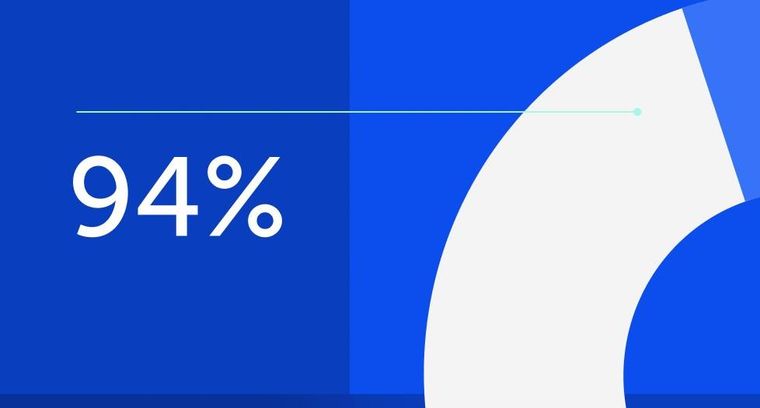
94% of researchers rate our articles as excellent or good
Learn more about the work of our research integrity team to safeguard the quality of each article we publish.
Find out more
ORIGINAL RESEARCH article
Front. Plant Sci., 30 July 2024
Sec. Plant Symbiotic Interactions
Volume 15 - 2024 | https://doi.org/10.3389/fpls.2024.1392934
This article is part of the Research TopicRhizosphere Interactions on Soil Carbon Cycle under Stress EnvironmentsView all 8 articles
Introduction: Rhizosphere effects (REs) have recently been identified as important regulators of root and microbial nutrient acquisition and are positively involved in nutrient cycling of belowground carbon (C), nitrogen (N), and phosphorus (P). Nutrient conditions of the fine roots and soil N are likely to influence REs. Still, it is unclear how REs of soil nutrients themselves variably impact the supply of nutrients to plants in terms of the responses to soil N due to succession.
Methods: In this study, we applied both fine roots and extracellular enzymes for vector analysis and stoichiometry of N:P to explore the metabolic limitations of roots and rhizospheric soil microbes and their relationships with REs across five levels of soil N (0, 5, 10, 15, and 20 kg N m−2 year−1) along successional age classes of 42, 55, and 65 years in a Pinus tabuliformis forest.
Results: Overall, the metabolism of root and rhizospheric soil microbes was mediated by soil N. N limitation of roots initially decreased before increasing, whereas that of microbes demonstrated opposite trends to the N levels owing to competition for inorganic N between them by REs of NO3−–N. However, N limitations of both roots and microbes were alleviated in young stands and increased with succession after the application of N. In addition, root N limitations were manipulated by REs of three different soil N-related indicators, i.e., total N, NH4+–N, and NO3−–N. Rhizospheric soil microbial N limitation was almost unaffected by REs due to their strong homeostasis but was an important driver in the regulation of root N limitation.
Discussion: Our results indicated that successional age was the most critical driver that directly and indirectly affected root N metabolism. However, the level of N application had a slight effect on root N limitation. Microbial N limitation and variations in the REs of N indicators regulated root N limitation in the rhizosphere. As a result, roots utilized REs to sequester N to alleviate N limitations. These findings contribute to novel mechanistic perspectives on the sustainability of N nutrition by regulating N cycling in a system of plant–soil–microbes in the rhizosphere to adapt to global N deposition or the heterogeneous distribution of bioavailable soil N with succession.
Over the last decade, there has been a rapid increase in the investigation of the rhizosphere, which is strongly affected by roots and serves as an important area of research on soil–plant–microbial interactions (Philippot et al., 2013; Vives-Peris et al., 2020; Ling et al., 2022; Jing et al., 2023; López et al., 2023) because of its critical role in terrestrial carbon (C) and nitrogen (N) cycling (Cheng et al., 2014; Finzi et al., 2015; Gan et al., 2021). Decoding the course of the rhizosphere connected with N cycling and their responses to exogenous N input has significant effects on plant physiological metabolism and growth in different life stages (Kuzyakov and Xu, 2013; Kang et al., 2022). The release of a diverse array of chemicals, including carbohydrates, organic acids, amino acids, mucilage, and exudative root cells, into the rhizosphere by living roots during plant growth and metabolism favors the improvement of soil fertility (Cheng and Kuzyakov, 2015; Carrillo et al., 2017; Vives-Peris et al., 2020). For example, Zea mays release more carbohydrates and c-aminobutyric acid into the rhizosphere to modify the P deficiency while decreasing the excretion of amino acids and the quantities of sugars to combat N and potassium (K) deficiencies (Carvalhais et al., 2011; Olanrewaju et al., 2019; Koshila Ravi and Muthukumar, 2024). This process leads to significant differences between rhizospheric soils that cling to root surfaces and bulk soils in terms of their physical, chemical, and biological properties (Kumar and Garkoti, 2022; Li et al., 2022; Herre et al., 2022a, 2022b). Specifically, it promotes the growth and development of plants, carbon sequestration, and functioning of terrestrial ecosystems by enhancing soil nutrient availability, influencing plant hormonal signaling, facilitating nutrient absorption and cycling, and alleviating abiotic stress through microorganisms in the rhizosphere (Kumar and Verma, 2019; Berger et al., 2020; Darriaut et al., 2022; Solomon et al., 2024; Zhuang et al., 2024). The magnitude of these modifications to rhizosphere attributes is often defined as the rhizosphere effect (RE) (Phillips and Fahey, 2006; Finzi et al., 2015; Gan et al., 2021).
The ecological stoichiometry of the N:P ratio, an effective indicator for detecting nutritional limitation, describes the interactions of essential elements in the global ecological processes of the balance between energy flow and nutrient cycles in terrestrial ecosystems (Elser et al., 2010; Chen et al., 2016; Ren et al., 2016; Cui et al., 2018). Nitrogen and phosphorus, two basic but the most restrictive nutritional elements, directly affect the growth and development of plants, the nutrient status of the soil, and the metabolism and activities of microbes (Elser et al., 2000, 2010; Sinsabaugh and Follstad Shah, 2011, 2012; Hu et al., 2018). Roots are essential organs that facilitate the absorption of N, P, and other elemental nutrients and serve as an interface between the soil and plants (Wang et al., 2020; Shi et al., 2021). The N:P ratios in fine roots are the most immediate and efficient reflections of growth rate and physiological adjustment to the environment, such as changes in N levels, warming, and elevated CO2 (Sardans et al., 2017; Wang et al., 2020), as well as nutrient availability and limitations (Su et al., 2019; Cao et al., 2020; Geng and Jin, 2022; Ma et al., 2022; Geng et al., 2023). Furthermore, the ecological stoichiometry of extracellular enzyme stoichiometry (EES) actively engaged in N and P cycling revealed equilibrium relationships between the relevant nutrient requirements for microbial metabolism and soil provision (Sinsabaugh et al., 2009; Sinsabaugh and Follstad Shah, 2012; Cui et al., 2019; Dong et al., 2021). Subsequently, the proposal and development of the threshold elemental ratio (TER) and enzymatic vector (V-T) models have thoroughly estimated the metabolic limitations of microbes based on traditional models, enhanced the uniformity of predictions for the metabolic limitations of microbes, and further confined the nutritional constraints of N and P (Sinsabaugh et al., 2009; Sinsabaugh and Follstad Shah, 2012; Cui et al., 2018, 2021).
Microbes use their ability to decompose soil organic matter (SOM) to acquire supplemental nutrients such as N and P via labile C as root exudates released by plant roots (Phillips et al., 2011; Dijkstra et al., 2021; He et al., 2021). REs are influenced by the availability of soil nutrients owing to the metabolic balance between microbes and fine roots (Phillips and Fahey, 2008) and may also be a useful nutritional adjunct to plants (Dijkstra et al., 2013; Hicks et al., 2020), which further affects soil C and N cycling (Dijkstra et al., 2013; He et al., 2021). REs also contribute to the maintenance of forest productivity during extra growth and provide a long-term enhancement response to elevated CO2 when forests endure a gradual increase in N limitation (Drake et al., 2011; Phillips et al., 2011; Meier et al., 2015). For example, a meta-analysis reported that roots could accelerate mineralization, and the priming of REs explained approximately 33.3% of the total soil C and N mineralization. Furthermore, the REs due to root-derived C accounted for up to 4% and 6% of gross and net primary production, respectively, in a temperate forest (Finzi et al., 2015). Therefore, the magnitude of REs is not only a crucial factor used to explain species coexistence and biodiversity (Lambers et al., 2009; Cheng et al., 2014; Hicks et al., 2020) but also a promising mechanism for evaluating the capacity of plants to accelerate soil organic carbon (SOC) turnover/accumulation (Han et al., 2020; Dijkstra et al., 2021) and resist future atmospheric N deposition (Phillips et al., 2011; Henneron et al., 2020; Gao et al., 2023). REs are emerging as a global nutritional acquisition strategy for different types of woody plants at large spatial scales and function as substitutional nutrients when bulk soil nutrients are insufficient for plant growth (Dijkstra et al., 2013; Sun et al., 2023).
