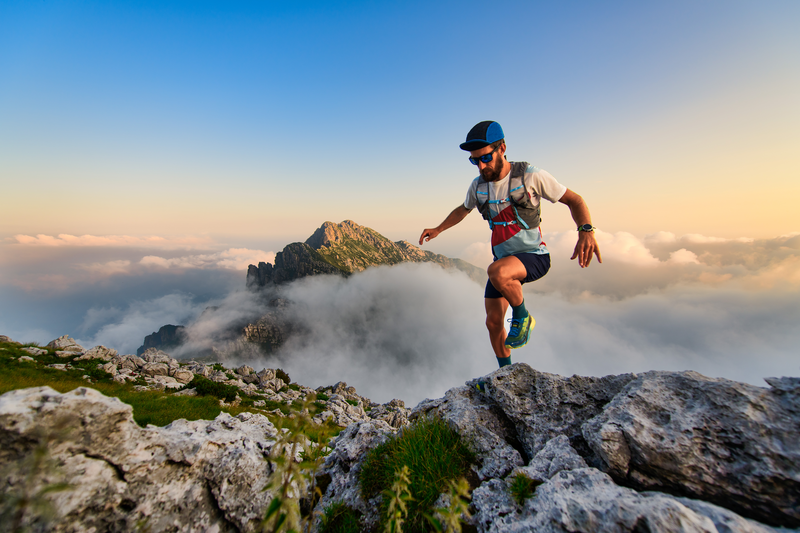
94% of researchers rate our articles as excellent or good
Learn more about the work of our research integrity team to safeguard the quality of each article we publish.
Find out more
ORIGINAL RESEARCH article
Front. Plant Sci. , 09 October 2024
Sec. Functional Plant Ecology
Volume 15 - 2024 | https://doi.org/10.3389/fpls.2024.1396004
This article is part of the Research Topic Flourishing in Arid Realms: Exploring the Adaptation of Plant Functional Traits to Drought Environments View all 16 articles
Introduction: The impacts of climate change can be profound in many ecosystems worldwide, including drylands such as arid and semi-arid scrublands and grasslands. Foundation plants such as shrubs can provide microclimatic refuges for a variety of taxa. These shrubs can directly influence micro6 environmental measures, and indirectly increase the local environmental heterogeneity as a result. We examined the hypothesis that, in comparison to an open gap, foundation shrubs improve the microclimate beneath their canopy and that microclimate is in turn a significant predictor of annual vegetation. The following predictions were made: 1) mean air temperature (NSAT), ground temperature (SGT), and vapour pressure deficit (VPD) will be significantly lower under the shrubs than in the open microsites; 2) shrub canopy size predicts microclimate; 3) site-level aridity estimates and percent shrub cover influence annual plant abundance and richness; and 4) the site13 level mean of NSAT and VPD predict annual plant abundance and richness.
Methods: Our study took place in Southwestern California, U.S.A. We used a handheld device with a probe to measure microclimatic variables such as near-surface air temperature (NSAT), near-surface relative humidity (NSRH), and surface ground temperature (SGT) at the shrub species Ephedra californica and in the open gap, across six sites in California, United States. Air temperature and RH were then used to calculate VPD. The mean number of vascular plant species across each site was also recorded.
Results & discussion: Only SGT was significantly reduced under shrub canopies. Canopy volume was not a significant predictor of all three microclimatic variables, demonstrating that even small, low-stature shrubs can have facilitative effects. Furthermore, total shrub cover and aridity at sites significantly predicted mean plant richness and abundance. There were significantly more plants associated with shrubs and there were significantly more species associated with the open. Mean air temperature and VPD at the site-level significantly predicted vegetation abundance and richness, though microsite-level differences were only significant for richness. Foundation shrubs are a focal point of resiliency in dryland ecosystems. Understanding their impact on microclimate can inform us of better management, conservation, and restoration frameworks.
Facilitation, defined as an interaction where one or more species (beneficiary) benefit from another species (benefactor) while none are harmed (Bertness and Leonard, 1997), is well-studied in community ecology (Brooker et al., 2007; McIntire and Fajardo, 2014). This interaction is often present in relatively high-stress environments, such as drylands, and generally, the magnitude of this interaction also increases with an increase in abiotic pressures (Bertness and Callaway, 1994). Foundation species like shrubs can act as structural agents of facilitation through many mechanistic pathways, one of which is microclimatic amelioration (Filazzola et al., 2017). Shrub understories provide more favorable environments for plants and animals by buffering against extreme temperatures, offering refuge from direct solar radiation, and increasing soil moisture (Filazzola and Lortie, 2014; Flores and Jurado, 2003; Holzapfel and Mahall, 1999). Thus, foundation shrubs are critical for community structure and diversity in many dryland ecosystems (Ivey et al., 2020; Zuliani et al., 2021).
