- 1Department of Biology and Biotechnology ‘L. Spallanzani’, University of Pavia, Pavia, Italy
- 2Trait and Genome Engineering Cluster, Rice Breeding Innovations, International Rice Research Institute, Metro Manila, Philippines
Water stress brought about by climate change is among the major global concerns threatening food security. Rice is an important staple food which requires high water resources. Being a semi-aquatic plant, rice is particularly susceptible to drought. The aim of this work was to develop techniques directed to promote rice resilience to water deprivation stress during germination by implementing specific seed priming treatments. Five popular Italian rice varieties were subjected to priming treatments using novel, sustainable solutions, like poly-gamma-glutamic acid (γ-PGA), denatured γ-PGA (dPGA), and iron (Fe) pulsing, alone or in combination. The effect of the developed priming methods was tested under optimal conditions as well as under water deprivation stress imposed by polyethylene glycol (PEG) treatments. The priming efficacy was phenotypically determined in terms of germination behavior by measuring a series of parameters (germinability, germination index, mean germination time, seed vigor index, root and shoot length, germination stress tolerance index). Biochemical analyses were carried out to measure the levels of iron uptake and accumulation of reactive oxygen species (ROS). Integrative data analyses revealed that the rice varieties exhibited a strong genotype- and treatment-specific germination behavior. PEG strongly inhibited germination while most of the priming treatments were able to rescue it in all varieties tested except for Unico, which can be defined as highly stress sensitive. Molecular events (DNA repair, antioxidant response, iron homeostasis) associated with the transition from seed to seedling were monitored in terms of changes in gene expression profiles in two varieties sensitive to water deprivation stress with different responses to priming. The investigated genes appeared to be differentially expressed in a genotype-, priming treatment-, stress- and stage-dependent manner. The proposed seed priming treatments can be envisioned as sustainable and versatile agricultural practices that could help in addressing the impact of climate challenges on the agri-food system.
1 Introduction
Re-emergence of global hunger is related to increasing obstacles in agricultural food production associated with the climate change scenario (https://www.fao.org/publications/sofi/2022/en/). Actions to mitigate these challenges are envisioned in the Sustainable Development Goals (SDG) Agenda 2030, mainly in SDG#2 - Zero hunger. Rice has high potential for aiding the accomplishment of SDG#2 due to its global consumption. This staple crop contributes to 35 - 59% of the daily caloric intake for billions of people, being the third most cultivated crop in the world (Sperotto et al., 2012). As a semiaquatic plant, rice is particularly sensitive to drought, a condition progressively worsening in the current climate scenario (Shahzad et al., 2021; Habib-Ur-Rahman et al., 2022). Hence, there is a need to develop strategies that could mitigate drought stress, starting from the germination stage (Panda et al., 2021).
Seed germination is one of the most important processes in the life of the plant, marking the transition from the quiescent state to a metabolically active state, initiating a series of biophysical, biochemical and molecular changes leading to radicle emergence and subsequent seedling growth (Han and Yang, 2015; Macovei et al., 2017; Yu et al., 2021). Within the agricultural context, optimal seed germination ensures seedling vigor and subsequently high yields during harvest, which are however negatively influenced by water deprivation stress (Osakabe et al., 2014; Reed et al., 2022). In the case of rice, numerous drought stress-related studies have been conducted at the reproductive stage due to its direct relation to yield (Rang et al., 2011; He and Serraj, 2012; Favreau et al., 2023; Lou et al., 2023) while less attention has been given to the germination stage.
Mature seeds contain an embryo in a quiescent state, characterized by reduced metabolism, although transcriptional and post-transcriptional modifications in response to the environmental signals remain partially active to sense and start the germination process under favorable conditions (Holdsworth et al., 2008). Seed germination is a triphasic process, where phase I is characterized by rapid water uptake, which in rice, corresponds to the first 20 h of imbibition. Phase II (lag phase) spans from 20 to 48 h, whereas phase III, representative of radicle emergence, starts after 48 h from imbibition (Yang et al., 2007). Complex physiological and biochemical events are involved in the transition from the dry, quiescent seed to the metabolically active state, including an intermediary state called pre-germinative metabolism, characterized by highly dynamic changes in carbohydrate metabolism, signal transduction, DNA synthesis, gene expression, regulation of redox homeostasis, and DNA repair (Howell et al., 2009; Macovei et al., 2017; Sano et al., 2022). Moreover, the de novo transcription happening during the early stages of germination is further subjected to additional regulation during seedling establishment (Rajjou et al., 2012; Sano et al., 2012). During the reactivation of the seed metabolism, reactive oxygen species (ROS) are produced at high levels due to rapid water uptake, and this can be potentially harmful if their levels pass a certain threshold (Baily et al., 2008, Pagano et al., 2022). To maintain ROS balance, the activation of antioxidant defense mechanisms are required to ensure successful seedling development (Kranner et al., 2010; Rajjou et al., 2012; Pagano et al., 2023). Moreover, efficient activation of the DNA damage response (DDR) pathway is necessary to repair DNA damage and safeguard seedling genome integrity (Balestrazzi et al., 2011; Pagano et al., 2017; Waterworth et al., 2019).
Generally, water deprivation stress can occur when water availability is low, potentially triggering loss of turgor pressure at a cellular level. In an agronomic context, water deprivation stress represents only a part of the complex drought conditions which highly restricts plant growth by reducing cellular elongation and expansion, detrimentally affecting seedling development and germination (Bhandari et al., 2023). Rice is highly sensitive to dry conditions during germination and the early phases of seedling growth. Low water potential in the soil prevents seedling from absorbing water, reducing seedling vigor (Kruthika and Jithesh, 2023; Székely et al., 2023). The effects of water deprivation stress include altered membrane transport, disruption of metabolic processes, decreased ATP synthesis, leading to aborted germination, or severe seedling growth reduction (Batlang et al., 2013; Oladosu et al., 2019; Kota et al., 2023). Transcriptional and hormonal activities, along with ROS levels, are highly affected when imbibition and seedling development occur during water stress conditions (Ishibashi et al., 2018; Kawaguchi et al., 2023).
