- 1Phytopathology- Applied Microbiology, Earth, and Life Institute, UCLouvain, Louvain-la-neuve, Belgium
- 2Unité de défense des végétaux, Institut des Sciences Agronomiques du Burundi, Bujumbura, Burundi
- 3Microbial Processes and Interactions, TERRA Teaching and Research Center, Gembloux Agro-Bio Tech, University of Liège, Gembloux, Belgium
- 4Chemical and Agricultural Industries, Faculty of Agricultural Sciences, University of Kinshasa, Kinshasa, Democratic Republic of Congo
- 5Laboratoire de Nutrition-Phytochimie, d’Ecologie et d’Environnement Appliquée, Centre Universitaire de Recherche et de Pédagogie Appliquées aux Sciences, Institut de Pédagogie Appliquée, Université du Burundi, Bujumbura, Burundi
- 6Département des Sciences et Technologie des Aliments, Faculté de Bio-Ingénierie, Université du Burundi, Bujumbura, Burundi
Agricultural productivity in the Great Lakes Countries of Central Africa, including Burundi, Rwanda, and the Democratic Republic of Congo, is affected by a wide range of diseases and pests which are mainly controlled by chemical pesticides. However, more than 30% of the pesticides used in the region are banned in European Union due to their high toxicity. Globally available safe and eco-friendly biological alternatives to chemicals are virtually non-existent in the region. Bacillus PGPR-based biocontrol products are the most dominant in the market and have proven their efficacy in controlling major plant diseases reported in the region. With this review, we present the current situation of disease and pest management and urge the need to utilize Bacillus-based control as a possible sustainable alternative to chemical pesticides. A repertoire of strains from the Bacillus subtilis group that have shown great potential to antagonize local pathogens is provided, and efforts to promote their use, as well as the search for indigenous and more adapted Bacillus strains to local agro-ecological conditions, should be undertaken to make sustainable agriculture a reality in the region.
1 Introduction
The importance of agriculture for the development of societies has been demonstrated for several centuries and it employs about 43% of the world’s working population (Roser, 2023). In the Great Lakes Countries of Central Africa (GLCCA), referred to in this report as Burundi, Rwanda, and the Democratic Republic of Congo (DRC), agriculture is the main activity employing more than 94%, 46% and 60% of the working population, while contributing to 40%, 30% and 36% of gross domestic product (GDP) in Burundi, Rwanda, and DRC respectively (PND, 2018; Lokuruka, 2020; FAAPA, 2021). However, this sector faces several constraints including low soil fertility and high incidence of diseases and pests leading to food insecurity (Bjornlund et al., 2020). For example, up to 52%, 43% and 38% of children under five in Burundi, DRC and Rwanda respectively are malnourished (Lokuruka, 2020; WFP, 2021; FAO, 2023). In the GLCCA, plant diseases and pests are generally controlled by chemical pesticides, especially those affecting cash crops such as coffee, cotton, tomatoes, potatoes, vegetables, and fruits (Niyongere et al., 2015; Muliele et al., 2018; Okonya et al., 2019a). However, some phytopathogens are inefficiently managed by traditional practices, such as the use of plant extracts, while others are not controlled (Rutikanga, 2015; MINAGRI-Burundi, 2018; Korangi Alleluya et al., 2021).
Biocontrol products are the most promoted tools within the Integrated Pest Management (IPM) framework, as they are generally recognized as safe (GRAS) compared to chemical pesticides (Raveau et al., 2020). Biocontrol products include biochemicals (semiochemicals, plant extracts, plant growth regulators and organic acids), macroorganisms (insects, mites, and nematodes) and microorganisms (beneficial bacteria, fungi, protozoa, viruses, yeasts) and their derivatives (cyclic lipopeptides, enzymes, chitosan oligopolysaccharides, etc…) (DunhamTrimmer, 2023). Among microorganisms, plant growth-promoting rhizobacteria (PGPR), including species of the Streptomyces, Paenibacillus, Pseudomonas and Bacillus genera, are the most commercially exploited for crop bioprotection, notably due to their efficient production of an arsenal of bioactive secondary metabolites (BSMs). These bacteria protect plants through direct antibiosis, competition with other pathogenic microbes for space and nutrients, and via their potential to induce plant systemic resistance (ISR) (Beneduzi et al., 2012; Wang et al., 2021; Dimkić et al., 2022). Bacillus-based products dominate the biocontrol market compared to their PGPR counterparts (DunhamTrimmer, 2023; Helepciuc and Todor, 2023) due to their ability to form resistant endospores, allowing stable formulations and ensuring long-term survival in the environment, especially in the current climate change context (Radhakrishnan et al., 2017; Miljaković et al., 2020). In this review, we provide a detailed topography of plant health and crop protection in the GLCCA, address constraints within the sector, and propose promising sustainable solutions. An overview of biological alternatives to chemical pesticides is discussed, with a focus on Bacillus PGPR for potential integration as biocontrol ingredients into local agricultural systems.
2 Diversity of phytopathogens and their control
We performed a comprehensive survey of the major diseases and causal agents affecting crop production in the GLCCA region by compiling data from published studies (articles and books) with those obtained from governmental and non-governmental agencies in the form of reports, newspapers, or online databases. Either in the field or in storage, agricultural crops are mainly affected by microbial pathogens consisting of fungi, oomycetes, bacteria, and viruses (Table 1). The most important fungal diseases encompass late blight of potato and tomato caused by Phytophthora infestans, angular leaf spot of bean caused by Pseudocercospora griseola, early blight of tomato caused by Alternaria solani, stem rot caused by Sclerotium rolfsii, late and early leaf spot caused by Nothopassalora personata and Cercospora arachidicola on peanut. These pathogens are most commonly controlled with the chemical mancozeb or its derivatives. Metalaxyl, benomyl, iprobenfos, and copper (II) chloride and metalaxyl are other important fungicides used in the region. One of the most common bacterial diseases prevailing in the region is Xanthomonas wilt of banana caused by X. campestris pv. musacearum, but unfortunately there is no effective pesticide available to control this pathogen (Table 1). Viral plant pathogens responsible for cassava mosaic disease, cassava brown streak disease, banana bunchy top disease and maize lethal necrosis, also cause serious threat to food security in the region but here again, no efficient chemical control is available.
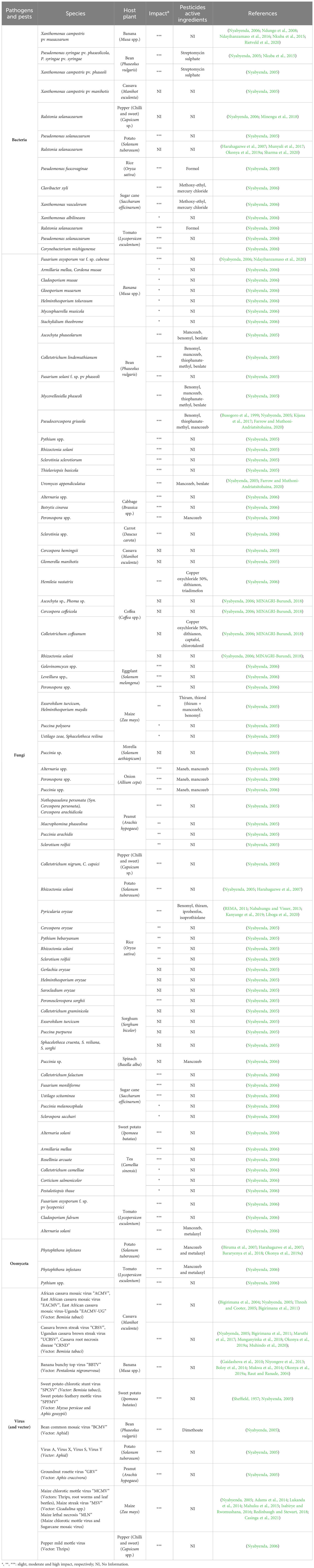
Table 1 Microbial pathogens affecting crop production in the GLCCA region and chemical pesticides used for their control.
Insects, nematodes, and weeds are also responsible for severe yield losses. Important insect pests affecting major crops in the region include fall armyworm (Spodoptera frugiperda), tomato leafminer (Tuta absoluta), coffee bugs (Antestiopsis orbitalis ghesquierei), whitefly (Bemisia tabaci), banana aphid (Pentalonia nigronervosa), and coton aphid (Aphis gossypii) (Nyabyenda, 2005; Dushimirimana et al., 2016; MINAGRI-Burundi, 2018; Niyibizi et al., 2019; Belga, 2020; Mukwa et al., 2020; Cokola et al., 2021; Niassy et al., 2021). The control of these insects relies heavily on the use of synthetic products such as acephate against S. frugiperda and T. absoluta, chlorpyrifos-ethyl against A. orbitalis ghesquierei and imidacloprid against aphids. Other major insecticides used in the region include lambda-cyhalothrin, cypermethrin, dimethoate, pirimiphos-methyl + permethrin, metaldehyde, abamectin and emamectin benzoate (MINAGRI-Burundi, 2018; ARECO-Rwanda Nziza, 2020; GUCE, 2022). Several species of nematodes also damage plants, including Meloidogyne javanica on various crops, Pratylenchus goodeyi and Helicotylenchus multicinctus on banana plants, and Ditylenchus spp. on potatoes (Coyne et al., 2018). These nematode-caused diseases are managed by using chemicals such as dazomet and terbufos. Parasitic plants or weeds that compete with crops such as sugarcane, rice, sorghum, and maize are also problematic and include among others Striga spp., Cyperus spp. and Echinochloa spp. Their control involves chemical herbicides like glyphosate, atrazine and dalapon (Nyabyenda, 2005; Rodenburg et al., 2016; Runo and Kuria, 2018).
The spread of some of these diseases and pests has led to historic famines in the GLCCA. For example, the Integrated Food Security Phase Classification (IPC) reported that nearly 13.1 million people in the DRC were acutely food insecure due to the spread of the fall armyworm on maize crops (MINAGRI-RDC, 2018; IPC, 2021). In 2004-2005, the outbreak of cassava mosaic disease led to severe food shortages that threatened many families in the north-eastern provinces of Kirundo and Muyinga in Burundi. As a result, 100 famine-related deaths were reported (Legg et al., 2006).
3 Limitations in local regulation of phytosanitary products and associated risks
Pesticide on the market must be regularly reconsidered by the relevant registration authorities and subsequently included in a list of approved or banned products. The aim is to ensure the quality, efficacy and safety of the chemicals used. However, there are some specific features of the management of pesticides in the GLCCA region that deserve special attention. First, many chemical pesticides banned in the European Union (EU Food Safety, 2023) are still officially registered in the GLCCA countries, representing up to 30% (Table 2) of the total number of products legally distributed (Table S1). It includes the highly hazardous but most commonly used fungicide mancozeb and the insecticides imidacloprid, acephate and dimethoate banned in EU (Lewis et al., 2016). Furthermore, some ingredients such as endosulfan and dichlorodiphenyltrichloroethane, which have been officially discarded by governments in the GLCCA region, are still available in local markets (Ngweme et al., 2019; Bassily, 2022). Second, malpractices in the handling of chemical pesticides are common, either among traders or end-users such as farmers or industrial workers. These malpractices include the sale of adulterated products, failure to use personal protective equipment, incorrect dosage and selection of products, and application at inappropriate times (Wipfler and ter Host, 2018; Okonya et al., 2019b; Balasha et al., 2023). One of the consequences is that residues of pesticides have been found at toxic levels in crops and vegetables such as tomato and amaranth (Kavatsurwa et al., 2014; Ngweme et al., 2021; Ndisanze et al., 2022).
Consequently, some acute symptoms and even death of humans and animals due to pesticide poisoning have been reported in the region. These symptoms include reddened eyes, itchy skin, teary eyes, burning eyes, runny nose, headache, difficulty breathing, and heavy sweating (Ndayambaje et al., 2019; Okonya et al., 2019b; Balasha et al., 2023). Chronic effects of pesticide exposure, including cancer, infertility, and neurological problems (Mahmood et al., 2016), may be a reality in the region or may occur in the near future, although no official survey has been conducted to date. Several reasons may explain this chaotic situation in the region such as poverty, ignorance, and illiteracy among users (Niyongere et al., 2015; Muliele et al., 2018; Ndayambaje et al., 2019; Balasha et al., 2023), inadequate governance in the pesticide sector, and possible socio-economic influence of agrochemical companies on officials (Gaberell and Viret, 2022).
4 Non-biological alternatives to chemical pesticides in GLLCA
The adverse effects of chemical pesticides have prompted the worldwide scientific community and policy makers to search for alternatives and promote the so-called IPM, which is defined as a holistic approach that aims to control plant pests and diseases by mobilizing all existing methods while reducing reliance on chemicals (Stenberg, 2017). IPM promotes the development of non-biological strategies such as prevention, monitoring, and rational use of chemical phytoprotectants products, and the implementation of biological control methods, which will be discussed later.
Good agricultural practices are preventive measures consisting, for example, in the use of certified varieties, crop rotation, field sanitation and balanced fertilization. These practices have been adopted to some extent in the GLCCA region and promising results have been obtained. For example, cassava mosaic disease and maize streak disease have been controlled using resistant varieties (Legg et al., 2006; Nkurunziza et al., 2012). The banana bunchy top disease has recently been reduced via macro- and micropropagation of healthy suckers (Niyongere et al., 2013; Tchatchambe et al., 2019; Paka et al., 2021). The incidence of banana Xanthomonas wilt and potato bacterial wilt caused by R. solani has been limited via crop rotation, field sanitation, sterilization of farm tools, and avoidance of their exchange (Harahagazwe et al., 2007; Ndayihanzamaso et al., 2016; Uwamahoro et al., 2020). However, despite many attempts involving certified varieties or other agricultural practices, no substantial results have been achieved to control fungal diseases in the region using these approaches. Moreover, implementation of good agricultural practices is unfortunately hampered by several factors, including demographic pressure on arable land, the relative time required to develop new varieties, the lack of durability of resistance in developed varieties, and the consequences of climate change in the agro-ecological context (Pandit et al., 2022).
