- 1School of Plant and Environmental Sciences, Virginia Tech, Blacksburg, VA, United States
- 2Department of Plant Pathology, Entomology and Microbiology, Iowa State University, Ames, IA, United States
- 3Soybean Genomics and Improvement Laboratory, Agricultural Research Service, Department of Agriculture, Beltsville, MD, United States
- 4Department of Plant Pathology, The Ohio State University, Wooster, OH, United States
For soybean, novel single dominant Resistance to Phytophthora sojae (Rps) genes are sought to manage Phytophthora root and stem rot. In this study, resistance to P. sojae was mapped individually in four recombinant inbred line (RIL) populations derived from crosses of the susceptible cultivar Williams with PI 407985, PI 408029, PI 408097, and PI424477 previously identified as putative novel sources of disease resistance. Each population was screened for resistance with five to seven isolates of P. sojae separately over multiple F7–F10 generations. Additionally, three of the populations were screened with inoculum from the combination of three P. sojae isolates (PPR), which comprised virulence to 14 Rps genes. Over 2,300 single-nucleotide polymorphism markers were used to construct genetic maps in each population to identify chromosomal regions associated with resistance to P. sojae. Resistance segregated as one or two genes to the individual isolates and one gene toward PPR in each population and mapped to chromosomes 3, 13, or 18 in one or more of the four RIL populations. Resistance to five isolates mapped to the same chromosome 3 region are as follows: OH7 (PI 424477 and PI408029), OH12168, OH7/8, PPR (PI 407985), and 1.S.1.1 (PI408029). The resistance regions on chromosome 13 also overlapped for OH1, OH25, OH-MIA (PI424477), PPR (PI 424477, PI 407985, and PI 408097), PPR and OH0217 (PI 408097), and OH4 (PI 408029), but were distinct for each population suggesting multiple genes confer resistance. Two regions were identified on chromosome 18 but all appear to map to known loci; notably, resistance to the combined inoculum (PPR) did not map at this locus. However, there are putative new alleles in three of four populations, three on chromosome 3 and two on chromosome 13 based on mapping location but also known virulence in the isolate used. This characterization of all the Rps genes segregating in these populations to these isolates will be informative for breeding, but the combined inoculum was able to map a novel loci. Furthermore, within each of these P. sojae isolates, there was virulence to more than the described Rps genes, and the effectiveness of the novel genes requires testing in larger populations.
1 Introduction
Soybean [Glycine max (L.) Merr.] is a major oil and protein crop that is grown worldwide. Soybean seed is composed mainly of 40% protein and 20% oil (Clemente and Cahoon, 2009). In the United States, the crop was planted on 87.5 million acres during the 2022 growing season and was worth $59.2 billion dollars in the 2021/2022 marketing year (http://www.soystats.com). Phytophthora sojae (Kaufmann and Gerdemann) is an economically important soybean pathogen in the United States and worldwide. Between 2015 and 2019, in 29 US states and Ontario, Canada, the estimated yield loss from P. sojae ranged from 25 to 29 million bushels annually (Bradley et al., 2021). The severity of loss depends on a wide variety of factors including soil type, compaction, and rainfall (Schmitthenner, 2000). P. sojae is a diverse organism with over 200 pathotypes (Dorrance, 2018a; Matthiesen et al., 2021; Hebb et al., 2022; McCoy et al., 2023). This pathogen causes root and stem rot in soybean plants, which can lead to stand and yield reduction in susceptible cultivars. Symptoms of Phytophthora root and stem rot (PRSR) early in the growing season include seed and seedling disease and pre- and post-emergence damping off. Later in the growing season, root rot and, in highly susceptible cultivars, stem lesions may develop if environmental and field conditions are favorable with P. sojae inoculum present (Schmitthenner, 1985; Dorrance et al., 2003a; Grau et al., 2004). Practices, such as crop rotation and tillage, are not as useful with this pathogen because oospores are able to persist in the soil and plant debris for years (Schmitthenner, 1985).
The two main types of resistance in soybean cultivars to P. sojae are single dominant resistance genes (Rps genes) and partial resistance. Partial resistance is quantitatively inherited, and several genes contribute to the level of resistance, whereas Rps genes are qualitatively inherited and are pathotype (formally race) specific (Schmitthenner, 1985; Grau et al., 2004; Dorrance, 2018b). The first Rps gene was identified in the 1950s (Bernard et al., 1957), and over 40 Rps genes and alleles have been mapped to date on 9 of the 20 soybean chromosomes (Dorrance, 2018a; Lin et al., 2022). In a genome-wide association study (GWAS), Van et al. (2020) identified 75 novel Rps loci for P. sojae on all chromosomes except 3 and 13 using 16 soybean panels, which included over 1,800 soybean accessions. However, only genes from three loci (Rps1, Rps3, and Rps6) have been used commercially, and these are Rps1a, Rps1b, Rps1c, Rps1k, Rps3a, and Rps6 (Grau et al., 2004; Slaminko et al., 2010). The use of Rps genes has allowed for shifts in pathotype diversity of P. sojae toward those that are adapted to specific Rps genes. In the north central region of the United States, complex pathotypes of P. sojae are now pervasive (Dorrance et al., 2003b, 2016; Yan and Nelson, 2019; Matthiesen et al., 2021; Hebb et al., 2022; McCoy et al., 2022). In a pathotype diversity study of P. sojae in 11 states in the United States, over 200 unique pathotypes were observed many of which could cause disease on soybean with any of the commercially available Rps genes (Dorrance et al., 2016). Depending on the soybean-growing region, certain Rps genes were not as effective as in the past (Matthiesen et al., 2021; McCoy et al., 2022), and this highlights the need for continuing to identify and combine effective Rps genes during the development of new soybean cultivars or ensuring cultivars also have high partial resistance. Dorrance and Schmitthenner (2000) identified multiple sources of potentially novel Rps and quantitative resistance to PRSR in soybean accessions. The majority of the lines that showed resistance were from South Korea and included PI 407985, PI 408097, PI 408029, and PI 424477. These same PIs were also resistant toward P. sojae in a combined inoculum approach (Matthiesen et al., 2016) making them a high priority source for new Rps genes. South Korean accessions are genetically distant from US genotypes (Burnham et al., 2002), which makes them good candidates to expand the gene pool available to US breeding programs.
Of the four PIs, Gordon et al. (2007a) evaluated PI 408097 in F2:3 and F2:4 recombinant inbred line (RIL) populations and proposed that this PI may have a combination of Rps genes of Rps1c plus one of the following Rps2, 3a, 3b, or 4. The objective of this study was to identify and map the potentially novel Rps loci in each of the RIL populations derived from crosses of Williams with PI 407985, PI 408029, PI 408097, and PI424477 using multiple generations. In this study, we used data from inoculations with single isolates of P. sojae and also three isolates of P. sojae that were combined before inoculation. This study allowed us to compare the results of these two different inoculation methods, construct high-density genetic maps, and determine chromosomal locations associated with resistance to PRSR using the four RIL populations. We utilized both single marker and quantitative approaches in mapping these loci. As qualitative and quantitative trait loci both have the same abbreviation (QTL), we have used Mendelian mapping as the term to indicate qualitative trait mapping. The findings from this study will expand the currently available Rps genes and genetics available for breeders to develop resistant cultivars.
2 Materials and methods
2.1 Plant material
Genetic material for this study included RIL populations PI 407985 × Williams, PI 408029 × Williams, PI 408097 × Williams, and PI 424477 × Williams, with 192, 188, 312, and 163 RILs, respectively. These RIL populations were created at Virginia Tech, Kentland Farm in Blacksburg, VA. The Plant Introduction (PI) lines: PI 407985, PI 408029, PI 408097, and PI 424477 were chosen based on results of previous P. sojae screens for resistance (Dorrance and Schmitthenner, 2000; Matthiesen et al., 2016). Williams is the differential with no Rps genes and is susceptible to all isolates of P. sojae (Dorrance et al., 2004). These four PI lines are from the US Soybean Germplasm Collection, and all are maturity group IV.
2.2 Rolled towel assay for phenotyping
Since PI 407985, PI 408029, PI 408097, and PI 424477 each contain several Rps genes, isolates of P. sojae were selected to potentially limit the expression to one or two Rps genes to enhance the possibility of fine mapping but also to identify one locus that would confer resistance to all the isolates. For each population, Table 1 summarizes the number of RILs, the isolates used to inoculate, and the number of generations screened for disease reaction in a rolled towel assay (Dorrance et al., 2008).
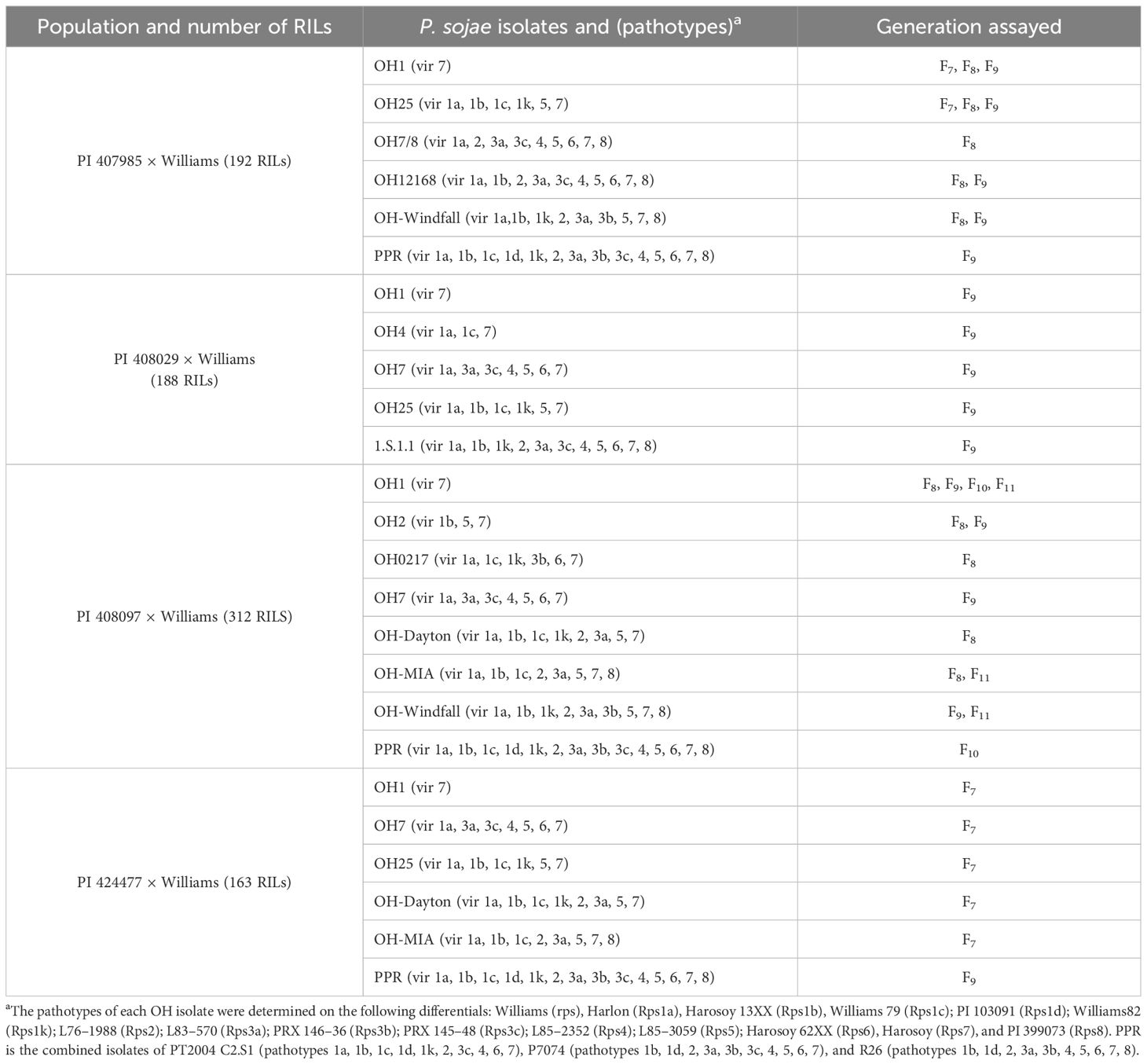
Table 1 The Phytophthora sojae isolates and their associated pathotypes to determine segregation ratios for the three RIL populations, PI 407985 × Williams, PI 408097 × Williams, and PI 424477 × Williams, the number of RILs in each population, and the generation(s) following hypocotyl inoculation in rolled towel assays.