Water deprivation stress at the germination stage can be addressed through several practices that include seed treatments to induce osmoprotection, limiting ROS accumulation, or enhancing the seed repair response. Among these treatments, seed priming is envisaged as a sustainable, cost-effective, and easy to use technique dedicated to improve seed germination even under adverse conditions. Seed priming is a pre-sowing technique that involves controlled imbibition, allowing seeds to reach the early stages of germination without radicle protrusion, followed by subsequent drying (Paparella et al., 2015; Devika et al., 2021). These treatments allow increased and uniform germination by acting on enhancing enzyme activation, metabolite production, repair of damaged DNA and osmotic regulation (Gerna et al., 2018; Forti et al., 2020a; Forti et al., 2020b; Wang et al., 2022). Even upon exposure to drought stress, this technique can speed up seedling emergence through the regulation of metabolic process in the early phases of germination (Marthandan et al., 2020). In rice, different priming methods, including hydropriming (Nakao et al., 2020), nanopriming (Waqas Mazhar et al., 2022), chemical priming applied through the use of salt solutions (Ali et al., 2021), plant extracts (Khan et al., 2022) or plant growth elicitors (Samota et al., 2017), have been applied and were shown to mitigate the effects of drought stress at different levels. Although the research in this field is steadily advancing, there is still the need to understand how priming works at a molecular level, and develop strategies that could mitigate water deprivation stress starting from the germination stage, taking into consideration also the intraspecific genetic variability (Pagano et al., 2023).
The priming protocols developed in this study utilize novel seed priming agents, namely, poly-gamma-glutamic acid (γ-PGA), denatured γ-PGA (dPGA), and iron (Fe) pulsing, applied to five economically important Italian rice varieties. Polyethylene glycol (PEG) was used to simulate water deprivation stress, as this is an inert and water-binding polymer with a non-ionic and impermeable long chain which mimics water and osmotic stress (Perez-Fernandez et al., 2006). γ-PGA, used as a chemical priming agent, has the potential to alleviate the effect of water stress due to its hygroscopic and water-holding qualities (Ogunleye et al., 2015). Additionally, dPGA can be used as a nutripriming agent given that it is constituted of single glutamic acid molecules released upon γ-PGA degradation. The combination of Fe pulsing with either γ-PGA or dPGA was also conducted to investigate a possible cumulative effect on rice germination under water deprivation stress. Phenotyping (germination, seedling growth, and stress parameters), biochemical (ROS detection, Fe quantification), and molecular analyses (gene expression profiling), were carried out to investigate the effect of priming and stress conditions on rice germination.
2 Materials and methods
2.1 Seed materials and water stress treatments
A selection of five popular Italian rice (Oryza sativa L.) varieties, namely Carnaroli, Cerere, Unico, Lomello, and Apollo, were used for the current study. Seeds were kindly supplied by Sardo Piemontese Sementi (Sa.Pi.Se, https://sapise.it/) and the University of Pavia Botanical Garden Plant Germplasm Bank (https://terraeambiente.dip.unipv.it/it/dipartimento/risorse/banca-del-germoplasma-vegetale).
To impose water deprivation stress during seed germination, a 25% PEG6000 (polyethylene glycol) solution was used to simulate an osmotic potential of -1215.9 kPa (Stanley and Strey, 2003) while distilled water (0 kPa) was used as a non-stress control. Before selecting this concentration, preliminary trials using 15%, 20% and 25% PEG were carried out on the Lomello variety. Petri dishes, containing 30 seeds/replicate, lined with a two-layer filter paper and 7 ml of PEG6000 or water, were placed in a growth chamber at 26°C under dark for two days and subsequently at 16 h light/8 h dark cycle for 10 days. The light conditions included a photon flux density of 150 μmol m-2s-1 and 70–80% relative humidity inside the containers. The system was laid out in a complete randomized design with three replicates.
2.2 Extraction of poly-gamma glutamic acid and denatured PGA
Recovery of crude γ-PGA was conducted by adapting a protocol from Massaiu et al. (2019). Bacillus subtilis strain PB5390 (Ermoli et al., 2021) was streaked on an LB plate and incubated at 37° overnight for single colonies. A pre-culture was prepared by incubating 6 colonies in LB liquid media containing 0.5% glucose at 37° with 233 rpm shaking for 8 h. The OD was measured at 600 nm to calculate the volume of the pre-culture that was added to the E-medium (40 g L-1 KC5H8NO4, 12 g L-1 Na3C6H5O7, 80 g L-1 glucose, 0.104 g L-1 MnSO4, 0.5 g L-1 MgSO4, 7 g L-1 K2HPO4, 0.04 g L-1 CaCl, 0.004 g L-1 FeCl, 0.05 g L-1 tryptophan, 0.05 g L-1 phenylalanine). The culture was incubated in the same conditions for 60 h. The culture was centrifuged (8,000 rpm, 15 min, 10°C) and the supernatant was collected. γ-PGA was precipitated with three volumes of cold methanol and kept at -20°C for 16 h. The mixture was later centrifuged to recover the γ-PGA pellet. The pellet was resuspended in 100 mM NaCl. The concentration of isolated crude γ-PGA was measured using a spectrophotometer at 216 nm in a quartz cuvette. A standard calibration curve with commercial γ-PGA was used to compute its concentration in g L-1. Absorbance measurement was carried out via the NanoPhotometer UV/Vis spectrophotometer (Implen, Schatzbogen, München, Germany), using 100 mM NaCl as blank. Denatured γ-PGA (dPGA) was prepared by incubating the extracted γ-PGA in a 120° oven for 72 h.
2.3 Priming treatments
For γ-PGA and dPGA priming treatments, sets of 30 rice seeds were placed in an open mouth flat bottomed tube and filled with 3 ml of 1 g L-1 γ-PGA or dPGA. Floating seeds on the surface were gently tapped to ensure total submergence in the soaking solution. The seeds were soaked for 16 hours at room temperature before direct sowing in Petri plates containing 25%PEG solution or water.