Phytosanitary products must be used in a rational way, taking into account the epidemiological factors of the specific pathogens, which are usually obtained from accurate field monitoring. Where chemicals are irreplaceable, careful consideration must be given to the dose applied and the less toxic products must be favored. For example, flupyradifurone has been proposed to replace the insecticide imidacloprid (Maloney et al., 2020) and copper hydroxide in substitution of mancozeb (FPS Health, 2023) in EU. Unfortunately, this practice is less widespread in the region, where the choice of pesticide is made at random without prior information on the disease status and on recommended products for optimal efficiency.
5 Implementation of biological control
Biological control is a promising approach for plant protection based on the use of living organisms and/or their derivatives. These include macroorganisms (predatory insects, mites, and nematodes), microorganisms (beneficial bacteria, fungi, protozoa, viruses, yeasts) and biochemicals (semiochemicals, plant extracts, plant growth regulators and organic acids) (DunhamTrimmer, 2023). The biocontrol market accounts for 10% of the global pesticide market, with North America leading the way in promoting BCPs, while African share is very insignificant led by countries like Nigeria, South Africa, and Kenya (Marrone, 2023; Mordor Intelligence, 2023). Biological control is a new paradigm in GLCCA and only seven biocontrol products (3%) are registered up to now (Table S1).
5.1 Macroorganisms
Macroorganisms are either predators or parasitoids of the same kind of pathogenic organisms (insects, mites, or nematodes). They are used in the form of eggs, larvae, pupae, or adults to kill or parasitize the target pest (Ramalakshmi et al., 2020; DunhamTrimmer, 2023). Insect predators have been successfully used in the region to control some pests. For instance, Epidinocarpis lopezi isolated in South America, has been used to control the cassava mealy bug (Phenacoccus Manihoti) (Neuenschwander, 2001; Nyabyenda, 2005), while Gyranusoidea tebygi and Anagyrus mangicola have been developed to control the devastating mango mealy bug in Burundi (FAO, 2022). Other common predators could be introduced locally to control a range of pests. Indeed, the green lacewing (Chrysoperla carnea), the Malaysian ladybird beetle (Chilocorus nigritus) and the mealybug ladybird (Cryptolaemus montrouzieri) have been reported to be effective in controlling the cotton aphid jassid, peanut thrips, sugarcane scales and coffee and mango mealybugs respectively (Ramalakshmi et al., 2020).
5.2 Biochemicals
Biochemicals in the form of plant extracts appear to be the most widely used biocontrol agents in the region. These extracts are prepared from various plant species like Azadirachta indica, Capsicum spp., Allium sativum, Tephrosia spp., Tithonia diversifolia or Ricinus communis and are used as insecticides to control various pests such as S. frugiperda on maize, Tuta absoluta on tomato, Ophiomya phaseoli, Aphis fabae on beans, etc. (Rutikanga, 2015; PES, 2021; Korangi Alleluya et al., 2021). In addition, two plant molecules azadirachtin and pyrethrin, and spinosad from actinomycetes have been registered as insecticides in Rwanda (Table S1). However, the use of these extracts is still globally rudimentary and based on traditional and community-based knowledge. Moreover, the use of this type of products requires increased land for growing plants to prepare the extracts, which would inevitably compete with staple and cash crops for agricultural land.
5.3 Microorganisms
Several microorganisms encompassing bacteria, fungi, and yeasts are commercialized as biocontrol agents. Fungal based agents mainly include the genera Trichoderma, Metarhizium and Beauveria; while yeasts include the genera Pichia, Yarrowia and Saccharomyces. The largest group of worldwide marketed microorganisms are bacteria (up to 75%), dominated by plant growth-promoting rhizobacteria (PGPR) of the genera Bacillus, Pseudomonas and Streptomyces (Saeed et al., 2021; Bonaterra et al., 2022; Dimkić et al., 2022). However, these globally adopted microbial-based alternatives are rarely used in the GLCCA region, and mainly as bioinsecticides such as B. thuringiensis sold in the three countries and B. bassiana and T. harzanium only registered in Rwanda. Here, an overview of the PGPR reported in literature with biocontrol activities and their mechanisms of action is presented for their possible integration into local agricultural systems in GLCCA.
6 Plant growth-promoting rhizobacteria in crop protection
Plant growth-promoting rhizobacteria are a group of bacteria found mainly in the vicinity of plant roots, the rhizosphere. The plant provides them with nutrients in the form of root exudates, while these bacteria protect the host and promote its growth. These benefits are mediated through various pathways, including biofertilization by nitrogen fixation and phosphate solubilization, phytostimulation by the production of phytohormones (cytokinin, abscisic acid, auxin, gibberellins, and ethylene), stress tolerance and biological control (Vejan et al., 2016; Anckaert et al., 2021; El-Saadony et al., 2022). PGPR include several species belonging to different genera, namely Rhizobium, Azotobacter, Streptomyces, Enterobacter, Klebsiella, Rhodococcus, Paenibacillus, Variovorax, Azosprillum, Bulkholderia, Serratia, Pseudomonas and Bacillus (Caulier et al., 2018). Bacterial strains of the genera Streptomyces, Paenibacillus, Pseudomonas and Bacillus are the best described and have been shown to be effective biocontrol agents against various plant pathogens through their multiple mechanisms of action such as nutrient and niche competition, antibiosis, signal interference and induction of host resistance (Wang et al., 2021).
6.1 Competition
Competition for nutrients and niches between pathogens and beneficial microbes is an important factor in limiting disease incidence and severity (Bishnoi, 2015). Indeed, rhizosphere microorganisms compete for a variety of plant root exudates, including sugars, amino acids and organic acids. These exudates also act as chemoattractants for bacteria to plant roots. These beneficial bacteria colonize the root and can inhibit the growth of soil-borne phytopathogenic microbes by competing for nutrients such as glucose, asparagine, and iron (Dutta and Lee, 2022). Besides exudates, competition for iron is the most well-studied mechanism and involves several bacterial siderophores, such as hydroxamates (Pseudomonas spp.), carboxylates (Rhizobium spp.) and catecholates (Pseudomonas spp., Bacillus spp.), which are used to extract iron from insoluble forms and sequester it from other competitors by chelation (Arguelles-Arias et al., 2009; Mazumdar et al., 2020). On the other hand, rhizobacteria outcompete other microbes for space through their ability to form biofilms, which also act as shelter-like against pathogens (Flemming et al., 2016; Singh et al., 2022).
6.2 Antibiosis
Antibiosis is a biological process in which antimicrobial substances synthesized by microorganisms inhibit or kill plant pathogens (Fira et al., 2018). These are toxins that interfere with the synthesis of the cell membrane or other metabolic processes of the pathogen and are variable depending on the PGPR species. For example, Pseudomonas spp. are known to produce 2,4-diacetylphloroglucinol, phenazine, pyoluteorin, pyrrolnitrin, hydrogen cyanide and a wide range of lipopeptides such as viscosin, amphisin, orfamide, bananamide and sessilin (Wang et al., 2021; Oni et al., 2022). Bacillus spp. produce various cyclic lipopeptides (CLPs), polyketides, dipeptides, RiPPs and volatile organic compounds (Arguelles Arias et al., 2013; Caulier et al., 2019; Anckaert et al., 2021). Streptomyces species are known producers of several antibiotics such as kasugamycin (antifungal and antibacterial), polyoxin B and D, validamycin (antifungal) families (Barka et al., 2016), as well as cyclic lipopeptides such as lipopeptins recently discovered with antagonistic activity against Fusarium oxysporum (Wang et al., 2023). Paenibacillus spp. are another important PGPR, producing mainly polymyxin active against Gram-negative bacteria, fusaricidin with anti-Gram-positive bacteria and antifungal activities (Ali et al., 2021), pelgipeptins and tridecaptins with antagonistic activity against fungi and bacteria (Le et al., 2020; Costa et al., 2022). PGPR also produce lytic enzymes (the so-called cell wall degrading enzymes) such as chitinase, glucanase, cellulase, xylanase, and pectinase, which are known for their antagonistic activities against pathogens (Abdelaziz et al., 2023).
6.3 Signal interference
Like other organisms, bacteria live in communities where they communicate to obtain nutrients and perform other metabolic functions. Cell-to-cell communication within bacteria is known as quorum sensing (QS), mediated by several molecules including N-acylhomoserine lactones, oligopeptides and LuxS/autoinducers (De Kievit, 2009; Rodríguez et al., 2020; Wang et al., 2021). QS is important for bacterial phenotype and virulence, biofilm formation and other physiological processes. QS-mediated metabolites from phytopathogenic bacteria can be chemically or enzymatically degraded by so-called quorum quenching (QQ) enzymes such as lactonase, acylase and oxidoreductase produced by some PGPR. This interferes with QS-regulated processes that are crucial for pathogen development (Sikdar and Elias, 2020) and some important bacterial diseases such as Pectobacterium carotovorum, Pseudomonas syringae, Ralstonia solanacearum, Erwinia amylovora have been successfully managed by using this approach (Rodríguez et al., 2020).
6.4 Induced systemic resistance
Induced systemic resistance (ISR) is the process by which plants treated with PGPR species or their secreted molecules induce defense genes and develop immunity to subsequent plausible pathogen attack. PGPR-induced ISR is phenotypically similar to the well-studied systemic acquired resistance (SAR), which is activated after a first infection by an incompatible or necrotizing pathogen (Pršić and Ongena, 2020). This process is regulated by the jasmonate or ethylene phytohormone pathways, whereas SAR is dependent on the salicylic acid regulation pathway. The main elicitors of ISR produced by PGPR are Acyl-Homoserine Lactones, cyclic lipopeptides, rhamnolipids, N-alkylated benzylamine derivative, siderophores, volatile compounds and other metabolites with antibiotic functions such as 2,4-diacetylphloroglucinol and phenazine (Pršić and Ongena, 2020; Wang et al., 2021; Zhu et al., 2022). These elicitors act as microbial associated molecular patterns and are perceived by specific transmembrane pattern recognition receptors, as it has been shown for the pathogen triggered-SAR. However, for a successful symbiosis with plant roots, PGPR have evolved to evade or suppress the pattern triggered immunity developed by the plant as the first line of defense (Yu et al., 2022; Seth et al., 2023). This PGPR-induced plant immunity ultimately leads to defense mechanisms such as cell wall reinforcement via deposition of lignin or callose, enhanced production of defense-related proteins (lipoxygenase, glucanase, chitinase, phenylalanine ammonia-lyase) and accumulation of phytoalexins as small-size antimicrobial metabolites (Abdelaziz et al., 2023).
7 Bacillus as successful PGPR for biological control
Bacillus is a rod-shaped, gram-positive and spore-forming bacterium that is widespread in a variety of ecological niches (Yin et al., 2023). Phylogenetically, the Bacillus genus can be broadly divided into two clades, namely the Bacillus cereus group, which includes the well-known and most commercialized bioinsecticide B. thuringiensis, and the Bacillus subtilis group, which includes most of Bacillus strains marketed for the biocontrol of microbial pathogens (Dunlap, 2019; Nikolaidis et al., 2022). Strains in the B. subtilis group are generally recognized as safe and have therefore been used as alternatives to chemical pesticides in agriculture (Etesami et al., 2023). For example, B. licheniformis SB3086 (Ecoguard®, Novozymes®, Biofungicide®, Green Relief®), B. pumilus GB34 (GB34®, Concentrated Biological Fungicide®, Ballad®), B. velezensis MBI600 (Subtilex®, Histick N/T®), B. velezensis GBO3 (Kodiak®, Companion®), B. velezensis QST713 (Serenade®, Rhapsody®) and B. velezensis FZB42 (Taegro®, Rhizovital®) are among the commercial biocontrol agents from the B. subtilis group (Lahlali et al., 2022; Yadav et al., 2022). These strains are continuously attracting researchers and industrials thanks to their intrinsic ability to form resistant endospores that allow stable formulations, relatively rapid growth on different substrates, and the secretion of a wealth of bioactive secondary metabolites retaining key biocontrol functions (Prasad et al., 2023). The biosynthesis of these biochemicals involves up to 10% of the bacterial genome and includes a huge diversity of compounds produced via non-ribosomal and ribosomal pathways (Grubbs et al., 2017; Borriss, 2020; Yin et al., 2023).
Cyclic lipopeptides are a group of compounds with a fatty acid and peptide moiety that are produced via the non-ribosomal pathway involving a multi-modular enzyme complex (Iqbal et al., 2023). This machinery gives rise to a large chemical diversity with different structures, based on their amino acid sequence or on the length of the fatty acid chain. These metabolites are classified into the heptapeptides surfactins (pumilacidins/lichenisins) and iturins (iturin A, mycosubtilin, bacillomycin) and the decapeptides fengycins (plipastatins); together with their homologues of different alkyl chain lengths (C12 - C17 for surfactins, C13-C17 for iturins and C14-C18 for fengycins) (Théatre et al., 2022). They are produced by members of the Bacillus subtilis group and are known to be involved in the induction of resistance in plants (Pršić and Ongena, 2020; Dimkić et al., 2022; Mahapatra et al., 2022) and, thanks to their amphiphilic nature, exhibit antimicrobial activities against a wide range of pathogenic microorganisms by penetrating and disrupting the cell membrane of target organisms (Fira et al., 2018; Ngalimat et al., 2021; Puan et al., 2023).
