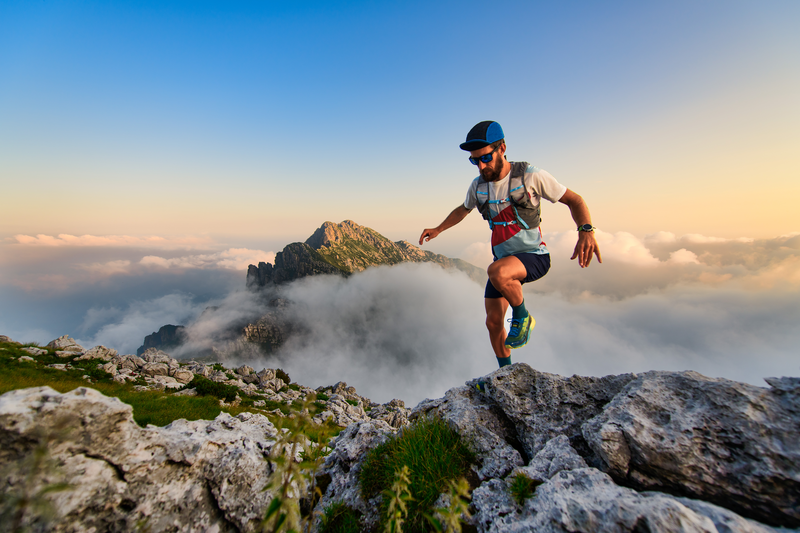
94% of researchers rate our articles as excellent or good
Learn more about the work of our research integrity team to safeguard the quality of each article we publish.
Find out more
OPINION article
Front. Plant Sci. , 16 February 2024
Sec. Photosynthesis and Photobiology
Volume 15 - 2024 | https://doi.org/10.3389/fpls.2024.1362814
This article is part of the Research Topic From Sunlight to Plant Health: Decoding Metabolic Responses View all 4 articles
The global food supply crisis is one of humanity’s most significant risks (World Economic Forum, 2023). Climate change is causing the loss of natural resources, which is closely related to this crisis (Mirzabaev et al., 2023). Therefore, it is crucial to implement sustainable food systems that ensure food security for both present and future generations. Thus, food should be available, accessible, and nutritious (Peng and Berry, 2018). Delaying the implementation of these goals will contribute to food insecurity and lead to a more polarized world.
Improving photosynthetic efficiency is critical to ensure food security because it generates 90% of plant biomass (van Bel et al., 2003) and increases crop yield (Brestic et al., 2021). However, photosynthesis is affected by high temperatures (Mathur et al., 2014), irregular rains (León-Sánchez et al., 2016), and drought (Wang et al., 2018), among others. The persistence and severity of these phenomena reduce the photosynthetic rate, exerting selection pressure mainly in C3 plants (Sello et al., 2019), affecting their adaptation biodiversity and could lead to an irreversible loss of genetic diversity (Demír, 2021), which is relevant to implement sustainable food production systems through genetic improvement (Salgotra and Chauhan, 2023).
The Solanaceae family is a prime example of climate change vulnerability because their centers of origin are in countries highly vulnerable to climate change (Samuels, 2015). In this regard, Solanaceae is among the 12 most diverse plant families, and more than 1,500 native species can be found in South America alone, and Peru standing out for its diversity (Palchetti et al., 2020). This richness translates into genetic and metabolic diversity that can be useful to improve the crop photosynthetic rate. Therefore, it is essential to identify the critical genes for light and dark phases.
Regarding the light phase (Figure 1), Leister (2023) proposes a list of genes, including the D1 gene of the photosystem II reaction center (PSII), that improve photosynthetic performance and plant growth when overexpressed. Thus, Chen et al. (2020) showed that Arabidopsis transgenic lines that overexpress D1 doubled their biomass under thermal stress (42°C). The D1 biosynthesis is mediated by genes that ensure psbA correct translation (Zhang et al., 2000). Thus, LPE1 binds to the 5’ end of psbA to facilitate association with HCF173 (Jin et al., 2018), which prevents exonucleotide degradation of psbA mRNA, ensuring its binding to the ribosome (Bollenbach, 2003). In addition, HCF244 is co-expressed with HCF173, which encodes a gene necessary for the translational initiation of psbA and stabilization of this messenger RNA (Link et al., 2012). These genes are relevant for plant development, in Arabidopsis lpe1-3 mutant showed a 70% reduction in the rosette size, and a drastic reduction in the ratio of variable fluorescence to maximum fluorescence (Fv/Fm) (Jin et al., 2018). For hcf173, Link et al. (2012) also had a similar reduction in rosette size in Arabidopsis than lpe1-3. RNA is highly unstable in hcf173, leading to a drastically impaired accumulation of PSII polypeptides (Schult et al., 2007). On the other hand, the hcf244 mutant cannot grow under autotrophic conditions due to a drastically impaired accumulation of PSII proteins (CP47, CP43, D1, and D2); reaching only about 10% to 20% of wild-type levels (Link et al., 2012). Both, HCF173 and HCF244 were identified by Bhattacharya et al. (2023) in tomato stromal proteome as part of the 29 orthologous proteins involved in the assembly, stability and repair of the PSII complex.