The iron (Fe) pulsing protocol used in this study was adapted from Dey et al. (2021). A volume of 3 ml of water was poured into an open mouth-flat bottom tube containing 30 rice seeds per replicate. The seeds were soaked for 16 hours at room temperature. Afterward, the seeds were sown directly on 85 mm plastic Petri dishes lined with 2 filter papers soaked in 7 ml of 10 mM ferrous sulphate heptahydrate (FeSO4.7H20). Alternatively, iron pulsing was combined with either γ-PGA (treatment PGA-Fe) or with dPGA (treatment dPGA-Fe) priming. For the PGA-Fe and dPGA-Fe priming, water was replaced with a soaking solution of either 0.001 g L-1 γ-PGA or 0.001 g L-1 dPGA. The sowing for Fe treatment started after soaking from Day 0 of the experiment. After 3 days, the seeds were transferred to new Petri plates with 2 filter papers and 7 ml of distilled water (non-stressed condition) or 25%PEG (stressed condition) and allowed to grow for a duration of 10 days.
Water soaking (WS) was included among the treatments to serve as a control for distinguish between the effect of the different agents used (PGA, dPGA, Fe) from that of water. Moreover, WS can also be considered as a priming treatment and it was previously used as a traditional pre-sowing technique in rice cultivation (Farooq et al., 2006). For the WS treatment, rice seeds were soaked in water for 16 hours at room temperature and subsequently directly sown in the 25%PEG- or water-containing Petri dishes.
A schematic representation of the experimental design based on different priming protocols and germination in the presence of 25%PEG-induced stress or non-stress conditions is given in Figure 1.
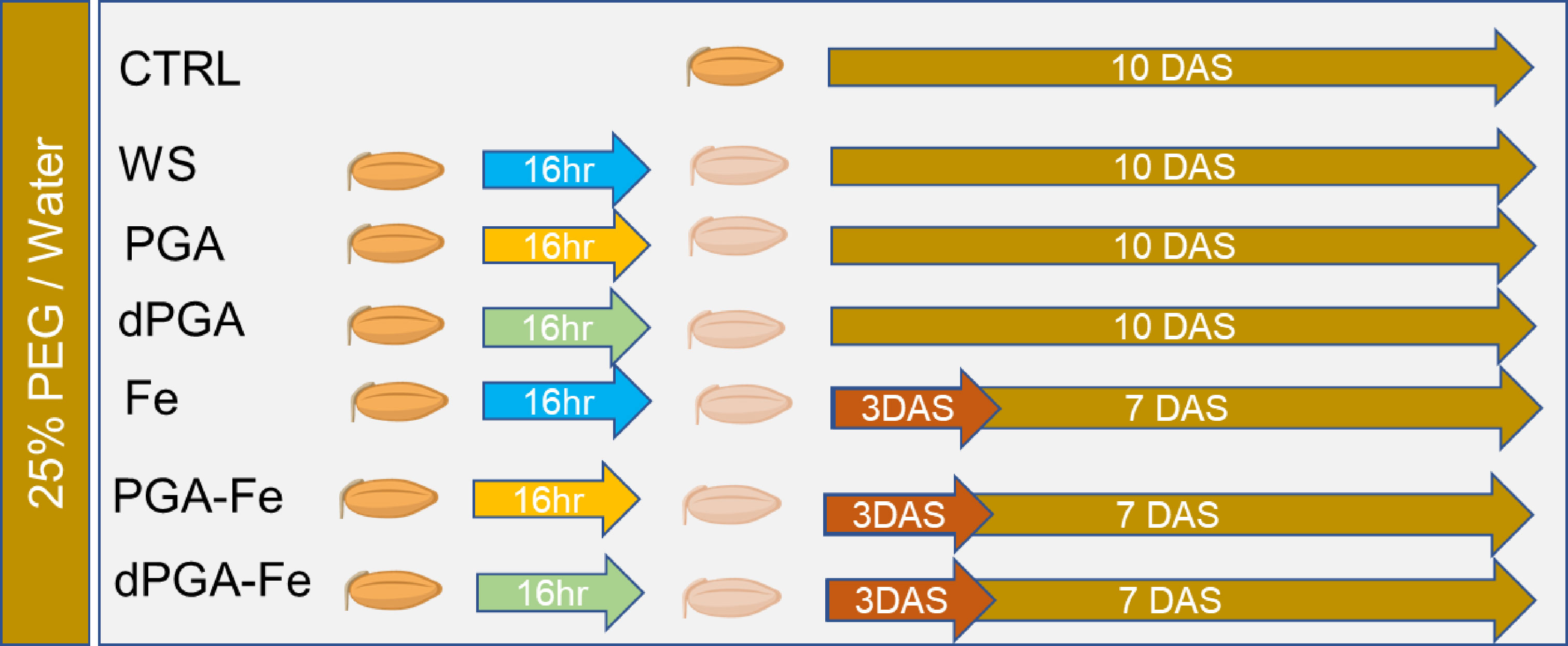
Figure 1 Overview of the experimental system developed to compare the effects of proposed seed priming protocols on the germination behavior of Italian rice varieties under 25%PEG-induced stress or non-stress (water) conditions. CTRL, unprimed control; WS, water soaking; PGA, poly-gamma-glutamic acid; dPGA, denatured poly-gamma-glutamic acid; Fe, iron pulsing; DAS, days after sowing. Blue arrow indicates that seeds were soaked for 16 h in water. Yellow arrow indicates that seeds were soaked for 16 h in PGA. Green arrow indicates that seeds were soaked for 16 h in dPGA.