Polyketides are bioactive metabolites synthetized via pathways catalyzed either by the polyketide synthases (difficidins and macrolactins) or by the hybrid polyketide synthases/non-ribosomal peptide synthetases (bacillaene) and are structurally composed of an alternation of polyenes and carbonyl groups (Iqbal et al., 2023). They retain consistent antibacterial activity against several phytopathogens either by interfering with protein synthesis or by damaging the bacterial cell wall (Fazle Rabbee and Baek, 2020; Miao et al., 2023; Zhang N. et al., 2023). The siderophore bacillibactin is a catecholate peptide also produced via the non-ribosomal pathway that retains a high affinity for iron. This property is very important in bacterial niche competition, helping bacilli to gain advantage over their competitors by chelating available iron in the environment (Yu et al., 2011). The dipeptide bacilysin is biosynthesized via a typical non-ribosomal pathway not shared by the lipopeptide/polyketide/siderophore trio. It exhibits toxicity against bacteria by blocking peptidoglycan biosynthesis (Borriss, 2015) and against fungi by probably inhibiting fungal glycans in a manner similar to the inhibition of bacterial peptidoglycan synthesis (Han X. et al., 2021).
Ribosomally produced and post-translationally modified peptides include bacteriocins and lantibiotics (such as amylocyclicin, amylolysin, plantazolicin, subtilin, pumilarin or LCI) known for their high inhibitory potential against bacterial pathogens, either by vascularization of the protoplasm or by pore formation or cell disruption (Sumi et al., 2015). In addition to these soluble metabolites, Bacillus volatile compounds (hydrocarbons nonane and tridecane, acetoin, 2,3-butanediol, benzaldehyde, 3-methylpropanoic acid, methylbenzene, benzothiazole, etc.) have been reported to inhibit the growth of some fungi, bacteria, and nematodes by disrupting cell wall integrity (Caulier et al., 2019; Kai, 2020; Iqbal et al., 2023).
8 The potential of Bacillus-based biocontrol in the GLCCA region, a myth or reality
The market for biocontrol agents based on strains of the B. subtilis group for the control of plant diseases is growing rapidly around the world but no product based on this bacterium is registered in the region. However, some promising preliminary studies using B. velezensis strains to control fungal diseases in Burundi and DRC have demonstrated the potential of these bacteria to adapt to local agro-ecological conditions, which could pave the way for their possible future dissemination and adoption by local farmers. Bacillus velezensis S499, isolated in Ituri/DRC in 1950s (Delcambe and Devignat, 1957), is effective against Fusarium sp. on tomato (65-70% of disease reduction) under field conditions in Burundi, and lipopeptide-mediated ISR is proposed as the main involved mechanism (Nihorimbere et al., 2010). Lipopeptides secreted by the same strain showed high in vitro antagonistic activity against pathogens isolated from maize cob such as Rhizopus stolonifer, Penicillium variable, Fusarium verticillioides. In the same study, S499 was able to efficiently reduce disease severity (39-67%) caused by these fungi in greenhouse and field conditions (Kulimushi et al., 2018). In addition, B. velezensis GA1 and its cyclic lipopeptides played an important role in the control of peanut stem rot disease caused by Athelia rolfsii, providing up to 60% of protection (Korangi Alleluya et al., 2023).
Furthermore, a number of studies have demonstrated the potential of Bacillus isolates in controlling a range of important and widespread phytopathogens reported in the GLCCA. For example, B. altitudinis and B. velezensis were shown to control rice blast caused by Pyricularia oryzae (15% and 25% of disease reduction, respectively) by direct antagonism and ISR involving iturin and fengycin (Lam et al., 2021). Volatiles from B. amyloliquefaciens NJN-6 reduce the mycelial growth of the banana pathogen F. oxysporum f.sp. cubense in vitro by up to 30-40% (Yuan et al., 2012). B. subtilis AUBB20 inhibited the growth of the coffee pathogen F. xylarioides through its secreted lytic enzymes (Muleta et al., 2007). Antagonistic activities of Bacillus subtilis strains have also been reported against fungi and oomycetes, including Alternaria linariae (da Silva Junior et al., 2023), Sclerotinia sclerotiorum, Rhizoctonia solani (Al-Mutar et al., 2023), Colletotrichum lindemuthianum (Martins et al., 2019), Mycosphaerella fijiensis (Gutierrez-Monsalve et al., 2015), Verticillium dahliae (Dhouib et al., 2019), Fusarium oxysporum f. sp. lycopersici (Aydi Ben Abdallah et al., 2017), Colletotrichum gloeosporioides (Luna-Bulbarela et al., 2018) and Phytophthora infestans (Zhang J. et al., 2023b).
The biocontrol activity of Bacillus has also been demonstrated against important bacterial phytopathogens. For example, the application of B. subtilis to potato plants infected with R. solanacearum reduced the incidence of wilt by about 50% (Elazouni et al., 2019). Tomato bacterial wilt caused by the same bacterium was efficiently controlled by B. methylotrophicus DR-08 due to its secreted metabolites oxydifficidin and difficidin (Im et al., 2019). Bacillus megaterium USB2103 efficiently controlled common bean bacterial wilt caused by Xanthomonas axonopodis pv. phaseoli through ISR mechanisms (Giorgio et al., 2016). Brown sheath blight of rice caused by Pseudomonas fuscovaginae was successfully controlled (76.6%) by B. amyloliquefaciens Bk7 (Ullah Kakar et al., 2014). Other examples of Bacillus spp. with potential applications in the control of fungal and bacterial diseases for crop protection in the GLCCA are shown in Table 3.
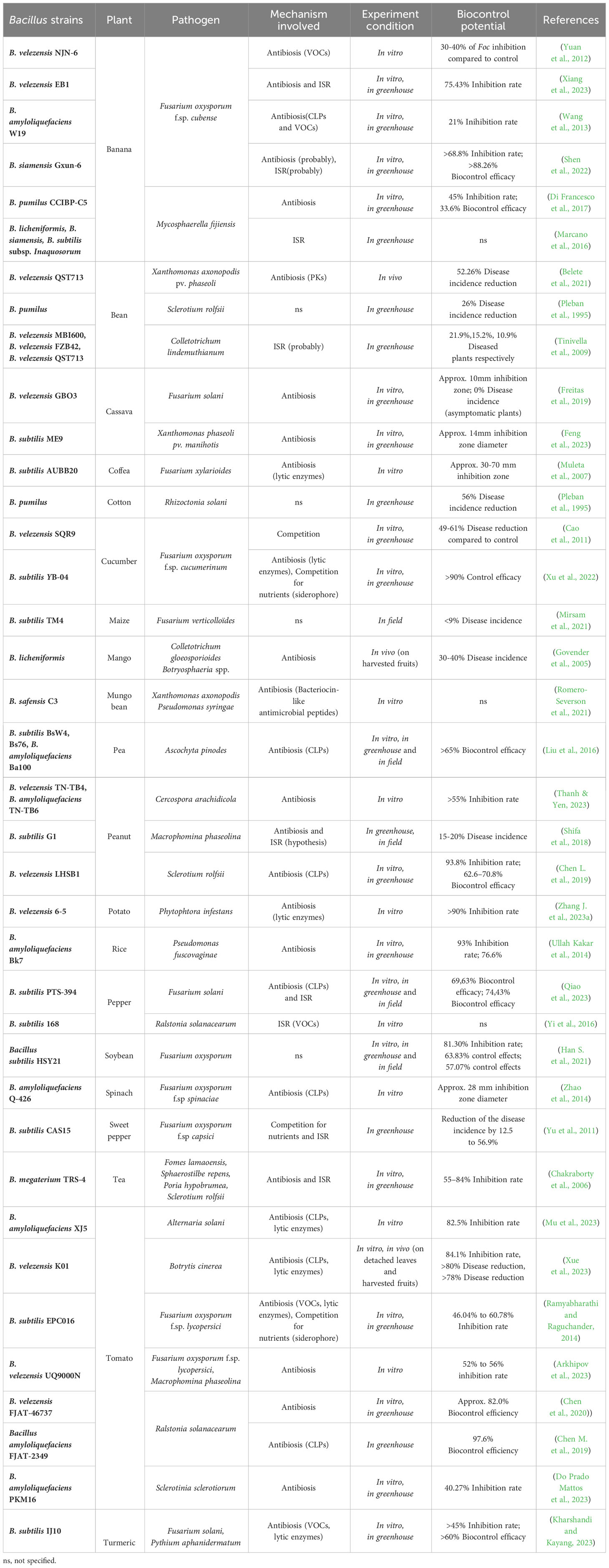
Table 3 Some global success stories of Bacillus isolates (subtilis group) in biocontrol of important crop pathogens reported in the GLCCA.
In addition to their efficacy against phytopathogenic fungi and bacteria, some Bacillus spp. have been reported for their potential to control nematodes, insects and weeds (Mnif and Ghribi, 2015). For instance, surfactins from B. velezensis S499 showed insecticidal activity against the fruit fly Drosophila melanogaster (Assié et al., 2002). The wheat rhizosphere Bacillus altitudinis D30202 was shown to have bioherbicidal potential on the grass Avena fatua L. (Ma et al., 2023). Bacillus strains such as B. altitudinis, B. pumilis, B. velezensis, B. mojavensis and B. megaterium showed nematicidal activity against Meloidogyne incognita (Padgham and Sikora, 2007; Huang et al., 2010; Xiang et al., 2017; El-Nagdi and Abd-El-Khair, 2019; Guimarães Pacifico et al., 2021; Ye et al., 2022) and Heterodera glycines, which threatens soybean production (Dalvan Do Nascimento et al., 2022). Cell-free culture supernatants and volatiles of B. amyloliquefaciens BV03, PTA4838 and B. velezensis MBI600 killed more than 85% of Helicotylenchus dihystera infesting soybean (Camatti et al., 2023). So even if very few products have been developed to the market scale so far, the results already available are promising for the design of bionematicides, bioinsecticides and bioherbicides based on Bacillus.
9 Conclusions
This review summarizes the current phytosanitary situation in the GLCCA region and the possibility of shifting to safer and more environmentally friendly alternatives to chemical pesticides for sustainable agriculture. Several diseases and pests are devastating many crops, leading to the use of chemical pesticides despite their known hazardous nature. Although pesticide regulations exist in the three countries, their implementation is problematic with one third of registered pesticides already banned from market in EU and numerous mishandling incidents. For the sake of the terrestrial and aquatic ecosystems, governments in the GLCCA need to take urgent action to mitigate the harmful effects of chemical pesticides. The regulatory systems should be reviewed and updated, and other less toxic pesticides or more sustainable control strategies should be promoted. Inspectors should be increased in number and motivation, and retailers and farmers should be made aware of pesticide regulations and the associated health and environmental risks.
Globally available alternatives inspired by integrated pest management (IPM), including good agricultural practices and biological control, are not widely used and/or in some cases non-existent. Although a limited number of biocontrol products are registered in the region, their use has yet to become a “reality”. Moreover, all available products are used against insect pests but there is no biological solution that has been developed in practical terms to fight highly prevalent fungal and bacterial diseases. Some Bacillus isolates clearly represent promising tools for the control of these microbial diseases, and their inherent ability to form resistant endospores, to grow on poor substrates such as agro-industrial residues in semi-solid systems, and their potential to secrete a vast array of bioactive secondary metabolites (Kumar et al., 2021; Bouassida et al., 2023) would allow designing stable, rich, and affordable formulations of products suitable for the local socio-economic context. Unfortunately, although some preliminary studies have demonstrated efficacy in controlling some important local pathogens, these Bacillus-based products are still a “myth” for local farmers. Efforts should be directed toward promoting the use of already available Bacillus-based products against endemic pathogens devastating crops in local farming systems. However, bioprospecting for indigenous Bacillus strains that are well-adapted to local agro-ecological conditions and retain strong biocontrol properties should be promoted to ensure sustainable plant disease management in the region. The great potential of indigenous Bacillus strains over the commercial ones has been shown, for example in the management of grapevine trunk disease (Langa-Lomba et al., 2023) and tropical fruit diseases (Reyes-Estebanez et al., 2020). Governments of the GLCCA countries should not only encourage the investment in such biocontrol products, which are virtually absent in the region, but also facilitate their registration and commercialization.
Author contributions
GN: Conceptualization, Writing – original draft. VKA: Conceptualization, Writing – original draft. FN: Conceptualization, Writing – original draft. VN: Funding acquisition, Writing – review & editing. AL: Conceptualization, Supervision, Writing – review & editing. MO: Conceptualization, Funding acquisition, Project administration, Supervision, Writing – review & editing.
Funding
The author(s) declare financial support was received for the research, authorship, and/or publication of this article. The work of FN and GN was supported by the Académie de Recherche et d’Enseignement Supérieur-Commission de Coopération au Développement (ARES-CDD), Fédération Wallonie-Bruxelles) through the PRD instrument (Title: Vers une agriculture plus performante et durable au Burundi: application de microorganismes pour améliorer la santé et la croissance des plantes). VKA was funded by ARES-CCD through the “Bourse exceptionnelle” program, University of Liège scholarship and by the Schlumberger Foundation Faculty for the Future program. MO is Research Director at the FRS-FNRS (National Fund for Scientific Research) in Belgium.
Acknowledgments
A particular gratitude is toward Mr. Christian Tebila and Mr. Alex Sulu of MINAGRI-DRC and the Crop Protection Department of MINAGRI-Burundi for their helpful contribution by providing data on pesticide regulation and importation.
Conflict of interest
The authors declare that the research was conducted in the absence of any commercial or financial relationships that could be construed as a potential conflict of interest.