Heterogeneity in the distribution of bioavailable N in soil across different regions and the incessant increase in N deposition in the atmosphere (Sun et al., 2022; McDonnell et al., 2023; Zhou et al., 2024) have led to differences in the nutrient limitation of roots and rhizospheric soil microbes, as well as rhizospheric effects that can extend through the entire ecosystem (Phillips et al., 2011; Zhang et al., 2019; Cai et al., 2023). Several studies have focused on regional and global climatic change (Dijkstra et al., 2013; Cheng et al., 2014; Terrer et al., 2016) and plant economic resource acquisition strategies (Keller et al., 2021; Sun et al., 2023). Although N deposition is a major global change, the response of REs to changes in soil N content remains poorly understood. Thus, an investigation into the effects of N application on the REs of soil nutrients linked to N and P and root and rhizospheric soil microbial nutrient limitations is critical. Specifically, it is critical to elucidate the ecological influences of N deposition on the nutritional balance and constraints in the rhizosphere of forest ecosystems.
Pinus tabuliformis is a widespread ectomycorrhizal (ECM) tree that occupies a pioneering niche in the coniferous forest ecosystems of northern China (Long et al., 2016; Taniguchi et al., 2021) and plays a dominant role as a carbon sink in forests (Song et al., 2021). This native tree species is drought-resistant and adaptable to harsh environments; therefore, it is typically used for revegetation (Song and Hou, 2020; Zhang et al., 2021). With continuous increases in N deposition in the atmosphere and the heterogeneity of the distribution of bioavailable N in the soil across different regions, it is essential to understand the responses of REs, the ecological stoichiometry of the C:N:P ratio, and the nutritional limitations of roots and microbes in the succession of P. tabuliformis forests. In this study, the metabolic limitations of both fine roots and rhizospheric soil microbes were explored by means of vector analysis of extracellular enzymes and roots based on the stoichiometry of N:P and their relationships with REs across five levels of N application (0, 5, 10, 15, and 20 kg N m−2 year−1) along a secondary succession of classes (42, 55, and 65) in a P. tabuliformis forest. Specifically, we hypothesized that 1) the application of N affects the nutritional limitations of rhizospheric soil microorganisms and fine roots, as well as REs. Furthermore, the response of nutritional microbial limitations in rhizospheric soil and roots of elder stands was more susceptible than that of young stands. 2) The nutritional limitation of roots with succession would be associated with microbial nutritional limitation in the rhizosphere and the REs.
This study was conducted at the Xiaowenshan Forest Farm (established in 1962) in the Guandishan Forest Region of Shanxi Province, northern China (Figure 1). The total area covers approximately 1.99 × 104 ha, with altitudes ranging from 1,460 to 1,610 m. This region is located in the inner continental mountain monsoon climate zone. The mean annual temperature is 4.2°C, the mean annual precipitation is 822.6 mm, and the relative humidity is 70.9%. The soil is a typical Alfisol, which is covered by a 3–7-cm humus layer. P. tabuliformis is a typical dominant tree species, with a few associated species such as Larix principis-rupprechtii, Picea wilsonii, Betula platyphylla, and Quercus mongolica. The understory species primarily comprise Spiraea salicifolia, Rosa xanthine, Corylus mandshurica, Rosa bella, and a few Lespedeza bicolor (Zhang et al., 2021).
In 2020, 42-year-old (42-year), 55-year-old (55-year), and 65-year-old (65-year) stands of P. tabuliformis forest were selected as experimental sites at the forest farm (111°24′E–112°37′E, 37°41′N–37°54′N) (Figure 1). A randomized block design with three replicate blocks of five levels of N application at each successional age was established before the experiment: N0 (0 kg N m−2 year−1, N1 (5 kg N m−2 year−1), N2 (10 kg N m−2 year−1), N3 (15 kg N m−2 year−1), and N4 (20 kg N m−2 year−1). Five 201-m2 circular plots (radius = 8 m) with a buffer zone (Song, 2017; Pretzsch, 2022) were included in each block. A backpack sprayer was used to apply various concentrations of urea solutions (CH4N2O) to the corresponding N application plots (N0, 0 g CH4N2O; N1, 6,608 g CH4N2O; N2, 8,913 g CH4N2O; N3, 11,217 g CH4N2O; and N4, 15,826 g CH4N2O; each added to 20 L of water) continuously before rain in mid-May, July, and September of each year (Geng and Jin, 2022). The details of the experimental sites are listed in Table 1.
In June 2022, after the continuous application of N for 1.5 years, three standard trees were randomly selected to collect the paired non-rhizospheric and rhizospheric soils, as well as the living fine roots (Ø ≤ 2 mm) in each plot of each stand age. Three 8 cm inside diameter soil cores (10 cm depth) were randomly extracted under the canopy area of each standard tree to ensure sufficient rhizospheric soil and living fine root samples following careful removal of understory plants and surface litter. The living roots of P. tabuliformis can be distinguished based on their features, including shape, color, taste, and elasticity. The fine roots were gently shaken to collect the adhered rhizospheric soil (Phillips et al., 2011). The fine roots were collected and placed in polyethylene bags. Finally, soil without any attachment to the roots was collected, which was regarded as non-rhizospheric soil. The living fine roots and paired non-rhizospheric and rhizospheric soils of three standard trees collected from each plot were pooled for a homogenized sample.
A cooler box was used to store the collected rhizospheric and non-rhizospheric soil samples, and it was ensured that their transport to the laboratory was complete within 24 h of analysis. Each soil sample was divided into two fractions. One fraction was stored at 4°C for <1 week and subsequently used for the analysis of soil enzyme activity. The other fraction was sieved using a 0.25-mm sieve after air-drying at room temperature (20°C–25°C) to analyze its chemical properties, including the concentration of SOC, total N (TN), total P (TP), NH4+–N, NO3−–N, and available P (AP). The root samples were first gently rinsed in distilled deionized water over a 0.5-mm wet sieve and then dried to a constant mass at 75°C for 48 h after heat-killing at 105°C for 15 min. Finally, the dried root samples were ground into fine powder to determine their chemical properties [TN, TP, and total C (TC)].
The SOC and TC of the roots were quantified using a TOC/TN analyzer (Multi N/C 3100; Analytik Jena, Jena, Germany). The TN and TP concentrations in the paired rhizospheric and non-rhizospheric soils were quantified using Kjeldahl digestion and molybdenum blue colorimetry, respectively. Root TN and TP contents were determined by initial digestion with H2SO4–H2O2 (Nelson and Sommers, 1973; Parkinson and Allen, 1975). The soil samples were extracted using a 2 M KCl and 0.5 M NaHCO3 solution under shaking and then passed through filter paper to determine the available N and P (NH4+–N, NO3−–N, and AP) of paired rhizospheric and non-rhizospheric soils (Olsen et al., 1954; Bremner and Keeney, 1966). These parameters were determined using an automated discrete analyzer (SmartChem 450, AMS Alliance, Rome, Italy).
The activities of four soil extracellular enzymes, i.e., β-1,4-glucosidase (BG), β-1,4-N-acetylglucosaminidase (NAG), l-leucine aminopeptidase (LAP), and acid phosphatase (ACP), representing one C-acquiring, two N-acquiring, and one P-acquiring enzymes, respectively, were determined using standard fluorometric techniques and enzyme calibration (M Plex, Tecan, Männedorf, Switzerland) (Cui et al., 2019, 2021; Huang et al., 2022). The enzymatic activity was expressed as nmol g−1 h−1.
The REs of each soil nutrient variable were calculated as the percentage difference between paired rhizospheric and non-rhizospheric soil samples using the Equation 1 (Phillips and Fahey, 2006):
where CR and CN are the concentrations of the measured variables that include one soil C-related indicator (SOC), three soil N-related indicators (TN, NH4+–N, and NO3−–N), and two soil P-related indicators (TP and AP) in the paired rhizospheric and non-rhizospheric soil samples, respectively.
Extracellular enzyme N:P stoichiometry in the rhizospheric soil was calculated using the Equation 2:
where N:PSEE represents the natural logarithm of the ratio of relative enzymatic activity, which indicates N acquisition and P acquisition in rhizospheric soil (Cui et al., 2018).
The vector analysis of soil extracellular enzymes was widely applied to determine the responses of soil microbial nutritional constraints of C, N, and P to internal plant succession and external soil environmental changes, which are always calculated with Equations 3 and 4 (Cui et al., 2021; Huang et al., 2022; Kang et al., 2022; Xu et al., 2022):
The vector length (dimensionless) quantified the relative microbial C limitation. The greater the vector length, the more severe the C limitation experienced by the microbes. The magnitude of the vector angle (°) determines the relative microbial N or P limitation. Vector angles (> 45° or < 45°) indicate that the microbes experienced relative P or N limitation, respectively. The greater the vector angle, the more P-limited the microbes and vice versa for N-limited microbes. Thus, we developed a new indicator to define microbial N limitation, as the formula, Equation 5:
where Degree represents the tangent angle of the line extending from the origin of the coordinates to the points with horizontal and vertical coordinates of Ln BG/Ln ACP and Ln BG/Ln (NAG + LAP), respectively.
The N:P stoichiometry of fine roots was calculated as described by Elser, with Equation 6 (Elser et al., 2010):
where N:PCON represents the mass ratio (g N/g P) of TN: TP in the fine roots.