Figure 1 Schematic representation of the photosynthetic electron transport chain and the Calvin Benson cycle. Key photosynthetic genes identified with red stars. Light phase: Low photosynthetic effciency 1 (LPE1), High Chlorophyll fluorescence phenotype 173 (HCF173), High Chlorophyll fluorescence phenotype 244 (HCF244), D1 reaction center (D1), Cytochrome b6f complex (Cyt b6f), NAD(P)H dehydrogenase-like (NDH) complex subunits (NdhC, NdhI, NdhJ, NdhL or CRR23, Ndh48 and Ndh45), ATP sintase gamma subunit (γ Subunit). Dark phase: Ribulose bisphosphate carboxylase oxygenase (RuBisCO), Glyceraldehyde-3-phosphate dehydrogenase subunits (GAPA and GAPB) and Triosephosphate isomerase (TPI). Electron flow represented with black lines.
Another critical component of the electron transport chain is the cytochrome b6/f complex (Rochaix, 2011). Mutants in tomatoes of the petM subunit of this complex showed lower electron transport rate, CO2 assimilation, and carotenoid content than the wild type. In addition, mutants of the petM-4 line showed late autotrophic growth (Bulut et al., 2023). In tobacco, petA, B, and D mutants showed a lower content of thylakoid membranes (Monde et al., 2000). Considering that the cytochrome b6f complex regulates the acclimation of photosynthetic organisms to changing light conditions (Malone et al., 2021) and that algae have an exceptional ability to adapt to such conditions (Sukenik et al., 1987), Yadav et al. (2020) identified that tobacco specimens transformed with cytochrome b6 gene from Kappaphycus alvarezii have a net photosynthetic rate higher than the wild type by approximately 60%. This improved performance was also evident in the growth and starch accumulation of the transgenic lines. Yadav et al. (2018) reported similar results with transforming tobacco specimens with the UfCytb6 gene from Ulva fasciata. These findings show that photosynthetic and growth enhancement of tobacco specimens through manipulation of cytochrome b6f subunits is a potential way to improve their performance in light-changing environments.
In addition to the structural genes of PSII, the NADPH dehydrogenase or NDH complex is also relevant for the light phase since it participates in the cyclic transport of electrons to maintain the balance of the redox system to mitigate oxidative stress in the photosynthetic apparatus (Ma et al., 2021). The CRR23, NDH48, and NDH45 subunits guarantee the accumulation and stabilization of this complex in Arabidopsis. Thus, the crr23 mutant showed a 12.5% reduction in the accumulation of the NDH complex (Shimizu et al., 2008), while ndh48 and ndh45 revealed functional deficiencies of this complex (Sirpiö et al., 2009). On the other hand, in tobacco, a 25% reduction in the photochemical efficiency of PSII was identified in mutants for the C, J, and K subunits due to the increase in ROS at -4°C and 42°C (Wang et al., 2006). It is crucial to study subunits that cause a decrease in photosynthetic efficiency because of temperature stress. Mutations in these subunits can negatively impact the plant’s ability to withstand frost. Therefore, it is essential to investigate these subunits in potato wild relatives as a potential solution to this problem (Nicolao et al., 2023).
On the other hand, ATPC1, the γ subunit of ATP synthase, induces conformational changes in the catalytic region of this enzyme that are necessary for ATP synthesis (Cheuk and Meier, 2021). It possesses two cysteine residues that regulate ATP synthase activity in response to fluctuating intracellular redox conditions due to the unstable activity of the photosynthetic electron transfer chain associated with changing light intensity (Akiyama et al., 2023). In tobacco, Rott et al. (2011) identified that atpc1 mutants showed a reduction of more than 50% in growth after 14 weeks and also a reduction in the chlorophyll a/b ratio; this change suggests a rearrangement of the photosynthetic apparatus. Furthermore (Kohzuma et al., 2013), identified that the knockout of atpc1 in Arabidopsis cannot perform autotrophic growth.
On the other hand, overexpression of enzymes in the dark phase (Figure 1) does not necessarily result in improved photosynthetic efficiency. According to Zhao et al. (2021), balancing the catalytic activity of the different enzymes in the Calvin Benson Cycle (CBC) is crucial. RuBisCO is one of the most essential enzymes studied for enhancing photosynthetic efficiency, biomass accumulation, and crop yield (Lin et al., 2021). An evaluated strategy to make the catalytic activity of RuBisCO more efficient is to increase the concentration of CO2 around this enzyme through synthetic engineering, as this could increase the photosynthetic efficiency of C3 plants by 25% (Zhu et al., 2010). In nature, a greater availability of foliar CO2 was observed in Solanum pennellii, a wild relative of tomato, where the distribution of its stomata limits the diffusion of CO2 by photorespiration, facilitating its fixation and a consequent higher photosynthetic rate (Muir et al., 2014). However, despite the greater availability of CO2, a limiting factor is the catalytic inefficiency of RuBisCO compared to CO2 and O2 as substrates. The short subunit of RuBisCO controls the affinity regulation of these molecules (Genkov et al., 2010). In tobacco, mutations in this subunit have been found to reduce the total content of RuBisCO by 93% and biomass accumulation by 90% compared to the wild-type.