2.4 Phenotyping analyses
The number of germinated seeds was recorded at 24 h intervals. Seeds were considered as germinated when they reached the S2 stage, when both the plumule and radicle were 2 mm long (Counce et al., 2000). Different sets of parameters were collected and calculated to evaluate germination performance, seedling growth, and stress tolerance (Kader, 2005; Ranal and Santana, 2006; Mahmood et al., 2022). For the evaluation of germination performance, the following parameters were used: germinability (G%), germination index (GI), mean germination time (MGT), and synchronization index (Z). To assess seedling growth, root length (Root), shoot length (Shoot), and seedling vigor index I (VII), were used. To test the water deprivation stress resilience or sensitivity, several tolerance indices were selected as follows: germination water stress tolerance index calculated at 7 days (GDTIMax), shoot length water stress tolerance index (SLWTI), and root length water stress tolerance index (RLWTI). The formulas used for the calculations of each parameter and the explanation of the obtained values are given in Supplementary Table 1. Measurements of shoot and root length were taken using the ImageJ program (https://imagej.nih.gov/ij/download.html).
2.5 Histochemical staining for Fe and H2O2 detection
The presence of Fe in the embryo axis was evaluated using Perl’s Prussian staining protocol adapted from Shobhana et al. (2013). Seedlings were removed from the plates after 10 days from sowing, thoroughly washed with water, and soaked in Perl’s stain containing 2% potassium ferrocyanide (K4[Fe(CN)6]) and 2% hydrochloric acid (HCl), for 30 min at room temperature under dark and vacuum conditions. Blue color develops in the presence of Fe in the tissue.
The levels of hydrogen peroxide (H2O2) in the embryo axis tissue were evaluated using the 3,3’-diaminobenzidine (DAB) staining method (Macovei et al., 2010). The DAB solution was prepared at a 0.005 g L-1 concentration by dissolving 3,3’-diaminobenzidine tetrahydrochloride in Tris-Buffered Saline (TBS, 50 mM Tris-Cl, pH 7.5, 150 mM NaCl) buffer. At the end of the treatments, 10-day old seedlings were soaked in 0.005 g L-1 DAB stain for 1 h under dark and vacuum conditions. Brown color develops in the presence of H2O2 in the tissue.
For both types of histochemical staining methods, a semi-quantitative approach was carried out to estimate the presence of Fe or H2O2 in the embryo axis, and data are presented as fold change to control. The intensity color gradient was measured using the ImageJ program.
2.6 RNA extraction and cDNA synthesis
Samples from the PEG-induced stress were collected at 3 time points, namely 16 h, 3 and 7 days after sowing (DAS), along with the embryo axis extracted from dry (untreated) mature seeds. Relatively 80 to 90 mg of tissue for embryo axis and 100 to 150 mg of tissue for seedlings were grinded in liquid N and collected into 1.5 ml tubes. For RNA isolation the TRIzol extraction method was applied following manufacturer’s indications. A volume of 750 μl of TRIzol reagent (Thermo Fisher Scientific, Monza, Italy) was added to each tube, vortexed for 5 s, and incubated in ice for 5 min. A volume of 150 μl chloroform was added, gently mixed, incubated for 3 min on ice, and centrifuged at 10,000 rpm for 15 min at 4°C. The upper phase was collected and transferred to new 1.5 ml tubes and an equal volume of isopropanol was added. The samples were incubated at -20°C for 20 min then centrifuged with the same settings and the supernatant was discarded to collect the RNA pellet. The pellet was washed with 500 μl ice-cold 70% ethanol and centrifuged, followed by two additional washes with 500 μl ice cold absolute ethanol. Finally, the pellet was air dried and resuspended in nuclease-free water. The concentration of each RNA sample was measured using a Biowave spectrophotometer (Biochrom Ltd., England), and the integrity of RNA samples was assessed on agarose gel. For cDNAs synthesis, the RevertAid First Strand cDNA Synthesis Kit (ThermoFisher Scientific, Monza, Italy) was used, according to the manufacturer’s recommendations.
2.7 Quantitative real-time PCR
The qRT-PCR reactions were carried out on a CFX Duet Real-time PCR system machine (Bio-rad Laboratories Inc., Milan, Italy) using the Maxima SYBR Green qPCR Master Mix (Thermo Fisher Scientific, Monza, Italy) as indicated by the manufacturer. The machine was operated using the Bio-rad CFX maestro software (Bio-rad Laboratories Inc.) and the following amplification conditions were applied: denaturation at 95°C, 10 min, and 40 cycles of 95°C, 15 s and 60°C, 30 s, final extension at 72°C, 30 s.
Oligonucleotide primers (Supplementary Table 2) were designed using Primer3plus (https://www.primer3plus.com/index.html) and subsequently verified with Oligo Analyzer (https://eu.idtdna.com/calc/analyzer), and Primer-Blast (https://www.ncbi.nlm.nih.gov/tools/primer-blast/index.cgi?GROUP_TARGET=on). The list of investigated genes include: LigVI (DNA LIGASE VI), FPG (FORMAMIDOPYRIMIDINE-DNA GLYCOSYLASE), NBS1 (NIJMEGEN BREAKAGE SYNDROME 1 MUTATED GENE), OGG1 (8-OXOGUANINE DNA GLYCOSYLASE), OPT7 (OLIGOPEPTIDE TRANSPORTER 7), PARP1 (POLY [ADP-RIBOSE] POLYMERASE 1), RAD51 (RADIATION SENSITIVE PROTEIN 51), TDP1α (TYROSYL-DNA PHOSPHODIESTERASE 1α), TDP1ß (TYROSYL-DNA PHOSPHODIESTERASE 1β), AoX1b (ALTERNATIVE OXIDASE 1B), APX6 (ASCORBATE PEROXIDASE 6), Cu/ZnSOD (CU/ZN SUPEROXID DISMUTASE), SODFe (FE SUPEROXID DISMUTASE), MIR (MITOCHONDRIAL-IRON REGULATED), NAS1 (NICOTIANAMINE SYNTHASE 1), NRAMP1 (NATURAL RESISTANCE-ASSOCIATED PROTEIN 1), VIT1 (VACUOLAR IRON TRANSPORTER), AAP1 (ASPARTATE AMINOTRANSFERASE 1), C3H50 (CYSTEINE 2 HISTIDINE). The 25sRNA was selected as a reference gene given that it was previously reported to be stable across different growth conditions and developmental stages in rice (Jain et al., 2006). The Thomsen method was used for relative quantification of transcript accumulation using the efficiency value of 1.8 (Thomsen et al., 2010). All reactions were carried out in triplicates. For data representation, Z-score was calculated on the linearized Ct values and used to generate heatmaps using the OriginPro 2023b software (OriginLab Corporation, Northampton, MA, USA).