A new vector analysis was developed to determine the nutritional limitations of N and P in plants, similar to that in a previous study (Xu et al., 2022), and was calculated with Equation 7:
The magnitude of the vector angle (°) determines the relative N or P limitation of the fine roots. A vector angle (> 4.09° or < 3.58°) indicated that the roots were experiencing relative N or P limitation, respectively, and (3.58° < vector angle < 4.09°) indicated that the relative P or N was free from limitation in fine roots. The greater the vector angle, the more serious the N-limitation experience for the roots and vice versa for the P limitation. The degree represents the tangent angle of the line extending from the origin of the coordinates to points with the horizontal and vertical coordinates of the TN and TP contents, respectively.
Differences in the parameters representing the metabolic limitations of root and rhizospheric soil microbes and the REs between different N application levels and successional ages were tested using a two-way analysis of variance (ANOVA). Multiple comparisons among the different N application levels and successional ages were conducted using the least significant difference (LSD) test (p < 0.05). Relationships between paired nutrient REs were determined using Pearson’s correlation analysis. The relationships between root and rhizospheric soil microbial nutrient limitations and REs were identified using linear regression analysis.
The relative importance of TC content in fine roots, rhizospheric soil microbial nutrient limitation, and REs in the regulation of root nutrient limitation was demonstrated using random forest analysis and the random forest R package. The complicated directions of multiple variables and the direct and indirect effects of the predicted variables on the metabolic limitations of the roots were quantified by constructing structural equation models (SEMs) based on our fundamental knowledge in conjunction with the results of linear regression analysis and random forest modeling (Zhao et al., 2022). Moreover, we used five metrics containing low chi-square value (χ2), high p-values (> 0.05), high Jöreskog’s goodness fit index (GFI > 0.95), and comparative fit index (CFI > 0.95), as well as low root mean square error of approximation (RMSEA < 0.05) to verify an ideal model fit. Construction and examination of the SEM model were based on the maximum likelihood estimations and performed through the R “lavaan” package (Zhao et al., 2022). All analyses were performed using the R version 4.2.2 (R Core Team, 2022).
Both the roots and microbes in the rhizosphere exhibited serious N restrictions [root N:PCON < 14, root vector angle (> 4.09°), enzyme N:P ratios > 1, and microbial vector angle (< 45°)] (Figures 2G–J, 3C–F). Typically, the application of N generally increased the root TC, TN and TP contents of three successional ages (Figures 2A, C, E), but significantly decreased the root vector angles in both 42-year and 55-year stands (p < 0.05) (Figure 2I). This indicated an enhancement in nutrient uptake and a mitigation in root N limitation following the application of N. However, microbial N limitation in the rhizosphere of stands of three successional ages and the root N limitation in the 65-year stand were not affected by the application of N (p > 0.05) (Figures 2I, 3G), although there was a slight decrease when the level of N application was >15 kg N m−2 year−1 in root N limitation of the 65-year stand. Vector length, representing a relative microbial C limitation, was only significantly different among the levels of N application in the 65-year stand (Figure 3A).
Figure 2 C, N, and P contents, N: P stoichiometry, and N limitations of fine roots for five levels of N application of stands of different ages (A, C, E, G, I) and their changes with succession (B, D, F, H, J) in Pinus tabuliformis forest. Different small letters denote the significant differences (p < 0.05) among different N application levels within the successional age, different capital letters denote the significant differences (p < 0.05) among three successional ages, and ns represents non-significance on the basis of ANOVA and LSD tests (p > 0.05). N0 = 0 kg N m−2 year−1; N1 = 5 kg N m−2 year−1; N2 = 10 kg N m−2 year−1; N3 = 15 kg N m−2 year−1; N4 = 20 kg N m−2 year−1. 42yr, 42-year-old stand; 55yr, 55-year-old stand; 65yr, 65-year-old stand. Red dashed lines represent the mean value of each variable in each successional age. LSD, least significant difference.
Figure 3 Vector analysis and stoichiometry N: P of extracellular enzyme, as well as N limitations of rhizospheric soil microbes for five levels of N application of stands of different ages (A, C, E, G) and their changes with succession (B, D, F, H) in Pinus tabuliformis forest. Different small letters denote the significant differences (p < 0.05) among different N application levels within the successional age, different capital letters denote the significant differences (p < 0.05) among three successional ages, and ns represents non-significance on the basis of ANOVA and LSD tests (p > 0.05). Red dashed lines represent the mean value of each variable in each successional age. LSD, least significant difference.
The average values of the natural logarithmic ratios of the extracellular enzyme activity represented stoichiometric C:N:P ratios of 1: 1.30: 1.08, 1: 1.28: 1.05, and 1: 1.34: 1.09 (Figure 4), demonstrating a slight increase with succession, although the effect was not significant (Figures 3B, D, F, H). However, succession had significant effects on root elements, except for the TP content, and significantly increased the root N limitation (Figures 2B, D, F, H, J). Besides, the root TN and TP contents were linearly correlated in all three stands (Figure 5). There were no interactive effects between the application of N and succession on root and rhizospheric soil microbial N limitations (p > 0.05; Table 2).
Figure 4 Standard major analysis of soil extracellular enzyme stoichiometry (C: N, C: P and N: P) (A–C) to identify the relative nutritional constraint for N or P of rhizospheric soil microbes. Black dashed lines denote referenced lines with slopes of 1.0 for the extracellular enzyme stoichiometry.
Figure 5 Simple linear regression analysis to identify the relative nutritional constraint for N or P of fine roots of stands of 42-, 55- (A), (B) and 65-year-old (C). Black dashed lines denote referenced lines with slopes of 14 for the N:P stoichiometry.
Table 2 Interaction effects of successional ages and the levels of N application tested by two-way ANOVA.
The application of N generally reduced the REs of SOC and TN in stands of three successional ages (except REs of TN in a 42-year stand), whereas it increased the REs of two soil P-related indicators, that is, TP and AP (except REs of AP in the 65-year stand) (Figures 6A, C, E, K). The REs of NH4+–N and NO3−–N demonstrated a similar pattern after the application of N, which decreased in the 42-year stand and increased in the 55-year and 65-year stands (Figures 6G, I).
Figure 6 REs on SOC, TN, and TP, as well as available nutrients (NH4+–N, NO3−–N, and AP) for five levels of N application of stands of different ages (A, C, E, G, I, K) and their changes with succession (B, D, F, H, J, L) in Pinus tabuliformis forest. Different small letters denote the significant differences (p < 0.05) among different N application levels within the successional age, different capital letters denote the significant differences (p < 0.05) among three successional ages, and ns represents non-significance on the basis of ANOVA and LSD tests (p > 0.05). N0 = 0 kg N m−2 year−1; N1 = 5 kg N m−2 year−1; N2 = 10 kg N m−2 year−1; N3 = 15 kg N m−2 year−1; N4 = 20 kg N m−2 year−1. 42yr, 42-year-old stand; 55yr, 55-year-old stand; 65yr, 65-year-old stand. White points and red dashed lines represent the mean value of each variable at each N application level and in each successional age, respectively. REs, rhizosphere effects; SOC, soil organic carbon; TN, total N; TP, total P; AP, available P; LSD, least significant difference.
The REs were generally significantly correlated with historical N rates according to the fitted model (Figure 7). The REs of the SOC and NH4+–N generally linearly decreased with N levels (except in the REs of NH4+–N in 65-year stand) (Figures 7A, D). The REs of TN and AP generally decreased non-linearly with increasing N levels and demonstrated a similar trend for both the 42-year and 55-year stands but differed from that of the 65-year stand (Figures 7B, F). However, the REs of NO3−–N generally increased non-linearly with higher N levels (Figure 7E). The REs of the TP increased linearly with the N level (Figure 7C), and the REs of the AP showed nearly no variation under different N levels (Figure 6K).
Figure 7 Regressions of the REs of six soil nutrients (SOC, TN, TP, NH4+–N, NO3−–N, and AP) along the levels of N application for three successional ages of Pinus tabuliformis forest (A–F), respectively. Lines in three colors imply the fitted linear relationships. R2 and P represent the coefficient of determination and level of significance, respectively. Gray-shaded areas indicate the 95% confidence intervals. REs, rhizosphere effects; SOC, soil organic carbon; TN, total N; TP, total P; AP, available P.
During the development of P. tabuliformis forests, REs differed following the application of N. The magnitudes of the REs of SOC and TN significantly increased with succession and were greater in the 65-year stands (Figures 6B, D). However, the REs of TP, NH4+–N, NO3−–N, and AP tended to decrease with succession and were only significant in REs of two soil P-related indicators, i.e., TP and AP (Figures 6F, H, J, L). The directions of TP and NO3−–N were positive and greater in magnitudes in 42-year stand compared with those of the other successional stages (Figures 6F, J). Generally, there were no interactive effects between N application and succession on REs, except for the REs of TP (p > 0.05; Table 2).
Pearson’s correlation analysis revealed that the root TC and TN contents were negatively correlated with the REs of SOC or REs of TN, respectively, but positively correlated with the REs of NO3−–N. However, these relationships were only significant in the REs of TN with root TC and TN contents (p < 0.05) (Figure 8). Root TN content also tended to decrease with the REs of NH4+–N; however, these relationships were not significant (p > 0.05) (Figure 8). In addition, the REs of NO3−–N were negatively correlated with the REs of NH4+–N (p < 0.05) (Figure 8).
