- 1College of Landscape Architecture, Zhejiang A & F University, Hangzhou, China
- 2Wenzhou Key laboratory of Resource Plant Innovation and Utilization, Institute of Subtropical Crops, Zhejiang Academy of Agricultural Sciences, Wenzhou, China
Clematis is the queen of the vines, being an ornamental plant with high economic value. Waterlogging stress reduces the ornamental value of the plant and limits its application. Melatonin plays an important role in plant resistance to abiotic stresses. In this study, the physiological responses and gene expression levels of two wild species, namely, Clematis tientaiensis and Clematis lanuginosa, and two horticultural varieties, namely, ‘Sen-No-Kaze’ and ‘Viva Polonia,’ under waterlogging stress were analyzed to determine the effect of melatonin on waterlogging tolerance. The results showed that the waterlogging tolerances of C. lanuginosa and ‘Sen-No-Kaze’ were relatively poor, but were significantly improved by concentrations of 100 μmol·L-1 and 50 μmol·L-1 melatonin. C. tientaiensis and ‘Viva Polonia’ had relatively strong tolerance to waterlogging, and this was significantly improved by 200 μmol·L-1 melatonin. Under waterlogging stress, the relative conductivity and H2O2 content of Clematis increased significantly; the photosynthetic parameters and chlorophyll contents were significantly decreased; photosynthesis was inhibited; the contents of soluble protein and soluble sugars were decreased. Effective improvement of waterlogging tolerance after exogenous melatonin spraying, the relative conductivity was decreased by 4.05%-27.44%; the H2O2 content was decreased by 3.84%-23.28%; the chlorophyll content was increased by 35.59%-103.36%; the photosynthetic efficiency was increased by 25.42%-45.86%; the antioxidant enzyme activities of APX, POD, SOD, and CAT were increased by 28.03%-158.61%; the contents of proline, soluble protein, and soluble sugars were enhanced, and cell homeostasis was improved. Transcription sequencing was performed on wild Clematis with differences in waterlogging tolerance, and nine transcription factors were selected that were highly correlated with melatonin and that had the potential to improve waterlogging tolerance, among which LBD4, and MYB4 were significantly positively correlated with the antioxidant enzyme system, and bHLH36, DOF36, and WRKY4 were significantly negatively correlated. Photosynthetic capacity was positively correlated with DOF36 and WRKY4 while being significantly negatively correlated with MYB4, MOF1, DOF47, REV1 and ABR1. Melatonin could enhance the flooding tolerance of Clematis by improving photosynthetic efficiency and antioxidant enzyme activity. This study provides an important basis and reference for the application of melatonin in waterlogging-resistant breeding of Clematis.
1 Introduction
Water is one of the key factors affecting plant growth and development as well as interactions with the environment. In recent years due to global warming, continuous destruction of the environment, and frequent occurrence of extreme weather events, the main causes of waterlogging stress have included extreme precipitation in a short period, poor soil drainage, and heavy soil structure (Fukao et al., 2019). Waterlogging affects crop production on about 10% of global arable land and has become one of the major natural disasters threatening the development of the agricultural industry (Tian et al., 2021; Liu et al., 2023). In southern China, especially in the middle and lower reaches of the Yangtze River, waterlogging disasters often occur due to excessive rainfall, high groundwater levels, and poor soil drainage. This has serious impacts on the external morphology, physiology, and biochemistry of plants (Xia and Chen, 2021; Jiang et al., 2022).
Clematis are mostly woody or grassy vines with attractive colors, a rich diversity of flower types, and a long flowering period, attributes that give the plants high ornamental and medicinal value. The plants are called the “Queen of Vines” and are widely used in landscaping and ecological restoration (Hu et al., 2021). Clematis is resistant to water and humidity. Long-term exposure to soils with saturated or near-saturated water will cause root rot, leaf wilting, and yellowness, inhibition of plant growth, the decline of ornamental quality, and plant death in severe cases (Rabiza-Świder et al., 2017). More rain in the plum rain season in southeastern coastal areas of China coincides with the flowering period of most Clematis, seriously affecting large-scale production and gardening applications (Jiang et al., 2010; Zhu et al., 2024). Therefore, analyzing the waterlogging tolerance of Clematis and establishing waterlogging-resistant varieties are key to breeding Clematis with high stress tolerance.
In waterlogging, the soil moisture content exceeds the field capacity, and the soil pores are filled with water, which inhibits and reduces the utilization rate of O2 by plant cells. As a result, the oxygen supply to the plant root cells is insufficient, and cellular aerobic respiration changes to anaerobic respiration, resulting in the accumulation of respiratory products, such as ethanol, acetaldehyde, and lactic acid, and resulting in the death of root cells. The number of rotten and blackened roots increases (Kurokawa et al., 2018; Hu et al., 2022). The impaired functions of the underground parts, such as water and nutrient absorption, indirectly lead to changes in the functions of the above-ground parts, including stomatal closure, chlorophyll degradation, and cell structure damage, thereby inhibiting gas exchange and photosynthetic efficiency of the leaves and seriously reducing the supply of plant energy materials (Peng et al., 2017; Zeng et al., 2021). The state of high O2 content and low CO2 content in photosynthetic tissues promotes the consumption of energy resources by enhanced photorespiration of plants, further hindering the accumulation of photosynthetic products and the absorption and transport of nutrients and aggravating the secondary stress responses of the plants (Tong et al., 2021; Zhong et al., 2023).
Under waterlogging stress, the dynamic balance of reactive oxygen species (ROS) is disturbed, resulting in metabolic disorders and electron leakage in vivo and accumulation of ROS, such as superoxide anions (O2−), hydroxyl radicals (OH−), hydrogen peroxide (H2O2), and singlet oxygen (1O2) (Singh et al., 2016). ROS accumulation has serious toxic effects on plant cells, and plants maintain ROS metabolic balance by initiating antioxidant and non-enzymatic antioxidant protection mechanisms (Bailey-Serres and Mittler, 2006; Mittler, 2017). Antioxidant enzymes include superoxide dismutase (SOD), peroxidase (POD), catalase (CAT), and ascorbate peroxidase (APX) (Wang et al., 2022a). SOD clears O2− to form H2O2 and O2; POD and CAT catalyze H2O2 to form H2O and O2, and the three enzymes work synergistically to protect plants from damage (Bela et al., 2015; Zhang et al., 2023a). Non-enzymatic antioxidant protective substances include soluble protein, soluble sugar, and proline, which can synergically enhance the activity of related enzymes or act as substrates for related enzymes to improve the survival ability of plants under abiotic stress (Pardo-Hernández et al., 2020; Wang et al., 2022).
Melatonin (MT), also known as pineal hormone, is an endogenous small-molecule substance that widely exists in animals and plants. It can easily cross the cell membrane and enter the subcellular compartment, and it has a wide range of physiological effects in animals and plants (Sun et al., 2021; Anitha et al., 2022; Eisa et al., 2023). Melatonin is present in more than 20 dicotyledonous and monocotyledonous plants (e.g., Allium cepa, Oryza sativa, Solanum lycopersicum, Musa nana, Malus pumila, and Cucumis sativus) and occurs in roots, stems, leaves, fruits, flowers, and seeds (Nawaz et al., 2016; Wei et al., 2018). Melatonin plays an important role in plant growth and development. Melatonin treatment improved the germination rates of Limonium bicolor and Cucumis melo L. seeds under salt stress by promoting soluble sugar utilization and the synthesis of new proteins and by increasing amylase and α-amylase activities, respectively (Castañares and Bouzo, 2019; Li et al., 2019).
Melatonin can alleviate chlorophyll degradation, maintain chlorophyll activity, and improve the plant photosynthetic rate in Arabidopsis thaliana, O. sativa, Triticum aestivum, and other plants under normal and abiotic stress conditions (Back, 2021; Talaat, 2021; Yang et al., 2021; Cao et al., 2023). Exogenous melatonin can significantly inhibit the decreases in chlorophyll degradation and photosynthetic rate under salt stress, balance the electron transfer in the donor, acceptor, and reaction center of the PSII system, and thus effectively alleviate the damage from salt stress on the cucumber photosynthetic system (Wang et al., 2016; Jahan et al., 2021). Exogenous application of melatonin can increase the expression of anthocyanin-related genes in tea leaves under arsenic stress, promote the production and accumulation of anthocyanins, and thus alleviate the effects of arsenic stress on the photosystem of tea trees (Li et al., 2021; Langaroudi et al., 2023).
Melatonin is also a powerful free radical scavenger and oxidizing agent that plays a direct role in scavenging free radicals, such as ROS and reactive nitrogen species (RNS), and maintaining the redox balance of plants (Tiwari et al., 2021; Ahmad et al., 2023). Melatonin can induce and enhance the activity of various antioxidant enzymes, thereby improving its efficiency as an antioxidant (Debnath et al., 2019), and its activity is five times that of glutathione (GSH) and 15 times that of mannitol (Bidabadi et al., 2020; NaveenKumar et al., 2020). Melatonin can increase the resistance of wheat to low-temperature stress (Turk et al., 2014; Kumar et al., 2021; Wu et al., 2022). Application of optimum rate of melatonin under high-temperature stress increased the activities of APX and POD by 43.7% and 45.5%, respectively, which had a positive effect on the growth of cherry radish (Jia et al., 2020).
In Prunus persica treated with 200 µmol· L-1 MT, the activities of superoxide dismutase and peroxidase were increased, while the contents of H2O2 and ethylene were decreased, indicating that melatonin has an antioxidant effect on waterlogging stress (Gu et al., 2021). Exogenous melatonin enhanced the resistance of pear and Solanum lycopersicum to waterlogging as well as to heavy metals and other stresses by increasing the activity of antioxidant enzymes and reducing the contents of hydrogen peroxide and malondialdehyde (Hasan et al., 2019; Liu et al., 2019) Melatonin also reduced low-salt-induced K+ efflux in O. sativa and reduced the sensitivity of plasma membrane K+ permeable channels to hydroxyl radicals (Liu et al., 2020).
There are about 355 species of Clematis germplasm resources in the world, and 147 species of wild Clematis in China. There are 33 species of wild Clematis plants in Zhejiang Province, among which Clematis tientaiensis is a unique wild germplasm resource, and Clematis lanuginosa is an important breeding parent. A previous study found that C. lanuginosa had relatively strong heat resistance, and cultivation showed that C. tientaiensis had strong adaptability and resistance to insect pests (Qian et al., 2022). The flower diameter of both species is more than 15 cm, giving the plants high ornamental and breeding value. In this study, two wild germplasm resources with excellent resistance, namely, C. tientaiensis and C. lanuginosa, as well as the horticultural varieties ‘Sen-No-Kaze’ and ‘Viva Polonia,’ were selected as materials, and exogenous melatonin was used to analyze the physiological responses of the photosynthetic and antioxidant systems of plants under waterlogging stress. The study provides an empirical basis and a reference for exploring the application of breeding new varieties of Clematis with high stress resistance.
2 Materials and methods
2.1 Plant materials and growing conditions
C. tientaiensis, C. lanuginosa, ‘Sen-No-Kaze’, and ‘Viva Polonia’ were stored in the National Clematis germplasm Resource Bank of Zhejiang Institute of Subtropical Crops. Three-year-old healthy Clematis plants with consistent growth, and free of pests and diseases were selected and planted in individual pots. The basin diameter was 11 cm, and the basin height was 18 cm. The planting medium contained peat, coconut husk, perlite, and light stone in the ratio 55:15:15:15. The test site was a greenhouse located at E 120°38 '30.99"; and N 28°0' 16.87". The area has a subtropical marine monsoon climate with four distinct seasons and abundant rainfall, with an annual average rainfall of 1700 mm and a frost-free period of 276 days. The average temperature was 31.4 ± 5.0°C. and the relative humidity was 68.0% ± 19.0%.
2.2 Waterlogging stress treatment
The double-set basin method was used to carry out flooding stress, and the test design is shown in Table 1. The control group (CK) was subjected to normal water management and was watered from 16:00–17:00 every afternoon to maintain the soil water content between 50%–60% until the end of the experiment. For the waterlogging treatment (T0), potted Clematis plants were placed in plastic boxes (42 cm long × 31.5 cm wide × 22.5 cm high), keeping the water level 4–5 cm above the soil surface. The medium was replenished regularly, and the water in the plastic box was changed once every seven days for 28 days. Exogenous spraying of melatonin (T1, T2, and T3) was calculated as the first day of stress on the day of waterlogging. The spraying was started at about 17:00 using a fine droplet sprayer and was evenly distributed on the upper and lower surfaces of the leaves, assuming that the surface and back of the leaves were uniformly wet and dripping, and the spraying lasted for seven days. The waterlogging treatment used distilled water instead of exogenous melatonin, and the control group underwent normal water management during spraying. The phenotypes of Clematis plants were recorded on the 29th day after the waterlogging treatment. All the healthy and intact leaves were collected and frozen in liquid nitrogen and stored at –80°C for physiological index detection and transcriptome sequencing. Each Clematis variety was set up with five potted plants in each treatment for technical replication, with a total of 100 plants in four varieties. The experiment was repeated three times.
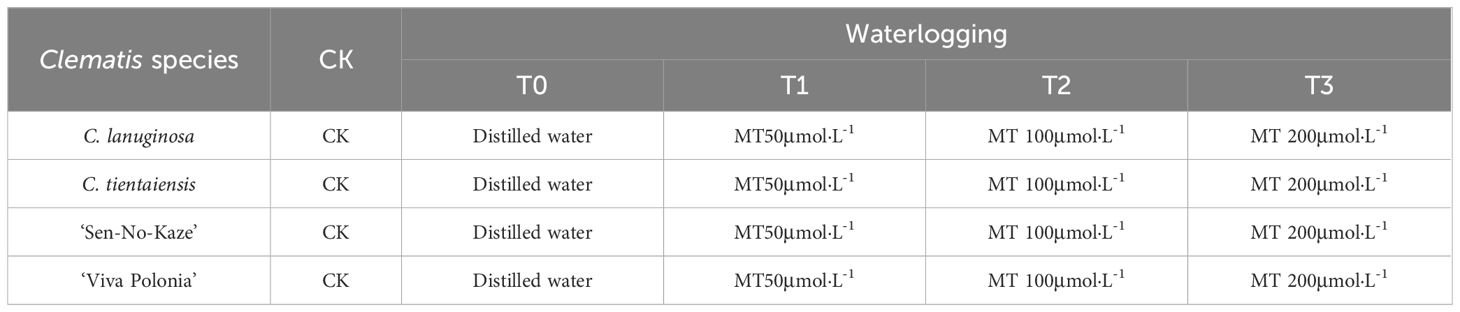
Table 1 Experimental design of effects of different concentrations of exogenous melatonin on Clematis under waterlogging stress.
2.3 Leaf gas exchange parameters and chlorophyll content
Healthy and fully developed leaves were randomly chosen for photosynthetic parameter measurements using an LI-6400 XT portable photosynthesis system (Li-Cor Inc., Lincoln, NE, USA), and equipped with a 6400–18 RGB LED light source. Five leaves were determined for each plant, and five plants were determined for each treatment. The measurements were carried out from 9:00 to 11:00 am. The photosynthetic photon flux density was 1200 μmol m−2s−1; the CO2 concentration was 450 ppm, and the relative humidity was 65%.
