fruit, and the effect is greater on flavonoids than on phenolic acids. Sun drying decreased the contents of phenolic acids and flavonoids in fruit by 29% and 86%, respectively (Bachir et al., 2016). Similarly, the total phenolic and total anthocyanin contents of a yellow- and a purple-colored fruit variety decreased significantly after drying, but the total flavonoid and proanthocyanidin contents of the yellow fruit variety significantly increased (Kamiloglu and Çapanoğlu, 2015). However, the levels of phenolic compounds, except apigenin-3-O-rutinoside, were high after oven drying, suggesting that oven drying can increase the total phenolic content and the antioxidant activity of fruit (Slatnar et al., 2011). The processing of fruit pulp into jam and honey also reduces the content of secondary metabolites (Tanwar et al., 2014). Although nectar processing, and jam processing reduce the levels of secondary metabolites, considerable quantities, particularly of carotenoids and phenols, remain after jam processing (Tanwar et al., 2014; Petkova et al., 2019). This suggests that the processing of fruit into jams maintains, at least in part, their contents of phenolics and carotenoids.
5 Enzymes involved in secondary metabolism in F. carica
The first preliminary genome sequence of F. carica was obtained by sequencing the genome of the Japanese traditional variety ‘Horaishi’ based on the Illumina platform. The first high-density linkage map was constructed using restriction enzyme site-associated sequencing (Mori et al., 2017). Further sequencing and assembly of the F. carica genome, ‘Dottato’, have been conducted using third-generation sequencing technologies (Usai et al., 2020). This version of the genome assembly of F. carica comprises 333 Mbp, 80% of which is anchored to 13 chromosomes (Usai et al., 2020). Later, the newest genome of F. carica ‘Orphan’ was built, with a total length of 366.34 Mb and a contig N50 of 9.78 Mb (Bao et al., 2023). Generally, ten families of structural genes associated with the anthocyanin biosynthesis pathway have been identified: FcPAL (two genes), FcC4H (one gene), Fc4CL (three genes), FcCHS (five genes), FcCHI (three genes), FcF3H (one gene), FcF3’H (three genes), FcDFR (three genes), FcANS (three genes), and FcUFGT (two genes) (Li et al., 2020) (Figure 3).
5.1 Chalcone synthase and chalcone isomerase
Chalcone synthase (CHS) is a key enzyme that catalyzes the first committed step in the anthocyanin biosynthesis pathway (Jez and Noel, 2001). As the receptacle tissues of F. carica develop and ripen, the contents of flavonoids increase, and the expression level of FcCHS is upregulated (Cui et al., 2021). FcCHS1 encodes 389 amino acid residues, including two phenylalanine residues essential for substrate specificity (Li et al., 2020). In addition, the transcription of FcCHS1 can be regulated by light-induced signal transduction elements such as HY5 (Wang et al., 2019). Subsequent to the reaction catalyzed by CHS, chalcone isomerase (CHI) catalyzes the conversion of chalcone into naringenin (Ralston et al., 2005). FcCHI1 encodes a 257 amino-acid type I chalcone isomerase, which includes four conserved active sites (Li et al., 2020). The expression levels of FcCHS1 and FcCHI1 increase with the accumulation of anthocyanins and are significantly higher in pigmented tissues (fruit peels and flowers) than non-pigmented tissues. The expression of FcCHS1 is very low in non-pigmented tissues (Li et al., 2020). FcCHS1 and FcCHI1, together with their homologs associated with anthocyanin accumulation, have similar molecular characteristics and secondary structures, implicating their involvement in F. carica fruit coloration.
5.2 Dihydroflavonol-4-reductase
Dihydroflavonol-4-reductase (DFR) is a key enzyme involved in the later steps of anthocyanin biosynthesis. It catalyzes the conversion of dihydroflavonols to colorless anthocyanins. Metabolomic and transcriptomic analyses showed that the expression levels of three FcDFR genes were significantly increased in fruit peel. Among them, the expression levels of FcDFR2 and FcDFR3 were upregulated by light deprivation, whereas FcDFR1 was downregulated (Wang et al., 2019). FcDFR1 encodes a 363-amino-acid protein encompassing a typical NADP-binding region and a substrate-binding region (Li et al., 2020). The secondary structure of FcDFR1 is similar to MnDFR1 (Morus notabilis), which has been implicated in anthocyanin accumulation, suggesting that FcDFR1 is involved in the anthocyanin biosynthesis of F. carica.
5.3 Anthocyanidin synthase and UDP-glycose flavonoid glycosyltransferase
Anthocyanidin synthase (ANS) catalyzes the penultimate step in the anthocyanin biosynthesis pathway, the conversion of colorless into colored anthocyanins. FcANS1 encodes a 358-amino-acid protein encompassing a conserved domain 2OG-FeII-Oxy (Cao et al., 2016). FcANS1 was significantly upregulated in F. carica fruit peel compared with flower tissue (Wang et al., 2019). The expression pattern of FcANS1 and the anthocyanin content are correlated during fruit development. Bagging treatment resulted in downregulation of FcANS1 expression in fruit peel and female flowers, suggesting that FcANS1 functions in anthocyanin biosynthesis (Cao et al., 2016). On the contrary, three FcANS genes FcANS1, FcANS2 and FcANS3 in female flower tissues were upregulated after bagging (Wang et al., 2021).
UFGT is the last key enzyme in the anthocyanin synthesis pathway, which can catalyze the glycosylation of unstable anthocyanins to form stable anthocyanins. The open reading frame of FcUFGT1 is 1374 bp. The expression level of FcUFGT1 in the fruit peel of F. carica at the mature stage was much higher than that in female flower tissue. However, the expression of FcUFGT1 was significantly downregulated in the fruit peel by light deprivation but significantly upregulated in the female flower tissue (Wang et al., 2019).
