- 1SINAGRI S.r.l. – Spin-Off of the University of Bari Aldo Moro, Bari, Italy
- 2Rete Italian Variety Club (IVC), Locorotondo, Italy
- 3Department of Biotechnology, University of Verona, Verona, Italy
- 4Department of Soil, Plant and Food Sciences, University of Bari Aldo Moro, Bari, Italy
- 5Istituto per la Protezione Sostenibile delle Piante (IPSP), CNR, Bari, Italy
The growing demand for sustainable and environmentally friendly viticulture is leading to a multiplication of breeding programs aimed at obtaining vines that are resistant to powdery mildew (PM) and downy mildew (DM), the two most damaging vine diseases. In Puglia, the most important Italian region for the production of table grapes, an extensive crossing program was launched in 2015 with 113 crosses, including elite table varieties, seedless varieties, and resistant varieties. The main seedling production parameters were measured for each cross. In particular, berries harvested as well as the number of seeds and seedlings obtained were considered. Approximately 103,119 seedlings were obtained and subjected to marker-assisted selection for seedlessness using the marker VvAGL11 and for resistance to PM and DM with appropriate markers. Approximately one third (32,638) of the progenies were selected as putative seedless and seventeen thousand five hundred-nine (17,509) were transferred to the field for phenotypic evaluation, including 527 seedless individuals putatively resistant, of which 208 confirmed to be resistant to DM, 22 resistant to PM, and 20 individuals that combined resistance and seedlessness traits. The work discusses the effects of parental combinations and other variables in obtaining surviving progeny and pyramiding genes in table grapes and provides useful information for selecting genotypes and increasing the efficiency of breeding programs for seedless disease-resistant grapes.
1 Introduction
Italy is the fourth largest table grape producer in the world after China, Turkey, and Chile and ahead of the United States and South Africa, with a production of 1,005,552 million tons in 2021, and the main European reference in this sector (OIV, https://www.oiv.int/what-we-do/country-report?oiv, accessed June 19, 2023). In Italy, table grape production is localized in the Southern Italian regions, especially Apulia and Sicily, which are characterized by a hot and dry summer climate, mild winters with rainfall not exceeding 500–600 mm/year, and winter temperatures rarely below 0°C. Almost 60% of the Italian production is concentrated in Apulia, mainly with the varieties ITALIA, REGINA BIANCA, VITTORIA, MICHELE PALIERI, and RED GLOBE, which carry the protected geographical indication “Uva di Puglia I.G.P.” (UE Reg. n. 680/2012, 2012; 2020, ISTAT). Seedlessness and other traits, such as crispness, berry shape, color, and bunch compactness, have become highly valued by consumers (Somogyi et al., 2020). Since the commercialization of the variety Sultanina under the name Thompson Seedless in the 1970s (Anderson, 2013), many other varieties have been successfully developed and cultivated worldwide, such as SUGRAONE, CRIMSON SEEDLESS, and AUTUMN ROYAL. These varieties all carry steno-spermocarpic seedlessness, which is a highly heritable and dominant trait that can be selected very early using the intragenic microsatellite marker, p3_VvAGL11, mapped in the regulatory region of the VviAGL11 gene (Mejía et al., 2011; Bergamini et al., 2013; Royo et al., 2018; di Rienzo et al., 2020). However, these varieties are sold under Plant Breeders’ Rights protection, which limits their availability and increases costs. They also have adaptation problems in Apulia, which lead to browning of the skin and splitting of the berries and require special agronomic procedures (Otto et al., 2022). Another important quality parameter for table grapes is the color variation of the berry skin, which has become highly diversified through hybridization and human selection. Berry skin color is mainly related to the content and composition of anthocyanins, which is determined by the allelic status of an important locus spanning a 200-kb region on chromosome 2 (Azuma et al., 2008; Fournier-Level et al., 2009; Matus et al., 2009). In the last decade, several studies have shown that the R2R3-MYB gene is the major genetic determinant of anthocyanin content and composition in grape berry skin. However, further studies are needed to understand the complex network acting on this trait to fully understand and apply it in inbreeding programs.
The control of diseases by genetic resistance sources, in particular powdery mildew (PM) and downy mildew (DM), caused by the biotrophic fungi Erysiphe necator (Schwein.) Burrill and Plasmopara viticola (Berk. & M.A. Curtis) Berl. and De Toni, respectively, has become necessary to obtain high-quality grape productions. The former usually attacks in warm and dry seasons, while the latter disease occurs in warmer and more humid climates (Miazzi et al., 1997; Hajjeh et al., 2008; Fröbel and Zyprian, 2019). Both diseases require a massive usage of pesticides, which is associated with high economic and environmental costs, including the emergence of resistance in pathogens (Hajjeh et al., 2005; Miazzi et al., 2008; Pedneault and Provost, 2016). The strict regulation of pesticides (Directive 2009/128/EC; Regulation 2002/473/EC) and the increasing demand for organic products have made sustainability an important issue in grape cultivation. Efforts to develop more sustainable viticulture have multiplied prompting private and public organizations to expand the use of resistant varieties introgressing genes from American non-vinifera species (such as Vitis riparia, Vitis rupestris, Muscadinia rotundifolia, Vitis cinerea, Vitis berlandieri, Vitis lincecumii, Vitis labrusca), Asian species (Vitis piasezkii, Vitis amurensis, Vitis romanetii), and Vitis vinifera Kishmish vatkana. These species have been used in breeding programs for decades (Bellin et al., 2009; Venuti et al., 2013; Feechan et al., 2015; Ibáñez et al., 2015; Ruehl et al., 2015; Merdinoglu et al., 2018; Vezzulli et al., 2018; Bove et al., 2019; Pomarici and Vecchio, 2019; Weinmann et al., 2019; Possamai et al., 2022; Schneider et al., 2019; Karn et al., 2021). After the first poor-quality hybrids, new resistant varieties, such as Regent or Solaris, have met the quality standards of the European market [30–32] (Töpfer et al., 2011, Buonassisi et al., 2017) paving the way for a wider use of genotypes coming from interspecific crosses also in the competitive table grape breeding programs, together with additional traits such as seedlessness. According to the VIVC database (https://www.vivc.de/index.php), 35 loci for downy mildew resistance (Rpv loci) and 13 loci for powdery mildew resistance (Run and Ren loci) have been mapped so far and are available for breeding even if only a few of them have been used so far. Associated markers are available for these loci, enabling marker-assisted selection (MAS) of resistant individuals at early stages of plant development (Eibach et al., 2007; Pathania et al., 2017; Cobb et al., 2019; Vezzulli et al., 2019; Zini et al., 2019), thus, greatly facilitating breeding programs.
This is particularly true for table grapes, where fungicides have to be applied repeatedly since early spring and throughout the growing season (Miazzi and Hajjeh, 2011; Chen et al., 2020). Over the last 20 years, developments in biotechnology and molecular biology have provided breeders with useful tools to speed up programs and make them more efficient. Genotyping and traceability methods based on molecular markers (Di Rienzo et al., 2017; Miazzi et al., 2020; Sanzani et al., 2016), and next-generation sequencing (NGS) technologies have led to more innovative and targeted studies in grape breeding (Butiuc and Coste, 2023; Vervalle et al., 2022). The intensive chemical treatments required to control them imposes expensive costs on growers and the environment, contribute significantly to pollution, and ultimately lead to a decline in the effectiveness of pesticides (Reynolds, 2015; Lykogianni et al., 2021).
This paper presents the results of a five-year breeding program carried out since 2015 by the Apulian winegrowers’ association “Italian Variety Club” (IVC, Bari, Italy) to obtain new grape varieties that combine seedlessness with resistance to PM and DM. The analysis of parental combinations/cultivation conditions for the development of new hybrids and an evaluation of the success of pyramidization will provide useful information to improve the efficiency of breeding seedless resistant grape varieties.
2 Material and methods
2.1 Plant material
For the crossing program, 38 stenospermocarpic seedless elite table grape varieties and pre-breeding material, and 42 elite seeded table varieties were selected (Table 1). Among them, seven were resistant to DM and PM, deriving from the species V. riparia, V. rupestris (Vitis International Variety Catalogue, VIVC, https://www.vivc.de/), and possibly M. rotundifolia. The identity of the parental varieties was preliminarily confirmed by PCR amplification with nine SSR markers established by the OIV for genotyping grapevines (OIV, accessed 10/5/2023) [36], according to the VIVC and DISSPA databases (Supplementary Table 1). All vines were 10 years old and were grown either at the Experimental Station “Centro di Ricerca e Formazione Basile Caramia” in Locorotondo (Bari, Italy) or at the IVC producer’s consortium (Bari, Italy) at a planting density of 1.0 × 2.5 m trained to a T-trellis. The vines were covered with a thin white net, and 0.16-mm-thick white polyethylene was applied from stage BBCH-11 (Lorenz et al., 1995). Irrigation was provided every 3 to 4 days by a drip irrigation system to keep the soil above 75% of field capacity. All agronomic practices were applied uniformly in all treatments and were consistent with standard commercial practice in the area.
