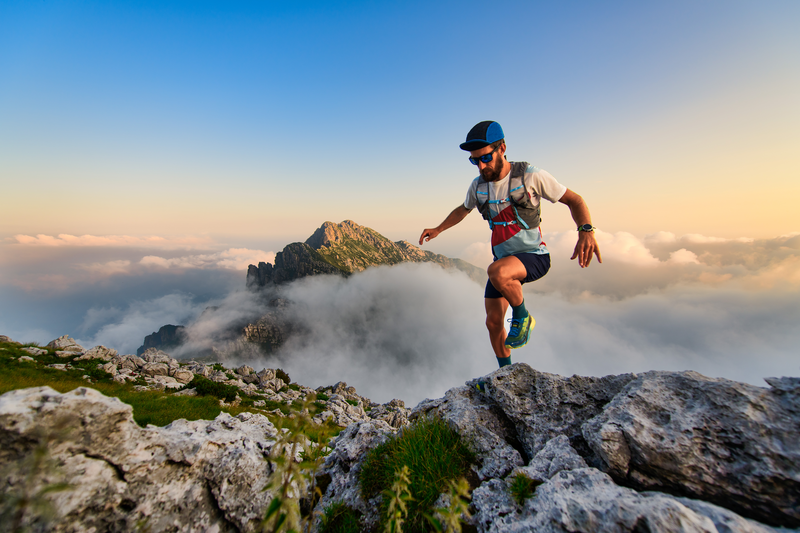
94% of researchers rate our articles as excellent or good
Learn more about the work of our research integrity team to safeguard the quality of each article we publish.
Find out more
ORIGINAL RESEARCH article
Front. Plant Sci. , 21 February 2024
Sec. Crop and Product Physiology
Volume 15 - 2024 | https://doi.org/10.3389/fpls.2024.1344647
This article is part of the Research Topic Enhancing Sustainable Crop Production: Biostimulants and Biotechnological Approaches in Challenging Climates View all 18 articles
Appropriate straw incorporation has ample agronomic and environmental benefits, but most studies are limited to straw mulching or application on the soil surface. To determine the effect of depth of straw incorporation on the crop yield, soil organic carbon (SOC), total nitrogen (TN) and greenhouse gas emission, a total of 4 treatments were set up in this study, which comprised no straw returning (CK), straw returning at 15 cm (S15), straw returning at 25 cm (S25) and straw returning at 40 cm (S40). The results showed that straw incorporation significantly increased SOC, TN and C:N ratio. Compared with CK treatments, substantial increases in the grain yield (by 4.17~5.49% for S15 and 6.64~10.06% for S25) were observed under S15 and S25 treatments. S15 and S25 could significantly improve the carbon and nitrogen status of the 0-40 cm soil layer, thereby increased maize yield. The results showed that the maize yield was closely related to the soil carbon and nitrogen index of the 0-40 cm soil layer. In order to further evaluate the environmental benefits of straw returning, this study measured the global warming potential (GWP) and greenhouse gas emission intensity (GHGI). Compared with CK treatments, the GWP of S15, S25 and S40 treatments was increased by 9.35~20.37%, 4.27~7.67% and 0.72~6.14%, respectively, among which the S15 treatment contributed the most to the GWP of farmland. GHGI is an evaluation index of low-carbon agriculture at this stage, which takes into account both crop yield and global warming potential. In this study, GHGI showed a different trend from GWP. Compared with CK treatments, the S25 treatments had no significant difference in 2020, and decreased significantly in 2021 and 2022. This is due to the combined effect of maize yield and cumulative greenhouse gas emissions, indicating that the appropriate straw returning method can not only reduce the intensity of greenhouse gas emissions but also improve soil productivity and enhance the carbon sequestration effect of farmland soil, which is an ideal soil improvement and fertilization measure.
In recent years, the impact of climate warming on natural economy and human life has become a global problem (Linquist et al., 2012). At present, it is generally believed that the increasing concentration of greenhouse gases (CO2, CH4, N2O) in the atmosphere was the main cause of climate warming. Among them, 10%-20% of the total anthropogenic greenhouse gas emissions had generated by agricultural activities (Smith et al., 2007). The emission of greenhouse gases from farmland comes from the direct emission of farmland soil and the indirect emission of agricultural management measures, such as tillage, irrigation, straw returning, fertilization, etc. (Baggs et al., 2003; Toma and Hatano, 2007; Saggar, 2010; Trost et al., 2013). Therefore, agricultural production is considered to be an important source of greenhouse gas emissions.
As a carrier of material, energy and nutrients, straw is a valuable renewable natural resources (Amaya et al., 2007). China is a large agricultural country, which had produced a huge amount of crop straw every year, more than 800 million tons (Xia et al., 2014; Liu et al., 2021; Zhong et al., 2022). The content of N, P, K and other nutrient elements in straw was rich. As an organic fertilizer resource, it can be equivalent to 40% of the amount of chemical fertilizer used in China (Zhuang et al., 2020). The traditional treatments of incineration will not only cause serious environmental pollution, but also a great waste of resources. In recent years, China ‘s farmland farming model has changed significantly. The crop straw is no longer used as fuel, and the common agricultural practice was returned the straw to the field, which not only improves soil fertility but also reduces air pollution caused by crop straw burning (Gao et al., 2011). Straw returning can make the carbon in the straw return to the soil to participate in the carbon cycle, which can not only reduced the carbon output of the farmland ecosystem but also increased the soil organic matter content and improve the soil fertility, so as to realize the reuse of agricultural resources (Mu et al., 2016; Liang et al., 2017; Adimassu et al., 2019; Smitha et al., 2019). Some studies have shown that straw returning can stimulate the microorganisms in the soil to produce a priming effect (Kuzyakov et al., 2000), increase microbial activity, accelerate the decomposition rate of soil organic matter, and thus affect the production and emission of soil greenhouse gases. However, the current research results on the increase or decrease of greenhouse gas emissions caused by straw returning are still uncertain.