Despite the recent improvements, Lin et al. (2021) suggest that the most effective way to enhance the RuBisCO efficiency is to modify the long subunit; the active site of the enzyme is located there, making it vulnerable to changes in temperature and humidity. In this context, previous studies have identified that high temperatures and dry environments can reduce RuBisCO efficiency by up to 40% (Parto and Lartillot, 2018). This scenario is common for many crops, which may experience a loss of productivity ranging from 3 to 13% for each one-degree increase in temperature (Zhao et al., 2017). Indeed, Lin et al. (2022) conducted a study to address a problem related to RuBisCO efficiency in hot and dry environments. They explored the potential of thermostable RuBisCO ancestors in Solanaceae and found that they have superior catalytic efficiency, suggesting that by utilizing the genetic diversity of their ancestors, it is possible to improve the enzymatic efficiency of RuBisCO.
Glyceraldehyde 3-phosphate dehydrogenase (GAPDH) is a crucial enzyme in the reduction stage of the CBC. According to Petersen et al. (2003), GAPDH plays a significant role in this process. Rius et al. (2006) has reported that if GAPDH is deficient, it can hinder glycolysis and reduce CO2 fixation by approximately 25%. The GAPDH is used to create photosynthates and regenerate Ribulose 1,5 bisphosphate. GAPA and GAPB are the two subunits that make up the GAPDH enzyme. Deleting either GAPA or GAPB can significantly reduce carbon assimilation in Arabidopsis. Simkin et al. (2020) have reported that carbon assimilation decreases by 73% by GAPA deletion, while the deletion of GAPB leads to a 34% reduction. In rice, GAPB overexpression increases CO2 assimilation and chlorophyll content even under low light conditions (Liu et al., 2020). In contrast, in Arabidopsis, carbon assimilation is higher for gapb than gapa.
Triose phosphate isomerase (TPI) is another essential enzyme in the CBC, playing a pivotal role in the first reaction of the regeneration stage (Johnson, 2016). TPI has a critical C-terminal region, vital for its catalytic, regulatory, or folding function. This region is essential for efficiently converting glyceraldehyde 3-phosphate molecules into dihydroxyacetone phosphate and vice versa (Castro-Torres et al., 2018). Mutations in TPI’s plastid form in Arabidopsis result in chlorotic leaves and almost no growth after ten weeks of evaluation because of the accumulation of methylglyoxal, which is twice that of the wild type. As a result, the transition from heterotrophic to autotrophic growth is delayed (Chen & Thelen, 2010). Moreover, TPI has cysteine residues similar to the GAPB subunit of the GAPDH enzyme. In Arabidopsis and photosynthetic microorganisms such as Synechocystis and Chlamydomonas, these residues facilitate its stability and activity by being close to the catalytic site (Dumont et al., 2016; Castro-Torres et al., 2018). In tomato, the mutation of its TPI genes only showed visible phenotype changes in double mutant lines tpi1tpi2. In these individuals, Chen et al. (2023) found reduced TPI activity, chlorotic variegation, and reduced carbon-assimilation efficiency in contrast to the wild type. However, assessing the thermostability of TPI1 and TPI2 proteins in tomatoes, the author found that TPI2 may be more stable than TPI1 under heat stress at 42°C.
In addition to ensuring food production, photosynthesis supports plant secondary metabolism since its products are precursors (Qaderi et al., 2023) of over 50 thousand secondary metabolites (Teoh, 2016). Because of their properties, there is growing interest in identifying new secondary metabolites as industry inputs to enhance agricultural sustainability and improve their production (Ozyigit et al., 2023). Thus, plant and food waste are processed as a promise source to obtain secondary metabolite for the cosmetic industry (Faria-Silva et al., 2020).
The cosmetic and personal care industry uses plant-derived secondary metabolites to formulate products (Ribeiro et al., 2015). This market has seen significant growth from 2016 to 2022 (Liyanaarachchi et al., 2018) as consumers prefer natural products (Nadeeshani Dilhara Gamage et al., 2022). Products containing lycopene for skin care are trendy (Choi et al., 2022) due to their antioxidant capacity, improving skin elasticity and hydration (Franco et al., 2021). Thus, lycopene price is over $6000 per kg (Zia-Ul-Haq et al., 2021). Unfortunately, competition with the food industry affects lycopene supplies (Khan et al., 2021). Although lycopene chemical synthesis can be an alternative, the chemical residues in this process affect its overall quality (Li et al., 2022). Therefore, it is relevant to increase its concentration (Costa et al., 2021) to generate a circular production system.