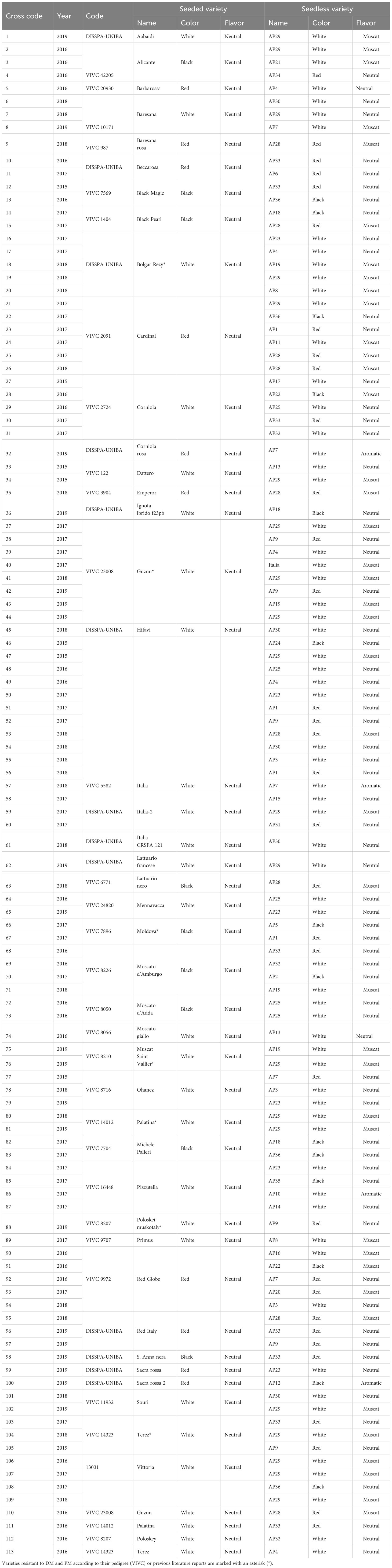
Table 1 List of V. vinifera cultivars used for the cross combinations between seeded (SD, female parent) and seedless (SL, male parent) cultivars carried out from 2015 to 2019.
2.2 Crosses and seeds recovery
From 2015 to 2019, a total of 113 crosses was made. A single variety was used in combination with several different varieties to compare the crossing performances of the different combinations (Table 1). Most of the cultivated grapes are hermaphroditic, and fertilization occurs mainly by self-pollination (Harst et al., 2009). In programmed crosses, therefore, the emasculation of plants to be used as female parents is necessary. Late-seeded varieties were chosen as female. Pollen was collected from fully flowering vines (at least 30%) at stages BBCH-65 and BBCH-68, while the female parent was used at stage BBCH-60 (first flower hoods detached from the receptaculum) (Lorenz et al., 1995). Pollen was collected avoiding humid, windy, and rainy conditions and stored at 18°C. Before use, pollen germinability was tested on paper in Petri dishes as percentage of granules producing germination tubes; samples with a germination of <50% were discarded.
A total of 1,612 bunches were pollinated. The inflorescences were emasculated with sterile tweezers and pollinated twice with a sterile brush within 48 h. The pollinated inflorescences were immediately packed until complete berry development. After first fruit development (10 days), bags were removed to allow regular growth of the grapes. Depending on the combination, fully ripe grapes with completely woody seeds were harvested from the end of August through September. The seeds were extracted from the ripe berries, washed in 1% hypochlorite solution, rinsed three times in sterile distilled water, and dried on paper at 25°C and low humidity for approximately 4–5 days until they were completely dry. Then they were stored in Petri dishes in the dark, under cool and dry conditions (4°C) for vernalization (Wang et al., 2022) (Figure 1).
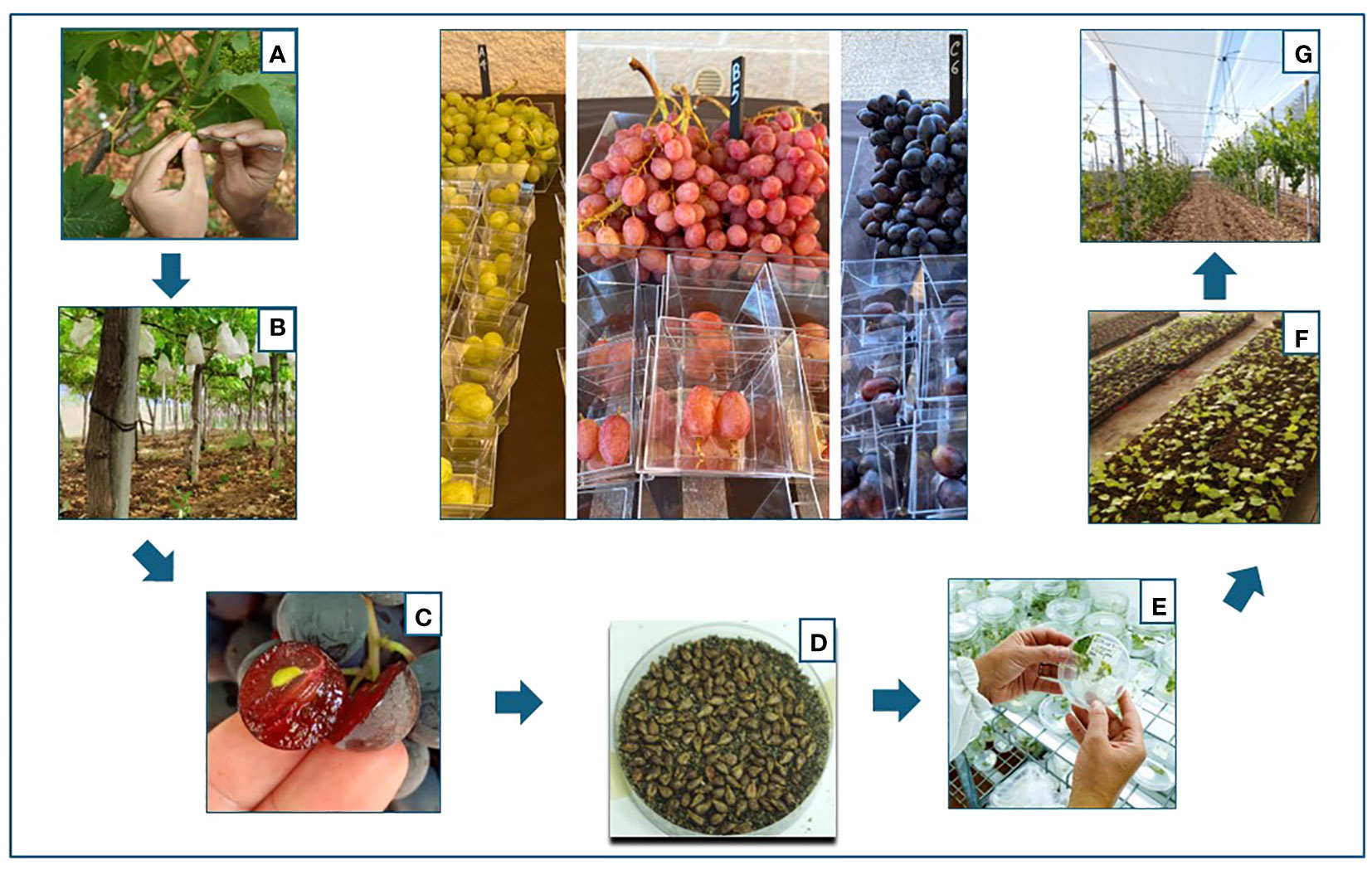
Figure 1 Flowchart of the breeding process followed to obtain the new varieties: (A) emasculation of the flowers of the female parents; (B) pollination and casing of the bunches with paper bags; (C) collection of the seeds; (D) stratification of the seeds in sand for vernalization; (E) germination of the seeds; (F) transfer and acclimatization of the vines in the greenhouse; (G) selection of the vines in the field.
2.3 Seed vernalization, germination, and plant development
Seeds were vernalized by stratification in thin, moistened river sand autoclaved at 120°C for 40 min. Seeds were stored in the dark at 4°C for approximately 1.5 months taking care not to let it mold or rot. For some varieties, this phase was extended to at least 3 months. After vernalization, seeds were transferred to paper discs in Petri dishes for germination, then transplanted into paper pots (250 ml) containing a soil mixture of vermiculite/peat soil/coconut shells (1:4:1) and placed in a greenhouse at 24°C and natural daylight for 1 month for acclimatization, after which they were transplanted into the open field (Figure 1).
2.4 DNA extraction and marker-assisted selection
Two young leaves were taken from each plantlet and used for DNA extraction following the protocol of Spadoni et al. (2019). The quantity and quality of extracted DNA was measured using the Nano-Drop™ 12000C spectrophotometer (Thermo Scientific, Waltham, MA) and 0.8% agarose gel electrophoresis. Seedlessness was selected using the SSR marker VvAGL11 with forward primer labeled with a 6-FAM, HEX, NED fluorescent dye (Bergamini et al., 2013). A final PCR volume of 12.5-μl mixture was used, including 2 ng/µl of DNA, dNTP 0.4 mM, 1.25 µl of PCR buffer 2×, 1.25 µl of primer mix forward and reverse (2.5 ng/µl), 0.1 U of DreamTaq polymerase. Reactions were performed under the following conditions: 95°C, 5 min; 10 cycles: 95°C, 30 s; 55°C, 45 s; 72°C, 45 s with a touch-down of 0.5°C per cycle; 25 cycles: 95°C, 30 s; 50°C, 45 s; 72°C, 45 s; final extension to 72°C, 15 min.
For resistance selection, a preliminary screening of the resistance genes present in the parents used for the crosses was carried out, as they were provided by Novisad University (Serbia), and the pedigrees of many of them were unknown. Therefore, four SSR markers associated with the Rpv1 and Rpv3 genes for DM and five SSR markers associated with the Run1, Run2, Ren1, and Ren4 genes for PM were selected based on a bibliographic review (VIVC, Di Gaspero et al., 2012; Li et al., 2012; Prazzoli et al., 2019; Zini et al., 2019) (Table 2). A preliminarily validation of markers on the resistant parents was carried out and for four among the resistant genotypes we got so far, robust and effective amplifications with markers UDV305 and UDV737 for DM, and VMC4f3.1 for PM giving the expected resistant allele profiles. Thus, these crosses were retained for subsequent MAS analysis in progenies (Table 3). PCRs were carried out in a final volume of 20 μl using the following concentrations: 2 ng/µl of DNA, dNTP 0.4 mM, PCR buffer 2×, 1 µl of primer forward (1 ng/µl), 3.2 µl of primer reverse (1 ng/µl), 1.6 µl of universal primer M13(-20) (1 ng/µl) labeled with a 6-FAM, HEX, NED fluorescent dye, and 0.25 U of DreamTaq polymerase. The following conditions were used: 95°C, 2 min; 10 cycles: 94°C, 20 s; 55°C, 20 s; 65°C, 40 s with a touch-down of 0.5°C per cycle; 25 cycles: 94°C, 20 s; 50°C, 20 s; 65°C, 40 s; final extension to 65°C, 30 min. Capillary electrophoresis was performed using the ABI PRISM 3100 Genetic Analyzer (Life Technologies) mixing 2 µl of the amplification products with 14.6 µl of formamide and 0.5 µl of the GeneScan 500 ROX size standards (Life Technologies, Carlsbad, CA, USA). Allele sizes were assigned using GeneMapper® software version 3.7 (Life Technologies).