Figure 8 The matrix of Pearson’s correlation coefficients among paired REs and the chemical property of fine roots in Pinus tabuliformis forests. *p < 0.05, ***p < 0.001. REs, rhizosphere effects.
Linear regression analysis indicated that REs of SOC, TN, TP, NH4+–N, and NO3−–N, as well as rhizospheric soil microbial N limitation, were linearly correlated with root N limitation, and their correlation coefficients were 0.30, 0.34, −0.44, 0.35, −0.33, and 0.34, respectively; p < 0.05) (Figures 9B, C). Among the variables, successional age (20.5% IncMSE), REs of TP (4.9% IncMSE), and microbial N limitation in the rhizosphere (3.8% IncMSE) were the three strongest predictors of root N limitation (Figure 10).
Figure 9 Linear regression analysis between the N limitations of rhizospheric soil microbes and root with the REs of each soil nutrient (SOC, TN, TP, NH4+–N, NO3−–N, and AP) (A, B). Linear regression analysis between the N limitations of root and rhizospheric soil microbes and their relationships with the rhizospheric soil microbial C limitation (C). The black lines with gray-shaded areas imply the least-squares linear regressions with the REs and limitations and their 95% confidence intervals. The values of R and p represent the corresponding Pearson’s correlation coefficients and level of significance, respectively. REs, rhizosphere effects; SOC, soil organic carbon; TN, total N; TP, total P; AP, available P.
Figure 10 Potential drivers of variation in regulation of root N limitation in Pinus tabuliformis forests. Percentage increases in the mean square error (%MSE) were used to estimate the relative importance of these different predicted variables as drivers in regulation of root N limitation, and higher %MSE values mean more important predictors. *p < 0.05, **p < 0.01, ns (0.05 < p < 0.1).
The results of the SEM model demonstrated that the explanatory factors cumulatively explained 64 variances in root N limitation (Figure 11A). Succession, which had the highest overall effect, was the most critical factor driving root N limitation, both directly and indirectly. The N limitation of microbes in the rhizosphere and REs of NH4+–N availability, with relatively high total effects, ranked following succession and had direct positive effects on root N limitation (Figures 11A, B). Succession had an indirect effect on root N limitation by manipulating root TC content to regulate the REs of NO3−–N availability and the N limitation of microbes (Figure 11A). In addition, we found that the REs of TN directly and positively regulated microbial N limitation in the rhizosphere (Figures 9A, 11A). However, the REs of TN and N levels had slight indirect effects on root N limitation (Figures 11A, B).
Figure 11 (A) Structural equation models (SEMs) describing multivariable effects of different predicted variables on REs of three different soil N-related indicators, i.e., total N, NH4+–N, and NO3−–N, limitations of rhizospheric soil microbes, successional ages, and roots TC content on root N limitation. Standardized path coefficients were expressed by numbers on the arrows. Proportion to the strengths of relationships is expressed by arrow widths. R2 represents the percentage of variance accounted for each response variable. CFI, GFI, and RMSEA represent comparative fit index, Jöreskog’s goodness fit index, and root mean square error of approximation, respectively. (B) Standardized direct, indirect, and total effects of each predicted variable on root N limitation calculated by SEM are demonstrated. *p < 0.05, **p < 0.01, ***p < 0.001.
Initially, we found that the roots were limited by soil N, which is consistent with our previous study on branches and leaves (Yan et al., 2023). This indicates that both the aboveground growth and underground growth were consistent. In the present study, root N limitation was significantly alleviated when N addition rates reached 20 kg N m−2 year−1 in the 42-year and 55-year stands. However, N application increased root N limitation in the 65-year stands (Figure 2I). As a fast-growing tree species prior to maturity, the increase in exogenous N in P. tabuliformis directly promoted the accumulation of root TN in the 42-year and 55-year stands (Figure 2C), which is consistent with previous studies (Kou et al., 2018; Liu et al., 2021; Geng et al., 2023). However, because the increased growth rate of P. tabuliformis caused by improved N availability exceeds the nutrient uptake rates during the maturation period, dilution effects have been observed (Deng et al., 2017; Geng et al., 2023).
Notably, the microbial vector angles were (< 45°), indicating N restriction rather than P restriction for microorganisms in the rhizospheres of stands at the three successional ages (Figures 3C, D). Similarly, application of N generally alleviated microbial N limitations in the 42-year and 55-year stands but exacerbated them in the 65-year stand, although they were not significantly affected by the application of N due to their strong homeostasis (Figures 3C, E, G) (Zhang et al., 2019). This correlated with root N limitation and was consistent with the SEM results, which suggested a key role of microorganisms in plant nutrient limitation and competitive utilization of resources between roots and rhizospheric microbes (Figure 11A). Roots and microbes in the rhizosphere are always in progressive N limitation (Figures 2I, J, 3C, D), which is attributed to the formation of strong depletion zones around the roots by continuous N uptake by plants (Kuzyakov and Xu, 2013). Similar patterns were also observed for root and microbial N limitation during succession, which increased with succession, consistent with previous studies (Zhou et al., 2017; Zhang et al., 2022), indicating that succession would increase N limitation in the root–soil–microbe system in the rhizosphere under the same level of soil N in this forest ecosystem.
The REs of SOC and TN decreased following N application, although sensitivity to N varied in P. tabuliformis stands of different ages (Figures 6A, C). Soil, a strong source of plant N acquisition, provides N resources to meet its high demand and conserves N for low demand and vice versa (He et al., 2021). The application of N reduces the mineralization of soil N in the rhizosphere, which is aligned with C inputs from root exudates (Dijkstra et al., 2013; Cheng et al., 2014; He et al., 2021). Thus, the improvement in soil N availability resulting from exogenous N input (i.e., fertilization or atmospheric N deposition) would enable plants to obtain N from the soil immediately rather than investing more C in belowground tissues and root exudates to stimulate microorganisms to decompose SOM for N acquisition (Phillips et al., 2009). However, the magnitude of the REs of SOC and TN in the 65-year stand was the greatest (Figures 6B, D, 12) in response to exacerbated root and microbial N limitations caused by lower root TC and TN contents in the roots of 65-year stands, in contrast to that in the 42-year and 55-year stands (Zhu et al., 2022) (Figure 8). This was consistent with some previous studies that showed that a dilution effect related to greater nutrient accumulation and biomass production would lead to lower N and P concentrations in plants (Guiz et al., 2016; Lü et al., 2019; Zhang et al., 2022). In addition to root exudates or rhizodeposits, microbes obtain N by assimilating the intractable decomposition of SOM to meet their elevated N requirements under intense N-limited conditions (Dijkstra et al., 2013; Kirkby et al., 2014; He et al., 2021), which is consistent with the preferential substrate utilization hypothesis (Phillips and Fahey, 2008; Dan et al., 2023).
Figure 12 Conceptual framework for exploration of the pathways of successional effects on N limitations of roots and rhizospheric soil microbes after the application of N. We displayed linkages with REs of three different soil N-related indicators, i.e., total N, NH4+–N, and NO3−–N, to demonstrate that development with succession increased the N limitations of microbes and roots. REs, rhizosphere effects.
The decreased REs of NH4+–N availability with the development of P. tabuliformis forests after the application of N was due to decreased root TN content (Figure 8), which is similar to previous findings (Hogberg et al., 1998; Jiang et al., 2020). However, positive REs of the NH4+–N availability in the 65-year stand and REs of the NO3−–N availability of all stands (Figures 6G–G, 7D, E) positively direct root TC content to the REs of the NO3−–N, positively correlate between the REs of the NO3−–N and root TN content, and positively affect the indirect REs of the NO3−–N to NH4+–N ratio induced by grave root N limitation (Figures 8, 11A) and demonstrated that differently aged natural secondary P. tabuliformis stands exhibited similar N preferences and dominantly relied on NO3−–N for its inorganic N nutrition. This was due to the greater mobile properties of NO3−–N than NH4+–N in soil solution (Kuzyakov and Xu, 2013). Our results also suggested that P. tabuliformis forests in 65-year-old absorbed NH4+–N from soils under severely N-limited conditions as a strategy to make full use of soil N sources, which enhances the primary productivity of forest ecosystems (Houle et al., 2014; Gao et al., 2020). Similarly, from this perspective, the REs of TN and NH4+–N availability alleviated root N limitation.
The REs of TP increased linearly with the N level, leading to a generally insignificant response signal of the REs of AP, which was further free from P-limited roots and microbes in the rhizosphere (Figures 6K, 7C). Lower soil pH owing to the application of N promoted phosphatase activity, which is consistent with the findings of Phillips and Fahey (Phillips and Fahey, 2006).