2.2.1 Screening with single P. sojae isolates
At Ohio State University (OSU), single isolates of P. sojae were used to map as many of the loci present in these PI lines as possible. In Ohio, P. sojae isolate OH1 was selected as it has virulence to only one known Rps gene, Rps7, and could identify the RILs that were lacking in Rps genes. This served as a check for subsequent inoculations to ensure the same lines were susceptible to all remaining isolates. Initially, isolates were transferred to Petri dishes with lima bean (Phaseolus lunatus L.) agar media (LBA) (lima beans 50 g/l, agar 12 g/l) and placed in a 25°C incubator. Isolates (virulence pathotype) OH1 (vir 7), OH2 (vir 1b, 7), OH4 (vir 1a, 1c, 7), OH7 (vir 1a, 3a, 3c, 4, 5, 6, 7), OH7/8 (vir 1a, 2, 3a, 3c, 4, 5, 6, 7, 8), OH12168 (vir 1a, 1b, 2, 3a, 3c, 4, 5, 6, 7, 8), OH0217 (vir 1a, 1c, 1k, 3b, 6, 7), OH25 (vir 1a, 1b, 1c, 1k, 7), OH-Dayton (vir 1a, 1b, 1c, 1k, 2, 3a, 5, 7), OH-MIA (vir 1a, 1b, 1c, 2, 3a, 5, 7, 8), 1.S.1.1 (vir 1a, 1b, 1k, 2, 3a, 3c, 4, 5, 6, 7, 8), and OH-Windfall (vir 1a, 1b, 1k, 2, 3a, 3b, 5, 7, 8) were used to inoculate the hypocotyls of 7-day-old seedlings in a rolled towel assay (10 to 15 seedlings/RIL) (Dorrance et al., 2008). After 7 days, germination towels were unrolled, and seed coats, twisted seedlings, and non-germinated seeds were removed. Petri dishes were fully colonized with 7-to-8-day old individual P. sojae. The colony was cut and placed in a syringe and forced through an 18-gauge hypodermic syringe to make a slurry. A small incision was made approximately 1 cm below the cotyledon on the surface of the hypocotyl on each seedling. The mycelial slurry (LBA and P. sojae mycelia) covered the wound, and the rolled towels were reassembled and incubated at 25°C and 50% HR in a growth chamber. Differential checks each having one known Rps gene or none included in each assay were Harlon (Rps1a), Harosoy 13XX (Rps1b), Williams 79 (Rps1c); PI 103091 (Rps1d); Williams82 (Rps1k); L76–1988 (Rps2); L83–570 (Rps3a); PRX 146–36 (Rps3b); PRX 145–48 (Rps3c); L85–2352 (Rps4); L85–3059 (Rps5); and Harosoy 62XX (Rps6), Harosoy (Rps7), PI 399073 (Rps8), and Williams (rps). Seven days after inoculation, seedlings were scored for presence of lesions. First, the response of the differential checks was examined to determine if the isolate had the expected virulence to the each known Rps gene. If the response was as expected, the RILs in the population were scored. The trait is a single dominant gene, and as such, a categorical score for the RILs was used based on the number of seedlings that developed expanding lesions from the total inoculated. For each isolate, where resistant (R), segregating (H), or susceptible (S), for 0% up to 20%, 21% to 79%, or 80% to 100%, respectively, scores were given. Due to the nature of the assay, inoculating the hypocotyl with a hypodermic, there are successful and unsuccessful infections, which is why the categories are broad. Due to the limited seed, the phenotype assay was done only once for isolates where a clear segregation pattern was observed and the differentials’ response as expected. Comparisons were made across isolates to ensure those RILs identified as susceptible to OH1 were consistent across all isolates. Furthermore, only data where differentials responded as expected were used.
2.2.2 Screening with a combination of three P. sojae isolates
At Iowa State University (ISU), isolates with all known virulence to Rps loci were combined prior to inoculation to identify the unique loci as previously described (Matthiesen et al., 2016). Briefly, three P. sojae isolates, PT2004 C2.S1 (pathotype 1a, 1b, 1c, 1d, 1k, 2, 3c, 4, 6, 7), P7074 (pathotype 1b, 1d, 2, 3a, 3b, 3c, 4, 5, 6, 7), and R26 (pathotype 1b, 1d, 2, 3a, 3b, 4, 5, 6, 7, 8), were grown on separate plates containing dilute DV8 media (40 ml of V-8 juice, 0.6 g of CaCO3, 0.2 g of Bacto yeast extract, 1 g of sucrose, 0.01 g of cholesterol, 20 g of Bacto agar, 1 L of distilled water) amended with neomycin sulfate (50 µg/ml) and chloramphenicol (10 µg/ml) for 7 to 8 days at 25°C in the dark. Each isolate slurry was prepared by combining each isolate grown separately in a 1:1:1 mixture used for the hypocotyl inoculation described above for each soybean line. The combined three isolates of PT2004 C2.S1, P7074, and R26 will be referred to as PPR, hereafter. Rolled towels with inoculated seedlings were kept at 25°C with a 16-h photoperiod for 7 days before percentage of killed seedlings for each RIL was scored. The pathogenicity of the 1:1:1 mixture was checked on the following 14 differentials: L88–8470 (Rps1a), L77–1863 (Rps1b), Williams 79 (Rps1c), L93–3312 (Rps1d), Williams 82 (Rps1k), L82–1449 (Rps2), L83–570 (Rps3a), L91–8347 (Rps3b), L92–7857 (Rps3c), L85–2352 (Rps4), L85–3059 (Rps5), L89–1581 (Rps6), L93–3258 (Rps7), PI 399073 (Rps8), and Sloan (rps). RIL responses were scored susceptible if at least 70% of seedlings died on all differentials checks. The experiment was replicated two times. The inoculated RILs were scored homozygous resistant if <30% and susceptible if more than 70% of the RILs developed symptoms. All others were classified as heterozygous.
2.3 Molecular marker assay
For single-nucleotide polymorphism (SNP) and simple sequence repeat (SSR) marker genotyping, unfolded first or second trifoliate leaves of greenhouse-grown plants were collected for DNA extraction. DNA from parental lines and at least 10 bulked plants from each individual RIL was isolated from lyophilized tissues using the CTAB method as described by Saghai-Maroof et al. (1984) with minor modifications. DNA concentration was measured with a Qubit 3.0 Fluorometer (Life Technologies, Carlsbad, CA).
To identify chromosomal regions associated with resistance to P. sojae, the four RIL populations and parents were genotyped using the Illumina Infinium BARCSoySNP6K BeadChip (Song et al., 2020) at USDA-ARS, Soybean Genomics and Improvement Lab, Beltsville, MD. The marker data sets were processed using GenomeStudio software (version 3.2.23). SNP markers that were monomorphic between parents of each RIL population and those that had more than 20% missing data were not used for linkage map construction. SSR markers were added to two critical P. sojae regions on chromosomes 3 and 18 in PI 407985 × Williams and PI 408097 × Williams. SSR markers were amplified by PCR with dye-labeled forward primers (Song et al., 2010) and analyzed by capillary electrophoresis using an Applied Biosystems 3130xl Genetic Analyzer (Foster City, CA).
2.4 Map construction, Mendelian and quantitative trait locus analysis
The genetic maps were constructed using JoinMap 4.0 (van Ooijen, 2006) based on an LOD threshold of 4.0 and a maximum recombination frequency of 50% for the original grouping. Marker order and their positions within each linkage group were determined using the maximum likelihood algorithm and Kosambi mapping function; those unassigned to any linkage group were excluded. Mendelian inheritance of the resistant trait was mapped with each isolate individually due to the number of different RILs used in each inoculation and generation.
MapQTL 5 software (van Ooijen, 2004) was used for the identification of each quantitative trait locus for reaction to each of the P. sojae isolates assayed in this study. Scores of individual RILs were used on the scale of 1 = homozygous resistant, 2 = heterozygous, and 3 = homozygous susceptible. The QTL analysis was performed in two stages: interval mapping (IM) to reveal critical chromosomal regions followed by more detailed QTL mapping provided by the enhanced power of composite interval mapping (CIM) with the walking speed set to 1 cM.
To identify levels of LOD significance thresholds on a genome-wide basis, 1,000 iteration permutation tests were conducted. Calculated genome-wide LOD thresholds were used as a base line in significant QTL justification. Surprisingly, some minor QTLs were identified, which is unexpected for this type of resistance. Our results focused on only the major QTL and those loci that could be mapped with both Mendelian and QTL mapping.
3 Results
3.1 Phenotypic data of RILs
Based on Chi-square analysis of the phenotypic data following inoculation, resistance toward one or more of the isolates alone or to the combined inoculum, PPR, in each of the RIL populations segregated as one to two Rps genes (Tables 2, 3). P. sojae isolate OH1 has virulence to one known Rps gene, Rps7, and resistance to OH1 segregated as two genes in each of these populations. This indicates that the total number of genes we expected to map in each of the populations was two. For each of these advanced RIL populations, segregation to the remaining isolates did not follow normal phenotypes for single or two-gene inheritance, with a maintenance of heterozygotes across the generations. This has been noted in other studies; thus, the chi-square analysis was based on the combination of RILs classified as heterozygous with either the homozygous-resistant or homozygous-susceptible RILs. Resistance was conferred by one Rps gene toward P. sojae OH12168, OH7/8, and the combined inoculum (PPR) in the PI 407985 × Williams based on segregation ratios. In addition, in this population, resistance toward P. sojae OH25 and OH-Windfall was conferred by one or two Rps genes. Resistance in the PI 408029 × Williams toward P. sojae isolates OH7 and 1.S.1.1 was conferred by one Rps gene, while for OH4 and OH25, resistance was conferred by either one or two Rps genes. In the PI 408097 × Williams population, resistance toward P. sojae isolates OH2, OH-Dayton, and PPR was controlled by two Rps genes, while resistance toward P. sojae isolates OH-MIA, OH0217, and OH-Windfall may be conferred by one or two Rps genes. In the PI 424477 × Williams population, resistance toward P. sojae isolates OH7, OH-MIA, OH25, and PPR were controlled by a single Rps gene.
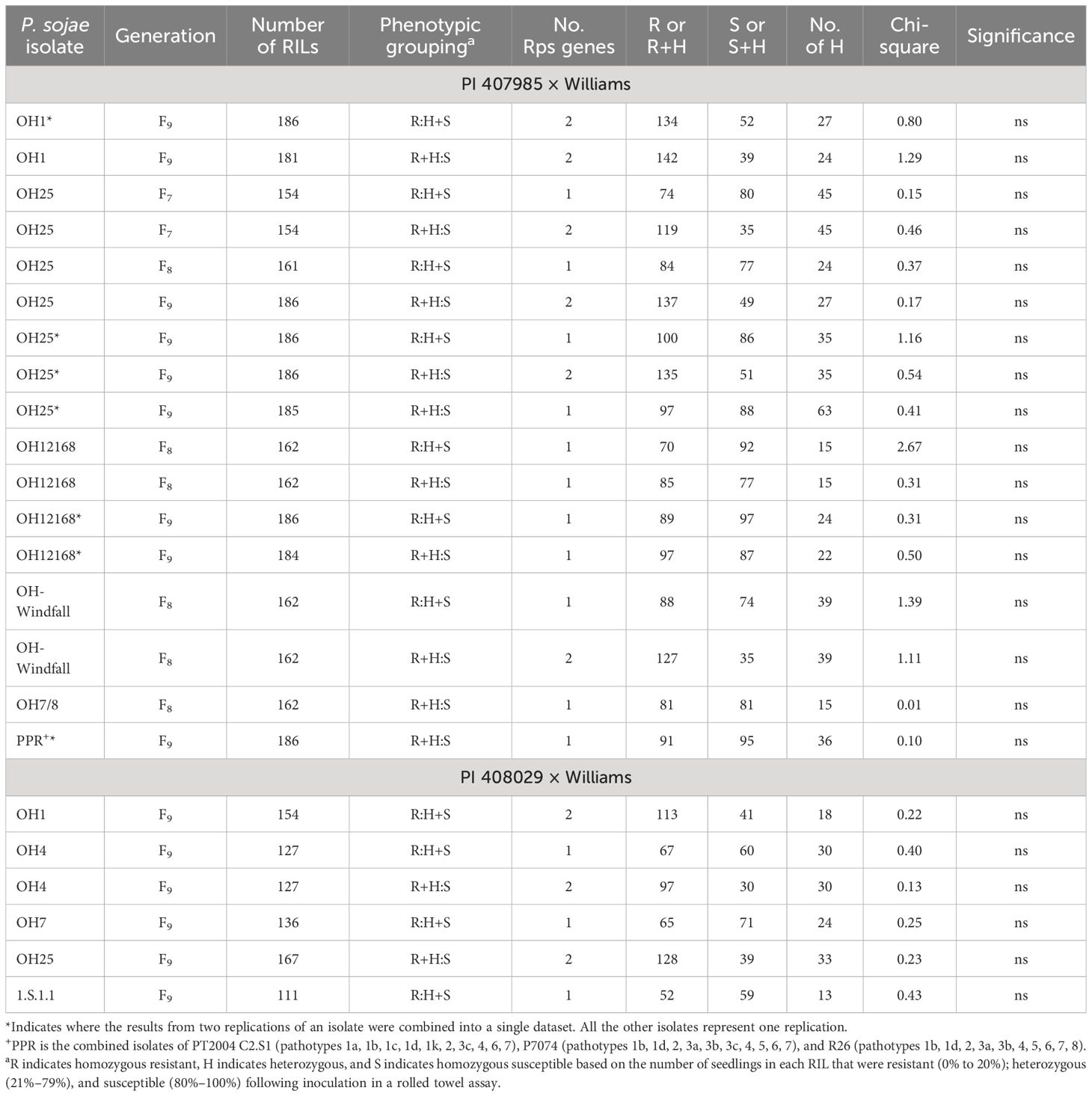
Table 2 Chi-square table for PI 407985 × Williams RIL and PI 408029 × Williams populations across generations following inoculation with five isolates of Phytophthora sojae separately and inoculation with inoculum of three isolates combined (PPR).