5.4 Other enzymes
Glutathione S-transferases (GSTs) are multifunctional enzymes involved in the transport of anthocyanins to vacuoles (Gullner et al., 2018). A total of 53 GST genes from five subfamilies are present in the F. carica genome. FcGSTF1 and FcGSTU5/6/7 may be important in anthocyanin accumulation in F. carica peel, but further research is needed (Liu et al., 2023).
Compared to anthocyanins, less research has focused on the regulation of the biosynthesis of other secondary metabolites in F. carica. Furanocoumarins (FCs), like anthocyanins, are synthesized via the phenylpropanoid pathway and are structurally classified as angular and linear furanocoumarins (Villard et al., 2019). The first step of the furanocoumarin pathway is catalyzed by the umbelliferone dimethylallyl transferase (UDT) and is important for the prenylation of umbelliferone (Figure 3). Comparative RNA-seq and enzyme activity analyses of F. carica latex organs have shown that FcPT1 has UDT activity and participates in the biosynthesis of furanocoumarins in F. carica, converting umbelliferone into demethylsuberosin (DMS) (Munakata et al., 2020). However, the functions of these enzymes need to be validated by in vitro studies. Marmesin synthase is a key enzyme in the biosynthesis of furanocoumarins and is involved in the production of the defense compound psoralen from p-coumaroyl coenzyme A (Villard et al., 2021). The cytochrome P450 gene CYP76F112 in F. carica encodes marmesin synthase, which can convert DMS into marmesin with high affinity. The discovery of CYP76F112, the first identified marmesin synthase, expands the potential applications of the furancoumarin pathway (Villard et al., 2021).
6 Transcription factors regulating the biosynthesis of secondary metabolites in F. carica
Transcription factors modulate the expression of structural genes associated with the biosynthesis of secondary metabolites. They bind to cis-acting regions, thereby inhibiting or promoting gene expression. These factors collaboratively or independently regulate the synthesis of enzymes involved in the biosynthesis of secondary metabolites, such as anthocyanins.
6.1 MYB transcription factors
MYB transcription factors are widely distributed in plants and are involved in a variety of aspects of plant growth and metabolism (Pratyusha and Sarada, 2022). Transcriptomic analysis revealed that light deprivation alters the expression of several F. carica genes, such as MYB114 and three R2R3MYB transcription factors, with FcMYB114 specifically regulating FcDFR1 and anthocyanin biosynthesis via promoter interaction (Wang et al., 2019). Virus-induced gene silencing of FcMYB114 led to significant downregulation of FcDFR1, FcANS1, and FcUFGT1, resulting in a reduction of anthocyanin content in F. carica fruit peel (Chen et al., 2023). Overexpression of two R2R3-MYB genes, FcMYB21 and FcMYB123, led to significantly increased anthocyanin content in apple peel and fruit callus tissues (Li et al., 2020). Furthermore, the significant promotion of MdMYC2 expression by overexpressing FcMYB21 suggests a potential close relationship between FcMYB21 and FcMYC2 in promoting F. carica fruit coloring.
6.2 Basic helix-loop-helix transcription factors
The bHLH family is the second largest group of plant transcription factors involved in plant growth, development, and signal transduction. bHLH transcription factors can interact with MYB transcription factors to regulate the biosynthesis of anthocyanins (Hichri et al., 2010). The genome of F. carica contains 118 bHLH genes (Song et al., 2021). According to the bHLH family classification in Arabidopsis, FcbHLH genes can be classified into 25 subgroups. bHLH genes of different subfamilies show different expression patterns in female flower tissues and fruit peel during F. carica fruit development. The transient transformation of FcbHLH42 in tobacco induced a significant increase in anthocyanin content, suggesting that FcbHLH42 promotes the accumulation of anthocyanins in F. carica. The levels of WD40 and other MYB transcription factors were positively correlated with the expression of FcbHLH42, providing insight into the regulatory mechanism of flavonoid biosynthesis in F. carica (Song et al., 2021).
6.3 WD40
The WD40 transcription factors are structurally stable and important regulators of plant development and physiology, including the biosynthesis of anthocyanins. TTG1 was the first WD40 protein discovered in Arabidopsis thaliana, and it forms a trimer with AtMYB123 and AtbHLH42 to promote anthocyanin biosynthesis (Baudry et al., 2004). The F. carica genome contains a total of 204 WD40 genes. Subcellular localization prediction showed that 109 FcWD40 proteins were localized to the cytoplasm, 69 to the nucleus, and 26 to other cellular compartments. FcWD4097 has been identified and named FcTTG1. The expression level of FcTTG1 was significantly highest in the flesh and peel, followed by the stem of F. carica (Fan et al., 2022).
MYB transcription factors form the MBW complex by binding to the FcMYB113, FcGL3, and FcWD40 proteins of the MYB, bHLH, and WD-repeat families, respectively. This complex directly activates the expression of genes involved in the biosynthesis of anthocyanins (Gonzalez et al., 2008). The MBW complex was identified in pollinated and parthenocarpic F. carica fruits. FcMYB113 was significantly downregulated, whereas FcGL3 and FcWD40 were moderately upregulated by exogenous ABA and ethylene. The high expression of FcMYB113, FcGL3, and FcWD40 during the later stages of anthocyanin biosynthesis may affect coloration (Lama et al., 2020). In addition, yeast two-hybrid assays and biomolecular fluorescence complementation experiments showed that FcWD40–97 and FcbHLH42 regulate the biosynthesis of anthocyanins by forming MBW complexes with FcMYB114 and FcMYB123 (Fan et al., 2022).