Foundation shrubs are key components of environmental heterogeneity. Foundation shrubs physically ameliorate the understory microclimate compared to the open gap (Anthelme et al., 2014; Filazzola et al., 2017; Zuliani et al., 2021); thus, resulting in environmental heterogeneity as a byproduct of this microclimatic difference. Environmental heterogeneity (EH), defined as non-uniformities in physical and ecological landscape characteristics (Dronova, 2017), can be divided into many subcomponents including climatic, vegetation, land cover, soil, and topography heterogeneity (Stein and Kreft, 2015). ‘Microclimate’ is the climate experienced in the lower 2m of the atmosphere and the upper 0.5-1m of the soil and is dependent on local topography, soil type, and vegetation (Stoutjesdijk and Barkman, 2014). Foundation plans provide resilience and microclimatic heterogeneity in drylands (Lortie et al., 2022), where elevated mean temperatures, fluctuations in temperature and precipitation, and prolonged drought episodes are on the rise (Maestre et al., 2021). Resiliency is a concept that refers to the ability of a system to resist changes in response to perturbations (Van Meerbeek et al., 2021). Plants can buffer perturbations that are the result of harsh climatic conditions (De Frenne et al., 2019). The impacts of plants in buffering climate have been primarily tested through landscape-level analyses, such as NDVI (Normalized Difference Vegetation Index) or vegetation land cover (Bagley et al., 2017); however, the structure of foundation species offers significant ecological functions that are crucial for offering climatic refuge at finer scales (Milling et al., 2018).
Like plant canopies in other systems, shrub canopies can, directly and indirectly, buffer climatic regimes. Plant canopies offer mediation from harsh climates by increasing environmental heterogeneity and cover (De Frenne et al., 2019). The quantity, quality, and temporal distribution of incoming sunlight are governed by the canopy’s structure, which also affects air movement, which in turn affects temperature and precipitation regimes via boundary layer effects (Jennings et al., 1998). Shrub volume is directly associated with micro-environmental conditions, with larger shrubs showing a greater environmental heterogeneity in their understory (Alday et al., 2014). Similar to other studies with plants (Yang et al., 2017), this is likely due to the greater heterogeneity of conditions beneath larger plant canopies. Many dryland organisms are vulnerable to small, fine-scale oscillations in addition to large-scale changes (Shrode and Gerking 1977; Hadley 1970), which can further push species past the point of no return. Thus, foundation shrubs, including Ephedra californica, can help facilitate some of these species (Lortie et al., 2018) through small-scale climatic amelioration. The idea of vegetation and microclimate in arid systems is well-explored (El-Bana et al., 2002; Jankju, 2013; Xue et al., 2019) but not necessarily concerning the size of the foundation species at multiple arid sites. Shrub volume measured and reported to such an extent at multiple arid sites in addition to its impact on annual plant richness and abundance is where the novelty of this study lies.
Ephedra Californica (Mormon Tea) is a foundation shrub native to the Southwestern regions of California that can occur dominantly or co-dominantly with Larrea tridentata (Sawyer et al., 2009), a flowering shrub that is often found in sandy soils, desert pavements, and the well-developed cryptogram layer of the Mojave (Braun et al., 2021; Sawyer et al., 2009). In this study, we measured microclimatic variables, including near-surface air temperature (NSAT), near-surface relative humidity (NSRH), and surface ground temperature (SGT) using a handheld device across six sites in California. NSAT is a point-station, air temperature measure that differs from a direct land surface measure of temperature and is a critical factor in the process of energy exchange between the land and the atmosphere (Xu et al., 2012). SGT (also referred to as Land Surface Temperature in the literature) is the measure of temperature directly on land as opposed to NSAT (Good et al., 2017). Similar to NSAT, NSRH refers to the measurement of the air moisture content near the surface (Pfahl and Niedermann, 2011). We used NSAT and NSRH to calculate vapor pressure deficit (VPD). We tested the hypothesis that foundation shrubs ameliorate the microclimate underneath their canopy relative to the open gap and that microclimate is a significant predictor of annual vegetation. The following predictions were made: 1) mean air temperature (NSAT), ground temperature (SGT), and vapor pressure deficit (VPD) will be significantly lower under the shrubs than in the open microsites; 2) shrub canopy size predicts microclimate; 3) site-level aridity estimates and percent shrub cover influence annual plant abundance and richness; and 4) the site-level mean of NSAT and VPD predict annual plant abundance and richness. Shrubs and vegetation improve microclimate and can thus provide key environmental heterogeneity that is crucial to the persistence of many dryland species given the current trajectory of climate change.
We surveyed a total of six sites across arid and semi-arid areas during the winter of 2023 (Table 1). The sites were located in Southwestern California, United States, and divided into two main ecological areas: Cuyama Valley and Carrizo Plain National Monument. The surveys took place between February 13 and 23, 2023. We surveyed a total of three sites in Cuyama Valley and three sites in the Carrizo Plain. Sites were always surveyed in the morning at approximately 9:00 AM. The year this study took place was considered a high precipitation year, characterized by a ‘superbloom’ and winter rainfall exceeding the long-term means by ≥70% (Jennings and Berry, 2023). We chose this study period because the early species of annuals normally begin germinating in March (Jennings and Berry, 2023), but because of the superbloom and high rainfall periods, they germinated earlier in late January (Jennings, 2001). We retrieved daily min and maximum temperatures (F) and precipitation (in) for each day of the study period from the National Oceanic and Atmospheric Administration (NOAA) (https://www.noaa.gov/; Supplementary Appendix C) using the nearest satellite located in New Cuyama, Cuyama Valley. Moreover, site-level mean annual temperature (MAP) and mean annual precipitation (MAP) were obtained from WorldClim (https://www.worldclim.org/data/index.html) at a 1km resolution. We further calculated DeMartonne’s aridity values using the following formula: aridity = P/(T + 10) where P = annual precipitation and T = mean annual temperature and classified the climate gradient according to Croitoru et al. (2013).