As shown by root exudation and nutrient absorption, REs control N cycling in the soil. The REs of the soil N indicators were susceptible to soil N in P. tabuliformis stands at the three successional ages. Numerous studies have shown that applying N to soil has variable effects on each RE indicator of N indicators (Cai et al., 2023; Dan et al., 2023; Gao et al., 2023). Moreover, REs affect the nutrient supply to plants and are essential drivers for alleviating root N limitation (Sun et al., 2014; Han et al., 2023). Linear regression and RF analyses revealed the importance of the REs of soil N indicators and the N limitation of microbes in the rhizosphere for root N limitation (Figures 9B, C, 10). SEMs revealed that the root TC content indirectly mitigated root N limitations by regulating the REs of NO3−–N availability and N limitations of microbes in the rhizosphere (Figure 11A). Thus, we put forward a conceptual diagram to summarize the effects of succession on the N limitation of roots and rhizospheric soil microbes (Figure 12). We determined the characteristics of stands of three successional ages by comparing their REs, microbial C and N limitations, and root N limitation among the five levels of N application using radar charts in P. tabuliformis forests after N input (Figure 13).
Figure 13 Comparison of REs of each soil nutrient (SOC, TN, TP, NH4+–N, NO3−–N, and AP), microbial C, N limitation, N:PSEE, and root N limitations among five N application levels of stands of 42-, 55- (A), (B) and 65-year-old (C). REs, rhizosphere effects; SOC, soil organic carbon; TN, total N; TP, total P; AP, available P.
Stand development enhanced the fine root biomass production, resulted in weaker fine root nutrient foraging ability, and caused lower soil N nutrient availability (Ryan et al., 2004; Lü et al., 2019; Zhu et al., 2022). These changes directly decreased root TC and TN concentrations, increased investment in belowground photosynthetic products such as root exudates, and increased microbial N limitation in the rhizosphere symbiosed with roots when plant growth was N-constrained (Figures 2B, D, 3F, H) (Guiz et al., 2016; Geng et al., 2021; He et al., 2021; Zhang et al., 2022; Geng et al., 2023). However, the increase in microbial N limitation with succession is insignificant owing to their faster turnover rates and strong homeostasis (Zhang et al., 2019, 2024). Root exudate inputs induced by succession promote the growth and activity of microorganisms and further stimulate stronger REs of SOC and TN and theoretically stronger REs of NH4+–N and NO3−–N availability to obtain more N resources (Figures 6B, D) (He et al., 2021; Zhu et al., 2022). In fact, continuous available N uptake by roots and microbes in the rhizosphere and the increased competitive utilization of resources between them led to strong depletion zones near the roots (Kuzyakov and Xu, 2013; Zhang et al., 2024), which ultimately decreased the REs of NH4+–N and NO3−–N availability with succession (Figures 6H, J). In addition, REs of NH4+–N also directly and positively drove the root N limitation and directly controlled the transformation from REs of NO3−–N (Figure 11A). This was because of the poor mobile properties in the soil solution of NH4+–N compared with NO3−–N and was not quickly absorbed by microbes in the rhizosphere but could be easily uptake by roots, making the roots outcompete in the uptake of N in the rhizosphere under N-limited condition (Kuzyakov and Xu, 2013; Huygens et al., 2016; Zhang et al., 2024).
The results of the radar charts showed that root N limitation of stands of the three ages and REs of TP in the 42-year stand generally increased with N levels, whereas root N limitation and REs of SOC greatly increased with succession (Figure 13). This result indicates that the adverse effect of N application on root N limitation in the 42-year stand was the smallest among the three stands of different ages. Therefore, the afforestation activities of the natural secondary P. tabuliformis forest in this area should prioritize middle-aged forests (40–50 years old) to adapt to global N deposition or the distribution heterogeneity of bioavailable soil N.
We assessed the responses of N limitation in the roots and rhizospheric soil microbes and the REs to N application of N-limited natural secondary P. tabuliformis forests of different successional ages. N limitation characterized the root and microbial communities. Older tree stands were subject to relatively strong root and microbial N limitations compared to younger stands in response to N. The magnitudes of the REs of SOC and TN were significantly greater in the 65-year stands. However, the magnitudes of the REs of P indicators and available N indicators, which were NH4+–N and NO3–N, were significantly lower in the 65-year stand.
Root N limitation was primarily contingent on variations in the REs of three different soil N-related indicators, TN, NH4+–N, and NO3−–N. Rhizospheric soil microbial N limitation was one of the most important factors driving root N limitation. The level of N application had only a slight effect on root N limitation. In addition, the root TC content, driven by successional age, directly affected microbial N limitation. This suggested that succession of P. tabuliformis forest increased microbial metabolic N limitation and further increased root N limitation owing to the formation of strong depletion zones near the roots but stimulated stronger REs to obtain more N in a N-limited forest ecosystem.
The raw data supporting the conclusions of this article will be made available by the authors, without undue reservation.
SD: Conceptualization, Formal analysis, Investigation, Software, Writing – original draft, Writing – review & editing. JG: Funding acquisition, Supervision, Writing – review & editing. YZ: Formal analysis, Investigation, Writing – review & editing. LL: Investigation, Writing – review & editing. RW: Investigation, Writing – review & editing. RZ: Investigation, Writing – review & editing.
The author(s) declare financial support was received for the research, authorship, and/or publication of this article. This work was supported by the National Natural Science Foundation of China (30970480).
We thank the Xiaowenshan Forest Farm for our fieldwork and our colleagues for their help in field sampling and laboratory analyses.
The authors declare that the research was conducted in the absence of any commercial or financial relationships that could be construed as a potential conflict of interest.
All claims expressed in this article are solely those of the authors and do not necessarily represent those of their affiliated organizations, or those of the publisher, the editors and the reviewers. Any product that may be evaluated in this article, or claim that may be made by its manufacturer, is not guaranteed or endorsed by the publisher.
REs, rhizosphere effects; SOM, soil organic matter; DBH, diameter at breast height; EES, extracellular enzyme stoichiometry; TER, threshold elemental ratio; V-T, enzymatic vector; ECM, ectomycorrhizal; SOC, soil organic carbon; TN, total N; TP, total P; NH4+–N, ammonium ions; NO3−–N, nitrates; AP, available P; TC, total C; BG, β-1,4-glucosidase; NAG, β-1,4-N-acetylglucosaminidase; LAP, l-leucine aminopeptidase; ACP, acid phosphatase.
Berger, S., Van Wees, S. C. M., Nybroe, O., Großkinsky, D. K. (2020). Editorial: cross-frontier communication: phytohormone functions at the plant-microbe interface and beyond. Front. Plant Sci. 11. doi: 10.3389/fpls.2020.00386
Bremner, J. M., Keeney, D. R. (1966). Determination and isotope-ratio analysis of different forms of nitrogen in soils: 3. Exchangeable ammonium, nitrate, and nitrite by extraction-distillation methods. Soil Sci. Soc. Amer J. 30, 577–582. doi: 10.2136/sssaj1966.03615995003000050015x
Cai, A., Tang, S., Waqas, M. A., Wang, B., Tian, D., Zhang, Y., et al. (2023). Magnitude, direction, and drivers of rhizosphere effect on soil nitrogen and phosphorus in global agroecosystem. Int. Soil Water Conserv. Res. 11, 482–493. doi: 10.1016/j.iswcr.2022.07.004
Cao, Y., Li, Y., Zhang, G., Zhang, J., Chen, M. (2020). Fine root C:N:P stoichiometry and its driving factors across forest ecosystems in northwestern China. Sci. Total Environ. 737, 140299. doi: 10.1016/j.scitotenv.2020.140299