2.8 Statistical analyses
Statistical analyses were carried out using the Statistical Tool for Agricultural Research 2.0.1 (STAR 2.0.1) software. Significant differences among varieties and treatments were determined though a two-way ANOVA (Analysis of Variance) method. The significance of the results was evaluated at a 0.05% level (p ≤ 0.05) using the Least Significant Difference (LSD) or Tukey’s Honest Significant Difference (HSD) tests. Means with the same designated letters are indicated as not significantly different. For the qRT-PCR data, the Student t-test (*, p ≤ 0.05) was used for statistical interpretation where each priming treatment was compared to the non-primed control (CTRL). A Principal Component Analysis (PCA) was conducted using the Originpro 2023b software (https://www.originlab.com/2023), following user’s guide specifications.
3 Results
3.1 Seed priming mitigates the negative effect of water stress during germination
Seed of Apollo, Carnaroli, Cerere, Lomello, and Unico varieties were treated with either water (WS), PGA, dPGA, Fe, PGA-Fe-, and dPGA-Fe. An unprimed control (CTRL) was also used. Primed and unprimed seeds were sown in Petri dishes under control conditions and PEG-induced stress. To evaluate the effect of the priming protocols, several germination parameters were analyzed.
In the absence of stress, no significant changes were observed in terms of germination percentage between the priming treatments and unprimed seeds (Supplementary Figure 1). The WS treatment showed a significant effect on the germination of all the tested varieties, specifically in terms of GI (Supplementary Table 3). A genotype-dependent effect of priming was observed. In Apollo, WS and PGA-Fe treatments decreased the germination time (MGT) by a day while the root length of seedlings from WS-, PGA-Fe-, and dPGA-Fe-treated seeds were significantly longer than the CTRL. The PGA, PGA-Fe, and dPGA-Fe treatments also resulted in high vigor (VII) values. For Carnaroli, increased shoot growth was observed in seedlings from WS- and dPGA-Fe-treated seeds while VII was improved only after WS treatment. The Cerere variety showed enhanced root and shoot length following WS, PGA, and dPGA treatments. The MGT decreased by 3 days in the Lomello variety, leading to a significant increase in root length following WS application. Treatments with PGA-Fe and dPGA-Fe also increased root length in Lomello. Unico showed no change in germination parameters across all priming methods applied, except for the GI values that were slightly improved upon WS treatments. Germinability (Gmax) and synchronicity (Z) did not significantly vary throughout the priming treatments in all varieties.
To simulate water deprivation stress, a PEG concentration of 25%, corresponding to -1215.9 kPa (Stanley and Strey, 2003), reported as the maximal limit in previous studies in rice (Islam et al., 2018; Sobahan et al., 2022) was used in this study. A preliminary test, conducted on the Lomello variety (Supplementary Figure 2) using 15%, 20%, and 25% PEG, indicated that 25% PEG allowed a better distinction between primed and unprimed seeds. Therefore, this concentration was chosen for subsequent analyses. Among the tested varieties, Lomello was identified as water deprivation stress tolerant as it showed germination values of 32.33 ± 5.03% under simulated drought, while Apollo, Carnaroli, Cerere, and Unico were classified as stress-sensitive since they did not germinate under these conditions (Supplementary Table 4, CTRL Gmax). However, the germinability of Apollo and Cerere seeds was rescued by iron pulsing (Fe) alone or in combination with PGA (PGA-Fe) and dPGA (dPGA-Fe). Significantly improved Gmax, GI, and VII values were registered following these priming techniques under PEG stress. The significant difference in MGT observed for Carnaroli and Cerere may be attributed to the events taking place during the first phases of germination, started during the 16 h soaking treatments applied before stress induction, while the CTRL was represented by dry seeds. The Unico variety can be considered as highly susceptible to water deprivation stress since even after the priming treatments seeds it did not germinate in the presence of PEG.
Since there was an increase in germinability among the stress-sensitive varieties upon priming, the germination water stress tolerance index (GWTI), root length, shoot length, and seedling vigor (VII) were assessed. Figure 2 shows the graphical progression of calculated values for each variety, while the statistical significance is provided in Supplementary Table 5. Overall, the previously observed trend was maintained among varieties also for these parameters. In terms of GWTI, Apollo showed a significant enhancement after treatments when Fe was applied either alone or in combination with PGA (PGA-Fe) and dPGA (dPGA-Fe) (Figure 2A). Carnaroli showed improved GWTI for most treatments (except dPGA) when compared to the CTRL conditions, and this was particularly significant for Fe pulsed seeds, reaching 58% at 10 DAS (Figure 2B). Cerere achieved elevated GWTI values up to 57%, specifically for the PGA-Fe-treated seeds (Figure 2C). For the highly-tolerant Lomello variety (Figure 2D) and the highly-sensitive Unico variety (Figure 2E), no significant changes were observed in terms of calculated GWTI values.