Northeast China is the main grain producing area in China. In recent years, with the increase of population growth and the improvement of living standards, higher requirements have been put forward for food production, environmental friendliness and sustainable development. People have made fruitful explorations in many fields such as high-yield cultivation, breeding and biotechnology. However, with the increase of crop yield, the biomass of straw has also increased significantly (Tian et al., 2020). In the past many years, due to the long-term shallow tillage of small agricultural machinery and the predatory production mode of large-scale application of chemical fertilizers, the comprehensive production capacity of farmland soil in Northeast China has declined sharply (Tian et al., 2019; Sui et al., 2020). Although the crop straw is the main source of organic materials for soil fertilization, straw burning is the most common straw treatment method, which was not only a waste of resources, but also caused serious environmental pollution. Therefore, aiming at the straw problem existing in the production of spring maize in Northeast China. A total of 4 treatments were set up in this study, which were no straw returning (CK), straw returning at 15 cm (S15), straw returning at 25 cm (S25) and straw returning at 40 cm (S40). By analyzing the effects of straw returning on the maize yield, physical and chemical properties of farmland soil and CO2 and N2O emissions, global warming potential (GWP) and greenhouse gas emission intensity (GHGI) were measured, and the regulation effect of straw returning depth on rice production potential and greenhouse gas emission reduction in paddy field was comprehensively evaluated to determine the optimal straw returning depth. It is expected that the research results will be of great significance to the scientific and rational use of straw and greenhouse gas emission reduction.
The experiment was conducted in the Qiqihar maize experimental station of the Heilongjiang Academy of Agricultural Sciences, which is located in Qiqihar, Heilongjiang Province, China (46°52′N, 123°46′E) during the maize growing season (May to October) from 2020 to 2022. The test area belongs to the mid-temperate continental monsoon climate, which is characterized by dry and windy spring and warm and rainy summer. The annual precipitation is 477 mm, and a frost-free period of approximately 130 days. The soil type was Argosols (FAO classification) and the basic key properties are shown in Table 1.
The experiment began in May 2020 and ended in October 2022. The test crop was maize and the variety was Nendan 29. The experiment comprised of four treatments as follows: no straw incorporation (CK), straw incorporation at 15 cm soil depth (S15), straw incorporation at 25 cm (S25) and straw incorporation at 40 cm (S40). Straw returning rate was 8000 kg hm-1. The treatments were arranged into a randomized block design and replicated three times. Nitrogen rates were 180 kg ha-1, and nitrogen fertilizer was applied according to the different stages, with base fertilizer and top-dressing fertilizer following a proportion of 1:2. Phosphorus (P2O5) rates were 90 kg ha-1 and potassium (K2O) rates were 120 kg ha-1, and phosphoru and potassium were applied as base fertilizer at one time. N, P, and K fertilizers was used urea, Ca(H2PO4)2 and K2SO4, respectively. Other management measures were consistent with local agronomic practices including weeding and spraying insecticides throughout the experiment.
Yield samples of maize were collected randomly from 1 m double rows per plot at maturity. Grain yield was standardized to a moisture content of 0.14 g H2O g−1.
After maize harvest, soil samples were collected in three layers (0-20 cm, 20-40 cm, and 40-60 cm) using a soil drill with a diameter of 3 cm. Five points were randomly selected from each micro-area, and the soil of the same soil layer was uniformly mixed as a sample. The soil samples were placed in a cool and ventilated place, dried and ground through a 0.15 mm sieve to determine soil organic carbon (SOC) and soil total nitrogen (TN) content. The SOC was determined by potassium dichromate external heating method (Lu, 2000), and the TN was determined by Kjeldahl apparatus (Kjeltec8400, FOSS, Denmark). The SOC and TN stock was calculated using the equal weight method (Ellert and Bettany, 1995; Xue et al., 2015), to eliminate the bias in the calculation of SOC and TN stocks caused by different plough layer thickness due to tillage. The ratio of SOC to TN was defined as soil carbon-nitrogen (C:N) ratio (Blanco-Canqui and Lal, 2008).
The emission fluxes of soil greenhouse gases CO2 and N2O were measured by static chamber method. Sampling once every 7 days during the growth period and once every 2 days after fertilization. Each treatment was placed in three static observation boxes, which were placed between two rows of corn. The sampling time was from 9:00 to 10:00 in the morning. The gas was collected every 10 min for a total of 5 times, and 30 mL of gas was collected in the trachea each time. Immediately after the sample collection was completed, the sample was taken back to the laboratory and analyzed within 24 hours using a gas chromatograph equipped with an ECD (Electron Capture Detector) and a FID (Flame Ionization Detector) detector (Agilent 7890A, Shanghai, China).The formula of CO2 and N2O emission flux was as follows:
F is CO2 emission flux or N2O emission flux; ρ is the density of CO2 or N2O in the standard state; H is the height of the closed box (m); ΔC/Δt is the change rate of CO2 or N2O concentration in the test chamber; T is the average temperature (°C) in the chamber during the sampling process.
The formula of cumulative CO2 or N2O emissions during the growing season was as follows:
CE is the cumulative emission of gas (CO2 or N2O), Fi and F(i+1) is the gas emission fluxes (mg m-2 h-1) in two consecutive adjacent sampling periods, and d is the number of days between two consecutive adjacent sampling periods.
On a 100-year timescale, the warming potential of N2O is 298. The formula of global warming potential (GWP) was as follows:
Greenhouse gas intensity (GHGI) is an index for comprehensive evaluation of greenhouse effect. The formula was as follows:
Data analyzes were performed using Excel 2019 and SPSS 23.0 software. Significant differences between treatments were indicated by different letters at p< 0.05 level according to Fisher’s LSD. Graphs were drawn with Origin 2018 software (OriginLab, Northampton, MA, USA), R software (Available online: http://www.r-project.org/) and Adobe Illustrator CS6 (Adobe Systems Inc., CA, USA).