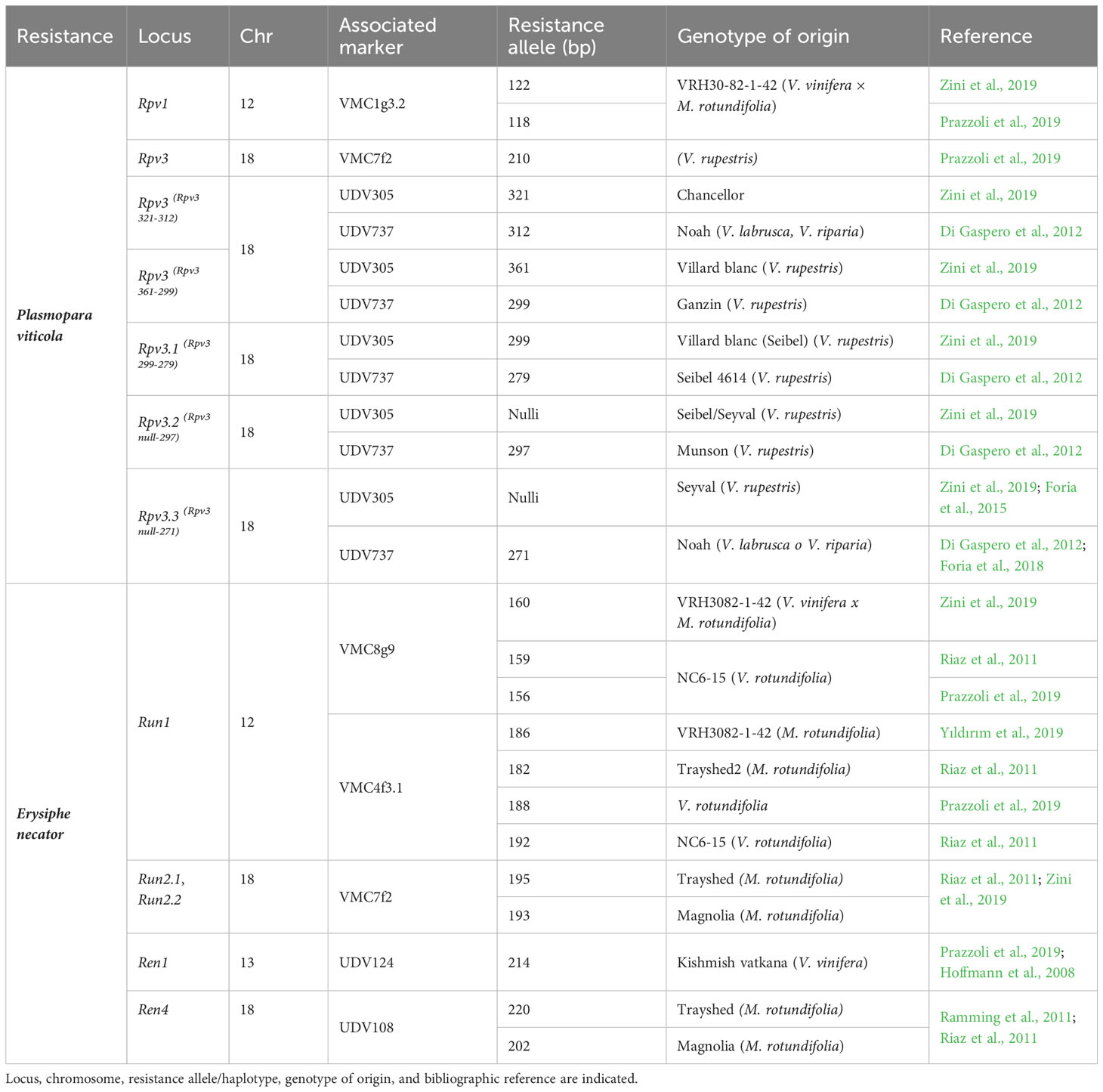
Table 2 List of markers selected for the analysis of loci associated with resistance (R) to DM and PM and used for a pre-screening on resistant parentals.
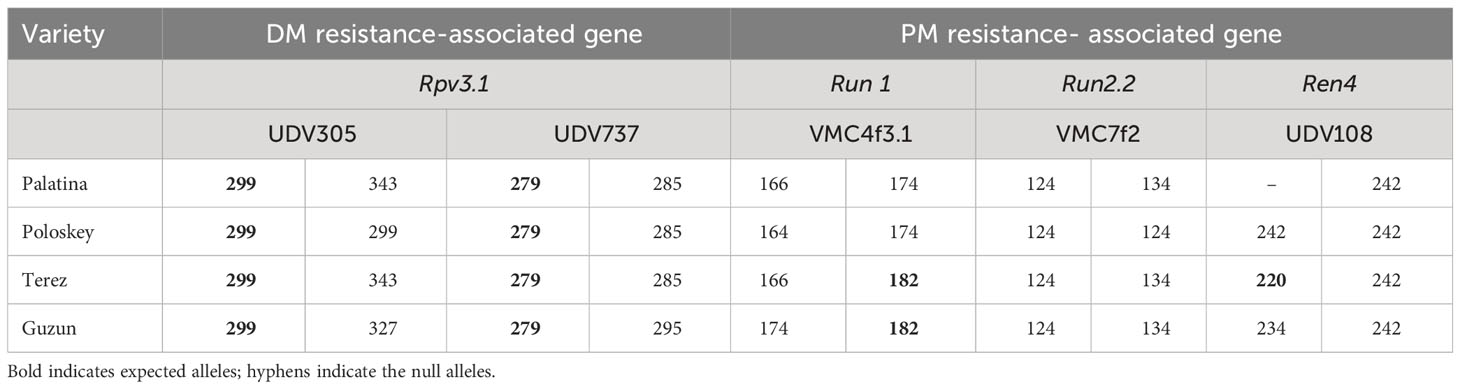
Table 3 Profiles of resistance obtained on parental varieties to confirm the presence of loci of resistance to DM and PM.
2.5 Evaluation of selected vines in the field
The selected progenies were propagated in 2016 and evaluated in a comparison field made in 2017 using 24 plants for each genotype according to the comparison criteria required for registration (Ministerial Decree n. 489243, Ministro delle Politiche Alimentari e Forestali, 30/09/2021). Vines were grown in the field for 3 years and then phenotyped in the field for seedlessness and for the important commercial traits of grape color and aroma. Putative seedless vines were transplanted into the field for phenotypic trait evaluation on each vine. After 3 years, seedlessness was assessed on 100 berries per vine according to Bergamini et al. (2013) and a three-class seed scale: aborted or vestigial (C1), herbaceous (C2), and woody (C3). Resistance to DM and PM was assessed during the highest disease pressure using the susceptible variety Italia as a control. Each vine was examined for symptoms classified according to the OIV455-1 (OIV, 1984) on a scale from 0 to 9 as follows: 1. Very low resistance: leaf with dense sporulation over the entire leaf surface and on more than 75% of the plants. 3. Low resistance: dense sporulation over the 65%–100% of the leaf surface, 50%–75% of the plants affected. 5. Medium resistance: sporulation over the 25%–65% of the leaf surface, 36%–50% of the plants affected; 7. Strong resistance: scant sporulation over the 5%–25% of the leaf surface, 25%–35% of the plants affected; 9. Very strong resistance: sporulation on 0%–5% of the leaf surface, <25% of the plants affected. On all the progenies, “berry color” (white, pink, red, black, neutral) and “berry flavor” (aromatic, neutral foxy) were also noted.
3 Results
3.1 Crosses
During the 5-year program, 109 crosses were successfully carried out by pollinating seeded varieties with seedless varieties yielding a total of 121,723 seeds. An average of 1,077 seeds per cross were obtained, ranging from 90 (Italia × AP28) to 4,600 (Red Globe × AP20). This combination also had the highest number of seeds per berry (2.6; average 1.5) (Supplementary Table 2).
3.2 Seed vernalization, germination, and plant development
All collected seeds were sent for vernalization during which some die-offs occurred. During vernalization, the average loss was 12.3%, but very high losses were observed in cross Victoria × AP36 (74.8), Cardinal × AP28 (62%), and Emperor × AP28 (69.3%) (Supplementary Table 2; Figure 2).