6.4 Other transcription factors
Other families of transcription factors, such as MADS-box and bZIP, have been identified in F. carica. MADS-box proteins are functionally diverse and regulate multiple processes, including floral organ development, fruit development and maturation, and seed coloring (Aerts et al., 2018). The F. carica genome has a total of 64 FcMADS-box genes, the expression levels of which were shown to be tissue specific (Kmeli et al., 2023). FcMADS9 has been cloned, and its regulation of the biosynthesis of anthocyanins in F. carica has been validated by heterologous expression in apple fruit and callus tissues (Li et al., 2021).
The bZIP family is one of the largest gene families in plants and is important for biological processes such as secondary metabolism, stress responses, and seed maturation. Y1 screening indicated that FcHY5 (bZIP) binds to the promoter region of FcCHS, implicating the latter in transcription of the structural genes linked to the biosynthesis of anthocyanins (Wang et al., 2019).
7 Concluding remarks and future perspectives
We have conducted a comprehensive review of the functions and biosynthesis of secondary metabolites in the economically significant F. carica. A diverse array of secondary metabolites in F. carica, such as flavonoids, terpenes, and coumarins, exhibit various beneficial activities. Dark-colored F. carica varieties and the fruit peel display elevated levels of secondary metabolites.
To fully exploit the potential application of these metabolites in food and medicine, it is crucial to acquire a more in-depth understanding of their biological activity and pharmacological efficacy. The functions of the flavonoids, terpenoids, and coumarins of F. carica require further investigation in cell-culture experiments, animal models, and clinical studies to identify potential health benefits. In addition, while F. carica is primarily consumed as fruit, the leaves contain higher levels of coumarin compounds, which are usually discarded as industrial byproducts without utilization; therefore, further research on the use and value of these byproducts is necessary.
The content of secondary metabolites undergoes substantial changes during the development and maturation of F. carica fruits, and it is also influenced by the genotype of F. carica. Additionally, a range of environmental factors (e.g., light), stresses (e.g., high temperature, low temperature, drought, nutrient deficiencies, salinity), hormone treatments (e.g., ABA, ethylene, NDGA, fluridone, and methyl jasmonate), as well as developmental factors, can affect the accumulation of secondary metabolites in F. carica. Post-harvest processing methods are worth further exploration as a low-cost and practical technique to maximize the retention of secondary metabolites in F. carica. Some cultivation and management practices can be applied to F. carica to achieve better fruit quality, including artificial light supplementation systems, fertilizer rationing, and the application of growth regulators.
Anthocyanins, as secondary metabolites in F. carica, play a significant role in determining the fruit’s appearance and nutritional value. The availability of the F. carica genome has facilitated the investigation of key genes involved in anthocyanin biosynthesis and their regulatory mechanisms. Transcriptomic and bioinformatics analyses have identified several structural genes, including FcPAL, FcCHS, FcCHI, FcF3H, FcDFR, FcANS, FcUFGT, and FcGST, which regulate anthocyanin synthesis. Furthermore, CYP76F112 and FcPT1 have been identified as crucial enzymes in the coumarin compound synthesis pathway. Moreover, MYB, bHLH, WD40, bZIP, and MADS-box transcription factors are implicated in the regulation of the biosynthesis of anthocyanins. Genetic and molecular studies are needed to further identify the functional genes involved in the regulation of secondary metabolite biosynthesis. The application of genetic transformation systems (root hair transformation, virus-induced gene silencing, and gene editing) will facilitate the development of biotechnological techniques for increased contents of bioactive secondary metabolites in F. carica varieties. Studies on the biosynthesis mechanisms of anticancer bioactive compounds such as psoralens and saponins in F. carica warrant further research.
Author contributions
YW: Writing – original draft, Writing – review & editing, Visualization. XL: Writing – review & editing, Visualization. SC: Writing – review & editing. QW: Writing – review & editing. BJ: Writing – review & editing. LW: Conceptualization, Writing – original draft, Writing – review & editing.
Funding
The author(s) declare that no financial support was received for the research, authorship, and/or publication of this article.
Conflict of interest
The authors declare that the research was conducted in the absence of any commercial or financial relationships that could be construed as a potential conflict of interest.
Publisher’s note
All claims expressed in this article are solely those of the authors and do not necessarily represent those of their affiliated organizations, or those of the publisher, the editors and the reviewers. Any product that may be evaluated in this article, or claim that may be made by its manufacturer, is not guaranteed or endorsed by the publisher.