Publisher’s note
All claims expressed in this article are solely those of the authors and do not necessarily represent those of their affiliated organizations, or those of the publisher, the editors and the reviewers. Any product that may be evaluated in this article, or claim that may be made by its manufacturer, is not guaranteed or endorsed by the publisher.
Supplementary material
The Supplementary Material for this article can be found online at: https://www.frontiersin.org/articles/10.3389/fpls.2024.1349357/full#supplementary-material
References
Abdelaziz, A. M., Hashem, A. H., El-Sayyad, G. S., El-Wakil, D. A., Selim, S., Alkhalifah, D. H. M., et al. (2023). Biocontrol of soil borne diseases by plant growth promoting rhizobacteria. Trop. Plant Pathol. 48, 105–127. doi: 10.1007/s40858-022-00544-7
Adams, I. P., Harju, V. A., Hodges, T., Hany, U., Skelton, A., Rai, S., et al. (2014). First report of maize lethal necrosis disease in Rwanda. New Dis. Rep. 29, 22. doi: 10.5197/j.2044-0588.2014.029.022
Ali, M. A., Lou, Y., Hafeez, R., Li, X., Hossain, A., Xie, T., et al. (2021). Functional analysis and genome mining reveal high potential of biocontrol and plant growth promotion in nodule-inhabiting bacteria within Paenibacillus polymyxa Complex. Front. Microbiol. 11. doi: 10.3389/fmicb.2020.618601
Al-Mutar, D. M. K., Noman, M., Alzawar, N. S. A., Qasim, H. H., Li, D., Song, F. (2023). The Extracellular lipopeptides and volatile organic compounds of Bacillus subtilis DHA41 display broad-spectrum antifungal activity against soil-borne phytopathogenic fungi. J. Fungi 9, 797. doi: 10.3390/jof9080797
Anckaert, A., Arguelles-Arias, A., Hoff, G., Calonne-Salmon, M., Declerck, S., Ongena, M. (2021). The use of Bacillus spp. as bacterial biocontrol agents to control plant diseases,” in Microbial Bioprotectants for Plant Disease Management. Eds. Köhl, J., Ravensberg, W. (Cambridge, Cambridgeshire: Burleigh Dodds Science Publishing), 1–54. doi: 10.19103/as.2021.0093.10
ARECO-Rwanda Nziza (2020) IPEN toxics-free SDGS campaign highly hazardous pesticides (HHPS) Rwanda situation report. Available at: https://ipen.org/sites/default/files/documents/final_Rwanda_hhps_report_areco_10_july_2020.pdf.
Arguelles Arias, A., Ongena, M., Devreese, B., Terrak, M., Joris, B., Fickers, P. (2013). Characterization of amylolysin, a novel lantibiotic from Bacillus amyloliquefaciens GA1. PloS One 8, e83037. doi: 10.1371/journal.pone.0083037
Arguelles-Arias, A., Ongena, M., Halimi, B., Lara, Y., Brans, A., Joris, B., et al. (2009). Bacillus amyloliquefaciens GA1 as a source of potent antibiotics and other secondary metabolites for biocontrol of plant pathogens. Microb. Cell Fact. 8, 63. doi: 10.1186/1475-2859-8-63
Arkhipov, A., Carvalhais, L. C., Schenk, P. M. (2023). PGPR control Phytophthora capsici in tomato through induced systemic resistance, early hypersensitive response and direct antagonism in a cultivar-specific manner. Eur. J. Plant Pathol. 167, 811–832. doi: 10.1007/s10658-023-02734-8
Assié, L. K., Deleu, M., Arnaud, L., Paquot, M., Thonart, P., Gaspar, C., et al. (2002). Insecticide activity of surfactins and iturins from a biopesticide Bacillus subtilis Cohn (S499 strain). Meded. Rijksuniv. Gent. Fak. Landbouwkd. Toegep. Biol. Wet. 67, 647–655. Avalaible at: https://pubmed.ncbi.nlm.nih.gov/12696433/
Aydi Ben Abdallah, R., Stedel, C., Garagounis, C., Nefzi, A., Jabnoun-Khiareddine, H., Papadopoulou, K. K., et al. (2017). Involvement of lipopeptide antibiotics and chitinase genes and induction of host defense in suppression of Fusarium wilt by endophytic Bacillus spp. in tomato. Crop Prot. 99, 45–58. doi: 10.1016/j.cropro.2017.05.008
Balasha, A. M., Mulume, D. A., Mwisha, S. W., Fyama, J. N. M., Kalumbu, J. T. (2023). Utilisation des pesticides en cultures maraîchères sur l’île d’Idjwi à l’est de la République démocratique du Congo: connaissances et pratiques des agriculteurs. Cahiers Agricultures 32, 5. doi: 10.1051/CAGRI/2022033
Bararyenya, A., Nduwimana, A., Nyawakira, D. (2018). Assessment of opportunities for Burundian Small-scale potato farmers to increase productivity and income. Potato Res. 61, 73–88. doi: 10.1007/s11540-018-9359-2
Barka, E. A., Vatsa, P., Sanchez, L., Gaveau-Vaillant, N., Jacquard, C., Klenk, H.-P., et al. (2016). Taxonomy, physiology, and natural products of actinobacteria. Microbiol. Mol. Biol. Rev. 80, 1–43. doi: 10.1128/mmbr.00019-15
Bassily, N. (2022) Burundi: Toxic pesticides threaten health and environment (Syfia Grands Lacs). Available at: https://wire.farmradio.fm/farmer-stories/3-Burundi-toxic-pesticides-threaten-health-and-environment-syfia-grands-lacs/ (Accessed September 12, 2022).
Belete, T., Kurtulus Bastas, K., Francesconi, S., Balestra, G. M. (2021). Biological effectiveness of Bacillus subtilis on common bean bacterial blight. J. Plant Pathol. 103, 249–258. doi: 10.1007/s42161-020-00727-8
Belga (2020) La RDC à son tour touchée par les criquets ravageurs, une première depuis 1944. Available at: https://www.rtbf.be/article/la-rdc-a-son-tour-touchee-par-les-criquets-ravageurs-une-premiere-depuis-1944-10443038 (Accessed February 27, 2022).
Beneduzi, A., Ambrosini, A., Passaglia, L. M. P. (2012). Plant growth-promoting rhizobacteria (PGPR): their potential as antagonists and biocontrol agents. Genet. Mol. Biol. 35, 1044–1051. doi: 10.1590/S1415-47572012000600020
Bigirimana, S., Barumbanze, P., Ndayihanzamaso, P., Shirima, R., Legg, J. P. (2011). First report of cassava brown streak disease and associated Ugandan cassava brown streak virus in Burundi. New Dis. Rep. 24, 26. doi: 10.5197/j.2044-0588.2011.024.026
Bigirimana, S., Barumbanze, P., Obonyo, R., Legg, J. P. (2004). First evidence for the spread of east African cassava mosaic virus - Uganda (EACMV-UG) and the pandemic of severe cassava mosaic disease to Burundi. Plant Pathol. 53, 231. doi: 10.1111/J.0032-0862.2004.00971.x
Biruma, M., Pillay, M., Tripathi, L., Blomme, G., Abele, S., Mwangi, M., et al. (2007). Banana Xanthomonas wilt: a review of the disease, management strategies and future research directions. Afr. J. Biotechnol. 6, 953–962. Available at: https://biblio.iita.org/documents/Biruma_et_al._-_BXW_review_paper_-_Afr_J_Biotech_April_2007.pdf-089232b3223892894f81a617723522f2.pdf
Bishnoi, U. (2015). “PGPR interaction: an ecofriendly approach promoting the sustainable agriculture system,” in Advances in botanical research. Eds. Bais, H., Sherrie, J. (London, UK: Academic Press, Elsevier), 81–113. doi: 10.1016/bs.abr.2015.09.006
Bjornlund, V., Bjornlund, H., Van Rooyen, A. F. (2020). Why agricultural production in sub-Saharan Africa remains low compared to the rest of the world – A historical perspective. Int. J. Water Resour. Dev. 36, 20–53. doi: 10.1080/07900627.2020.1739512
Boloy, F. N., Nkosi, B. I., Losimba, J. K., Bungamuzi, C. L., Siwako, H. M., Walunkonka Balowe, F., et al. (2014). Assessing incidence, development and distribution of banana bunchy top disease across the main plantain and banana growing regions of the Democratic Republic of Congo. Afr. J. Agric. Res. 9, 2611–2623. doi: 10.5897/AJAR2014.8751
Bonaterra, A., Badosa, E., Daranas, N., Francés, J., Roselló, G., Montesinos, E. (2022). Bacteria as biological control agents of plant diseases. Microorganisms 10, 1759. doi: 10.3390/microorganisms10091759
Borriss, R. (2015). “Bacillus, a plant-beneficial bacterium,” in Principles of plant-microbe interactions. Ed. Lugtenberg, B. (Cham, Bavaria: Springer International Publishing), 379–391. doi: 10.1007/978-3-319-08575-3_40
Borriss, R. (2020). “Phytostimulation and biocontrol by the plant-associated Bacillus amyloliquefaciens FZB42: An update,” in Phyto-microbiome in stress regulation. Environmental and microbial biotechnology. Eds. Kumar, M., Kumar, V., Prasad, R. (Singapore: Springer). doi: 10.1007/978-981-15-2576-6_1
Bouassida, M., Mnif, I., Hammami, I., Triki, M. A., Ghribi, D. (2023). Bacillus subtilis SPB1 lipopeptide biosurfactant: antibacterial efficiency against the phytopathogenic bacteria Agrobacterium tumefaciens and compared production in submerged and solid state fermentation systems. Food Sci. Biotechnol. 32, 1595–1609. doi: 10.1007/s10068-023-01274-5
Busogoro, J. P., Jijakli, M. H., Lepoivre, P. (1999). Identification of a novel source of resistance to angular leaf spot disease of common bean within the secondary gene pool. Plant Breed. 118, 417–423. doi: 10.1046/J.1439-0523.1999.00413.X
Camatti, G., Mulinari dos Santos, F., dos Santos Rodrigues Júnior, G.L., Pereira Camargo, D., Stefanello Manfio, G., Rodrigo Pereira Santos, J., et al. (2023). Bacillus-and Trichoderma-based products control the spiral nematode Helicotylenchus dihystera in soybean. Rhizosphere 27, 100717. doi: 10.1016/j.rhisph.2023.100717
Cao, Y., Zhang, Z., Ling, N., Yuan, Y., Zheng, X., Shen, B., et al. (2011). Bacillus subtilis SQR 9 can control Fusarium wilt in cucumber by colonizing plant roots. Biol. Fertility Soils 47, 495–506. doi: 10.1007/s00374-011-0556-2
Casinga, C. M., Shirima, R. R., Mahungu, N. M., Tata-Hangy, W., Bashizi, K. B., Munyerenkana, C. M., et al. (2021). Expansion of the cassava brown streak disease epidemic in Eastern Democratic Republic of Congo. Plant Dis. 105, 2177–2188. doi: 10.1094/PDIS-05-20-1135-RE
Caulier, S., Gillis, A., Colau, G., Licciardi, F., Liépin, M., Desoignies, N., et al. (2018). Versatile antagonistic activities of soil-borne Bacillus spp. and Pseudomonas spp. against Phytophthora infestans and other potato pathogens. Front. Microbiol. 9. doi: 10.3389/fmicb.2018.00143
Caulier, S., Nannan, C., Gillis, A., Licciardi, F., Bragard, C., Mahillon, J. (2019). Overview of the antimicrobial compounds produced by members of the Bacillus subtilis Group. Front. Microbiol. 10. doi: 10.3389/fmicb.2019.00302
Chakraborty, U., Chakraborty, B., Basnet, M. (2006). Plant growth promotion and induction of resistance in Camellia sinensis by Bacillus megaterium. J. Basic Microbiol. 46, 186–195. doi: 10.1002/jobm.200510050
Chen, M., Wang, J., Liu, B., Zhu, Y., Xiao, R., Yang, W., et al. (2020). Biocontrol of tomato bacterial wilt by the new strain Bacillus velezensis FJAT-46737 and its lipopeptides. BMC Microbiol. 20, 160. doi: 10.1186/s12866-020-01851-2
Chen, M. C., Wang, J. P., Zhu, Y. J., Liu, B., Yang, W. J., Ruan, C. Q. (2019). Antibacterial activity against Ralstonia solanacearum of the lipopeptides secreted from the Bacillus amyloliquefaciens strain FJAT-2349. J. Appl. Microbiol. 126, 1519–1529. doi: 10.1111/jam.14213
Chen, L., Wu, Y. D., Chong, X. Y., Xin, Q. H., Wang, D. X., Bian, K. (2019). Seed-borne endophytic Bacillus velezensis LHSB1 mediate the biocontrol of peanut stem rot caused by Sclerotium rolfsii. J. Appl. Microbiol. 128, 803–813. doi: 10.1111/jam.14508
Cokola, M. C., Ndjadi, S. S., Bisimwa, E. B., Ahoton, L. E., Francis, F. (2021). First report of Spodoptera frugiperda (Lepidoptera: Noctuidae) on Onion (Allium cepa L.) in South Kivu, Eastern DR Congo. Rev. Bras. Entomologia 65, e20200083. doi: 10.1590/1806-9665-RBENT-2020-0083
Costa, A., Corallo, B., Amarelle, V., Stewart, S., Pan, D., Tiscornia, S., et al. (2022). Paenibacillus sp. Strain UY79, isolated from a root nodule of Arachis villosa, displays a broad spectrum of antifungal activity. Appl. Environ. Microbiol. 88, e01645–e01621. doi: 10.1128/AEM.01645-21
Coyne, D. L., Cortada, L., Dalzell, J. J., Claudius-Cole, A. O., Haukeland, S., Luambano, N., et al. (2018). Plant-parasitic nematodes and food security in sub-saharan Africa. Annu. Rev. Phytopathol. 56, 381–403. doi: 10.1146/annurev-phyto-080417-045833
Dalvan Do Nascimento, D., Rodrigues, M., Ferreira, R. J., Marchioro, V., Macedo Da Silva, E., Alberto, C., et al. (2022). Soybean growth-promotion and Heterodera glycines suppression in two application methods of Bacillus strains. Biol. Control 175, 1049–9644. doi: 10.1016/j.biocontrol.2022.105039
da Silva Junior, A. L., Borges, Á.V., da Silva, H. A. O., Leite, I. C. H. L., Alves, K. S., de Medeiros, L. S., et al. (2023). Lipopeptide-enriched extracts of Bacillus velezensis B157 for controlling tomato early blight. Crop Prot. 172, 106317. doi: 10.1016/j.cropro.2023.106317
De Kievit, T. R. (2009). Quorum sensing in Pseudomonas aeruginosa biofilms. Environ. Microbiol. 11, 279–288. doi: 10.1111/j.1462-2920.2008.01792.x
Delcambe, L., Devignat, R. (1957). L’iturine, nouvel antibiotique d’origine congolaise. Acad. R. Sci. Coloniales 6, 1–77. Available at: https://www.kaowarsom.be/fr/node/5930
Dhouib, H., Zouari, I., Ben Abdallah, D., Belbahri, L., Taktak, W., Triki, M. A., et al. (2019). Potential of a novel endophytic Bacillus velezensis in tomato growth promotion and protection against Verticillium wilt disease. Biol. Control 139, 104092. doi: 10.1016/j.biocontrol.2019.104092
Di Francesco, A., Milella, F., Mari, M., Roberti, R. (2017). A preliminary investigation into Aureobasidium pullulans as a potential biocontrol agent against Phytophthora infestans of tomato. Biol. Control 114, 144–149. doi: 10.1016/J.BIOCONTROL.2017.08.010
Dimkić, I., Janakiev, T., Petrović, M., Degrassi, G., Fira, D. (2022). Plant-associated Bacillus and Pseudomonas antimicrobial activities in plant disease suppression via biological control mechanisms-A review. Physiol. Mol. Plant Pathol. 117, 101754. doi: 10.1016/j.pmpp.2021.101754
Do Prado Mattos, A., Rissato, B. B., Itako, A. T., Júnior, J. B. T., Estrada, K. R. F. S. (2023). Bacillus amyloliquefaciens PKM16 acts as an antagonist of white mold and an inducer of defense enzymes in tomato plants. Acta Scientiarum. Agron. 45, e59586. doi: 10.4025/actasciagron.v45i1.59586
DunhamTrimmer (2023) DunhamTrimmer® Global biocontrol report: market overview, trends, drivers and insights. Available at: https://dunhamtrimmer.com/reports/global-biocontrol-market-report/.