As shown in Figure 1, depth of straw returning significantly affected the maize yield. Compared with CK treatments, S15 and S25 treatments were significantly increased the maize yield, and was the highest under S25 treatments. In 2020, the maize yield increased significantly by 6.64% under S25 treatments and 5.22% under S15 treatments, respectively. In 2021, the maize yield increased significantly by 6.99% under S25 treatments and 5.49% under S15 treatments, respectively. In 2022, the maize yield increased significantly by 10.06% under S25 treatments and 4.17% under S15 treatments, respectively. While the S40 treatments had little effect on the maize yield, the maize yield increased significantly by 1.18% in 2020 and decreased by 1.51% in 2022.
Figure 1 Effects of depth of straw returning on maize yield. For each year, bars followed by the different letters are significantly different at P< 0.05. CK: no straw returning; S15: straw returning at 15 cm soil depth; S25: straw returning at 25 cm; S40: straw returning at 40 cm.
The depth distribution of SOC and SOC stocks was significantly affected by depth of straw returning (Table 2). Compared with the CK treatments, at the 0-20 cm depth, the SOC was the largest under S15 treatments, which increased by 8.50~14.22%, and the SOC stock was the largest under S25 treatments, which increased by 9.71~22.34%. Compared with the CK treatments, at the 20-40 cm depth, the SOC was the largest under S25 treatments, which increased by 9.91~22.55%, and the SOC stock was the largest under S40 treatments, which increased by 4.15~16.61%. Compared with the CK treatment, at the 40-60 cm depth, the SOC was the largest under S40 treatments, which increased by 7.93~18.60%, and the SOC stock was the largest under S40 treatments, which increased by 4.88~17.30%.
Table 2 Depth distribution of SOC (g kg-1) and SOC stocks under different straw returning treatments.
The depth distribution of TN and STN stocks were significantly affected by depth of straw returning (Table 3). Compared with the CK treatments, at the 0-20 cm depth, the TN and STN stock was the largest under S15 treatments, which increased by 3.02~10.18% and 2.15~8.32%, respectively. Compared with the CK treatments, at the 20-40 cm depth, the TN and STN stock was the largest under S25 treatments, which increased by 7.32~12.11% and 6.77~12.65%, respectively. Compared with the CK treatments, at the 40-60 cm depth, the TN and STN stock was the largest under S40 treatments, which increased by 4.17~14.88% and 2.47~14.98%, respectively.
Table 3 Depth distribution of TN (g kg-1) and STN stocks under different straw returning treatments.
The depth distribution of C:N ratio was significantly affected by depth of straw returning. Compared with the CK treatments, at the 0-20 cm depth, the soil C:N ratio was the largest under S15 treatments, which increased by 3.66~5.32%. Compared with the CK treatments, at the 20-40 cm depth, the soil C:N ratio was the largest under S25 treatments, which increased by 2.41~9.48%. Compared with the CK treatments, at the 40-60 cm depth, the soil C:N ratio was the largest under S40 treatment which increased by 5.32% in 2020, which was the largest under S40 treatments which increased by7.24 ~8.29% in 2021 and 2022 (Figure 2).
Figure 2 Effects of depth of straw returning on C:N ratio. For each soil layer, bars with different letters differ significantly at P< 0.05. CK, no straw returning; S15, straw returning at 15 cm soil depth; S25, straw returning at 25 cm; S40, straw returning at 40 cm.
Correlation analysis results also showed that the grain yield was significant related to the SOC, TN and soil C:N ratio (Figure 3). The grain yield had significantly positive correlations with the SOC, TN and soil C:N ratio at the 0-20 cm and 20-40 cm depth, while was not significantly correlation with the SOC, TN and soil C:N ratio at the 40-60 cm depth.
Figure 3 The relationship between Grain yield and SOC, TN and C:N ratio at different soil depths from 2020 to 2022. GN, grain yield, SOC, soil organic carbon, TN, total nitrogen, C:N ratio, carbon-nitrogen ratio.
The dynamic changes of the soil CO2 flux and CO2 emission in maize growing season under all depth of straw returning treatments were shown in Figure 4. The soil CO2 flux of each treatments showed an obvious bimodal change trend during the whole maize growing season. In the early stage of maize growth, the soil CO2 flux was larger and then gradually decreased, and reached the peak of emission flux in the middle stage of growth, and then the emission flux decreased. As shown in Figure 4, depth of straw returning was significantly increased the CO2 emission, and was the highest under S15 treatments, which was increased by 7.67~19.54% compared with the CK treatments.
Figure 4 Effects of depth of straw returning on dynamics of CO2 fluxes and cumulative CO2 emissions. For each year, bars followed by the different letters are significantly different at P< 0.05. CK, no straw returning; S15, straw returning at 15 cm soil depth; S25, straw returning at 25 cm; S40, straw returning at 40 cm.
The dynamic changes of the soil N2O flux and N2O emission in maize growing season under all depth of straw returning treatments were shown in Figure 5. The soil N2O flux of each treatment showed an obvious bimodal change trend during the whole maize growing season. In the early stage of maize growth, the soil N2O flux was larger and then gradually decreased, and reached the peak of emission flux in the middle stage of growth, and then the emission flux decreased. It can be seen that the peak value of the soil N2O flux is roughly the same as that of fertilization period. The first peak appears after base fertilizer, and the second peak appears after top-dressing fertilizer, indicating that fertilization is the main factor affecting the soil N2O flux. As shown in Figure 5, depth of straw returning treatments was significantly increased the N2O emission, and was the highest under S15 treatments, which increased by 15.41~26.56% compared with the CK treatments.
Figure 5 Effects of depth of straw returning on dynamics of N2O fluxes and cumulative N2O emissions. For each year, bars followed by the different letters are significantly different at P< 0.05. CK, no straw returning; S15, straw returning at 15 cm soil depth; S25, straw returning at 25 cm; S40, straw returning at 40 cm.