Table 1. Sites. List of study sites in California, United States surveyed for this study and their respective geographical coordinates.
We selected 30 pairs of shrub-open microsites at each study site. Ephedra californica was the dominant shrub across all these sites and there were no other dominant or co-dominant shrub species present. The length (at longest axis), width (perpendicular to length), and height to the tallest vegetation branch that had green tissue (Mormon tea is a shrub with many branches) were measured for every shrub (Lortie et al., 2018). We used the equation for the volume of a hemisphere () to calculate canopy volume and the equation for the area of a circle () to calculate the canopy area (Liczner et al., 2016; Lortie et al., 2018). We recorded the percent shrub cover at each site within a 20m radius at the center of the site via Google Earth composite satellite images at a spatial resolution of 30cm (Hestrio et al., 2021; Owen et al., 2024). Within each circular plot (20m in radius), the length at the longest axis of every shrub was measured and used to calculate the individual shrub cover area using a formula of a circle (Liczner et al., 2016; Lortie et al., 2018). Shrub covers were then summed and divided by the total area of the circular plot to produce percent shrub cover estimates of each site (Supplementary Appendix D, L).
We used the Mengshen Digital Temperature and Humidity Meter (Mengshen, 2023) to record near-surface air temperature (NSAT, °C) and near-surface relative humidity (NSRH, %). To record these microclimatic variables, the handheld probe was positioned at approximately ∼10-15cm above the soil at the north side of the shrub, immediately within the canopy dripline (inside), and 1m away from a shrub in the adjacent, interstitial open area (outside). We used a cover to stop direct sunlight on the probe bulbs when air temperature and RH were being recorded. Furthermore, we also used the Etekcity Laser Thermometer Gun (Etekcity, 2023) to record surface ground temperature (SGT, °C) both underneath the shrub canopy at the north side of the shrub, and in the open area located adjacently 1m away from a shrub. We then used air temperature and RH to calculate the vapor pressure deficit (VPD) as vegetation structure and microtopography, directly and indirectly, impact this measurement (Novick et al., 2024). We used the following equation: where saturation vapor pressure (SVP) is the maximum amount of water vapor that air can hold as a certain recorded temperature and RH = relative humidity.
To further explore if microclimate changes depending on the site-level differences in vegetation, we conducted vegetation surveys in shrub and open microsites at each study site. We identified all vascular plant species present in four paired 1 m2 quadrats at each microsite (8 total per site). In shrub microsites, one of the paired quadrats was placed under the drip line of randomly selected shrubs on the south-facing side and the paired open quadrat was placed due south with 1m spacing between the two quadrats. In open microsites, each pair of quadrats was randomly placed in what we deemed as the center of the site. We summarized the results of the eight plots to determine the mean annual plant abundance and richness.
All statistical analyses were done using R version 4.3.1 (R Core Team, 2024). Data and codes are publicly available on Zenodo (Ghazian and Lortie, 2023). Data distribution was examined using Q-Q plots, and homoscedasticity and normality were tested (Schützenmeister et al., 2012). The relationship between NSAT, SGT, NSRH, and VPD was examined using Pearson’s correlation (Benesty et al., 2009). The relationship between canopy area and volume was also examined using Pearson’s correlation, as well. We fit Generalized Linear Models (GLM) to test for differences in shrub volume and its effects on air temperature, VPD, and ground temperature for the open versus the shrub. GLM dispersion parameters with AIC scores were used to compare and select the appropriate family to fit to models (Richards et al., 2011). Post-hoc tests were done using the function emmeans from the emmeans R package (Lenth and Herve, 2019). We then used Generalized Linear Mixed Models (GLMM) to model shrub volume as a predictor of each microclimatic variable, with microsite nested in site to serve as a covariate. We further explored if mean plant richness and abundance were predicted by percent shrub cover, aridity, mean NSAT, and mean VPD using GLMs. We included predictors in these models by testing multicollinearity using the Performance package and excluded those that lead to high variance inflation factors (VIF) (Daoud, 2017), and hence high collinearity (Supplementary Appendix A, B).
