- Agricultural College, Inner Mongolia Agricultural University, Hohhot, China
Lodging reduces maize yield and quality. The improvement in maize lodging resistance has proven to be instrumental in maximizing the yield potential of maize varieties under high-density planting. Tillage practices accommodate larger groups by enhancing soil conditions. This study aimed to elucidate the impact of subsoil tillage in reducing the maize stalk lodging rate. The maize cultivars Xianyu 335 (XY335) and Zhongdan2 (ZD2) were selected for field experiments including two tillage methods, shallow rotary (RT) and subsoil (SS), and two densities, 75,000 plants ha−1 (D1) and 105,000 plants ha−1 (D2), were set up to investigate and analyze the changes of maize lodging rate and the related indexes of lodging resistance under SS and RT conditions. The findings revealed that under high density, as compared to rotary tillage, SS tillage decreased the plant and ear height by 9.01–9.20 cm and 3.50–4.90 cm, respectively. The stalk dry matter accumulation was enhanced by 8.98%–24.98%, while stalk diameter between two and seven internodes increased by 0.47– 4.15 mm. Stalk cellulose increased by 11.83% –12.38%, hemicellulose increased by 6.7%–15.97%, and lignin increased by 9.86%–15.9%. The rind puncture and crushing strength improved by 3.11%–20.06% and 11.90%–27.07%, respectively. The bending strength increased by 6.25%–27.96% and the lodging rate decreased by 1.20%–6.04%. Yield increased by 7.58%–8.17%. At SS tillage when density increased, the index changes in ZD2 were mostly less than those in XY335. The rind penetration strength, bending strength, crushing strength, stalk diameter, and dry matter accumulation all had a negative correlation with the lodging rate. It suggested that SS tillage was beneficial to lodging resistance and, in combination with stalk lodging-resistant varieties, can effectively alleviate the problem of stalk lodging after increased planting density.
1 Introduction
Maize is one of the world’s and China’s major crops in both plant area and yield and plays a pivotal role in national food security. With the development of the Chinese economy and population growth, the demand for grain has shown a steady increase, and maize output will need to rise to fulfill the needs (Ghose, 2014; Tigchelaar et al., 2018). Increasing planting density and the number of effective harvested ears per unit area is one of the key cultivation measures to increase maize yield (Ma et al., 2014). In China, the average planting density of maize rose from 15,000 plants ha−1 in the 1950s to 60,000 plants ha−1 in 2010, and the average grain yield elevated from 1,000 kg hm−1 in the 1940s to 6,000 kg hm−1 in 2010 (Li et al., 2016). In recent years, the planting density of maize in China has grown from 99,500 plants ha−1 to 105,000 plants ha−1 (Kamran et al., 2018; Liu et al., 2021; Zhang et al., 2022).
Increasing planting density is an effective approach to increase maize yield with minimum input. However, as density increases, increased competitive pressure between plants changes the structure and function of maize plants and populations, thus affecting maize stalk morphology, carbohydrate accumulation and distribution, chemical components, root morphology and structure, and mechanical strength of stalks, and increasing the risk of lodging (Xue et al., 2020a; Sher et al., 2018; Zheng et al., 2021; Qi et al., 2023). Stalk lodging causes annual yield loss of up to 75% (Li et al., 2015) and reduces the quality of grain production (Da et al., 2016), becoming an important concern in modern maize production. The direct mechanized harvest and grain drying into storage can simplify the production link, improve production efficiency, aid in achieving large-scale production, and help realize progressive advancement in agricultural practices (Xue et al.,2018a). However, the high lodging rate hinders the large-scale popularization and application of whole-mechanized processes. Grain loss due to straw fall is higher than root fall because straw fall disrupts the transport of photosynthetic products, nutrients, and water (Shah et al., 2021). Lodging at silking and milk-ripening stages (R1 and R3) influence grain filling and yield, while stalk lodging (R6) at the dewatering stage after physiological maturity adversely impacts mechanical yield (Xie et al., 2022). Morphological characteristics of the plant, including plant height and ear height, and mechanical strength of the stalk, including crushing strength (CS) and bending strength (BS), are often strong indicators of maize stalk resistance, and these indicators are often closely related to tillage practices (Shi et al., 2016; Li et al., 2023).
Tillage affects soil structure, moisture, fertility, gases, heat, and other factors that influence crop root growth and yield formation. However, there is little information on how maize stalk traits respond to tillage practices and their effects on maize stalk resistance to lodging. Deep tillage (e.g., subsoiling, deep ploughing, or ripping, to a depth > 20 cm) has been shown to enhance the structure and health of compacted soils (Hamza and Anderson, 2005; Hall et al., 2010). Tillage affects various soil physical, biological, and chemical properties and thus also impacts crop yield. Previous studies have shown that deep tillage improves the soil properties in the tilled layer by reducing soil bulk density and penetration resistance (Varsa et al., 1997), increasing soil porosity, hydraulic conductivity, and infiltration rate (Laddha and Totawat, 1997), and creating a more favorable soil environment for root growth and crop production than shallow tillage. Conventional tillage with soil compaction resulted in barren summer maize, leading to low root stress resistance (Bian et al., 2016). As compared to shallow tillage and no tillage, subsoil (SS) tillage can break up the compacted hardpan layer without inverting it, decrease the soil bulk density and soil compaction, and increase the soil water content. This improves soil structure and, thus, promotes root growth and boosts maize yield (Sun et al., 2018; Xu et al., 2019; Wang et al., 2020b). Root fixation properties and stalk development complement each other, promoting the accumulation of material and mechanical strength formation of aboveground stalks, effectively reducing the rate of lodging. Liu et al. reported that subsoil significantly improved the vascular transport efficiency of the stalk and enhanced stalk strength. On the other hand, it also improves root vigor, facilitates water and nutrient uptake, promotes dry matter accumulation, and increases the mechanical strength of the stalks, ultimately improving resistance to lodging (Liu et al., 2013). Ma et al. demonstrated that subsoil can reduce the ear height of maize under dense planting conditions, slow down the densification pressure, and increase maize stalk puncture strength, CS, and BS to varying degrees, so as to optimize stalk morphology and mechanical traits, which is conducive to improving the resistance to lodging and efficiently accommodating larger populations (Liu et al., 2013; Ma et al., 2014; Zhang et al., 2015). Yu et al. showed that subsoil improves the soil environment, which promotes the growth of the root system and helps to absorb water and nutrients from deeper layers of the soil, and that the use of subsoil technology can further increase the planting density, giving full play to the yield potential of varieties (Yu et al., 2013). It can be seen that SS tillage can be one of the effective ways to exploit the potential of maize for densification and resistance to lodging. Therefore, an in-depth investigation of the effect of SS tillage on maize stalk resistance after densification will be of great significance for the application of SS tillage measures to improve maize resistance in production and for the realization of the full mechanization of maize.
The average plough layer is 18.5 cm in China (Li and Wang, 2010) and lower than 35 cm in the United States (Li and Wang, 2010). At a high density, constructing a reasonable soil topsoil layer can promote material accumulation during the formation of stem mechanical strength, and thus reduce the lodging risk. Therefore, it was hypothesized that SS tillage could improve the lodging resistance of maize stalks under high-density conditions. The present study was carried out to (1) elucidate the responses of plant morphology, dry matter accumulation, and mechanical characteristics to subsoiling tillage; and (2) evaluate the response of different maize varieties to SS tillage at different densities. The study’s findings provide ideas and technical assistance for the mechanized harvesting of maize as well as the densification and yield increase.
2 Materials and methods
2.1 Experimental site
During 2019–2020, the experiment was conducted in the Modern Agriculture Expo Park of China at Tu met Right Banner, Baotou City, Inner Mongolia (40°28′ 28′′, 110°29′ 5′′). The previous crop was maize and the soil type was sandy loam. Table 1 reflects the main meteorological elements in the growing period, while soil nutritional contents are listed in Table 2.
2.2 Experimental design
The experiment was conducted utilizing a re-split zone experiment design with three factors (Table 3): tillage method, planting density, and variety. In the main area of cultivation, two treatments were set: subsoil (SS) (35 cm) and traditional rotary tillage (RT) (15 cm);, the total area of the plot is 2,160 m2. The sub-plot included a density of 75,000 plants ha−1 (D1) (plot area, 180 m2; length, 30 m; width, 6 m; row space, 60 cm; and plant space, 22.22 cm) and 105,000 plants ha−1 (D2) (plot area, 180 m2; length, 30 m; width, 6 m; row space, 60 cm; and plant space, 15.87 cm). Two varieties with different lodging resistance were selected, namely, Xian yu335 (XY335) and Zhong dan2 (ZD2). Three replicates per treatment were established (15 m × 6 m, 90 m2 plots). The plots were subjected to continuous tillage on the same site with the same plots in 2019 and 2020.
The sowing dates were 23 April 2019– and 26 April 2020–. Nitrogen fertilizer (225.0 kg hm−2), P2O5 (150.0 kg hm−2), and K2O (150.0 kg hm−2) were used in the test. Nitrogen fertilizer was applied at the jointing, V12, and silking stages in a ratio of 3:6:1, respectively. P2O5 and K2O were used as base fertilizers at one time. RT is representative of the typical tillage practice in the study area, and the soil was tilled to a depth of 20 cm with a rotary cultivator in the spring. The SS treatment was sub-soiled to a depth of 35 cm with an interval of 60 cm using a subsoiler in the autumn and tilled to a depth of 20 cm with a rotary cultivator in the spring. The growth period was irrigated four times: pre-sowing, V12, R1, and R3 stage, with 750 m3 hm−2 irrigation water. Other management was the same as in field production.
2.3 Sampling and measurements
2.3.1 Field stalk lodging rate
Field lodging of maize was evaluated at the silking stage (R1), 30 days after spinning (R3), physiological maturity stage (R6), and 15 days after ripening (R6–15d).
2.3.2 Plant morphological indexes
Plant height (from the ground to the top of the tassel) and ear height (from the ground to the node bearing the ear) were measured using a ruler. The stalk diameter (d) was measured using a Vernier caliper at the midpoint of the internode at its narrowest side (d1) and widest side (d2). It was calculated as d = (d1+d2)/2.
2.3.3 Sampling and determination of internode mechanical strength
At the R1 stage, three maize plants were randomly chosen from each treatment. The stalks were cut from the ground, and leaves and other parts were removed, followed by sorting and marking according to the nodes. The mechanical indexes of the stalks were determined as follows:
Rind Puncture Strength (RPS): The SY-S03 probe (1 mm−2) was slowly pressed perpendicular to the stalk at a constant rate to read the maximum penetration (N mm−2) of the stalk epidermis. It was measured at the base of the second to seventh internodes.
Crushing strength (CS): The SY-S03 type stalk strength measuring instrument was used. The 1 cm−2 probe perpendicular to the stalk axis was used to press slowly and evenly in the middle of the internode with the pressure head of the testing machine so that it could reach the CS value (N cm−2) when it was torn. It was measured at the base of the third, fifth, and seventh internodes.
Bending strength (BS): The 0.5 cm−2 probe of the SY-S03 stalk strength measuring instrument was perpendicular to the stalk axis, and the pressure head of the testing machine was pressed in the middle of the internode at a slow and uniform speed so that its bending reached the maximum value (N cm−2). It was measured at the base of the second, fourth, and sixth internodes.
2.3.4 Stalk dry matter accumulation
A total of four periods were measured, which were at the silking stage (R1), 30 days of silking (R3), physiological maturity stage (R6), and 15 days after maturity (R6–15d); the stalk samples were intercepted and placed in the oven at 105°C for 30 min, dried at 80°C, and weighed dry.
2.4 Data and statistical analysis
Data were processed using Microsoft Excel 2019 (Microsoft, Inc., Redmond, WA, USA). The statistical software SAS 9.4 (SAS Institute Inc., Raleigh, CA, USA) was used for data variance and correlation analysis. One-way ANOVA was used to compare the differences between treatments each year at the same growth stage. Three-way ANOVA was used to assess the effects of individual factors and interactions between factors on the indicators. The significance test was performed using LSD (least significant difference) and Duncan’s method. Pearson’s correlation coefficient was used for correlation analysis. Additionally, figures were created using Sigma plot 12.5.
3 Results
3.1 Lodging rate of the stalks
ANOVA results (Table 4) indicated that maize stalk lodging rates varied significantly based on density, variety, tillage, density × variety, and tillage × variety. After 15 days of maturity, the lodging rate of maize under the SS plot was 2.55% and 2.01% points lower than RT in 2019 and 2020, respectively. The planting density increased from D1 to D2, resulting in a significant increase in lodging rate, which climbed by 10.18% and 3.61% points in 2019 and 2020, respectively, at maturity. At 15 days after maturity, it elevated by 11.23% and 3.76% points. XY335 had a lower lodging rate than ZD2. Table 5 shows that, after increasing the density, the lodging rate of ZD2 increased by 1.67%–21.30% under the RT plot, while it increased by 2.53%–18.67% under the SS plot. However, in comparison to RT, SS with increased density exhibited a reduction in lodging rate ranging from 0.40% to 6.49%. Thus, employing SS may minimize the maize lodging rate despite the increase in planting density.