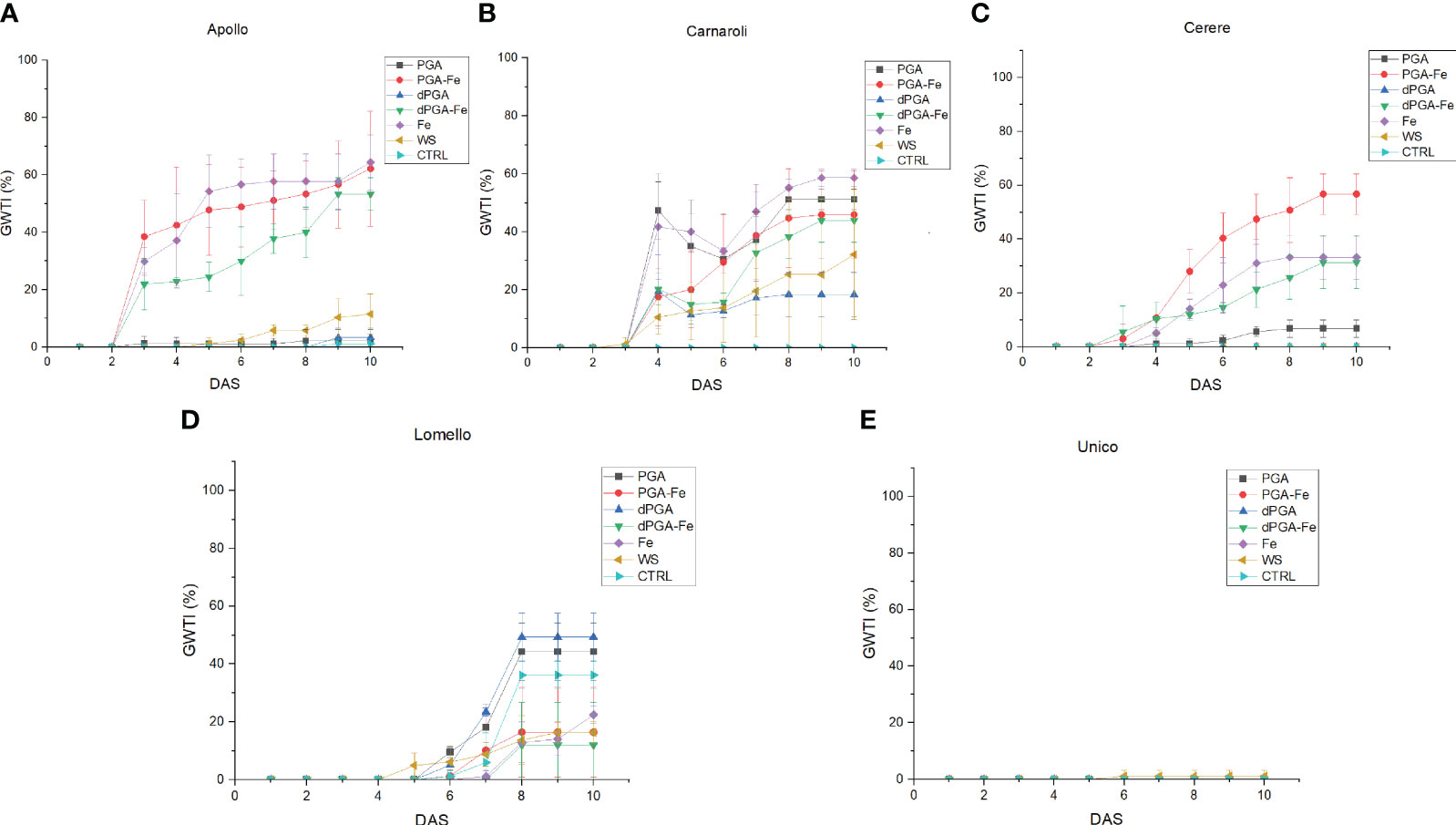
Figure 2 Germination water stress tolerance index (GWTI) for (A) Apollo, (B) Carnaroli, (C) Cerere, (D) Lomello, and (E) Unico. Data represent the means ± st.dev. of three independent replicates of 30 seeds each. CTRL, unprimed control; PGA, poly-gamma-glutamic acid; dPGA, denatured poly-gamma-glutamic acid; Fe, iron pulsing; WS, water soaking; DAS, days after sowing. Statistical analysis is provided in Supplementary Table 5.
When considering the calculated stress vigor indexes (Figure 3) similar patterns were observed among varieties. The elevated root length upon PEG-induced stress indicates that all seed priming treatments (except WS) applied on Carnaroli (Figure 3B), and iron pulsing (Fe, PGA-Fe, and dPGA-Fe) applied on Cerere (Figure 3C) and Apollo (Figure 3A), were able to mitigate the negative effect of water stress. The stress-tolerant Lomello variety showed significant differences compared to the CTRL for PGA and Fe pulsing treatments (Figure 3D), while no growth was registered in the case of Unico (Figure 3E) variety.