A total of 180 shrub-open microsites were surveyed for microclimatic measures (n = 180). Mean shrub volume and area for E. californica were 39.64 ± 27.80 m3 and 37.16 ± 22.06 m2, respectively (Supplementary Appendix F). Shrub area and canopy volume were significantly, and positively related (Pearson’s product-moment correlation = 0.92, p<0.01; Supplementary Appendix G); thus, we used shrub volume as a proxy for size in all our models. Percent shrub cover at study sites ranged from 6.68-31.18% (Supplementary Appendix D). Table 2 provides the mean air temperature and VPD under the shrub and in the open at each site. The lowest mean air temperature was recorded in the open at Carrizo_soda_shrub (15.06 ± 3.48 °C) and the lowest mean VPD was recorded under the shrub (0.55 ± 0.16 kPa) in Cuyama_3. Figure 1 depicts the frequency distribution of change in air temperature between the open and the shrub in Kelvin. Mean ground temperature was the only microclimatic parameter significantly lower under the shrub than in the open (Estimated Marginalized Mean (EMM) 13.5 ± 0.39 °C, p<0.01; Figure 2).
Figure 1. Frequency distribution. The frequency distribution of temperature differences between the open and shrub is presented. The x-axis represents the difference in temperature in Kelvin while the y-axis represents the particular frequency ΔT that was observed. Dashed red line is the mean of temperature changes.
Figure 2. Shrub volume vs. microclimatic measures. The relationship between shrub volume (m3) and microclimatic measures including NSAT (°C), NSRH (%), and SGT (°C) are presented using points. Smoothed means are fitted using the linear method. Colors represent the different key microclimatic variables.
Overall, shrub volume did not have a significant effect on air temperature in fixed-effect models (GLMM estimated β = 0; Table 3). The same was observed for VPD (GLMM estimated (β = 0) and ground temperature (GLMM estimated β = -0.01). The confidence intervals for all three estimates were also narrow, which further confirms the weak effects of shrub volume on these microclimatic variables.
A total of 27 different annual plant species were found under shrubs and in the open (Supplementary Appendix E). Bromus madritensis rubens (red brome) was the most abundant species observed under the shrubs, while Lasthenia gracilis (needle goldfield) was the most abundant species in the open. Astragalus lentiginosus nigricalycis (spotted locoweed), Brassica nigra (black mustard), Lactuca serriola (prickly lettuce), Lupinus microcarpus (chick lupine) were the equally the least abundant species under the shrub. Lupinus microcarpus (chick lupine) was also the least abundant species in the open. There were four species exclusively found under shrubs, including Brassica nigra (black mustard), Eremalche exilis (white mallow), Lactuca serriola (prickly lettuce), Pholistoma membranaceum (white fiesta flower). Both site-level shrub cover and aridity were significant predictors of plant abundance and richness (Figure 3 and Table 4). Mean plant abundance was significantly greater under the shrubs (EMM 23 ± 1.82, p<0.01), while mean richness was significantly greater in the open (EMM 6.82 ± 0.16, p<0.01). Site-level mean air temperature and mean VPD were significant predictors of plant richness both under the shrub and in the open, but not abundance (Figure 4, Table 5).
Figure 3. Vegetation measures (I) Mean plant abundance and richness regressed against percent shrub cover (%) (A, B) and aridity (C, D). Smoothed means are fitted using the linear method.
Figure 4. Vegetation measures II. Mean plant abundance and richness regressed against mean NSAT (°C) (A, C) and mean VPD (kPa) (B, D). Smoothed means are fitted using the linear method. Color represents the microsite.
Abiotic amelioration via foundation shrubs is a key component of many dryland ecosystems. Herein, we examined the microclimate of the foundation shrub species, Ephedra californica in order to determine the relative significance of shrub volume on microclimate and the role of microclimate in predicting vegetation richness and abundance. These were tested across six sites in the drylands of California. We hypothesized that, in comparison to an open gap, foundation shrubs improve the microclimate beneath their canopy and that microclimate is in turn a significant predictor of annual vegetation. Despite our prediction that all microclimatic variables under the shrub would be significantly lower than in the open, we only observed significantly lower ground temperature under the shrub. Shrub volume was not a significant predictor of air temperature, ground temperature, and VPD. Thus, we propose that E. californica is a good benefactor species when it comes to facilitating some micro-environmental measures compared to the open gap; though, its role in microclimatic amelioration is weakly dependent on size. Furthermore, we concluded that site-level percent shrub cover and aridity are significant predictors of vascular plant abundance and richness. Mean air temperature and VPD at the site level significantly predicted vegetation richness and abundance, however, microsite-level differences were only significant to plant richness. Mean plant abundance was greater under shrubs suggesting that shrub cover has an impact on microclimate, and the environmental heterogeneity induced by shrubs that may act on different spatial scales, hence altering the dynamics of distribution and establishment of the herbaceous plant community (Madrigal et al., 2008). We suggest that one factor may be microclimatic amelioration, such as cooler ground temperatures, by foundation species that allow for the presence of species that would otherwise be absent. This is consistent with the results of Soliveres and Maestre (2014) who observed 29% more species associated with shrubs in drylands compared to the open. Furthermore, we observed that mean plant richness was higher in the open. We believe that this may be due to the fact some shrubs restrict the establishment and survivorship of certain annual plants because the reduction of light by shrub canopy can limit the photosynthetic activity of annuals (Swank and Oechel, 1991). Semi-arid annual plants attain high net photosynthetic rates by having very high light saturation levels (Werk et al., 1983). A reduction in radiation by shrubs can reduce resource acquisition (Jackson and Caldwell, 1992). Thus, we suggest that plant-plant interactions and their responses to ongoing climate change are more complex than previously thought (Seiferling et al., 2014), but these interactions are nonetheless crucial in maintaining biodiversity in dryland ecosystems, particularly given the number of species that exclusively associate with shrubs. Though beyond the scope of our study, we suggest that future experiments consider plant traits as a tool to understand why some species are more benefited than others.