The biosynthesis of lycopene and other carotenoids begins with the 2-C-methyl-D erythritol 4-phosphate pathway that uses glyceraldehyde 3-phosphate (GAP) and pyruvate (Sathasivam et al., 2021) to form 1-deoxy-D-xylulose 5-phosphate (DXP) via 1-deoxy-D-xylulose-5-phosphate synthase (DXS) (Simpson et al., 2016). The manipulation of DXS increases lycopene production (Kang et al., 2005), and its overexpression results in a twofold increase in the carotenoid content (Morris, 2006). The activity of DXS depends on the availability of GAP, GAPDH being the photosynthetic enzyme that generates this molecule (Petersen et al., 2003). GAPDH has predominant activity in photosynthetically active tissues (Kelly and Gibbs, 1973), favoring the biosynthesis of carotenoids such as lutein, beta-carotene, violaxanthin, and neoxanthin (Sun et al., 2018) that protect the photosynthetic apparatus from oxidative photodamage (Kim et al., 2018). In green tissues of plants, the regulation of carotenoid biosynthesis must occur in a coordinated manner with the assembly of the photosynthesis apparatus (Lu and Li, 2008). On the other hand, phytoene synthase (PSY) knock-out, a critical enzyme for carotenoid biosynthesis, completely suppresses photosynthesis (Sun et al., 2018).
The cosmetic and personal care industry uses plant-derived secondary metabolites, like lycopene, to avert skin photodamage and aging. Therefore, it is necessary to link diversity in plant secondary metabolism with the underlying genetic architecture related to photosynthetic gene diversity (LPE1, HCF173, HCF244, D1, Cytochrome b6f complex and NDH complex subunits, APTase γ subunit, RuBisCO, GAPA, GAPB and TPI) to add value to the Solanaceae biodiversity to develop new crops and thus prevent competition with the food industry.
CA-B: Writing – original draft, Writing – review & editing. GZ: Conceptualization, Writing – original draft, Writing – review & editing.
The author(s) declare financial support was received for the research, authorship, and/or publication of this article. This work was supported by Vicerectorado de Investigacion de la Universidad Nacional Agraria La Molina (TR. N° 0373-2022-R-UNALM).
The authors declare that the research was conducted in the absence of any commercial or financial relationships that could be construed as a potential conflict of interest.
All claims expressed in this article are solely those of the authors and do not necessarily represent those of their affiliated organizations, or those of the publisher, the editors and the reviewers. Any product that may be evaluated in this article, or claim that may be made by its manufacturer, is not guaranteed or endorsed by the publisher.
Akiyama, K., Ozawa, S.-I., Takahashi, Y., Yoshida, K., Suzuki, T., Kondo, K., et al. (2023). Two specific domains of the γ subunit of chloroplast F o F 1 provide redox regulation of the ATP synthesis through conformational changes. Proc. Natl. Acad. Sci. 120, 1–8. doi: 10.1073/pnas.2218187120
Bhattacharya, O., Ortiz, I., Hendricks, N., Walling, L. L. (2023). The tomato chloroplast stromal proteome compendium elucidated by leveraging a plastid protein-localization prediction Atlas. Front. Plant Sci. 14. doi: 10.3389/fpls.2023.1020275
Bollenbach, T. J. (2003). Divalent metal-dependent catalysis and cleavage specificity of CSP41, a chloroplast endoribonuclease belonging to the short chain dehydrogenase/reductase superfamily. Nucleic Acids Res. 31, 4317–4325. doi: 10.1093/nar/gkg640
Brestic, M., Yang, X., Li, X., Allakhverdiev, S. I. (2021). Crop photosynthesis for the twenty-first century. Photosynth Res. 150, 1–3. doi: 10.1007/s11120-021-00869-5
Bulut, M., Nunes-Nesi, A., Fernie, A. R., Alseekh, S. (2023). Characterization of PetM cytochrome b6f subunit 7 domain-containing protein in tomato. Hortic. Res. 10, 1–12. doi: 10.1093/hr/uhad224
Castro-Torres, E., Jimenez-Sandoval, P., Fernández-de Gortari, E., López-Castillo, M., Baruch-Torres, N., López-Hidalgo, M., et al. (2018). Structural basis for the limited response to oxidative and thiol-conjugating agents by triosephosphate isomerase from the photosynthetic bacteria synechocystis. Front. Mol. Biosci. 5. doi: 10.3389/fmolb.2018.00103