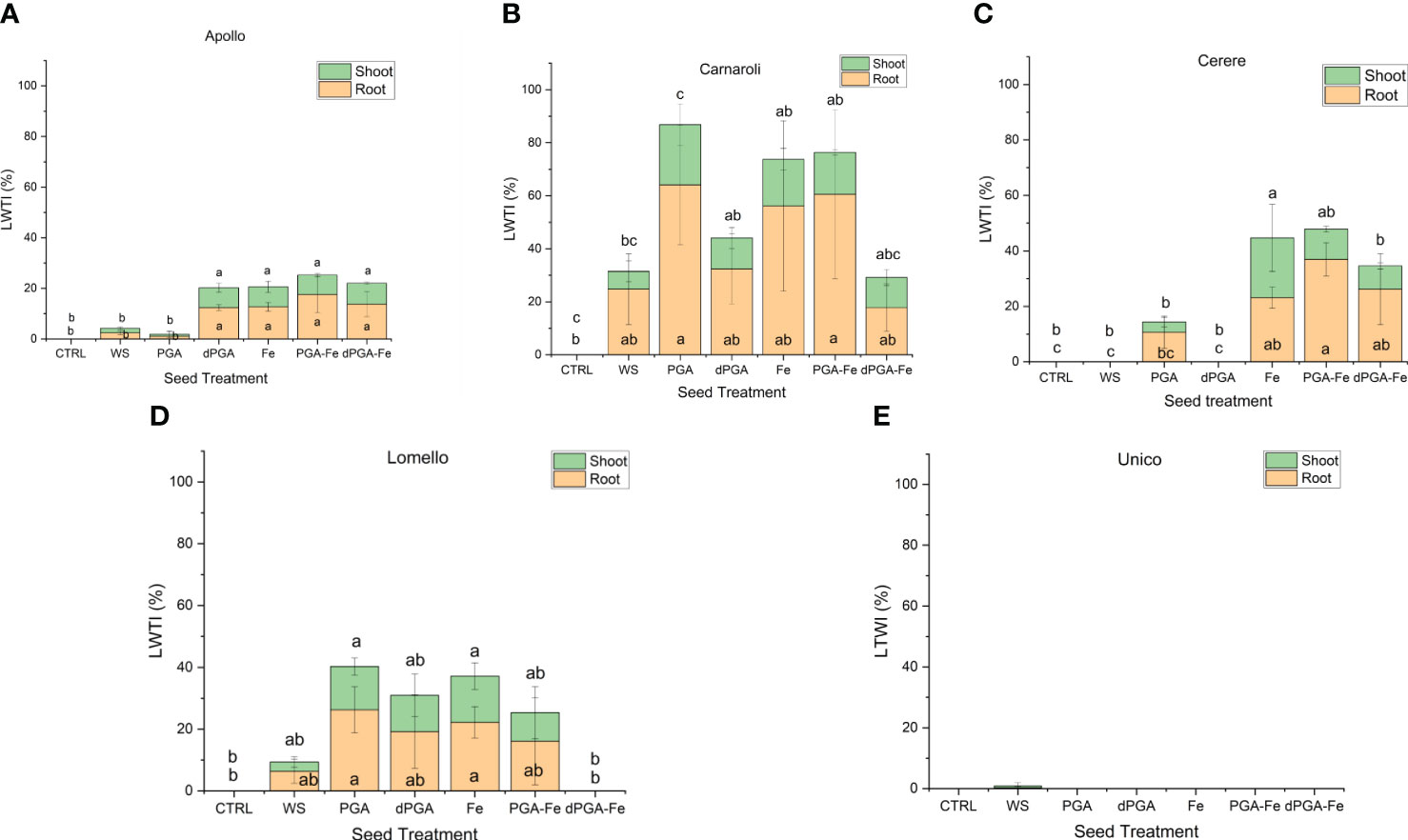
Figure 3 Calculated vigor tolerance indexes under stress in terms of shoot and root length (LTWI, length tolerance water stress index) for (A) Apollo, (B) Carnaroli, (C) Cerere, (D) Lomello, and (E) Unico. Data represent the means ± st.dev. of three independent replicates of 30 seeds each. CTRL, unprimed control; PGA, poly-gamma-glutamic acid; dPGA, denatured poly-gamma-glutamic acid; Fe, iron pulsing; WS, water soaking; DAS, days after sowing. Samples followed by different letters indicate statistically significant (p ≤ 0.05) differences determined using the ANOVA Tukey’s test.
Overall, the proposed seed priming treatments were able to improve rice germination under both stress and non-stress conditions in a genotype-specific manner. The 25% PEG-induced stress strongly inhibited germination while most of the priming treatments were able to rescue it in all varieties tested except for Unico, which can be defined as highly sensitive to water deprivation stress. Importantly, the identification of Lomello as being highly stress tolerant and responsive to priming may help in enhancing its adoption not only for cultivation but also in breeding programs to further promote this trait.
3.2 The genotype-specific responses to priming are supported by PCA integrative analyses
To better evidence the genotype-specific behavior, an integrative analysis was carried out by applying a Principal Component Analysis (PCA) based on all the germination parameters collected from 1 to 10 DAS. Two PCA clusters were generated relative to the data regarding non-stressed (Figure 4A) and stressed (Figure 4B) conditions. The two principal components (PC) contributed to 70.76% (in which 47.38% belongs to PC1 and 23.38% belongs to PC2) of the observed variability for non-stressed conditions and 79.86% (in which 67.31% belongs to PC1 and 12.55% belongs to PC2) of the variability in case of PEG-induced stress. The clustering pattern obtained from the germination behavior of the seeds grown under optimal condition (Figure 4A) grouped the varieties according to the genetic clades described by Mongiano et al. (2018); namely, Carnaroli and Lomello were grouped around G2 clade, Apollo to G4, while Cerere and Unico were grouped to clade G5.
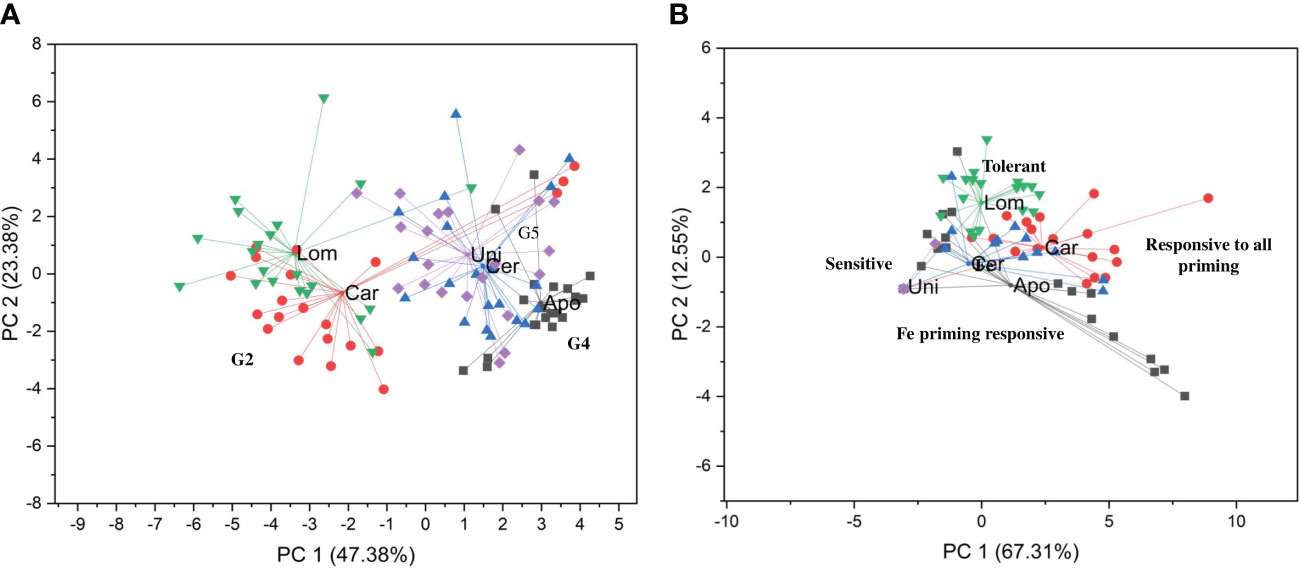
Figure 4 PCA clustering generated using all germination parameters collected from 1 to 10 DAS for the five Italian rice varieties and seed priming treatments. (A) PCA plot for optimal germination conditions. (B) PCA plot for PEG-induced water stress. Lom, Lomello; Car, Carnaroli; Uni, Unico; Apo, Apollo; Cer, Cerere; G2, G4, G5 genetic clades described by Mongiano et al. (2018) for variety development.