More than 30% of the total land area in California is defined as a semi-arid ecoregion (Syphard et al., 2022). Shrubs create heterogeneity in these regions by creating variation in physical structure and thus provide a generalized facilitation function of ameliorating microclimate (Braun et al., 2021). Shrub canopies create micro-environmental benefits including increasing shade, reducing radiation load, increasing night-time winter temperature, and increasing soil moisture, and canopy structure play an important role in differences observed in these micro-environmental parameters (Jankju, 2013). In this study, we observed the facilitative effects of E. californica are not dependent on size. This shows that even the canopy of smaller, low-stature, long-lived ancient shrubs can nonetheless provide fine-scale climatic refuges for other species (Ivey et al., 2020; Lortie et al., 2018, 2020). Maintaining the biodiversity of semi-arid regions is crucial as it translates to the overall biodiversity of the entire region of California. There is a direct relationship between environmental heterogeneity (EH) and biodiversity, with species richness increasing with greater EH (Agra et al., 2021). Maintaining E. californica presence and promoting its persistence in the region through conservation, restoration, and management will result in greater heterogeneity in the region and thus higher regional biodiversity. Shrubs and annual plants enable small areas in the species niche that can allow species persistence in a shifting climate (Brooker et al., 2018). Thus providing animal species with the option to move around and behaviorally mitigate climate change effects. Hence, management and restoration efforts need to focus on not only protecting existing shrub species but also restoring and managing disturbed scrubland and they are key to the persistence of biodiversity. Supporting the persistence of other taxa through foundation shrubs like E. californica that both directly and indirectly influence microclimatic heterogeneity is crucial in the future well-being of dryland ecosystems under the current climate change paradigms.
The datasets presented in this study can be found in online repositories. The names of the repository/repositories and accession number(s) can be found in the article/Supplementary Material.
NG: Conceptualization, Data curation, Formal analysis, Investigation, Methodology, Project administration, Visualization, Writing – original draft, Writing – review & editing. RK: Conceptualization, Data curation, Investigation, Methodology, Writing – review & editing. MZ: Conceptualization, Investigation, Methodology, Writing – review & editing. CL: Conceptualization, Formal analysis, Funding acquisition, Investigation, Project administration, Resources, Supervision, Writing – review & editing.
The author(s) declare financial support was received for the research, authorship, and/or publication of this article. This research was made possible through a Natural Sciences and Engineering Research Council of Canada (NSERC) grant awarded to CL and the Ontario Graduate Scholarship (OGS) awarded to NG.
We are thankful to the United States Bureau of Land Management (BLM) for allowing us to conduct research on their land.
The authors declare that the research was conducted in the absence of any commercial or financial relationships that could be construed as a potential conflict of interest.
All claims expressed in this article are solely those of the authors and do not necessarily represent those of their affiliated organizations, or those of the publisher, the editors and the reviewers. Any product that may be evaluated in this article, or claim that may be made by its manufacturer, is not guaranteed or endorsed by the publisher.