The leaves of Clematis were washed and dried quickly, and the midvein was cut off. A mixed sample of 0.1 g chopped leaves was weighed and placed in a 10 ml test tube with 8 ml of 80% acetone. Chlorophyll was extracted at 4°C in the dark. The absorbance of the supernatant was measured at 663, 645, and 470 nm using a spectrophotometer (Shimadzu UV-2550, Kyoto, Japan). The total chlorophyll content was calculated according to the Arnon formula (Palta, 1990).
2.4 H2O2 content and relative conductivity
The H2O2 content was measured according to the method previously described (Ma et al., 2017).
Samples of leaves (0.1 g) were weighed, and 10 ml of distilled water was added. The leaves were soaked for 1 h after vacuuming, and then tested for the conductivity (C1). The tubes with the leaf samples were placed in a boiling water bath at 100°C for 10 min, cooled to room temperature, and tested again for conductivity (C2). The relative conductivity was calculated as C1/C2 (Zhai et al., 2016).
2.5 Proline, soluble sugar, and soluble protein contents
The proline content was determined using 0.5 g of fresh leaves and 5 ml of 3% sulfamesalicylic acid homogenate, centrifuged at 4000 × g for 10 min, and the filtrate (2 ml) was mixed with indanhydrin (2 ml) and acetic acid (2 ml) in a test tube. The reaction mixture was incubated in a water bath at 98°C for 30 minutes and then extracted with toluene (5 ml). Toluene with chromophores was aspirated, cooled to room temperature, and the absorbance was measured at 520 nm (Bates et al., 1973).
A soluble sugar test kit (Nanjing Jiancheng Bioengineering Institute, Jiangsu, China) was used for determination. For the extraction, 0.2 g leaf samples were ground with 2 ml of distilled water until evenly mixed, heated in a water bath at 98°C for 10 min, centrifuged at room temperature at 4000 r/min after cooling for 10 min, and the supernatant was collected. The substrate was added with concentrated sulfuric acid, and the reaction was carried out in a boiling water bath at 98°C for 10 min. After cooling to room temperature, the absorbance was measured at 620 nm to calculate the soluble sugar content (Zhao et al., 2019).
Determination of soluble protein content in leaves was performed according to the Coomassie brilliant blue (CBB) G250 method described by Read (Read and Northcote, 1981). After adding 5 ml of CBB G-250 reagent to 0.1 ml of enzyme solution, the soluble protein content was determined at 595 nm colorimetric wavelength after standing for 2 min.
2.6 Antioxidant enzyme activity
To determine antioxidant enzyme activity, 0.3 g of fresh leaf tissue was homogenized with 8 ml of phosphate buffer (0.1 M, pH 6.8). The mixed liquid sample was centrifuged at 12,000 × g at 4°C for 20 minutes. The obtained supernatant was used to determine the activities of SOD, POD, CAT, and APX. The activity of SOD was determined according to the instructions of a total superoxide dismutase (T-SOD) detection kit (Nanjing Jiancheng Bioengineering Institute, Jiangsu, China). One unit of SOD activity is defined as the amount of enzyme required to inhibit 50% of the reduction rate monitored at 550 nm. The activity of APX was determined using the APX detection kit (Nanjing Jiancheng Bioengineering Institute, Jiangsu, China). APX activity was determined based on the absorbance variation of 290 nm (A10s and A130s). CAT and POD activities were determined by CAT assay kit and POD assay kit (Nanjing Jiancheng Bioengineering Institute, Jiangsu, China), respectively.
2.7 Transcriptome analysis
RNA was extracted from C. tientaiensis and C. lanuginosa with Trizol reagent (Takara, Beijing, China). Total RNA was extracted from tissue samples, and the concentration and purity of the extracted RNA were detected by using a Nanodrop 2000 (Thermo Scientific, Waltham, MA, USA). RNA integrity was detected by agarose gel electrophoresis, and RIN values were determined using an Agilent 5300 analyzer (Agilent Technologies, Palo Alto, CA, USA). After the fragmentation buffer was added, the mRNA was randomly fragmented, and the fragments of about 300 bp were separated by magnetic bead screening. A cDNA library was synthesized by reverse transcription. Double-ended sequencing was performed by second-generation sequencing (Illumina Novaseq 6000, San Diego, CA, USA), and sequencing was performed by Majorbio Biomedical Technology Co., Ltd. (Shanghai, China).
Trinity software (r20140717) was used to splice clean reads to obtain the transcripts for subsequent analysis. NR, Swiss-Prot, eggnog, and KEGG databases (www.kegg.jp/kegg/) were used to annotate all of the unigenes (E value < 1.0 e−5). Gene expression was analyzed by FPKM (Fragments Per Kilobase of exon model per Million mapped reads). The criteria for screening DEGs were a P-value ≤ 0.05, a false discovery rate (FDR) < 0.001, and a fold difference ≥ 2 or a fold difference ≤ 0.5 (Winfield et al., 2010; MacWilliams et al., 2023).
Using the FPKM values of differentially expressed genes obtained by RNA-seq sequencing, filtering unannotated differentially expressed genes and five genes with the sum of treated expression amounts less than 500, combined with the determined relevant physiological indicators, weighted correlation network analysis (WGCNA) was performed to construct a correlation matrix between phenotypes and gene modules (Azam et al., 2023; Wang et al., 2023).
Total RNA was extracted from leaves, and cDNA was synthesized with a reverse transcription kit (Thermo Scientific, Waltham, MA, USA). RT-PCR was performed using an ABI PRISM 7500 real-time PCR system (Applied Biosystems, Foster City, CA, USA) and AceQ qPCR SYBR Green Master Mix (Vazyme, Nanjing, Jiangsu Province, China). The RT-PCR reaction system was as follows: 5 min at 95°C, 40 cycles at 95°C for 15 s, and 30 cycles at 60°C. Each sample was repeated three times, and the reference gene was GAPDH. The specificity of each pair of primers was verified by a melting curve analysis, and the gene expression levels were calculated by the 2−△△Ct method.
2.8 Statistical analysis
SPSS22.0 statistical software was used to conduct two-way ANOVA for all of the data, and all of the charts were produced by GraphPad Prism 9.5.1 and SigmaPlot 12.5 software. Different letters on the histograms between different treatments indicate a significant difference at P ≤ 0.05.
3 Results
3.1 Exogenous melatonin improved the growth of Clematis after waterlogging stress
C. tientaiensis, C. lanuginosa, ‘Sen-No-Kaze’, and ‘Viva Polonia’ showed different degrees of leaf wilting, drooping, yellowing, and drying under waterlogging stress. C. lanuginosa and ‘Sen-No-Kaze’ were the most sensitive; most of the leaves withered, drooped, and rotted, while C. tientaiensis and ‘Viva Polonia’ were relatively better, where only a few leaves turned brown and withered. More than 60% of the leaves remained green. The growth of the four species of Clematis was improved after exogenous melatonin spraying. Under waterlogging stress, C. lanuginosa grew best under T2, while some leaves wilted and yellowed under T3. C. tientaiensis and ‘Viva Polonia’ showed good growth under melatonin treatments, and T3 produced the best growth. ‘Sen-No-Kaze’ grew best under T1, and wilting and yellowing occurred with T3 (Figure 1).
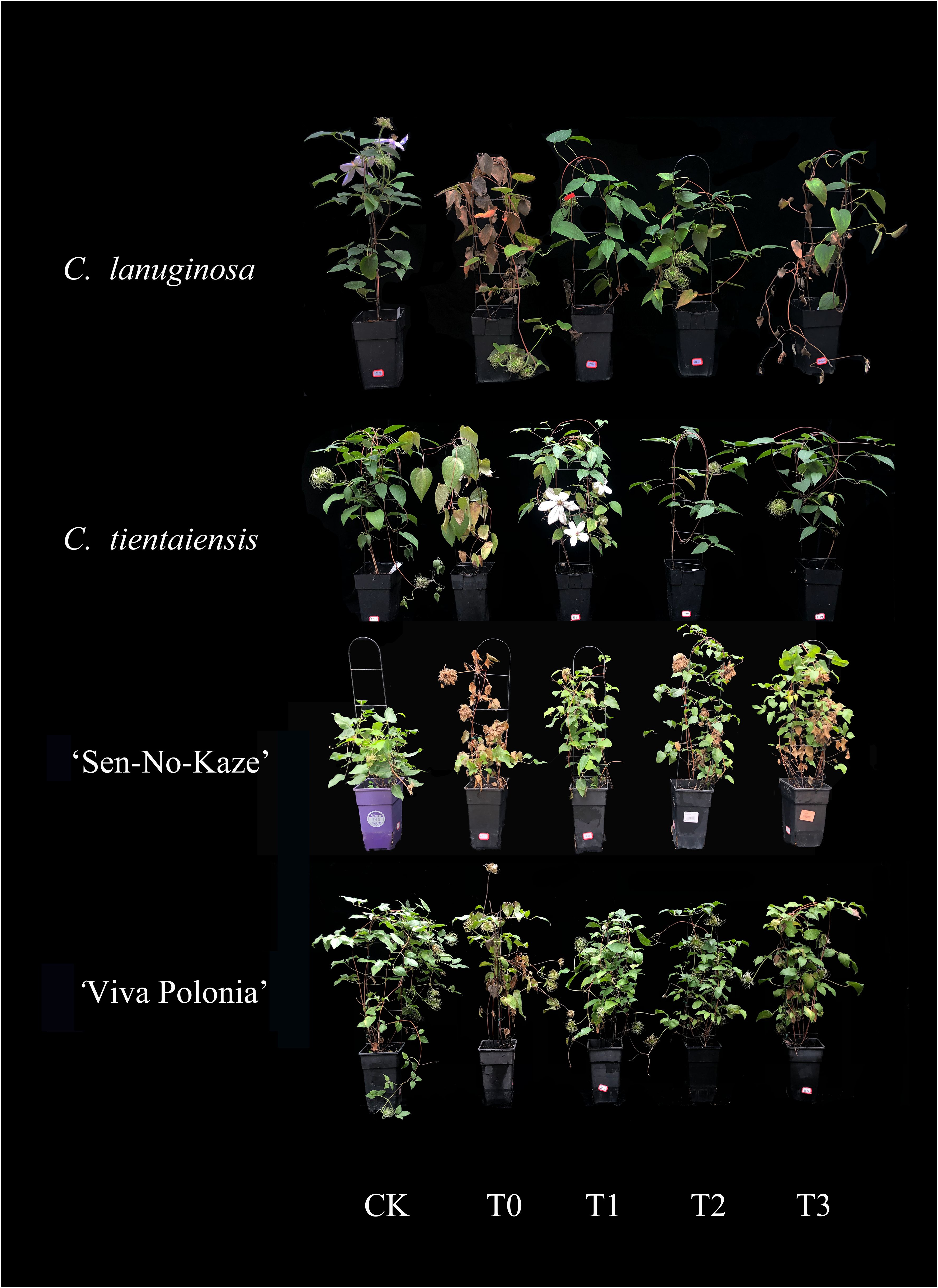
Figure 1 Plant growth of Clematis under waterlogging stress treated with different concentrations of exogenous melatonin.
Waterlogging stress significantly increased the relative electrical conductivity (REC) of Clematis leaves, and after exogenous melatonin spraying, the REC of the leaves of the four Clematis leaves decreased significantly, among which the relative electrical conductivity of C. lanuginosa under T2 decreased significantly by 28.51%, and that of C. tientaiensis under T3 decreased significantly by 26.99%. ‘Sen-No-Kaze’ decreased significantly under T1 by 30.75%. ‘Viva Polonia’ had a significant 32.66% decrease under T3 (Figure 2A). After waterlogging stress, the REC of C. tientaiensis was significantly lower than that of the other three Clematis, and the REC of C. lanuginosa under the T1 treatment was the highest, while the REC of C. tientaiensis and ‘Viva Polonia’ under the T3 treatment was significantly lower than those of C. lanuginosa and ‘Sen-No-Kaze’(Figure 2B).
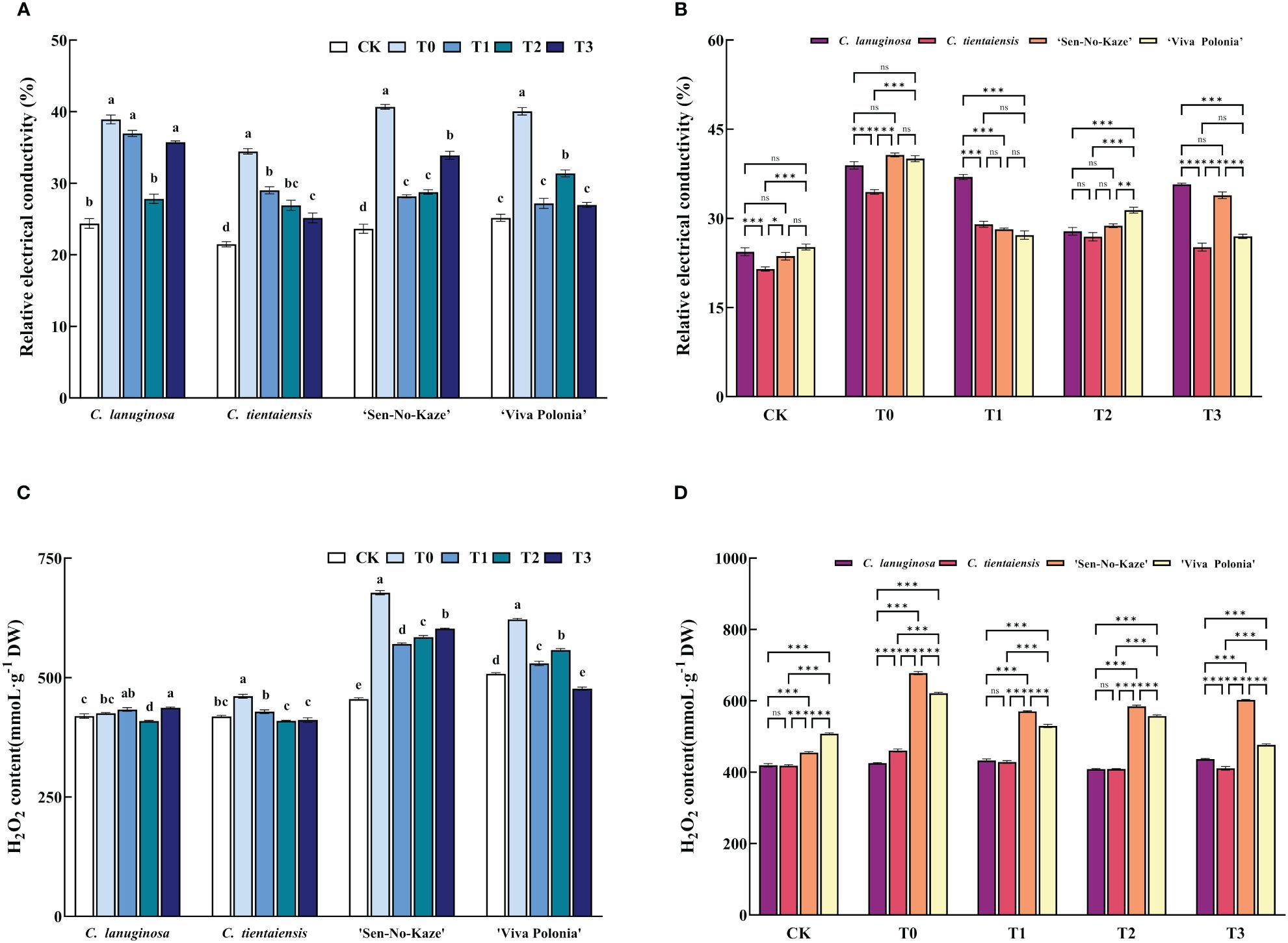
Figure 2 The effects of waterlogging stress and different concentrations of exogenous melatonin on REC and H2O2 contents of Clematis. (A, B) Relative electrical conductivity; (C, D) H2O2 contents. Error bars indicate SE (n = 5 plants). Different letters indicate significant differences based on two-way ANOVA followed by Tukey’s multiple comparison test (P ≤ 0.05). *means a significant difference at the P ≤ 0.05 level; **means a significant difference at the P ≤ 0.01 level, ***means a significant difference at the P ≤ 0.001 level, and ns means no significant difference at the P ≤ 0.05 level.