3.2 Plant height, ear height, and ear ratio of maize
ANOVA results showed significant differences in maize plant and ear height across tillage, density, and variety. The plant height varied significantly among tillage × variety, density × variety, and tillage × variety × density.
Subsoil tillage effectively reduced plant and ear height, but there was no significant effect on the ear ratio. In comparison to RT, the plant and ear heights were reduced by 9.39 cm and 5.32 cm, respectively, under SS. From D1 to D2, the plant and ear height increased by 10.09 cm in 2019 and 4.99 cm in 2020. The plant and ear height of XY335 were lower than ZD2 (Table 6).
According to Table 7, under the RT plot, when the density increased, the plant and ear height grew by 10.66 cm and 5.13 cm for ZD2, while it was raised by 11.63 cm and 7.20 cm for XY335. SS reduced the rise in plant height and ear height of XY335 and ZD2 due to increased density.
3.3 Diameter of the second to seventh internodes
ANOVA results (Table 8) revealed that the diameter from the second to seventh internodes varied significantly among density, variety, tillage, and density × variety. SS increased diameter from the second to seventh internodes, respectively. When the density increased from D1 to D2, the diameter from the second to seventh internodes decreased significantly. The diameter of the second to seventh internodes of XY335 is larger than that of ZD2. Table 9 depicts that the stalk diameter between the second and seventh nodes at the base of the maize plant decreases as the node ascends and diminishes with increasing planting density. Under the RT plot, after the increase in density, the reduction in stalk diameter ranged from 9.04% to 17.47% for XY335 and from 10.71% to 22.32% for ZD2. Conversely, under the SS plot, the reduction in stalk diameter was in the range of 2.97%–14.79% for XY335 and 7.43%–17.51% for ZD2. Consequently, it can be inferred that SS mitigates the impact of reduced diameter resulting from increased planting density. Under high density (D2), SS increased diameter in XY335 by 4.65%–20.28% and in ZD2 by 0.72%–16.35% as compared to RT. The higher increase in the diameter of XY335 indicated that it was more receptive to subsoil tillage than ZD2.
3.4 Rind puncture strength from the second to seventh internodes
ANOVA results indicated that the RPS from the second to seventh internodes varied significantly among density, variety, tillage, density × variety, tillage × variety, and density × variety. In comparison to RT, SS significantly enhanced the RPS from the second to seventh internodes by 2.68–8.71 N mm−2 in 2019 and increased by 4.07–12.32 N mm−2 in 2020. When the density increased from D1 to D2, the RPS from the second to seventh internodes dropped considerably. The XY335 cultivar had a higher RPS than the ZD2 cultivar (Table 10).
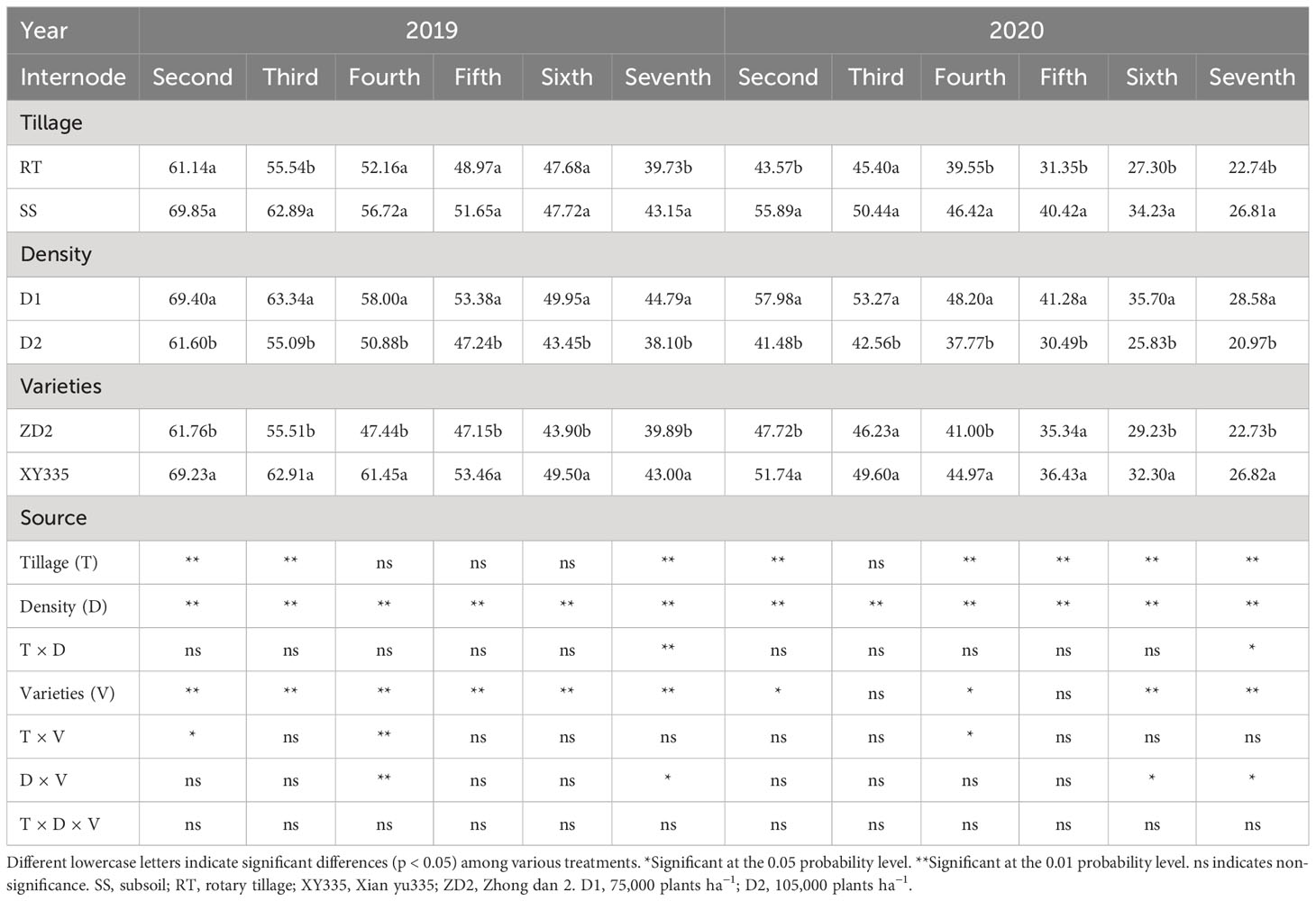
Table 10 ANOVA results for rind puncture strength (N mm−2) from the second to seventh internodes during 2019 to 2020.
Figures 1, 2 depict that the RPS of the stalk internodes at the base of the maize plant decreases with the upward position of the node and drops significantly as the planting density increases. According to the RT plot, the reduction in RPS after increased density varied from 10.67% to 32.66% for XY335 and from 10.93% to 41.76% for ZD2. In contrast, under the SS plot, the reduction in RPS after increased density was in the range of 9.05%–23.36% for XY335 and 8.18%–29.71% for ZD2, with XY335 exhibiting a more pronounced effect. Consequently, it can be inferred that SS tillage mitigates the decrease in RPS between the second and seventh stalk nodes following higher planting density. Under high density (D2), SS increased XY335 RPS by 6.55%–37.59% and ZD2 by 3.11%–33.91% compared to RT. The larger increase in XY335 indicates that the response of XY335 RPS to subsoil tillage was greater than that of ZD2.
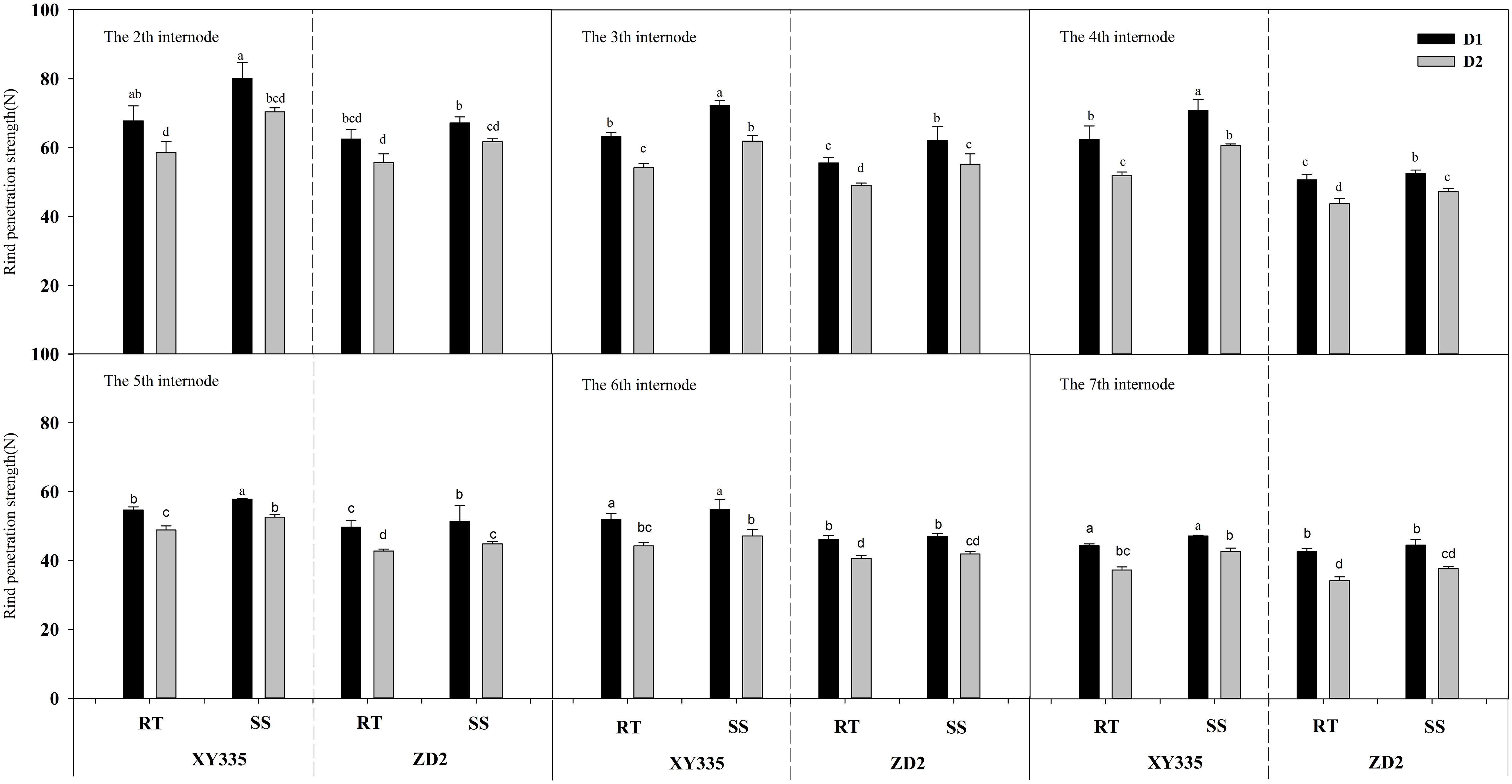
Figure 1 Effect of subsoil tillage and planting density on the penetration strength of maize stalk internodes in 2019. Different lowercase letters indicate significant differences (p < 0.05) among various treatments; SS, subsoil; RT, rotary tillage; XY335, Xian yu335; ZD2, Zhong dan 2. D1, 75,000 plants ha−1; D2, 105,000 plants ha−1.