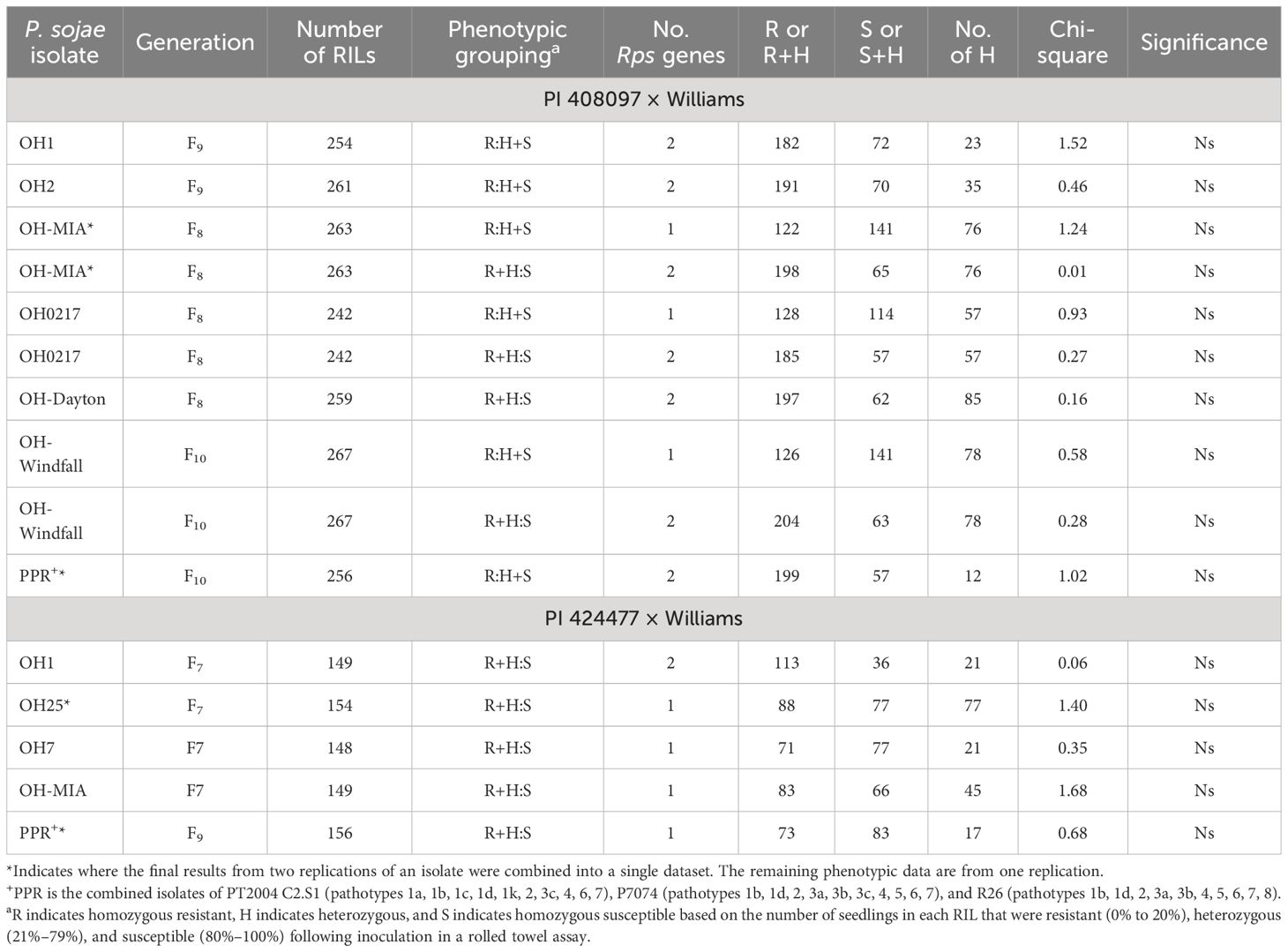
Table 3 Chi-square table for PI 408097 × Williams and for PI 424477 × Williams RIL populations across generations following inoculation with six and four isolates of Phytophthora sojae and inoculation with inoculum of three isolates combined (PPR).
3.2 Mendelian inheritance (qualitative trait loci) and quantitative trait loci for single Rps gene mapping
Prior to mapping the resistance phenotype, the accuracy of the constructed genetic map for each of the four RIL populations was verified against the soybean genome sequence assembly version Wm82.a2 (http://soybase.org, Brown et al., 2021). The total number of mapped markers was 2,316 for the population PI 407985 × Williams, 2,670 for PI 408029 × Williams, 2,644 for PI 408097 × Williams, and 2,477 for PI 424477 × Williams. The high-density genetic maps were subsequently used to identify chromosomal regions associated with resistance to P. sojae isolates in these populations.
For the PI 407985 × Williams population, 192 RILs were phenotyped over three generations, F7, F8, and F9 (Table 4; Supplementary Table 1). Resistance toward OH7/8, OH12168, and combined inoculum (PPR) all segregated as a single gene and mapped to the same region on chromosome 3, as well as resistance toward the remaining isolates OH1 and OH-Windfall, which were confirmed via QTL mapping (Figure 1A, Supplementary Figure 1; Table 4). The SNP marker, ss715585782, was at the maximum LOD for OH1, OH7/8, OH12168, and PPR consistently across the F8 and F9 generations (Supplementary Figure 1A) indicating that the same Rps gene may be conferring resistance and could be a new allele based on the virulence pathotypes. The maximum LOD for OH-Windfall mapped ~3.6 cM below ss715585782 near the marker ss715586806 (Table 4). This region covers 1.31 Mb and is enriched for genes encoding leucine-rich repeat containing proteins as well as serine threonine kinase based on the Wm82.a2 sequence assembly (www.soybase.org, Brown et al., 2021) (Supplementary Table 2).
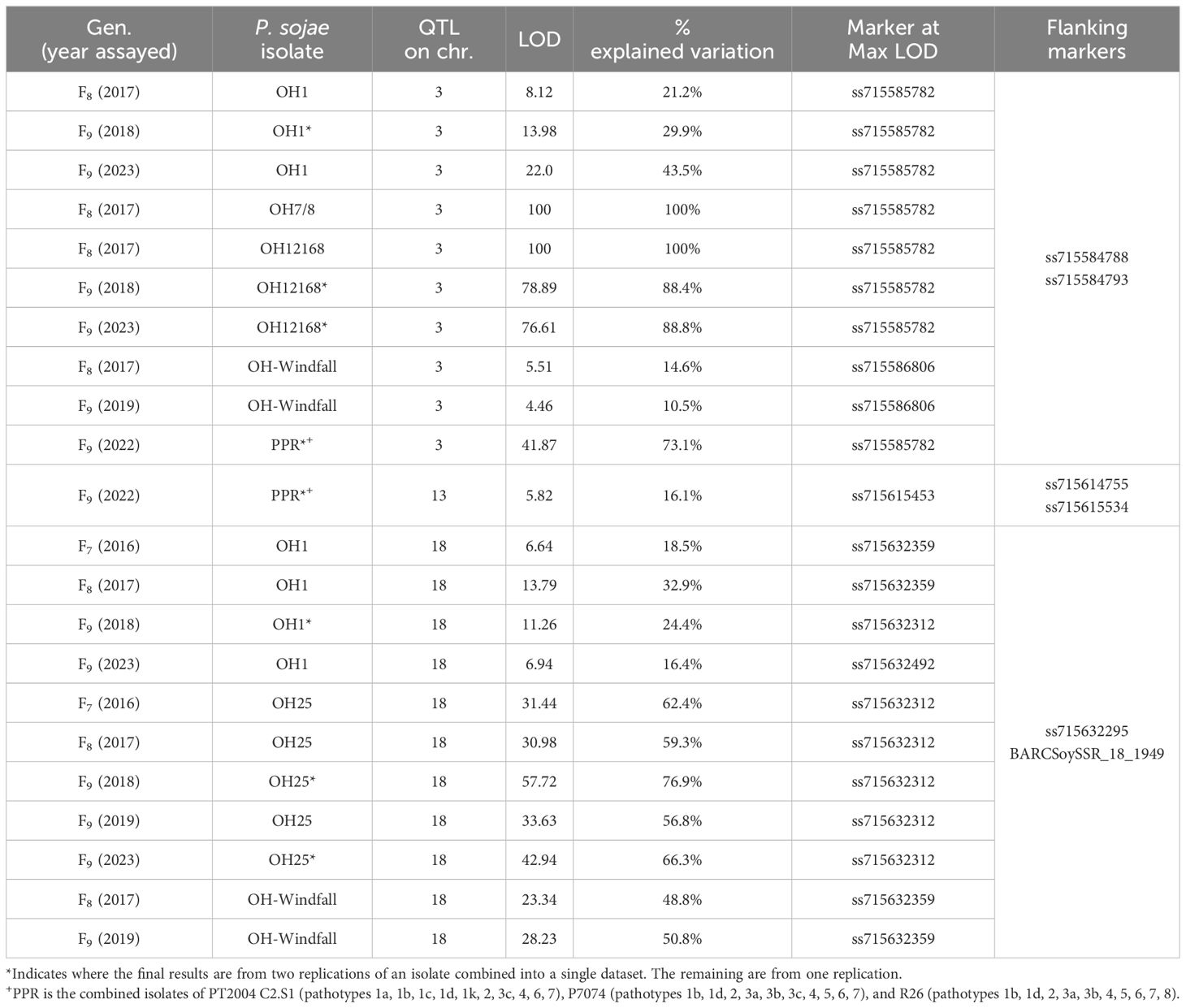
Table 4 Resistance in PI 407985 × Williams toward Phytophthora sojae in F7, F8, and F9 of the RIL population based on QTL mapping.