Waterlogging stress also increased the H2O2 content of Clematis. After the addition of exogenous melatonin, the H2O2 content of C. lanuginosa was significantly decreased under T2 (3.84%), and those of C. tientaiensis and ‘Viva Polonia’ were significantly decreased under T3 (10.82% and 23.28%). ‘Sen-No-Kaze’ decreased significantly in T1 by 15.83% (Figure 2C). For the different Clematis species, the H2O2 contents of ‘Viva Polonia’ and ‘Sen-No-Kaze’ were significantly higher than those of C. lanuginosa and C. tientaiensis. With the increase of the melatonin concentration, the H2O2 content in ‘Viva Polonia’ was similar to those in C. lanuginosa and C. tientaiensis, but the H2O2 content in ‘Sen-No-Kaze’ was significantly higher than that in the other species of Clematis (Figure 2D).
3.2 Exogenous melatonin enhances photosynthesis of Clematis under waterlogging stress
Under waterlogging stress, the contents of Chla, Chlb, Car, and total chlorophyll in the leaves of the four Clematis were significantly decreased, and the pigment content was increased by melatonin, but there were differences in the responses to different concentrations. Under the T2 treatment, the contents of Chla, Chlb, Car, and total chlorophyll increased the most compared with waterlogging stress in C. lanuginosa and were significantly increased, by 67.32%, 62.96%, 59.99%, and 66.00%, respectively. Under the T3 treatment, the contents of Chla, Chlb, Car, and total Chlorophyll increased the most in C. tientaiensis, by 38.14%, 31.56%, 29.88%, and 36.93%, respectively, and in ‘Viva Polonia’, the respective values were 35.10%, 36.95%, 34.97%, and 35.59%. Under the T1 treatment, the contents of Chla, Chlb, Car, and total Chlorophyll increased the most in ‘Sen-No-Kaze’, by 83.58%, 93.05%, 102.65%, and 103.36%, respectively (Figure 3).
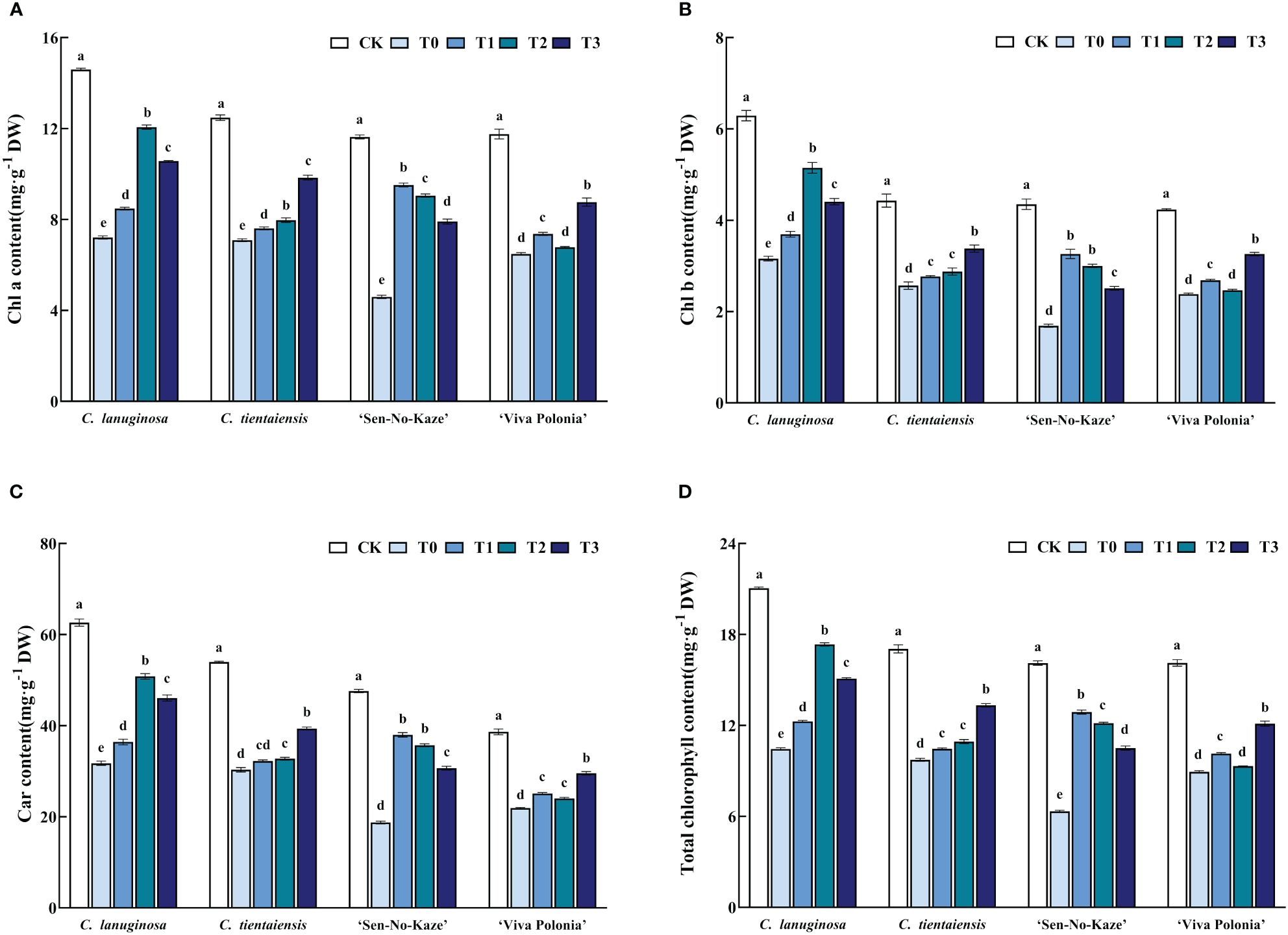
Figure 3 Effects of waterlogging stress and different concentrations of exogenous melatonin on the pigment of Clematis leaves. (A) Chla content; (B) Chlb content; (C) Car content; (D) Total chlorophyll content. Error bars indicate SE (n = 5 plants). Different letters indicate significant differences based on two-way ANOVA followed by Tukey’s multiple comparison test (P ≤ 0.05).
Waterlogging stress and different concentrations of exogenous melatonin significantly decreased the net photosynthetic rate (Pn), stomatal conductance (Gs), intercellular CO2 concentration (Ci), and transpiration rate (Tr) of Clematis, and the addition of exogenous melatonin significantly increased photosynthetic gas exchange parameters. Compared to T0, the values of Pn, Gs, Ci, and Tr were increased by 25.42%, 63.22%, 18.47%, and 102.80%, respectively, in the T1 treatment of C. lanuginosa. Under T3 conditions, the values of Pn, Gs, Ci, and Tr were significantly increased by 29.57%, 45.86%, 62.77%, and 62.93%, respectively, in C. tientaiensis, and by 18.19%, 52.38%, 95.36%, and 132.44% in ‘Viva Polonia’, respectively. In ‘Sen-No-Kaze’, Pn, Gs, Ci, and Tr were increased by 44.25%, 75.64%, 52.11%, and 139.01% under T1 conditions (Figure 4).
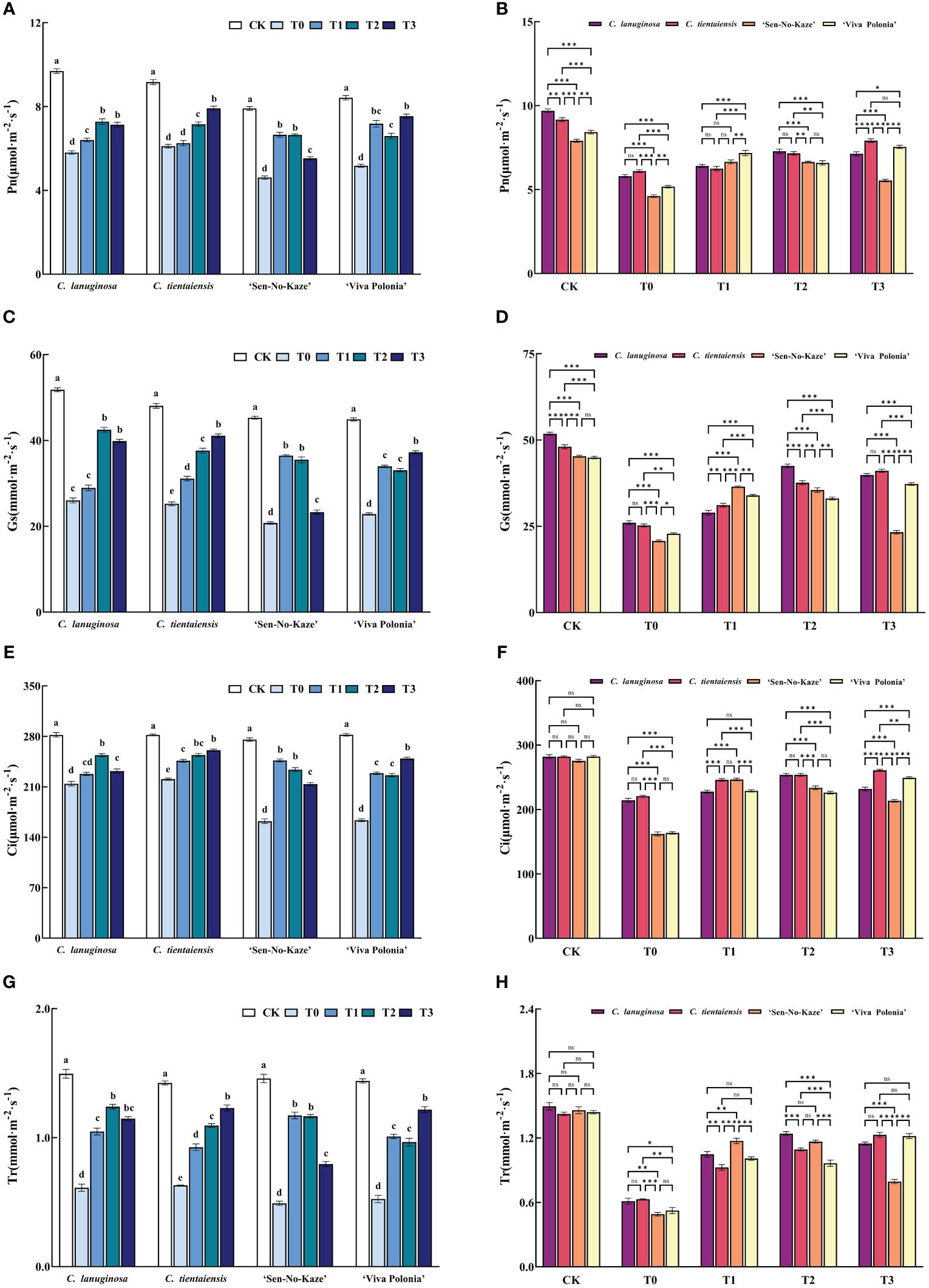
Figure 4 Effects of waterlogging stress and different concentrations of exogenous melatonin on photosynthetic parameters of Clematis. (A, B) Net photosynthetic rate (Pn); (C, D) Stomatal conductance (Gs); (E, F) Intercellular CO2 concentration (Ci); (G, H) Transpiration rate (TR). Error bars indicate SE (n = 5 plants). Different letters indicate significant differences based on two-way ANOVA followed by Tukey’s multiple comparison test (P ≤0.05). *means a significant difference at the P ≤ 0.05 level. **means a significant difference at the P ≤ 0.01 level. ***means a significant difference at the P ≤ 0.001 level, and ns means no significant difference at the P ≤ 0.05 level.
3.3 Exogenous melatonin increased antioxidant enzyme activity of Clematis under waterlogging stress
Waterlogging stress increased the activities of APX, POD, SOD, and CAT in Clematis plants, and exogenous melatonin increased the antioxidant activity of Clematis under waterlogging stress. Under the T2 treatment of C. lanuginosa, the activity of antioxidant enzymes increased the most; APX increased by 58.22%; POD increased by 141.91%; SOD increased by 43.90%, and CAT increased by 111.45%. C. tientaiensis and ‘Viva Polonia’ had the highest antioxidant enzyme activities under T3; APX increased by 59.14% and 35.89%, POD increased by 117.23% and 59.85%; SOD increased by 33.21% and 28.03%, and CAT increased by 54.65% and 70.64%, respectively. Under the T1 treatment, the activities of antioxidant enzymes in ‘Sen-No-Kaze’ increased the most, with APX being increased by 158.61%, POD increased by 123.91%, SOD increased by 62.99% and CAT increased by 141.72% (Figure 5).