Carrillo, Y., Bell, C., Koyama, A., Canarini, A., Boot, C. M., Wallenstein, M., et al. (2017). Plant traits, stoichiometry and microbes as drivers of decomposition in the rhizosphere in a temperate grassland. J. Ecol. 105, 1750–1765. doi: 10.1111/1365-2745.12772
Carvalhais, L. C., Dennis, P. G., Fedoseyenko, D., Hajirezaei, M., Borriss, R., Von Wirén, N. (2011). Root exudation of sugars, amino acids, and organic acids by maize as affected by nitrogen, phosphorus, potassium, and iron deficiency. Z. Pflanzenernähr. Bodenk. 174, 3–11. doi: 10.1002/jpln.201000085
Chen, Y., Chen, L., Peng, Y., Ding, J., Li, F., Yang, G., et al. (2016). Linking microbial C:N:P stoichiometry to microbial community and abiotic factors along a 3500-km grassland transect on the Tibetan Plateau. Global Ecol. Biogeogr. 25, 1416–1427. doi: 10.1111/geb.12500
Cheng, W., Kuzyakov, Y. (2015). “Root effects on soil organic matter decomposition,” in Agronomy monographs. Eds. Zobel, R. W., Wright, S. F. (American Society of Agronomy, Crop Science Society of America, Soil Science Society of America, Madison, WI, USA), 119–143. doi: 10.2134/agronmonogr48.c7
Cheng, W., Parton, W. J., Gonzalez-Meler, M. A., Phillips, R., Asao, S., McNickle, G. G., et al. (2014). Synthesis and modeling perspectives of rhizosphere priming. New Phytol. 201, 31–44. doi: 10.1111/nph.12440
Cui, Y., Bing, H., Fang, L., Jiang, M., Shen, G., Yu, J., et al. (2019). Extracellular enzyme stoichiometry reveals the carbon and phosphorus limitations of microbial metabolisms in the rhizosphere and bulk soils in alpine ecosystems. Plant Soil 458, 7–20. doi: 10.1007/s11104-019-04159-x
Cui, Y., Fang, L., Guo, X., Wang, X., Zhang, Y., Li, P., et al. (2018). Ecoenzymatic stoichiometry and microbial nutrient limitation in rhizosphere soil in the arid area of the northern Loess Plateau, China. Soil Biol. Biochem. 116, 11–21. doi: 10.1016/j.soilbio.2017.09.025
Cui, Y., Moorhead, D. L., Guo, X., Peng, S., Wang, Y., Zhang, X., et al. (2021). Stoichiometric models of microbial metabolic limitation in soil systems. Global Ecol. Biogeogr. 30, 2297–2311. doi: 10.1111/geb.13378
Dan, X., He, M., Meng, L., He, X., Wang, X., Chen, S., et al. (2023). Strong rhizosphere priming effects on N dynamics in soils with higher soil N supply capacity: The ‘Matthew effect’ in plant-soil systems. Soil Biol. Biochem. 178, 108949. doi: 10.1016/j.soilbio.2023.108949
Darriaut, R., Lailheugue, V., Masneuf-Pomarède, I., Marguerit, E., Martins, G., Compant, S., et al. (2022). Grapevine rootstock and soil microbiome interactions: Keys for a resilient viticulture. Horticulture Res. 9, uhac019. doi: 10.1093/hr/uhac019
Deng, Q., Hui, D., Dennis, S., Reddy, K. C. (2017). Responses of terrestrial ecosystem phosphorus cycling to nitrogen addition: A meta-analysis. Global Ecol. Biogeogr. 26, 713–728. doi: 10.1111/geb.12576
Dijkstra, F. A., Carrillo, Y., Pendall, E., Morgan, J. A. (2013). Rhizosphere priming: a nutrient perspective. Front. Microbiol. 4. doi: 10.3389/fmicb.2013.00216
Dijkstra, F. A., Zhu, B., Cheng, W. (2021). Root effects on soil organic carbon: a double-edged sword. New Phytol. 230, 60–65. doi: 10.1111/nph.17082
Dong, H., Ge, J., Sun, K., Wang, B., Xue, J., Wakelin, S. A., et al. (2021). Change in root-associated fungal communities affects soil enzymatic activities during Pinus massoniana forest development in subtropical China. For. Ecol. Manage. 482, 118817. doi: 10.1016/j.foreco.2020.118817
Drake, J. E., Gallet-Budynek, A., Hofmockel, K. S., Bernhardt, E. S., Billings, S. A., Jackson, R. B., et al. (2011). Increases in the flux of carbon belowground stimulate nitrogen uptake and sustain the long-term enhancement of forest productivity under elevated CO2: C fluxes belowground and long-term FACE productivity. Ecol. Lett. 14, 349–357. doi: 10.1111/ele.2011.14.issue-4
Elser, J. J., Fagan, W. F., Denno, R. F., Dobberfuhl, D. R., Folarin, A., Huberty, A., et al. (2000). Nutritional constraints in terrestrial and freshwater food webs. Nature 408, 578–580. doi: 10.1038/35046058
Elser, J. J., Fagan, W. F., Kerkhoff, A. J., Swenson, N. G., Enquist, B. J. (2010). Biological stoichiometry of plant production: metabolism, scaling and ecological response to global change. New Phytol. 186, 593–608. doi: 10.1111/j.1469-8137.2010.03214.x
Finzi, A. C., Abramoff, R. Z., Spiller, K. S., Brzostek, E. R., Darby, B. A., Kramer, M. A., et al. (2015). Rhizosphere processes are quantitatively important components of terrestrial carbon and nutrient cycles. Global Change Biol. 21, 2082–2094. doi: 10.1111/gcb.12816
Gan, D., Feng, J., Han, M., Zeng, H., Zhu, B. (2021). Rhizosphere effects of woody plants on soil biogeochemical processes: a meta-analysis. Soil Biol. Biochem. 160, 108310. doi: 10.1016/j.soilbio.2021.108310
Gao, L., Cui, X., Hill, P. W., Guo, Y. (2020). Uptake of various nitrogen forms by co-existing plant species in temperate and cold-temperate forests in northeast China. Appl. Soil Ecol. 147, 103398. doi: 10.1016/j.apsoil.2019.103398
Gao, Y., Tan, Z., Wang, H., Zhu, Y. (2023). Nitrogen fertilization and the rhizosphere effect on nitrogen cycling: A meta-analysis. Appl. Soil Ecol. 186, 104788. doi: 10.1016/j.apsoil.2022.104788
Geng, P., Jin, G. (2022). Fine root morphology and chemical responses to N addition depend on root function and soil depth in a Korean pine plantation in Northeast China. For. Ecol. Manage. 520, 120407. doi: 10.1016/j.foreco.2022.120407
Geng, Q., Ma, X., Liao, J., Wu, W., Niu, S., Luo, Y., et al. (2021). Contrasting nutrient-mediated responses between surface and deep fine root biomass to N addition in poplar plantations on the east coast of China. For. Ecol. Manage. 490, 119152. doi: 10.1016/j.foreco.2021.119152
Geng, Q., Ma, X., Peng, F., Zhu, Z., Li, Q., Xu, D., et al. (2023). Consistent responses of the C:N:P stoichiometry of green leaves and fine roots to N addition in poplar plantations in eastern coastal China. Plant Soil. 485, 377–394. doi: 10.1007/s11104-022-05836-0
Guiz, J., Hillebrand, H., Borer, E. T., Abbas, M., Ebeling, A., Weigelt, A., et al. (2016). Long-term effects of plant diversity and composition on plant stoichiometry. Oikos 125, 613–621. doi: 10.1111/oik.02504
Han, M., Chen, Y., Sun, L., Yu, M., Li, R., Li, S., et al. (2023). Linking rhizosphere soil microbial activity and plant resource acquisition strategy. J. Ecol. 111, 875–888. doi: 10.1111/1365-2745.14067
Han, M., Sun, L., Gan, D., Fu, L., Zhu, B. (2020). Root functional traits are key determinants of the rhizosphere effect on soil organic matter decomposition across 14 temperate hardwood species. Soil Biol. Biochem. 151, 108019. doi: 10.1016/j.soilbio.2020.108019
He, W., Yuan, Y., Zhang, Z., Xiao, J., Liu, Q., Laiho, R., et al. (2021). Effect of N addition on root exudation and associated microbial N transformation under Sibiraea angustata in an alpine shrubland. Plant Soil 460, 469–481. doi: 10.1007/s11104-020-04753-4
Henneron, L., Kardol, P., Wardle, D. A., Cros, C., Fontaine, S. (2020). Rhizosphere control of soil nitrogen cycling: a key component of plant economic strategies. New Phytol. 228, 1269–1282. doi: 10.1111/nph.16760
Herre, M., Heinze, S., Heitkötter, J., Marschner, B. (2022a). Different factors control organic matter degradation in bulk and rhizosphere soil from the top- and subsoils of three forest stands. Soil Biol. Biochem. 172, 108775. doi: 10.1016/j.soilbio.2022.108775
Herre, M., Heitkötter, J., Heinze, S., Rethemeyer, J., Preusser, S., Kandeler, E., et al. (2022b). Differences in organic matter properties and microbial activity between bulk and rhizosphere soil from the top- and subsoils of three forest stands. Geoderma 409, 115589. doi: 10.1016/j.geoderma.2021.115589
Hicks, L. C., Leizeaga, A., Rousk, K., Michelsen, A., Rousk, J. (2020). Simulated rhizosphere deposits induce microbial N-mining that may accelerate shrubification in the subarctic. Ecology 101, e03094. doi: 10.1002/ecy.3094
Hogberg, P., Hogbom, L., Schinkel, H. (1998). Nitrogen-related root variables of trees along an N-deposition gradient in Europe. Tree Physiol. 18, 823–828. doi: 10.1093/treephys/18.12.823