In the maize growing season, the GWP mainly comes from the CO2 and N2O emissions. In this study, the estimated results of the GWP and GHGI under all depth of straw returning treatments in maize growing season were shown in Figure 6. Compared with CK treatments, the GWP of S15, S25 and S40 treatments was increased by 9.35~20.37%, 4.27~7.67% and 0.72~6.14%, respectively, among which S15 treatment contributed the most to the GWP of farmland. The GHGI is an evaluation index of low-carbon agriculture at this stage, which takes into account both crop yield and global warming potential. In this study, the GHGI was shown a different trend from the GWP. Compared with CK treatments, S25 treatments was no significant difference in 2020, and was decreased significantly in 2021 and 2022. This is due to the combined effect of the maize yield and cumulative greenhouse gas emissions, indicating that the appropriate straw returning method can not only reduce the intensity of greenhouse gas emissions but also improve soil productivity and enhance the carbon sequestration effect of farmland soil, which is an ideal soil improvement and fertilization measure.
Figure 6 Effects of depth of straw returning on GWP and GHGI. For each year, bars followed by the different letters are significantly different at P< 0.05. CK, no straw returning; S15, straw returning at 15 cm soil depth; S25, straw returning at 25 cm; S40, straw returning at 40 cm. GWP, global warming potential, GHGI, greenhouse gas intensity.
Different straw returning methods will affect the distribution of straw in the tillage layer, and the position of straw will affect the spatial distribution of the SOC and TN (Puget and Lal, 2005; Du et al., 2010). In this study, the SOC and TN near the straw position were higher than those without straw. Studies have also shown that the SOC and TN in the soil profile is affected by the content of straw and soil organic matter (Turmel et al., 2015). Some studies have shown that straw returning can cause deep soil disturbance and promote the mineralization of organic matter contained in the soil itself (Devêvre and Horwáth, 2000). Therefore, the carbon and nitrogen released by straw decomposition and the mineralization of soil organic matter may be the two main reasons for the influence of the SOC and TN in the different soil layers. The soil C:N ratio directly affects the carbon-nitrogen cycle, carbon-nitrogen interaction and the stability of soil organic matter in farmland ecosystem (Russell et al., 2005; Tong et al., 2009). Similar to the SOC and TN, different tillage depths had a significant effect on the soil C:N ratio, and the soil layer near the straw had a higher C:N ratio. Consistent with previous studies (Puget and Lal, 2005), this study found that depth of straw returning treatments helped to improve the soil C:N ratio. According to the analysis, the improvement effect is mainly due to the fact that the carbon release rate of straw is higher than the nitrogen release rate. The phenomenon of carbon fixation and nitrogen mineralization increase is common in depth of straw returning treatments under the environment of high carbon-nitrogen ratio, which may be mainly due to straw return treatments changed the soil carbon and nitrogen status (Kramer et al., 2013; Laird and Chang, 2013). Similar to the SOC and TN, the SOC and STN stocks were also higher in the position close to the straw returning. Compared with S40 treatments, S15 and S25 treatments significantly increased the SOC and STN stocks in the 0-40 cm layer. Previous studies have shown that this may be because under the straw returning treatments, the higher carbon and nitrogen release rate in the soil layer of straw returning promoted the significant increase of the SOC and STN content, thus increasing the upper the SOC and STN stocks.
Increasing the yield per unit area on the basis of limited cultivated land is helpful to ensure food security. Important factors affecting the crop yield include temperature, sunshine, precipitation, fertilization management and tillage pattern (Hou et al., 2012; Jat et al., 2018; Tian S. Z., et al., 2016). Improving soil nutrient status and nutrient use efficiency is of great significance to ensure high and stable yield of crops and sustainable production of farmland (Xin et al., 2019). Existing studies have shown that in most soil use types, straw returning treatments can increase the soil nitrogen content, crop nitrogen use efficiency compared with no straw returning treatments (Liang et al., 2017; Smitha et al., 2019). Under the condition of dry farmland soil environment in Northeast China, the maize yield was effectively improved under the condition of conventional shallow straw returning. In this study showed that the maize yield increased significantly with straw returning treatments, especially under S25 treatments, which is similar to some previous research results (Cai et al., 2014; Tian S. Z., et al., 2016; Zhang et al., 2017). Depth of straw returning treatments was affected soil bulk density and improved crop root architecture, thus promoted the absorption and utilization of nutrients and water to ensure the healthy growth and development of crops (Huang et al., 2013). In this study, the correlation analysis showed that the maize yield was significantly positively correlated with the STC, TN and C:N ratio in the 0-20cm and 20-40cm soil layers, and not significantly correlated with the 40-60cm soil layers. These results indicate that straw returning was beneficial to increase the fixation of the STC and TN in the plough layer, thereby increasing the maize yield. It was further explained that the SOC and TN in the 0 ~ 40 cm soil layer could be used as key parameters for maize growth (Kautz et al., 2013; Raiesi, 2017; Zhang et al., 2018).
The ultimate goal of agricultural production is to take into account both economic and environmental benefits, and to ensure the sustainable development of agriculture while increasing the economic yield of crops. In this study, the emission of soil CO2 increased under all depth of straw returning treatments, which was the same conclusion as some study (Oorts et al., 2007; Bavin et al., 2009; Lenka and Lal, 2013). It shows that straw returning accelerates the decomposition of organic matter and the conversion rate of mineral nutrients by soil microorganisms, thus increased the emission of CO2. Depth of straw returning treatments have different effects on the environment of different soil layers, and the effects on the CO2 emissions were also different. In this study, the CO2 emissions decreased significantly with the increase of straw returning depth. Compared with S15 and S25 treatments, the CO2 emission flux under the S40 treatment was lower under S40 treatments. This study believes that on the one hand, when the straw were returned to the 15 cm and 25 cm soil layers, the soil temperature was higher than that of the 40 cm soil layers, which promotes the CO2 emissions. On the other hand, when the straw was returned to 40 cm, the deep water content of the soil layer greatly reduced the diffusion rate of CO2 in the soil pores, so the diffusion of CO2 to the ground decreased. In addition, some studies have also shown that with the increase of soil depth, soil catalase activity gradually decreased. When straw was returned to 40 cm, aerobic microorganisms increased less, respiration was relatively weak, and the CO2 emissions were reduced.