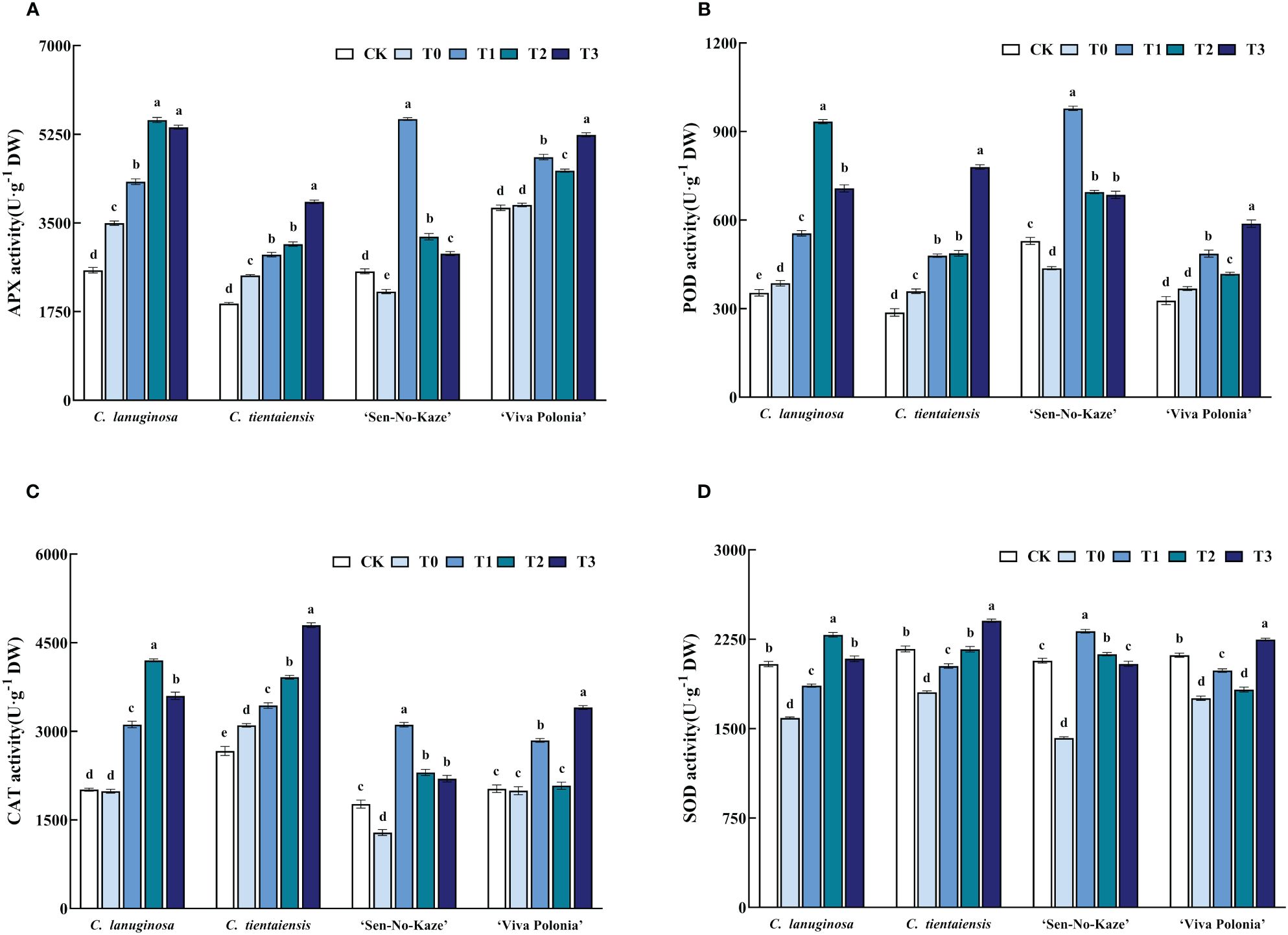
Figure 5 Effects of waterlogging stress and different concentrations of exogenous melatonin on antioxidant enzyme activity of Clematis. (A) APX activity; (B) POD activity; (C) CAT activity; (D) SOD activity. Error bars indicate SE (n = 5 plants). Different letters indicate significant differences based on two-way ANOVA followed by Tukey’s multiple comparison test (P ≤ 0.05).
3.4 Exogenous melatonin increased the content of osmoregulatory substances in Clematis under waterlogging stress
Waterlogging stress significantly increased the proline content in Clematis, and after exogenous melatonin spraying, the proline content was also significantly increased compared to waterlogging stress. For the optimal concentration of melatonin, C. lanuginosa significantly increased the proline content by 92.01%, and C. tientaiensis significantly increased proline by 68.35%. ‘Sen-No-Kaze’ showed a significant increase of 131.86%, and ‘Viva Polonia’ showed a significant increase of 52.98% (Figure 6A).
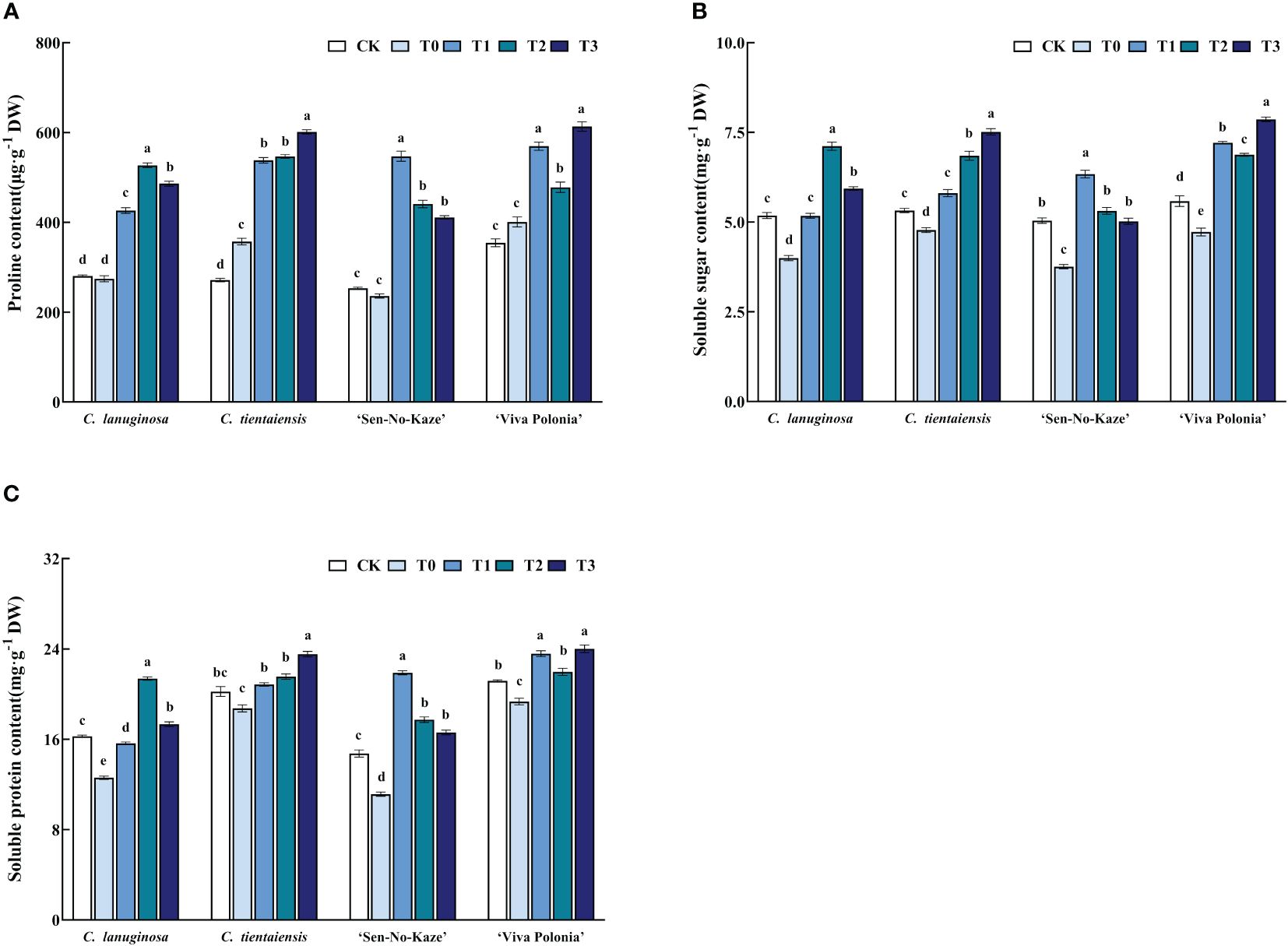
Figure 6 Effects of waterlogging stress and different concentrations of exogenous melatonin on the content of osmoregulatory substances in Clematis. (A) Proline content; (B) Soluble sugar content; (C) Soluble protein content. Values are the means ± standard error (n = 5 plants). Different letters indicate significant differences based on two-way ANOVA followed by Tukey’s multiple comparison test (P ≤ 0.05).
Soluble sugar content was significantly increased after melatonin spraying. In C. lanuginosa, soluble sugar was significantly increased by 77.97%; in C. tientaiensis by 57.21%, in ‘Sen-No-Kaze’ by 68.64%, and in ‘Viva Polonia’ by 66.35% (Figure 6B). Waterlogging stress and exogenous melatonin spraying also increased the soluble protein content in Clematis leaves, by 69.83%, 25.67%, 96.67%, and 24.14%, respectively (Figure 6C).
3.5 Transcriptome analysis of exogenous melatonin supplementation under waterlogging stress
C. lanuginosa and C. tientaiensis were the original breeding parents of Clematis, and the two had large differences in waterlogging tolerance. Therefore, transcriptome analysis was conducted for C. lanuginosa and C. tientaiensis. A total of 199,498 transcriptomes were assembled, comprising 239,096,966 bp of transcript data, of which 129,833 Unigenes were assembled from 153,185,608 bp (Supplementary Tables S1, S2). In C. lanuginosa, 478 Unigenes were up-regulated, and 2625 were down-regulated under waterlogging stress. For the melatonin treatments T1, T2, and T3, 2356, 2084, and 244 Unigenes were up-regulated, and 18148, 13592, and 329 Unigenes were down-regulated, respectively (Figure 7A). In C. tientaiensis, 2961 Unigenes were up-regulated, and 2889 were down-regulated under waterlogging stress; 1112, 1454, and 809 Unigenes were up-regulated, and 748, 1391, and 1007 Unigenes were down-regulated under the T1, T2, and T3 treatments, respectively (Figure 7B).
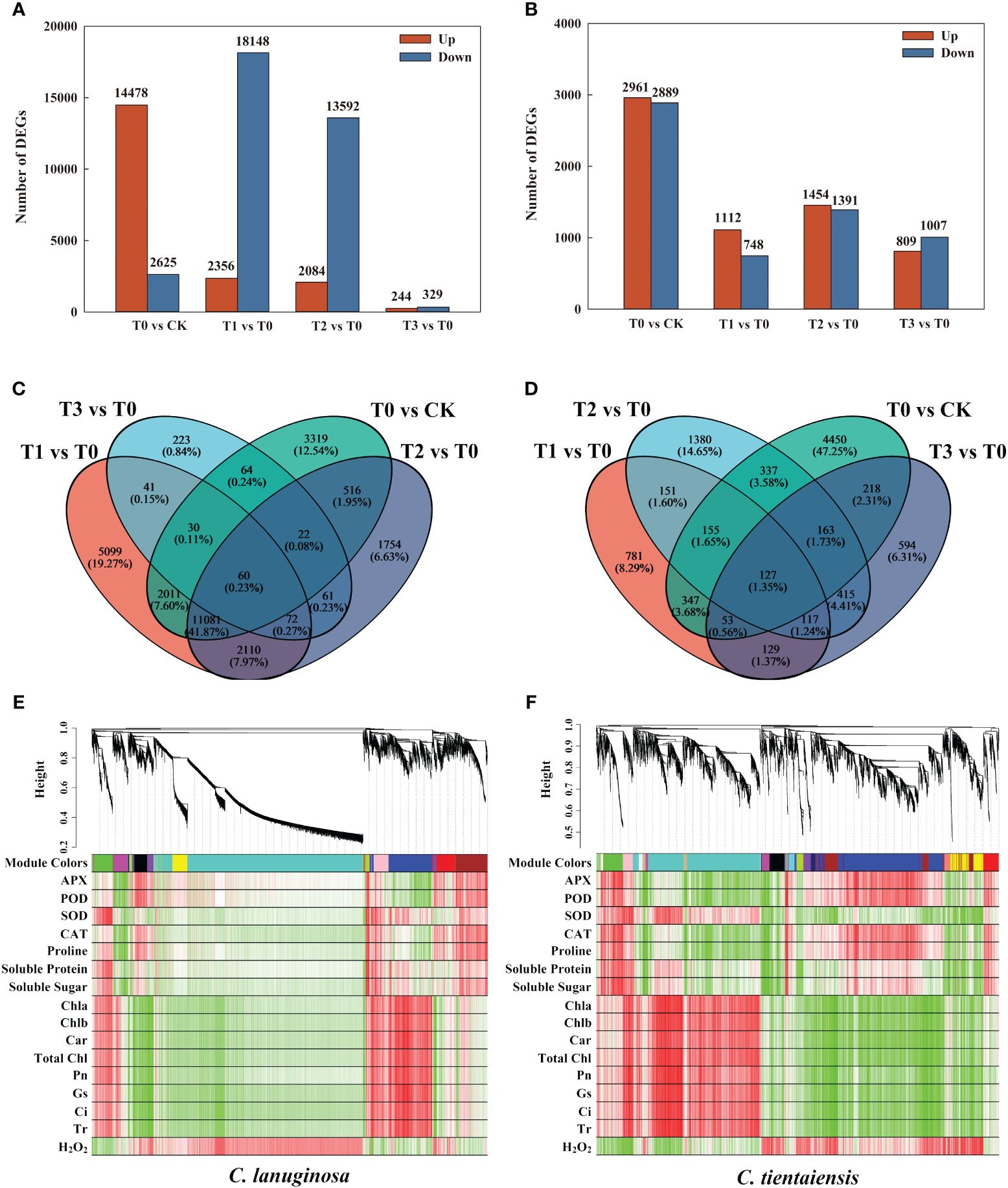
Figure 7 Transcriptome differential genes and association analysis under waterlogging stress and exogenous melatonin treatments. (A) The number of DEGs for C. lanuginosa; (B) The number of DEGs for C. tientaiensis; (C) Venn diagrams for DEGs in the four comparison groups of C. lanuginosa; (D) Venn diagrams for DEGs in the four comparison groups of C. tientaiensis; (E) WGCNA analysis of C. lanuginosa; (F) WGCNA analysis of C. tientaiensis.
A total of 60 genes were differentially expressed (DEGs) in all of the treatments of C. lanuginosa; 132 genes (0.50%) were differentially expressed in the melatonin treatment, and 5099 DEGs (19.27%) were specifically expressed in T1 (Figure 7C). In C. tientaiensis, a total of 127 genes (1.35%) were differentially expressed in all of the treatments; there were 244 DEGs (2.59%) in the melatonin treatment, and 781 DEGs (8.29%) in the T1 treatment (Figure 7D). GO analysis revealed that the up-regulated DEGs were annotated in the processes of heterocyclic compound binding, organic cyclic compound binding, and ion binding after the addition of melatonin to C. lanuginosa and C. tientaiensis under waterlogging stress (Supplementary Figures S4A, B). The down-regulated DEGs were annotated in the processes of organic substance metabolic process, primary metabolic process, and organic cyclic compound binding (Supplementary Figures S4C, D).