Houle, D., Moore, J.-D., Ouimet, R., Marty, C. (2014). Tree species partition N uptake by soil depth in boreal forests. Ecology 95, 1127–1133. doi: 10.1890/14-0191.1
Hu, Y., Shu, X., He, J., Zhang, Y., Xiao, H., Tang, X., et al. (2018). Storage of C, N, and P affected by afforestation with Salix cupularis in an alpine semiarid desert ecosystem. Land Degrad Dev. 29, 188–198. doi: 10.1002/ldr.2862
Huang, Y.-X., Wu, Z.-J., Zong, Y.-Y., Li, W.-Q., Chen, F.-S., Wang, G. G., et al. (2022). Mixing with coniferous tree species alleviates rhizosphere soil phosphorus limitation of broad-leaved trees in subtropical plantations. Soil Biol. Biochem. 175, 108853. doi: 10.1016/j.soilbio.2022.108853
Huygens, D., Díaz, S., Urcelay, C., Boeckx, P. (2016). Microbial recycling of dissolved organic matter confines plant nitrogen uptake to inorganic forms in a semi-arid ecosystem. Soil Biol. Biochem. 101, 142–151. doi: 10.1016/j.soilbio.2016.07.006
Jiang, Q., Guo, R., Song, T., Chen, T., Chen, Y., Jia, L., et al. (2020). Effects of warming and nitrogen addition on nitrogen uptake kinetics of fine roots of Cunninghamia lanceolata seedlings in different seasons. Acta Ecologica Sin. 40 (09), 2996–3005. doi: 10.5846/stxb201905070924
Jing, H., Wang, H., Wang, G., Liu, G., Cheng, Y. (2023). The mechanism effects of root exudate on microbial community of rhizosphere soil of tree, shrub, and grass in forest ecosystem under N deposition. ISME Commun. 3, 120. doi: 10.1038/s43705-023-00322-9
Kang, H., Xue, Y., Yan, C., Lu, S., Yang, H., Zhu, J., et al. (2022). Contrasting patterns of microbial nutrient limitations between rhizosphere and bulk soil during stump sprout restoration in a clear-cut oak forest. For. Ecol. Manage. 515, 120241. doi: 10.1016/j.foreco.2022.120241
Keller, A. B., Brzostek, E. R., Craig, M. E., Fisher, J. B., Phillips, R. P. (2021). Root-derived inputs are major contributors to soil carbon in temperate forests, but vary by mycorrhizal type. Ecol. Lett. 24, 626–635. doi: 10.1111/ele.13651
Kirkby, C. A., Richardson, A. E., Wade, L. J., Passioura, J. B., Batten, G. D., Blanchard, C., et al. (2014). Nutrient availability limits carbon sequestration in arable soils. Soil Biol. Biochem. 68, 402–409. doi: 10.1016/j.soilbio.2013.09.032
Koshila Ravi, R., Muthukumar, T. (2024). “Root exudates and their importance in arbuscular mycorrhizal symbiosis and nutrients navigation from inaccessible soil: an efficient mediator of mineral acquisition in nutrient deprived soil,” in Mycorrhizal symbiosis and agroecosystem restoration. Eds. Ansari, R. A., Rizvi, R., Mahmood, I. (Springer Nature Singapore, Singapore), 101–123. doi: 10.1007/978-981-99-5030-0_5
Kou, L., Chen, W., Jiang, L., Dai, X., Fu, X., Wang, H., et al. (2018). Simulated nitrogen deposition affects stoichiometry of multiple elements in resource-acquiring plant organs in a seasonally dry subtropical forest. Sci. Total Environ. 624, 611–620. doi: 10.1016/j.scitotenv.2017.12.080
Kumar, A., Verma, J. P. (2019). “The role of microbes to improve crop productivity and soil health,” in Ecological wisdom inspired restoration engineering. Eds. Achal, V., Mukherjee, A. (Springer Singapore, Singapore), 249–265. doi: 10.1007/978-981-13-0149-0_14
Kumar, S., Garkoti, S. C. (2022). Rhizosphere influence on soil microbial biomass and enzyme activity in banj oak, chir pine and banj oak regeneration forests in the central Himalaya. Geoderma 409, 115626. doi: 10.1016/j.geoderma.2021.115626
Kuzyakov, Y., Xu, X. (2013). Competition between roots and microorganisms for nitrogen: mechanisms and ecological relevance. New Phytol. 198, 656–669. doi: 10.1111/nph.12235
Lambers, H., Mougel, C., Jaillard, B., Hinsinger, P. (2009). Plant-microbe-soil interactions in the rhizosphere: an evolutionary perspective. Plant Soil 321, 83–115. doi: 10.1007/s11104-009-0042-x
Li, W., Li, Y., Lv, J., He, X., Wang, J., Teng, D., et al. (2022). Rhizosphere effect alters the soil microbiome composition and C, N transformation in an arid ecosystem. Appl. Soil Ecol. 170, 104296. doi: 10.1016/j.apsoil.2021.104296
Ling, N., Wang, T., Kuzyakov, Y. (2022). Rhizosphere bacteriome structure and functions. Nat. Commun. 13, 836. doi: 10.1038/s41467-022-28448-9
Liu, G., Xing, Y., Wang, Q., Wang, L., Feng, Y., Yin, Z., et al. (2021). Long-term nitrogen addition regulates root nutrient capture and leaf nutrient resorption in Larix gmelinii in a boreal forest. Eur. J. For. Res. 140, 763–776. doi: 10.1007/s10342-021-01364-1
Long, D., Liu, J., Han, Q., Wang, X., Huang, J. (2016). Ectomycorrhizal fungal communities associated with Populus simonii and Pinus tabuliformis in the hilly-gully region of the Loess Plateau, China. Sci. Rep. 6, 24336. doi: 10.1038/srep24336
López, J. L., Fourie, A., Poppeliers, S. W. M., Pappas, N., Sánchez-Gil, J. J., De Jonge, R., et al. (2023). Growth rate is a dominant factor predicting the rhizosphere effect. ISME J. 17, 1396–1405. doi: 10.1038/s41396-023-01453-6
Lü, X., Hu, Y., Wolf, A. A., Han, X. (2019). Species richness mediates within-species nutrient resorption: Implications for the biodiversity–productivity relationship. J. Ecol. 107, 2346–2352. doi: 10.1111/1365-2745.13180
Ma, Y., Wang, D., Guo, X., Zhu, Y.-G., Delgado-Baquerizo, M., Chu, H. (2022). Root stoichiometry explains wheat endophytes and their link with crop production after four decades of fertilization. Sci. Total Environ. 846, 157407. doi: 10.1016/j.scitotenv.2022.157407
McDonnell, T. C., Phelan, J., Talhelm, A. F., Cosby, B. J., Driscoll, C. T., Sullivan, T. J., et al. (2023). Protection of forest ecosystems in the eastern United States from elevated atmospheric deposition of sulfur and nitrogen: A comparison of steady-state and dynamic model results. Environ. Pollut. 318, 120887. doi: 10.1016/j.envpol.2022.120887
Meier, I. C., Pritchard, S. G., Brzostek, E. R., McCormack, M. L., Phillips, R. P. (2015). The rhizosphere and hyphosphere differ in their impacts on carbon and nitrogen cycling in forests exposed to elevated CO2. New Phytol. 205, 1164–1174. doi: 10.1111/nph.13122
Nelson, D. W., Sommers, L. E. (1973). Determination of total nitrogen in plant material 1. Agron. J. 65, 109–112. doi: 10.2134/agronj1973.00021962006500010033x
Olanrewaju, O. S., Ayangbenro, A. S., Glick, B. R., Babalola, O. O. (2019). Plant health: feedback effect of root exudates-rhizobiome interactions. Appl. Microbiol. Biotechnol. 103, 1155–1166. doi: 10.1007/s00253-018-9556-6
Olsen, S. R., Cole, C. V., Watanabe, F. S., Dean, L. A. (1954). Estimation of available phosphorus in soils by extraction with sodium bicarbonate. USDA Circ. 939, 1–19.
Parkinson, J. A., Allen, S. E. (1975). A wet oxidation procedure suitable for the determination of nitrogen and mineral nutrients in biological material. Commun. Soil Sci. Plant Anal. 6, 1–11. doi: 10.1080/00103627509366539
Philippot, L., Raaijmakers, J. M., Lemanceau, P., van der Putten, W. H. (2013). Going back to the roots: the microbial ecology of the rhizosphere. Nat. Rev. Microbiol. 11, 789–799. doi: 10.1038/nrmicro3109
Phillips, R. P., Bernhardt, E. S., Schlesinger, W. H. (2009). Elevated CO2 increases root exudation from loblolly pine (Pinus taeda) seedlings as an N-mediated response. Tree Physiol. 29, 1513–1523. doi: 10.1093/treephys/tpp083
Phillips, R. P., Fahey, T. J. (2006). Tree species and mycorrhizal associations influence the magnitude of rhizosphere effects. Ecology 87, 1302–1313. doi: 10.1890/0012-9658(2006)87[1302:Tsamai]2.0.CO;2
Phillips, R. P., Fahey, T. J. (2008). The influence of soil fertility on rhizosphere effects in Northern hardwood forest soils. Soil Sci. Soc. Amer J. 72, 453–461. doi: 10.2136/sssaj2006.0389
Phillips, R. P., Finzi, A. C., Bernhardt, E. S. (2011). Enhanced root exudation induces microbial feedbacks to N cycling in a pine forest under long-term CO 2 fumigation. Ecol. Lett. 14, 187–194. doi: 10.1111/j.1461-0248.2010.01570.x
Pretzsch, H. (2022). Mixing degree, stand density, and water supply can increase the overyielding of mixed versus monospecific stands in Central Europe. For. Ecol. Manage. 503, 119741. doi: 10.1016/j.foreco.2021.119741
R Core Team (2022). R: A language and environment for statistical computing (Vienna, Austria: R Foundation for Statistical Computing).