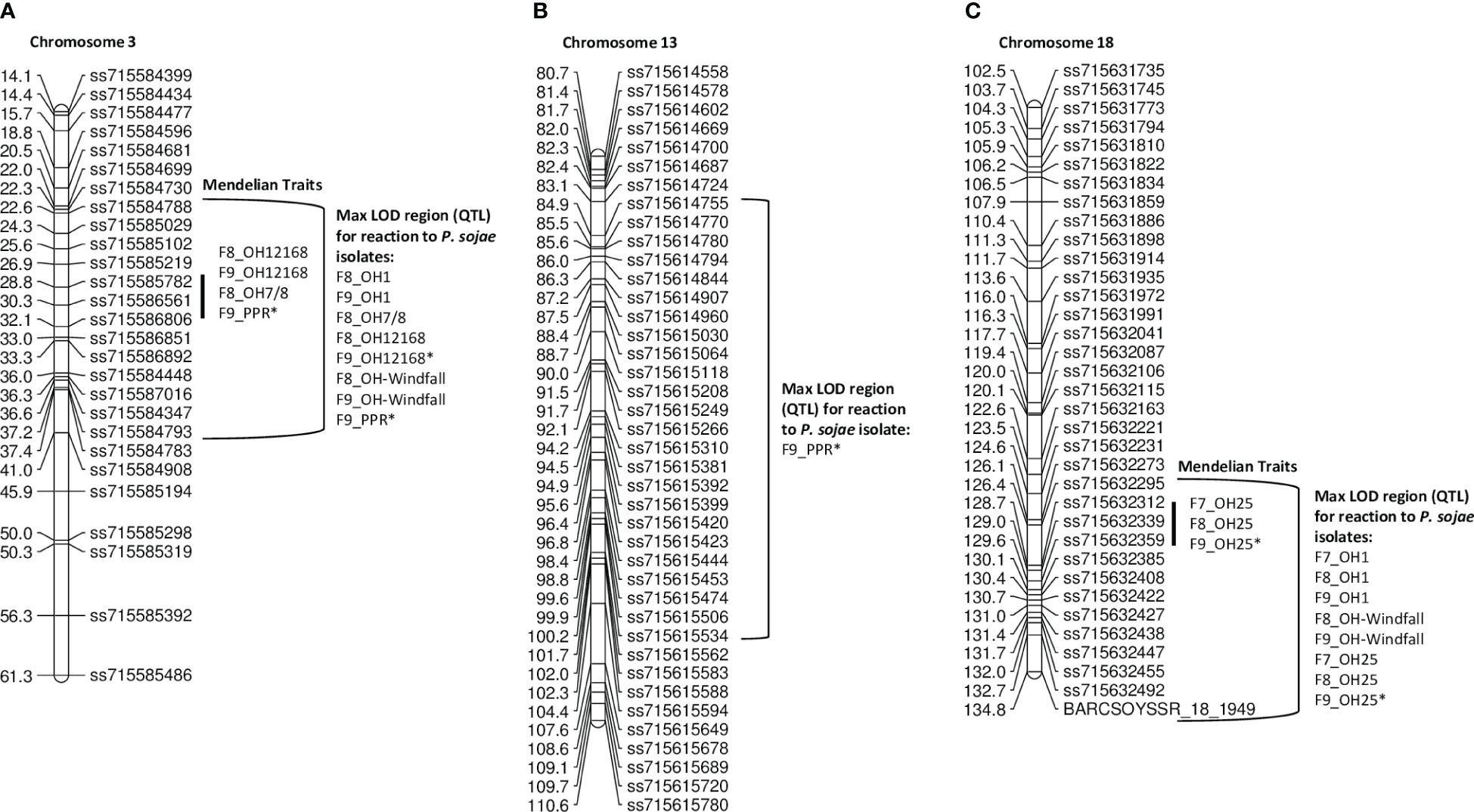
Figure 1 (A) Chromosome 3 regional map for Mendelian and QTL mapping in PI 407985 × Williams (positions of Rps loci in the inner bracket denoted by a black line). The max LOD region for reaction to isolates OH1, OH7/8, OH12168, OH-Windfall, and PPR are denoted by the bracketed region to the right side of the map. (B) Chromosome 13 regional map for QTL mapping in the PI 407985 × Williams. The bracketed region is the max LOD region for reaction to the combined inoculum, PPR. (C) Chromosome 18 regional map for Mendelian and QTL mapping in PI 407985 × Williams (positions of Rps loci in the inner bracket denoted by a black line). The max LOD region for the reaction to isolates OH1, OH25, and OH-Windfall are denoted by the bracketed region to the right side of the map. Isolates designated with an asterisk (*) indicate that disease screening results from two replications were combined into a single dataset; the remaining are from one replication. All isolates were mapped separately and then placed on the map.
Interestingly, resistance toward the combined inoculum, PPR, segregated as a single gene in the F9 generation, but a minor QTL on chromosome 13 explaining 16.1% of the phenotypic variation with an LOD of 5.82 was identified (Table 4). This region was flanked by SNP markers, ss715614755 and ss715615534 (Figure 1B). There are 28 genes that encode for leucine-rich repeats in this large region (5.4 Mb) based on the Wm82.a2 sequence (soybase.org).
Resistance to P. sojae isolates OH1, OH25, and OH-Windfall mapped on chromosome 18. The flanking markers were the same across the F7, F8, and F9 generations (Table 4, Figure 1C; Supplementary Figure 1B). Based on Mendelian mapping, resistance toward OH-Windfall mapped 9 cM below the end marker BARCSOYSSR_18_1949 on chromosome 18, which was also a flanking marker based on QTL mapping. However, the marker at the peak LOD for this isolate was ss715632359, which maps near the other isolates (Figure 1C, bottom of Table 4). Within this physical region (271,334 bp), there are four annotated disease resistance genes (Glyma.18g282100, Glyma.18g282600, Glyma.18g283200, and Glyma.18g284100) based on Glyma82.a2.v1 (soybase.org). It is important to note that the RILs did not respond consistently to these isolates for resistance or susceptibility indicating the possibility that this region may have several Rps genes to P. sojae that confer resistance to these isolates. Additionally, any of the known Rps genes (Rps4, 5, and 6) on chromosome 18 would confer resistance to OH25. However, OH-Windfall has virulence to Rps5, thus Rps4 and Rps6 are likely candidates.
In the PI 408029 × Williams population, 188 RILs were screened in the F9 generation with five single isolates (Table 5). Resistance toward OH1 mapped to chromosomes 3 and 13 (Figures 2A, B). The marker at the maximum LOD score with QTL mapping was ss715585348 for the three isolates (OH1, OH7, 1.S.1.1) potentially indicating the same gene confers resistance (Table 5; Supplementary Figure 2A). This region from ss715585348 to ss715586444 covers less than 1 Mb at 805,038 bp and partially overlaps the Rps gene region identified in the PI 407985 × Williams RIL population, which contains sequences of many NBS-LRR genes. Based on the virulence to known Rps genes in these isolates (Rps1a, Rps1b, and Rps1k), there is the possibility that Rps1c could be in PI 408029 and contributing to resistance.
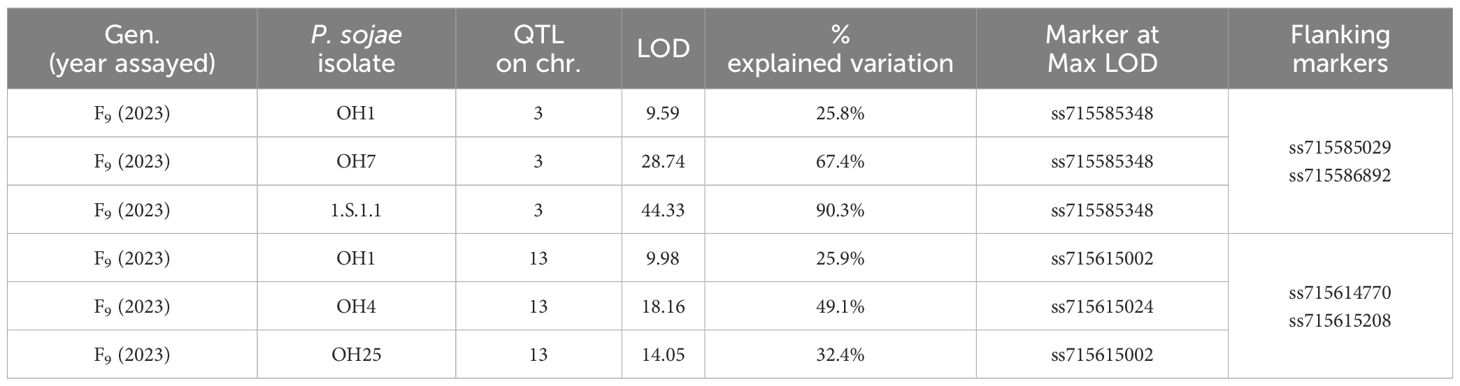
Table 5 Resistance in PI 408029 × Williams toward Phytophthora sojae in the F9 of the RIL population based on QTL mapping.
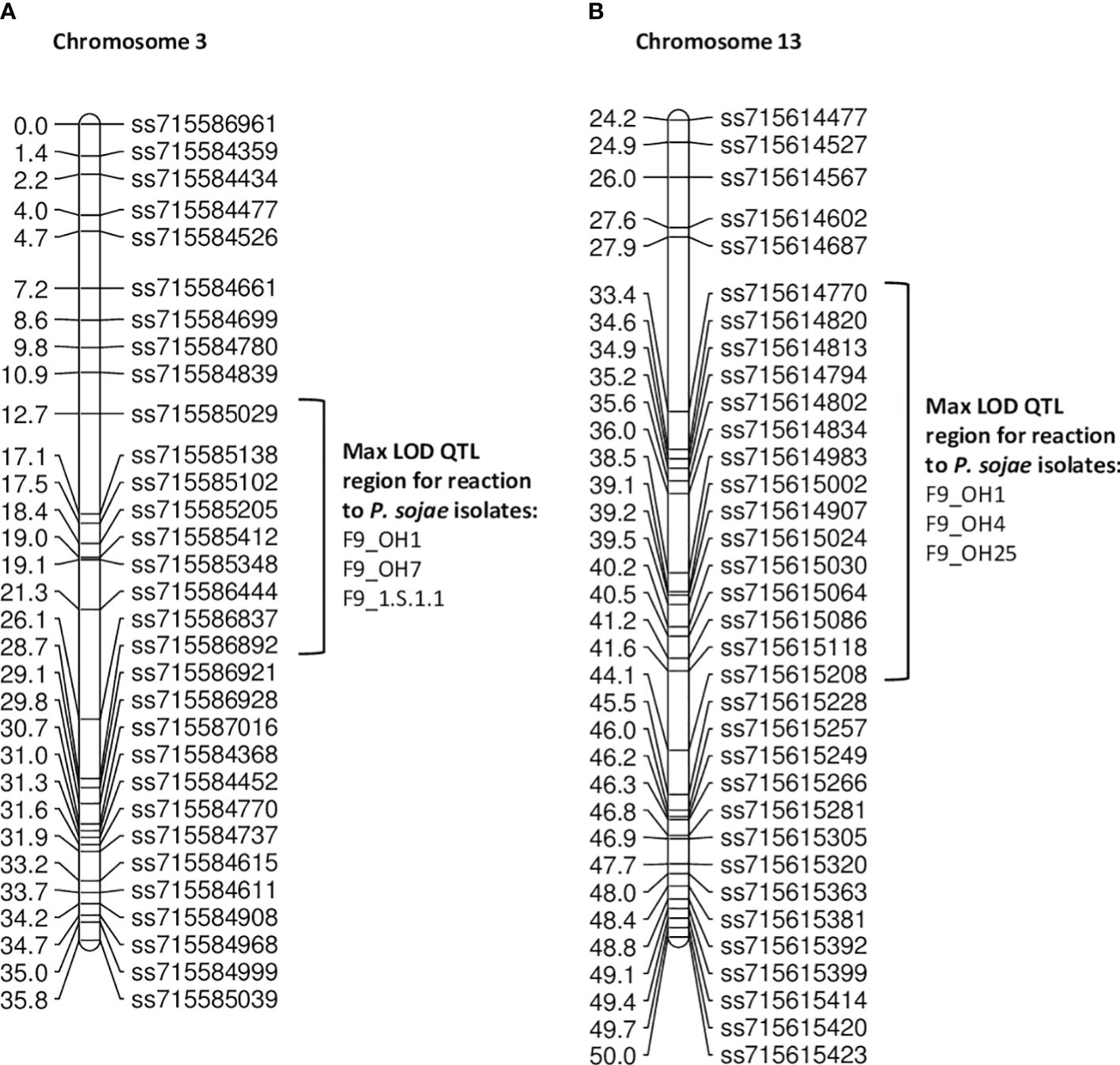
Figure 2 (A) Chromosome 3 regional map for QTL mapping in PI 408029 × Williams. The max LOD region for reaction to isolates OH1, OH7, and 1.S.1.1 are denoted by the bracketed region to the right side of the map. (B) Chromosome 13 regional map for QTL mapping in PI 408029 × Williams. The max LOD region for reaction to isolates OH1, OH4, and OH25 are denoted by the bracketed region to the right side of the map.
Resistance towards P. sojae isolate OH4 mapped below that of OH1 and OH25 on chromosome 13 with SNP marker, ss715615024, at the maximum LOD (Figure 2B). The similarity of the three LOD curves for this chromosomal region emphasizes the consistency of the map location over all three isolates (Supplementary Figure 2B). Based on the Wm82.a2 assembled sequence, this region encompasses ~0.8 Mb, and there are 12 genes with annotations of leucine-rich repeat and defense related. These three isolates, OH1, OH4, and OH25, do not have any virulence to known Rps genes that map to this region, but this does not necessarily preclude that there are no additional alleles of these genes (Rps3a, 3b, 3c, and 8) present in this PI.
The largest of the four RIL populations, PI 408097 × Williams 312 RILs were screened over four generations (F8, F9, F10, F11) with seven single isolates and combined inoculum (PPR). For this population, QTL mapping was primarily used as there were two genes segregating for resistance toward P. sojae isolates OH1, OH2, OH-Dayton, and PPR, while OH-MIA, OH0217, and OH-Windfall were difficult to discern between one and two Rps genes. As such, resistance was mapped to three regions on chromosomes 3, 13, and 18 (Table 6, Figures 3A–C; Supplementary Figure 3A, B) with QTL mapping only.