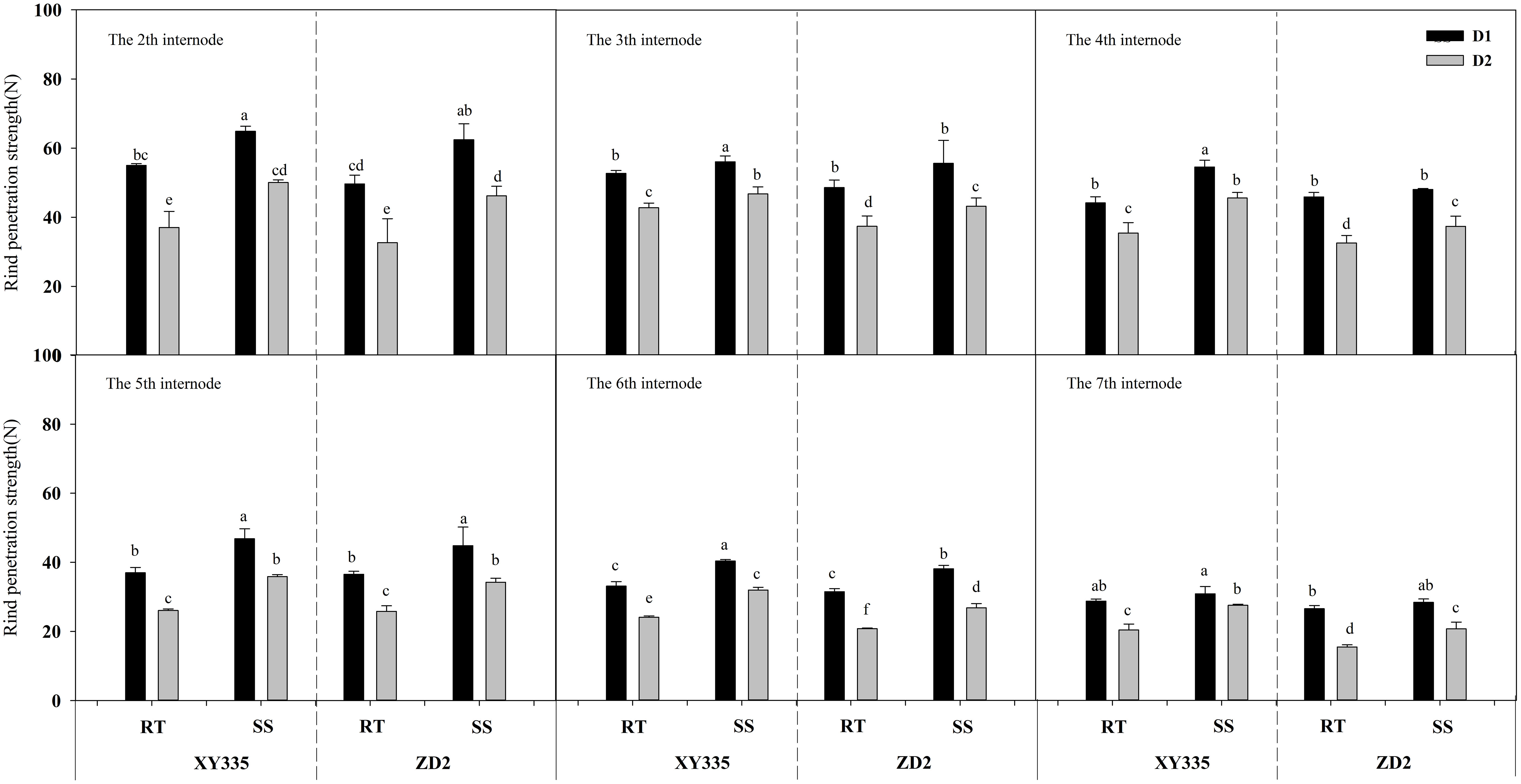
Figure 2 Effect of subsoil tillage and planting density on the penetration strength of maize stalk internodes in 2020. Different lowercase letters indicate significant differences (p < 0.05) among various treatments; SS, subsoil; RT, rotary tillage; XY335, Xian yu335; ZD2, Zhong dan 2. D1, 75,000 plants ha−1; D2, 105,000 plants ha−1.
3.5 Crushing strength
ANOVA results indicated that the CS of the second, fourth, and sixth internodes differed significantly across density, variety, tillage, tillage × variety, and density × variety. Comparing SS to RT, it significantly increased the CS of the second, fourth, and sixth internodes by 55.7, 55.34, and 37.56 N cm−2 in 2019, and by 64.16, 71.2, and 32.67 N cm−2 in 2020, respectively. When the density increased from D1 to D2, the CS of the second, fourth, and sixth internodes decreased significantly. The XY335 cultivar had higher CS than the ZD2 cultivar (Table 11).
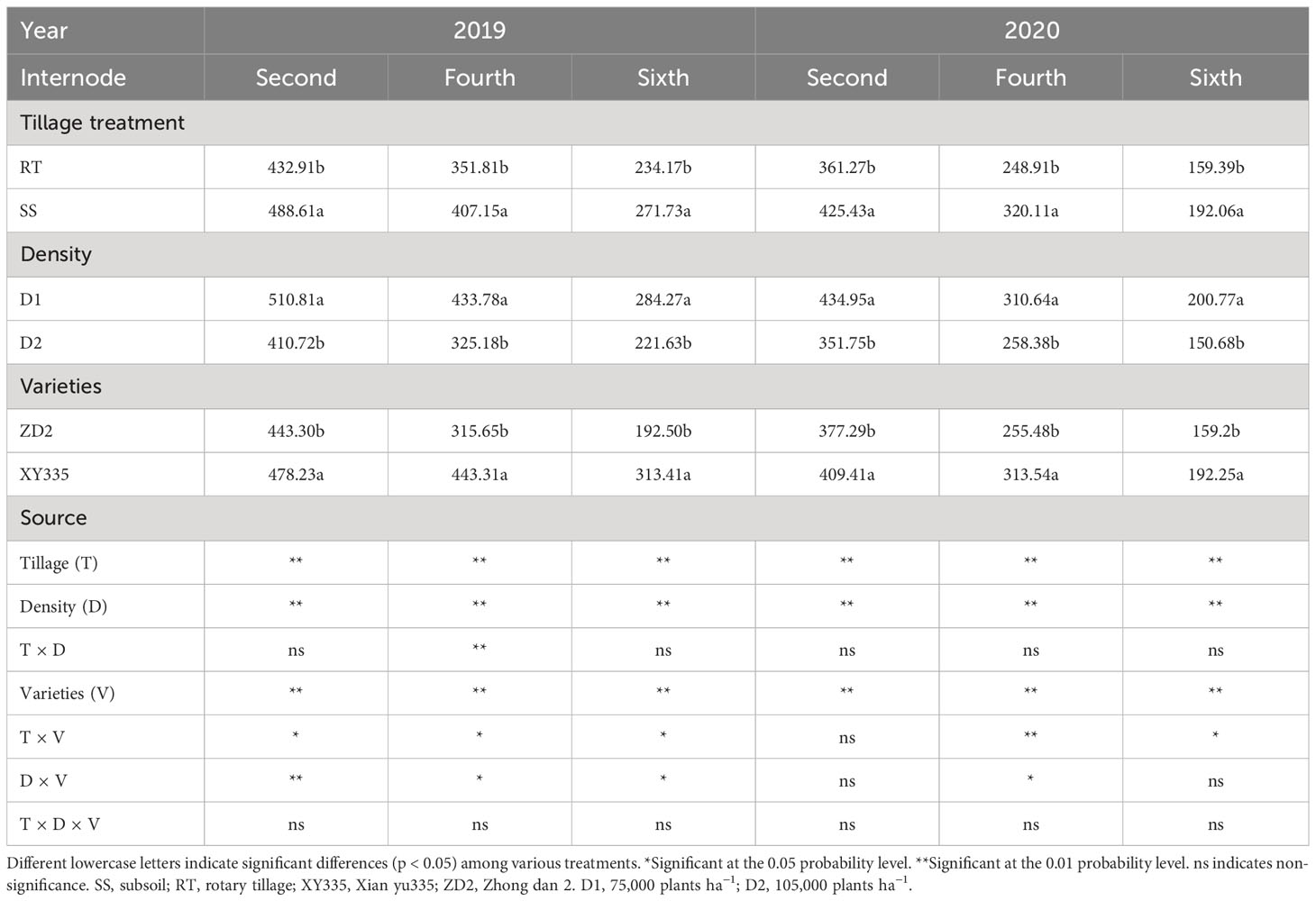
Table 11 Variance analysis of the impact of subsoil tillage and planting density on internode crushing strength (N cm−2) during 2019–2020.
The CS of maize stalk internodes decreased with the upward position of the node and reduced significantly with increasing planting density, as seen in Figures 3, 4. Under the RT plot, the reduction in CS after increased density ranged from 10.67% to 32.66% for XY335 and from 10.93% to 41.76% for ZD2. Conversely, under the SS plot, the reduction in CS after increased density varied from 9.05% to 23.36% for XY335 and from 8.18% to 29.71% for ZD2, with XY335 exhibiting a more pronounced effect. Consequently, it can be inferred that SS tillage attenuates the decrease in CS between the second, fourth, and sixth stalk nodes following increased planting density. Under high density (D2), SS increased CS by 16.96–37.57% for XY335 and 11.90–23.41% for ZD2 compared to RT. The larger increase for XY335 suggested that changes in its CS responded more favorably to subsoil tillage as compared to ZD2.
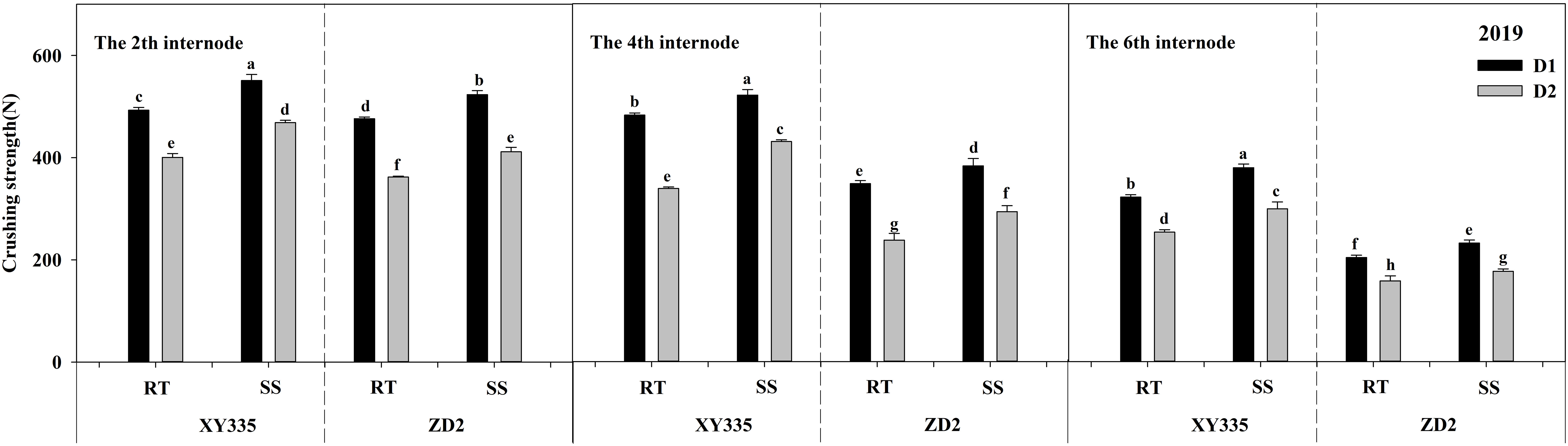
Figure 3 Effect of subsoil tillage and planting density on the crushing strength (N cm−2) of maize stalk internodes in 2019. Different lowercase letters indicate significant differences (p < 0.05) among various treatments; SS, subsoil; RT, rotary tillage; XY335, Xian yu335; ZD2, Zhong dan 2. D1, 75,000 plants ha−1; D2, 105,000 plants ha−1.
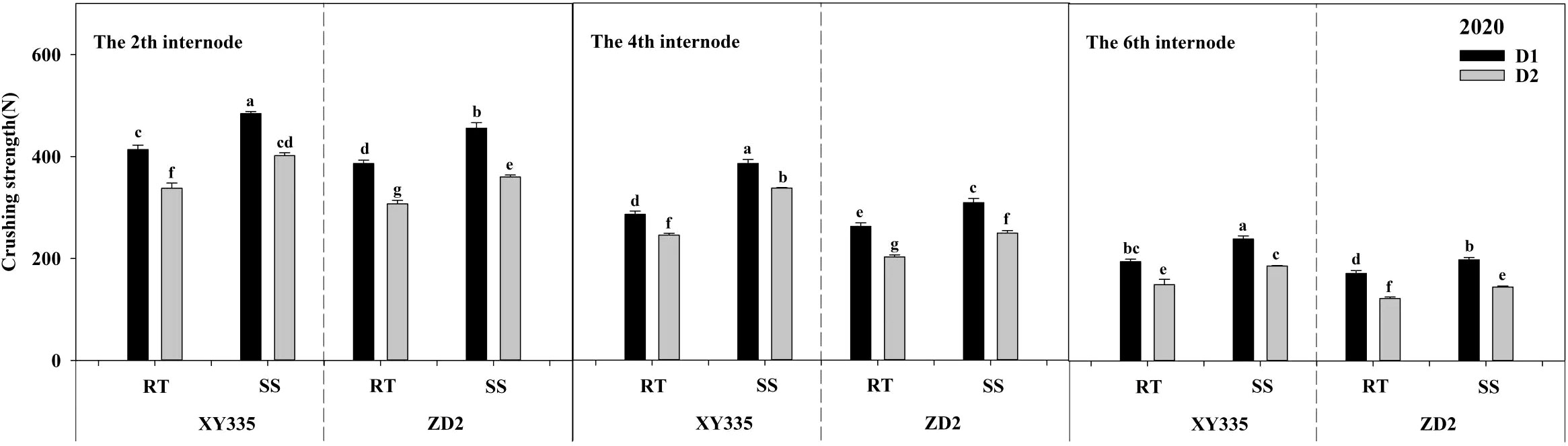
Figure 4 Effect of subsoil tillage and planting density on the crushing strength (N cm−2) of maize stalk internodes in 2020. Different lowercase letters indicate significant differences (p < 0.05) among various treatments; SS, subsoil; RT, rotary tillage; XY335, Xian yu335; ZD2, Zhong dan 2. D1, 75,000 plants ha−1; D2, 105,000 plants ha−1.