Chen, C., Zhang, M., Ma, X., Meng, Q., Zhuang, K. (2023). Differential heat-response characteristics of two plastid isoforms of triose phosphate isomerase in tomato. Plant Biotechnol. J. 1–12. doi: 10.1111/pbi.14212
Chen, J.-H., Chen, S.-T., He, N.-Y., Wang, Q.-L., Zhao, Y., Gao, W., et al. (2020). Nuclear-encoded synthesis of the D1 subunit of photosystem II increases photosynthetic efficiency and crop yield. Nat. Plants 6, 570–580. doi: 10.1038/s41477-020-0629-z
Chen, M., Thelen, J. J. (2010). The plastid isoform of triose phosphate isomerase is required for the postgerminative transition from heterotrophic to autotrophic growth in Arabidopsis. Plant Cell 22, 77–90. doi: 10.1105/tpc.109.071837
Cheuk, A., Meier, T. (2021). Rotor subunits adaptations in ATP synthases from photosynthetic organisms. Biochem. Soc. Trans. 49, 541–550. doi: 10.1042/BST20190936
Choi, Y.-H., Kim, S. E., Lee, K.-H. (2022). Changes in consumers’ awareness and interest in cosmetic products during the pandemic. Fashion Textiles 9, 1. doi: 10.1186/s40691-021-00271-8
Costa, A., Marques, M., Congiu, F., Paiva, A., Simões, P., Ferreira, A., et al. (2021). Evaluating the presence of lycopene-enriched extracts from tomato on topical emulsions: physico-chemical characterization and sensory analysis. Appl. Sci. 11, 5120. doi: 10.3390/app11115120
Demír, A. (2021). The impacts of climate change on genetic diversity. Biol. Divers. Conserv. 14/3, 511–518. doi: 10.46309/biodicon.2021.1032772
Dumont, S., Bykova, N. V., Pelletier, G., Dorion, S., Rivoal, J. (2016). Cytosolic triosephosphate isomerase from arabidopsis thaliana is reversibly modified by glutathione on cysteines 127 and 218. Front. Plant Sci. 7. doi: 10.3389/fpls.2016.01942
Faria-Silva, C., Ascenso, A., Costa, A. M., Marto, J., Carvalheiro, M., Ribeiro, H. M., et al. (2020). Feeding the skin: A new trend in food and cosmetics convergence. Trends Food Sci. Technol. 95, 21–32. doi: 10.1016/j.tifs.2019.11.015
Franco, L., Marchena, A., Rodríguez, A. (2021). Skin health properties of lycopene and melatonin. J. Dermatol. Skin Sci. 3, 26–29. doi: 10.29245/2767-5092/2021/1.1126
Genkov, T., Meyer, M., Griffiths, H., Spreitzer, R. J. (2010). Functional hybrid rubisco enzymes with plant small subunits and algal large subunits. J. Biol. Chem. 285, 19833–19841. doi: 10.1074/jbc.M110.124230
Jin, H., Fu, M., Duan, Z., Duan, S., Li, M., Dong, X., et al. (2018). LOW PHOTOSYNTHETIC EFFICIENCY 1 is required for light-regulated photosystem II biogenesis in Arabidopsis. Proc. Natl. Acad. Sci. 115, E6075–E6084. doi: 10.1073/pnas.1807364115
Kang, M. J., Lee, Y. M., Yoon, S. H., Kim, J. H., Ock, S. W., Jung, K. H., et al. (2005). Identification of genes affecting lycopene accumulation in Escherichia coli using a shot-gun method. Biotechnol. Bioeng 91, 636–642. doi: 10.1002/bit.20539
Kelly, G. J., Gibbs, M. (1973). Nonreversible d-glyceraldehyde 3-phosphate dehydrogenase of plant tissues. Plant Physiol. 52, 111–118. doi: 10.1104/pp.52.2.111
Khan, U. M., Sevindik, M., Zarrabi, A., Nami, M., Ozdemir, B., Kaplan, D. N., et al. (2021). Lycopene: food sources, biological activities, and human health benefits. Oxid. Med. Cell Longev 2021, 1–10. doi: 10.1155/2021/2713511
Kim, H. S., Ji, C. Y., Lee, C.-J., Kim, S.-E., Park, S.-C., Kwak, S.-S. (2018). Orange: a target gene for regulating carotenoid homeostasis and increasing plant tolerance to environmental stress in marginal lands. J. Exp. Bot. 69, 3393–3400. doi: 10.1093/jxb/ery023
Kohzuma, K., Dal Bosco, C., Kanazawa, A., Kramer, D. M., Meurer, J. (2013). A potential function for the γ2 subunit (atpC2) of the chloroplast ATP synthase. 576–578. Berlin, Heidelberg: Springer. doi: 10.1007/978-3-642-32034-7_123
Leister, D. (2023). Enhancing the light reactions of photosynthesis: Strategies, controversies, and perspectives. Mol. Plant 16, 4–22. doi: 10.1016/J.MOLP.2022.08.005
León-Sánchez, L., Nicolás, E., Nortes, P. A., Maestre, F. T., Querejeta, J. I. (2016). Photosynthesis and growth reduction with warming are driven by nonstomatal limitations in a Mediterranean semi-arid shrub. Ecol. Evol. 6, 2725–2738. doi: 10.1002/ece3.2074