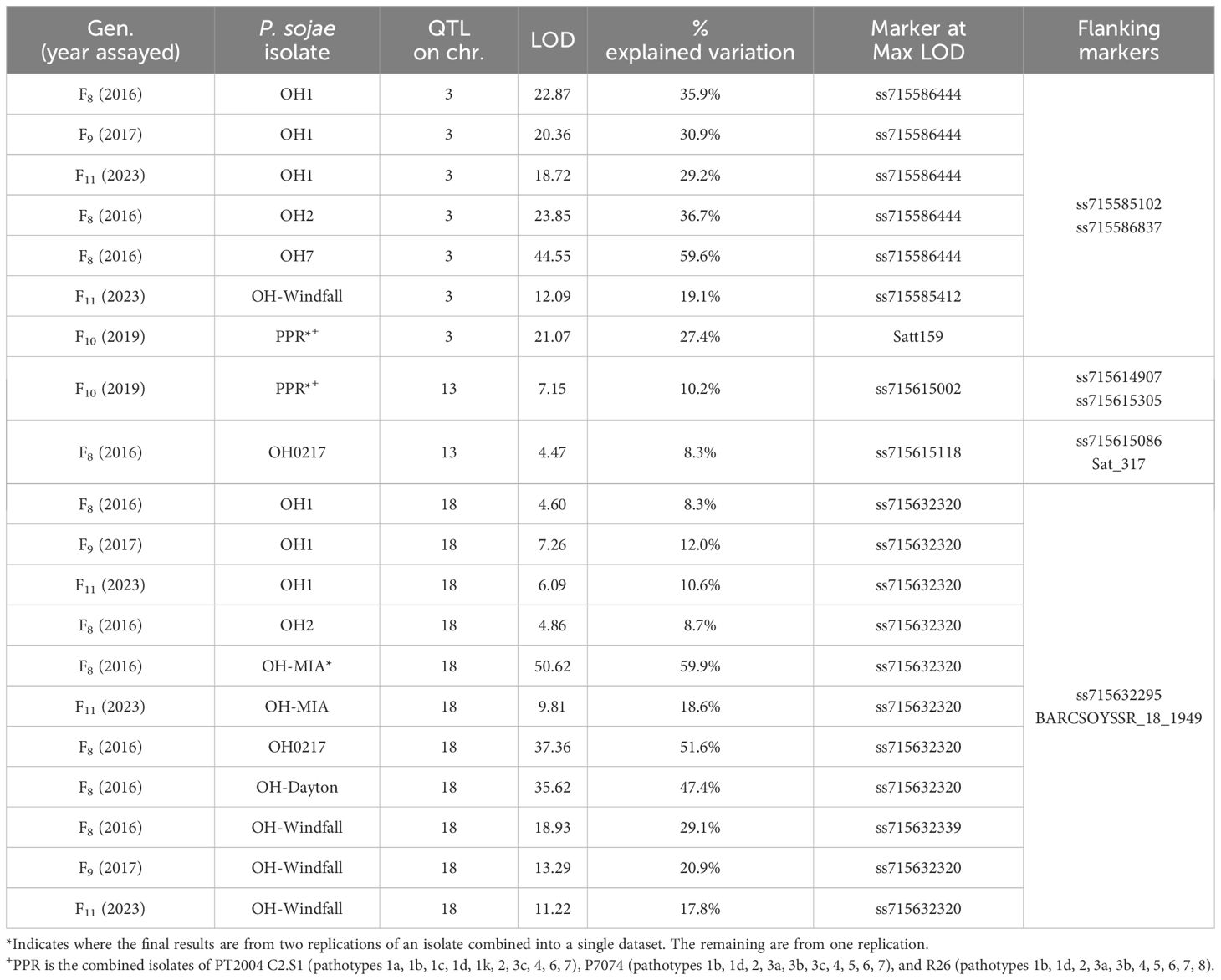
Table 6 Resistance in PI 408097 × Williams toward Phytophthora sojae in F8, F9, F10, and F11 of the RIL population based on QTL mapping.
The max LOD QTL region on chromosome 3 was flanked by SNP markers ss715585102 and ss715586837 for OH1, OH2, OH7, OH-Windfall, and PPR (Figure 3A, Table 6). However, the peak SNP marker differed for OH-Windfall and PPR from that of the others. In terms of physical distance, this interval covers 2.2 Mb. There are six NBS-LRR genes within a 240-kb region of the peak markers ss71586444, Glyma.03g035300, Glyma.03g037000, Glyma.03g037100, Glyma.03g037300, Glyma.03g037400, and Glyma.03g037900. The resistance genes, Rps1a, Rps1b, Rps1c, Rps1d, Rps1k, and Rps7, do not confer resistance to both of these isolates, thus potentially, resistance to PPR and OH-Windfall could be new Rps genes, as there were no NBS-LRR genes within the 240 kb of each of these markers in the Wm82.a2 sequence (soybase.org). Also noteworthy is that more than one location on chromosome 3 was mapped at this locus and could be contributing to the maintenance of heterozygosity in this population to these isolates.
Resistance was mapped toward isolates OH0217 and PPR on chromosome 13 near markers ss715615002 and ss715615118, respectively (Figure 3B). These two resistance loci were approximately 1 cM apart, and for both, the LOD was <7.15 and explained ¾10.2% of the genetic variation (Table 6). This is unexpectedly low for a dominant R-gene. This could be a novel gene or potentially incomplete expression of an R gene on chromosome 13 as Rps3a, 3b, 3c, and 8 are not effective against PPR, while Rps3a, 3c, and 8 are effective toward OH0217. This region of 2 Mb has 26 genes annotated with LRR domains.
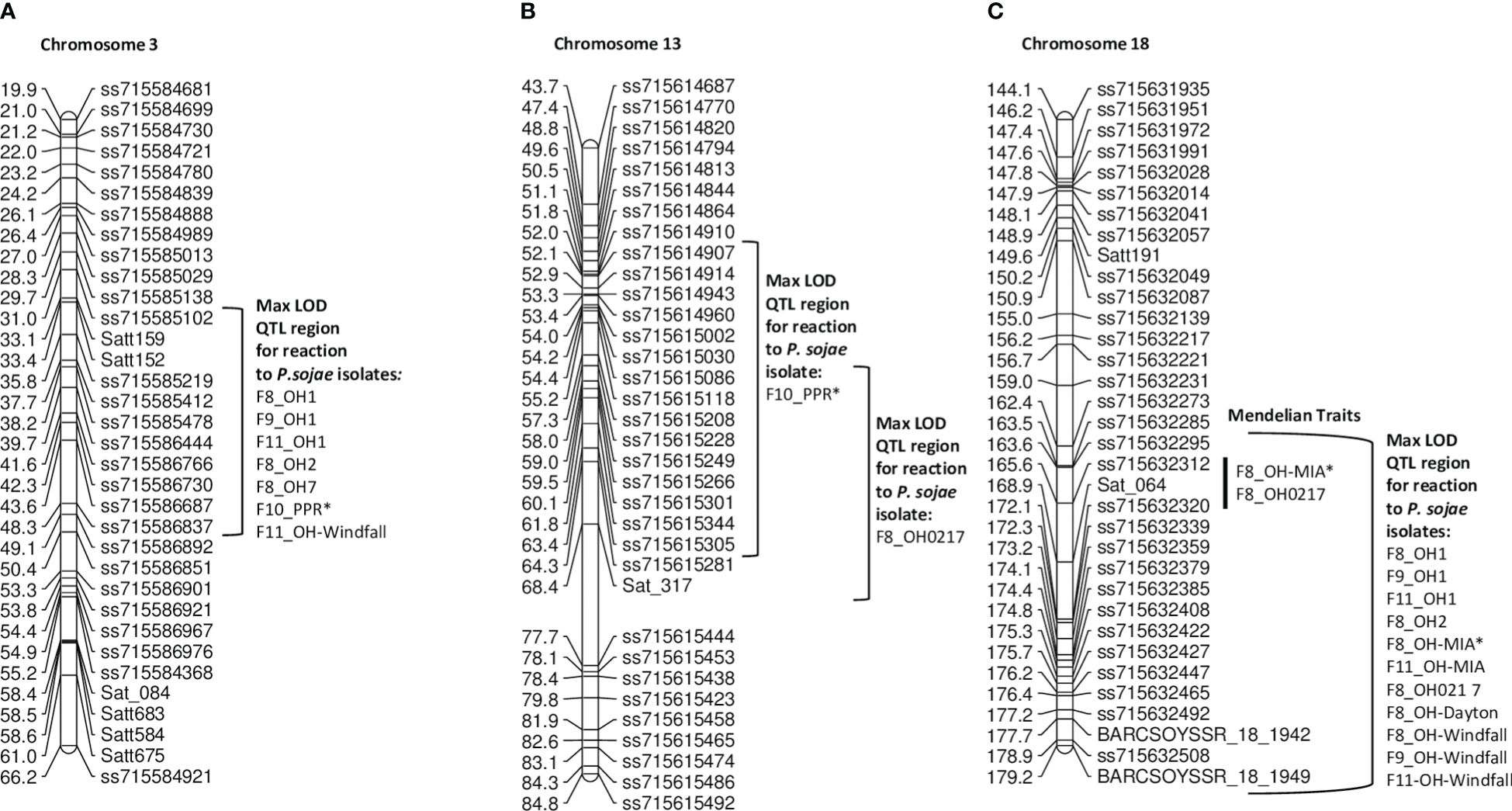
Figure 3 (A) Chromosome 3 regional map for Mendelian and QTL mapping in PI 408097 × Williams (positions of Rps loci in the inner bracket denoted by a black line). The max LOD region for reaction to isolates OH1, OH2, OH7, OH-Windfall, and PPR are denoted by the bracketed region to the right side of the map. (B) Chromosome 13 regional map for QTL mapping in PI 408097 × Williams. The max LOD region for reaction to isolates PPR and OH0217 are denoted by the bracketed regions to the right side of the map. (C) Chromosome 18 regional map for Mendelian and QTL mapping in PI 408097 × Williams (positions of Rps loci in the inner bracket denoted by a black line). The max LOD region for reaction to isolates OH1, OH2, OH0217, OH-Windfall, OH-MIA, and OH-Dayton are denoted by the bracketed region to the right side of the map. Isolates designated with an asterisk (*) indicate that disease screening results from two replications were combined into a single dataset; the remaining are from one replication.
Resistance to OH1, OH2, OH-MIA, OH0217, OH-Dayton, and OH-Windfall all mapped to chromosome 18 (Figures 3C). As mentioned above, resistance was segregating as two genes toward these isolates; thus, the second gene was detected in the mapping process. The marker at the maximum LOD, ss715632320, was the same for all but one isolate in one generation (OH-Windfall F8) (Table 6). There are nine genes within 240 kb of this SNP marker with LRR domains. This was the largest of the four populations with each phenotypic data set containing 242 or more RILs, so it is surprising that these loci were not better defined.
In the PI424477 × Williams population, 163 RILs were phenotyped individually with five P. sojae isolates separately in the F7 generation and with the combined inoculum, PPR, in the F9 generation (Table 7, Figures 4A, B). Based on chi-square analysis, the resistance response to isolates OH-MIA, OH7, OH25, and PPR segregated as single Rps genes, while OH1 segregated as two. QTL for resistance to OH1 mapped on chromosomes 3 and 13.
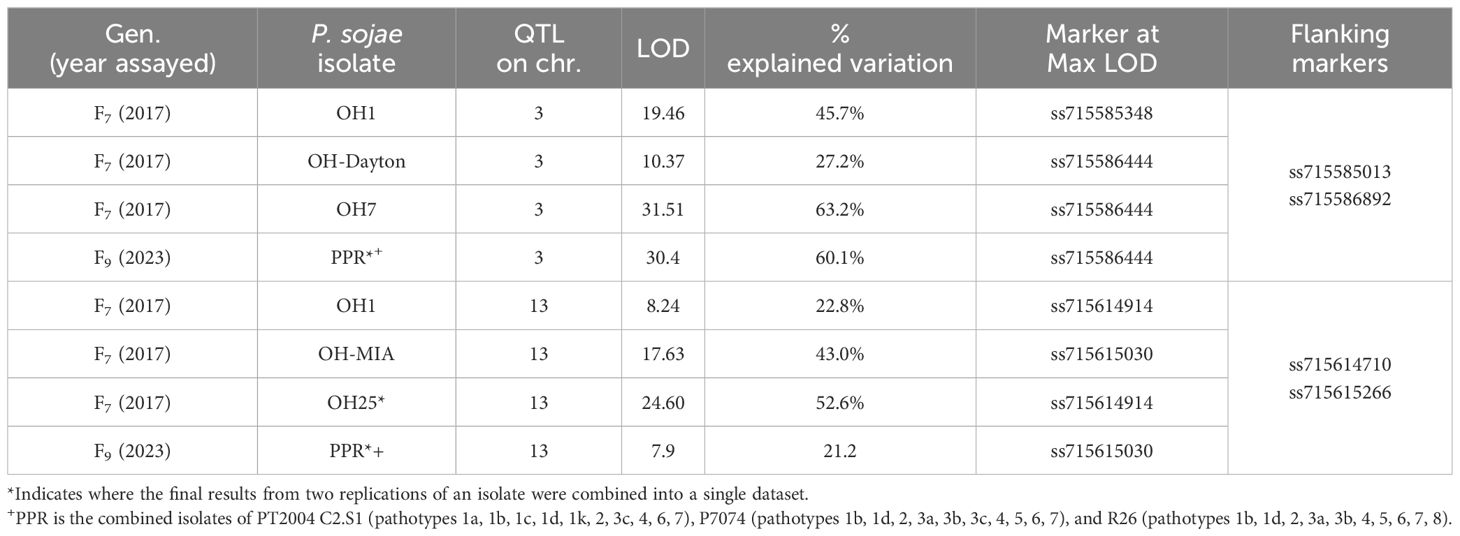
Table 7 Resistance in PI 424477 × Williams toward Phytophthora sojae in F7 and F9 of the RIL population based on QTL mapping.