3.6 Bending strength
The ANOVA results exhibited significant differences in the BS of the third, fifth, and seventh internodes based on density, variety, tillage, tillage × variety, and density × variety. When compared with RT, SS tillage significantly raised the BS of the third, fifth, and seventh internodes by 35.77, 69.72, and 61.52 N cm−2 in 2019, and by 59.44, 38.40, and 34.37 N cm−2 in 2020. As the planting density progressed from D1 to D2, the BS of the third, fifth, and seventh internodes decreased significantly. The BS of the XY335 cultivar was higher than that of the ZD2 cultivar (Table 12).
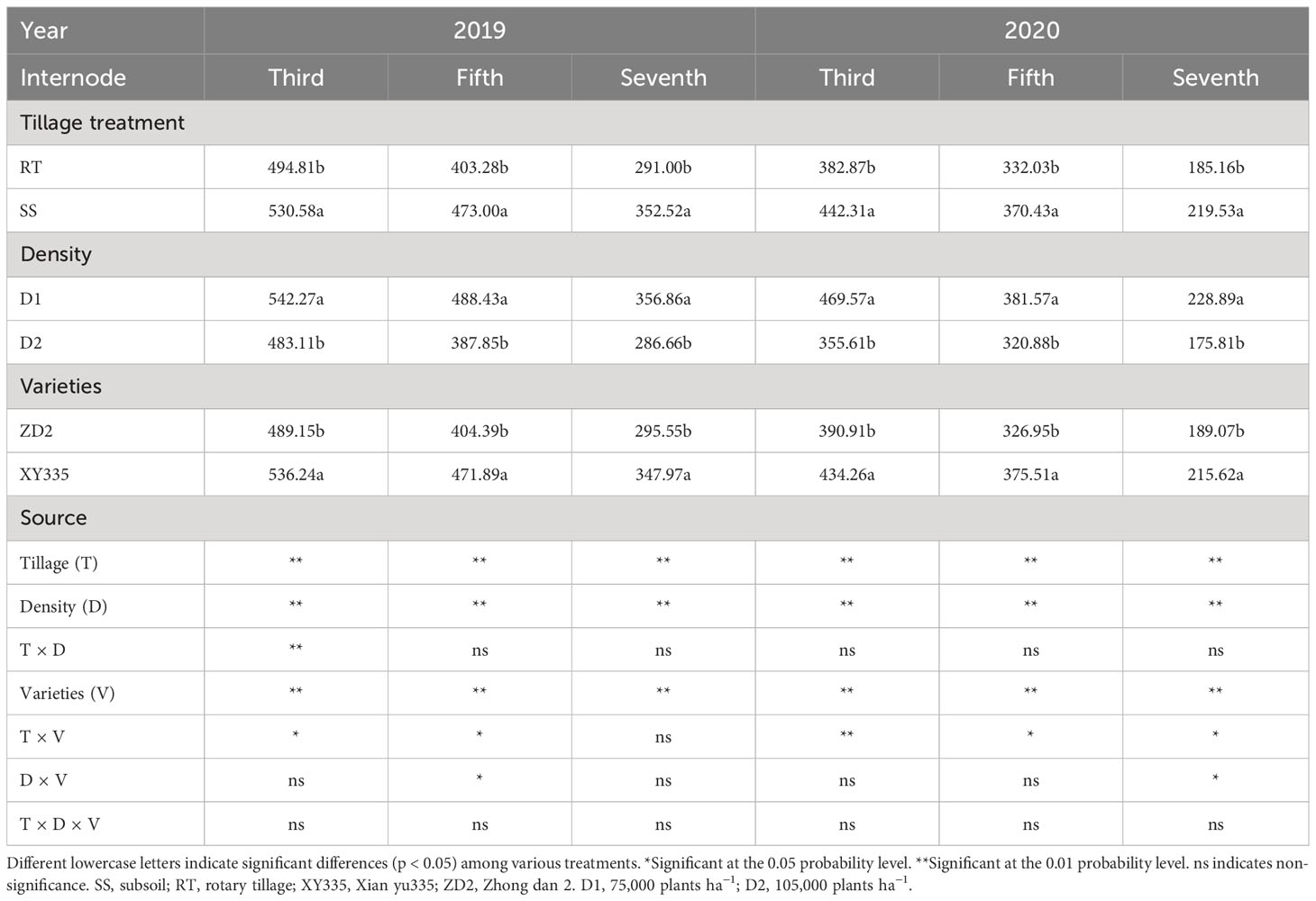
Table 12 Variance analysis of effects of subsoil tillage and planting density on internode bending strength (N cm−2) during 2019–2020.
The BS of stalk internodes decreased with the upward position of the node and reduced substantially with increased planting density, as reflected in Figures 5, 6. Under the RT plot, the drop in BS after increased planting density ranged from 12.92% to 27.59% for XY335 and from 13.81% to 30.37% for ZD2. On the other hand, under the SS plot, XY335 showed a more noticeable effect, with the reduction in BS varying from 7.91% to 20.66%, while it ranged from 9.29% to 24.48% for ZD2. Thus, it may be concluded that SS tillage offsets the decrease in BS of the third, fifth, and seventh internodes following increased planting density. Under the high density (D2), SS increased CS by 11.33–27.96% for XY335 and 6.25–26.39% for ZD2 compared to RT. The higher increase for XY335 implied that the BS response of XY335 was more receptive to subsoil tillage than ZD2.
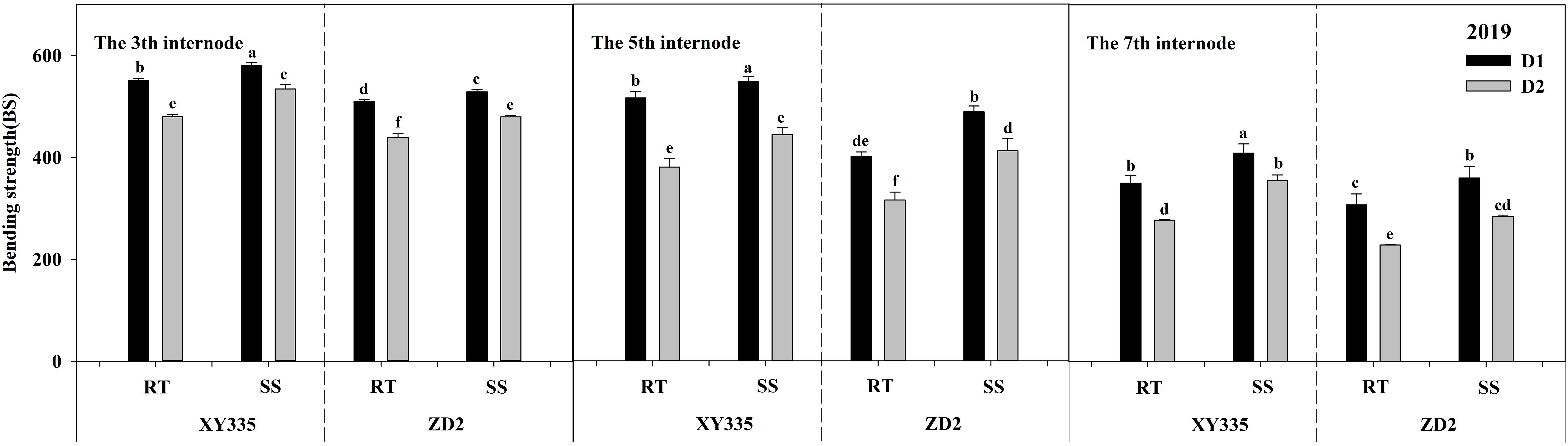
Figure 5 Effect of subsoil tillage and planting density on the bending strength (N cm−2) of maize stalk internodes in 2019. Different lowercase letters indicate significant differences (p < 0.05) among various treatments; SS, subsoil; RT, rotary tillage; XY335, Xian yu335; ZD2, Zhong dan 2. D1, 75,000 plants ha−1; D2, 105,000 plants ha−1.
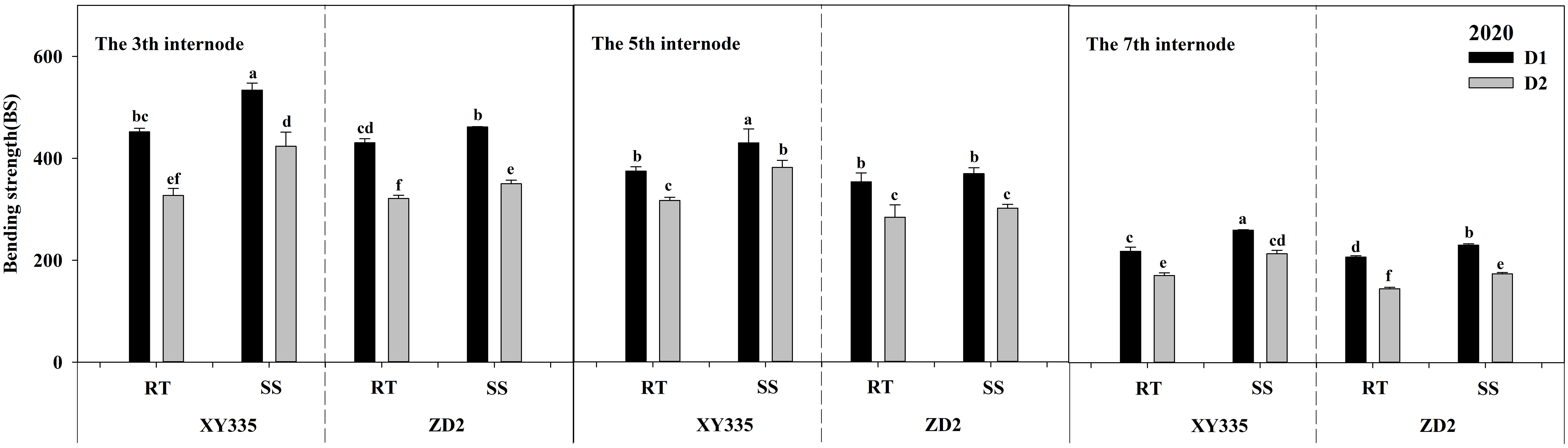
Figure 6 Effect of subsoil tillage and planting density on the bending strength (N cm−2) of maize stalk internodes in 2020. Different lowercase letters indicate significant differences (p < 0.05) among various treatments; SS, subsoil; RT, rotary tillage; XY335, Xian yu335; ZD2, Zhong dan 2. D1, 75,000 plants ha−1; D2, 105,000 plants ha−1.
3.7 Effects of tillage methods and planting density on stalk dry matter accumulation
The ANOVA results exhibited significant variations in stalk dry matter accumulation (SDMA) per plant depending on density, variety, tillage, tillage × variety, and density × variety. Subsoil tillage substantially raised the SDMA by 4.24–7.37 g in 2019 and by 7.88–10.42 g in 2020, compared with RT. As the planting density progressed from D1 to D2, the SDMA decreased significantly. The XY335 cultivar had greater SDMA than the ZD2 cultivar (Table 13).