Dunlap, C. A. (2019). Taxonomy of registered Bacillus spp. strains used as plant pathogen antagonists. Biol. Control 134, 82–86. doi: 10.1016/j.biocontrol.2019.04.011
Dushimirimana, S., Gasogo, A., Kazitsa, E.-G., Hance, T. (2016). Dynamics and seasonal variability of Bemesia tabaci colonies in cassava. Modern Agric. Sci. Technol. 2, 26–32. doi: 10.15341/mast(2375-9402)/01.02.2016/004
Dutta, S., Lee, Y. H. (2022). High-throughput identification of genes influencing the competitive ability to obtain nutrients and performance of biocontrol in Pseudomonas putida JBC17. Sci. Rep. 12, 872. doi: 10.1038/s41598-022-04858-z
Elazouni, I., Abdel-Aziz, S., Rabea, A. (2019). Microbial efficacy as biological agents for potato enrichment as well as bio-controls against wilt disease caused by Ralstonia solanacearum. World J. Microbiol. Biotechnol. 35, 30. doi: 10.1007/s11274-019-2596-y
El-Nagdi, W. M. A., Abd-El-Khair, H. (2019). Application of Bacillus species for controlling root-knot nematode Meloidogyne incognita in eggplant. Bull. Natl. Res. Cent. 43, 154. doi: 10.1186/s42269-019-0187-6
El-Saadony, M. T., Saad, A. M., Soliman, S. M., Salem, H. M., Ahmed, A. I., Mahmood, M., et al. (2022). Plant growth-promoting microorganisms as biocontrol agents of plant diseases: Mechanisms, challenges and future perspectives. Front. Plant Sci. 13. doi: 10.3389/fpls.2022.923880
Etesami, H., Ryong Jeong, B., Glick, B. R. (2023). Potential use of Bacillus spp. as an effective biostimulant against abiotic stresses in crops-A review. Curr. Res. Biotechnol. 5, 100128. doi: 10.1016/j.crbiot.2023.100128
EU Food Safety (2023) EU pesticides database. Available at: https://food.ec.europa.eu/plants/pesticides/eu-pesticides-database_en (Accessed September 18, 2023).
FAAPA (2021) Le secteur agricole contribue à 36% dans la formation du PIB en RDC. Available at: http://www.faapa.info/blog/le-secteur-agricole-contribue-a-36-dans-la-formation-du-pib-en-rdc/ (Accessed January 20, 2021).
FAO (2022) FAO employs innovative plant pest control solutions in Eastern Africa. Available at: https://reliefweb.int/report/Burundi/fao-employs-innovative-plant-pest-control-solutions-eastern-africa/ (Accessed April 10, 2022).
FAO (2023) En Afrique centrale, environ 42,7 millions de personnes sont en situation d’insécurité alimentaire et nutritionnelle. Available at: https://www.fao.org/africa/news/detail-news/fr/c/1273669// (Accessed June 20, 2023).
Farrow, A., Muthoni-Andriatsitohaina, R. (2020). Atlas for common bean production in Africa. 2nd Edition (Nairobi: CIAT Publication).
Fazle Rabbee, M., Baek, K.-H. (2020). Antimicrobial activities of lipopeptides and polyketides of Bacillus velezensis for Agricultural Applications. Molecules 25, 4973. doi: 10.3390/molecules25214973
Feng, Y., Zhang, Y., Shah, O. U., Luo, K., Chen, Y. (2023). Isolation and identification of endophytic bacteria Bacillus sp. ME9 that exhibits biocontrol activity against Xanthomonas phaseoli pv. manihotis. Biology 12, 1231. doi: 10.3390/biology12091231
Fira, D., Dimki, I., Beri, T., Lozo, J. (2018). Biological control of plant pathogens by Bacillus species. J. Biotechnol. 285, 44–55. doi: 10.1016/j.jbiotec.2018.07.044
Flemming, H. C., Wingender, J., Szewzyk, U., Steinberg, P., Rice, S. A., Kjelleberg, S. (2016). Biofilms: an emergent form of bacterial life. Nat. Rev. Microbiol. 14, 563–575. doi: 10.1038/nrmicro.2016.94
FPS Health (2023) Phytoweb | Plant protection and Fertilising Products. Available at: https://fytoweb.be/en (Accessed September 11, 2023).
Freitas, M. A., Medeiros, F. H. V., Melo, I. S., Pereira, P. F., Peñaflor, M. F. G. V., Bento, J. M. S., et al. (2019). Stem inoculation with bacterial strains Bacillus amyloliquefaciens (GB03) and Microbacterium imperiale (MAIIF2a) mitigates Fusarium root rot in cassava. Phytoparasitica 47, 135–142. doi: 10.1007/s12600-018-0706-2
Gaberell, L., Viret, G. (2022) Les géants de l’agrochimie gagnent des milliards grâce à des pesticides cancérogènes ou néfastes pour les abeilles. Available at: https://www.publiceye.ch/fr/thematiques/pesticides/analyse-ventes-pesticides-2018 (Accessed November 23, 2022).
Gaidashova, S., Karemera, F., Karamura, E. (2010). Agronomic performance of introduced banana varieties in lowlands of Rwanda. Afr. Crop Sci. J. 16, 9–16. doi: 10.4314/acsj.v16i1.54321
Giorgio, A., Lo Cantore, P., Shanmugaiah, V., Lamorte, D. (2016). Rhizobacteria isolated from common bean in southern Italy as potential biocontrol agents against common bacterial blight. Eur. J. Plant Pathol. 144, 297–309. doi: 10.1007/s10658-015-0767-8
Govender, V., Korsten, L., Sivakumar, D. (2005). Semi-commercial evaluation of Bacillus licheniformis to control mango postharvest diseases in South Africa. Postharvest Biol. Technol. 38, 57–65. doi: 10.1016/j.postharvbio.2005.04.005
Grubbs, K. J., Bleich, R. M., Santa Maria, K. C., Allen, S. E., Farag, S., Shank, E. A., et al. (2017). Large-scale bioinformatics Analysis of Bacillus genomes uncovers conserved roles of natural products in bacterial physiology. MSystems 2, 10–1128. doi: 10.1128/mSystems.00040-17
GUCE (2022) Types et quantités des pesticides importés en RDC. Available at: https://invoice.segucerdc.cd/invoice/Account/Login (Accessed February 02, 2021).
Guimarães Pacifico, M., Eckstein, B., Bettiol, W. (2021). Screening of Bacillus for the development of bioprotectants for the control of Fusarium oxysporum f. sp. vasinfectum and Meloidogyne incognita. Biol. Control 164, 104764. doi: 10.1016/j.biocontrol.2021.104764
Gutierrez-Monsalve, J. A., Mosquera, S., González-Jaramillo, L. M., Mira, J. J., Villegas-Escobar, V. (2015). Effective control of black sigatoka disease using a microbial fungicide based on Bacillus subtilis EA-CB0015 culture. Biol. Control 87, 39–46. doi: 10.1016/j.biocontrol.2015.04.012
Han, S., Chen, J., Zhao, Y., Cai, H., Guo, C. (2021). Bacillus subtilis HSY21 can reduce soybean root rot and inhibit the expression of genes related to the pathogenicity of Fusarium oxysporum. Pesticide Biochem. Physiol. 178, 104916. doi: 10.1016/j.pestbp.2021.104916
Han, X., Shen, D., Xiong, Q., Bao, B., Zhang, W., Dai, T., et al. (2021). The plant-beneficial rhizobacterium Bacillus velezensis FZB42 controls the soybean pathogen Phytophthora sojae due to bacilysin production. Appl. Environ. Microbiol. 87, e01601–e01621. doi: 10.1128/AEM.01601-21
Harahagazwe, D., Ndayiragije, P., Ntimpirangeza, M. (2007). Les maladies et ravageurs de quelques cultures à racines et tubercules (ISABU) Bujumbura. Available at: https://www.researchgate.net/publication/317175756_Pests_and_diseases_of_selected_root_and_tuber_crops_grown_in_Burundi_in_French.
Helepciuc, F. E., Todor, A. (2023). Making the best of research investment in pathogens control through biocontrol. How is research correlated with agricultural microbial biological control product availability? PloS Pathog. 19, e1011071. doi: 10.1371/journal.ppat.1011071
Huang, Y., Xu, C. K., Ma, L., Zhang, K. Q., Duan, C. Q., Mo, M. H. (2010). Characterisation of volatiles produced from Bacillus megaterium YFM3.25 and their nematicidal activity against Meloidogyne incognita. Eur. J. Plant Pathol. 126, 417–422. doi: 10.1007/s10658-009-9550-z
Im, S. M., Yu, N. H., Won Joen, H., Kim, S. O., Park, H. W., Park, A. R., et al. (2019). Biological control of tomato bacterial wilt by oxydifficidin and difficidin-producing Bacillus methylotrophicus DR-08. Pesticide Biochem. Physiol. 163, 130–137. doi: 10.1016/j.pestbp.2019.11.007
IPC (2021) Analyse de la sécurité alimentaire aiguë et de la malnutrition aiguë de l’IPC en RDC. Available at: https://www.ipcinfo.org/fileadmin/user_upload/ipcinfo/docs/IPC_DRC_Acute_FoodInsec_Malnutrition_2021Sept2022Aug_Report_French.pdf.