There are different views on the impact of straw returning on the N2O emissions. Some studies have suggested that straw returning has increased the N2O emissions by changing soil characteristics and stimulating soil microbial activity, thereby promoting denitrification (Sey et al., 2008; Xu et al., 2017; Hu et al., 2019). This study found that depth of straw returning treatments increased the soil N2O emissions, with significant peaks on the 6th and 52nd days depth of straw returning treatments, which may be related to fertilization. Fertilization provided a large amount of available nitrogen for soil microorganisms, accelerate nitrification, denitrification and mineralization, and thus promoted the N2O emissions (Qin et al., 2012; Hu et al., 2019). Soil NH4+-N and NO3–N are the direct substrates of nitrification and denitrification, and also directly affected the amount of the N2O emissions (Azeem et al., 2014). Therefore, the emission of N2O is based on the concentration of available nitrogen in the soil. Straw returning to different soil layers increased the concentration of available nitrogen and the N2O emissions (Karen and Keith, 2003; Horváth et al., 2010; Hu et al., 2013). When straw returning to the 15 cm soil layers, the cumulative emission of N2O was the largest, which may be due to the fact that the soil layer was close to the ground and the dry-wet alternation was frequent, and the suitable temperature was conducive to the reproduction of microorganisms, which accelerated the decomposition of straw and promoted the emission of N2O (Jacinthe and Lal, 2003; Castro et al., 2010). When straw returning to the 15 cm soil layers, the emission of N2O was relatively small. On the one hand, it is because deep returning reduced soil bulk density, releases nutrients to the deep layer, and increased NO3–N, thereby inhibited the activity of denitrifying enzymes. On the other hand, the degree of soil nutrient deficiency in the 40-60 cm soil layer was higher. After straw returning, the fixation of nitrogen by microorganisms was increased, and the concentration of available nitrogen in soil was reduced, thus inhibited the nitrification and denitrification processes and reduced the N2O emissions (Gebauer, 2013). Some studies have shown that the emission of CH4 in dryland soil was lower, and it is mostly absorbed (Zheng et al., 2021). This may be because the dryland soil was relatively dry, the ventilation condition was good, and oxygen was more likely to diffuse into the soil, so that the CH4 was oxidized. It may also be due to the high decomposition rate of organic matter in dryland soil, which is not easy to accumulate organic carbon, thus affecting the production and emission of CH4. Therefore, the CH4 emissions were not measured in this study.
The cumulative emissions of the soil CO2 and N2O increased after depth of straw returning treatments, which promoted the GWP of S15, S25 and S40 treatments to be significantly higher than CK treatment. It is worth noting that the GWP was decreased with the increase of straw returning depth. The GHGI is an evaluation index of low-carbon agriculture, which takes into account both the crop yield and global warming potential. In this study, the GHGI was shown a different trend from the GWP. Compared with CK treatments, S25 treatments was no significant difference in 2020, and decreased significantly in 2021 and 2022. This is due to the combined effect of the maize yield and cumulative greenhouse gas emissions, indicating that the appropriate straw returning method can not only be further improved crop yield without the cost of environmental benefits but also improve soil productivity and enhance the carbon sequestration effect of farmland soil, which is an ideal soil improvement and fertilization measure.
In this study, compared with CK treatments, depth of straw returning were increased the soil SOC and TN, and improved soil quality. The soil quality-related traits were highly correlated with the maize yield, among which S15 and S25 increased yield more obviously, indicating that the improvement of soil quality by depth of straw returning helped to increase maize yield. The analysis of the greenhouse gas emissions showed that the global warming potential gradually decreased with the increase of straw returning depth, and were significantly higher than that of CK treatments. In order to further evaluate the environmental benefits of straw returning, this study measured the GHGI, and the results showed that S25 treatments were decreased significantly compared with CK treatments. These results indicating that the appropriate straw returning depth of can not only be further improved crop yield without the cost of environmental benefits but also improve soil productivity and enhance the carbon sequestration effect of farmland soil, which is an ideal soil improvement and fertilization measure.
We ensure that all maize seeds used in this study originated from Qiqihar Branch of Heilongjiang Academy of Agricultural Sciences in Heilongjiang Province, China. The legality of these seeds complies with the IUCN Policy Statement on Research Involving Species at Risk of Extinction and the Convention on the Trade in Endangered Species of Wild Fauna and Flora. The maize seeds collected in the study are all cultivated maize in China rather than endangered and wild species. These varieties have passed the legal variety certification procedures in China and are licensed for production, planting, and market operations. The authors declare that the cultivation of plants and carrying out study in the Qiqihar maize experiment base of Heilongjiang academy of agricultural sciences complies with all relevant institutional, national and international guidelines and treaties.
The original contributions presented in the study are included in the article/supplementary material. Further inquiries can be directed to the corresponding authors.
JW: Writing – original draft. YH: Data curation, Writing – review & editing. CZ: Formal analysis, Writing – original draft. TX: Investigation, Writing – review & editing. ZQ: Supervision, Writing – original draft. BM: Software, Writing – review & editing. MY: Methodology, Writing – review & editing. LW: Methodology, Writing – original draft. YL: Software, Writing – original draft. QL: Software, Writing – review & editing. XD: Methodology, Writing – review & editing. CQ: Software, Writing – review & editing. BXM: Software, Writing – review & editing.
The author(s) declare financial support was received for the research, authorship, and/or publication of this article. This work was supported by grants from the National Maize Industry Technology system-Qiqihar integrated test station (CARS-02-46), Heilongjiang agricultural science and Technology Innovation Leap Engineering Agricultural Science and Technology Foundation Innovation Youqing Project (CX22YQ15), Heilongjiang provincial research institutes scientific research business expense project (CZKYF2023-1-C013).
The authors declare that the research was conducted in the absence of any commercial or financial relationships that could be construed as a potential conflict of interest.