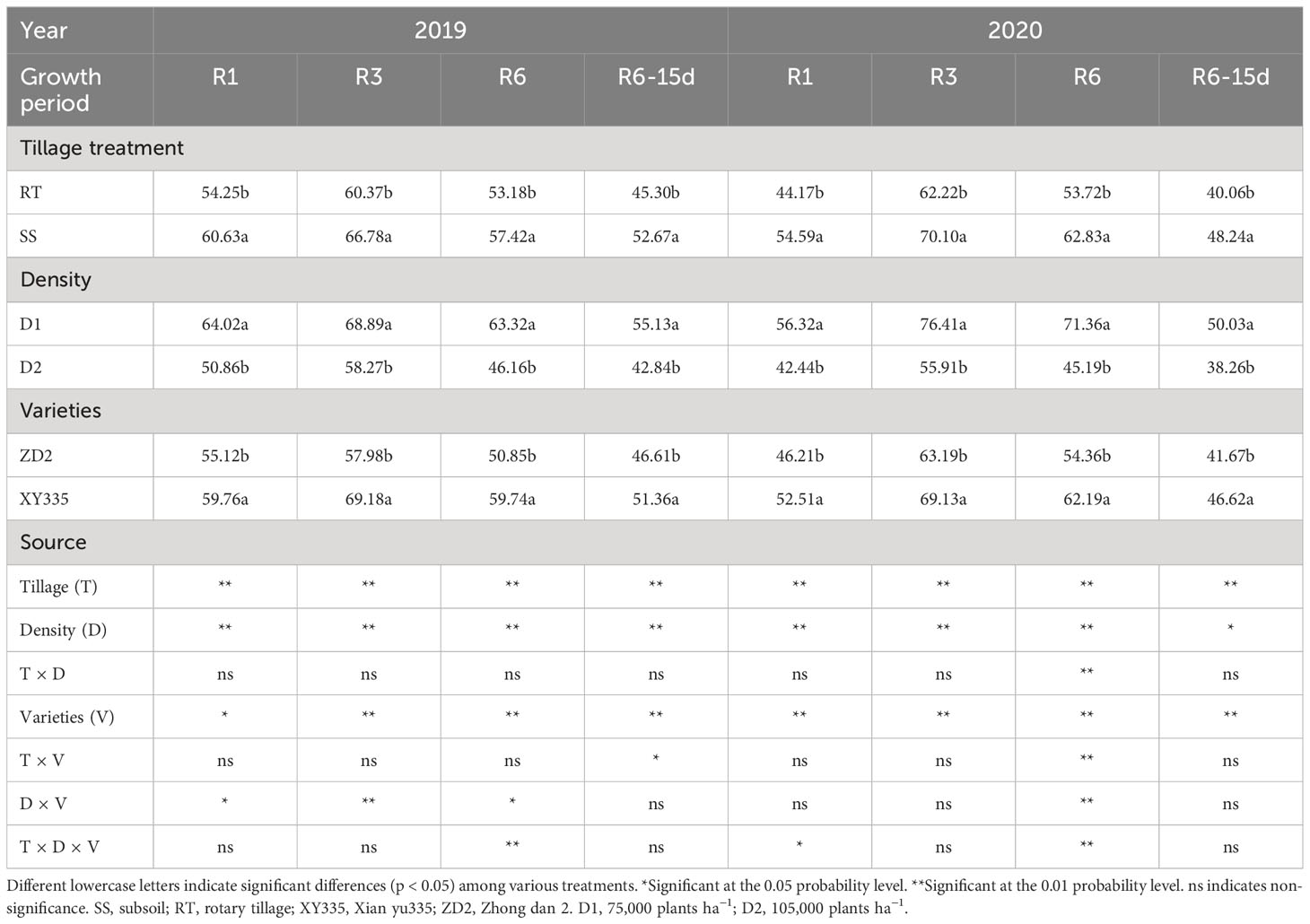
Table 13 Variance analysis of effects of subsoil tillage and planting density on stalk dry matter accumulation (g) in 2019–2020.
Figures 7, 8 depicts that SDMA decreased with increasing planting density. Under the RT plot, SDMA reduced by 17.64%–30.23% for XY335 and 28.28%–41.49% for ZD2 with increasing density. Conversely, under the SS plot, the increase in density decreased SDMA by 11.83%–27.17% for XY335 and 21.70%–33.14% for ZD2. The decrease in SDMA after increased density under SS was lower than that under RT, implying that SS tillage alleviates the decline in SDMA due to the rise in planting density. Under high density (D2), in comparison to RT, SS increased SDMA in XY335 by 13.47%–41.39% and by 7.1%–24.17% in ZD2. The larger increase in SDMA of XY335 suggested that it responded more positively to SS tillage than ZD2.
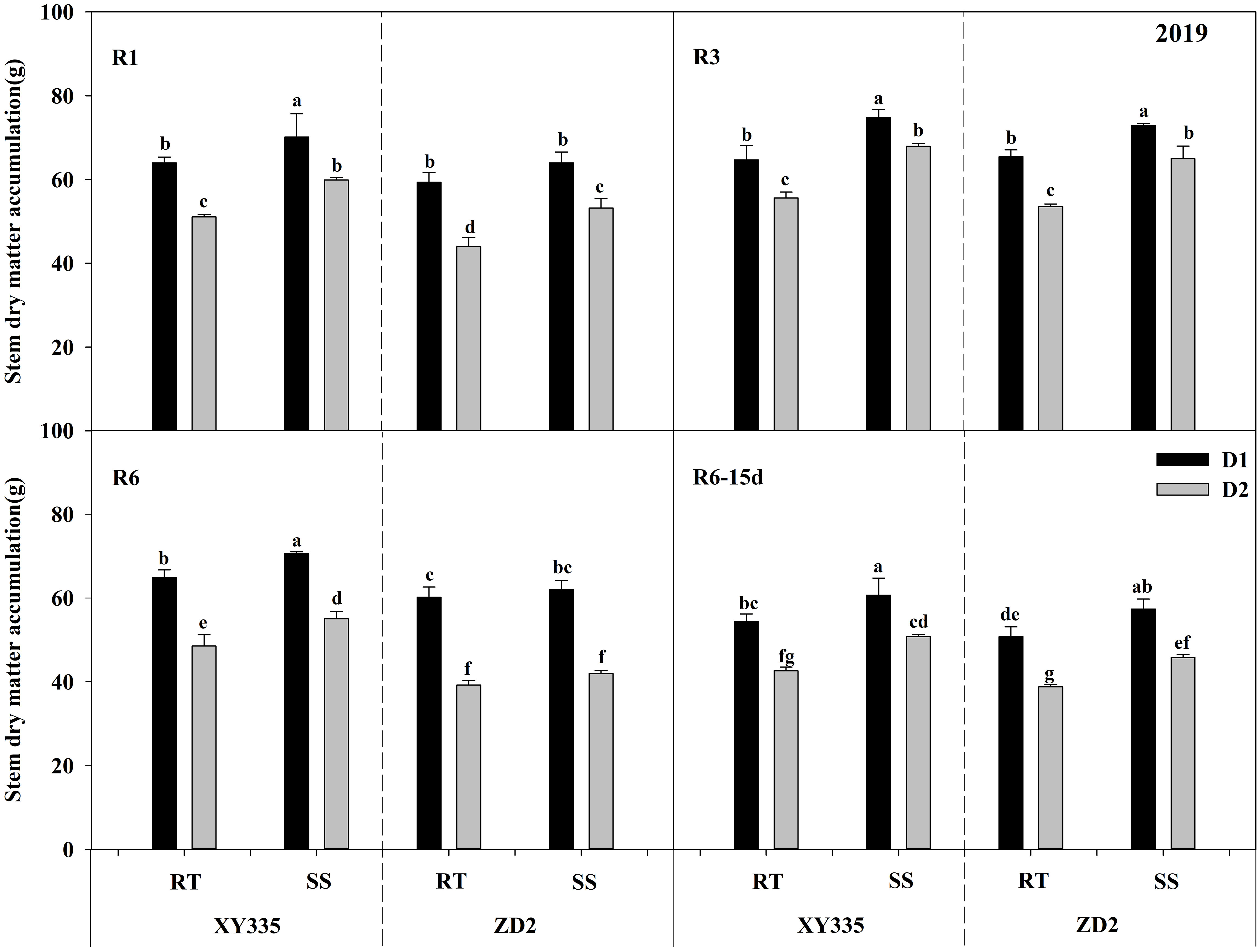
Figure 7 Effect of subsoil tillage and planting density on stalk dry matter accumulation (g) in 2019. Different lowercase letters indicate significant differences (p < 0.05) among various treatments; SS, subsoil; RT, rotary tillage; XY335, Xian yu335; ZD2, Zhong dan 2. D1, 75,000 plants ha−1; D2, 105,000 plants ha−1. R3, milk stage; R6, physiological maturity; R6–15d, 15 days after physiological maturity.
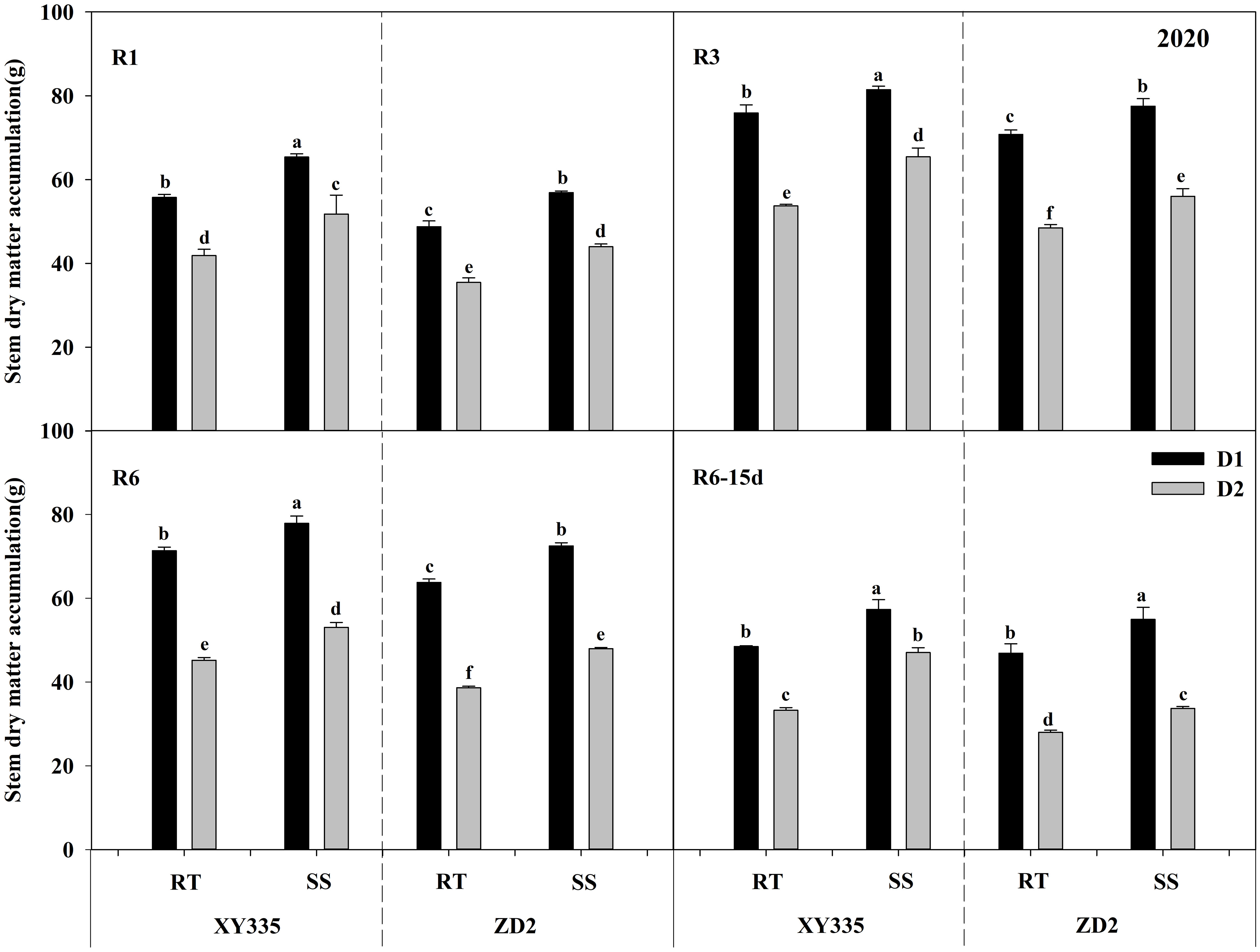
Figure 8 Effect of subsoil tillage and planting density on stalk dry matter accumulation (g) in 2020. Different lowercase letters indicate significant differences (p < 0.05) among various treatments; SS, subsoil; RT, rotary tillage; XY335, Xian yu335; ZD2, Zhong dan 2. D1, 75,000 plants ha−1; D2, 105,000 plants ha−1. R3, milk stage; R6, physiological maturity; R6–15d, 15 days after physiological maturity.
3.8 Correlation between plant morphology, internode mechanical strength, stalk dry matter accumulation, and maize lodging rate
As shown in Figure 9, plant height, ear height, and ear ratio were significantly and positively correlated with lodging rate. Nevertheless, the RPS of the fourth internode; the CS of the second, fourth, and sixth internodes; the BS of the fifth internode; and the diameter of the third, fifth, and seventh internodes were negatively associated with lodging rate. Furthermore, the CS of the fourth and sixth internodes and accumulation of dry matter of the stalks were negatively and significantly correlated with lodging rate. Significant positive correlations were also found among internode RPS, CS, and BS. There was a significant negative correlation between internode RPS, CS, BS, plant height, and ear height. Additionally, a significant positive correlation was exhibited between SDMA and internode diameter.