The Supplementary Material for this article can be found online at: https://www.frontiersin.org/articles/10.3389/fpls.2024.1396004/full#supplementary-material
Agra, J., Ligeiro, R., Heino, J., Macedo, D. R., Castro, D. M. P., Linares, M. S., et al. (2021). Anthropogenic disturbances alter the relationships between environmental heterogeneity and biodiversity of stream insects. Ecol. Indic. 121, 107079. doi: 10.1016/j.ecolind.2020.107079
Alday, J. G., Santana, V. M., Marrs, R. H., Martínez-Ruiz, C. (2014). Shrub-induced understory vegetation changes in reclaimed mine sites. Ecol. Eng. 73, 691–698. doi: 10.1016/j.ecoleng.2014.09.079
Anthelme, F., Cavieres, L. A., Dangles, O. (2014). Facilitation among plants in alpine environments in the face of climate change. Front. Plant Sci. 5. doi: 10.3389/fpls.2014.00387
Bagley, J. E., Kueppers, L. M., Billesbach, D. P., Williams, I. N., Biraud, S. C., Torn, M. S. (2017). The influence of land cover on surface energy partitioning and evaporative fraction regimes in the U.S. Southern Great Plains. J. Geophys. Res.: Atmospheres. 122, 5793–5807. doi: 10.1002/2017JD026740
Benesty, J., Chen, J., Huang, Y., Cohen, I. (2009). “Pearson correlation coefficient,” in Noise Reduction in Speech Processing, vol. 2. Eds. Cohen, I., Huang, Y., Chen, J., Benesty, J. (Berlin, Heidelberg: Springer), 1–4. doi: 10.1007/978-3-642-00296-0_5
Bertness, M. D., Callaway, R. (1994). Positive interactions in communities. Trends Ecol. Evol. 9, 191–193. doi: 10.1016/0169-5347(94)90088-4
Bertness, M. D., Leonard, G. H. (1997). The Role of Positive Interactions in Communities: Lessons from intertidal habitats. Ecology 78, 1976–1989. doi: 10.1890/0012-9658(1997)078[1976:TROPII]2.0.CO;2
Braun, J., Westphal, M., Lortie, C. J. (2021). The shrub Ephedra californica facilitates arthropod communities along a regional desert climatic gradient. Ecosphere 12 (9), 1–16. doi: 10.1002/ecs2.3760
Brooker, R. W., Brewer, M. J., Britton, A. J., Eastwood, A., Ellis, C., Gimona, A., et al. (2018). Tiny niches and translocations: The challenge of identifying suitable recipient sites for small and immobile species. J. Appl. Ecol. 55, 621–630. doi: 10.1111/1365-2664.13008
Brooker, R. W., Maestre, F. T., Callaway, R. M., Lortie, C. L., Cavieres, L. A., Kunstler, G., et al. (2007). Facilitation in plant communities: The past, the present, and the future. J. Ecol. 96 (1), 18–34. doi: 10.1111/j.1365-2745.2007.01295.x
Croitoru, A.-E., Piticar, A., Imbroane, A. M., Burada, D. C. (2013). Spatiotemporal distribution of aridity indices based on temperature and precipitation in the extra-Carpathian regions of Romania. Theor. Appl. Climatol. 112, 597–607. doi: 10.1007/s00704-012-0755-2
Daoud, J. I. (2017). Multicollinearity and regression analysis. J. Phys.: Conf. Ser. 949, 12009. doi: 10.1088/1742-6596/949/1/012009
De Frenne, P., Zellweger, F., Rodríguez-Sánchez, F., Scheffers, B. R., Hylander, K., Luoto, M., et al. (2019). Global buffering of temperatures under forest canopies. Nat. Ecol. Evol. 3, 744–749. doi: 10.1038/s41559-019-0842-1
Dronova, I. (2017). Environmental heterogeneity as a bridge between ecosystem service and visual quality objectives in management, planning and design. Landscape Urban. Plann. 163, 90–106. doi: 10.1016/j.landurbplan.2017.03.005
El-Bana, M. I., Nijs, I., Kockelbergh, F. (2002). Microenvironmental and vegetational heterogeneity induced by phytogenic nebkhas in an arid coastal ecosystem. Plant Soil 247, 283–293. doi: 10.1023/A:1021548711206
Etekcity (2023). Available online at: https://www.amazon.ca/Etekcity-Thermometer-Temperature-Non-contact-58%E2%84%89-716%E2%84%89/dp/B00837ZGRY/ref=sr_1_1_sspa?keywords=infrared+thermometer+gun&qid=1688497101&s=industrial&sprefix=infare+%2Cindustrial%2C80&sr=1-1-spons&sp_csd=d2lkZ2V0TmFtZT1zcF9hdGY&psc=1.
Filazzola, A., Lortie, C. J. (2014). A systematic review and conceptual framework for the mechanistic pathways of nurse plants: A systematic review of nurse-plant mechanisms. Global Ecol. Biogeogr. 23, 1335–1345. doi: 10.1111/geb.12202
Filazzola, A., Westphal, M., Powers, M., Liczner, A. R., (Smith) Woollett, D. A., Johnson, B., et al. (2017). Non-trophic interactions in deserts: Facilitation, interference, and an endangered lizard species. Basic. Appl. Ecol. 20, 51–61. doi: 10.1016/j.baae.2017.01.002
Flores, J., Jurado, E. (2003). Are nurse-protégé interactions more common among plants from arid environments? J. Vegetation. Sci. 14, 911–916. doi: 10.1111/j.1654-1103.2003.tb02225.x
Ghazian, N., Lortie, C. J. (2023). A test of microclimatic facilitation and shrub canopy volume across the Californian aridity gradient. doi: 10.5281/zenodo.8116950
Good, E. J., Ghent, D. J., Bulgin, C. E., Remedios, J. J. (2017). A spatiotemporal analysis of the relationship between near-surface air temperature and satellite land surface temperatures using 17 years of data from the ATSR series. J. Geophys. Res.: Atmospheres. 122, 9185–9210. doi: 10.1002/2017JD026880
Hadley, N. F. (1970). Micrometeorology and energy exchange in two desert arthropods. Ecology 51 (3), 434–444. doi: 10.2307/1935378
Hestrio, Y. F., Soleh, M., Hidayat, A., Afida, H., Gunawan, H., Maryanto, A. (2021). Satellite data receiving antenna system for pleiades neo observation satellite. J. Phys.: Conf. Ser. 1763, 12019. doi: 10.1088/1742-6596/1763/1/012019
Holzapfel, C., Mahall, B. E. (1999). Bidirectional facilitation and interference between shrubs and annuals in the mojave desert. Ecology 80, 1747–1761. doi: 10.1890/0012-9658(1999)080[1747:BFAIBS]2.0.CO;2
Ivey, K. N., Cornwall, M., Crowell, H., Ghazian, N., Nix, E., Owen, M., et al. (2020). Thermal ecology of the federally endangered blunt-nosed leopard lizard (Gambelia sila). Conserv. Physiol. 8, coaa014. doi: 10.1093/conphys/coaa014
Jackson, R. B., Caldwell, M. M. (1992). Shading and the capture of localized soil nutrients: Nutrient contents, carbohydrates, and root uptake kinetics of a perennial tussock grass. Oecologia 91, 457–462. doi: 10.1007/BF00650316
Jankju, M. (2013). Role of nurse shrubs in restoration of an arid rangeland: Effects of microclimate on grass establishment. J. Arid. Environ. 89, 103–109. doi: 10.1016/j.jaridenv.2012.09.008
Jennings, B. (2001). Comparative flowering phenology of plants in the western Mojave Desert. Madroño 48 (3), 162–171.