Ren, C., Zhao, F., Kang, D., Yang, G., Han, X., Tong, X., et al. (2016). Linkages of C:N:P stoichiometry and bacterial community in soil following afforestation of former farmland. For. Ecol. Manage. 376, 59–66. doi: 10.1016/j.foreco.2016.06.004
Ryan, M. G., Binkley, D., Fownes, J. H., Giardina, C. P., Senock, R. S. (2004). An experimental test of the causes of forest growth decline with stand age. Ecol. Monogr. 74, 393–414. doi: 10.1890/03-4037
Sardans, J., Grau, O., Chen, H. Y. H., Janssens, I. A., Ciais, P., Piao, S., et al. (2017). Changes in nutrient concentrations of leaves and roots in response to global change factors. Global Change Biol. 23, 3849–3856. doi: 10.1111/gcb.13721
Shi, L., Li, Q., Fu, X., Kou, L., Dai, X., Wang, H. (2021). Foliar, root and rhizospheric soil C:N:P stoichiometries of overstory and understory species in subtropical plantations. CATENA 198, 105020. doi: 10.1016/j.catena.2020.105020
Sinsabaugh, R. L., Follstad Shah, J. J. (2011). Ecoenzymatic stoichiometry of recalcitrant organic matter decomposition: the growth rate hypothesis in reverse. Biogeochemistry 102, 31–43. doi: 10.1007/s10533-010-9482-x
Sinsabaugh, R. L., Follstad Shah, J. J. (2012). Ecoenzymatic stoichiometry and ecological theory. Annu. Rev. Ecol. Evol. Syst. 43, 313–343. doi: 10.1146/annurev-ecolsys-071112-124414
Sinsabaugh, R. L., Hill, B. H., Follstad Shah, J. J. (2009). Ecoenzymatic stoichiometry of microbial organic nutrient acquisition in soil and sediment. Nature 462, 795–798. doi: 10.1038/nature08632
Solomon, W., Janda, T., Molnár, Z. (2024). Unveiling the significance of rhizosphere: Implications for plant growth, stress response, and sustainable agriculture. Plant Physiol. Biochem. 206, 108290. doi: 10.1016/j.plaphy.2023.108290
Song, Y., Zhai, J., Zhang, J., Qiao, L., Wang, G., Ma, L., et al. (2021). Forest management practices of Pinus tabulaeformis plantations alter soil organic carbon stability by adjusting microbial characteristics on the Loess Plateau of China. Sci. Total Environ. 766, 144209. doi: 10.1016/j.scitotenv.2020.144209
Song, Z., Hou, J. (2020). Provenance differences in functional traits and N: P stoichiometry of the leaves and roots of Pinus tabulaeformis seedlings under N addition. Global Ecol. Conserv. 21, e00826. doi: 10.1016/j.gecco.2019.e00826
Su, L., Du, H., Zeng, F., Peng, W., Rizwan, M., Núñez-Delgado, A., et al. (2019). Soil and fine roots ecological stoichiometry in different vegetation restoration stages in a karst area, southwest China. J. Environ. Manage. 252, 109694. doi: 10.1016/j.jenvman.2019.109694
Sun, L., Tsujii, Y., Xu, T., Han, M., Li, R., Han, Y., et al. (2023). Species of fast bulk-soil nutrient cycling have lower rhizosphere effects: A nutrient spectrum of rhizosphere effects. Ecology 104, e3981. doi: 10.1002/ecy.3981
Sun, Y., Xu, X.-L., Kuzyakov, Y. (2014). Mechanisms of rhizosphere priming effects and their ecological significance. Chin. J. Plant Ecol. 38, 62–75. doi: 10.3724/SP.J.1258.2014.00007
Sun, Z.-C., Ma, T.-Y., Xu, S.-Q., Guo, H.-R., Hu, C.-C., Chen, C.-J., et al. (2022). Levels and variations of soil bioavailable nitrogen among forests under high atmospheric nitrogen deposition. Sci. Total Environ. 838, 156405. doi: 10.1016/j.scitotenv.2022.156405
Taniguchi, T., Yuzawa, T., HuiPing, M., Yamamoto, F., Yamanaka, N. (2021). Plantation soil inoculation combined with straw checkerboard barriers enhances ectomycorrhizal colonization and subsequent growth of nursery grown Pinus tabulaeformis seedlings in a dryland. Ecol. Eng. 163, 106191. doi: 10.1016/j.ecoleng.2021.106191
Terrer, C., Vicca, S., Hungate, B. A., Phillips, R. P., Prentice, I. C. (2016). Mycorrhizal association as a primary control of the CO 2 fertilization effect. Science 353, 72–74. doi: 10.1126/science.aaf4610
Vives-Peris, V., De Ollas, C., Gómez-Cadenas, A., Pérez-Clemente, R. M. (2020). Root exudates: from plant to rhizosphere and beyond. Plant Cell Rep. 39, 3–17. doi: 10.1007/s00299-019-02447-5
Wang, Z., Lv, S., Song, H., Wang, M., Zhao, Q., Huang, H., et al. (2020). Plant type dominates fine-root C:N:P stoichiometry across China: A meta-analysis. J. Biogeography 47, 1019–1029. doi: 10.1111/jbi.13791
Xu, H., Qu, Q., Li, G., Liu, G., Geissen, V., Ritsema, C. J., et al. (2022). Impact of nitrogen addition on plant-soil-enzyme C–N–P stoichiometry and microbial nutrient limitation. Soil Biol. Biochem. 170, 108714. doi: 10.1016/j.soilbio.2022.108714
Yan, X., Duan, S., Zheng, R., Liu, L., Yue, G., Zhang, Y., et al. (2023). Effects of soil nitrogen concentration on carbon,nitrogen and phosphorus stoichiometry and homeostasis of new branches and leaves in natural Pinus tabulaeformis Forest. J. Soil Water Conserv. 37 (02), 294–300. doi: 10.13870/j.cnki.stbcxb.2023.02.034
Zhang, J., Ai, Z., Liang, C., Wang, G., Liu, G., Xue, S. (2019). How microbes cope with short-term N addition in a Pinus tabuliformis forest-ecological stoichiometry. Geoderma 337, 630–640. doi: 10.1016/j.geoderma.2018.10.017
Zhang, L., Liu, J., Xi, J., Pang, R., Gunina, A., Zhou, S. (2024). Competition for nitrogen between plants and microorganisms in grasslands: effect of nitrogen application rate and plant acquisition strategy. Biol. Fertil Soils. 60, 227–236. doi: 10.1007/s00374-023-01782-w
Zhang, P., Lü, X.-T., Li, M.-H., Wu, T., Jin, G. (2022). N limitation increases along a temperate forest succession: evidences from leaf stoichiometry and nutrient resorption. J. Plant Ecol. 15, 1021–1035. doi: 10.1093/jpe/rtac017
Zhang, Y., Liu, T., Guo, J., Tan, Z., Dong, W., Wang, H. (2021). Changes in the understory diversity of secondary Pinus tabulaeformis forests are the result of stand density and soil properties. Global Ecol. Conserv. 28, e01628. doi: 10.1016/j.gecco.2021.e01628
Zhao, X., Tian, P., Liu, S., Sun, Z., Zeng, Z., Wang, Q. (2022). Cunninghamia lanceolata and understory ferns had positive rhizosphere effects on the temperature sensitivity of soil microbial respiration in a subtropical forest. Geoderma 408, 115593. doi: 10.1016/j.geoderma.2021.115593
Zhou, W., Li, C., Zhao, W., Stringer, L. C., Fu, B. (2024). Spatial distributions of soil nutrients affected by land use, topography and their interactions, in the Loess Plateau of China. Int. Soil Water Conserv. Res. 12, 227–239. doi: 10.1016/j.iswcr.2023.02.005
Zhou, Z., Wang, C., Jiang, L., Luo, Y. (2017). Trends in soil microbial communities during secondary succession. Soil Biol. Biochem. 115, 92–99. doi: 10.1016/j.soilbio.2017.08.014
Zhu, L., Sun, J., Yao, X., Wang, X., Huang, J., Xiong, D., et al. (2022). Fine root nutrient foraging ability in relation to carbon availability along a chronosequence of Chinese fir plantations. For. Ecol. Manage. 507, 120003. doi: 10.1016/j.foreco.2021.120003
Keywords: rhizosphere effects, N:P stoichiometry, N limitation, stand ages, Pinus tabuliformis
Citation: Duan S, Guo J, Zhang Y, Liu L, Wang R and Zheng R (2024) Rhizosphere effects and microbial N limitations drive the root N limitations in the rhizosphere during secondary succession in a Pinus tabuliformis forest in North China. Front. Plant Sci. 15:1392934. doi: 10.3389/fpls.2024.1392934
Received: 28 February 2024; Accepted: 27 June 2024;
Published: 30 July 2024.
Edited by:
Chuntao Yin, United States Department of Agriculture, United StatesReviewed by:
Busayo Joshua Babalola, University of Georgia, United StatesCopyright © 2024 Duan, Guo, Zhang, Liu, Wang and Zheng. This is an open-access article distributed under the terms of the Creative Commons Attribution License (CC BY). The use, distribution or reproduction in other forums is permitted, provided the original author(s) and the copyright owner(s) are credited and that the original publication in this journal is cited, in accordance with accepted academic practice. No use, distribution or reproduction is permitted which does not comply with these terms.
*Correspondence: Jinping Guo, Z3VvamlucGluZ0BzeGF1LmVkdS5jbg==
Disclaimer: All claims expressed in this article are solely those of the authors and do not necessarily represent those of their affiliated organizations, or those of the publisher, the editors and the reviewers. Any product that may be evaluated in this article or claim that may be made by its manufacturer is not guaranteed or endorsed by the publisher.
Research integrity at Frontiers
Learn more about the work of our research integrity team to safeguard the quality of each article we publish.