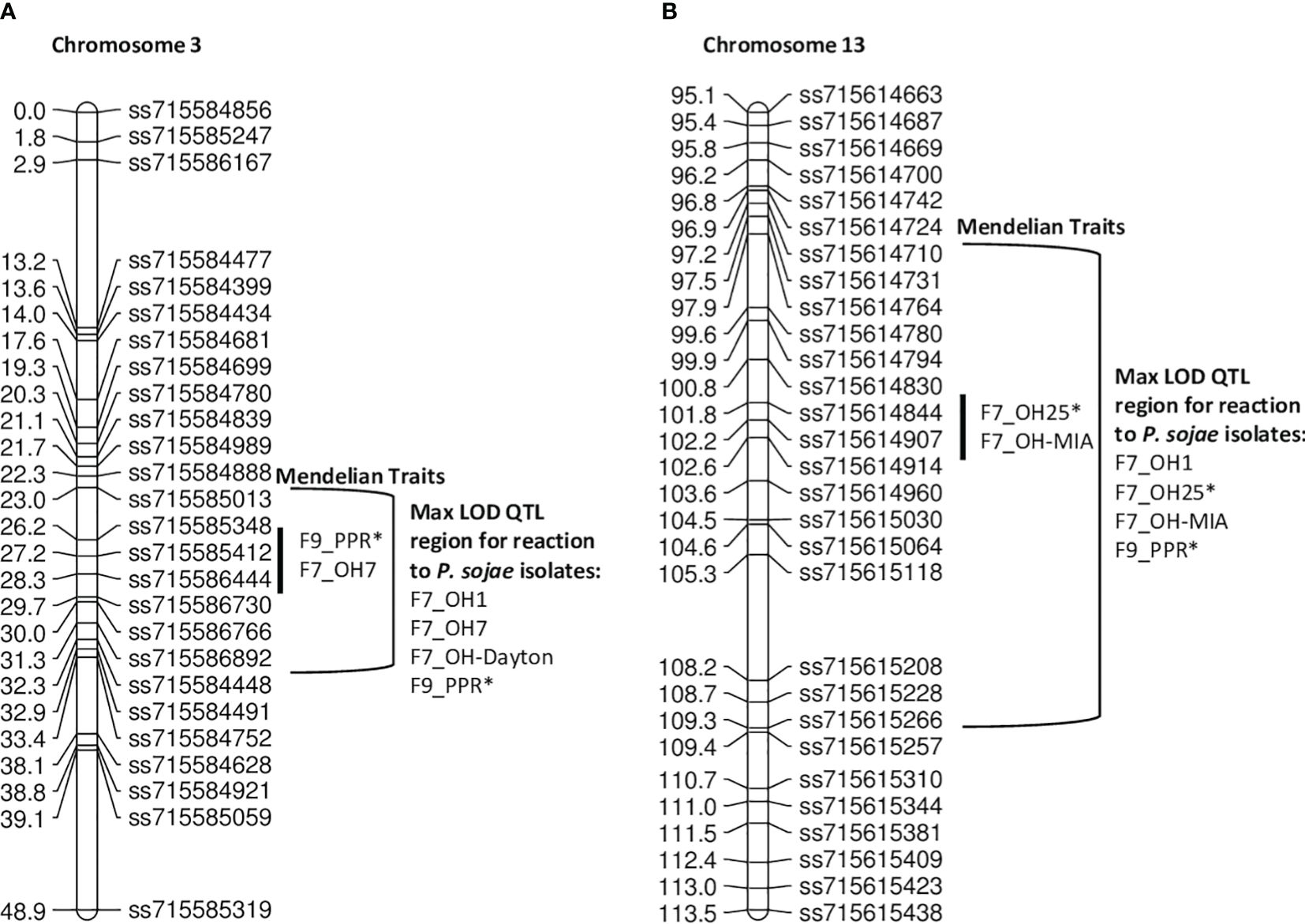
Figure 4 (A) Chromosome 3 regional map for Mendelian and QTL mapping in PI 424477 × Williams (positions of Rps loci in the inner bracket denoted by a black line). The max LOD region for reaction to isolates OH1, OH7, OH-Dayton, and PPR are denoted by the bracketed region to the right side of the map. Those isolates with an * indicates where the final results from two replications of an isolate were combined into a single dataset. (B) Chromosome 13 regional map for Mendelian and QTL mapping in PI 424477 × Williams (positions of Rps loci in the inner bracket denoted by a black line). The max LOD region for reaction to isolates OH1, OH25, OH-MIA, and PPR are denoted by the bracketed regions to the right side of the map. Isolates designated with an asterisk (*) indicate that disease screening results from two replications were combined into a single dataset; the remaining are from one replication.
The P. sojae isolates OH7 and PPR mapped within 1 cM between ss715585412 and ss715586444 on chromosome 3 based on Mendelian mapping (Supplementary Table 1). More importantly, the marker at the max LOD score with QTL mapping for P. sojae isolates OH7, OH-Dayton, and PPR was ss715586444 on chromosome 3 (Table 7). For these three isolates, LOD scores ranged from 10.37 to 31.51, and QTL mapping explained between 27.2% and 63.2% of the phenotypic variation (Table 7; Supplementary Figure 4A). There are 28 genes annotated with leucine-rich repeats and disease resistance within this region based on Wm82.a2 sequence. Thus, there could potentially be one to two novel Rps genes in this region between SNP markers ss715585412 and ss715586444 that covers 1.3 Mb. Both OH-Dayton and PPR have virulence to known Rps genes, Rps1a, Rps1b, Rps1c, Rps1d, Rps1k, and Rps7, thus indicating a high probability of a new allele at this locus.
With Mendelian mapping, P. sojae isolates OH25 and OH-MIA were 1 cM apart on chromosome 13 (Figure 4B). The isolates OH25 and OH-MIA mapped between ss715614844 and ss715614907 (Supplementary Table 1). This potentially indicates that two different genes that confer resistance are present. This is supported through QTL mapping of OH1, OH-MIA, OH25, and PPR where two different markers have the maximum LOD score, ss715614914 (OH1 and OH25) and ss715615030 (OH-MIA and PPR) on chromosome 13 (Table 7; Supplementary Figure 4B). The distance between ss715614830 and ss715615030 on W82.a2 sequence assembly is 1.13 Mb and is known to be R-gene rich. There are at least 17 genes annotated with leucine-rich repeats in the W82.a2 assembly, and both OH-MIA and PPR have virulence toward Rps3a and 8 and PPR toward Rps3b and 3c. Thus, the allele closest to ss715615030 based on QTL mapping would have the highest probability of being novel.
As noted throughout these results, we used a combination of Mendelian and QTL mapping to identify the key regions in the soybean genome for these Rps genes. For these advanced populations, the expected proportions of heterozygosity for a single gene should range from 0.78 to 0.19 for the F7 and F9 RIL populations, respectively. However, this did not change for the phenotypic screening and, in some cases (isolate by population), remained quite high (Tables 2, 3).
Mapping by Mendelian or QTL in all four populations detected at least two resistance loci to regions covering 1 to 2 cM or 1 to 1.4 Mb on chromosomes 3, 13, and/or 18. The combined inoculum (PPR), which has virulence to all known Rps genes, was mapped to one or more locations in each population. Except for the chromosome 13 locus in population PI 407985 × Williams, mapping of resistance toward PPR was confirmed by another isolate mapped to the same region.
None of these loci conferred resistance to all isolates used in this study. For example, while PPR mapped toward one or more loci, other isolates had resistance segregating as single genes and mapping to alternative chromosomes. This strongly suggests that there are additional undescribed virulences or Avr effectors in these isolates toward more Rps genes than what are currently described and that differentials are available for.
4 Discussion
There is an essential need for continuing to identify and combine effective Rps genes, which have been highlighted by the fact that the widely used Rps genes, Rps1a, Rps1c, and Rps1k, are no longer effective management tools in the United States, Canada, and Argentina since the diversity of pathotypes has changed significantly over time in these areas (Dorrance et al., 2016; Grijalba et al., 2020a, b; Matthiesen et al., 2021; Tremblay et al., 2021; Hebb et al., 2022; McCoy et al., 2022, 2023). We were able to map resistance toward one and two genes toward five to seven P. sojae isolates in each of the RIL populations derived from four plant introductions (PI 407985, PI 408029, PI 408097, and PI 424477) previously identified as putative novel sources of disease resistance for P. sojae (Dorrance and Schmitthenner, 2000; Gordon et al., 2007a, 2007b; Matthiesen et al., 2016). Additionally, PI 408097 and PI 408029 are also sources of disease resistance for Pythium sylvaticum and Py. irregulare (Clevinger et al., 2021). PI 408097 is also a source of resistance to Py. torulosum (Clevinger et al., 2021).
Due to the number of genes segregating and the maintenance of heterozygous class in these RIL populations, both Mendelian and quantitative trait mapping were used across several generations. The mapping was similar for the LOD range and peak SNP across generations and in some populations across isolates. This phenomenon has been reported previously in studies of crosses with PIs and Williams (Gordon et al., 2007a; Ortega and Dorrance, 2011). This could be in part due to meiotic incompatibility (Ortega and Dorrance, 2011) or for other chromosomal challenges in the complex R gene loci.
Resistance toward P. sojae mapped in each of the four RIL populations to chromosome 3. Populations PI 407985 and PI 424477 mapped resistance qualitatively and quantitatively on chromosome 3, while in populations PI 408029 and PI 408097, resistance was mapped quantitatively. Soybean chromosome 3 continues to be the most valuable carrier of Rps genes against numerous isolates of P. sojae as resistance in this study as well as others. The SSR marker Satt009 has been reported to be closely linked to previously reported Rps loci on chromosome 3 including Rps1a, Rps7, Rps14, and Rps1? (Weng et al., 2001; Sugimoto et al., 2012; Lin et al., 2013; Chen et al., 2021). An SNP marker, ss715585782, in our study, is separated by 2,506 bp from Satt009 on the physical map of chromosome 3. Thus, resistance mapped to genomic regions in this study either partially or mostly overlapped where a number of Rps loci were previously identified including Rps1, Rps7, Rps9, Rps14, RpsHC18, RpsHN, RpsQ, RpsUN1, RpsYD29, RpsYU25, RpsWY, RpsX, and Rps of cv. Waseshiroge and Rps of cv. Daewon, and Rps identified in PI 407974B (Bolaños-Carriel et al., 2022; Lin et al., 2022).
A relatively large Rps gene containing interval on chromosome 3 was detected in the PI 424477 population, which starts at 2,921,152 and ends at 4,642,708 bp. This interval completely covers the one mapped in the PI 408097 and PI 408029 populations and mostly overlaps the Rps region detected in the PI 407985 population (Supplementary Table 3). Rps9, RpsQ, RpsX, and RpsWY loci can be added to those already mentioned above regarding commonalities between Rps loci map locations reported earlier and those identified in this study. Jang et al. (2020), through linkage analysis, located an Rps locus to a 573-kb region from 3,893,390 to 4,466,635 bp on chromosome 3 in the Daepung × Daewon RIL population. The Rps resistance-related sequences identified in the current study encompass this interval, which contains 10 leucine-rich repeat- and four serine/threonine protein kinase-coding genes based on Glyma.W82.a2.v1 (Jang et al., 2020).
Resistance toward P. sojae mapped to the Rps3 region of chromosome 13 in all four populations. In populations PI 407985 and PI 408097, resistance loci were detected by QTL mapping for the combined inoculum, PPR, on chromosome 13. In population PI 408029, resistance loci were detected on chromosome 13 by QTL mapping for isolates OH4 and OH25. Population PI 424477 detected resistance toward isolates OH-MIA and OH25 on chromosome 13 using both Mendelian and QTL mapping. According to earlier published reports, the Rps3 region was mapped between Satt334 (29,609,521 bp) and Satt510 (32,196,800 bp) in one study (Demirbas et al., 2001) and slightly narrowed down to Sct_033 (30,739,608 bp) to Sat_317 (32,196,800) in another report (Sugimoto et al., 2012). In this study, all isolates affiliated with chromosome 13 were mapped in the 28,859,734- to 32,225,680-bp interval as QTL and in the 29,630,754- to 30,465,386-bp interval as a Mendelian trait. The maximum LOD region on chromosome 13 for these populations is enriched with disease-related genes based on the Williams82 version sequence. Similar to the results for chromosome 3, we do expect that these loci are new alleles to these genes based on the PPR inoculum and OH-MIA as they have virulence toward Rps3a, 3b, 3c, and 8.