To determine the correlation between phenotype and gene expression and to identify key waterlogging tolerance candidate genes, a WGCNA analysis showed that the DEGs and physiological indicators in C. lanuginosa were clustered into 20 modules, among which 45 grey60 modules composed of DEGs were significantly positively correlated with antioxidant enzyme activity and soluble sugar, and 142 magenta modules composed of DEGs were significantly negatively correlated. Pink modules containing 144 DEGs were positively correlated with leaf pigment content, photosynthetic capacity, and negatively correlated with H2O2 content (Figure 7E). The DEGs and physiological indexes of C. tientaiensis were grouped into 12 modules.
Antioxidant enzyme activity and soluble sugar were significantly negatively correlated with pink modules (77 DEGs), while the black modules (82 DEGs) were significantly positively correlated with leaf pigment content, photosynthetic system parameters, and soluble protein, and negatively correlated with H2O2 (Figure 7F).
3.6 Screening of transcription factors for improving waterlogging tolerance of Clematis by melatonin
The transcription factors in the association analysis were screened. Under waterlogging stress and melatonin treatment, 23 transcription factors belonging to 12 gene families were differentially expressed in C. lanuginosa. These included five MYB, three bZIP, three C2H2, two FAR1, two ERF, two C2C2, one bHLH, one WRKY, one MADS, one HSF, one LOB, and one B3. There were 14 transcription factors in C. tientaiensis, five MYB, two C2H2, two ERF, one FAR1, one bHLH, one WRKY, one LOB, and one B3. There were 14 differentially expressed transcription factors in both C. lanuginosa and C. tientaiensis, and nine were differentially expressed in C. lanuginosa (Supplementary Table S3).
Transcription factors in significantly correlated modules were further identified. In C. lanuginosa, LBD4, MYB4, and DREB1b were significantly positively correlated with antioxidant enzyme activity; bHLH36 was significantly negatively correlated with antioxidant enzyme activity and soluble sugar content, and the transcription factors that were significantly positively correlated with photosynthetic system parameters were MYB5, DOF36 and WRKY4. The negative correlations involved MOF1, DOF47, REV1 and ABR1 (Figures 8A, B). In C. tientaiensis, LBD4, REV1, FRS5, antioxidant oxidase activity, and soluble sugar content were significantly positively correlated; DOF36 and WRKY4 was significantly negatively correlated, and photosynthetic system parameters were significantly negatively correlated with MYB4, MOF1, and DOF47 (Figures 8C, D). To further verify the expression patterns of waterlogging tolerance candidate genes under waterlogging stress, RT-qPCR was performed, and the quantitative results were consistent with the expression trends of RNA-seq gene expression data.
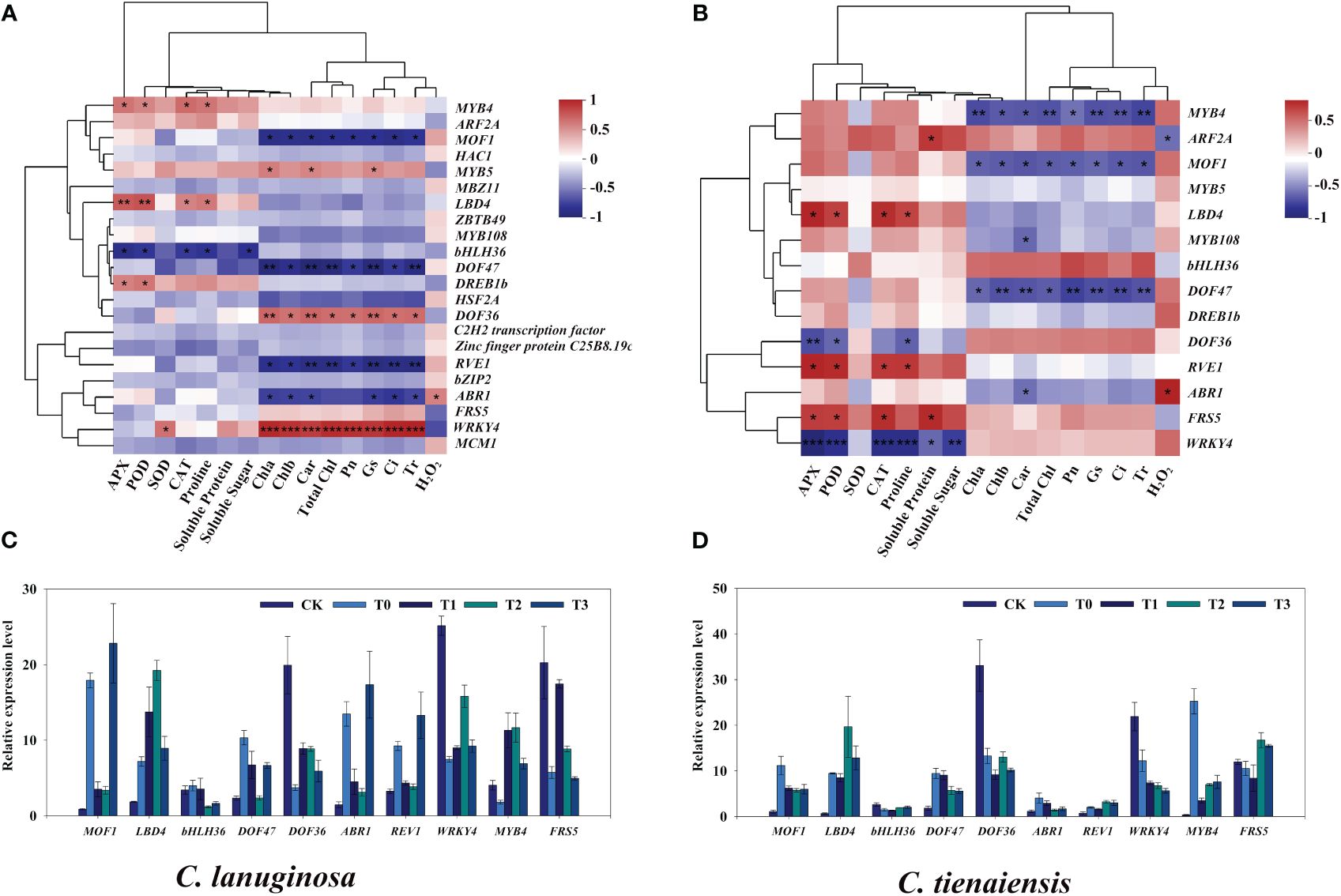
Figure 8 Screening of transcription factors related to waterlogging tolerance of Clematis. (A) Correlation analysis of different expressed transcription factors and physiological indexes of C. lanuginosa; (B) Correlation analysis of different expressed transcription factors and physiological indexes of C. tientaiensis; (C) Transcription factor expression in C. lanuginosa; (D) Transcription factor expression in C. tientaiensis. *means a significant difference at the P ≤ 0.05 level. **means a significant difference at the P ≤ 0.01 level. ***means a significant difference at the P ≤ 0.001 level.
4 Discussion
Water is one of the key factors affecting plant growth and development, and leaves are the organs whose external morphology is most sensitive to water stress. Long-term exposure of plants to soil environments saturated with water or near saturation will cause leaf wilting, yellowing, and loss (Ashraf, 2012). To explore the effects of waterlogging stress on the growth and ornamental value of Clematis, four varieties in the flowering stage were subjected to waterlogging stress treatment. The results showed that the flowers of the plants were wilted and drooped after waterlogging stress, and C. lanuginosa, C. tientaiensis, and ‘Viva Polonia’ all maintained the ability to produce seeds, but some seeds were green and sterile. Studies have shown that melatonin plays a role in reproductive protection during flowering, and its content is highest in young flower organs or during flower development (Wang et al., 2022d). In Datura metel L., the highest levels of melatonin were found in the least-developed buds, and the concentration of melatonin decreased as the buds matured. When exposed to low-temperature stress, the content of melatonin in young flowers increased significantly, indicating that melatonin was involved in the protection of new reproductive organs (Murch et al., 2009). After exogenous melatonin spraying, C. tientaiensis had strong waterlogging tolerance, was able to flower, and could maintain normal growth, while the seeds of ‘Viva Polonia’ recovered the green color, indicating that melatonin may also play a role in protection of reproductive structures of Clematis under waterlogging stress and in maintaining normal reproductive growth.
Under normal conditions, the four types of Clematis showed differences in Pn and Gs, but no significant differences in Tr or Ci (Figures 4B, D, F, H). There were significant differences in leaf pigment content (Supplementary Figure S1), possibly due to differences in physiological characteristics among different species and varieties of Clematis. Waterlogging stress leads to hypoxia, stomatal conductance decrease, stomatal closure, leaf wilting, and decreased water evaporation. With the continuous waterlogging stress, the chlorophyll content decreases; leaves undergo premature aging, photosynthetic parameters (Pn, Gs, Ci, and Tr) are decreased, and the rate of photosynthesis decreases. Under waterlogging stress, the Chlb and total Chlorophyll content in the leaves of C. lanuginosa was significantly higher than in C. tientaiensis, the contents of Chla and Car were not significantly different (Supplementary Figure S1), and there were no significant differences in photosynthetic gas exchange parameters (Figure 4B), indicating that the photosynthetic system of C. tientaiensis may be more efficient than that of C. lanuginosa, or Clematis mainly absorbs and uses red light for photosynthesis. The specific differences still need to be further studied.
Melatonin can inhibit the degradation of chlorophyll under abiotic stress, improve the photosynthetic rate of leaves by enhancing the activity of Rubisco, and promote the accumulation of plant dry matter (Yang et al., 2018). Melatonin can decrease the expression of the key gene of chlorophyll degradation, pheophorbide A oxygenase (PAO), and increase the expression of the key photosynthetic gene RBCS, thereby improving the photosynthetic efficiency of plants under drought stress (Cherono et al., 2021). Exogenous foliar spraying of melatonin increased the chlorophyll content and the maximum mass seed yield (Fv/Fm) of photosystem II photochemistry of Sorghum bicolor seedlings, thereby increasing the rate of photosynthesis (Zhang et al., 2022). The net photosynthetic rate of waterlogged Medicago sativa pretreated with melatonin significantly increased (Zhang et al., 2019). Melatonin maintained the chlorophyll content in Clematis under waterlogging stress and improved the photosynthetic capacity. The Pn of the four types of Clematis under the T1 treatment had the least difference (Figure 4B). Under waterlogging stress, the Ci values of ‘Sen-No-Kaze’ and ‘Viva Polonia’ were significantly lower than those of C. lanuginosa and C. tientaiensis, and melatonin spraying narrowed the gap between the varieties (Figure 4F). Different Clematis showed variation in melatonin concentration. C. tientaiensis and ‘Viva Polonia’ had relatively strong waterlogging tolerance, and the chlorophyll content and photosynthetic capacity were significantly improved under the T3 treatment, while the low melatonin concentration did not result in a significant effect. C. lanuginosa and ‘Sen-No-Kaze’ were improved by the medium and low melatonin concentrations (Figures 3; 4). The results indicated that suitable addition of melatonin could improve the photosynthetic efficiency of Clematis to provide the energy needed for growth under waterlogging stress.
When plants grow under normal conditions, ROS activity is maintained. Under waterlogging stress, the original dynamic balance of ROS in plants is broken, resulting in membrane lipid peroxidation, increased membrane permeability, and leakage of plant cellular contents. Eventually, root rot, leaf wilting, and other forms of plant damage occur (Pan et al., 2021; Peláez-Vico et al., 2023). Plants can clear ROS through both enzymatic and non-enzymatic systems. Enzyme systems include SOD, CAT, POD, APX, glutathione peroxidase (GPX), glutathione reductase (GR), dehydroascorbate reductase (DHAR), and monodehydroascorbate reductase (MDHAR). Non-enzymatic systems eliminate ROS through the circulation of Ascorbate (AsA) and Glutathione (GSH) (Hossain et al., 2009; Foyer and Noctor, 2011). SOD, APX, and CAT activities of Musa acuminata, Citrus reticulata, and Chrysanthemum morifolium in leaves under waterlogging stress were higher than those of controls (Teoh et al., 2022; Li et al., 2023). The APX, POD, CAT, and SOD activities of Clematis were higher than those of the control group under waterlogging stress consistent with results from other species.
Melatonin can regulate the activity of antioxidant enzymes, remove free radicals, improve the redox state of cells, reduce ROS and RNS levels, and stabilize biofilms (Li et al., 2015). In Malus pumila, external application of melatonin reduced the physiological damage to the plant by ROS, thereby improving its stain resistance (Zheng et al., 2017). Exogenous melatonin effectively increased the activities of SOD, POD, and APX in Zea mays, Prunus persica, and Sorghum bicolor under waterlogging stress, and improved plant tolerance (Gu et al., 2021; Ahmad et al., 2022). In this study, exogenous melatonin improved the activities of APX, POD, SOD, and CAT in Clematis under waterlogging stress. By increasing the ability to remove ROS, the level of H2O2 was reduced, and the value of REC was decreased (Figure 2), and reduced the effect of waterlogging stress on the cell membrane system and enzyme function, indicating that oxidative damage was alleviated. Melatonin can inhibit the accumulation of ROS in Clematis under waterlogging stress to a certain extent, reduce the degree of membrane lipid peroxidation, reduce the damage to cell membranes, and alleviate the damage from waterlogging.
In this study, Clematis varieties with different waterlogging stress tolerance had different responses in antioxidant enzyme activity. The APX and SOD activity of ‘Sen-No-Kaze’ increased most significantly after melatonin spraying (Supplementary Figure S2), and the POD activity of ‘Sen-No-Kaze’ was higher than that of the other three types under normal culture conditions, waterlogging stress, and low melatonin concentration. With the increase in the melatonin concentration, the POD enzyme activity of ‘Sen-No-Kaze’ in the T2 and T3 treatments increased less than those of C. lanuginosa and C. tientaiensis. The POD activity of C. lanuginosa increased most significantly after melatonin spraying (Supplementary Figure S2B). The CAT antioxidant oxidase activity levels of C. lanuginosa and C. tientaiensis were higher than those of the other two varieties under waterlogging stress and melatonin treatment (Supplementary Figures S2C, D). The antioxidant enzyme activity of Clematis with strong waterlogging tolerance increased significantly after the addition of melatonin, while the antioxidant enzyme activity of Clematis with poor waterlogging tolerance was inhibited under the high melatonin concentration compared to the low concentration, a result that may be related to differences in tolerance.
The variety and growth stages of plants may have different effects on the amount of melatonin, and a high concentration of melatonin may inhibit plant growth (Arnao and Hernández-Ruiz, 2018). The leaf pigment content, APX, POD, and other antioxidant enzyme activities of C. lanuginosa under the T3 treatment were higher than those under the T1 treatment, but the relative water content of T3 was lower than that of T1 (Supplementary Figure S5A). This difference resulted in relatively high leaf pigment content, stomatal conductance, net photosynthetic rate, and antioxidant enzyme activity. Leaf pigment content, photosynthetic reaction gas parameters, and antioxidant enzyme activity decreased with the increase of melatonin concentration in ‘Sen-No-Kaze’, C. lanuginosa under T3 displayed partially mature leaf tips with yellowing. The reason for this may be that the high melatonin concentration inhibited the growth and development of Clematis, and the plants did not respond to waterlogging stress or the low melatonin treatment as under the relatively high melatonin concentration. Moreover, the ‘Sen-No-Kaze’ variety was more sensitive to the high melatonin concentration, but the specific differences in response need to be further studied.