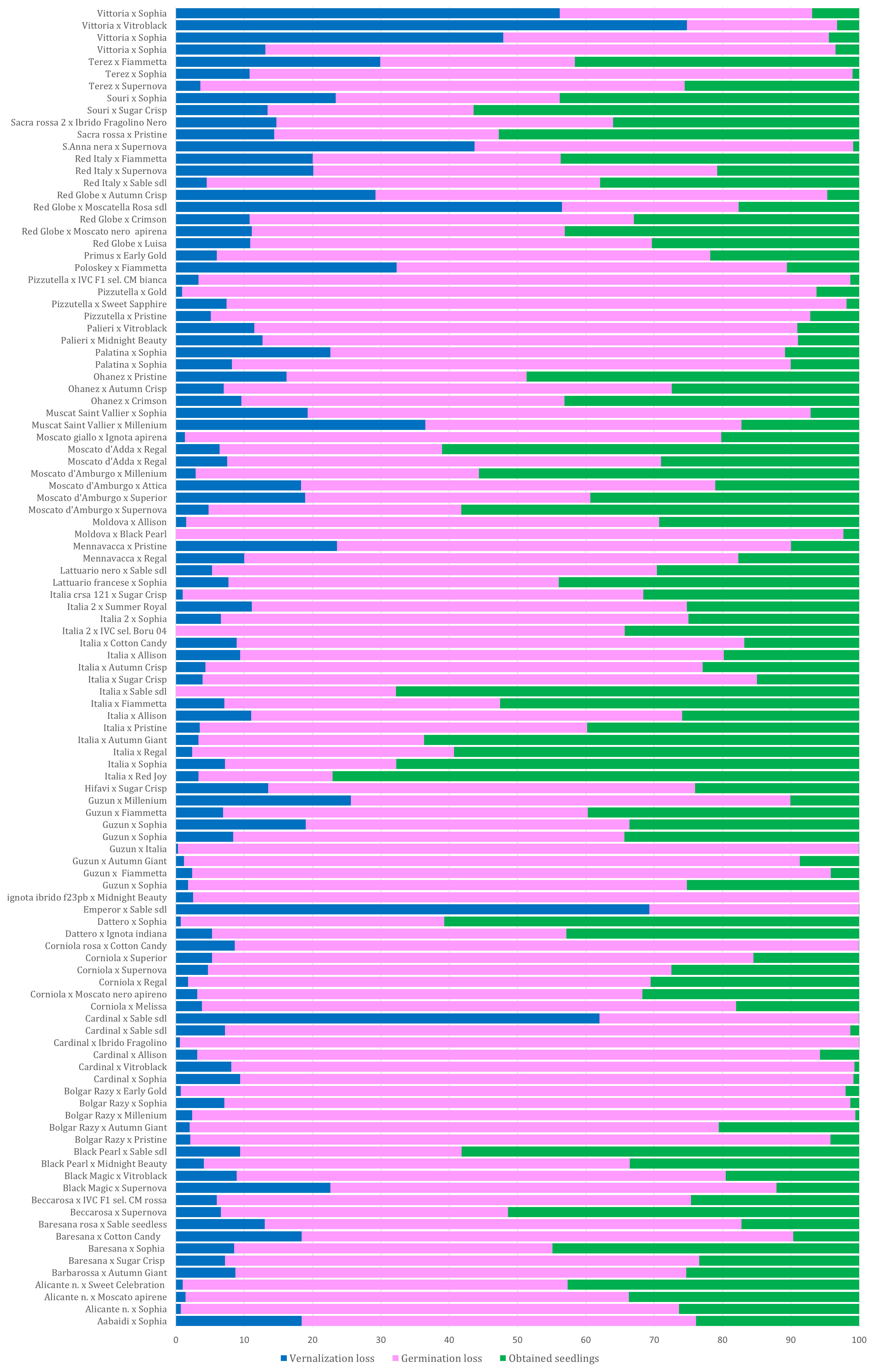
Figure 2 Percentage of seed loss observed during vernalization and germination for each cross; the percentage of viable seedlings transferred to the greenhouse is shown in green. The squares with a solid line indicate some of the best crosses; the dotted squares indicate some of the worst crosses. Overall, the crosses with cultivars Victoria and Red Globe showed losses >50%, while very low losses were observed in the progenies of cultivars Italia (× AP24, 3.3%; × AP23, 3.5%; × AP30, 3.9%; × AP29, 7.2%; × AP25, 2.4%; × AP4, 3.3%) as well as cultivars ALICANTE, BOLGAR RAZY, CORNIOLA, and GUZUN (Supplementary Table 2; Figure 2).
A total of 103,119 seeds were brought into the greenhouse for germination and acclimatization. During these stages, a further average loss of 62% was observed reducing the number of seedlings to 32,638 (Supplementary Table 2). The highest losses were observed in the progeny of varieties, such as CARDINAL, BOLGAR RAZY, MOLDOVA, PIZZUTELLA, and Victoria, as female plants. In contrast, the lowest losses were observed in progenies of ITALIA, SOURI, SACRA ROSSA, RED ITALY, RED GLOBE, DATTERO, MOSCATO D’AMBURGO, among others (see Figure 2). Progenies from the crosses BOLGAR RAZY × AP19, GUZUN × ITALIA, IGNOTA IBRIDO F23PB × AP18, CORNIOLA ROSA × AP7, and EMPEROR × AP28 were cleared (Supplementary Table 2; Figure 2). An effect of the combination “female × pollen donor” was observed in the percentage of progeny surviving in the different developmental stages. For example, a few progenies from crosses of Italia with AP1, AP7, and AP30 survived, while approximately 70% of the progeny from crosses with AP4, AP24, and AP28 survived with a small loss during the germination stage. Losses in the greenhouse and in the field were negligible, as good crop protection and cultivation practices were applied.
3.3 Molecular analysis of seedlessness
Molecular analysis of seedlessness was carried out on 60 selected crosses of the program, including four crosses for resistance to DM and PM. A total of 17,509 individuals were tested with the marker p3_VvAGL11 associated with the major seedlessness gene identifying 8,223 putative seedless individuals corresponding to 47% of the total progeny (Supplementary Table 3). This, matched with the expected segregation rate of 1:1 for crosses between seeded parents (woody seeds, homozygous at the p3_VvAGL11 locus, allele profile 184/184 bp) × seedless parents (herbaceous or aborted seeds, heterozygous at the p3_VvAGL11 locus, allele profile 184/196 bp) resulted in genotypes 184/184 (seeded) and 184/196 (seedless or herbaceous seeds) (Bennici et al., 2019). However, large deviations from the expected values were also observed, such as the 3% in the cross BECCAROSA × AP33 and the 87% in the cross PIZZUTELLA × AP23, as well as in crosses with the varieties CORNIOLA and VITTORIA (Supplementary Table 3). The seedlessness obtained also varied greatly within the crosses of the female variety in relation to the pollinator variety, as, for example, in the crosses of cv Italia where seedless progeny ranged from 31% in the cross with AP7 to 80% in the cross with AP30.
3.4 Molecular analysis of the resistance
The 527 seedless individuals obtained from the four crosses, including a resistant variety as parent, were subjected to MAS for resistance too. The haplotype 299-279, associated with the DM resistance gene Rpv3.1, was found in a total of 206 individuals, while the allele 182, associated with the PM resistance gene Run1, was observed in 22 individuals (Table 4; Supplementary Table 4). None of the resistant progeny to PM was obtained in crosses PALATINA × AP33 and POLOSKEY × AP32, while pyramidization of the two resistances was achieved in 20 individuals obtained from the crosses GUZUN × AP28 and TEREZ × AP4 (Table 4; Supplementary Table 4).
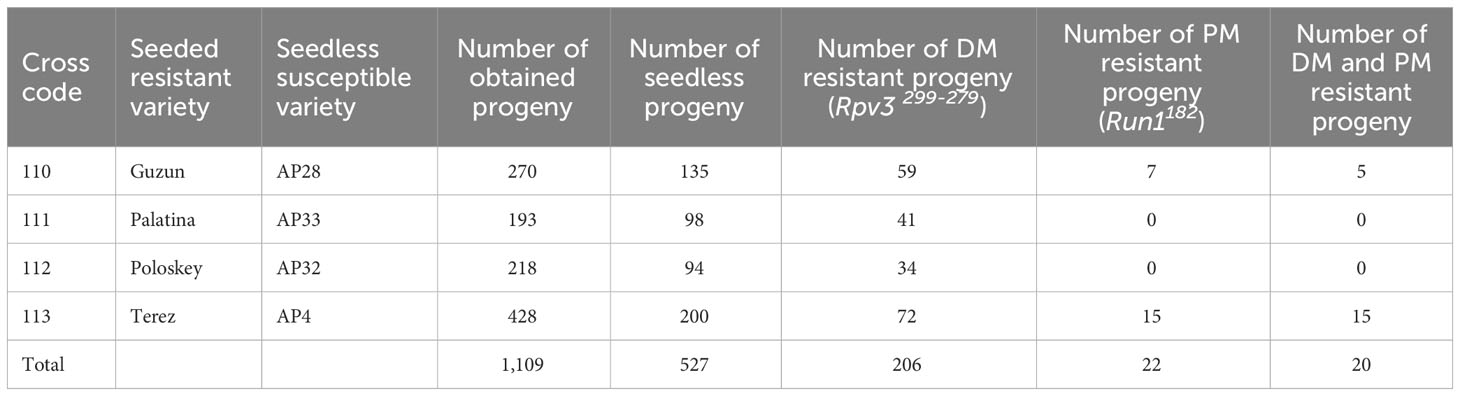
Table 4 Summary of the results obtained in the grapevine cross-program for pyramidation of seedlessness and resistance to DM and PM.
3.5 In-field phenotypic evaluations
The progenies selected just for seedlessness were propagated in 2016 and evaluated in a comparison field made in 2017 using 24 plants for each genotype according to the criteria required for registration (see Material and Methods). Overall, seedlessness was confirmed in 79% of the vines, while 21% of the vines carried herbaceous (C1) or woody seeds (C3). In particular, herbaceous seeds (C1) were observed in the progeny of ITALIA × AP29 (14%), MENNAVACCA × AP25 (9%), PIZZUTELLA × AP23 (10%), and PIZZUTELLA × AP35 (15%), and woody seeds (C3) were found in the progeny of BLACK MAGIC × AP33 (31%), BLACK MAGIC × AP36 (6%), CORNIOLA × AP17 (26%), CORNIOLA × AP25 (52%), ITALIA × AP24 (13%), MENNAVACCA × AP25 (26%), MOSCATO D’ADDA × AP25 (37%), and MOSCATO GIALLO × AP13 (36%) (Supplementary Table 3). As for color, the breeding program produced 55.1% white, 3.6% pink, 24.6% red, and 4.1% black vines (Supplementary Table 5), with recombination occurring in 34 out of 56 crosses (Figure 3), particularly in crosses with BLACK MAGIC, MOSCATO, and RED GLOBE.