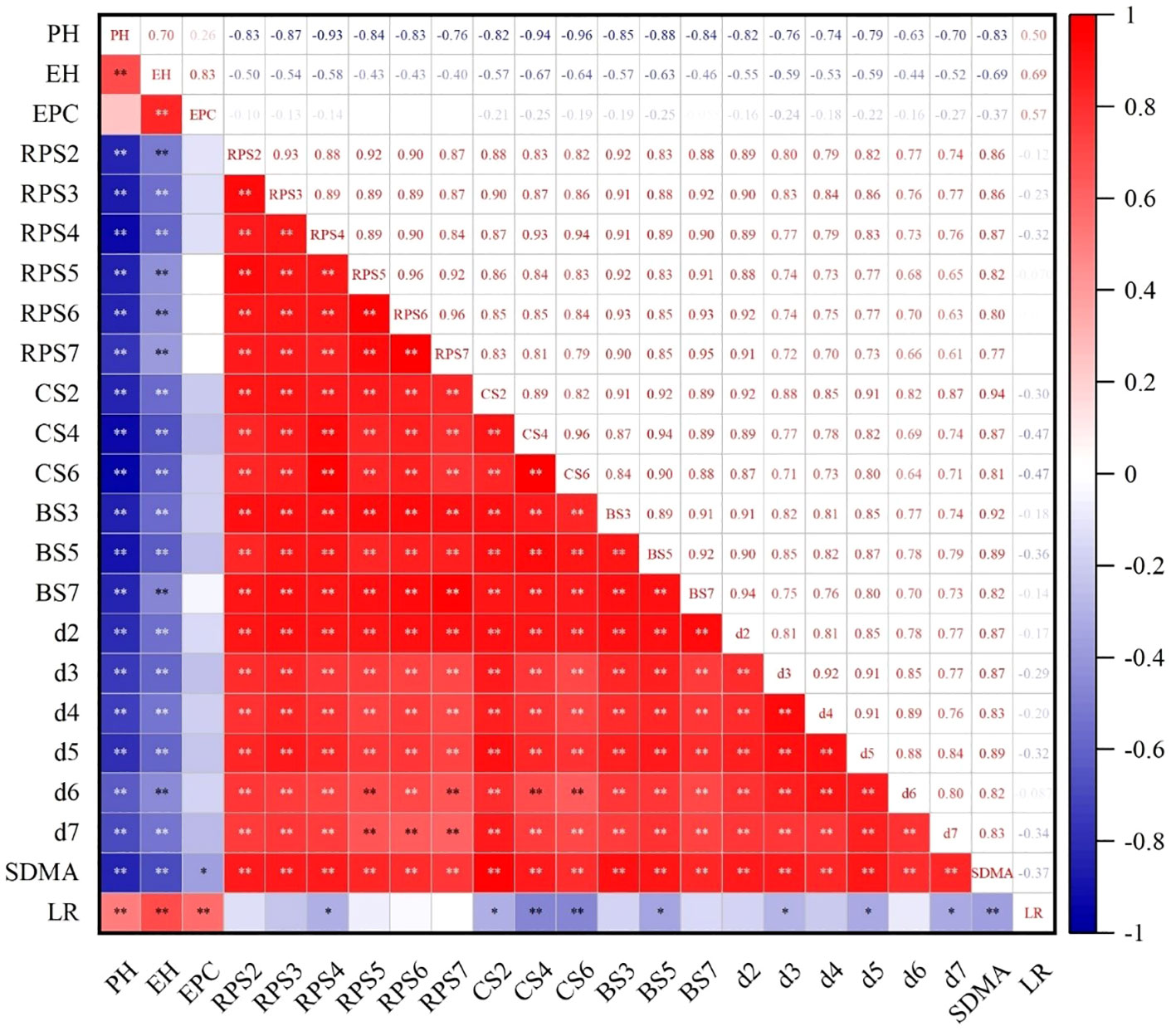
Figure 9 Correlation analysis among indicators. PH, plant height; EH, ear height; ER, ear ratio; BS, bending strength; CS, crushing strength; RPS, rind penetration strength; d, internode diameter; SDMA, stalk dry matter accumulation; LR, lodging rate. ** and *, significances at the 0.01 and 0.05 probability levels, respectively.
3.9 Effects of tillage and planting density on stalk internal chemical component content
As can be seen in Figure 10, stalk cellulose, hemicellulose, and lignin content decreased significantly at increasing density. Compared to RT, stalk cellulose, hemicellulose, and lignin content increased under SS conditions, which was more significant at D2 density. XY335 and ZD2 cellulose increased by 11.83% and 12.38%, respectively, hemicellulose increased by 15.97% and 6.7%, and lignin increased by 15.9% and 9.86%.
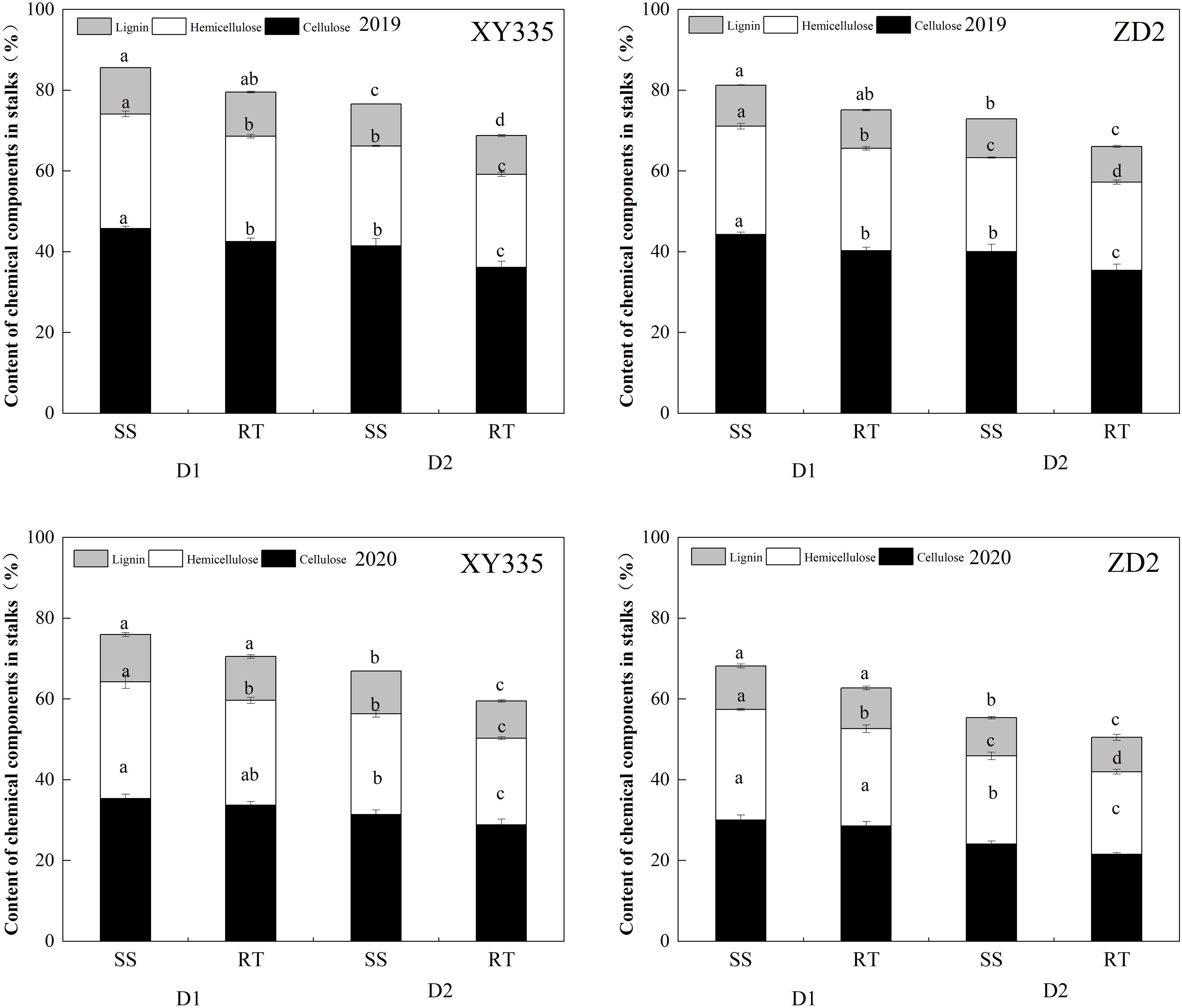
Figure 10 Stalk internal chemical component content under different treatments in 2019–2020. Different lowercase letters indicate significant differences(p < 0.05) among various treatments; SS, subsoil; RT, rotary tillage; XY335, Xian yu335; ZD2, Zhong dan 2. D1, 75,000 plants ha−1; D2, 105,000plants ha−1.
3.10 Correlation between stalk chemical content and stalk strength
Correlation analysis between the chemical component content in the stalks of maize and stalk bending strength showed that cellulose, hemicellulose, and lignin content were all highly significantly and positively correlated with stalk bending strength (Figure 11), with lignin content having the greatest correlation of 0.868 with stalk strength.
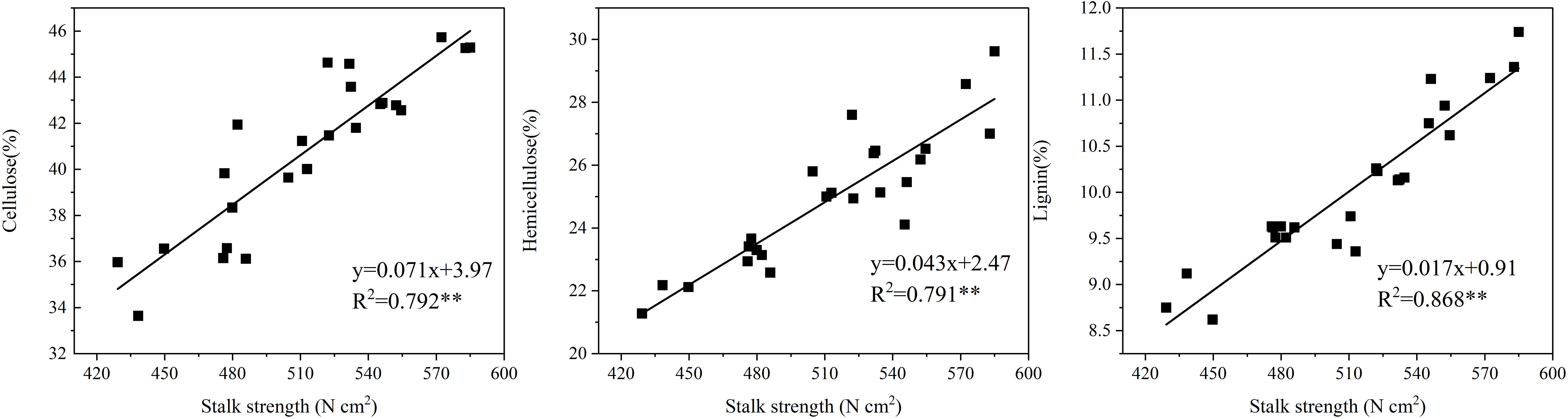
Figure 11 Correlation between stalk chemical content and stalk strength. **indicates significant correlation.
3.11 Effects of subsoil tillage and planting density on maize yield
Because the 2-year trend was consistent, the 2-year average was used to describe the results. Under D1 density, compared with RT, the yields of SS condition XY335 and ZD2 increased by 6.92% and 6.84%, and under D2 density, they increased by 8.17% and 7.58%, respectively. The yield increase of SS was greater under high density (Figure 12).