Non-enzymatic antioxidant protective substances include proline, soluble protein, and soluble sugar. As the main osmoregulatory substances of plants, they can synergistically improve the activity of related enzymes or act as the substrates of related enzymes while reducing the osmotic potential of cell cytoplasm, promoting the absorption of water from the outside of cells, and regulating cell osmotic pressure to reduce the damage from waterlogging stress. Thus, the survival ability of plants under waterlogging stress can be improved (Wang et al., 2019). In studies of kiwifruit, Capsicum annuum, and other plants, exogenous application of melatonin increased the content of osmoregulatory substances in plants under stress, thus alleviating the damage caused by stress and helping the plants survive (Moustafa-Farag et al., 2020; Huo et al., 2022; Khosravi et al., 2023). Waterlogging stress significantly increased the contents of proline, soluble protein, and soluble sugar in the four Clematis (Figure 6). The levels of osmoregulatory substances in C. tientaiensis were significantly higher than those in C. lanuginosa, and those in ‘Viva Polonia’ were significantly higher than those in ‘Sen-No-Kaze’ Among the four species, the proline content of C. tientaiensis maintained relatively high level after waterlogging stress and melatonin induction (Supplementary Figure S3A). The soluble sugar contents in C. lanuginosa and ‘Sen-No-Kaze’ were decreased by the increase of melatonin concentration (Supplementary Figure S3B), indicating that the proline and soluble protein of Clematis with strong waterlogging tolerance were most significantly responsive to waterlogging stress, and may also improve the efficiency of energy use. Melatonin can be used as an effective plant biostimulant to combat biological and abiotic stresses because it can promote the accumulation of soluble sugar and proline. In this study, after spraying melatonin, the proline content, soluble sugar, and soluble protein content of Clematis were enhanced, thus effectively maintaining cell homeostasis and improving the adaptability of Clematis to waterlogging stress.
Transcription factors (TFs) are key regulators of gene expression, and they play a crucial role in regulating plant responses to various abiotic stresses (He et al., 2023). C. lanuginosa and C. tientaiensis are the original breeding parents of Clematis. In this study, stress resistance genes in wild Clematis were further extracted by transcriptome analysis. Under waterlogging stress and melatonin treatment, 23 and 14 transcription factors were differentially expressed in C. lanuginosa and C. tientaiensis, respectively. The two differentially expressed genes were distributed in MYB, Zinc finger protein, ERF, FAR1, bHLH, WRKY, LOB, and B3 gene families (Figure 8). These differentially expressed transcription factors may be the key to the regulation of waterlogging tolerance of Clematis tiantianensis.
The transcription factor LOB (Lateral organ boundaries domain) has been shown to respond to waterlogging stress. Expression levels of LOB domain (LBD) family genes in Solanum L. and Arabidopsis thaliana increased under waterlogging stress (Dawood et al., 2016; Voesenek et al., 2016). In this study, LBD4 in C. lanuginosa and C. tientaiensis were significantly positively correlated with the antioxidant enzyme system and were significantly up-regulated under waterlogging stress as well as being induced by melatonin, indicating that LBD4 played an important role in improving the antioxidant enzyme activity of Clematis under waterlogging stress.
The AP2/ERF (APETALA2/ethylene-responsive factor) transcription factor family is critical for plant abiotic stress response (Licausi et al., 2013; Wang et al., 2022b). The family contains four subfamilies of AP2, RAV, ERF, and DREB, among which ABR1 was responsive to ABA and stress conditions including cold, high salt, and drought (Pandey et al., 2005). In Arabidopsis, AtABR1 was shoot specifically induced upon submergence and hypoxia, and overexpressing plants showed morphological phenotypes like increased root hair length and number, and directly or interaction with ABF4 promoted starch degradation to resist drought stress (Bäumler et al., 2019; Zhang et al., 2023b). In this study, ABR1 was significantly negatively correlated with the photosynthetic system in C. lanuginosa and C. tientaiensis, and its expression was up-regulated by waterlogging stress induction, and the expression was inhibited by melatonin. These results suggest that ABR1 may play an important role in Clematis waterlogging stress, and that melatonin can regulate the expression of ABR1 to improve the tolerance to waterlogging.
MYB (v-MYB avian myeloblastosis viral oncogene homolog) transcription factor is one of the largest transcription factors in plants, and it plays important roles in the plant cell cycle, environmental response, stress response, primary metabolism, and secondary metabolism (Jung et al., 2008; Dubos et al., 2010; An et al., 2020). Studies have shown that the MYB protein REVEILLE/LHY-CCA1-Like (RVE1/LCL1), which is related to the biological clock, binds to the Evening element (EE) in the DREB1 promoter region, and RVE1/RVE2 plays a negative regulatory role in plant cold tolerance. It can also mediate the expression of the downstream DREB1 gene under cold stress (Kidokoro et al., 2021). In this study, RVE1 was significantly negatively correlated with the photosynthetic system in C. lanuginosa and significantly positively correlated with the antioxidant system in C. tientaiensis, suggesting that RVE1 in Clematis may negatively regulate waterlogging tolerance and mediate the expression of DREB1b. MOF1 is a transcriptional suppressor that can interact with three TOPLESS related proteins, namely, OsTPR1, OsTPR2, and OsTPR3, inhibit the expression of DL and OsMADS6, and thus inhibit flower organogenesis and spikelet meristem development in rice (Li et al., 2020; Ren et al., 2020). The expression of MOF1 was up-regulated under waterlogging stress, and the appropriate concentration of melatonin inhibited the expression of MOF1, which restored the organ development ability of Clematis.
Transposase-derived transcription factors FRS-FRF family proteins play crucial roles in plant growth and development in processes, such as oxidation reactions, starch synthesis, flowering time, flower development, drought stress, low phosphorus response, and ABA response (Ma and Li, 2018). FRS-FRF family proteins are divided into six subgroups according to their protein structure. FRS5 is in the V subfamily (Lin et al., 2007; Li et al., 2011; Tang et al., 2013; Aguilar-Martínez et al., 2015; Liu et al., 2017). Up-regulation of the FRS5 gene in Triticum aestivum increased its tolerance to nitrogen deficiency (Wang et al., 2021). In this study, waterlogging stress inhibited the expression of FRS5 (Figure 8), while melatonin induced up-regulation of FRS5, and this improved the waterlogging tolerance of Clematis.
The plant-specific transcription factor DOF (DNA binding with one finger) protein family is a classic plant-specific transcription factor family belonging to the single zinc finger structural protein superfamily. The proteins are involved in the regulation of different plant processes during growth and development (Gupta et al., 2015; Boccaccini et al., 2016). Overexpression of MdDOF54 in apple (Malus × domestica) would result in a higher photosynthetic rate and stronger water transport capacity under long-term drought conditions than that of the wild type, which greatly increased the survival rate under short-term drought conditions (Chen et al., 2020). DOF proteins are also key regulatory centers of various plant hormone pathways, integrating abscisic acid, jasmonic acid, salicylic acid, and redox signaling (Wang et al., 2022c). In this study, the inhibition of DOF36 expression by waterlogging stress was positively correlated with photosynthesis, while the significant upregulation of DOF47 was negatively correlated with photosynthesis, suggesting that DOF36 may positively regulate photosynthesis, similar to MdDOF54, while DOF47 negatively regulates photosynthesis.
WRKYs are the largest and most important family of transcription factors in plants and are primarily involved in responses to various stresses (Rushton et al., 2010; Wang et al., 2021). Recent studies have shown that members of this gene family are involved in hypoxic stress responses in many plant species, including Oryza sativa (Shiono et al., 2014), Helianthus annuus (Raineri et al., 2015), and Diospyros kaki (Zhu et al., 2019). WRKY33 in Arabidopsis thaliana roots was induced after hypoxia treatment, and wrky33 mutants were sensitive to hypoxia stress treatment (Hwang et al., 2011). In this study, WRKY4 in C. lanuginosa was significantly positively correlated with the photosynthetic system, while WRKY4 in C. tientaiensis was significantly negatively correlated with the antioxidant system, suggesting that WRKY4 may play a positive and positive regulating role in the photosynthetic system and negatively regulating the antioxidant system, thereby improving the tolerance to waterlogging in Clematis.
In this study, waterlogging stress damaged the membrane system, the photosynthetic system, and the oxidation balance of Clematis, significantly reducing photosynthetic production. Exogenous melatonin effectively reduced lipid peroxidation of the membrane of flooded Clematis, maintained photosynthetic production capacity, improved antioxidant enzyme activity, and improved the adaptability of Clematis to waterlogging stress. From the perspective of economy and production, 50 μmol·L-1 can enhance the waterlogging tolerance of Clematis to a certain extent to meet the production demand, and nine transcription factors that can improve the waterlogging tolerance of Clematis were identified through transcriptome analysis. The findings thus provide an important basis and reference for the further application of melatonin in the cultivation and resistance breeding of Clematis. In future studies, it will be necessary to explore the regulatory role of these transcription factors on Clematis waterlogging tolerance to further improve our understanding of the underlying mechanisms.
Data availability statement
The raw transcriptome data have been deposited at the NCBI Sequence Read Archive with accession number PRJNA1070854 (https://www.ncbi.nlm.nih.gov/bioproject/PRJNA1070854).
Author contributions
KC: Conceptualization, Data curation, Formal analysis, Writing – original draft. QH: Funding acquisition, Project administration, Resources, Writing – review & editing. XM: Investigation, Methodology, Writing – original draft. XZ: Investigation, Methodology, Writing – original draft. RQ: Writing – review & editing. JZ: Writing – review & editing.
Funding
The author(s) declare financial support was received for the research, authorship, and/or publication of this article. This work was supported by Zhejiang Province Public Welfare Project (LGN20C150001); National Natural Science Foundation of China (32102428); Zhejiang Science and Technology Major Program on Agricultural New Variety Breeding (2021C02071-6); Wenzhou Major Technological Innovation Project (ZS2020002); Zhejiang Province Rare and Endangered Wild Animals and Plants Rescue and Protection Action Project.
Acknowledgments
The authors declare that the research was conducted in the absence of any commercial or financial relationships that could be construed as a potential conflict of interest.
Conflict of interest
The authors declare that the research was conducted in the absence of any commercial or financial relationships that could be construed as a potential conflict of interest.
Publisher’s note
All claims expressed in this article are solely those of the authors and do not necessarily represent those of their affiliated organizations, or those of the publisher, the editors and the reviewers. Any product that may be evaluated in this article, or claim that may be made by its manufacturer, is not guaranteed or endorsed by the publisher.
Supplementary material
The Supplementary Material for this article can be found online at: https://www.frontiersin.org/articles/10.3389/fpls.2024.1385165/full#supplementary-material
Supplementary Figure 1 | Effects of waterlogging stress on the chlorophyll content of Clematis.
Supplementary Figure 2 | Effects of waterlogging stress on antioxidant enzyme activity of Clematis.
Supplementary Figure 3 | Effect of waterlogging stress on proline, soluble sugar, and soluble protein content of Clematis.
Supplementary Figure 4 | GO annotation analysis of DEGs in C. lanuginosa and Clematis.
Supplementary Figure 5 | Effect of waterlogging stress on relative water content of Clematis.