All claims expressed in this article are solely those of the authors and do not necessarily represent those of their affiliated organizations, or those of the publisher, the editors and the reviewers. Any product that may be evaluated in this article, or claim that may be made by its manufacturer, is not guaranteed or endorsed by the publisher.
Adimassu, Z., Alemu, G., Tamene, L. (2019). Effects of tillage and crop residue management on runoff; soil loss and crop yield in the Humid Highlands of Ethiopia. Agric. Sys. 168, 11–18. doi: 10.1016/j.agsy.2018.10.007
Amaya, A., Medero, N., Néstor, T., Hugo, S., Cristina, D. (2007). Activated carbon briquettes from biomass materials. Biores. Technol. 98 (8), 1635–1641. doi: 10.1016/j.biortech.2006.05.049
Azeem, B., KuShaari, K., Man, Z. B., Basit, A., Thanh, T. H. (2014). Review on materials & methods to produce controlled release coated urea fertilizer. J. Controlled Release 181, 11–21. doi: 10.1016/j.jconrel.2014.02.020
Baggs, E. M., Stevenson, M., Pihlatie, M., Bhattacharyya, R. (2003). Nitrous oxide emissions following application of residues and fertiliser under zero and conventional tillage. Plant Soil 254 (2), 361–370. doi: 10.1023/A:1025593121839
Bavin, T. K., Griffis, T. J., Baker, J. M., Venterea, R. T. (2009). Impact of reduced tillage and cover cropping on the greenhouse gas budget of a maize/soybean rotation ecosystem. Agricult. Ecosyst. Environ. 134, 234–242. doi: 10.1016/j.agee.2009.07.005
Blanco-Canqui, H., Lal, R. (2008). No-tillage and soil-profile carbon sequestration: An on-farmassessment. Soil Sci. Soc. America J. 72 (3), 693–701. doi: 10.2136/sssaj2007.0233
Cai, H. G., Zhang, X. Z., Ping, J. Q., Yan, X. G., Liu, J. Z., Yuan, J. C., et al. (2014). Effect of subsoil tillage depth on nutrient accumulation, root distribution, and grain yield in spring maize. Crop J. 2, 297–307. doi: 10.1016/j.cj.2014.04.006
Castro, H. F., Classen, A. T., Austin, E. E., Norby, R. J., SChadt, C. W. (2010). Soil microbial community responses to multiple experimental climate change drivers. Appl. Environ. Microbiol. 76 (4), 999–1007. doi: 10.1128/AEM.02874-09
Devêvre, O. C., Horwáth, W. R. (2000). Decomposition of rice straw and microbial carbon use efficiency under different soil temperatures and moistures. Soil Biol. Biochem. 32, 1773–1785. doi: 10.1016/S0038-0717(00)00096-1
Du, Z. L., Ren, T. S., Hu, C. S. (2010). Tillage and residue removal effects on soil carbon and nitrogen storage in the north China plain. Soil Sci. Soc. America J. 74, 196–202. doi: 10.2136/sssaj2009.0048
Ellert, B. H., Bettany, J. R. (1995). Calculation of organic matter and nutrients stored in soils under contrasting management regimes. Can. J. Soil Sci. 75, 529–538. doi: 10.4141/cjss95-075
Gao, B., Ju, X. T., Zhang, Q., Christie, P., Zhang, F. S. (2011). New estimates of direct N2O emissions from Chinese croplands from 1980 to 2007 using localized emission factors. Biogeosciences 8 (10), 3011–3024. doi: 10.5194/bg-8-3011-2011
Gebauer, K. G. (2013). Plastic mulching in agriculture-Friend or foe of N2O emissions? Agricult. Ecosyst. Environ. 167, 43–51. doi: 10.1016/j.agee.2013.01.010
Horváth, L., Grosz, B., Machon, A., Tuba, Z., Nagy, Z., Czóbel, S. Z., et al. (2010). Estimation of nitrous oxide emission from Hungarian semi-arid sandy and loess grasslands; effect of soil parameters, grazing, irrigation and use of fertilizer. Agricult. Ecosyst. Environ. 139 (1), 255–263. doi: 10.1016/j.agee.2010.08.011
Hou, X. Q., Li, R., Jia, Z. K., Han, Q. F., Wang, W., Yang, B. P. (2012). Effects of rotational tillage practices on soil properties, winter wheat yields and water-use efficiency in semi-arid areas of north-west China. Field Crops Res. 129, 7–13. doi: 10.1016/j.fcr.2011.12.021
Hu, N. J., Chen, Q., Zhu, L. Q. (2019). The responses of soil N2O emissions to residue returning systems: a meta-analysis. Sustainability 11 (3), 1–7. doi: 10.3390/su11030748
Hu, X. K., Su, F., Ju, X. T., Gao, B., Oenema, O., Christie, P., et al. (2013). Greenhouse gas emissions from a wheat-maize double cropping system with different nitrogen fertilization regimes. Environ. pollut. 176, 198–207. doi: 10.1016/j.envpol.2013.01.040
Huang, Y., Bi, S. Y., Zou, H. T., Dou, S. (2013). Effect of straw deep returning on corn root system and yield. J. Maize Sci. 21, 109–112. doi: 10.13597/j.cnki.maize.science.2013.05.025
Jacinthe, P. A., Lal, R. (2003). Nitrogen fertilization of wheat residue affecting nitrous oxide and methane emission from a central Ohio Luvisol. Biol. Fertil. Soils 37 (6), 338–347. doi: 10.1007/s00374-003-0607-4
Jat, R. D., Jat, H. S., Nanwal, R. K., Yadav, A. K., Bana, A., Choudhary, K. M., et al. (2018). Conservation agriculture and precision nutrient management practices in maize-wheat system: Effect on crop and water productivity and economic profitability. Field Crops Res. 222, 111–120. doi: 10.1016/j.fcr.2018.03.025
Karen, E. D., Keith, A. S. (2003). Nitrous oxide emission factors for agricultural soils in Great Britain: the impact of soil water-filled pore space and other controlling variables. Global Change Biol. 9 (2), 00563.x. doi: 10.1046/j.1365-2486.2003.00563.x