Jennings, S. B., Brown, N. D., Sheil, D. (1998). Assesing forest canopies and understory illumination: Canopy closure, canopy cover and other measures. Forestry 72 (1) 59–73. doi: 10.1093/forestry/72.1.59
Jennings, W. B., Berry, K. H. (2023). Selection of microhabitats, plants, and plant parts eaten by a threatened tortoise: Observations during a superbloom. Front. Amphibian. Reptile. Sci. 1. doi: 10.3389/famrs.2023.1283255
Lenth, R., Herve, M. (2019). Emmeans, estimated marginal means, aka least-squared means. R Core Team (Version 1.1.2). Indianapolis, IN [Computer software].
Liczner, A. R., Sotomayor, D. A., Filazzola, A., Lortie, C. J. (2016). Germination response of desert annuals to shrub facilitation is species specific but not ecotypic. J. Plant Ecol. 10 (2), 364–374. doi: 10.1093/jpe/rtw030
Lortie, C. J., Braun, J., Westphal, M., Noble, T., Zuliani, M., Nix, E., et al. (2020). Shrub and vegetation cover predict resource selection use by an endangered species of desert lizard. Sci. Rep. 10, 4884. doi: 10.1038/s41598-020-61880-9
Lortie, C. J., Filazzola, A., Westphal, M., Butterfield, H. S. (2022). Foundation plant species provide resilience and microclimatic heterogeneity in drylands. Sci. Rep. 12, 18005. doi: 10.1038/s41598-022-22579-1
Lortie, C. J., Gruber, E., Filazzola, A., Noble, T., Westphal, M. (2018). The Groot Effect: Plant facilitation and desert shrub regrowth following extensive damage. Ecol. Evol. 8, 706–715. doi: 10.1002/ece3.3671
Madrigal, J., García-Rodriguez, J. A., Julian, R., Puerto, A., Fernández-Santos, B. (2008). Exploring the influence of shrubs on herbaceous communities in a Mediterranean climatic context of two spatial scales. Plant Ecol. 195, 225–234. doi: 10.1007/s11258-007-9321-x
Maestre, F. T., Benito, B. M., Berdugo, M., Concostrina-Zubiri, L., Delgado-Baquerizo, M., Eldridge, D. J., et al. (2021). Biogeography of global drylands. New Phytol. 231, 540–558. doi: 10.1111/nph.17395
McIntire, E. J. B., Fajardo, A. (2014). Facilitation as a ubiquitous driver of biodiversity. New Phytol. 201, 403–416. doi: 10.1111/nph.12478
Mengshen (2023). Available online at: https://www.amazon.ca/Mengshen-Digital-Temperature-Humidity-Meter/dp/B01CY29SC2 (accessed April 01, 2023).
Milling, C. R., Rachlow, J. L., Olsoy, P. J., Chappell, M. A., Johnson, T. R., Forbey, J. S., et al. (2018). Habitat structure modifies microclimate: An approach for mapping fine-scale thermal refuge. Methods Ecol. Evol. 9, 1648–1657. doi: 10.1111/2041-210X.13008
Novick, K. A., Ficklin, D. L., Grossiord, C., Konings, A. G., Martínez-Vilalta, J., Sadok, W., et al. (2024). The impacts of rising vapour pressure deficit in natural and managed ecosystems. Plant. Cell Environ. 47, 3561–3589. doi: 10.1111/pce.14846
Owen, E., Lortie, C. J., Zuliani, M. (2024). Native shrub densities predict burrow co-occurrence patterns in Central California Drylands. BMC Ecol. Evol. 24, 68. doi: 10.1186/s12862-024-02259-6
Pfahl, S., Niedermann, N. (2011). Daily covariations in near-surface relative humidity and temperature over the ocean. J. Geophys. Res. 116, D19104. doi: 10.1029/2011JD015792
Richards, S. A., Whittingham, M. J., Stephens, P. A. (2011). Model selection and model averaging in behavioural ecology: The utility of the IT-AIC framework. Behav. Ecol. Sociobiol. 65, 77–89. doi: 10.1007/s00265-010-1035-8
Sawyer, J. O., Keeler-Wolf, T., Evens, J. (2009). A manual of California vegetation (Sacaramento, Californica: California Native Plant Society Press). Available online at: http://books.google.com/books?id=y40lAQAAMAAJ.