References
Aguilar-Martínez, J. A., Uchida, N., Townsley, B., West, D. A., Yanez, A., Lynn, N., et al. (2015). Transcriptional, posttranscriptional, and posttranslational regulation of SHOOT MERISTEMLESS gene expression in Arabidopsis determines gene function in the shoot apex. Plant Physiol. 167, 424–442. doi: 10.1104/pp.114.248625
Ahmad, N., Naeem, M., Ali, H., Alabbosh, K. F., Hussain, H., Khan, I., et al. (2023). From challenges to solutions: The impact of melatonin on abiotic stress synergies in horticultural plants via redox regulation and epigenetic signaling. Scientia Hortic. 321, 112369. doi: 10.1016/j.scienta.2023.112369
Ahmad, S., Wang, G. Y., Muhammad, I., Zeeshan, M., Zhou, X. B. (2022). Melatonin and KNO3 application improves growth, physiological and biochemical characteristics of maize seedlings under waterlogging stress conditions. Biology 11, 99. doi: 10.3390/biology11010099
An, J. P., Wang, X. F., Zhang, X. W., Xu, H. F., Bi, S. Q., You, C. X., et al. (2020). An apple MYB transcription factor regulates cold tolerance and anthocyanin accumulation and undergoes MIEL1-mediated degradation. Plant Biotechnol. J. 18, 337–353. doi: 10.1111/pbi.13201
Anitha, K., Senthil, A., Kalarani, M. K., Senthil, N., Marimuthu, S., Djanaguiraman, M., et al. (2022). Exogenous melatonin improves seed germination and seedling growth in greengram under drought stress. J. Appl. Natural Sci. 14, 1190–1197. doi: 10.31018/jans.v14i4.3818
Arnao, M. B., Hernández-Ruiz, J. (2018). Melatonin and its relationship to plant hormones. Ann. Bot. 121, 195–207. doi: 10.1093/aob/mcx114
Ashraf, M. A. (2012). Waterlogging stress in plants: A review. Afr. J. Agric. Res. 7, 1976–1981. doi: 10.5897/AJARX11.084
Azam, M., Zhang, S., Li, J., Ahsan, M., Agyenim-Boateng, K. G., Qi, J., et al. (2023). Identification of hub genes regulating isoflavone accumulation in soybean seeds via GWAS and WGCNA approaches. Front. Plant Sci. 14. doi: 10.3389/fpls.2023.1120498
Back, K. (2021). Melatonin metabolism, signaling and possible roles in plants. Plant J. 105, 376–391. doi: 10.1111/tpj.14915
Bailey-Serres, J., Mittler, R. (2006). The roles of reactive oxygen species in plant cells. Plant Physiol. 141, 311–311. doi: 10.1104/pp.104.900191
Bates, L. S., Waldren, R. P. A., Teare, I. D. (1973). Rapid determination of free proline for water-stress studies. Plant Soil 39, 205–207. doi: 10.1007/BF00018060
Bäumler, J., Riber, W., Klecker, M., Müller, L., Dissmeyer, N., Weig, A. R., et al. (2019). At ERF# 111/ABR 1 is a transcriptional activator involved in the wounding response. Plant J. 100, 969–990. doi: 10.1111/tpj.14490
Bela, K., Horváth, E., Gallé, Á., Szabados, L., Tari, I., Csiszár, J. (2015). Plant glutathione peroxidases: emerging role of the antioxidant enzymes in plant development and stress responses. J. Plant Physiol. 176, 192–201. doi: 10.1016/j.jplph.2014.12.014
Bidabadi, S. S., VanderWeide, J., Sabbatini, P. (2020). Exogenous melatonin improves glutathione content, redox state and increases essential oil production in two Salvia species under drought stress. Sci. Rep. 10, 6883. doi: 10.1038/s41598–020-63986–6
Boccaccini, A., Lorrai, R., Ruta, V., Frey, A., Mercey-Boutet, S., Marion-Poll, A., et al. (2016). The DAG1 transcription factor negatively regulates the seed-to-seedling transition in Arabidopsis acting on ABA and GA levels. BMC Plant Biol. 16, 1–11. doi: 10.1186/s12870–016-0890–5
Cao, Y., Du, P., Yin, B., Zhou, S., Li, Z., Zhang, X., et al. (2023). Melatonin and dopamine enhance waterlogging tolerance by modulating ROS scavenging, nitrogen uptake, and the rhizosphere microbial community in Malus hupehensis. Plant Soil 483, 475–493. doi: 10.1007/s11104–022-05759-w
Castañares, J. L., Bouzo, C. A. (2019). Effect of exogenous melatonin on seed germination and seedling growth in melon (Cucumis melo L.) under salt stress. Hortic. Plant J. 5, 79–87. doi: 10.1016/j.hpj.2019.01.002
Chen, P., Yan, M., Li, L., He, J., Zhou, S., Li, Z. (2020). The apple DNA-binding one zinc-finger protein MdDof54 promotes drought resistance. Horticulture Res. 7, 195. doi: 10.1038/s41438–020-00419–5
Cherono, S., Ntini, C., Wassie, M., Mollah, M. D., Belal, M. A., Ogutu, C., et al. (2021). Exogenous application of melatonin improves drought tolerance in coffee by regulating photosynthetic efficiency and oxidative damage. J. Am. Soc. Hortic. Sci. 146, 24–32. doi: 10.21273/JASHS04964–20
Dawood, T., Yang, X., Visser, E. J., Te Beek, T. A., Kensche, P. R., Cristescu, S. M., et al. (2016). A co-opted hormonal cascade activates dormant adventitious root primordia upon flooding in Solanum dulcamara. Plant Physiol. 170, 2351–2364. doi: 10.1104/pp.15.00773
Debnath, B., Islam, W., Li, M., Sun, Y., Lu, X., Mitra, S., et al. (2019). Melatonin mediates enhancement of stress tolerance in plants. Int. J. Mol. Sci. 20 (5), 1040. doi: 10.3390/ijms20051040
Dubos, C., Stracke, R., Grotewold, E., Weisshaar, B., Martin, C., Lepiniec, L. (2010). MYB transcription factors in Arabidopsis. Trends Plant Sci. 15, 573–581. doi: 10.1016/j.tplants.2010.06.005
Eisa, E. A., Honfi, P., Tilly-Mándy, A., Gururani, M. A. (2023). Exogenous application of melatonin alleviates drought stress in ranunculus asiaticus by improving its morphophysiological and biochemical attributes. Horticulturae 9, 262. doi: 10.3390/horticulturae9020262
Foyer, C. H., Noctor, G. (2011). Ascorbate and glutathione: the heart of the redox hub. Plant Physiol. 155, 2–18. doi: 10.1104/pp.110.167569
Fukao, T., Barrera-Figueroa, B. E., Juntawong, P., Peña-Castro, J. M. (2019). Submergence and waterlogging stress in plants: a review highlighting research opportunities and understudied aspects. Front. Plant Sci. 10. doi: 10.3389/fpls.2019.00340
Gu, X., Xue, L., Lu, L., Jiao, J., Song, G., Xie, M., et al. (2021). Melatonin enhances the waterlogging tolerance of Prunus persica by modulating antioxidant metabolism and anaerobic respiration. J. Plant Growth Regul. 40, 2178–2190. doi: 10.1007/s00344–020-10263–5
Gupta, S., Malviya, N., Kushwaha, H., Nasim, J., Bisht, N. C., Singh, V. K., et al. (2015). Insights into structural and functional diversity of Dof (DNA binding with one finger) transcription factor. Planta 241, 549–562. doi: 10.1007/s00425–014-2239–3
Hasan, M. K., Ahammed, G. J., Sun, S., Li, M., Yin, H., Zhou, J. (2019). Melatonin inhibits cadmium translocation and enhances plant tolerance by regulating sulfur uptake and assimilation in Solanum lycopersicum L. J. Agric. Food Chem. 67, 10563–10576. doi: 10.1021/acs.jafc.9b02404
He, H., Yang, M., Li, S., Zhang, G., Ding, Z., Zhang, L., et al. (2023). Mechanisms and biotechnological applications of transcription factors. Synthetic Syst. Biotechnol. 8, 565–577. doi: 10.1016/j.synbio.2023.08.006
Hossain, Z., López-Climent, M. F., Arbona, V., Pérez-Clemente, R. M., Gómez-Cadenas, A. (2009). Modulation of the antioxidant system in citrus under waterlogging and subsequent drainage. J. Plant Physiol. 166, 1391–1404. doi: 10.1016/j.jplph.2009.02.012
Hu, Q., Qian, R., Zhang, Y., Zhang, X., Ma, X., Zheng, J. (2021). Physiological and gene expression changes of Clematis crassifolia and Clematis cadmia in response to heat stress. Front. Plant Sci. 12. doi: 10.3389/fpls.2021.624875
Hu, C. H., Zheng, Y., Tong, C. L., Zhang, D. J. (2022). Effects of exogenous melatonin on plant growth, root hormones and photosynthetic characteristics of trifoliate orange subjected to salt stress. Plant Growth Regul. 97, 551–558. doi: 10.1007/s10725–022-00814-z
Huo, L., Wang, H., Wang, Q., Gao, Y., Xu, K., Sun, X. (2022). Exogenous treatment with melatonin enhances waterlogging tolerance of kiwifruit plants. Front. Plant Sci. 13. doi: 10.3389/fpls.2022.1081787
Hwang, J. H., Lee, M. O., Choy, Y. H., Ha-Lee, Y. M., Hong, C. B., Lee, D. H. (2011). Expression profile analysis of hypoxia responses in Arabidopsis roots and shoots. J. Plant Biol. 54, 373–383. doi: 10.1007/s12374–011-9172–9
Jahan, M. S., Guo, S., Sun, J., Shu, S., Wang, Y., Abou El-Yazied, A., et al. (2021). Melatonin-mediated photosynthetic performance of tomato seedlings under high-temperature stress. Plant Physiol. Biochem. 167, 309–320. doi: 10.1016/j.plaphy.2021.08.002
Jia, C., Yu, X., Zhang, M., Liu, Z., Zou, P., Ma, J., et al. (2020). Application of melatonin-enhanced tolerance to high-temperature stress in cherry radish (Raphanus sativus L. var. radculus pers). J. Plant Growth Regul. 39, 631–640. doi: 10.1007/s00344–019-10006–1
Jiang, Z., Yang, S., Liu, Z., Xu, Y., Xiong, Y., Qi, S., et al. (2022). Coupling machine learning and weather forecast to predict farmland flood disaster: A case study in Yangtze River basin. Environ. Model. Software 155, 105436. doi: 10.1016/j.envsoft.2022.105436
Jiang, N., Yu, W. B., Li, H. Z., Guan, K. Y. (2010). Floral traits, pollination ecology and breeding system of three Clematis species (Ranunculaceae) in Yunnan province, southwestern China. Aust. J. Bot. 58, 115–123. doi: 10.1071/BT09163
Jung, C., Seo, J. S., Han, S. W., Koo, Y. J., Kim, C. H., Song, S. I., et al. (2008). Overexpression of AtMYB44 enhances stomatal closure to confer abiotic stress tolerance in transgenic Arabidopsis. Plant Physiol. 146, 623. doi: 10.1104/pp.107.110981
Khosravi, S., Haghighi, M., Mottaghipisheh, J. (2023). Effects of melatonin foliar application on hot pepper growth and stress tolerance. Plant Stress 9, 100192. doi: 10.1016/j.stress.2023.100192
Kidokoro, S., Hayashi, K., Haraguchi, H., Ishikawa, T., Soma, F., Konoura, I., et al. (2021). Posttranslational regulation of multiple clock-related transcription factors triggers cold-inducible gene expression in Arabidopsis. Proc. Natl. Acad. Sci. 118, e2021048118. doi: 10.1073/pnas.2021048118
Kumar, G., Saad, K. R., Arya, M., Puthusseri, B., Mahadevappa, P., Shetty, N. P., et al. (2021). The Synergistic role of serotonin and melatonin during temperature stress in promoting cell division, ethylene and isoflavones biosynthesis in Glycine max. Curr. Plant Biol. 26, 100206. doi: 10.1016/j.cpb.2021.100206
Kurokawa, Y., Nagai, K., Huan, P. D., Shimazaki, K., Qu, H., Mori, Y., et al. (2018). Rice leaf hydrophobicity and gas films are conferred by a wax synthesis gene (LGF1) and contribute to flood tolerance. New Phytol. 218, 1558–1569. doi: 10.1111/nph.15070
Langaroudi, I. K., Piri, S., Chaeikar, S. S., Salehi, B. (2023). Evaluating drought stress tolerance in different Camellia sinensis L. cultivars and effect of melatonin on strengthening antioxidant system. Scientia Hortic. 307, 111517. doi: 10.1016/j.scienta.2022.111517
Li, X., Ahammed, G. J., Zhang, X. N., Zhang, L., Yan, P., Zhang, L. P., et al. (2021). Melatonin-mediated regulation of anthocyanin biosynthesis and antioxidant defense confer tolerance to arsenic stress in. J. Hazardous Materials 403, 123922. doi: 10.1016/j.jhazmat.2020.123922
Li, J., Liu, J., Zhu, T., Zhao, C., Li, L., Chen, M. (2019). The role of melatonin in salt stress responses. Int. J. Mol. Sci. 20, 1735. doi: 10.3390/ijms20071735
Li, G., Siddiqui, H., Teng, Y., Lin, R., Wan, X. Y., Li, J., et al. (2011). Coordinated transcriptional regulation underlying the circadian clock in Arabidopsis. Nat. Cell Biol. 13, 616–622. doi: 10.1038/ncb2219
Li, C., Su, J., Zhao, N., Lou, L., Ou, X., Yan, Y., et al. (2023). CmERF5-CmRAP2. 3 transcriptional cascade positively regulates waterlogging tolerance in Chrysanthemum morifolium. Plant Biotechnol. J. 21, 270–282. doi: 10.1111/pbi.13940
Li, C., Tan, D. X., Liang, D., Chang, C., Jia, D., Ma, F. (2015). Melatonin mediates the regulation of ABA metabolism, free-radical scavenging, and stomatal behaviour in two Malus species under drought stress. J. Exp. Bot. 66, 669–680. doi: 10.1093/jxb/eru476
Li, Y. F., Zeng, X. Q., Li, Y., Wang, L., Zhuang, H., Wang, Y., et al. (2020). MULTI-FLORET SPIKELET 2, a MYB transcription factor, determines spikelet meristem fate and floral organ identity in rice. Plant Physiol. 184, 988–1003. doi: 10.1104/pp.20.00743
Licausi, F., Ohme-Takagi, M., Perata, P. (2013). APETALA 2/Ethylene Responsive Factor (AP 2/ERF) transcription factors: Mediators of stress responses and developmental programs. New Phytol. 199, 639–649. doi: 10.1111/nph.12291
Lin, R., Ding, L., Casola, C., Ripoll, D. R., Feschotte, C., Wang, H. (2007). Transposase-derived transcription factors regulate light signaling in Arabidopsis. Science 318, 1302–1305. doi: 10.1126/science.1146281
Liu, K., Harrison, M. T., Yan, H., Liu, D. L., Meinke, H., Hoogenboom, G., et al. (2023). Silver lining to a climate crisis in multiple prospects for alleviating crop waterlogging under future climates. Nat. Commun. 14, 765. doi: 10.1038/s41467–023-36129–4
Liu, J., Shabala, S., Zhang, J., Ma, G., Chen, D., Shabala, L., et al. (2020). Melatonin improves rice salinity stress tolerance by NADPH oxidase-dependent control of the plasma membrane K+ transporters and K+ homeostasis. Plant Cell Environ. 43, 2591–2605. doi: 10.1111/pce.13759
Liu, Y., Xie, Y., Wang, H., Ma, X., Yao, W., Wang, H. (2017). Light and ethylene coordinately regulate the phosphate starvation response through transcriptional regulation of PHOSPHATE STARVATION RESPONSE1. Plant Cell 29, 2269–2284. doi: 10.1105/tpc.17.00268
Liu, J., Yue, R., Si, M., Wu, M., Cong, L., Zhai, R., et al. (2019). Effects of exogenous application of melatonin on quality and sugar metabolism in ‘Zaosu’pear fruit. J. Plant Growth Regul. 38, 1161–1169. doi: 10.1007/s00344–019-09921–0
Ma, L., Li, G. (2018). FAR1-related sequence (FRS) and FRS-related factor (FRF) family proteins in Arabidopsis growth and development. Front. Plant Sci. 9. doi: 10.3389/fpls.2018.00692
Ma, X., Zheng, J., Zhang, X., Hu, Q., Qian, R. (2017). Salicylic acid alleviates the adverse effects of salt stress on Dianthus superbus (Caryophyllaceae) by activating photosynthesis, protecting morphological structure, and enhancing the antioxidant system. Front. Plant Sci. 8. doi: 10.3389/fpls.2017.00600