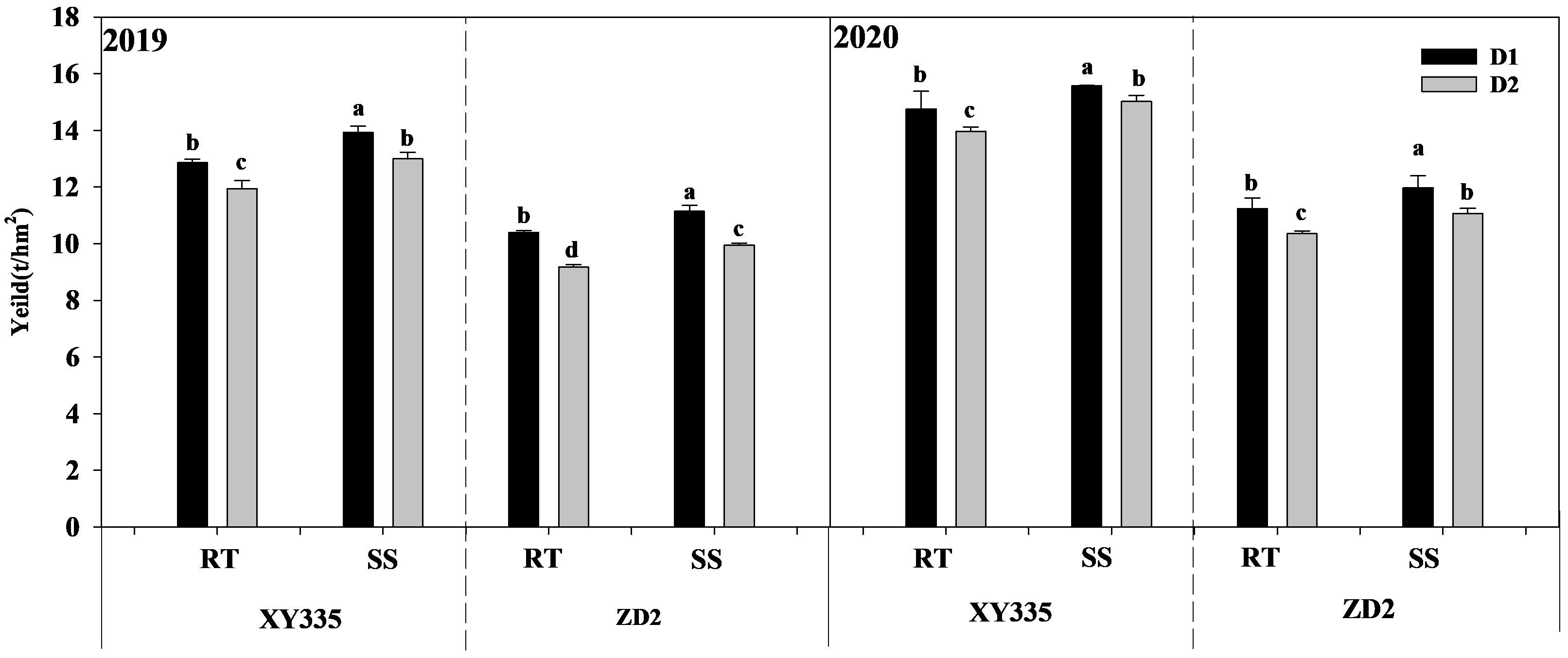
Figure 12 Effect of subsoil tillage and planting density on maize yield (t ha−1). Different lowercase letters indicate significant differences(p < 0.05) among various treatments; SS, subsoil; RT, rotary tillage; XY335, Xian yu335; ZD2, Zhong dan 2. D1, 75,000 plants ha−1; D2, 105,000plants ha−1.
4 Discussion
Maize crop lodging is detrimental to both yield and quality and is influenced by maize varieties and cultivation practices (Ma et al., 2014). Increased planting density will increase the risk of lodging. Implementing subsoil tillage measures has a substantial impact on reducing lodging. In this study, as the planting density grew, so did maize lodging rates. However, after SS tillage, the lodging rate of ZD2 decreased by 6.04%, indicating alleviation of the lodging pressure induced by increased plant density.
Research on maize stalk lodging has mainly focused on plant morphology, stalk mechanical properties, chemical composition, and anatomical structure, among other aspects (Zhang et al., 2021a). Plant height and ear height (EH) are considered the most crucial morphological features related to stalk lodging. Previous studies have also reported that lowering plant height can enhance a crop’s resistance to lodging (Shekoofa et al., 2008; Peng et al., 2014). Additionally, maize with high resistance to stalk lodging should also have a lower ear position to decrease its center of gravity (Shah et al., 2021). Xie et al. (2022) concluded that reducing the ear ratio significantly decreased the length of internodes below the ear, thereby improving the lodging resistance of maize stalks in China over the past 70 years. Furthermore, maize lodging is closely associated with SDMA (Xue et al., 2020b), stalk diameter, and mechanical properties of the stalk (Xue et al., 2020a). Currently, enhancing stalk mechanical strength, stalk diameter, RPS, and the unit length basal internode dry weight (DWUL) are considered important approaches to improve maize lodging resistance (Robertson et al., 2014; Xue et al., 2018b; Wang et al., 2020a). The significant positive correlation between plant lodging resistance to lignin content and its related enzyme activities and mechanical properties has been generally confirmed (Zhang et al., 2014; Sekhon et al., 2020). In this study, the correlation analysis indicated a significant negative correlation between plant height, ear height, and stalk lodging rate. Stalk thickness, SDMA, RPS, BS, and compressive strength exhibited a significant positive correlation with lodging rate. There was a significant positive correlation between lignin, hemicellulose, and cellulose within the stalks and the mechanical strength of the stalks. These findings are in basic agreement with previous studies.
In this study, as the planting density grew, the plant and ear height of maize increased. Compared to RT, SS tillage could reduce both plant and ear height, thereby lowering the center of gravity of maize. A lower center of gravity is more favorable for the lodging resistance of maize stalks (Shah et al., 2021). With increasing density, the stalk internode diameter, SDMA, and mechanical strength of stalk internodes decreased. In comparison to RT, SS practices could increase the stalk internode diameter and SDMA, enhancing internal lignin and cellulose content of the stalks, thus increasing the mechanical strength of the stalks. This effect was more pronounced under high-density conditions, and the response of lodging-resistant varieties to SS tillage was more evident. Moreover, under SS conditions, the negative impact of high-density stress on stalk dry matter and mechanical strength was less than that under shallow rotation. This indicates that SS tillage can attenuate the negative effects of high-density stress on lodging-related indicators of stalk resistance.
SS tillage can improve the lodging problem caused by high-density planting, possibly because subsoil practices effectively break the plough pan, reduce soil volume (Yu et al., 2023), and decrease soil bulk density and compaction (Lou et al., 2021). This enhances soil porosity and cultivation thickness (Wang et al., 2019), altering soil physical properties and establishing a reasonable tillage layer structure. This is more conducive to the downward growth of maize roots, leading to variations in maize root characteristics. Compared to shallow rotation, subsoiling techniques are more favorable for the growth of maize roots, thereby increasing root biomass and anchorage capacity (Sun et al., 2017). Additionally, SS tillage can enhance root vitality, facilitate water and nutrient absorption, and delay root aging (Yin et al., 2021). A strong root system could increase root anchorage and absorptive capacity for water and nutrients, leading to high yield and resistance to root lodging (Manzur et al., 2014). Root development and stalk development complement each other, and well-developed roots promote the accumulation of stalk material and the formation of mechanical strength, ultimately improving maize lodging resistance.
Previous studies have shown that SS tillage is advantageous for regulating soil nutrients and breaking up soil compaction (Zhang et al., 2021b). Bian et al. (2016) reported that under shallow rotation, soil compaction caused poor root development in summer maize, leading to low lodging resistance of summer maize. Moreover, SS tillage can increase maize emergence rate, plant height, and uniformity, and enhance leaf area index (LAI), affecting photosynthetic characteristics. SS tillage enhances the photosynthetic rate, stomatal conductance, and transpiration rate, and promotes the accumulation of aboveground biomass (Wu et al., 2020). Various studies have supported that SS tillage significantly improves the transport efficiency of stalk vascular bundles, influences maize stalk characteristics, strengthens stalk strength, and ultimately enhances lodging resistance (Liu et al., 2013). SS tillage can also increase soil water storage (Brunel-Saldias et al., 2018; Zhou et al., 2019). As maize reaches the high-growth period, the demand for water increases and soil water storage may decrease. SS tillage is more conducive to the downward movement of water after irrigation or rainfall, effectively increasing moisture content in the deep soil layer (Wang et al., 2019), promoting the formation and accumulation of plant biomass. These factors could contribute to improving maize lodging resistance.
5 Conclusion
Subsoil tillage improved the maize lodging resistance, and its effects were more pronounced under high planting density. The lodging rate of maize was significantly negatively correlated with plant height, ear height, and ear position coefficient. It also significantly negatively correlated with stalk diameter, mechanical strength of the base internode, and biomass accumulation. The CS at the fourth and sixth internodes exhibited relatively high correlation coefficients with the lodging rate. Subsoil increases the mechanical strength of stalks by increasing the internal cellulose, hemicellulose, and lignin content of the stalks. SS tillage under high density reduces plant and ear height while elevating stalk diameter, dry matter accumulation, and the mechanical strength of the stalk, leading to an effective reduction in the maize lodging rate. In combination with stalk lodging-resistant varieties (XY335), SS tillage can effectively alleviate the problem of stalk lodging after intensive planting. The findings of this study provide new ideas for improving maize’s resistance to lodging.
Data availability statement
The raw data supporting the conclusions of this article will be made available by the authors, without undue reservation.
Author contributions
XF: Data curation, Formal analysis, Investigation, Writing – original draft, Writing – review & editing. DM: Conceptualization, Methodology, Writing – review & editing. TL: Investigation, Writing – review & editing. SH: Investigation, Writing – review & editing. XY: Project administration, Writing – review & editing. JG: Project administration, Writing – review & editing.
Funding
The author(s) declare financial support was received for the research, authorship, and/or publication of this article. This study was funded by the National Corn Industry Technology System Project (CARS-02-50); the Inner Mongolia Autonomous Region Science and Technology Major Special Project (2021ZD0003); the Key Laboratory of Crop Cultivation and Genetic Improvement in Inner Mongolia Autonomous Region (2021PT0004); the Key Laboratory of High Yield and Efficient Cultivation and Cultivation of Crops in Northern Cold and Arid Regions of the Ministry of Agriculture and Rural Affairs; the Inner Mongolia Autonomous Region Graduate Research Innovation Funding Project (DC2300002869); and the Inner Mongolia Autonomous Region Graduate Research Innovation Funding Project (DC2300002049). Basic Research Funds Program for University of the Inner Mongolia (BR22-12-02, BR221019) and Program for improving the Scientific Research Ability of Youth Teachers of Inner Mongolia Agricultural University (BR220121, BR220119).
Conflict of interest
The authors declare that the research was conducted in the absence of any commercial or financial relationships that could be construed as a potential conflict of interest.
Publisher’s note
All claims expressed in this article are solely those of the authors and do not necessarily represent those of their affiliated organizations, or those of the publisher, the editors and the reviewers. Any product that may be evaluated in this article, or claim that may be made by its manufacturer, is not guaranteed or endorsed by the publisher.
References
Bian, D. H., Jia, G. P., Cai, L. J., Ma, Z. Y., Eneji, A. E., Cui, Y. H. (2016). Effects of tillage practices on root characteristics and root lodging resistance of maize. Field Crops Res. 185, 89–96. doi: 10.1016/j.fcr.2015.10.008
Brunel-Saldias, N., Seguel, O., Ovalle, C., Acevedo, E., Martínez, I. (2018). Tillage effects on the soil water balance and the use of water by oats and wheat in a Mediterranean climate. Soil Tillage Res. 184, 68–77. doi: 10.1016/j.still.2018.07.005
Da, B. X., Zhao, G., Wan, Y., Tan, M. L., Song, C., Song, Y. (2016). Effect of planting density on lodging-related morphology, lodging rate, and yield of tartary buckwheat (Fagopyrum tataricum). Plant Product. Sci. 19, 479–488. doi: 10.1080/1343943X.2016.1188320
Ghose, B. (2014). Food security and food self-sufficiency in China: from past to 2050. Food Energy Secur. 3, 86–95. doi: 10.1002/fes3.48
Hall, D. J. M., Jones, H. R., Crabtree, W. L., Daniels, T. L. (2010). Claying and deep ripping can increase crop yields and profits on water repellent sands with marginal fertility in southern Western Australia. Soil Res. 48, 178–187. doi: 10.1071/SR09078
Hamza, M. A., Anderson, W. K. (2005). Soil compaction in crop** systems: A review of the nature, causes and possible solutions. Soil tillage Res. 82, 121–145. doi: 10.1016/j.still.2004.08.009
Kamran, M., Ahmad, I., Wang, H., Wu, X., Xu, J., et al. (2018). Mepiquat chloride application increases lodging resistance of maize by enhancing stem physical strength and lignin biosynthesis. Field Crops Res. 224, 148–159. doi: 10.1016/j.fcr.2018.05.011
Laddha, K. C., Totawat, K. L. (1997). Effects of deep tillage under rainfed agriculture on production of sorghum (Sorghum biocolor L. Moench) intercropped with green gram (Vigna radiata L. Wilczek) in western India. Soil tillage Res. 43, 241–250. doi: 10.1016/S0167-1987(97)00027-5
Li, L., He, L. X., Li, Y. Q., Wang, Y. F., Ashraf, U., Hamoud, Y. A., et al. (2023). Deep fertilization combined with straw incorporation improved rice lodging resistance and soil properties of paddy fields. Eur. J. Agron. 142, 126659. doi: 10.1016/j.eja.2022.126659
Li, S., Wang, C. (2010). Innovation and diffusion of corn production technology (Science Press: Beijing).