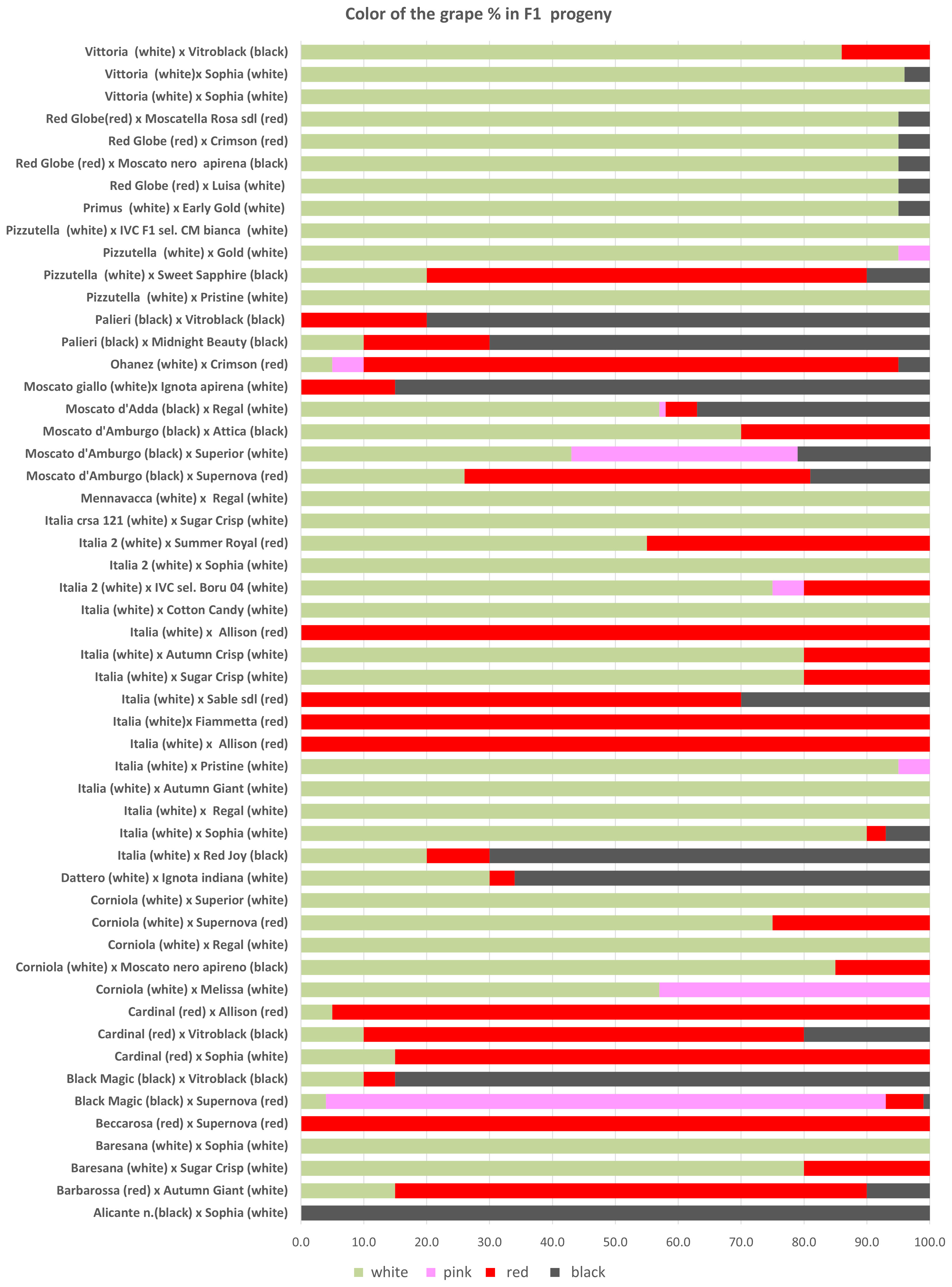
Figure 3 The percentages of genotypes with different berry color observed in F1 progenies obtained in the crosses.
In terms of flavor, 48.2% of the offspring were neutral, 17.9% were aromatic, while the remaining 33.9% were partly aromatic and partly neutral (Figure 4).
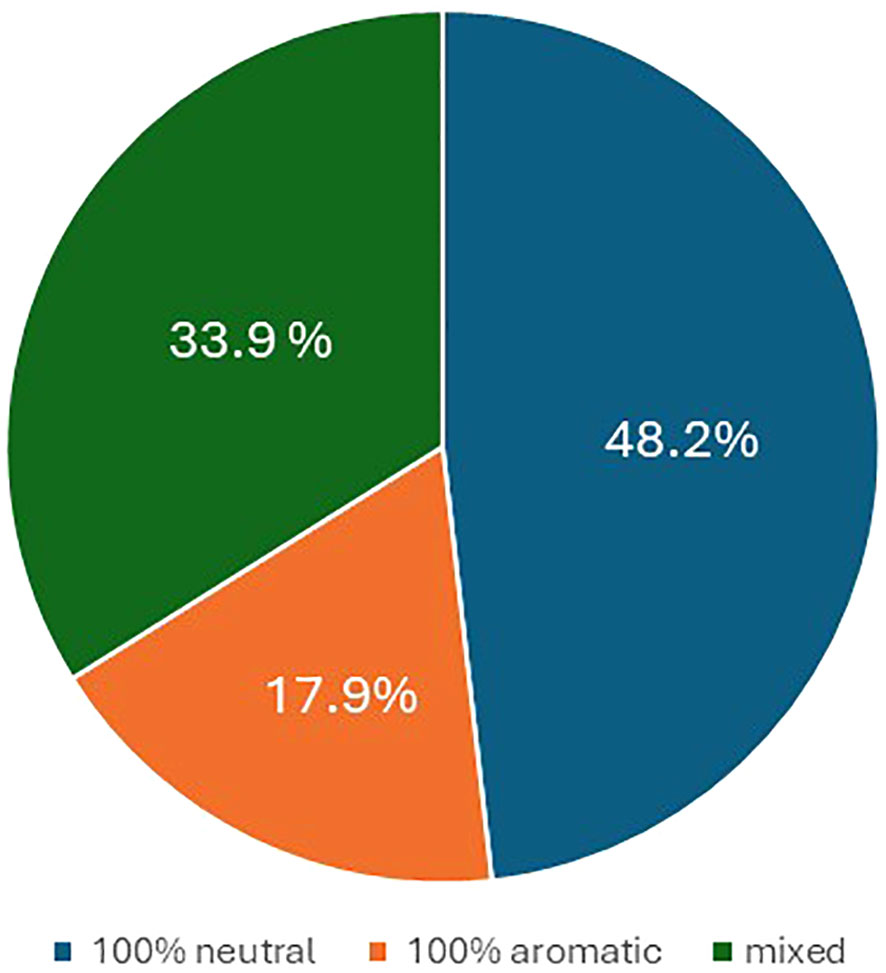
Figure 4 Pie chart illustrating the percentage of progenies with berry flavor neutral (blue), aromatic (orange), and mixed (gray).
The seedless progenies of the crosses also selected for DM and PM resistance were scored in the field during the peak disease pressure using a scale from 0 to 9. The results generally confirmed the molecular data for both DM and PM and showed medium-high score on the resistance scale, although some contradictory data were also found (Supplementary Table 4). Further investigation is needed both in molecular screening with more markers and markers for additional loci and in the field for symptoms. Indeed, the absence of R loci stands solely for the loci analyzed and leaves open the possibility that other markers may be useful for detecting additional sources of resistance.
4 Discussion
In Apulia, table grapes are of crucial economic importance, and growers demand good table grape varieties that are adapted to the local climate and combine seedlessness with resistance to destructive diseases such as powdery mildew and downy mildew. To meet these expectations, the Apulian winegrower’s association “Italian Variety Club” launched an extensive crossing program in 2015 to combine seedlessness with durable resistance in new grape varieties. The plan comprised 113 crosses, which included seedless and elite varieties as parents, as well as four varieties with resistance to PM and DM diseases. A total of 121,723 seeds were collected with the crossing program, but vernalization resulted in an average loss of 12.1% of the seeds, which proved to be a very critical phase. Nevertheless, the results were encouraging considering that the germination capacity of grapevine seeds is generally approximately 30%–50% (Gao et al., 2014; Wang et al., 2022). Seed germination is one of the most important determinants of reproductive performances and depends on various factors [8], such as stratification time, amount of moisture, mold development, amount of inhibitory phenolic compounds, seed hardness, and cultivation conditions (Lin et al., 2009; Zhang et al., 2009). A complete understanding of dormancy, germination, and seedling formation in grape seeds is lacking, and more comprehensive studies are needed for perennial species (Leida et al., 2010; Graeber et al., 2012). Indeed, large differences were found between crosses, with the progeny of cv Italia showing only minor losses, while the progeny of varieties, such as VICTORIA and RED GLOBE, reached 74.8%. Heavy losses (up to 59%) were also observed in seedlings in the first stages of growth, especially in the progeny of cv. CARDINAL, BOLGAR REZY, MOLDOVA, and PIZZUTELLA, while the progeny of cv. ITALIA, SOURI, and SACRA ROSSA were hardly affected. Here, too, diseases, unsuitable growing conditions, and transplanting methods can have a major influence on the establishment rate of the seedlings, but the variety also seems to play a decisive role (Butiuc and Coste, 2023). Overall, the Italia variety provided very encouraging results. Italia is a late-maturing table grape bred in 1911 from a cross of BICANE × MUSCAT AMBURG. It is the most important Apulian variety and is known for its large bunches and tasty, crunchy berries, as well as its good transportability and storability (Torres et al., 2017). The good performance observed makes this variety a good candidate for table grape breeding programs.