Kautz, T., Amelung, W., Ewert, F., Gaiser, T., Horn, R., Jahn, R. (2013). Nutrient acquisition from arable subsoils in temperate climates: A review. Soil Biol. Biochem. 57, 1003–1022. doi: 10.1016/j.soilbio.2012.09.014
Kramer, S., Marhan, S., Haslwimmer, H., Ruess, L., Kandeler, E. (2013). Temporal variation in surface and subsoil abundance and function of the soil microbial community in an arable soil. Soil Biol. Biochem. 61, 76–85. doi: 10.1016/j.soilbio.2013.02.006
Kuzyakov, Y., Friedel, J. K., Stahr, K. (2000). Review of mechanisms and quantification of priming effects. Soil Biol. Biochem. 32 (11-12), 1485–1498. doi: 10.1016/S0038-0717(00)00084-5
Laird, D. A., Chang, C. W. (2013). Long-term impacts of residue harvesting on soil quality. Soil Tillage Res. 134, 33–40. doi: 10.1016/j.still.2013.07.001
Lenka, N. K., Lal, R. (2013). Soil aggregation and greenhouse gas flux after 15 years of wheat straw and fertilizer management in a no-till system. Soil Tillage Res. 126, 78–89. doi: 10.1016/j.still.2012.08.011
Liang, G., Wu, H., Houssou, A. A., Cai, D., Wu, X., Gao, L., et al. (2017). Soil respiration, glomalin content, and enzymatic activity response to straw application in a wheat-maize rotation system. J. Soil Sediment 18, 697–707. doi: 10.1007/s11368-017-1817-y
Linquist, B., Van-Groenigen, K. J., Adviento-Borbe, M. A., Pittelkow, C., Van, K. C. (2012). An agronomic assessment of greenhouse gas emissions from major cereal crops. Global Change Biol. 18, 194–209. doi: 10.1111/j.1365-2486.2011.02502.x
Liu, N., Li, Y., Cong, P., Wang, J., Wei, H., Zhang, L. (2021). Depth of straw incorporation significantly alters crop yield, soil organic carbon and total nitrogen in the north China plain. Soil Tillage Res. 205 (1), 104772. doi: 10.1016/j.still.2020.104772
Lu, R. K. (2000). The analysis method of soil agriculture chemistry (Beijing: China Agricultural Science and Technology Press), 106–110.
Mu, X. Y., Zhao, Y. L., Liu, K., Ji, B. Y., Guo, H. B., Xue, Z. W., et al. (2016). Responses of soil properties, root growth and crop yield to tillage and crop residue management in a wheat-maize cropping system on the North China Plain. Europ. J. Agron. 78, 32–43. doi: 10.1016/j.eja.2016.04.010
Oorts, K., Merckx, R., Gréhan, E., Labreuche., J., Nicolardot, B. (2007). Determinants of annual fluxes of CO2 and N2O in long-term no-tillage and conventional tillage systems in northern France. Soil Tillage Res. 95, 133–148. doi: 10.1016/j.still.2006.12.002
Puget, P., Lal, R. (2005). Soil organic carbon and nitrogen in a Mollisol in central Ohio as affected by tillage and land use. Soil Tillage Res. 80, 201–213. doi: 10.1016/j.still.2004.03.018
Qin, S., Wang, Y., Hu, C., Oenema, O., Li, X., Zhang, Y., et al. (2012). Yield-scaled N2O emissions in a winter wheat-summer corn double-cropping system. Atmos Environ. 55, 240–244. doi: 10.1016/j.atmosenv.2012.02.077
Raiesi, F. (2017). A minimum data set and soil quality index to quantify the effect of land use conversion on soil quality and degradation in native rangelands of upland arid and semiarid regions. Ecol. Indic. 75, 307–320. doi: 10.1016/j.ecolind.2016.12.049
Russell, A. E., Laird, D. A., Parkin, T. B., Mallarino, A. P. (2005). Impact of nitrogen fertilization and cropping system on carbon sequestration in Midwestern Mollisols. Soil Sci. Soc. America J. 69, 413–422. doi: 10.2136/sssaj2005.0413
Saggar, S. (2010). Estimation of nitrous oxide emission from ecosystems and its mitigation technologies. Agric. Ecosyst. Environ. 136 (3-4), 189–191. doi: 10.1016/j.agee.2010.01.007
Sey, B. K., Whalen, J. K., Gregorich, E. G., Rochette, P., Cue, R. I. (2008). Carbon dioxide and nitrous oxide content in soils under corn and soybean. Soil Sci. Soc. America J. 72 (4), 931–938. doi: 10.2136/sssaj2007.0093
Smith, D. M., Cusack, S., Colman, A. W., Folland, C. K., Harris, G. R., Murphy, J. M. (2007). Improved surface temperature prediction for the coming decade from a global climate model. Science 317 (5839), 796–799. doi: 10.1126/science.1139540
Smitha, G. R., Basak, B. B., Thondaiman, V., Saha, A. (2019). Nutrient management through organics, bio-fertilizers and crop residues improves growth, yield and quality of sacred basil (Ocimum sanctum Linn). Ind. Crop Prod. 128, 599–606. doi: 10.1016/j.indcrop.2018.11.058
Sui, P., Tian, P., Lian, H., Wang, Z., Ma, Z., Qi, H., et al. (2020). Straw incorporation management affects maize grain yield through regulating nitrogen uptake, water use efficiency, and root distribution. Agronomy 10 (3), 324. doi: 10.3390/agronomy10030324
Tian, P., Lian, H., Wang, Z., Jiang, Y., Li, C., Sui, P., et al. (2020). Effects of deep and shallow tillage with straw incorporation on soil organic carbon, total nitrogen and enzyme activities in Northeast China. Sustainability 12, 8679. doi: 10.3390/su12208679
Tian, P., Sui, P., Lian, H., Wang, Z., Meng, G., Sun, Y., et al. (2019). Maize straw returning approaches affected straw decomposition and soil carbon and nitrogen storage in Northeast China. Agronomy 9, 818. 2. doi: 10.3390/agronomy9120818