References
Abdel-Aty, A. M., Hamed, M. B., Salama, W. H., Ali, M., Fahmy, A. S., Mohamed, S. A. (2019). Ficus carica, Ficus sycomorus and Euphorbia tirucalli latex extracts: Phytochemical screening, antioxidant and cytotoxic properties. Biocatal. Agric. Biotechnol. 20, 101199. doi: 10.1016/J.BCAB.2019.101199
Aerts, N., de Bruijn, S., van Mourik, H., Angenent, G. C., van Dijk, A. D. J. (2018). Comparative analysis of binding patterns of MADS-domain proteins in Arabidopsis thaliana. BMC Plant Biol. 18, 131. doi: 10.1186/s12870–018-1348–8
Ammar, S., del Mar Contreras, M., Belguith-Hadrich, O., Belguith-Hadrich, O., Segura-Carretero, A., Bouaziz, M. (2015). Assessment of the distribution of phenolic compounds and contribution to the antioxidant activity in Tunisian fig leaves, fruits, skins and pulps using mass spectrometry-based analysis. Food Funct. 6, 3663–3677. doi: 10.1039/c5fo00837a
Arvaniti, O. S., Samaras, Y., Gatidou, G., Thomaidis, N. S., Stasinakis, A. S. (2019). Review on fresh and dried figs: Chemical analysis and occurrence of phytochemical compounds, antioxidant capacity and health effects. Food Res. Int. 119, 244–267. doi: 10.1016/j.foodres.2019.01.055
Bachir, B. M., Richard, G., Meziant, L., Fauconnier, M. L., Louaileche, H. (2016). Effects of sun-drying on physicochemical characteristics, phenolic composition and in vitro antioxidant activity of dark fig varieties. J. Food Process. Preserv. 41, e13164. doi: 10.1111/jfpp.13164
Bao, Y., He, M., Zhang, C., Jiang, S., Zhao, L., Ye, Z., et al. (2023). Advancing understanding of Ficus carica: a comprehensive genomic analysis reveals evolutionary patterns and metabolic pathway insights. Front. Plant Sci. 14. doi: 10.3389/fpls.2023.1298417
Baudry, A., Heim, M. A., Dubreucq, B., Caboche, M., Weisshaar, B., Lepiniec, L. (2004). TT2, TT8, and TTG1 synergistically specify the expression of BANYULS and proanthocyanidin biosynthesis in Arabidopsis thaliana. Plant J. 39, 366–380. doi: 10.1111/j.1365–313X.2004.02138.x
Calani, L., Bresciani, L., Rodolfi, M., Del Rio, D., Petruccelli, R., Faraloni, C., et al. (2022). Characterization of the (poly)phenolic fraction of fig peel: comparison among twelve cultivars harvested in Tuscany. Plants 11, 3073. doi: 10.3390/plants11223073
Çalişkan, O., Polat, A. A. (2011). Phytochemical and antioxidant properties of selected fig (Ficus carica l.) accessions from the eastern Mediterranean region of Turkey. Sci. Hortic. 128, 473–478. doi: 10.1016/j.scienta.2011.02.023
Cao, L., Xu, X., Chen, S., Ma, H. (2016). Cloning and expression analysis of Ficus carica anthocyanidin synthase 1 gene. Sci. Hortic. 211, 369–375. doi: 10.1016/j.scienta.2016.09.015
Castañeda-Ovando, A., Pacheco-Hernández, M. D. L., Páez-Hernández, M. E., Rodríguez, J. A., Galán-Vidal, C. A. (2009). Chemical studies of anthocyanins: a review. Food Chem. 113, 859–871. doi: 10.1016/j.foodchem.2008.09.001
Chang, S. K., Jiang, Y., Yang, B. (2021). An update of prenylated phenolics: Food sources, chemistry and health benefits. Trends Food Sci. Tech. 108, 197–213. doi: 10.1016/j.tifs.2020.12.022
Chen, Z. Y., Gao, W. K., Li, M. H., He, D. N., Long, Y. D., Huang, L., et al. (2023). Function of FcMYB114 gene in fig (Ficus carica L.) by virus-induced gene silencing. Southwest. China J. Agric. Sci. 36, 623–628. doi: 10.16213/j.cnki.scjas.2023.3.021
Chung, I. M., Kim, S. J., Yeo, M. A., Park, S. W., Moon, H. I. (2011). Immunotoxicity activity of natural furocoumarins from milky sap of Ficus carica L. against Aedes aEgypti L. Immunopharmacol. Immunotoxicol. 33, 515–518. doi: 10.3109/08923973.2010.543907
Conforti, F., Menichini, G., Zanfini, L., Tundis, R., Statti, G. A., Provenzano, E., et al. (2012). Evaluation of phototoxic potential of aerial components of the fig tree against human melanoma. Cell Prolif. 45, 279–285. doi: 10.1111/j.1365–2184.2012.00816.x
Cui, Y., Wang, Z., Chen, S., Vainstein, A., Ma, H. (2019). Proteome and transcriptome analyses reveal key molecular differences between quality parameters of commercial-ripe and tree-ripe fig (Ficus carica L.). BMC Plant Biol. 19, 146. doi: 10.1186/s12870–019-1742-x
Cui, Y., Zhai, Y., Flaishman, M., Li, J., Chen, S., Zheng, C., et al. (2021). Ethephon induces coordinated ripening acceleration and divergent coloration responses in fig (Ficus carica L.) flowers and receptacles. Plant Mol. Biol. 105, 347–364. doi: 10.1007/s11103–020-01092-x
Deepa, P., Sowndhararajan, K., Kim, S., Park, S. J. (2018). A role of Ficus species in the management of diabetes mellitus: a review. J. Ethnopharmacol. 215, 210–232. doi: 10.1016/j.jep.2017.12.045
Del Caro, A., Piga, A. (2008). Polyphenol composition of peel and pulp of two Italian fresh fig fruits cultivars (Ficus carica L.). Eur. Food Res. Technol. 226, 715–719. doi: 10.1007/s00217–007-0581–4
Dueñas, M., Pérez-Alonso, J. J., Santos-Buelga, C., Escribano-Bailón, T. (2008). Anthocyanin composition in fig (Ficus carica L.). J. Food. Compost. Anal. 21, 107–115. doi: 10.1016/j.jfca.2007.09.002