Li, S. Y., Wang, Y., Hu, C., Yan, Y. (2015). Effects of strong wind lodging at pre-and post-tasseling stages on growth and yield of summer maize. Yingyong Sheng tai Xue bao 26 (08), 2405–2413. doi: 10.13287/j.1001-9332.20150521.018
Li, S. K., Wang, K. R., Xie, R. Z., Hou, P., Ming, B., Yang, X. X., et al. (2016). Implementing higher population and full mechanization technologies to achieve high yield and high efficiency in maize production. Crops 4, 1–6.
Liu, X. M., Gu, W. R., Li, C. F., Li, J., Shi, W. E. I. (2021). Effects of nitrogen fertilizer and chemical regulation on spring maize lodging characteristics, grain filling and yield formation under high planting density in Heilongjiang Province, China. J. Integr. Agric. 20, 511–526. doi: 10.1016/S2095-3119(20)63403-7
Liu, M., Qi, H., Zhang, W., Zhang, Z., Li, X., Song, Z., et al. (2013). Effects of deep loosening and nitrogen application on anatomical structures of stalk and lodging in maize. J. Maize Sci. 21, 57–63.
Lou, S., He, J., Li, H., Wang, Q., Lu, C., Liu, W., et al. (2021). Current knowledge and future directions for improving subsoiling quality and reducing energy consumption in conservation fields. Agriculture 11, 575. doi: 10.3390/agriculture11070575
Ma, D., Xie, R., Liu, X., Niu, X., Hou, P., Wang, K., et al. (2014). Lodging-related stalk characteristics of maize varieties in China since the 1950s. Crop Sci. 54, 2805–2814. doi: 10.2135/cropsci2014.04.0301
Manzur, M. E., Hall, A. J., Chimenti, C. A. (2014). Root lodging tolerance in Helianthus annuus (L.): associations with morphological and mechanical attributes of roots. Plant Soil 381, 71–83. doi: 10.1007/s11104-014-2122-9
Peng, D., Chen, X., Yin, Y., Lu, K., Yang, W., Tang, Y., et al. (2014). Lodging resistance of winter wheat (Triticum aestivum L.): Lignin accumulation and its related enzymes activities due to the application of paclobutrazol or gibberellin acid. Field Crops Res. 157, 1–7. doi: 10.1016/j.fcr.2013.11.015
Qi, B., Hu, J., Zhu, L., Duan, Y., Zhang, W., Gou, L. (2023). Response of maize stalk to plant density on cellulose accumulation by modulating enzymes activities. Field Crops Res. 304, 109152. doi: 10.1016/j.fcr.2023.109125
Robertson, D., Smith, S., Gardunia, B., Cook, D. (2014). An improved method for accurate phenotyping of corn stalk strength. Crop Sci. 54, 2038–2044. doi: 10.2135/cropsci2013.11.0794
Sekhon, R. S., Joyner, C. N., Ackerman, A. J., McMahan, C. S., Cook, D. D., Robertson, D. J. (2020). Stalk bending strength is strongly associated with maize stalk lodging incidence across multiple environments. Field Crop Res. 249, 107737. doi: 10.1016/j.fcr.2020.107737
Shah, A. N., Tanveer, M., Abbas, A., Yildirim, M., Shah, A. A., Ahmad, M. I., et al. (2021). Combating dual challenges in maize under high planting density: Stem lodging and kernel abortion. Front. Plant Sci. 12. doi: 10.3389/fpls.2021.699085
Shekoofa, A., Emam, Y. (2008). Plant growth regulator (ethephon) alters maize (Zea maps L.) growth. J. Agron. 7. doi: 10.3389/fpls.2018.010047
Sher, A., Khan, A., Ashraf, U., Liu, H. H., Li, J. C. (2018). Characterization of the effect of increased plant densit484 y on canopy morphology and stalk lodging risk. Frontiers in Plant Science 9, 1047. doi: 10.3389/fpls.2018.014850047
Shi, D. Y., Li, Y. H., Zhang, J. W., Liu, P., Zhao, B., Dong, S. T. (2016). Effects of plant density and nitrogen rate on lodging-related stalk traits of summer maize. Plant Soil Environ. 62, 299–306. doi: 10.17221/720/2015-PSE
Sun, X., Ding, Z., Wang, X., Hou, H., Zhou, B., Yue, Y., et al. (2017). Subsoiling practices change root distribution and increase post-anthesis dry matter accumulation and yield in summer maize. PloS One 12, e0174952. doi: 10.1371/journal.pone.0174952
Sun, J., Gao, J., Wang, Z., Hu, S., Zhang, F., Bao, H., et al. (2018). Maize canopy photosynthetic efficiency, plant growth, and yield responses to tillage depth. Agronomy 9, 3. doi: 10.3390/agronomy9010003
Tigchelaar, M., Battisti, D. S., Naylor, R. L., Ray, D. K. (2018). Future warming increases probability of globally synchronized maize production shocks. Proc. Natl. Acad. Sci. 115, 6644–6649. doi: 10.1073/pnas.1718031115
Varsa, E. C., Chong, S. K., Abolaji, J. O., Farquhar, D. A., Olsen, F. J. (1997). Effect of deep tillage on soil physical characteristics and corn (Zea mays L.) root growth and production. Soil Tillage Res. 43, 219–228. doi: 10.1016/S0167-1987(97)00041-X
Wang, S., Guo, L., Zhou, P., Wang, X., Shen, Y., Han, H., et al. (2019). Effect of subsoiling depth on soil physical properties and summer maize (Zea mays L.) yield. Plant Soil Environ. 65 (3), 131–137. doi: 10.17221/703/2018-PS
Wang, S., Wang, H., Hafeez, M. B., Zhang, Q., Yu, Q., Wang, R., et al. (2020b). No-tillage and subsoiling increased maize yields and soil water storage under varied rainfall distribution: A 9-year site-specific study in a semi-arid environment. Field Crops Res. 255, 107867. doi: 10.1016/j.fcr.2020.107867
Wang, Q., Xue, J., Chen, J. L., Fan, Y. H., Zhang, G. Q., Xie, R. Z., et al. (2020a). Key indicators affecting maize stalk lodging resistance of different growth periods under different sowing dates. J. Integr. Agric. 19, 2419–2428. doi: 10.1016/S2095-3119(20)63259-2
Wu, W., Wang, S., Zhang, L., Li, J., Song, Y., Peng, C., et al. (2020). Subsoiling improves the photosynthetic characteristics of leaves and water use efficiency of rainfed summer maize in the Southern Huang-Huai-Hai Plain of China. Agronomy 10, 465. doi: 10.3390/agronomy10040465
Xie, R. Z., Yu, X. F., Li, S. K., Gao, J. L. (2022). Historical trends in maize morphology from the 1950s to the 2010s in China. J. Integr. Agric. 21, 2159–2167. doi: 10.1016/S2095-3119(21)63697-3
Xu, J., Han, H., Ning, T., Li, Z., Lal, R. (2019). Long-term effects of tillage and straw management on soil organic carbon, crop yield, and yield stability in a wheat-maize system. Field Crops Res. 233, 33–40. doi: 10.1016/j.fcr.2018.12.016
Xue, J., Gao, S., Fan, Y., Li, L., Ming, B., Wang, K., et al. (2020a). Traits of plant morphology, stalk mechanical strength, and biomass accumulation in the selection of lodging-resistant maize cultivars. Eur. J. Agron. 117, 126073. doi: 10.1016/j.eja.2020.126073
Xue, J., Li, L., Xie, R., Wang, K., Hou, P., Ming, B., et al. (2018a). Effect of lodging on maize grain losing and harvest efficiency in mechanical grain harvest. Acta Agronom. Sin. 44, 1774–1781. doi: 10.3724/SP.J.1006.2018.01774
Xue, J., Ming, B., Xie, R., Wang, K., Hou, P., Li, S. (2020b). Evaluation of maize lodging resistance based on the critical wind speed of stalk breaking during the late growth stage. Plant Methods 16, 1–12. doi: 10.1186/s13007-020-00689-z
Xue, J., Wang, Q., Li, L., Zhang, W., Xie, R., Wang, K., et al. (2018b). Changes of maize lodging after physiological maturity and its influencing factors. Acta Agronom. Sin. 44, 1782–1792. doi: 10.3724/SP.J.1006.2018.01782
Yin, B., Hu, Z., Wang, Y., Zhao, J., Pan, Z., Zhen, W. (2021). Effects of optimized subsoiling tillage on field water conservation and summer maize (Zea mays L.) yield in the North China Plain. Agric. Water Manage. 247, 106732. doi: 10.1016/j.agwat.2020.106732
Yu, X., Qu, J., Hu, S., Xu, P., Chen, Z., Gao, J., et al. (2023). The effect of tillage methods on soil physical properties and maize yield in Eastern Inner Mongolia. Eur. J. Agron. 147, 126852. doi: 10.1016/j.eja.2023.126852
Yu, X. F., Sun, H. L., Gao, J. L., Wang, Z. G., Yang, H. S., Zhang, R. F., et al. (2013). Mechanism behind densification and yield increase of spring maize with different density-tolerance regulated by subsoiling. Trans. Chin. Soc. Agric. Eng. (Transactions CSAE) 35, 35–46. doi: 10.11975/j.issn.1002-6819.2019.13.004
Zhang, P., Gu, S., Wang, Y., Yang, R., Yan, Y., Zhang, S., et al. (2021a). Morphological and mechanical variables associated with lodging in maize (Zea mays L.). Field Crops Res. 269, 108178. doi: 10.1016/j.fcr.2021.108178
Zhang, J., Li, G. H., Song, Y. P., Liu, Z. H., Yang, C. D., Tang, S., et al. (2014). Lodging resistance characteristics of high-yielding rice populations. Field Crop Res. 161, 64–74. doi: 10.1016/j.fcr.2014.01.012
Zhang, Y., Tan, C., Wang, R., Li, J., Wang, X. (2021b). Conservation tillage rotation enhanced soil structure and soil nutrients in long-term dryland agriculture. Eur. J. Agron. 131, 126379. doi: 10.1016/j.eja.2021.126379
Zhang, P., Yan, Y., Gu, S., Wang, Y., Xu, C., Sheng, D., et al. (2022). Lodging resistance in maize: A function of root–shoot interactions. Eur. J. Agron. 132, 126393. doi: 10.1016/j.eja.2021.126393
Zhang, R. F., Yang, H. S., Gao, J. L., Zhang, Y. Q., Wang, Z. G., Fan, X. Y., et al. (2015). Effect of subsoiling on root morphological and physiological characteristics of spring maize. Trans. Chin. Soc. Agric. Eng. (Transactions CSAE) 31, 78–84. doi: 10.3969/j.issn.1002-6819.2015.05.012
Zheng, Y. X., Chen, D., Wei, P. C., Lu, P., Yang, J. Y., Li, S. K., et al. (2021). Effects of planting density on lodging resistance and grain yield of spring maize stalks in Guizhou province. Acta agronom. Sin. 47, 738751. doi: 10.3724/SP.J.1006.2021.03044
Keywords: maize, subsoil tillage, stalk morphology, stalk strength, lodging rate
Citation: Feng X, Ma D, Lei T, Hu S, Yu X and Gao J (2024) Subsoil tillage improved the maize stalk lodging resistance under high planting density. Front. Plant Sci. 15:1396182. doi: 10.3389/fpls.2024.1396182
Received: 05 March 2024; Accepted: 12 June 2024;
Published: 17 July 2024.
Edited by:
Miroslav Zoric, LoginEKO Research and Development Center, SerbiaReviewed by:
Baoyuan Zhou, Institute of Crop Sciences (CAAS), ChinaAlam Sher, Anhui Agricultural University, China
Agnieszka Synowiec, University of Agriculture in Krakow, Poland
Congfeng Li, Chinese Academy of Agricultural Sciences, China
Copyright © 2024 Feng, Ma, Lei, Hu, Yu and Gao. This is an open-access article distributed under the terms of the Creative Commons Attribution License (CC BY). The use, distribution or reproduction in other forums is permitted, provided the original author(s) and the copyright owner(s) are credited and that the original publication in this journal is cited, in accordance with accepted academic practice. No use, distribution or reproduction is permitted which does not comply with these terms.
*Correspondence: Xiaofang Yu, eXV4aWFvZmFuZzc1QDE2My5jb20=; Julin Gao, bm1nYW9qdWxpbkAxNjMuY29t