Schützenmeister, A., Jensen, U., Piepho, H.-P. (2012). Checking normality and homoscedasticity in the general linear model using diagnostic plots. Commun. Stat - Simulation. Comput. 41, 141–154. doi: 10.1080/03610918.2011.582560
Seiferling, I., Proulx, R., Wirth, C. (2014). Disentangling the environmental-heterogeneity–species-diversity relationship along a gradient of human footprint. Ecology 95, 2084–2095. doi: 10.1890/13-1344.1
Shrode, J. B., Gerking, S. D. (1977). Effects of constant and fluctuating temperatures on reproductive performance of a desert pupfish, cyprinodon n. nevadensis. Physiol. Zool. 50 (1), 1–10. doi: 10.1086/physzool.50.1.30155710
Soliveres, S., Maestre, F. T. (2014). Plant–plant interactions, environmental gradients and plant diversity: A global synthesis of community-level studies. Perspect. Plant Ecol. Evol. Syst. 16, 154–163. doi: 10.1016/j.ppees.2014.04.001
Stein, A., Kreft, H. (2015). Terminology and quantification of environmental heterogeneity in species-richness research: Environmental heterogeneity and species richness. Biol. Rev. 90, 815–836. doi: 10.1111/brv.12135
Stoutjesdijk, P., Barkman, J. J. (2014). Microclimate, vegetation and fauna (Netherlands: KNNV Publishing).
Swank, S. E., Oechel, W. C. (1991). Interactions among the effects of herbivory, competition, and resource limitation on chaparral herbs. Ecology 72, 104–115. doi: 10.2307/1938906
Syphard, A. D., Keeley, J. E., Gough, M., Lazarz, M., Rogan, J. (2022). What makes wildfires destructive in california? Fire 5, 133. doi: 10.3390/fire5050133
Van Meerbeek, K., Jucker, T., Svenning, J. (2021). Unifying the concepts of stability and resilience in ecology. J. Ecol. 109, 3114–3132. doi: 10.1111/1365-2745.13651
Werk, K. S., Ehleringer, J., Forseth, I. N., Cook, C. S. (1983). Photosynthetic characteristics of Sonoran Desert winter annuals. Oecologia 59, 101–105. doi: 10.1007/BF00388081
Xu, Y., Qin, Z., Shen, Y. (2012). Study on the estimation of near-surface air temperature from MODIS data by statistical methods. Int. J. Remote Sens. 33, 7629–7643. doi: 10.1080/01431161.2012.701351
Xue, J., Gui, D., Lei, J., Sun, H., Zeng, F., Mao, D., et al. (2019). Oasis microclimate effects under different weather events in arid or hyper arid regions: A case analysis in southern Taklimakan desert and implication for maintaining oasis sustainability. Theor. Appl. Climatol. 137, 89–101. doi: 10.1007/s00704-018-2567-5
Yang, Y., Chen, J.-G., Schöb, C., Sun, H. (2017). Size-mediated interaction between a cushion species and other non-cushion species at high elevations of the hengduan mountains, SW China. Front. Plant Sci. 08. doi: 10.3389/fpls.2017.00465
Keywords: facilitation, foundation shrub, microclimate, heterogeneity, richness, abundance
Citation: Ghazian N, King R, Zuliani M and Lortie CJ (2024) The microclimatic effects of the native shrub Ephedra californica (Mormon tea) in California drylands. Front. Plant Sci. 15:1396004. doi: 10.3389/fpls.2024.1396004
Received: 05 March 2024; Accepted: 23 September 2024;
Published: 09 October 2024.
Edited by:
Sebastian Leuzinger, Auckland University of Technology, New ZealandReviewed by:
Clayton Kranawetter, University of Missouri, United StatesCopyright © 2024 Ghazian, King, Zuliani and Lortie. This is an open-access article distributed under the terms of the Creative Commons Attribution License (CC BY). The use, distribution or reproduction in other forums is permitted, provided the original author(s) and the copyright owner(s) are credited and that the original publication in this journal is cited, in accordance with accepted academic practice. No use, distribution or reproduction is permitted which does not comply with these terms.
*Correspondence: Nargol Ghazian, bmFyZ29sZzFAbXkueW9ya3UuY2E=
Disclaimer: All claims expressed in this article are solely those of the authors and do not necessarily represent those of their affiliated organizations, or those of the publisher, the editors and the reviewers. Any product that may be evaluated in this article or claim that may be made by its manufacturer is not guaranteed or endorsed by the publisher.
Research integrity at Frontiers
Learn more about the work of our research integrity team to safeguard the quality of each article we publish.