Dureshahwar, K., Mubashir, M., Upaganlwar, A., Sangshetti, J. N., Upasani, C. D., Une, H. D. (2019). Quantitative assessment of tactile allodynia and protective effects of flavonoids of Ficus carica Lam. leaves in diabetic neuropathy. Pharmacogn. Mag. 15, 128–134. doi: 10.4103/pm.pm_553_18
Ercişli, S., Tosun, M., Karlidag, H., Dzubur, A., Hadziabulic, S., Aliman, Y. (2012). Color and antioxidant characteristics of some fresh fig (Ficus carica L.) genotypes from northeastern Turkey. Plant Foods. Hum. Nutr. 67, 271–276. doi: 10.1007/s11130–012-0292–2
Fan, Z., Zhai, Y., Wang, Y., Zhang, L., Song, M., Flaishman, M. A., et al. (2022). Genome-wide analysis of anthocyanin biosynthesis regulatory WD40 gene FcTTG1 and related family in Ficus carica L. Front. Plant Sci. 13. doi: 10.3389/fpls.2022.948084
Francini, A., Sodini, M., Vicario, G., Raffaelli, A., Gucci, R., Caruso, G., et al. (2021). Cations and phenolic compounds concentrations in fruits of fig plants exposed to moderate levels of salinity. Antioxid 10, 1865. doi: 10.3390/antiox10121865
Garza-Alonso, C. A., Niño-Medina, G., Gutiérrez-Díez, A., García-López, J. I., Vázquez-Alvarado, R. E., López-Jiménez, A., et al. (2020). Physicochemical characteristics, minerals, phenolic compounds, and antioxidant capacity in fig tree fruits with macronutrient deficiencies. Not. Bot. Horti. Agrobo. 48, 1585–1599. doi: 10.15835/nbha48311867
Gholami, M., Rahemi, M., Kholdebarin, B., Rastegar, S. (2012). Biochemical responses in leaves of four fig cultivars subjected to water stress and recovery. Sci. Hortic. 148, 109–117. doi: 10.1016/j.scienta.2012.09.005
Ginwala, R., Bhavsar, R., Chigbu, D., Jain, P., Khan, Z. K. (2019). Potential role of flavonoids in treating chronic inflammatory diseases with a special focus on the anti-inflammatory activity of apigenin. Antioxid 8, 35. doi: 10.3390/antiox8020035
Gonzalez, A., Zhao, M., Leavitt, J. M., Lloyd, A. M. (2008). Regulation of the anthocyanin biosynthetic pathway by the TTG1/bHLH/Myb transcriptional complex in Arabidopsis seedlings. Plant J. 53, 814–827. doi: 10.1111/j.1365–313X.2007.03373.x
Gullner, G., Komives, T., Király, L., Schröder, P. (2018). Glutathione s-transferase enzymes in plant-pathogen interactions. Front. Plant Sci. 9. doi: 10.3389/fpls.2018.01836
Gündeli, M. A., Kafkas, N. E., Güney, M., Ercişli, S. (2021). Determination of phytochemicals from fresh fruits of fig (Ficus carica L.) at different maturity stages. Acta Sci. Pol. Hortorum. Cultus. 20, 73–81. doi: 10.24326/asphc.2021.2.8
Guo, Z. B., Han, B. M., Guo, Q. (2017). Effects of methyl jasmonate on high temperature stress and biological encroachment of fig seedlings. JiangSu. Agric. Sci. 45, 138–143. doi: 10.15889/j.issn.1002–1302.2017.20.033
Hajam, T. A., Saleem, H. (2022). Phytochemistry, biological activities, industrial and traditional uses of fig (Ficus carica): A review. Chem. Biol. Interact. 368, 110237. doi: 10.1016/j.cbi.2022.110237
Hao, G., Dong, Y., Huo, R., Wen, K., Zhang, Y., Liang, G. (2016). Rutin inhibits neuroinflammation and provides neuroprotection in an experimental rat model of subarachnoid hemorrhage, possibly through suppressing the RAGE-NF-κB inflammatory signaling pathway. Neurochem. Res. 41, 1496–1504. doi: 10.1007/s11064–016-1863–7
Harzallah, A., Bhouri, A. M., Amri, Z., Soltana, H., Hammami, M. (2016). Phytochemical content and antioxidant activity of different fruit parts juices of three figs (Ficus carica L.) varieties grown in Tunisia. Ind. Crops Prod. 83, 255–267. doi: 10.1016/j.indcrop.2015.12.043
Hichri, I., Heppel, S. C., Pillet, J., Léon, C., Czemmel, S., Delrot, S., et al. (2010). The basic helix-loop-helix transcription factor MYC1 is involved in the regulation of the flavonoid biosynthesis pathway in grapevine. Mol. Plant 3, 509–523. doi: 10.1093/mp/ssp118
Hoxha, L., Kongoli, R. (2016). Evaluation of antioxidant potential of Albanian fig varieties “Kraps Zi” and “Kraps Bardhe” cultivated in the region of Tirana. Int. J. Hydrog. Energy 16, 70–74.
Hoxha, L., Kongoli, R., Dervishi, J. (2022). Influence of maturity stage on polyphenolic content and antioxidant activity of fig (Ficus carica L.) fruit in native Albanian varieties. Chem. Proc. 10, 49. doi: 10.3390/IOCAG2022–12199
Hssaini, L., Charafi, J., Razouk, R., Hernández, F., Hanine, H. (2020). Assessment of morphological traits and fruit metabolites in eleven fig varieties (Ficus carica L.). Int. J. Fruit Sci. 20, 8–28. doi: 10.1080/15538362.2019.1701615
Hssaini, L., Elfazazi, K., Razouk, R., Ouaabou, R., Hernandez, F., Hanine, H., et al. (2021). Combined effect of cultivar and peel chromaticity on figs’ primary and secondary metabolites: preliminary study using biochemical and ftir fingerprinting coupled to chemometrics. Biology 10, 573. doi: 10.3390/biology10070573