The early application of MAS led to the identification of 7,696 seedless putative plants (49% of the total progeny) of which 79% were confirmed in the field trials indicating a good efficiency of the marker p3_VvAGL11 and its usefulness in long-term, labor-intensive, and expensive grapevine selection programs (Bergamini et al., 2013). However, the lower predictive power of the marker observed in the CORNIOLA and AP25 crosses suggests that alternative/additional modifications at the associated 196-bp locus may be involved in the determinism of seedlessness. A single nucleotide modification in the VvAGL11 gene has been shown to be responsible for seedlessness in V. vinifera (Royo et al., 2018; Ocarez et al., 2020). However, it is very likely that other recombination events and SNPs in the coding region, with small but stable effects, are involved in the complex genetic architecture of apyrenia leading to the failure of the marker (Ocarez et al., 2020; Li et al., 2015). Further characterization of the seedlessness locus and further genetic analyses will help to clarify the reasons for the failure of the marker’s predictive power in crosses with these varieties.
Regarding the berry colors of the progeny, recombination was observed in most crosses. Such variability is not surprising in table grape, a crop in which crosses between different varieties are widespread resulting in extensive gene recombination. In grapevine, differences in berry color are generally due to somatic mutations associated with the VvMybA gene family, which is highly polymorphic and determines the variation in anthocyanin content in berries (Lijavetzky et al., 2006; Fournier-Level et al., 2009; Carbonell-Bejerano et al., 2017; Ferreira et al., 2020; Röckel et al., 2022). Here, too, it is likely that minor loci also play a role, which would explain the controversial results especially for the varieties BLACK MAGIC, MOSCATO, and RED GLOBE. On the other hand, these varieties seem to be a very good resource for increasing color variability in table grape breeding programs. As far as the berry flavor trait is concerned, half of the crosses between neutral female and muscadine male resulted in 49% of neutral offspring, 18% in aromatic offspring, and 34% in partly aromatic and partly neutral progeny. In grapevine, monoterpenes are the key compounds responsible for the Muscat flavor, and a major QTL was co-localized with the 1-deoxy-D-xylulose 5-phosphate synthase (VvDXS) gene, encoding for the 1-deoxy-D-xylulose 5-phosphate synthase enzyme, which is involved in the plastidial pathway of terpene biosynthesis (Battilana et al., 2009; Emanuelli et al., 2010; Li et al., 2023). Our results are difficult to interpret, and further genetic studies of this trait and its heritability will be carried out in the future.
The crosses carried out to obtain the pyramiding of seedlessness and resistance to DM and PM resulted in 230 putative resistant seedless genotypes, 20 of which were resistant to both diseases. The preliminary field observations yielded results that were generally consistent with molecular selection with offspring showing medium-to-high scores of resistance. However, some inconsistencies were also recorded, which makes further evaluation crucial in the coming years. It will also be necessary to extend the analysis to other resistance alleles involved in PM such as Ren3 and Ren9 (Zini et al., 2019). The pedigree of parental varieties, such as PÖLÖSKEI MUSKOTÁLY, TERÉZ, and PALATINA, created in Hungary in 1957 from back-crosses of Seyve-Villard and other French–American hybrids, lack information on the origin of their resistance and should be integrated. This would help to establish an efficient protocol for the early identification of resistance genes in V. vinifera, and would facilitate the lengthy and costly process of grapevine breeding (Hoffmann et al., 2007; Tóth-Lencsés et al., 2015).
5 Conclusions
In recent years, a more sustainable and environmentally friendly management of table grape production has also become possible through the use of resistant varieties. The use of multiple sources of resistance and MAS facilitates the pyramiding of key resistance genes. The breeding program described here has enabled the selection of 10 new selections (Supplementary Figure 1) that combine seedlessness with phenotypically important commercial traits such as color and aroma. These selections are in the process of patent registration in the National Register of Vine Varieties. Others will be the subject of an evaluation plan in the coming years, which will lead to the selection of varieties that meet the ever-changing requests of producers and consumers, also with regard to the new diseases that continue to appear in the Mediterranean area, such as Xylella fastidiosa fastidiosa, the causal agent of Pierce’s disease, which has recently also appeared in Puglia (https://www.osservatoriofitosanitario.regione.puglia.it/). The availability of new tools, such as genomic selection approaches using genome-wide molecular markers, will certainly be better suited to capture the complex genetic architecture of resistance and other quantitative traits. The implementation of these approaches in grape breeding will provide new opportunities to promote more sustainable and environmentally friendly viticulture.
Data availability statement
The original contributions presented in the study are included in the article/Supplementary Material. Further inquiries can be directed to the corresponding authors.
Author contributions
MMM: Investigation, Methodology, Validation, Writing – original draft, Writing – review & editing. LP: Data curation, Investigation, Writing – original draft. CP: Data curation, Investigation, Writing – review & editing. VR: Data curation, Writing – review & editing. DB: Investigation, Validation, Writing – review & editing. IM: Visualization, Writing – review & editing. PLN: Validation, Visualization, Writing – review & editing. CM: Funding acquisition, Methodology, Validation, Writing – review & editing, Resources.
Funding
The author(s) declare financial support was received for the research, authorship, and/or publication of this article. The program of grapevine breeding was funded by the Italian Variety Club (IVC). This study was carried out within the Agritech National Research Center and received funding from the European Union Next-Generation EU (PIANO NAZIONALE DI RIPRESA E RESILIENZA (PNRR)—MISSIONE 4 COMPONENTE 2, INVESTIMENTO 1.4—D.D. 1032 17/06/2022, CN00000022). This study was supported by the BANDO Joint Project 2018 from the University of Verona.
Conflict of interest
Authors LP, CP were employed by the Spin Off of the University of Bari SINAGRI S.r.l.; authors LP, CP, VR were employed by company Rete Italian Variety Club (IVC). CM is a founder member of the Spin Off of the University of Bari SINAGRI S.r.l.; CM and MMM were scientific consultants in kind for the company Rete Italian Variety Club (IVC).
The remaining authors declare that the research was conducted in the absence of any commercial or financial relationships that could be construed as a potential conflict of interest.
The authors declare that this study received funding from Rete Italian Variety Club (IVC). The funder had the following involvement in the study: study design, data collection and analysis.
Publisher’s note
All claims expressed in this article are solely those of the authors and do not necessarily represent those of their affiliated organizations, or those of the publisher, the editors and the reviewers. Any product that may be evaluated in this article, or claim that may be made by its manufacturer, is not guaranteed or endorsed by the publisher.
Supplementary material
The Supplementary Material for this article can be found online at: https://www.frontiersin.org/articles/10.3389/fpls.2024.1379642/full#supplementary-material
References
Anderson, K. (2013). Which Winegrape Varieties are Grown Where? (Adelaide, Australia: University of Adelaide Press). doi: 10.20851/j.ctt1t304tz
Azuma, A., Kobayashi, S., Mitani, N., Shiraishi, M., Yamada, M., Ueno, T., et al. (2008). Genomic and genetic analysis of myb-related genes that regulate anthocyanin biosynthesis in grape berry skin. Theor. Appl. Genet. 117 (6), 1009–1019. doi: 10.1007/s00122-008-0840-1
Battilana, J., Costantini, L., Emanuelli, F., Sevini, F., Segala, C., Moser, S., et al. (2009). The 1-deoxy-D: -xylulose 5-phosphate synthase gene co-localizes with a major QTL affecting monoterpene content in grapevine. Theor. Appl. Genet. 118, 653–669. doi: 10.1007/s00122-008-0927-8
Bellin, D., Peressotti, E., Merdinoglu, D., Wiedemann-Merdinoglu, S., Adam-Blondon, A. F., Cipriani, G., et al. (2009). Resistance to Plasmopara viticola in grapevine ‘Bianca’ is controlled by a major dominant gene causing localis ed necrosis at the infection site. Theor. Appl. Genet. 120, 163–176. doi: 10.1007/s00122-009-1167-2
Bennici, S., Di Guardo, M., Distefano, G., La Malfa, S., Puglisi, D., Arcidiacono, F., et al. (2019). Influence of the genetic background on the performance of molecular markers linked to seedlessness in table grapes. Sci. Hortic. 252, 316–323. doi: 10.1016/j.scienta.2019.03.060
Bergamini, C., Cardone, M. F., Anaclerio, A., Perniola, R., Pichierri, A., Genghi, R., et al. (2013). Validation assay of p3_VvAGL11 marker in a wide range of genetic background for early selection of stenospermocarpy in Vitis vinifera. L. Mol. Biotechnol. 54, 1021–1030. doi: 10.1007/s12033-013-9654-8
Bove, F., Bavaresco, L., Caffi, T., Rossi, V. (2019). Assessment of resistance components for improved phenotyping of grapevine varieties resistant to downy mildew. Front. Plant Sci. 10. doi: 10.3389/fpls.2019.01559
Buonassisi, D., Colombo, M., Migliaro, D., Dolzani, C., Peressotti, E., Mizzotti, C., et al. (2017). Breeding for grapevine downy mildew resistance: a review of “omics” approaches. Euphytica 213, 103. doi: 10.1007/s10681-017-1882-8
Butiuc-Keul, A., Coste, A. (2023). Biotechnologies and strategies for grapevine improvement. Horticulturae 9, 62. doi: 10.3390/horticulturae9010062
Carbonell-Bejerano, P., Royo, C., Torres-Pérez, R., Grimplet, J., Fernandez, L., Martínez, J., et al. (2017). Catastrophic unbalanced genome rearrangements cause somatic loss of berry color in grapevine. Plant Physiol. 175, 786–801. doi: 10.1104/pp.17.00715
Chen, M., Brun, F., Raynal, M., Makowski, D. (2020). Delaying the first grapevine fungicide application reduces exposure on operators by half. Sci. Rep. 10, 6404. doi: 10.1038/s41598-020-62954-4171
Cobb, J. N., Biswas, P. S., Platten, J. D. (2019). Back to the future: revisiting MAS as a tool for modern plant breeding. Theor. Appl. Genet. 132, 647–667. doi: 10.1007/s00122-018-3266-4
Di Gaspero, G., Copetti, D., Coleman, C., Castellarin, S. D., Eibach, R., Kozma, P., et al. (2012). Selective sweep at the Rpv3 locus during grapevine breeding for downy mildew resistance. Theor. Appl. Genet. 124, 277–286. doi: 10.1007/s00122-011-1703-8
Di Rienzo, V., Fanelli, V., Miazzi, M. M., Savino, V., Pasqualone, A., Summo, C., et al. (2017). A reliable analytical procedure to discover table grape DNA adulteration in industrial wines and musts. Acta Hortic. X Int. Symp. Grapevine Physiol. Biotechnol. 1188-49, 365–370. doi: 10.17660/ActaHortic.2017.1188.49
Eibach, R., Zyprian, E., Welter, L., Töpfer, R. (2007). The use of molecular markers for pyramiding resistance genes in grpevine breeding. Vitis 46, 120–124. doi: 10.5073/VITIS
Emanuelli, F., Battilana, J., Costantini, L., Le Cunff, L., Boursiquot, J. M., This, P., et al. (2010). A candidate gene association study on muscat flavor in grapevine (Vitis vinifera L.). BMC Plant Biol. 10, 241. doi: 10.1186/1471-2229-10-241
Feechan, A., Kocsis, M., Riaz, S., Zhang, W., Gadoury, D. M., Walker, M. A., et al. (2015). Strategies for RUN1 deployment using RUN2 and REN2 to manage grapevine powdery mildew informed by studies of race specificity. Phytopathology 105, 1104–1113. doi: 10.1094/PHYTO-09-14-0244-R
Ferreira, S. S., Silva, P., Silva, A. M., Nunes, F. M. (2020). Effect of harvesting year and elderberry cultivar on the chemical composition and potential bioactivity: A three-year study. Food Chem. 302, 125366. doi: 10.1016/j.foodchem.2019.125366
Foria, S. (2015). The Rpv3 locus in grapevine: DNA variation and relevance for conventional breeding. Udine Italy: University of Udine.