The region 28,859,734–32,225,680 bp on chromosome 13 contains 33 NBS-LRR-type genes (soybase.org) and has been well studied at the sequence level (Innes et al., 2008; Ashfield et al., 2012). Resistance to several soybean pathogens is located in this region and includes Rsv1 (Hayes et al., 2004), Rpg1 (Ashfield et al., 1998), Rpv1 (Gore et al., 2002), and Rps3 (Diers et al., 1992). QTLs conferring resistance to root-knot nematodes (Tamulonis et al., 1997a, 1997b), Sclerotinia stem rot (Arahana et al., 2001) and corn ear worm (Rector et al., 1999), also map near this cluster of R genes on chromosome 13.
These locations on chromosomes 3 and 13 were somewhat unexpected in part due to the complexity and diversity of the pathotypes of the isolates used in these studies and a previous genome-wide association analysis (Van et al., 2020). Based on this analysis, 75 novel Rps loci were reported on all soybean chromosomes except 3 and 13 (Van et al., 2020). Chromosomes 3 and 13 were not identified in this GWAS study because many of the isolates showed virulence to Rps loci on chromosomes 3 and 13. In the current study, the combined inoculum, PPR, is virulent on most known Rps genes (vir 1a, 1b, 1c, 1d, 1k, 2, 3a, 3b, 3c, 4, 5, 6, 7, 8) and detected resistance factors on both chromosomes 3 and 13 in two of the populations, PI 407985 and PI 408097, via QTL mapping. Resistance to the combined inoculum, PPR, was on chromosome 3 in the PI 407985 and PI 424477 populations utilizing both Mendelian and QTL mapping. It is important to note that while all of the PIs were resistant to all of the individual isolates and the combination of isolates (PPR), based on the reaction of the RILs, the individual resistance genes identified in these populations, would not be effective alone toward all of the isolates. This emphasizes the need to consider stacking of Rps genes moving forward in cultivar development.
In two populations, PI 407985 and PI 408097, resistance to P. sojae was mapped to the lower arm of chromosome 18, in the same region as known Rps genes: Rps4 and Rps6 (Demirbas et al., 2001; Sandhu et al., 2004; Sugimoto et al., 2012; Zhang et al., 2013). Earlier-mapped Rps genes also associated with these same regions are Rps12 and RpsJS (Sun et al., 2014; Sahoo et al., 2017, 2021). Rps4 and Rps6 are thought to be allelic, and Rps4 co-segregates with Sat_064 (Figure 3C of this study: Figure 2 of Sandhu et al., 2004). In a later study, Rps13 was also shown to be allelic to Rps4 and Rps6 (Sahoo et al., 2021). The Rps loci identified in the current study could be allelic to these Rps loci in the PI 408097 population as well since they are located in the same region on chromosome 18. Rps loci for multiple generations of OH-Windfall and one generation of OH-MIA were mapped past the known end of the long arm of chromosome 18 in both the PI 407985 and PI 408097 populations. Resistance toward isolates OH4 and OH25 also mapped to the end of chromosome 18 in the Williams × PI 407974B RIL population by Bolaños-Carriel et al. (2022).
5 Conclusions
Novel Rps genes are required to continue to use this form of resistance in areas where Phytophthora root and stem rot can be yield limiting. In this study, four RIL populations derived from sources of putative novel resistance with at least 163 RILs and the largest having over 240 RILs were screened for disease reaction and subsequent mapping of Rps genes following inoculation with P. sojae. Multiple single isolates of P. sojae were used in screening over multiple generations in three of the four populations, and a combined inoculum of three isolates (PPR) was also used to screen in these populations. PI 408029 × Williams was screened in one generation with five single isolates. The similar results observed using both Mendelian and QTL mapping and the consistency of the identified resistance-related chromosomal regions over multiple generations of each population strengthen the mapping conclusions. Based on the map location and virulence pathotype of the isolates, three novel loci were identified on chromosome 3 (PI 407985, PI 408097, and PI 424477) and two on chromosome 13 (PI 408097 and PI 424477). As the known Rps genes, Rps4 and Rps6, on chromosome 18, could confer resistance to the isolates that mapped to this region, it is unlikely that we identified a novel gene, but an additional source of this resistance. The regions on chromosome 3 identified in three of the PIs (PI 407985, PI 408097, and PI 424477) are very important as these have multiple Rps genes in these regions that are closely linked together.
Several of these are putative new alleles in these regions since the populations were screened with isolates that have known virulence to these Rps loci in the differentials. They could be the same alleles with the more recently published Rps genes, but we do not have access to those genotypes currently. Sequencing is needed to verify if these results are truly the same or new alleles to some of the newly reported Rps genes.
As the combined inoculum had known virulence to all known Rps genes, this study suggests that development of new cultivars with Rps-mediated resistance would require the incorporation of all resistance genes from a PI.
Data availability statement
The raw data supporting the conclusions of this article will be made available by the authors, without undue reservation.
Author contributions
EC: Data curation, Formal analysis, Writing – original draft. RB: Data curation, Formal analysis, Investigation, Methodology, Visualization, Writing – review & editing. CS: Data curation, Investigation, Writing – review & editing. QS: Data curation, Writing – review & editing. AB: Data curation, Formal analysis, Writing – review & editing. CB-C- Data curation, Formal analysis, Writing – review & editing. AR: Methodology, Supervision, Writing – review & editing. AD: Conceptualization, Resources, Supervision, Writing – review & editing. MS: Conceptualization, Resources, Supervision, Writing – review & editing.
Funding
The author(s) declare financial support was received for the research, authorship, and/or publication of this article. This project was funded in part by the United Soybean Board (projects 1420–532-5659, 1720–172-0125, 1920–172-0110, and 2020–172-0138), the Iowa Soybean Association, the Virginia Soybean Board (467250, 467416, 453313), and the Ohio Soybean Council. MS and AR acknowledge funding from USDA-NIFA Agriculture and Food Research Initiative Competitive Grant no. 2011–68004-30104. MS, AR, and AD acknowledge support from the National Institute of Food and Agriculture, U.S. Department of Agriculture, Hatch projects at VT, ISU and OSU.
Acknowledgments
MS acknowledges additional contributions from the Agricultural Experiment Station Hatch Program and Open Access Subvention Fund—both at Virginia Tech. We wish to thank Deloris Veney and Corey Ziegler for the technical support for all of the inoculations performed at The Ohio State University.
Conflict of interest
The authors declare that the research was conducted in the absence of any commercial or financial relationships that could be construed as a potential conflict of interest.
The handling editor AF and reviewer JJ declared a past co-authorship with the author AR.
Publisher’s note
All claims expressed in this article are solely those of the authors and do not necessarily represent those of their affiliated organizations, or those of the publisher, the editors and the reviewers. Any product that may be evaluated in this article, or claim that may be made by its manufacturer, is not guaranteed or endorsed by the publisher.
Supplementary material
The Supplementary Material for this article can be found online at: https://www.frontiersin.org/articles/10.3389/fpls.2024.1394676/full#supplementary-material
References
Arahana, V. S., Graef, G. L., Specht, J. E., Steadman, J. R., Eskridge, K. M. (2001). Identification of QTLs for resistance to Sclerotinia sclerotiorum in soybean. Crop Sci. 41, 180–188. doi: 10.2135/cropsci2001.411180x
Ashfield, T., Danzer, J., Held, D., Clayton, K., Keim, P., Saghai Maroof, M. A., et al. (1998). Rpg1, a soybean gene effective against races of bacterial blight, maps to a cluster of previously identified disease resistance genes. Theor. Appl. Genet. 96, 1013–1021. doi: 10.1007/s001220050833
Ashfield, T., Egan, A. N., Pfeil, B. E., Chen, N. W. G., Podicheti, R., Ratnaparkhe, M. B., et al. (2012). Evolution of a complex disease resistance gene cluster in diploid Phaseolus and tetraploid Glycine. Plant Physiol. 159, 336–354. doi: 10.1104/pp.112.195040
Bernard, R. L. S., Kaufmann, P. E., Schmitthenner, A. F. (1957). Inheritance of resistance to Phytophthora root and stem rot in the soybean. Agron. J. 49, 391. doi: 10.2134/agronj1957.00021962004900070016x
Bolaños-Carriel, C., Batnini, A., McHale, L. K., Dorrance, A. E. (2022). Identification of resistance loci toward Phytophthora sojae in South Korean soybean plant introductions 407974B and 424487B. Crop Sci. 62, 275–285. doi: 10.1002/csc2.20596
Bradley, C. A., Allen, T. W., Sisson, A. J., Bergstrom, G. C., Bissonnette, K. M., Bond, J., et al. (2021). Soybean yield loss estimates due to diseases in the United States and Ontario, Canada, from 2015 to 2019. Plant Health Prog. 22, 483–495. doi: 10.1094/PHP-01-21-0013-RS
Brown, A. V., Conners, S. I., Huang, W., Wilkey, A. P., Grant, D., Weeks, N. T., et al. (2021). A new decade and new data at SoyBase, the USDA-ARS soybean genetics and genomics database. Nucl. Acids Res. 49, D1496–D15012. doi: 10.1093/nar/gkaa1107
Burnham, K. D., Francis, D. M., Dorrance, A. E., Fioritto, R. J., St Martin, S. K. (2002). Genetic diversity patterns among Phytophthora resistant soybean plant introductions based on ssr markers. Crop Sci. 42, 338–343. doi: 10.2135/cropsci2002.3380
Chen, L., Wang, W., Ping, J., Fitzgerald, J. C., Cai, G., Clark, C. B., et al. (2021). Identification and molecular mapping of Rps14, a gene conferring broad-spectrum resistance to Phytophthora sojae in soybean. Theor. Appl. Genet. 134, 3863–3872. doi: 10.1007/s00122-021-03933-9
Clemente, T. E., Cahoon, E. B. (2009). Soybean oil: Genetic approaches for modification of functionality and total content. Plant Physiol. 151, 1030–1040. doi: 10.1104/pp.109.146282
Clevinger, E. M., Biyashev, R., Lerch-Olson, E., Yu, H., Quigley, C., Song, Q., et al. (2021). Identification of quantitative disease resistance loci toward four Pythium species in soybean. Front. Plant Sci. 12. doi: 10.3389/fpls.2021.644746
Demirbas, A., Rector, B. G., Lohnes, D. G., Fioritto, R. J., Graef, G. L., Cregan, P. B., et al. (2001). Simple sequence repeat markers linked to the soybean Rps genes for Phytophthora resistance. Crop Sci. 41, 1220–1227. doi: 10.2135/cropsci2001.4141220x
Diers, B. W., Mansur, L., Imsande, J., Shoemaker, R. C. (1992). Mapping Phytophthora resistance loci in soybean with restriction fragment length polymorphism markers. Crop Sci. 32, 377–383. doi: 10.2135/cropsci1992.0011183X003200020020x
Dorrance, A. E. (2018a). Management of Phytophthora sojae of soybean: A review and future perspectives. Can. J. Plant Pathol. 40, 210–219. doi: 10.1080/07060661.2018.1445127
Dorrance, A. E. (2018b). ““Oomycete and fungal pathogens of soybean,”,” in Achieving sustainable cultivation of soybeans, vol. II . Ed. Ngyuen, H. T. (Burleigh Dodds Science Publishing Limited, Philadelphia, PA), 3–25.