Jaina, R., Jain, S., Bhagchandani, T., Yadav, N. (2013). New furanocoumarins and other chemical constituents from Ficus carica root heartwood. Z. für. Naturforschung. C. 68, 3–7. doi: 10.1515/znc-2013–1-201
Jez, J., Noel, J. (2001). Mechanism of chalcone synthase - pK(a) of the catalytic cysteine and the role of the conserved histidine in a plant polyketide synthase. J. Biol. Chem. 275, 39640–39646. doi: 10.1074/jbc.M008569200
Jucá, M., Cysne, F. F., de Almeida, J. C., Mesquita, D. D. S., Barriga, J. R. M., Dias, K. C. F., et al. (2020). Flavonoids: biological activities and therapeutic potential. Nat. Prod. Res. 34, 692–705. doi: 10.1080/14786419.2018.1493588
Kamiloglu, S., Çapanoğlu, E. (2015). Polyphenol content in figs (Ficus carica L.): effect of sun-drying. Int. J. Food Prop. 18, 521–535. doi: 10.1080/10942912.2013.833522
Kaulmann, A., Bohn, T. (2014). Carotenoids, inflammation, and oxidative stress–implications of cellular signaling pathways and relation to chronic disease prevention. Nutr. Res. 34, 907–929. doi: 10.1016/j.nutres.2014.07.010
Kmeli, N., Hamdi, J., Bouktila, D. (2023). Genome-wide characterization of Ficus carica MADS-box transcription factors with a focus on their roles during fruit development. Hortic. Environ. Biotechnol. 64, 311–329. doi: 10.1007/s13580–022-00478–8
Lama, K., Harlev, G., Shafran, H., Peer, R., Flaishman, M. A. (2020). Anthocyanin accumulation is initiated by abscisic acid to enhance fruit color during fig (Ficus carica L.) ripening. J. Plant Physiol. 251, 153192. doi: 10.1016/j.jplph.2020.153192
Lama, K., Yadav, S., Rosianski, Y., Shaya, F., Lichter, A., Chai, L., et al. (2019). The distinct ripening processes in the reproductive and non-reproductive parts of the fig syconium are driven by ABA. J. Exp. Bot. 70, 115–131. doi: 10.1093/jxb/ery333
Lazreg-Aref, H., Mars, M., Fekih, A., Aouni, M., Said, K. (2012). Chemical composition and antibacterial activity of a hexane extract of Tunisian caprifig latex from the unripe fruit of Ficus carica. Pharm. Biol. 50, 407–412. doi: 10.3109/13880209.2011.608192
Li, J., An, Y., Wang, L. (2020). Transcriptomic analysis of Ficus carica peels with a focus on the key genes for anthocyanin biosynthesis. Int. J. M. Sci. 21, 1245. doi: 10.3390/ijms21041245
Li, J., Ma, N., An, Y., Wang, L. (2021). FcMADS9 of fig regulates anthocyanin biosynthesis. Sci. Hortic. 278, 109820. doi: 10.1016/j.scienta.2020.109820
Liu, Y. P., Guo, J. M., Yan, G., Zhang, M. M., Zhang, W. H., Qiang, L., et al. (2019). Anti-inflammatory and antiproliferative prenylated isoflavone derivatives from the fruits of Ficus carica. J. Agric. Food. Chem. 67, 4817–4823. doi: 10.1021/acs.jafc.9b00865
Liu, H. P., Li, D., Xu, W. C., Fu, Y., Liao, R., Shi, J., et al. (2020). Effect of packaging design with two functional films on the quality of figs stored at ambient temperature. J. Food Process. Preserv. 44, e14836. doi: 10.1111/jfpp.14836
Liu, L., Zheng, S., Yang, D., Zheng, J. (2023). Genome-wide in silico identification of glutathione S-transferase (GST) gene family members in fig (Ficus carica L.) and expression characteristics during fruit color development. PeerJ 11, e14406. doi: 10.7717/peerj.14406
Magalhães, A. F., Tozzi, A. M. G. A., Magalhães, E. G., Souza-Neta, L. C. (2006). New prenylated metabolites of Deguelia longeracemosa and evaluation of their antimicrobial potential. Planta. Med. 72, 358–363. doi: 10.1055/s-2005–916232
Maghsoudlou, E., Esmaeilzadeh Kenari, R., Raftani Amiri, Z. (2017). Evaluation of antioxidant activity of fig (Ficus carica) pulp and skin extract and its application in enhancing oxidative stability of canola oil. J. Food Process. Pres. 41, e13077. doi: 10.1111/jfpp.13077
Mahmoudi, S., Khali, M., Benkhaled, A., Boucetta, I., Dahmani, Y., Attallah, Z., et al. (2018). Fresh figs (Ficus carica L.): pomological characteristics, nutritional value, and phytochemical properties. Eur. J. Hortic. Sci. 83, 104–113. doi: 10.17660/eJHS.2018/83.2.6
Mao, D., Zhong, L., Zhao, X., Wang, L. (2023). Function, biosynthesis, and regulation mechanisms of flavonoids in Ginkgo biloba. Fruit Res. 3, 18. doi: 10.48130/FruRes-2023–0018
Marrelli, M., Menichini, F., Statti, G. A., Bonesi, M., Duez, P., Menichini, F., et al. (2012). Changes in the phenolic and lipophilic composition, in the enzyme inhibition and antiproliferative activity of Ficus carica L. cultivar Dottato fruits during maturation. Food Chem. Toxicol. 50, 726–733. doi: 10.1016/j.fct.2011.12.025
Mawa, S., Husain, K., Jantan, I. (2013). Ficus carica L. (Moraceae): Phytochemistry, traditional uses and biological activities. Evid. Based. Complementary. Altern. Med. 2013, 974256. doi: 10.1155/2013/974256
Mopuri, R., Islam, M. S. (2016). Antidiabetic and anti-obesity activity of Ficus carica: in vitro experimental studies. Diabetes Metab. J. 42, 300–300. doi: 10.1016/j.diabet.2016.07.020
Mordente, A., Guantario, B., Meucci, E., Silvestrini, A., Lombardi, E., Martorana, G. E., et al. (2011). Lycopene and cardiovascular diseases: an update. Curr. Med. Chem. 18, 1146–1163. doi: 10.2174/092986711795029717