MacWilliams, J. R., Nabity, D., Mauck, K. E., Kaloshian, I. (2023). Transcriptome analysis of aphid-resistant and susceptible near isogenic lines reveals candidate resistance genes in cowpea (Vigna unguiculata). BMC Plant Biol. 23, 22. doi: 10.1186/s12870–022-04021-w
Moustafa-Farag, M., Mahmoud, A., Arnao, M. B., Sheteiwy, M. S., Dafea, M., Soltan, M., et al. (2020). Melatonin-induced water stress tolerance in plants: Recent advances. Antioxidants 9, 809. doi: 10.3390/antiox9090809
Murch, S. J., Alan, A. R., Cao, J., Saxena, P. K. (2009). Melatonin and serotonin in flowers and fruits of Datura metel L. J. Pineal Res. 47, 277–283. doi: 10.1111/j.1600–079X.2009.00711.x
NaveenKumar, S. K., Hemshekhar, M., Jagadish, S., Manikanta, K., Vishalakshi, G. J., Kemparaju, K., et al. (2020). Melatonin restores neutrophil functions and prevents apoptosis amid dysfunctional glutathione redox system. J. Pineal Res. 69, e12676. doi: 10.1111/jpi.12676
Nawaz, M. A., Huang, Y., Bie, Z., Ahmed, W., Reiter, R. J., Niu, M., et al. (2016). Melatonin: current status and future perspectives in plant science. Front. Plant Sci. 6. doi: 10.3389/fpls.2015.01230
Palta, J. P. (1990). Leaf chlorophyll content. Remote Sens. Rev. 5, 207–213. doi: 10.1080/02757259009532129
Pan, J., Sharif, R., Xu, X., Chen, X. (2021). Mechanisms of waterlogging tolerance in plants: Research progress and prospects. Front. Plant Sci. 11. doi: 10.3389/fpls.2020.627331
Pandey, G. K., Grant, J. J., Cheong, Y. H., Kim, B. G., Li, L., Luan, S. (2005). ABR1, an APETALA2-domain transcription factor that functions as a repressor of ABA response in Arabidopsis. Plant Physiol. 139, 1185–1193. doi: 10.1104/pp.105.066324
Pardo-Hernández, M., López-Delacalle, M., Rivero, R. M. (2020). ROS and NO regulation by melatonin under abiotic stress in plants. Antioxidants. 9, 1078. doi: 10.3390/antiox9111078
Peláez-Vico, M.Á., Tukuli, A., Singh, P., Mendoza-Cózatl, D. G., Joshi, T., Mittler, R. (2023). Rapid systemic responses of Arabidopsis to waterlogging stress. Plant Physiol. 193, 2215–2231. doi: 10.1093/plphys/kiad433
Peng, Y., Zhou, Z., Tong, R., Hu, X., Du, K. (2017). Anatomy and ultrastructure adaptations to soil flooding of two full-sib poplar clones differing in flood-tolerance. Flora 233, 90–98. doi: 10.1016/j.flora.2017.05.014
Qian, R., Hu, Q., Ma, X., Zhang, X., Ye, Y., Liu, H., et al. (2022). Comparative transcriptome analysis of heat stress responses of Clematis lanuginosa and Clematis crassifolia. BMC Plant Biol. 22, 1–16. doi: 10.1186/s12870–022-03497-w
Rabiza-Świder, J., Skutnik, E., Jędrzejuk, A. (2017). The effect of preservatives on water balance in cut clematis flowers. J. Hortic. Sci. Biotechnol. 92, 270–278. doi: 10.1080/14620316.2016.1276863
Raineri, J., Ribichich, K. F., Chan, R. L. (2015). The sunflower transcription factor HaWRKY76 confers drought and flood tolerance to Arabidopsis thaliana plants without yield penalty. Plant Cell Rep. 34, 2065–2080. doi: 10.1007/s00299–015-1852–3
Read, S. M., Northcote, D. H. (1981). Minimization of variation in the response to different proteins of the Coomassie blue G dye-binding assay for protein. Analytical Biochem. 116, 53–64. doi: 10.1016/0003–2697(81)90321–3
Ren, D., Rao, Y., Yu, H., Xu, Q., Cui, Y., Xia, S., et al. (2020). MORE FLORET1 encodes a MYB transcription factor that regulates spikelet development in rice. Plant Physiol. 184, 251–265. doi: 10.1104/pp.20.00658
Rushton, P. J., Somssich, I. E., Ringler, P., Shen, Q. J. (2010). WRKY transcription factors. Trends Plant Sci. 15, 247–258. doi: 10.1016/j.tplants.2010.02.006
Shiono, K., Yamauchi, T., Yamazaki, S., Mohanty, B., Malik, A. I., Nagamura, Y., et al. (2014). Microarray analysis of laser-microdissected tissues indicates the biosynthesis of suberin in the outer part of roots during formation of a barrier to radial oxygen loss in rice (Oryza sativa). J. Exp. Bot. 65, 4795–4806. doi: 10.1093/jxb/eru235
Singh, R., Singh, S., Parihar, P., Mishra, R. K., Tripathi, D. K., Singh, V. P., et al. (2016). Reactive oxygen species (ROS): beneficial companions of plants’ developmental processes. Front. Plant Sci. 7. doi: 10.3389/fpls.2016.01299
Sun, C., Liu, L., Wang, L., Li, B., Jin, C., Lin, X. (2021). Melatonin: A master regulator of plant development and stress responses. J. Integr. Plant Biol. 63, 126–145. doi: 10.1111/jipb.12993
Talaat, N. B. (2021). Polyamine and nitrogen metabolism regulation by melatonin and salicylic acid combined treatment as a repressor for salt toxicity in wheat (Triticum aestivum L.) plants. Plant Growth Regul. 95, 315–329. doi: 10.1007/s10725–021-00740–6
Tang, W., Ji, Q., Huang, Y., Jiang, Z., Bao, M., Wang, H., et al. (2013). FAR-RED ELONGATED HYPOCOTYL3 and FAR-RED IMPAIRED RESPONSE1 transcription factors integrate light and abscisic acid signaling in Arabidopsis. Plant Physiol. 163, 857–866. doi: 10.1104/pp.113.224386
Teoh, E. Y., Teo, C. H., Baharum, N. A., Pua, T. L., Tan, B. C. (2022). Waterlogging stress induces antioxidant defense responses, aerenchyma formation and alters metabolisms of banana plants. Plants 11, 2052. doi: 10.3390/plants11152052
Tian, L. X., Zhang, Y. C., Chen, P. L., Zhang, F. F., Li, J.. (2021). How does the waterlogging regime affect crop yield? A global meta-analysis. Frontiers in Plant Science. 12, 634898. doi: 10.3389/fpls.2021.634898
Tiwari, R. K., Lal, M. K., Kumar, R., Chourasia, K. N., Naga, K. C., Kumar, D., et al. (2021). Mechanistic insights on melatonin-mediated drought stress mitigation in plants. Physiologia Plantarum 172, 1212–1226. doi: 10.1111/ppl.13307
Tong, C., Hill, C. B., Zhou, G., Zhang, X. Q., Jia, Y., Li, C. (2021). Opportunities for improving waterlogging tolerance in cereal crops—physiological traits and genetic mechanisms. Plants 10, 1560. doi: 10.3390/plants10081560
Turk, H., Erdal, S., Genisel, M., Atici, O., Demir, Y., Yanmis, D. (2014). The regulatory effect of melatonin on physiological, biochemical and molecular parameters in cold-stressed wheat seedlings. Plant Growth Regul. 74, 139–152. doi: 10.1007/s10725–014-9905–0
Voesenek, L. A., Sasidharan, R., Visser, E. J., Bailey-Serres, J. (2016). Flooding stress signaling through perturbations in oxygen, ethylene, nitric oxide and light. New Phytol. 209, 39–43. doi: 10.1111/nph.13775
Wang, L., Dossa, K., You, J., Zhang, Y., Li, D., Zhou, R., et al. (2021). High-resolution temporal transcriptome sequencing unravels ERF and WRKY as the master players in the regulatory networks underlying sesame responses to waterlogging and recovery. Genomics 113, 276–290. doi: 10.1016/j.ygeno.2020.11.022
Wang, L. Y., Liu, J. L., Wang, W. X., Sun, Y. (2016). Exogenous melatonin improves growth and photosynthetic capacity of cucumber under salinity-induced stress. Photosynthetica 54, 19–27. doi: 10.1007/s11099–015-0140–3
Wang, H., Liu, X., Yang, P., Wu, R., Wang, S., He, S., et al. (2022a). Potassium application promote cotton acclimation to soil waterlogging stress by regulating endogenous protective enzymes activities and hormones contents. Plant Physiol. Biochem. 185, 336–343. doi: 10.1016/j.plaphy.2022.06.019
Wang, H., Ni, D., Shen, J., Deng, S., Xuan, H., Wang, C., et al. (2022b). Genome-wide identification of the AP2/ERF gene family and functional analysis of GmAP2/ERF144 for drought tolerance in soybean. Front. Plant Sci. 13. doi: 10.3389/fpls.2022.848766
Wang, J., Sun, H., Sheng, J., Jin, S., Zhou, F., Hu, Z., et al. (2019). Transcriptome, physiological and biochemical analysis of Triarrhena sacchariflora in response to flooding stress. BMC Genet. 20, 1–15. doi: 10.1186/s12863–019-0790–4
Wang, Y., Wang, D., Tao, Z., Yang, Y., Gao, Z., Zhao, G., et al. (2021). Impacts of nitrogen deficiency on wheat (Triticum aestivum L.) grain during the medium filling stage: Transcriptomic and metabolomic comparisons. Front. Plant Sci. 12. doi: 10.3389/fpls.2021.674433
Wang, Z., Wong, D. C. J., Chen, Z., Bai, W., Si, H., Jin, X. (2022c). Emerging roles of plant DNA-binding with one finger transcription factors in various hormone and stress signaling pathways. Front. Plant Sci. 13. doi: 10.3389/fpls.2022.844201
Wang, R., Xie, M., Zhao, W., Yan, P., Wang, Y., Gu, Y., et al. (2023). WGCNA reveals genes associated with lignification in the secondary stages of wood formation. Forests 14, 99. doi: 10.3390/f14010099
Wang, K., Xing, Q., Ahammed, G. J., Zhou, J. (2022d). Functions and prospects of melatonin in plant growth, yield, and quality. J. Exp. Bot. 73, 5928–5946. doi: 10.1093/jxb/erac233
Wang, S., Zhou, H., Feng, N., Xiang, H., Liu, Y., Wang, F., et al. (2022). Physiological response of soybean leaves to uniconazole under waterlogging stress at R1 stage. J. Plant Physiol. 268, 153579. doi: 10.1016/j.jplph.2021.153579
Wei, J., Li, D. X., Zhang, J. R., Shan, C., Rengel, Z., Song, Z. B., et al. (2018). Phytomelatonin receptor PMTR 1-mediated signaling regulates stomatal closure in Arabidopsis thaliana. J. Pineal Res. 65, e12500. doi: 10.1111/jpi.12500
Winfield, M. O., Lu, C., Wilson, I. D., Coghill, J. A., Edwards, K. J. (2010). Plant responses to cold: transcriptome analysis of wheat. Plant Biotechnol. J. 8, 749–771. doi: 10.1111/j.1467–7652.2010.00536.x
Wu, P., Ma, Y., Ahammed, G. J., Hao, B., Chen, J., Wan, W., et al. (2022). Insights into melatonin-induced photosynthetic electron transport under low-temperature stress in cucumber. Front. Plant Sci. 13. doi: 10.3389/fpls.2022.1029854
Xia, J., Chen, J. (2021). A new era of flood control strategies from the perspective of managing the 2020 Yangtze River flood. Sci. China Earth Sci. 64, 1–9. doi: 10.1007/s11430–020-9699–8
Yang, X. L., Xu, H., Li, D., Gao, X., Li, T. L., Wang, R. (2018). Effect of melatonin priming on photosynthetic capacity of tomato leaves under low-temperature stress. Photosynthetica 56, 884–892. doi: 10.1007/s11099–017-0748–6
Yang, L., You, J., Li, J., Wang, Y., Chan, Z. (2021). Melatonin promotes Arabidopsis primary root growth in an IAA-dependent manner. J. Exp. Bot. 72, 5599–5611. doi: 10.1093/jxb/erab196
Zeng, R., Chen, T., Wang, X., Cao, J., Li, X., Xu, X., et al. (2021). Physiological and expressional regulation on photosynthesis, starch and sucrose metabolism response to waterlogging stress in peanut. Front. Plant Sci. 12. doi: 10.3389/fpls.2021.601771
Zhai, Y., Zhang, L., Xia, C., Fu, S., Zhao, G., Jia, J., et al. (2016). The wheat transcription factor, TabHLH39, improves tolerance to multiple abiotic stressors in transgenic plants. Biochem. Biophys. Res. Commun. 473, 1321–1327. doi: 10.1016/j.bbrc.2016.04.071
Zhang, Q., Liu, X., Zhang, Z., Liu, N., Li, D., Hu, L. (2019). Melatonin improved waterlogging tolerance in Alfalfa (Medicago sativa) by reprogramming polyamine and ethylene metabolism. Front. Plant Sci. 10. doi: 10.3389/fpls.2019.00044
Zhang, B., Sun, M., Liu, W., Lian, M., Yang, S., Peng, F., et al. (2023a). Waterlogging resistance and evaluation of physiological mechanism of three peach (Prunus persica) rootstocks. Protoplasma 260, 1375–1388. doi: 10.1007/s00709–023-01850-w
Zhang, R., Yue, Z., Chen, X., Wang, Y., Zhou, Y., Xu, W., et al. (2022). Foliar applications of urea and melatonin to alleviate waterlogging stress on photosynthesis and antioxidant metabolism in sorghum seedlings. Plant Growth Regul. 97, 429–438. doi: 10.1007/s10725–021-00705–9
Zhang, Y., Zhu, J., Khan, M., Wang, Y., Xiao, W., Fang, T., et al. (2023b). Transcription factors ABF4 and ABR1 synergistically regulate amylase-mediated starch catabolism in drought tolerance. Plant Physiol. 191, 591–609. doi: 10.1093/plphys/kiac428
Zhao, L., Yang, T., Xing, C., Dong, H., Qi, K., Gao, J., et al. (2019). The β-amylase PbrBAM3 from pear (Pyrus betulaefolia) regulates soluble sugar accumulation and ROS homeostasis in response to cold stress. Plant Sci. 287, 110184. doi: 10.1016/j.plantsci.2019.110184
Zheng, X., Zhou, J., Tan, D. X., Wang, N., Wang, L., Shan, D., et al. (2017). Melatonin improves waterlogging tolerance of Malus baccata (Linn.) Borkh. seedlings by maintaining aerobic respiration, photosynthesis and ROS migration. Front. Plant Sci. 8. doi: 10.3389/fpls.2017.00483
Zhong, Y. H., Guo, Z. J., Wei, M. Y., Wang, J. C., Song, S. W., Chi, B. J., et al. (2023). Hydrogen sulfide upregulates the alternative respiratory pathway in mangrove plant Avicennia marina to attenuate waterlogging-induced oxidative stress and mitochondrial damage in a calcium-dependent manner. Plant Cell Environ. 46, 1521–1539. doi: 10.1007/s11104–022-05759-w
Zhu, Q. G., Gong, Z. Y., Huang, J., Grierson, D., Chen, K. S., Yin, X. R. (2019). High-CO2/hypoxia-responsive transcription factors DkERF24 and DkWRKY1 interact and activate DkPDC2 promoter. Plant Physiol. 180, 621–633. doi: 10.1104/pp.18.01552
Keywords: Clematis tientaiensis, Clematis lanuginosa, waterlogging, physiological analysis, transcriptome analysis, transcription factor
Citation: Chen K, Hu Q, Ma X, Zhang X, Qian R and Zheng J (2024) The effect of exogenous melatonin on waterlogging stress in Clematis. Front. Plant Sci. 15:1385165. doi: 10.3389/fpls.2024.1385165
Received: 12 February 2024; Accepted: 30 May 2024;
Published: 18 June 2024.
Edited by:
Fernanda Fidalgo, University of Porto, PortugalReviewed by:
Lia Dinis, University of Trás-os-Montes and Alto Douro, PortugalBruno Sousa, University of Porto, Portugal
Copyright © 2024 Chen, Hu, Ma, Zhang, Qian and Zheng. This is an open-access article distributed under the terms of the Creative Commons Attribution License (CC BY). The use, distribution or reproduction in other forums is permitted, provided the original author(s) and the copyright owner(s) are credited and that the original publication in this journal is cited, in accordance with accepted academic practice. No use, distribution or reproduction is permitted which does not comply with these terms.
*Correspondence: Jian Zheng, zjyzs@126.com
†These authors have contributed equally to this work and share first authorship