Iqbal, S., Begum, F., Rabaan, A. A., Aljeldah, M., Al Shammari, B. R., Alawfi, A., et al. (2023). Classification and multifaceted potential of secondary metabolites produced by Bacillus subtilis Group: A Comprehensive Review. Molecules 28, 927. doi: 10.3390/molecules28030927
Isabirye, B. E., Rwomushana, I. (2016). Current and future potential distribution of maize chlorotic mottle virus and risk of maize lethal necrosis disease in Africa. J. Crop Prot. 2016, 215–228. doi: 10.18869/modares.jcp.5.2.215
Kai, M. (2020). Diversity and distribution of volatile secondary metabolites throughout Bacillus subtilis Isolates. Front. Microbiol. 11. doi: 10.3389/fmicb.2020.00559
Kanyange, L., Kamau, J., Ombori, O., Ndayiragije, A., Muthini, M. (2019). Genotyping for blast (Pyricularia oryzae) resistance genes in F2 population of supa aromatic rice (Oryza sativa L.). Int. J. Genomics 2019, 5246820. doi: 10.1155/2019/5246820
Kavatsurwa, S. M., Kiremire, B., Wasswa, J., Mpiana, P. T. (2014). Dithiocarbamates residues level in selected vegetables from Bukavu, Democratic Republic of Congo. J. Phys. Chem. Sci. 1, 1–7. Available at: https://scienceq.org/dithiocarbamates-residues-level-in-selected-vegetables-from-bukavu-democratic-republic-of-congo.php
Kharshandi, F., Kayang, H. (2023). Antagonistic potential of rhizobacterial isolates against fungal pathogens causing rhizome rot in turmeric. Arch. Microbiol. 205, 221. doi: 10.1007/s00203-023-03565-1
Kijana, R., Abang, M., Edema, R., Mukankusi, C., Buruchara, R. (2017). Prevalence of angular leaf spot disease and sources of resistance in common bean in eastern Democratic Republic of Congo. Afr. Crop Sci. J. 25, 109–122. doi: 10.4314/acsj.v25i1.8
Korangi Alleluya, V., Argüelles Arias, A., Ribeiro, B., De Coninck, B., Helmus, C., Delaplace, P., et al. (2023). Bacillus lipopeptide-mediated biocontrol of peanut stem rot caused by Athelia rolfsii. Front. Plant Sci. 14. doi: 10.3389/fpls.2023.1069971
Korangi Alleluya, V., Kubindana, G., Fingu-mabola, J. C., Sulu, A., Kasereka, G., Matamba, A., et al. (2021). Utilisation des biopesticides pour une agriculture durable en République Démocratique du Congo (Synthèse bibliographique). Rev. Africaine d’Environnement d’Agriculture 2, 53–67. Available at: https://hdl.handle.net/2268/264393
Kulimushi, P. Z., Chuma Basime, G., Mushagalusa Nachigera, G., Thonart, P., Ongena, M. (2018). Efficacy of Bacillus amyloliquefaciens as biocontrol agent to fight fungal diseases of maize under tropical climates: from lab to field assays in south Kivu. Environ. Sci. pollut. Res. 25, 29808–29821. doi: 10.1007/s11356-017-9314-9
Kumar, V., Ahluwalia, V., Saran, S., Kumar, J., Patel, A. K., Singhania, R. R. (2021). Recent developments on solid-state fermentation for production of microbial secondary metabolites: Challenges and solutions. Bioresource Technol. 323, 124566. doi: 10.1016/j.biortech.2020.124566
Lahlali, R., Ezrari, S., Radouane, N., Kenfaoui, J., Esmaeel, Q., El Hamss, H., et al. (2022). Biological control of plant pathogens: A Global Perspective. Microorganisms 10, 596. doi: 10.3390/microorganisms10030596
Lam, V. B., Meyer, T., Arias, A. A., Ongena, M., Oni, F. E., Höfte, M. (2021). Bacillus cyclic lipopeptides iturin and fengycin control rice blast caused by Pyricularia oryzae in potting and acid sulfate soils by direct antagonism and induced systemic resistance. Microorganisms 9, 1441. doi: 10.3390/microorganisms9071441
Langa-Lomba, N., González-García, V., Venturini-Crespo, M. E., Casanova-Gascón, J., Barriuso-Vargas, J. J., Martín-Ramos, P. (2023). Comparison of the efficacy of Trichoderma and Bacillus strains and commercial biocontrol products against grapevine Botryosphaeria dieback pathogens. Agronomy 13, 533. doi: 10.3390/agronomy13020533
Le, K. D., Kim, J., Yu, N. H., Kim, B., Lee, C. W., Kim, J. C. (2020). Biological control of tomato bacterial wilt, Kimchi cabbage soft rot, and red pepper bacterial leaf spot Using Paenibacillus elgii JCK-5075. Front. Plant Sci. 11. doi: 10.3389/fpls.2020.00775
Legg, J. P., Owor, B., Sseruwagi, P., Ndunguru, J. (2006). Cassava mosaic virus disease in east and central Africa: epidemiology and management of a regional pandemic. Adv. Virus Res. 67, 355–418. doi: 10.1016/S0065-3527(06)67010-3
Lewis, K. A., Tzilivakis, J., Warner, D. J., Green, A. (2016). An international database for pesticide risk assessments and management. Hum. Ecol. Risk Assess. 22, 1050–1064. doi: 10.1080/10807039.2015.1133242
Liboga, O. B., Litucha, B. J., Ngama, B. F., Balimo, I. F., Kayawa, L. J. (2020). Comportement de cinq variétés de riz pluvial (Oryza sativa L.) à la pyriculariose et la verse dans les conditions naturelles à Kisangani, République Démocratique du Congo. J. Appl. Biosci. 145, 14965–11497. doi: 10.35759/JABs.v145.11
Liu, N., Xu, S., Yao, X., Zhang, G., Mao, W., Hu, Q., et al. (2016). Studies on the control of Ascochyta blight in field peas (Pisum sativum L.) caused by Ascochyta pinodes in Zhejiang province, China. Front. Microbiol. 7. doi: 10.3389/fmicb.2016.00481
Lokuruka, N. I. M. (2020). “Food and nutrition security in east Africa (Rwanda, Burundi and South Sudan): status, challenges and prospects,” in Food security in Africa. Ed. Mahmoud, B. (London: IntechOpen), 75–92. Available at: https://www.intechopen.com/books/food-security-in-africa/food-and-nutrition-security-in-east-africa-Rwanda-Burundi-and-south-Sudan-status-challenges-and-pros.
Lukanda, M., Owati, A., Ogunsanya, P., Valimunzigha, K., Katsongo, K., Ndemere, H., et al. (2014). First report of maize chlorotic mottle virus infecting maize in the Democratic Republic of the Congo. Plant Dis. 98, 1448. doi: 10.1094/PDIS-05-14-0484-PDN
Luna-Bulbarela, A., Tinoco-Valencia, R., Corzo, G., Kazuma, K., Konno, K., Galindo, E., et al. (2018). Effects of bacillomycin D homologues produced by Bacillus amyloliquefaciens 83 on growth and viability of Colletotrichum gloeosporioides at different physiological stages. Biol. Control 127, 145–154. doi: 10.1016/j.biocontrol.2018.08.004
Ma, X.-H., Shen, S., Li, W., Wang, J. (2023). Bioherbicidal potential of Bacillus altitudinis D30202 on Avena fatua L.: a whole-genome sequencing analysis. J. Appl. Genet. 64, 809–817. doi: 10.1007/s13353-023-00788-2
Mahapatra, S., Chakraborty, S., Samanta, M., Das, S., Islam, T., Mahapatra, S., et al. (2022). “Current understanding and future directions of biocontrol of plant diseases by Bacillus spp., with special reference to induced systemic resistance,” in Bacilli in agrobiotechnology. Bacilli in climate resilient agriculture and bioprospecting. Eds. Islam, M. T., Rahman, M., Pandey, P. (Cham, Bavaria: Springer International Publishing), 127–150. doi: 10.1007/978-3-030-85465-2_6
Mahmood, I., Imadi, S. R., Shazadi, K., Gul, A., Hakeem, K. R. (2016). “Effects of pesticides on environment,” in Plant, soil and microbes. Eds. Hakeem, R. K., Akhtar, S. M., Abdullah, A. N. S. (Cham, Bavaria: Springer International publishing), 253–269. doi: 10.1007/978-3-319-27455-3_13
Mahuku, G., Lockhart, B. E., Wanjala, B., Jones, M. W., Kimunye, J. N., Stewart, L. R., et al. (2015). Maize lethal necrosis (MLN), an emerging threat to maize-based food security in sub-saharan Africa. Phytopathology 105, 956–965. doi: 10.1094/PHYTO-12-14-0367-FI
Maloney, E. M., Sykes, H., Morrissey, C., Peru, K. M., Headley, J. V., Liber, K. (2020). Environmental toxicology comparing the acute toxicity of imidacloprid with alternative systemic insecticides in the aquatic insect Chironomus dilutus. Environ. Toxicol. Chem. 39, 587–594. doi: 10.1002/etc.4639
Marcano, I. E., Díaz-Alcántara, C. A., Seco, V., Urbano, B., González-Andrés, F. (2016). “Induced systemic resistance could explain the reduction in the incidence of black sigatoka (Mycosphaerella Fijiensis) in banana plants inoculated with bacteria isolated from banana tree roots in the Dominican Republic,” in Biological nitrogen fixation and beneficial plant-microbe interaction. Eds. González-Andrés, F., James, E. (Cham, Bavaria: Springer International Publishing), 155–170. doi: 10.1007/978-3-319-32528-6_14
Marrone, P. G. (2023). Mini-review status of the biopesticide market and prospects for new bioherbicides. Pest. Manage. Sci 80, 81–86. doi: 10.1002/ps.7403
Martins, S. J., Faria, A. F., Pedroso, M. P., Cunha, M. G., Rocha, M. R., Medeiros, F. H. V. (2019). Microbial volatiles organic compounds control anthracnose (Colletotrichum lindemuthianum) in common bean (Phaseolus vulgaris L.). Biol. Control 131, 36–42. doi: 10.1016/j.biocontrol.2019.01.003
Maruthi, M. N., Jeremiah, S. C., Mohammed, I. U., Legg, J. P. (2017). The role of the whitefly, Bemisia tabaci (Gennadius), and farmer practices in the spread of cassava brown streak ipomoviruses. J. Phytopathol. 165, 707–717. doi: 10.1111/JPH.12609
Mazumdar, D., Saha, S. P., Ghosh, S. (2020). Isolation, screening and application of a potent PGPR for enhancing growth of chickpea as affected by nitrogen level. Int. J. Vegetable Sci. 26, 333–350. doi: 10.1080/19315260.2019.1632401
Miao, S., Liang, J., Xu, Y., Yu, G., Shao, M. (2023). Bacillaene, sharp objects consist in the arsenal of antibiotics produced by Bacillus. Cell. Physiol. 2023, 1–15. doi: 10.1002/jcp.30974
Miljaković, D., Marinković, J., Balešević-Tubić, S. (2020). The Significance of Bacillus spp. in disease suppression and growth promotion of field and vegetable crops. Microorganisms 8, 1037. doi: 10.3390/microorganisms8071037
MINAGRI-Burundi (2018) Plan de Gestion des Pestes (PGP). Available at: https://bi.chm-cbd.net/sites/bi/files/2020-09/plan-gest-pestes-bi.pdf.
MINAGRI-RDC (2018) Sécurité alimentaire, niveau de production agricole et Animale, évaluation de la campagne agricole 2017-2018 et Bilan Alimentaire de la RD Congo. Available at: https://reliefweb.int/report/democratic-republic-congo/s-curit-alimentaire-niveau-de-production-agricole-et-animale.
Minengu, J. D. D., Mwengi, I., Maleke, M. (2018). Agriculture familiale dans les zones péri-urbaines de Kinshasa : analyse, enjeux et perspectives (synthèse bibliographique). Rev. Africaine d’Environnement d’Agriculture 1, 60–69. Available at: https://rafea-congo.com/admin/pdfFile/RAFEA-Article-Minengu-et-al-2018-ok.pdf
Mnif, I., Ghribi, D. (2015). Potential of bacterial derived biopesticides in pest management. Crop Protection 77, 52–64. doi: 10.1016/j.cropro.2015.07.017
Mirsam, H., Hary Kalqutny, S., Aqil, M., Azrai, M., Pakki, S., Muis, A., et al. (2021). Indigenous fungi from corn as a potential plant growth promoter and its role in Fusarium verticillioides suppression on corn. Heliyon 7, e07926. doi: 10.1016/j.heliyon.2021.e07926
Mordor intelligence (2023) Africa biopesticides market-size, share, COVID-19 impact and forecasts up to 2029. Available at: https://www.mordorintelligence.com/industry-reports/africa-biopesticides-market (Accessed December 02, 2023).