Mori, K., Shirasawa, K., Nogata, H., Hirata, C., Tashiro, K., Habu, T., et al. (2017). Identification of RAN1 orthologue associated with sex determination through whole genome sequencing analysis in fig (Ficus carica L.). Sci. Rep. 7, 41124. doi: 10.1038/srep41124
Munakata, R., Kitajima, S., Nuttens, A., Tatsumi, K., Takemura, T., Ichino, T., et al. (2020). Convergent evolution of the UbiA prenyltransferase family underlies the independent acquisition of furanocoumarins in plants. New Phytol. 225, 2166–2182. doi: 10.1111/nph.16277
Oliveira, A. P., Silva, L. R., Andrade, P. B., Valentão, P., Silva, B. M., Gonçalves, R. F., et al. (2010a). Further insight into the latex metabolite profile of Ficus carica. J. Agr. Food Chem. 58, 10855–10863. doi: 10.1021/jf1031185
Oliveira, A. P., Silva, L. R., Ferreres, F., Pinho, P. G. D., Valentão, P., Silva, B. M. (2010b). Chemical assessment and in vitro antioxidant capacity of Ficus carica latex. J. Agr. Food Chem. 58, 3393–3398. doi: 10.1021/jf9039759
Oliveira, A. P., Silva, L. R., Guedes de Pinho, P., Gil-Izquierdo, A., Valentão, P., Silva, B. M., et al. (2010c). Volatile profiling of Ficus carica varieties by HS-SPME and GC-IT-MS. Food Chem. 123, 548–557. doi: 10.1016/j.foodchem.2010.04.064
Palmeira, L., Pereira, C., Dias, M. I., Abreu, R. M. V., Corrêa, R. C. G., Pires, T. C. S. P., et al. (2019). Nutritional, chemical and bioactive profiles of different parts of a Portuguese common fig (Ficus carica L.) variety. Food Res. Int. 126, 108572. doi: 10.1016/j.foodres.2019.108572
Parhiz, H., Roohbakhsh, A., Soltani, F., Rezaee, R., Iranshahi, M. (2015). Antioxidant and anti-inflammatory properties of the citrus flavonoids hesperidin and hesperetin: an updated review of their molecular mechanisms and experimental models. Phytother. Res. 29, 323–331. doi: 10.1002/ptr.5256
Pereira, C., López-corrales, M., Serradilla, M. J., Villalobos, M. D., Ruíz-Moyano, S., Martín, A. (2017). Influence of ripening stage on bioactive compounds and antioxidant activity in nine fig (Ficus carica L.) varieties grown in Extremadura, Spain. J. Food Compost. Anal. 64, 203–212. doi: 10.1016/j.jfca.2017.09.006
Petkova, N., Ivanov, I., Denev, P. (2019). Changes in phytochemical compounds and antioxidant potential of fresh, frozen, and processed figs (Ficus carica L.). Int. Food Res. J. 26, 1881–1888.
Pratyusha, D. S., Sarada, D. V. L. (2022). MYB transcription factors-master regulators of phenylpropanoid biosynthesis and diverse developmental and stress responses. Plant Cell Rep. 41, 2245–2260. doi: 10.1007/s00299–022-02927–1
Ralston, L., Subramanian, S., Matsuno, M., Yu, O. (2005). Partial reconstruction of flavonoid and isoflavonoid biosynthesis in yeast using soybean type I and type II chalcone isomerases. Plant Physiol. 137, 1375–1388. doi: 10.1104/pp.104.054502
Reinsvold, R. E. (2010). Expression of the Artemisia annua beta-caryophyllene synthase in Synechocystis sp. strain PCC6803 results in cyanobacterial sesquiterpene accumulation. (master’s thesis). University of Northern Colorado, Greeley (CO).
Rodov, V., Vinokur, Y., Horev, B. (2012). Brief postharvest exposure to pulsed light stimulates coloration and anthocyanin accumulation in fig fruit (Ficus carica L.). Postharvest. Biol. Technol. 68, 43–46. doi: 10.1016/j.postharvbio.2012.02.001
Rubnov, S., Kashman, Y., Rabinowitz, R., Schlesinger, M., Mechoulam, R. (2001). Suppressors of cancer cell proliferation from fig (Ficus carica) resin: isolation and structure elucidation. J. Nat. Prod. 64, 993–996. doi: 10.1021/np000592z
Russo, F., Caporaso, N., Paduano, A., Sacchi, R. (2014). Phenolic compounds in fresh and dried figs from Cilento (Italy), by considering breba crop and full crop, in comparison to Turkish and Greek dried figs. J. Food Sci. 79, C1278–C1284. doi: 10.1111/1750–3841.12505
Sandhu, A. K., Islam, M., Edirisinghe, I., Burton-Freeman, B. (2023). Phytochemical composition and health benefits of figs (fresh and dried): a review of literature from 2000 to 2022. Nutrients 15, 2623. doi: 10.3390/nu15112623
Shahrajabian, M. H., Sun, W., Cheng, Q. (2021). A review of chemical constituents, traditional and modern pharmacology of fig (Ficus carica L.), a super fruit with medical astonishing characteristics. Pol. J. Agron. 44, 22–29. doi: 10.26114/PJA.IUNG.452.2021.452.04
Siyadatpanah, A., Mirzaei, F., Hossain, R., Islam, M. T., Fatemi, M., Norouzi, R. (2022). Anti-parasitic activity of the olea europaea and Ficus carica on leishmania major: new insight into the anti-leishmanial agents. Biologia 77, 1795–1803. doi: 10.1007/s11756–022-01066-y
Slatnar, A., Klancar, U., Stampar, F., Veberic, R. (2011). Effect of drying of figs (Ficus carica L.) on the contents of sugars, organic acids, and phenolic compounds. J. Agric. Food Chem. 59, 11696–11702. doi: 10.1021/jf202707y