Li, M., Xia, Q., Lv, S., Tong, J., Wang, Z., Nie, Q., et al. (2022). Enhanced CO 2 capture for photosynthetic lycopene production in engineered Rhodopseudomonas palustris, a purple nonsulfur bacterium. Green Chem. 24, 7500–7518. doi: 10.1039/D2GC02467E
Lin, M. T., Orr, D. J., Worrall, D., Parry, M. A. J., Carmo-Silva, E., Hanson, M. R. (2021). A procedure to introduce point mutations into the Rubisco large subunit gene in wild-type plants. Plant J. 106, 876–887. doi: 10.1111/tpj.15196
Lin, M. T., Salihovic, H., Clark, F. K., Hanson, M. R. (2022). Improving the efficiency of Rubisco by resurrecting its ancestors in the family Solanaceae. Sci. Adv. 8, 1–12. doi: 10.1126/sciadv.abm6871
Link, S., Engelmann, K., Meierhoff, K., Westhoff, P. (2012). The Atypical Short-Chain Dehydrogenases HCF173 and HCF244 Are Jointly Involved in Translational Initiation of the psbA mRNA of Arabidopsis. Plant Physiol. 160, 2202–2218. doi: 10.1104/pp.112.205104
Liu, Y., Pan, T., Tang, Y., Zhuang, Y., Liu, Z., Li, P., et al. (2020). Proteomic analysis of rice subjected to low light stress and overexpression of osGAPB increases the stress tolerance. Rice 13, 30. doi: 10.1186/s12284-020-00390-8
Liyanaarachchi, G. D., Samarasekera, J. K. R. R., Mahanama, K. R. R., Hemalal, K. D. P. (2018). Tyrosinase, elastase, hyaluronidase, inhibitory and antioxidant activity of Sri Lankan medicinal plants for novel cosmeceuticals. Ind. Crops Prod 111, 597–605. doi: 10.1016/j.indcrop.2017.11.019
Lu, S., Li, L. (2008). Carotenoid metabolism: biosynthesis, regulation, and beyond. J. Integr. Plant Biol. 50, 778–785. doi: 10.1111/j.1744-7909.2008.00708.x
Ma, M., Liu, Y., Bai, C., Yong, J. W. H. (2021). The significance of chloroplast NAD(P)H dehydrogenase complex and its dependent cyclic electron transport in photosynthesis. Front. Plant Sci. 12. doi: 10.3389/fpls.2021.661863
Malone, L. A., Proctor, M. S., Hitchcock, A., Hunter, C. N., Johnson, M. P. (2021). Cytochrome b6f – Orchestrator of photosynthetic electron transfer. Biochim. Biophys. Acta (BBA) - Bioenergetics 1862, 148380. doi: 10.1016/j.bbabio.2021.148380
Mathur, S., Agrawal, D., Jajoo, A. (2014). Photosynthesis: Response to high temperature stress. J. Photochem. Photobiol. B 137, 116–126. doi: 10.1016/J.JPHOTOBIOL.2014.01.010
Mirzabaev, A., Bezner Kerr, R., Hasegawa, T., Pradhan, P., Wreford, A., Cristina Tirado von der Pahlen, M., et al. (2023). Severe climate change risks to food security and nutrition. Clim Risk Manag 39, 1–10. doi: 10.1016/j.crm.2022.100473
Monde, R., Zito, F., Olive, J., Wollman, F., Stern, D. B. (2000). Post-transcriptional defects in tobacco chloroplast mutants lacking the cytochrome b 6/f complex. Plant J. 21, 61–72. doi: 10.1046/j.1365-313x.2000.00653.x
Morris, W. L. (2006). Overexpression of a bacterial 1-deoxy-D-xylulose 5-phosphate synthase gene in potato tubers perturbs the isoprenoid metabolic network: implications for the control of the tuber life cycle. J. Exp. Bot. 57, 3007–3018. doi: 10.1093/jxb/erl061
Muir, C. D., Pease, J. B., Moyle, L. C. (2014). Quantitative genetic analysis indicates natural selection on leaf phenotypes across wild tomato species (Solanum sect. Lycopersicon; solanaceae). Genetics 198, 1629–1643. doi: 10.1534/genetics.114.169276
Nadeeshani Dilhara Gamage, D. G., Dharmadasa, R. M., Chandana Abeysinghe, D., Saman Wijesekara, R. G., Prathapasinghe, G. A., Someya, T. (2022). Global perspective of plant-based cosmetic industry and possible contribution of Sri Lanka to the development of herbal cosmetics. Evidence-Based Complementary Altern. Med. 2022, 1–26. doi: 10.1155/2022/9940548
Nicolao, R., Gaiero, P., Castro, C. M., Heiden, G. (2023). Solanum malmeanum, a promising wild relative for potato breeding. Front. Plant Sci. 13. doi: 10.3389/fpls.2022.1046702
Ozyigit, I. I., Dogan, I., Hocaoglu-Ozyigit, A., Yalcin, B., Erdogan, A., Yalcin, I. E., et al. (2023). Production of secondary metabolites using tissue culture-based biotechnological applications. Front. Plant Sci. 14. doi: 10.3389/fpls.2023.1132555