Mu, F., Chen, X., Fu, Z., Wang, X., Guo, J., Zhao, X., et al. (2023). Genome and transcriptome Analysis to elucidate the biocontrol mechanism of Bacillus amyloliquefaciens XJ5 against Alternaria solani. Microorganisms 11, 2055. doi: 10.3390/microorganisms11082055
Muhindo, H., Yasenge, S., Casinga, C., Songbo, M., Dhed’a, B., Alicai, T., et al. (2020). Incidence, severity and distribution of Cassava brown streak disease in northeastern Democratic Republic of Congo. Cogent Food Agric. 6, 1789422. doi: 10.1080/23311932.2020.1789422
Mukwa, L. F. T., Muengula, M., Zinga, I., Kalonji, A., Iskra-Caruana, M. L., Bragard, C. (2014). Occurrence and distribution of banana bunchy top virus related agro-ecosystem in South Western, Democratic Republic of Congo. Am. J. Plant Sci. 5, 647–658. doi: 10.4236/ajps.2014.55079
Mukwa, L. F. T., Mukendi, J., Adakate, F. G., Bugeme, D. M., Kalonji-Mbuyi, A., Ghimire, S. (2020). First report of the South American tomato pinworm Tuta absoluta (Meyrick) (Lepidoptera: Gelechiidae) and its damage in the Democratic Republic of Congo. BioInvasions Records 10, 33–44. doi: 10.1007/s12600-020-00841-4
Muleta, D., Assefa, F., Granhall, U. (2007). In vitro antagonism of rhizobacteria isolated from Coffea arabica L. against emerging fungal coffee pathogens. Eng. Life Sci. 7, 577–586. doi: 10.1002/elsc.200700004
Muliele, T. M., Manzenza, C. M., Ekuke, L. W., Diaka, C. P., Ndikubwayo, D. M., Kapalay, O. M., et al. (2018). Utilisation et gestion des pesticides en cultures maraîchères : cas de la zone de Nkolo dans la province du Kongo Central, République Démocratique du Congo. J. Appl. Biosci. 119, 11954. doi: 10.4314/jab.v119i1.11
Munganyinka, E., Ateka, E. M., Kihurani, A. W., Kanyange, M. C., Tairo, F., Sseruwagi, P., et al. (2018). Cassava brown streak disease in Rwanda, the associated viruses and disease phenotypes. Plant Pathol. 67, 377–387. doi: 10.1111/ppa.12789
Munyuli, T., Cihire, K., Rubabura, D., Mitima, K., Kalimba, Y., Tchombe, N., et al. (2017). Farmers’ perceptions, believes, knowledge and management practices of potato pests in South-Kivu Province, eastern of Democratic Republic of Congo. Open Agric. 2, 362–385. doi: 10.1515/opag-2017-0040
Nabahungu, N. L., Visser, S. M. (2013). Farmers’ knowledge and perception of agricultural wetland management in Rwanda. Land Degradation Dev. 24, 363–374. doi: 10.1002/ldr.1133
Ndayambaje, B., Amuguni, H., Coffin-Schmitt, J., Sibo, N., Ntawubizi, M., VanWormer, E. (2019). Pesticide application practices and knowledge among small-scale local rice growers and communities in Rwanda: A cross-sectional study. Int. J. Environ. Res. Public Health 16, 4770. doi: 10.3390/ijerph16234770
Ndayihanzamaso, P., Mostert, D., Matthews, M. C., Mahuku, G., Jomanga, K., Mpanda, H. J., et al. (2020). Evaluation of mchare and matooke bananas for resistance to Fusarium oxysporum f. Sp. cubense race 1. Plants 9, 1–15. doi: 10.3390/plants9091082
Ndayihanzamaso, P., Niko, N., Niyongere, C., Bizimana, S., Nibasumba, A., Lepoint, P., et al. (2016). Distribution, incidence and farmers knowledge of banana Xanthomonas wilt in Burundi. Afr. J. Agric. Res. 11, 3615–3621. doi: 10.5897/AJAR2016.11210
Ndisanze, A. M., Kamana, E., Nirere, C., Ilkay, K. (2022). Assessment of the pesticides utilization and the pesticide residues presence in fresh and tomato products for the tomato supply chain in Rwanda. Food Nutr. Sci. 13, 963–972. doi: 10.4236/fns.2022.1312067
Ndungo, V., Fiaboe, K. K. M., Mwangi, M. (2008). Banana Xanthomonas wilt in the DR Congo : Impact, spread and management. J. Appl. Biosci. 1, 1–7. Available at: https://hdl.handle.net/10568/90751
Neuenschwander, P. (2001). Biological control of the cassava mealybug in Africa: A review. Biol. Control 21, 214–229. doi: 10.1006/bcon.2001.0937
Ngalimat, M. S., Yahaya, R. S. R., Baharudin, M. M. A., Yaminudin, S.M., Karim, M., Ahmad, S. A., et al. (2021). A Review on the biotechnological applications of the operational group Bacillus amyloliquefaciens. Microorganisms 9, 614. doi: 10.3390/microorganisms9030614
Ngweme, G. N., Al Salah, D. M. M., Laffite, A., Sivalingam, P., Grandjean, D., Konde, J. N., et al. (2021). Occurrence of organic micropollutants and human health risk assessment based on consumption of Amaranthus viridis, Kinshasa in the Democratic Republic of the Congo. Sci. Total Environ. 754, 142175. doi: 10.1016/j.scitotenv.2020.142175
Ngweme, G. N., Mbela, G. K., Sikulisimwa, C. P., Kyela, C. M., Komanda, J. A. (2019). Analyse des connaissances, attitudes et pratiques des maraîchers de la ville de Kinshasa en rapport avec l’utilisation des pesticides et l’impact sur la santé humaine et sur l’environnement. Afrique Sci. 15, 122–133. Available at: https://www.afriquescience.net/PDF/15/4/11.pdf
Niassy, S., Agbodzavu, M. K., Kimathi, E., Mutune, B., Abdel-Rahman, E. F. M., Salifu, D., et al. (2021). Bioecology of fall armyworm Spodoptera frugiperda (J. E. Smith), its management and potential patterns of seasonal spread in Africa. PloS One 16, e0249042. doi: 10.1371/journal.pone.0249042
Nihorimbere, V., Ongena, M., Cawoy, H., Brostaux, Y., Kakana, P., Jourdan, E., et al. (2010). Beneficial effects of Bacillus subtilis on field-grown tomato in Burundi: Reduction of local Fusarium disease and growth promotion. Afr. J. Microbiol. Res. 4, 1135–1142. Available at: https://hdl.handle.net/2268/92891
Nikolaidis, M., Hesketh, A., Mossialos, D., Iliopoulos, I., Oliver, S. G., Amoutzias, G. D. (2022). A Comparative analysis of the core proteomes within and among the Bacillus subtilis and Bacillus cereus evolutionary groups reveals the patterns of lineage- and species-specific adaptations. Microorganisms 10, 1720. doi: 10.3390/microorganisms10091720
Niyibizi, G. P., Gakuru, S. J. B., Rizinde, H. J. C., Munenwa, S.A., Lwanzo, L. (2019). État de lieux des caféières face à la menace d’Antestiopsis orbitalis dans le territoire de Kalehe, à l’Est de la RD Congo (Annales de l’UNIGOM), Goma 9, 87–99. Available at: https://pugoma.com/index.php/UNIGOM/article/view/145.
Niyongere, C., Losenge, T., Ateka, E. M., Ntukamazina, N., Ndayiragije, P., Simbare, A., et al. (2013). Understanding banana bunchy top disease epidemiology in Burundi for an enhanced and integrated management approach. Plant Pathol. 62, 562–570. doi: 10.1111/J.1365-3059.2012.02676.X
Niyongere, C., Mbonihankuye, C., Mutshail, G., Yamuremye, A. (2015). Utilization of pesticides in smallholder horticulture production pinpoint the need for cropping system changes in Burundi. Acta Hortic. 1105, 213–220. doi: 10.17660/ActaHortic.2015.1105.30
Nkuba, J., Tinzaara, W., Night, G., Niko, N., Jogo, W., Mukandala, L., et al. (2015). Adverse impact of banana Xanthomonas Wilt on farmers’ livelihoods in Eastern and Central Africa. Afr. J. Plant Sci. 9, 279–286. doi: 10.5897/AJPS2015.1292
Nkurunziza, G., Ndayisenga, M., Ndayihanzamaso, P., Ndayiragije, P., Niyongabo, D. (2012). Techniques de culture, de protection et de conservation du maïs – Cas des variétés à pollinisation libre (Manuel. ISABU). Bujumbura. Available at: https://isabu.bi/wp-content/uploads/2021/09/Manuel-de-formation_-Mais.pdf.
Nyabyenda, P. (2005). Les Plantes cultivées en régions tropicales d A’ltitude d’Afrique: Généralités, légumineuses alimentaires, Plantes à tubercules et racines, Céréales (Gembloux: Presses Agronomiques de Gembloux).
Nyabyenda, P. (2006). Les plantes cultivées en régions tropicales d’altitude d’Afrique : Cultures industrielles et d’exportation, cultures fruitières, cultures maraîchères (Gembloux: Presses Agronomiques de Gembloux).
Okonya, J., Ocimati, W., Nduwayezu, A., Kantungeko, D., Niko, N., Blomme, G., et al. (2019a). Farmer reported pest and disease impacts on root, tuber, and banana crops and livelihoods in Rwanda and Burundi. Sustainability 11, 1592. doi: 10.3390/su11061592
Okonya, J., Petsakos, A., Suarez, V., Nduwayezu, A., Kantungeko, D., Blomme, G., et al. (2019b). Pesticide use practices in root, tuber, and banana crops by smallholder farmers in Rwanda and Burundi. Int. J. Environ. Res. Public Health 16, 400. doi: 10.3390/ijerph16030400
Oni, F. E., Esmaeel, Q., Onyeka, J. T., Adeleke, R., Jacquard, C., Clement, C., et al. (2022). Pseudomonas lipopeptide-mediated biocontrol: Chemotaxonomy and biological activity. Molecules 27, 372. doi: 10.3390/molecules27020372
Padgham, J. L., Sikora, R. A. (2007). Biological control potential and modes of action of Bacillus megaterium against Meloidogyne graminicola on rice. Crop Prot. 26, 971–977. doi: 10.1016/j.cropro.2006.09.004
Paka, G. V., Mobambo, P. K., Omondi, B. A., Staver, C. (2021). Evaluation de l’efficacité de la macro-propagation des cultivars de bananiers les plus préférés au Kongo Central, en RD Congo. Afrique Sci. 19, 76–88. Available at: https://hdl.handle.net/10568/118143
Pandit, M. A., Kumar, J., Gulati, S., Bhandari, N., Mehta, P., Katyal, R., et al. (2022). Major biological control strategies for plant pathogens. Pathogens 11, 273. doi: 10.3390/pathogens11020273
PES (2021) Rapport National sur la situation des Pesticides Hautement Dangereux (PHD) au Burundi. Available at: https://ipen.org/sites/default/files/documents/rapport_ped_au_burundi-fr.pdf.
Pleban, S., Ingel, F., Chet, I. (1995). Control of Rhizoctonia solani and Sclerotium rolfsii in the greenhouse using endophytic Bacillus spp. Eur. J. Plant Pathol. 101, 665–672. doi: 10.1007/BF01874870
PND (2018) Plan National de Développement du Burundi 2018-2027. Available at: https://www.presidence.gov.bi/wp-content/uploads/2018/08/PND-Burundi-2018-2027-Version-Finale.pdf.
Prasad, B., Sharma, D., Kumar, P., Chandra Dubey, R. (2023). Biocontrol potential of Bacillus spp. for resilient and sustainable agricultural systems. Physiol. Mol. Plant Pathol. 128, 102173. doi: 10.1016/j.pmpp.2023.102173
Pršić, J., Ongena, M. (2020). Elicitors of plant immunity triggered by beneficial bacteria. Front. Plant Sci. 11. doi: 10.3389/fpls.2020.594530
Puan, S. L., Erriah, P., Baharudin, M. M. A., Yahaya, N. M., Kamil, W. N. I. W. A., Ali, M. S. M., et al. (2023). Antimicrobial peptides from Bacillus spp. and strategies to enhance their yield. Appl. Microbiol. Biotechnol. 107, 5569–5593. doi: 10.1007/s00253-023-12651-9
Qiao, J., Zhang, R., Liu, Y., Liu, Y. (2023). Evaluation of the biocontrol efficiency of Bacillus subtilis wettable powder on pepper root rot caused by Fusarium solani. Pathogens 12, 225. doi: 10.3390/pathogens12020225
Radhakrishnan, R., Hashem, A., Abd Allah, E. F. (2017). Bacillus: A biological tool for crop improvement through bio-molecular changes in adverse environments. Front. Physiol. 8. doi: 10.3389/fphys.2017.00667
Ramalakshmi, V., Dash, L., Padhy, D., Kumar, K. A. (2020). “Pest control by macroorganisms,” in Recent trends in insect pest management. Eds. Raju, S. V. S., Sharma, K. R. (New Delhi: AkiNik Publications), 135–156.
Ramyabharathi, S., Raguchander, T. (2014). Efficacy of secondary metabolites produced by Bacillus subtilis EPCO16 against tomato wilt pathogen Fusarium oxysporum f.sp. lycopersici. J. Mycol. Plant Pathol. 44, 148–153.
Raut, S. P., Ranade, S. (2004). “Diseases of banana and their management,” in Diseases of fruits and vegetables. Ed. Naqvi, S. A. M. H. (Dordrecht: Kluwer Academic Publishers), 37–52.
Raveau, R., Fontaine, J., Lounès, A., Sahraoui, H. (2020). Essential oils as potential alternative biocontrol products against plant pathogens and weeds: A review. Foods 9, 365. doi: 10.3390/foods9030365
Redinbaugh, M. G., Stewart, L. R. (2018). Maize lethal necrosis: An emerging, synergistic viral disease. Annu. Rev. Virol. 5, 301–322. doi: 10.1146/annurev-virology-092917-043413
REMA (2011) The national integrated pest Management (IPM) framework for Rwanda. Available at: https://www.rema.gov.rw/rema_doc/LVEMP/IPM_Latest%20Version-2.pdf.
Reyes-Estebanez, M., Sanmartin, P., Camacho-Chab, J. C., Susana, C., Chan-Bacab, M. J., Águila-Ramírez, R. N., et al. (2020). Characterization of a native Bacillus velezensis-like strain for the potential biocontrol of tropical fruit pathogens. Biol. Control 141, 104127. doi: 10.1016/j.biocontrol.2019.104127
Rietveld, A. M., Dusingizimana, P., Blomme, G., Gaidashova, S. V., Ocimati, W., Ntamwira, J. (2020) A superior technology to control Banana Xanthomonas Wilt (BXW) in Rwanda. RTB Research Brief 03. Available at: https://hdl.handle.net/10568/110019.
Rodenburg, J., Demont, M., Zwart, S. J., Bastiaans, L. (2016). Parasitic weed incidence and related economic losses in rice in Africa. Agriculture Ecosyst. Environ. 235, 306–317. doi: 10.1016/j.agee.2016.10.020
Rodríguez, M., Torres, M., Blanco, L., Béjar, V., Sampedro, I., Llamas, I. (2020). Plant growth-promoting activity and quorum quenching-mediated biocontrol of bacterial phytopathogens by Pseudomonas segetis strain P6. Sci. Rep. 10, 4121. doi: 10.1038/s41598-020-61084-1
Romero-Severson, J., Moran, T. E., Shrader, D. G., Fields, F. R., Pandey-Joshi, S., Thomas, C. L., et al. (2021). A seed-endophytic Bacillus safensis strain with antimicrobial activity has genes for novel bacteriocin-like antimicrobial peptides. Front. Microbiol. 12. doi: 10.3389/fmicb.2021.734216
Roser, M. (2023) Employment in agriculture. Available at: https://ourworldindata.org/employment-in-agriculture (Accessed September 20, 2023).