Solomon, A., Golubowicz, S., Yablowicz, Z., Grossman, S., Bergman, M., Gottlieb, H. E., et al. (2006). Antioxidant activities and anthocyanin content of fresh fruits of common fig (Ficus carica L.). J. Agric. Food Chem. 54, 7717–7723. doi: 10.1021/jf060497h
Son, J. H., Jin, H., You, H. S., Shim, W. H., Kim, J. M., Kim, G. W., et al. (2017). Five cases of phytophotodermatitis caused by fig leaves and relevant literature review. Ann. Dermatol. 29, 86–90. doi: 10.5021/ad.2017.29.1.86
Song, M., Wang, H., Wang, Z., Huang, ,. H. ,. T., Chen, S. W., Ma, H. Q. (2021). Genome-wide characterization and analysis of bHLH transcription factors related to anthocyanin biosynthesis in fig. Front. Plant Sci. 12. doi: 10.3389/fpls.2021.730692
Su, Q., Rowley, K. G., Itsiopoulos, C., O’Dea, K. (2002). Identification and quantitation of major carotenoids in selected components of the Mediterranean diet: green leafy vegetables, figs and olive oil. Eur. J. Clin. Nutr. 56, 1149–1154. doi: 10.1038/sj.ejcn.1601472
Takahashi, T., Okiura, A., Kohno, M. (2017). Phenylpropanoid composition in fig (Ficus carica L.) leaves. J. Nat. Med. 71, 770–775. doi: 10.1007/s11418–017-1093–6
Tanwar, B., Andallu, B., Modgil, R. (2014). Influence of processing on physicochemical, nutritional and phytochemical composition of Ficus carica L. (fig) products. Asian J. Dairy. Foods. Res. 33, 37–43. doi: 10.5958/j.0976–0563.33.1.008
Usai, G., Mascagni, F., Giordani, T., Vangelisti, A., Bosi, E., Zuccolo, A., et al. (2020). Epigenetic patterns within the haplotype phased fig (Ficus carica L.) genome. Plant J. 102, 600–614. doi: 10.1111/tpj.14635
Vallejo, F., Marín, J. G., Tomás-Barberán, F. A. (2012). Phenolic compound content of fresh and dried figs (Ficus carica L.). Food Chem. 130, 485–492. doi: 10.1016/j.foodchem.2011.07.032
Veberic, R., Colaric, M., Stampar, F. (2008). Phenolic acids and flavonoids of fig fruit (Ficus carica L.) in the northern Mediterranean region. Food Chem. 106, 153–157. doi: 10.1016/j.foodchem.2007.05.061
Villard, C., Larbat, R., Munakata, R., Hehn, A. (2019). Defence mechanisms of Ficus: pyramiding strategies to cope with pests and pathogens. Planta 249, 617–633. doi: 10.1007/s00425–019-03098–2
Villard, C., Munakata, R., Kitajima, S., van Velzen, R., Schranz, M. E., Larbat, R., et al. (2021). A new P450 involved in the furanocoumarin pathway underlies a recent case of convergent evolution. New Phytol. 231, 1923–1939. doi: 10.1111/nph.17458
Wang, Z., Song, M., Li, Y., Chen, S., Ma, H. (2019). Differential color development and response to light deprivation of fig (Ficus carica L.) syconia peel and female flower tissues: transcriptome elucidation. BMC Plant Biol. 19, 217. doi: 10.1186/s12870–019-1816–9
Wang, Z., Song, M., Wang, Z., Chen, S., Ma, H. (2021). Metabolome and transcriptome analysis of flavor components and flavonoid biosynthesis in fig female flower tissues (Ficus carica L.) after bagging. BMC Plant Biol. 21, 396. doi: 10.1186/s12870–021-03169–1
Yahiaoui, S., Kati, D. E., Chaalal, M., Ali, L. M. A., Cheikh, K. E., Depaepe, G., et al. (2024). Antioxidant, antiproliferative, anti-inflammatory, and enzyme inhibition potentials of Ficus carica wood bark and related bioactive phenolic metabolites. Wood Sci. Technol 58, 1-25. doi: 10.1007/s00226-024-01549-y
Yang, J., Guo, J., Yuan, J. (2008). In vitro antioxidant properties of rutin. LWT 41, 1060–1066. doi: 10.1016/j.lwt.2007.06.010
Yin, W. P., Chen, H. M., Wang, T. X., Cai, M. S. (1997). 9,19-Cyclopropyl-24,25-epoxyethane-5-ene-3β the chemical structure and anticancer activity of spirosterols. Chin. J. Med. Chem. 7, 49–50. doi: 10.14142/j.cnki.cn21–1313/r.1997.01.010
Zhang, Y., Wan, Y., Huo, B., Li, B., Jin, Y., Hu, X. (2018). Extracts and components of Ficus carica leaves suppress survival, cell cycle, and migration of triple-negative breast cancer MDA-MB-231 cells. Oncotarg. Ther. 11, 4377–4386. doi: 10.2147/OTT.S17160
Keywords: Ficus carica, secondary metabolite, anthocyanin, coumarin, biosynthesis
Citation: Wang Y, Liu X, Chen S, Wang Q, Jin B and Wang L (2024) Functions, accumulation, and biosynthesis of important secondary metabolites in the fig tree (Ficus carica). Front. Plant Sci. 15:1397874. doi: 10.3389/fpls.2024.1397874
Received: 08 March 2024; Accepted: 14 June 2024;
Published: 02 July 2024.
Edited by:
Weiwei Zhang, Yangtze University, ChinaReviewed by:
Sarahani Harun, National University of Malaysia, MalaysiaNing Tang, Chongqing University of Arts and Sciences, China
Copyright © 2024 Wang, Liu, Chen, Wang, Jin and Wang. This is an open-access article distributed under the terms of the Creative Commons Attribution License (CC BY). The use, distribution or reproduction in other forums is permitted, provided the original author(s) and the copyright owner(s) are credited and that the original publication in this journal is cited, in accordance with accepted academic practice. No use, distribution or reproduction is permitted which does not comply with these terms.
*Correspondence: Li Wang, bGl3YW5nQHl6dS5lZHUuY24=