Palchetti, M. V., Cantero, J. J., Barboza, G. E. (2020). Solanaceae diversity in South America and its distribution in Argentina. Acad. Bras. Cienc 92, 1–17. doi: 10.1590/0001-3765202020190017
Parto, S., Lartillot, N. (2018). Molecular adaptation in Rubisco: Discriminating between convergent evolution and positive selection using mechanistic and classical codon models. PloS One 13, e0192697. doi: 10.1371/journal.pone.0192697
Peng, W., Berry, E. M. (2018). “The concept of food security,” in Encyclopedia of food security and sustainability (Elsevier), 1–7. doi: 10.1016/B978-0-08-100596-5.22314-7
Petersen, J., Brinkmann, H., Cerff, R. (2003). Origin, evolution, and metabolic role of a novel glycolytic GAPDH enzyme recruited by land plant plastids. J. Mol. Evol. 57, 16–26. doi: 10.1007/s00239-002-2441-y
Qaderi, M. M., Martel, A. B., Strugnell, C. A. (2023). Environmental factors regulate plant secondary metabolites. Plants 12, 447. doi: 10.3390/plants12030447
Ribeiro, A., Estanqueiro, M., Oliveira, M., Sousa Lobo, J. (2015). Main benefits and applicability of plant extracts in skin care products. Cosmetics 2, 48–65. doi: 10.3390/cosmetics2020048
Rius, S. P., Casati, P., Iglesias, A. A., Gomez-Casati, D. F. (2006). Characterization of an Arabidopsis thaliana mutant lacking a cytosolic non-phosphorylating glyceraldehyde-3-phosphate dehydrogenase. Plant Mol. Biol. 61, 945–957. doi: 10.1007/s11103-006-0060-5
Rochaix, J.-D. (2011). Reprint of: Regulation of photosynthetic electron transport. Biochim. Biophys. Acta (BBA) - Bioenergetics 1807, 878–886. doi: 10.1016/j.bbabio.2011.05.009
Rott, M., Martins, N. F., Thiele, W., Lein, W., Bock, R., Kramer, D. M., et al. (2011). ATP synthase repression in tobacco restricts photosynthetic electron transport, CO 2 assimilation, and plant growth by overacidification of the thylakoid lumen. Plant Cell 23, 304–321. doi: 10.1105/tpc.110.079111
Salgotra, R. K., Chauhan, B. S. (2023). Genetic diversity, conservation, and utilization of plant genetic resources. Genes (Basel) 14, 174. doi: 10.3390/genes14010174
Samuels, J. (2015). Biodiversity of food species of the solanaceae family: A preliminary taxonomic inventory of subfamily solanoideae. Resources 4, 277–322. doi: 10.3390/resources4020277
Sathasivam, R., Radhakrishnan, R., Kim, J. K., Park, S. U. (2021). An update on biosynthesis and regulation of carotenoids in plants. South Afr. J. Bot. 140, 290–302. doi: 10.1016/j.sajb.2020.05.015
Schult, K., Meierhoff, K., Paradies, S., Toöller, T., Wolff, P., Westhoff, P. (2007). The Nuclear-Encoded Factor HCF173 Is Involved in the Initiation of Translation of the psbA mRNA in Arabidopsis thaliana. Plant Cell 19, 1329–1346. doi: 10.1105/tpc.106.042895
Sello, S., Meneghesso, A., Alboresi, A., Baldan, B., Morosinotto, T. (2019). Plant biodiversity and regulation of photosynthesis in the natural environment. Planta 249, 1217–1228. doi: 10.1007/s00425-018-03077-z
Shimizu, H., Peng, L., Myouga, F., Motohashi, R., Shinozaki, K., Shikanai, T. (2008). CRR23/ndhL is a subunit of the chloroplast NAD(P)H dehydrogenase complex in Arabidopsis. Plant Cell Physiol. 49, 835–842. doi: 10.1093/pcp/pcn058
Simkin, A. J., Faralli, M., Ramamoorthy, S., Lawson, T. (2020). Photosynthesis in non-foliar tissues: implications for yield. Plant J. 101, 1001–1015. doi: 10.1111/tpj.14633
Simpson, K., Quiroz, L. F., Rodriguez-Concepción, M., Stange, C. R. (2016). Differential contribution of the first two enzymes of the MEP pathway to the supply of metabolic precursors for carotenoid and chlorophyll biosynthesis in carrot (Daucus carota). Front. Plant Sci. 7. doi: 10.3389/fpls.2016.01344
Sirpiö, S., Allahverdiyeva, Y., Holmström, M., Khrouchtchova, A., Haldrup, A., Battchikova, N., et al. (2009). Novel nuclear-encoded subunits of the chloroplast NAD(P)H dehydrogenase complex. J. Biol. Chem. 284, 905–912. doi: 10.1074/JBC.M805404200
Sukenik, A., Bennett, J., Falkowski, P. (1987). Light-saturated photosynthesis — Limitation by electron transport or carbon fixation? Biochim. Biophys. Acta (BBA) - Bioenerg. 891, 205–215. doi: 10.1016/0005-2728(87)90216-7