Tian, S. Z., Ning, T. Y., Wang, Y., Liu, Z., Li, G., Li, Z. J., et al. (2016). Crop yield and soil carbon responses to tillage method changes in North China. Soil Till. Res. 163, 207–213. doi: 10.1016/j.still.2016.06.005
Toma, Y., Hatano, R. (2007). Effect of crop residue C:N ratio on N2O emissions from Gray Lowland Soil in Mikasa, Hokkaido, Japan. Soil Sci. Plant Nutr. 53 (2), 198–205. doi: 10.1111/j.1747-0765.2007.00125.x
Tong, C. L., Xiao, H. A., Tang, G. R., Wang, H. Q., Huang, T. P., Xia, H. A., et al. (2009). Long-term fertilizer effects on organic carbon and total nitrogen and coupling relationships of C and N in paddy soils in subtropical China. Soil Tillage Res. 106, 8–14. doi: 10.1016/j.still.2009.09.003
Trost, B., Prochnow, A., Drastig, K., Andreas, M. A., Frank, E., Michael, B. (2013). Irrigation, soil organic carbon and N2O emissions. A review. Agron. Sustain. Dev. 33 (4), 733–749. doi: 10.1007/s13593-013-0134-0
Turmel, M. S., Speratti, A., Baudron, F., Verhulst, N., Govaerts, B. (2015). Crop residue management and soil health: A systems analysis. Agric. Syst. 134, 6–16. doi: 10.1016/j.agsy.2014.05.009
Xia, L. L., Wang, S. W., Yan, X. Y. (2014). Effects of long-term straw incorporation on the net global warming potential and the net economic benefit in a rice-wheat cropping system in China. Agric. Ecosys. Environ. 197, 118–127. doi: 10.1016/j.agee.2014.08.001
Xin, W., Zhang, L., Zhang, W., Gao, J., Yi, J., Zhen, X., et al. (2019). An integrated analysis of the rice transcriptome and metabolome reveals root growth regulation mechanisms in response to nitrogen availability. Int. J. Mol. Sci. 20, 5893. doi: 10.3390/ijms20235893
Xu, C., Han, X., Bol, R., Smith, P., Wu, W. L., Meng, F. Q. (2017). Impacts of natural factors and farming practices on greenhouse gas emissions in the North China Plain: a meta analysis. Ecol. Evol. 7 (17), 3211. doi: 10.1002/ece3.3211
Xue, J. F., Pu, C., Liu, S. L., Chen, Z. D., Chen, F., Xiao, X. P., et al. (2015). Effects of tillage systems on soil organic carbon and total nitrogen in a double paddy cropping system in Southern China. Soil Tillage Res. 153, 161–168. doi: 10.1016/j.still.2015.06.008
Zhang, Y. J., Wang, R., Wang, S. L., Wang, H., Xu, Z. G., Jia, G. C., et al. (2017). Effects of different sub-soiling frequencies incorporated into no-tillage systems on soil properties and crop yield in dry land wheat-maize rotation system. Field Crops Res. 2017, 151–158. doi: 10.1016/j.fcr.2017.05.002
Zhang, X. F., Zhu, A. N., Xin, X. L., Yang, W. L., Zhang, J. B., Ding, S. J. (2018). Tillage and residue management for long-term wheat-maize cropping in the North China Plain: I. Crop yield integrated Soil fertil. index. Field Crops Res. 221, 157–165. doi: 10.1016/j.fcr.2018.02.025
Zheng, J., Fan, J. L., Zhang, F. C., Guo, J. J., Yan, S., Zhuang, Q., et al. (2021). Interactive effects of mulching practice and nitrogen rate on grain yield, water productivity, fertilizer use efficiency and greenhouse gas emissions of rainfed summer maize in northwest China. Agric. Water Manage. 248, 106778. doi: 10.1016/j.agwat.2021.106778
Zhong, L., Li, G. Y., Chen, G. Y. (2022). Research progress on the distribution characteristics of crop straws and the preparation and application of straw carbon-based fertilizers in China. J. Agric. Resour. Environ. 39, 575–585. doi: 10.13254/j.jare.2021.0070
Keywords: straw returning, maize, yield potential, greenhouse gases, soil organic carbon
Citation: Wang J, Han Y, Zhou C, Xu T, Qu Z, Ma B, Yuan M, Wang L, Liu Y, Li Q, Ding X, Qian C and Ma B (2024) Effects of depth of straw returning on maize yield potential and greenhouse gas emissions. Front. Plant Sci. 15:1344647. doi: 10.3389/fpls.2024.1344647
Received: 26 November 2023; Accepted: 30 January 2024;
Published: 21 February 2024.
Edited by:
Günter Neumann, University of Hohenheim, GermanyReviewed by:
Kailou Liu, Jiangxi Institute of Red Soil, ChinaCopyright © 2024 Wang, Han, Zhou, Xu, Qu, Ma, Yuan, Wang, Liu, Li, Ding, Qian and Ma. This is an open-access article distributed under the terms of the Creative Commons Attribution License (CC BY). The use, distribution or reproduction in other forums is permitted, provided the original author(s) and the copyright owner(s) are credited and that the original publication in this journal is cited, in accordance with accepted academic practice. No use, distribution or reproduction is permitted which does not comply with these terms.
*Correspondence: Chunrong Qian, cWlhbmppYW55aTMxOEAxNjMuY29t; Baoxin Ma, bmpzbTkxNzBAMTI2LmNvbQ==
†These authors have contributed equally to this work
Disclaimer: All claims expressed in this article are solely those of the authors and do not necessarily represent those of their affiliated organizations, or those of the publisher, the editors and the reviewers. Any product that may be evaluated in this article or claim that may be made by its manufacturer is not guaranteed or endorsed by the publisher.
Research integrity at Frontiers
Learn more about the work of our research integrity team to safeguard the quality of each article we publish.