Foria, S., Magris, G., Morgante, M., Di Gaspero, G. (2018). The genetic background modulates the intensity of Rpv3-dependent downy mildew resistance in grapevine. Plant Breed. 137, 220–228. doi: 10.1111/pbr.12564
Fournier-Level, A., Le Cunff, L., Gomez, C., Doligez, A., Ageorges, A., Roux, C., et al. (2009). Quantitative genetic bases of anthocyanin variation in grape (Vitis vinifera L. ssp. sativa) berry: a quantitative trait locus to quantitative trait nucleotide integrated study. Genetics 183, 1127–1139. doi: 10.1534/genetics.109.103929
Fröbel, S., Zyprian, E. (2019). Colonization of different grapevine tissues by plasmopara viticola-A histological study. Front. Plant Sci. 24. doi: 10.3389/fpls.2019.00951
Gao, K., Li, H., Nan, H. (2014). Study on chilling requirements and germination characteristics of Chardonnay grape seeds. North. Fruits 04, 4–6. doi: 10.3969/j.issn.1001-5698.2014.04.002
Graeber, K., Nakabayashi, K., Miatton, E., Leubner-Metzger, G., Soppe, W. J. (2012). Molecular mechanisms of seed dormancy. Plant Cell Environ. 35 (10), 1769–1786. doi: 10.1111/j.1365-3040.2012.02542.x
Hajjeh, H., Miazzi, M., De Guido, M. A., Faretra, F. (2005). Specific SCAR primers for the "flag shoot" and "ascospore" biotypes of the grape powdery mildew fungus Erysiphe necator. J. Pl. Path. 87, 71–74. doi: 10.4454/JPP.V87I1.899
Hajjeh, H., Miazzi, M., Faretra, F. (2008). Overwintering of erysiphe necator schw. in southern Italy. J. Plant Pathol. 89, 323–330. doi: 10.4454/JPP.V90I2.668
Harst, M., Cobanov, B., Hausmann, L., Eibach, R., Töpfer, R. (2009). Evaluation of pollen dispersal and cross pollination using transgenic grapevine plants. Environ. Biosafety Res. 8, 87 –99. doi: 10.1051/ebr/2009012
Hoffmann, S., Cindric, P., Kozma, P. (2007). “Breeding resistante cultivars to downy and powdery mildew,” in Agricultural and Food Sciences (Research Institute for Viticulture & Oenology, Faculty of Agriculture, Novi Sad, Serbia Pázmány Péter u. 4, 7634 Pécs, Hungary).
Hoffmann, S., Di Gaspero, G., Kovacs, L., Howard, S., Kiss, E., Galbacs, Z., et al. (2008). Resistance to Erysiphe necator in the grapevine “Kishmish vatkana” is controlled by a single locus through restriction of hyphal growth. Theor. Appl. Genet. 116, 427–438. doi: 10.1007/s00122-007-0680-4
Ibáñez, J., Carreño, J., Yuste, J., Martínez-Zapater, J. M. (2015). Grapevine breeding and clonal selection programmes in Spain. Grapevine breeding programs for the wine industry. Food Sci., 183–209. doi: 10.1016/B978-1-78242-075-0.00009-0
Karn, A., Zou, C., Brooks, S., Fresnedo-Ramírez, J., Gabler, F., Sun, Q., et al. (2021). Discovery of the REN11 locus from Vitis aestivalis for stable resistance to grapevine powdery mildew in a family segregating for several unstable and tissue-specific quantitative resistance loci. Front. Plant Sci. 12. doi: 10.3389/fpls.2021.733899
Leida, C., Terol, J., Martí, G., Agustí, M., Llácer, G., Badenes, M. L., et al. (2010). Identification of genes associated with bud dormancy release in prunus persica by suppression subtractive hybridization. Tree Physiol. 30 (5), 655–666. doi: 10.1093/treephys/tpq008
Li, C., Erwin, A., Pap, D., Coleman, C., Higgins, A. D., Kiss, E., et al. (2012). Selection for Run1-Ren1 dihybrid grapevines using microsatellite markers. Am. J. Enol. Vitic 64, 152–155. doi: 10.5344/ajev.2012.12060
Li, Y., He, L., Song, Y., Zhang, P., Chen, D., Guan, L., et al. (2023). Comprehensive study of volatile compounds and transcriptome data providing genes for grape aroma. BMC Plant Biol. 23, 171. doi: 10.1186/s12870-023-04191-1
Lijavetzky, D., Ruiz-García, L., Cabezas, J. A., De Andrés, M. T., Bravo, G., Ibáñez, A., et al. (2006). Molecular genetics of berry colour variation in table grape. Mol. Genet. Genomics 276, 427–435. doi: 10.1007/s00438-006-0149-1
Lin, L., Zhang, Y., Huang, Y., Peng, H., Hu, J. (2009). A comparative study on seed germination in different grape cultivars. Guangxi Agric. Sci. 40, 1590–1592. doi: 10.3969/j.issn.2095-1191.2009.12.021
Lorenz, D. H., Eichhorn, K. W., Bleiholder, H., Klose, R., Meier, U., Weber, E. (1995). Growth Stages of the Grapevine: phenological growth stages of the grapevine (Vitis vinifera L. ssp. vinifera) - Codes and descriptions according to the extended BBCH scale. Aust. J. Grape Wine Res. 1, 100–103. doi: 10.1111/j.1755-0238.1995.tb00085.x
Lykogianni, M., Bempelou, E., Karamaouna, F., Aliferis, K. A. (2021). Do pesticides promote or hinder sustainability in agriculture? The challenge of sustainable use of pesticides in modern agriculture. Sci. Total Environ. 795, 148625. doi: 10.1016/j.scitotenv.2021.148625
Matus, J. T., Loyola, R., Vega, A., Peña-Neira, A., Bordeu, E., Arce-Johnson, P., et al. (2009). Post-veraison sunlight exposure induces MYB-mediated transcriptional regulation of anthocyanin and flavonol synthesis in berry skins of vitis vinifera. J. Exp. Bot. 60 (3), 853–867. doi: 10.1093/jxb/ern336
Mejía, N., Soto, B., Guerrero, M., Casanueva, X., Houel, C., Miccono, M. A., et al. (2011). Molecular, genetic and transcriptional evidence for a role of VvAGL11 in stenospermocarpic seedlessness in grapevine. BMC Plant Biol. 11, 57. doi: 10.1186/1471-2229-11-57
Merdinoglu, D., Schneider, C., Prado, E., Wiedemann-Merdinoglu, S., Mestre, P. (2018). Breeding for durable resistance to downy and powdery mildew in grapevine. OENO One 52, 203–209. doi: 10.20870/oeno-one.2018.52.3.2116
Miazzi, M. M., Hajjeh, H. R. (2011). Differential sensitivity to triadimenol of erysiphe necator isolates belonging to different genetic groups. J. Pl. Pathol. 93, 729–735.
Miazzi, M. M., D’Agostino, N., di Rienzo, V., Venerito., P., Savino, V. N., Fucilli, V., et al. (2020). Marginal grapevine germplasm from Apulia (Southern Italy) represents an unexplored source of genetic diversity. Agronomy 10, 563. doi: 10.3390/agronomy10040563
Miazzi, M. M., Hajjeh, H., Faretra, F. (2008). Occurrence and distribution of “flag shoot” and “ascospore” biotypes in populations of Erysiphe necator Schw. In Southern Italy. J. Pl. Pathol. 90, 563–573. doi: 10.4454/JPP.V90I3.701
Miazzi, M., Natale, P., Pollastro, S., Faretra, F. (1997). Handling of the biotrophic pathogen Uncinula necator (Schw.) Burr. under laboratory conditions and observation on its mating system. J. Pl. Pathol. 78, 71–77. Available at: https://www.jstor.org/stable/41999052.