Dorrance, A. E., Berry, S. A., Anderson, T. R., Meharg, C. (2008). Isolation, storage, pathotype characterization, and evaluation of resistance for Phytophthora sojae in soybean. Plant Health Prog. 9. doi: 10.1094/PHP-2008-0118-01-DG
Dorrance, A. E., Jia, H., Abney, T. S. (2004). Evaluation of soybean differentials for their interaction with Phytophthora sojae. Plant Health Prog. 5. doi: 10.1094/PHP-2004-0309-01-RS
Dorrance, A. E., Kurle, J., Robertson, A. E., Bradley, C. A., Giesler, L., Wise, K., et al. (2016). Pathotype diversity of Phytophthora sojae in eleven states in the United States. Plant Dis. 100, 1429–1437. doi: 10.1094/PDIS-08-15-0879-RE
Dorrance, A. E., Mcclure, S. A., Desilva, A. (2003b). Pathogenic diversity of Phytophthora sojae in Ohio soybean fields. Plant Dis. 87, 139–146. doi: 10.1094/PDIS.2003.87.2.139
Dorrance, A. E., Mcclure, S. A., Martin, S. K. S. (2003a). Effect of partial resistance on Phytophthora stem rot incidence and yield of soybean in Ohio. Plant Dis. 87, 308–312. doi: 10.1094/PDIS.2003.87.3.308
Dorrance, A. E., Schmitthenner, A. F. (2000). New sources of resistance to Phytophthora sojae in the soybean plant introductions. Plant Dis. 84, 1303–1308. doi: 10.1094/PDIS.2000.84.12.1303
Gordon, S. G., Berry, S. A., St Martin, S. K., Dorrance, A. E. (2007a). Genetic analysis of soybean plant introductions with resistance to Phytophthora sojae. Phytopathology 97, 106–112. doi: 10.1094/PHYTO-97-0106
Gordon, S. G., Kowitwanich, K., Pipatpongpinyo, W., St. Martin, S. K., Dorrance, A. E. (2007b). Molecular marker analysis of soybean plant introductions with resistance to Phytophthora sojae. Phytopathology 97, 113–118. doi: 10.1094/PHYTO-97-0113
Gore, M. A., Hayes, A. J., Jeong, S. C., Yue, Y. G., Buss, G. R., Saghai Maroof, M. A. (2002). Mapping tightly linked genes controlling potyvirus infection at the Rsv1 and Rpv1 region in soybean. Genome 45, 592–599. doi: 10.1139/g02-009
Grau, C. R., Dorrance, A. E., Bond, J., Russin, J. S. (2004). “Fungal Diseases,” in Soybeans: Improvement, Production, and Uses. Eds. Shibles, R. M., Harper, J. E., Wilson, R. F., Shoemaker, R. C. (American Society of Agronomy, Madison, WI), 679–763. doi: 10.2134/agronmonogr16.3ed.c14
Grijalba, P. E., del C. Ridao, A., Guillin, E., Steciow, M. (2020a). Pathogenic diversity of Phytophthora sojae in the southeast of the Province of Buenos Aires. Trop. Plant Pathol. 45, 397–401. doi: 10.1007/s40858-020-00364-7
Grijalba, P. E., Martinez, M. C., Guillin, E. (2020b). Pathotype and SSR variation in Phytophthora sojae from the Argentinean Pampas. J. Phytopathol. 168, 228–243. doi: 10.1111/jph.12885
Hayes, A. J., Jeong, S. C., Gore, M. A., Yu, Y. G., Buss, G. R., Tolin, S. A., et al. (2004). Recombination within a nucleotide-binding-site/leucine-rich-repeat gene cluster produces new variants conditioning resistance to Soybean mosaic virus in soybeans. Genetics 166, 493–503. doi: 10.1534/genetics.166.1.493
Hebb, L. M., Bradley, C. A., Mideros, S. X., Telenko, D. E. P., Wise, K., Dorrance, A. E. (2022). Pathotype complexity and genetic characterization of Phytophthora sojae populations in Illinois, Indiana, Kentucky, and Ohio. Phytopathology 112, 663–681. doi: 10.1094/PHYTO-12-20-0561-R
Innes, R. W., Ameline-Torregrosa, C., Ashfield, T., Cannon, E., Cannon, S. B., Chacko, B., et al. (2008). Differential accumulation of retroelements and diversification of NB-LRR disease resistance genes in duplicated regions following polyploidy in the ancestor of soybean. Plant Physiol. 148, 4, 1740–1759. doi: 10.1104/pp.108.127902
Jang, I. H., Kang, I. J., Kim, J. M., Kang, S. T., Jang, Y. E., Lee, S. (2020). Genetic mapping of a resistance locus to Phytophthora sojae in the Korean soybean cultivar Daewon. Plant Path J. 36, 591–599. doi: 10.5423/PPJ.OA.09.2020.0173
Lin, F., Chhapekar, S. S., Vieira, C. C., Da Silva, M. P., Rojas, A., Lee, D., et al. (2022). Breeding for disease resistance in soybean: A global perspective. Theor. Appl. Genet. 135, 3773–3872. doi: 10.1007/s00122-022-04101-3
Lin, F., Zhao, M., Ping, J., Johnson, A., Zhang, B., Abney, T. S., et al. (2013). Molecular mapping of two genes conferring resistance to Phytophthora sojae in a soybean landrace PI 567139B. Theor. Appl. Genet. 126, 2177–2185. doi: 10.1007/s00122-013-2127-4
Matthiesen, R. L., Abeysekara, N. S., Ruiz-Rojas, J. J., Biyashev, R. M., Saghai-Maroof, M. A., Robertson, A. E. (2016). A method for combining isolates of Phytophthora sojae to screen for novel sources of resistance to Phytophthora stem and root rot in soybean. Plant Dis. 100, 1424–1428. doi: 10.1094/PDIS-08-15-0916-RE
Matthiesen, R. L., Schmidt, C., Garnica, V. C., Giesler, L. J., Robertson, A. E. (2021). Comparison of Phytophthora sojae populations in Iowa and Nebraska to identify effective Rps genes for Phytophthora stem and root rot management. Plant Health Prog. 22, 300–308. doi: 10.1094/PHP-02-21-0016-FI
McCoy, A. G., Belanger, R. R., Bradley, C. A., Cerritos-Garcia, D. G., Garnica, V. C., Giesler, L. J., et al. (2023). A global-temporal analysis on Phytophthora sojae resistance-gene efficacy. Nat. Commun. 14, 6043. doi: 10.1038/s41467-023-41321-7
McCoy, A. G., Noel, Z. A., Jacobs, J. L., Clouse, K. M., Chilvers, M. I. (2022). Phytophthora sojae pathotype distribution and fungicide sensitivity in Michigan. Plant Dis. 106, 425–431. doi: 10.1094/PDIS-03-21-0443-RE
Ortega, M. A., Dorrance, A. E. (2011). Microsporogenesis of Rps8/rps8 heterozygous soybean lines. Euphytica 181, 77–88. doi: 10.1007/s10681-011-0422-1
Rector, B. G., All, J. N., Parrott, W. A., Boerma, H. R. (1999). Quantitative trait loci for antixenosis resistance to corn earworm in soybean. Crop Sci. 39, 531–538. doi: 10.2135/cropsci1999.0011183X003900020038x
Saghai-Maroof, M. A., Soliman, K. M., Jorgensen, R. A., Allard, R. W. (1984). Ribosomal DNA spacer-length polymorphisms in barley: Mendelian inheritance, chromosomal location, and population dynamics. Proc. Natl. Acad. Sci. U.S.A. 81, 8014–8018. doi: 10.1073/pnas.81.24.8014
Sahoo, D. K., Abeysekara, N. S., Cianzio, S. R., Robertson, A. E., Bhattacharyya, M. K. (2017). A novel Phytophthora sojae resistance Rps12 gene mapped to a genomic region that contains several Rps genes. PloS One 12, e0169950. doi: 10.1371/journal.pone.0169950
Sahoo, D. K., Das, A., Huang, X., Cianzio, S., Bhattacharyya, M. K. (2021). Tightly linked Rps12 and Rps13 genes provide broad-spectrum Phytophthora resistance in soybean. Sci. Rep. 11, 16907. doi: 10.1038/s41598–021-96425–1
Sandhu, D., Gao, H., Cianzio, S., Bhattacharyya, M. K. (2004). Deletion of a disease resistance nucleotide-binding-site leucine-rich-repeat-like sequence is associated with the loss of the Phytophthora resistance gene Rps4 in soybean. Genetics 168, 2157–2167. doi: 10.1534/genetics.104.032037
Schmitthenner, A. F. (1985). Problems and progress in control of Phytophthora root-rot of soybean. Plant Dis. 69, 362–368. doi: 10.1094/PD-69-362
Schmitthenner, A. F. (2000). Phytophthora rot of soybean. Plant Health Prog. 1. doi: 10.1094/PHP-2000-0601-01-HM
Slaminko, T. L., Bowen, C. R., Hartman, G. L. (2010). Multi-year evaluation of commercial soybean cultivars for resistance to Phytophthora sojae. Plant Dis. 94, 368–371. doi: 10.1094/PDIS-94-3-0368
Song, Q., Jia, G., Zhu, Y., Grant, D., Nelson, R. T., Hwang, E. Y., et al. (2010). Abundance of SSR motifs and development of candidate polymorphic SSR markers (BARCSOYSSR_1.0) in soybean. Crop Sci. 50, 1950–1960. doi: 10.2135/cropsci2009.10.0607
Song, Q., Yan, L., Quigley, C., Fickus, E., Wei, H., Chen, L., et al. (2020). Soybean BARCSoySNP6K: An assay for soybean genetics and breeding research. Plant J. 104, 800–811. doi: 10.1111/tpj.14960
Sugimoto, T., Kato, M., Yoshida, S., Matsumoto, I., Kobayashi, T., Kaga, A., et al. (2012). Pathogenic diversity of Phytophthora sojae and breeding strategies to develop Phytophthora-resistant soybeans. Breed Sci. 61, 511–522. doi: 10.1270/jsbbs.61.511
Sun, J., Li, L., Zhao, J., Huang, J., Yan, Q., Xing, H., et al. (2014). Genetic analysis and fine mapping of RpsJS, a novel resistance gene to Phytophthora sojae in soybean [Glycine max (L.) Merr.]. Theor. Appl. Genet. 127, 913–919. doi: 10.1007/s00122-014-2266-2
Tamulonis, J. P., Luzzi, B. M., Hussey, R. S., Parrot, W. A., Boerma, H. R. (1997a). DNA markers associated with resistance to Javanese root-knot nematode in soybean. Crop Sci. 37, 783–788. doi: 10.2135/cropsci1997.0011183X003700030015x
Tamulonis, J., Luzzi, B. M., Hussey, R. S., Parrot, W. A., Boerma, H. R. (1997b). DNA marker analysis of loci conferring resistance to peanut root-knot nematode in soybean. Theor. Appl. Genet. 95, 664–670. doi: 10.1007/s001220050610
Tremblay, V., McLaren, D. L., Kim, Y. M., Strelkov, S. E., Conner, R. L., Wally, O., et al. (2021). Molecular assessment of pathotype diversity of Phytophthora sojae in Canada highlights declining sources of resistance in soybean. Plant Dis. 105, 4006–4013. doi: 10.1094/PDIS-04-21-0762-RE
Van, K., Rolling, W., Biyashev, R. M., Matthiesen, R. L., Abeysekara, N. S., Robertson, A. E., et al. (2020). Mining germplasm panels and phenotypic datasets to identify loci for resistance to Phytophthora sojae in soybean. Plant Genome 14, e20063. doi: 10.1002/tpg2.20063
van Ooijen, J. W. (2004). MapQTL 5: Software for the Mapping of Quantitative Trait Loci in Experimental Populations (Wageningen, Netherlands: Kyasma B.V).
van Ooijen, J. W. (2006). Joinmap 4.0: Software for the Calculation of Genetic Linkage Maps in Experimental Populations (Wageningen, Netherlands: Kyasma B.V).
Weng, C., Yu, K., Anderson, T. R., Poysa, V. (2001). Mapping genes conferring resistance to Phytophthora root rot of soybean, Rps1a and Rps7. J. Hered 92, 442–446. doi: 10.1093/jhered/92.5.442
Yan, H., Nelson, B. (2019). Adaptation of Phytophthora sojae to Rps resistance genes over the past two decades in North Dakota. Plant Health Prog. 20, 88–93. doi: 10.1094/PHP-10-18-0062-RS
Keywords: Phytophthora sojae, Rps genes, Glycine max, soybean, resistance breeding, recombinant inbred line population
Citation: Clevinger EM, Biyashev R, Schmidt C, Song Q, Batnini A, Bolaños-Carriel C, Robertson AE, Dorrance AE and Saghai Maroof MA (2024) Comparison of Rps loci toward isolates, singly and combined inocula, of Phytophthora sojae in soybean PI 407985, PI 408029, PI 408097, and PI424477. Front. Plant Sci. 15:1394676. doi: 10.3389/fpls.2024.1394676
Received: 04 March 2024; Accepted: 05 June 2024;
Published: 01 July 2024.
Edited by:
Ahmad Fakhoury, Southern Illinois University Carbondale, United StatesReviewed by:
Dechun Wang, Michigan State University, United StatesJanette Jacobs, Michigan State University, United States
Tatsuhiko Shiraiwa, Kyoto University, Japan
Copyright © 2024 Clevinger, Biyashev, Schmidt, Song, Batnini, Bolaños-Carriel, Robertson, Dorrance and Saghai Maroof. This is an open-access article distributed under the terms of the Creative Commons Attribution License (CC BY). The use, distribution or reproduction in other forums is permitted, provided the original author(s) and the copyright owner(s) are credited and that the original publication in this journal is cited, in accordance with accepted academic practice. No use, distribution or reproduction is permitted which does not comply with these terms.
*Correspondence: M. A. Saghai Maroof, c21hcm9vZkB2dC5lZHU=
†Present address: Carlos Bolanos Carriel, Universidad Central del Ecuador, Facultad de Ciencias Agricolas, Quito, Ecuador
‡These authors have contributed equally to this work and share first authorship