Runo, S., Kuria, E. K. (2018). Habits of a highly successful cereal killer, Striga. PloS Pathog. 14, e1006731. doi: 10.1371/journal.ppat.1006731
Rutikanga, A. (2015). Pesticides use and regulations in Rwanda status and potential for promotion of biological control methods (Aberdeenshire, (Scotland: University of Aberdeen).
Saeed, Q., Xiukang, W., Haider, F. U., Kučerik, J., Mumtaz, M. Z., Holatko, J., et al. (2021). Rhizosphere bacteria in plant growth promotion, biocontrol, and bioremediation of contaminated sites: A comprehensive review of effects and mechanisms. Int. J. Mol. Sci. 22, 10529. doi: 10.3390/ijms221910529
Seth, K., Vyas, P., Deora, S., Gupta, A. K., Meena, M., Swapnil, P., et al. (2023). “Understanding plant-plant growth-promoting rhizobacteria (PGPR) interactions for inducing plant defense,” in Plant-microbe interaction - recent advances in molecular and biochemical approaches. Eds. Swapnil, P., Meena, M., Harish, Marwal, A., Vijayalakshmi, S., Zehra, A. (London, UK: Academic Press, Elsevier), 201–226. doi: 10.1016/B978-0-323-91876-3.00010-5
Sharma, K., Kreuze, J., Abdurahman, A., Parker, M., Nduwayezu, A., Rukundo, P. (2020). Molecular diversity and pathogenicity of Ralstonia solanacearum species complex associated with bacterial wilt of potato in Rwanda. Plant Dis. 105, 770–779. doi: 10.1094/PDIS-04-20-0851-RE
Sheffield, F. M. L. (1957). Virus diseases of sweet potato in East Africa. I. Identification of the viruses and their insect vectors. Phytopathology 47, 582–590. Available at: https://worldveg.tind.io/record/7663?ln=en
Shen, N., Li, S., Li, S. Y., Zhang, H., Jiang, M. (2022). The siderophore-producing bacterium, Bacillus siamensis Gxun-6, has an antifungal activity against Fusarium oxysporum and promotes the growth of banana. Egypt. J. Biol. Pest Control 32, 34. doi: 10.1186/s41938-022-00533-7
Shifa, H., Gopalakrishnan, C., Velazhahan, R. (2018). Management of late leaf spot (Phaeoisariopsis personata) and root rot (Macrophomina phaseolina) diseases of groundnut (Arachis hypogaea L.) with plant growth-promoting rhizobacteria, systemic acquired resistance inducers and plant extracts. Phytoparasitica 46, 19–30. doi: 10.1007/s12600-018-0644-z
Sikdar, R., Elias, M. (2020). Quorum quenching enzymes and their effects on virulence, biofilm, and microbiomes: a review of recent advances. Expert Rev. Anti-infective Ther. 18, 1221–1233. doi: 10.1080/14787210.2020.1794815
Singh, P., Singh, R. K., Zhou, Y., Wang, J., Jiang, Y., Shen, N., et al. (2022). Unlocking the strength of plant growth promoting Pseudomonas in improving crop productivity in normal and challenging environments: a review. J. Plant Interact. 17, 220–238. doi: 10.1080/17429145.2022.2029963
Stenberg, J. A. (2017). A conceptual framework for integrated pest management. Trends Plant Sci. 22, 759–769. doi: 10.1016/j.tplants.2017.06.010
Sumi, C. D., Yang, B. W., Yeo, I. C., Hahm, Y. T. (2015). Antimicrobial peptides of the genus Bacillus: A new era for antibiotics. Can. J. Microbiol. 61, 93–103. doi: 10.1139/cjm-2014-0613
Tchatchambe, J. N. B., Losimba, J. K., Kirongozi, F. B., Adheka, J. G., Onautshu, D. O. (2019). Macro-propagation and micro-propagation of BBTV-free plants in Kisangani, DR Congo. Scholars Bull. 9771, 178–183. doi: 10.21276/sb.2019.5.5.1
Thanh, K., Yen, T. T. (2023). Isolation and selection of antagonistic bacteria against Cercospora arachidicola causing brown spot on peanut. HAYATI J. Biosci. 30, 927–936. doi: 10.4308/hjb.30.5.927-936
Théatre, A., Hoste, A. C. R., Rigolet, A., Benneceur, I., Bechet, M., Ongena, M., et al. (2022). “Bacillus sp.: A remarkable source of bioactive lipopeptides,” in Biosurfactants for the biobased economy. Eds. Hausmann, R., Henkel, M. (Cham, Bavaria: Springer International Publishing), 123–180.
Thresh, J. M., Cooter, R. J. (2005). Strategies for controlling cassava mosaic virus disease in Africa. Plant Pathol. 54, 587–614. doi: 10.1111/J.1365-3059.2005.01282.X
Tinivella, F., Hirata, L. M., Celan, M. A., Wright, S. A. I., Amein, T., Schmitt, A., et al. (2009). Control of seed-borne pathogens on legumes by microbial and other alternative seed treatments. Eur. J. Plant Pathol. 123, 139–151. doi: 10.1007/s10658-008-9349-3
Ullah Kakar, K., Duan, Y., Nawaz, Z., Sun, G., Almoneafy, A. A., Auwal Hassan, M., et al. (2014). A novel rhizobacterium Bk7 for biological control of brown sheath rot of rice caused by Pseudomonas fuscovaginae and its mode of action. Eur. J. Plant Pathol. 138, 819–834. doi: 10.1007/s10658-013-0356-7
Uwamahoro, F., Berlin, A., Bucagu, C., Bylund, H., Yuen, J. (2020). Ralstonia solanacearum causing potato bacterial wilt: host range and cultivars’ susceptibility in Rwanda. Plant Pathol. 69, 559–568. doi: 10.1111/ppa.13140
Vejan, P., Abdullah, R., Khadiran, T., Ismail, S., Nasrulhaq Boyce, A. (2016). Role of plant growth promoting rhizobacteria in agricultural sustainability-A review. Molecules 21, 573. doi: 10.3390/molecules21050573
Wang, X., Du, Z., Chen, C., Guo, S., Mao, Q., Wu, W., et al. (2023). Antifungal effects and biocontrol potential of lipopeptide-producing Streptomyces against banana Fusarium wilt fungus Fusarium oxysporum f. sp. cubense. Front. Microbiol. 14. doi: 10.3389/fmicb.2023.1177393
Wang, H., Liu, R., You, M. P., Barbetti, M. J., Chen, Y. (2021). Pathogen biocontrol using plant growth-promoting bacteria (PGPR): role of bacterial diversity. Microorganisms 9, 1988. doi: 10.3390/microorganisms9091988
Wang, B., Yuan, J., Zhang, J., Shen, Z., Zhang, M., Li, R., et al. (2013). Effects of novel bioorganic fertilizer produced by Bacillus amyloliquefaciens W19 on antagonism of Fusarium wilt of banana. Biol. Fertil. Soils 49, 435–446. doi: 10.1007/s00374-012-0739-5
WFP (2021) Burundi annual country report. Available at: https://docs.wfp.org/api/documents/WFP-0000137887/download/ (Accessed October 01, 2021).
Wipfler, L., ter Horst, M. (2018) Pesticide management in Rwanda analysis of the current pest control products administration and management system. Wageningen Environmental Research report 2904. Available at: https://edepot.wur.nl/457874.
Xiang, N., Lawrence, K. S., Kloepper, J. W., Donald, P. A., McInroy, J. A., Lawrence, G. W. (2017). Biological control of Meloidogyne incognita by spore-forming plant growth-promoting rhizobacteria on cotton. Plant Dis. 101, 774–784. doi: 10.1094/PDIS-09-16-1369-RE
Xiang, D., Yang, X., Liu, B., Chu, Y., Liu, S., Li, C. (2023). Bio-priming of banana tissue culture plantlets with endophytic Bacillus velezensis EB1 to improve Fusarium wilt resistance. Front. Microbiol. 14. doi: 10.3389/fmicb.2023.1146331
Xu, W., Yang, Q., Yang, F., Xie, X., Goodwin, P. H., Deng, X., et al. (2022). Evaluation and genome analysis of Bacillus subtilis YB-04 as a potential biocontrol agent against Fusarium wilt and growth promotion agent of cucumber. Front. Microbiol. 13. doi: 10.3389/fmicb.2022.885430
Xue, Y., Zhang, Y., Huang, K., Wang, X., Xing, M., Xu, Q., et al. (2023). A novel biocontrol agent Bacillus velezensis K01 for management of gray mold caused by Botrytis cinerea. AMB Expr. 13, 91. doi: 10.1186/s13568-023-01596-x
Yadav, R., Singh, S., Singh, A. N. (2022). Biopesticides: Current status and future prospects. Proc. Int. Acad. Ecol. Environ. 12, 211–233. Available at: http://www.iaees.org/publications/journals/piaees/articles/2022-12(3)/biopesticides-current-status-and-future-prospects.pdf
Ye, L., Wang, J. Y., Liu, X. F., Guan, Q., Dou, N. X., Li, J., et al. (2022). Nematicidal activity of volatile organic compounds produced by Bacillus altitudinis AMCC 1040 against Meloidogyne incognita. Arch. Microbiol. 204, 521. doi: 10.1007/s00203-022-03024-3
Yi, H. S., Ahn, Y. R., Song, G. C., Ghim, S. Y., Lee, S., Lee, G., et al. (2016). Impact of a bacterial volatile 2,3-butanediol on Bacillus subtilis rhizosphere robustness. Front. Microbiol. 7. doi: 10.3389/fmicb.2016.00993
Yin, Q. J., Ying, T. T., Zhou, Z. Y., Hu, G. A., Yang, C. L., Hua, Y., et al. (2023). Species-specificity of the secondary biosynthetic potential in Bacillus. Front. Microbiol. 14. doi: 10.3389/fmicb.2023.1271418
Yu, X., Ai, C., Xin, L., Zhou, G. (2011). The siderophore-producing bacterium, Bacillus subtilis CAS15, has a biocontrol effect on Fusarium wilt and promotes the growth of pepper. Eur. J. Soil Biol. 47, 138–145. doi: 10.1016/j.ejsobi.2010.11.001
Yu, Y., Gui, Y., Li, Z., Jiang, C., Guo, J., Niu, D. (2022). Induced systemic resistance for improving plant immunity by beneficial microbes. Plants 11, 386. doi: 10.3390/plants11030386
Yuan, J., Raza, W., Shen, Q., Huang, Q. (2012). Antifungal activity of Bacillus amyloliquefaciens NJN-6 volatile compounds against Fusarium oxysporum f. sp. cubense. Appl. Environ. Microbiol. 78, 5942–5944. doi: 10.1128/AEM.01357-12
Zhang, J., Huang, X., Hou, Y., Xia, X., Zhu, Z., Huang, A., et al. (2023a). Isolation and screening of antagonistic endophytes against Phytophthora infestans and preliminary exploration on anti-oomycete mechanism of Bacillus velezensis 6-5. Plants 12, 909. doi: 10.3390/plants12040909
Zhang, J., Huang, X., Yang, S., Huang, A., Ren, J., Luo, X., et al. (2023b). Endophytic Bacillus subtilis H17-16 effectively inhibits Phytophthora infestans, the pathogen of potato late blight, and its potential application. Pest Manage. Sci. 79, 5073–5086. doi: 10.1002/ps.7717
Zhang, N., Wang, Z., Shao, J., Xu, Z., Liu, Y., Xun, W., et al. (2023). Biocontrol mechanisms of Bacillus: Improving the efficiency of green agriculture. Microbial. Biotechnol. 16, 2250–2263. doi: 10.1111/1751-7915.14348
Zhao, P., Quan, C., Wang, Y., Wang, J., Fan, S. (2014). Bacillus amyloliquefaciens Q-426 as a potential biocontrol agent against Fusarium oxysporum f. sp. spinaciae. J. Basic Microbiol. 54 (5), 448–456. doi: 10.1002/jobm.201200414
Keywords: Burundi, Rwanda, DRC, diseases and pests, pesticide, IPM, Bacillus spp., biocontrol
Citation: Nihorimbere G, Korangi Alleluya V, Nimbeshaho F, Nihorimbere V, Legrève A and Ongena M (2024) Bacillus-based biocontrol beyond chemical control in central Africa: the challenge of turning myth into reality. Front. Plant Sci. 15:1349357. doi: 10.3389/fpls.2024.1349357
Received: 04 December 2023; Accepted: 08 January 2024;
Published: 06 February 2024.
Edited by:
Choong-Min Ryu, Korea Research Institute of Bioscience and Biotechnology (KRIBB), Republic of KoreaReviewed by:
Amelia Cristina Montoya Martinez, Instituto Tecnológico de Sonora (ITSON), MexicoBrent L. Nielsen, Brigham Young University, United States
Copyright © 2024 Nihorimbere, Korangi Alleluya, Nimbeshaho, Nihorimbere, Legrève and Ongena. This is an open-access article distributed under the terms of the Creative Commons Attribution License (CC BY). The use, distribution or reproduction in other forums is permitted, provided the original author(s) and the copyright owner(s) are credited and that the original publication in this journal is cited, in accordance with accepted academic practice. No use, distribution or reproduction is permitted which does not comply with these terms.
*Correspondence: Gaspard Nihorimbere, nihorimbereg@yahoo.fr; Virginie Korangi Alleluya, virginiekorangi@gmail.com; François Nimbeshaho, francois.nimbeshaho@gmail.com; Venant Nihorimbere, venant.nihorimbere@gmail.com; Anne Legrève, anne.legreve@uclouvain.be; Marc Ongena, marc.ongena@uliege.be
†These authors have contributed equally to this work