Ocarez, N., Jiménez, N., Núñez, R., Perniola, R., Marsico, A. D., Cardone, M. F., et al. (2020). Unraveling the deep genetic architecture for seedlessness in grapevine and the development and validation of a new set of markers for vviAGL11-based gene-assisted selection. Genes 11, E151. doi: 10.3390/genes11020151
OIV. (1984). International Organisation of Vine and Wine. (Paris). Available at: https://www.oiv.int/.
Otto, T., Botelho, R., Biasi, L., Milijić, U., Correia, A. C., Jordão, A. M. (2022). “Adaptability of Different International Grape Varieties in Diverse Terroirs: Impact on Grape and Wine Composition,” in Recent Advances in Grapes and Wine Production - New Perspectives for Quality Improvement. Eds. Jordão, A., Botelho, R., Miljic, U. (IntechOpen, London). doi: 10.5772/intechopen.108204
Pathania, A., Rialch, N., Sharma, P. N. (2017). “Marker-assisted selection in disease resistance breeding: a boon to enhance agriculture production,” in Current developments in biotechnology and bioengineering: crop modification, nutrition, and food production. Eds. Dubey, S. K., Pandey, A., Sangwan, R. S. (Amsterdam, Netherlands: Elsevier), 187–213. doi: 10.1016/B978-0-444-63661-4.00009-8
Pedneault, K., Provost, C. (2016). Fungus resistant grape varieties as a suitable alternative for organic wine production: Benefits, limits, and challenges. Sci. Horticult. 208, 57–77. doi: 10.1016/j.scienta.2016.03.016
Pomarici, E., Vecchio, R. (2019). Corrigendum to “Will sustainability shape the future wine market?” Wine Econ. Policy. 8, 216. doi: 10.1016/j.wep.2019.05.001
Possamai, T., Wiedemann-Merdinoglu, S. (2022). Phenotyping for QTL identification: A case study of resistance to Plasmopara viticola and Erysiphe necator in grapevine. Front. Plant Sci. 13. doi: 10.3389/fpls.2022.930954
Prazzoli, M. L., Lorenzi, S., Perazzolli, M., Toffolatti, S., Failla, O., Grando, M. S. (2019). “Identification of disease resistance-linked alleles in Vitis vinifera germplasm,” in BIO Web of Conferences, Vol. 13. CO.NA.VI. 2018 - 7° Convegno Nazionale di Viticoltura. (EDP Sciences), 01004. doi: 10.1051/bioconf/20191301004
Ramming, D. W., Gabler, F., Smilanick, J., Cadle-Davidson, M., Barba, P., Mahanil, S., et al. (2011). A single dominant locus, Ren4, confers rapid non-race-specific resistance to grapevine powdery mildew. Phytopathology 101, 502–508. doi: 10.1094/PHYTO-09-10-0237
Reynolds, A. G. (2015). “Grapevine breeding in France - a historical perspective,” in Grapevine breed programs for the wine industry publisher. Ed. A. G. Reynolds (Amsterdam, Netherlands: Woodhead Publishing), 65–76. doi: 10.1016/B978-1-78242-075-0.00004-1
Riaz, S., Tenscher, A. C., Ramming, D. W., Walker, M. A. (2011). Using a limited mapping strategy to identify major QTLs for resistance to grapevine powdery mildew (Erysiphe necator) and their use in marker-assisted breeding. Theor. Appl. Genet. 122, 1059–1073. doi: 10.1007/s00122-010-1511-6
Royo, C., Torres-Pérez, R., Mauri, N., Diestro, D., Cabezas, J. A., Marchal, C., et al. (2018). The major origin of seedless grapes is associated with a missense mutation in the MADS-box gene VviAGL11. Plant Physiol. 177, 1234–1253. doi: 10.1104/pp.18.00259
Röckel, F., Moock, C., Schwander, F., Maul, E., Töpfer, R., Hausmann, L. (2022). A 69 kbp deletion at the berry color locus is responsible for berry color recovery in vitis vinifera l. cultivar ‘Riesling rot’. Int. J. Mol. Sci. 23, 3708. doi: 10.3390/ijms23073708
Ruehl, E., Schmid, J., Eibach, R., Töpfer, R. (2015). Grapevine breeding programmes in Germany. Grapevine Breed Prog. Wine Ind., 77–101. doi: 10.1016/B978-1-78242-075-0.00005-3
Sanzani, S. M., Miazzi, M. M., Di Rienzo, V., Fanelli, V., Gambacorta, G., Taurino, M. R., et al. (2016). A rapid assay to detect toxigenic Penicillium spp. Contamination in wine and musts. Toxins 8, E235. doi: 10.3390/toxins8080235
Schneider, C., Spring, J. L., Onimus, C., Prado, E., Verdenal, T., Lemarquis, G., et al. (2019). “French-Swiss collaborative program for breeding new grapevine varieties durably resistant to downy and powdery mildew,” in BIO Web of Conf. 42nd World Congress of Vine and Wine. (EDP Sciences). doi: 10.1051/bioconf/20191501018
Somogyi, E., Lazar, J., Bordor, P., Kaszab, T. (2020). Colour of grapevine (Vitis vinifera L.) accessions influenced by the length of cold storage. Progress. Agric. Eng. Sci. 16 (S2). doi: 10.1556/446.2020.20013
Spadoni, A., Sion, S., Gadaleta, S., Savoia, M. A., Piarulli, L., Fanelli, V., et al. (2019). A simple and rapid method for genomic DNA extraction and microsatellite analysis in tree plants. J. Agric. Sci. Technol. 21, 1215–1226. doi: 10.1016/J.SCIENTA.2008.03.024
Töpfer, R., Hausmann, L., Eibach, R. (2011). “Molecular Breeding,” in Genetics, genomics and breeding of grapes. Eds. Adam-Blondon, A. F., Martinez-Zapater, J. M., Kole, C. (Science Publishers, Enfield). doi: 10.1201/b10948
Torres, R., Ferrara, G., Soto, F., López, J. A., Sanchez, F., Mazzeo, A., et al. (2017). Effects of soil and climate in a table grape vineyard with cover crops. Irrigation management using sensors networks. Cienc. Tec. Vitiv. 32, 72–81. doi: 10.1051/ctv/20173201072
Tóth-Lencsés, A. K., Kozma, P., Szoke, A., Kerekes, A., Veres, A., Kiss, E. (2015). Parentage analysis in Hungarian grapevine cultivars of 'Seibel'-'Seyve-Villard'origin. Vitis 54, 27–29.
Venuti, S., Copetti, D., Foria, S., Falginella, L., Hoffmann, S., Bellin, D., et al. (2013). Historical introgression of the downy mildew resistance gene Rpv12 from the Asian species Vitis amurensis into grapevine varieties. PloS One 8, 4. doi: 10.1371/journal.pone.0061228
Vervalle, J. A., Costantini, L., Lorenzi, S., Pindo, M., Mora, R., Bolognesi, G., et al. (2022). A high-density integrated map for grapevine based on three mapping populations genotyped by the Vitis18K SNP chip. Theor. Appl. Genet. 135, 4371–4390. doi: 10.1007/s00122-022-04225-6
Vezzulli, S., Doligez, A., Bellin, D. (2019). “Molecular mapping of grapevine genes,” in The grape genome (Springer, Cham), 103–136.
Vezzulli, S., Vecchione, A., Stefanini, M., Zulini, L. (2018). Downy mildew resistance evaluation in 28 grapevine hybrids promising for breeding programs in Trentino region (Italy). Eur. J. Plant Pathol. 150, 485–495. doi: 10.1007/s10658-017-1298-2
Wang, Z. L., Hui, M., Shi, X. Q., Wu, D., Wang, Y., Han, X., et al. (2022). Characteristics of the seed germination and seedlings of six grape varieties (V. vinifera). Plants 11, 479. doi: 10.3390/plants11040479
Weinmann, E., Boos, M., Ehret, P., Flubacher, L., Schneider, C., Veith, L. (2019). “Breeding of new disease-tolerant grape varieties–Viticulture in times of climatic change,” in BIO Web of Conferences, Vol. 15. 42nd World Congress of Vine and Wine (EDP Sciences), 01035.
Yıldırım, Z., Atak, A., Akkurt, M. (2019). Determination of downy and powdery mildew resistance of some Vitis spp. Ciec. Téc. Vitiv. 34, 15–24. doi: 10.1051/ctv/20193401015
Zhang, J., Wang, Y., Shi, L., Cheng, L., Li, K. (2009). Comparison of grape seed seedling methods. Sino-Overseas Grapevine Wine. 7, 33–37.
Keywords: table grape, seedlessness, Erysiphe necator, Plasmopara viticola, resistance, marker-assisted selection
Citation: Piarulli L, Pirolo C, Roseti V, Bellin D, Mascio I, La Notte P, Montemurro C and Miazzi MM (2024) Breeding new seedless table grapevines for a more sustainable viticulture in Mediterranean climate. Front. Plant Sci. 15:1379642. doi: 10.3389/fpls.2024.1379642
Received: 31 January 2024; Accepted: 14 March 2024;
Published: 05 April 2024.
Edited by:
Marco Landi, University of Pisa, ItalyReviewed by:
Darko Preiner, University of Zagreb, CroatiaFrancesco Sunseri, Mediterranea University of Reggio Calabria, Italy
Patricia Leao, Brazilian Agricultural Research Corporation (EMBRAPA), Brazil
Ermes Lo Piccolo, University of Pisa, Italy
Copyright © 2024 Piarulli, Pirolo, Roseti, Bellin, Mascio, La Notte, Montemurro and Miazzi. This is an open-access article distributed under the terms of the Creative Commons Attribution License (CC BY). The use, distribution or reproduction in other forums is permitted, provided the original author(s) and the copyright owner(s) are credited and that the original publication in this journal is cited, in accordance with accepted academic practice. No use, distribution or reproduction is permitted which does not comply with these terms.
*Correspondence: Monica Marilena Miazzi, Monicamarilena.miazzi@uniba.it; Cinzia Montemurro, cinzia.montemurro@uniba.it