Sun, T., Yuan, H., Cao, H., Yazdani, M., Tadmor, Y., Li, L. (2018). Carotenoid metabolism in plants: the role of plastids. Mol. Plant 11, 58–74. doi: 10.1016/j.molp.2017.09.010
Teoh, E. S. (2016). “Secondary metabolites of plants,” in Medicinal orchids of asia (Springer International Publishing, Cham), 59–73. doi: 10.1007/978-3-319-24274-3_5
van Bel, A. J. E., Offler, C. E., Patrick, J. W. (2003). “PHOTOSYNTHESIS AND PARTITIONING | Sources and sinks,” in Encyclopedia of applied plant sciences (Elsevier), 724–734. doi: 10.1016/B0-12-227050-9/00089-2
Wang, P., Duan, W., Takabayashi, A., Endo, T., Shikanai, T., Ye, J.-Y., et al. (2006). Chloroplastic NAD(P)H dehydrogenase in tobacco leaves functions in alleviation of oxidative damage caused by temperature stress. Plant Physiol. 141, 465–474. doi: 10.1104/pp.105.070490
Wang, Z., Li, G., Sun, H., Ma, L., Guo, Y., Zhao, Z., et al. (2018). Effects of drought stress on photosynthesis and photosynthetic electron transport chain in young apple tree leaves. Biol. Open. 7, 1–9. doi: 10.1242/bio.035279
Yadav, S. K., Khatri, K., Rathore, M. S., Jha, B. (2018). Introgression of UfCyt c6, a thylakoid lumen protein from a green seaweed Ulva fasciata Delile enhanced photosynthesis and growth in tobacco. Mol. Biol. Rep. 45, 1745–1758. doi: 10.1007/s11033-018-4318-1
Yadav, S. K., Khatri, K., Rathore, M. S., Jha, B. (2020). Ectopic Expression of a Transmembrane Protein KaCyt b 6 from a Red Seaweed Kappaphycus alvarezii in Transgenic Tobacco Augmented the Photosynthesis and Growth. DNA Cell Biol. 1–15. doi: 10.1089/dna.2020.5479
Zhang, L., Paakkarinen, V., van Wijk, K. J., Aro, E.-M. (2000). Biogenesis of the chloroplast-encoded D1 protein: regulation of translation elongation, insertion, and assembly into photosystem II. Plant Cell 12, 1769–1781. doi: 10.1105/tpc.12.9.1769
Zhao, C., Liu, B., Piao, S., Wang, X., Lobell, D. B., Huang, Y., et al. (2017). Temperature increase reduces global yields of major crops in four independent estimates. Proc. Natl. Acad. Sci. 114, 9326–9331. doi: 10.1073/pnas.1701762114
Zhao, H., Tang, Q., Chang, T., Xiao, Y., Zhu, X.-G. (2021). Why an increase in activity of an enzyme in the Calvin–Benson cycle does not always lead to an increased photosynthetic CO2 uptake rate?—a theoretical analysis. In Silico Plants 3, 1–13. doi: 10.1093/insilicoplants/diaa009
Zhu, X.-G., Long, S. P., Ort, D. R. (2010). Improving photosynthetic efficiency for greater yield. Annu. Rev. Plant Biol. 61, 235–261. doi: 10.1146/annurev-arplant-042809-112206
Keywords: photosynthesis, Solanaceae, sustainability, biodiversity, cosmetics
Citation: Aguirre-Bottger C and Zolla G (2024) The best of both worlds: photosynthesis and Solanaceae biodiversity seeking a sustainable food and cosmetic industry. Front. Plant Sci. 15:1362814. doi: 10.3389/fpls.2024.1362814
Received: 29 December 2023; Accepted: 05 February 2024;
Published: 16 February 2024.
Edited by:
Roland Valcke, University of Hasselt, BelgiumReviewed by:
Kusum Khatri, Ben-Gurion University of the Negev, IsraelCopyright © 2024 Aguirre-Bottger and Zolla. This is an open-access article distributed under the terms of the Creative Commons Attribution License (CC BY). The use, distribution or reproduction in other forums is permitted, provided the original author(s) and the copyright owner(s) are credited and that the original publication in this journal is cited, in accordance with accepted academic practice. No use, distribution or reproduction is permitted which does not comply with these terms.
*Correspondence: Gaston Zolla, Z2VtemJAeWFob28uY29t
Disclaimer: All claims expressed in this article are solely those of the authors and do not necessarily represent those of their affiliated organizations, or those of the publisher, the editors and the reviewers. Any product that may be evaluated in this article or claim that may be made by its manufacturer is not guaranteed or endorsed by the publisher.
Research integrity at Frontiers
Learn more about the work of our research integrity team to safeguard the quality of each article we publish.