On the other side, the clustering pattern based on the data collected from PEG-induced stress revealed a treatment-based grouping (Figure 4B). Apollo and Cerere were grouped closely as responsive to iron treatments (Fe, PGA-Fe, and dPGA-Fe), while Carnaroli clustered separately since this variety was responsive to most seed treatments. Unico, was placed close to Apollo, and Cerere, forming the group of varieties sensitive to PEG-induced stress, as reflected from all the germination data. The difference between Unico and the other sensitive varieties consists in the fact that while Apollo and Cerere were positively affected by priming, Unico was not. Lomello represented another cluster as majority of the germination parameters investigated pointed towards an enhanced tolerance to water deprivation stress. Its position close to Carnaroli is in agreement with the fact that the priming treatments resulted in a further improvement of stress tolerance.
Based on the PCA results, it can be inferred that the G2 clade (including Lomello and Carnaroli) is a potential source of genetic materials that can be used for developing water deprivation stress tolerant varieties. Results from this analysis suggest that the trends in priming responsiveness could reflect the breeding treads presented by Mongiano et al. (2018).
3.3 Determination of iron and hydrogen peroxide accumulation during the imposed treatments
Perls Prussian blue staining was performed to measure Fe accumulation in 10 DAS seedlings while DAB staining was used to measure the accumulation of H2O2.
Based on the results of Perls Prussian blue staining, it can be observed that, as expected, only the Fe-related priming methods (Fe, PGA-Fe, and dPGA-Fe) displayed Fe accumulation in the germinating seedlings (Figure 5). High levels of Fe can be detected in the Cerere variety both in the absence (Figure 5A) and presence of PEG (Figure 5B). This provides further evidence in support of the previous observation regarding the high response of this variety to priming treatments that includes Fe pulsing (Fe, PGA-Fe, dPGA-Fe). In the absence of stress, most varieties accumulated high Fe levels in the seedlings, except for Carnaroli, which is less responsive to Fe pulsing as evidenced by the phenotyping data. Under PEG-induced stress, Fe levels seem to decrease in most varieties, except Cerere and Unico (Figure 5B). This may be due to the fact that Fe requirement is generally enhanced during stress as Fe is needed to supply the energy and redox power necessary to support seedling growth and antioxidant activity (Rout and Sahoo, 2015).
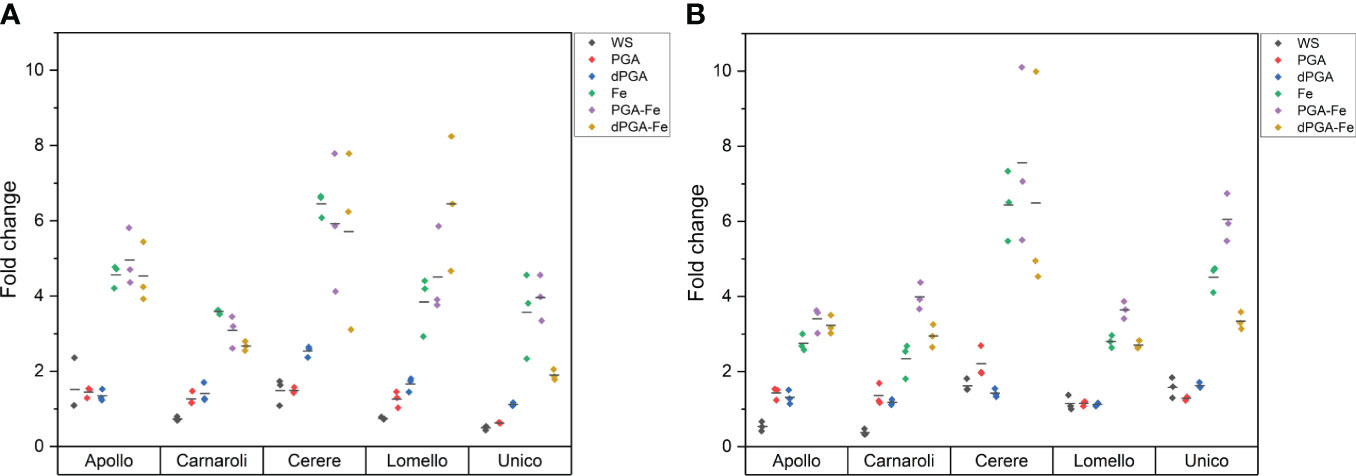
Figure 5 Semiquantitative measurement of iron accumulation through Perls Prussian Blue staining in 10 DAS rice seedlings in the (A) absence and (B) presence of 25% PEG-induced stress. Horizontal bars indicate the average fold change with respect to the control from the three replicates. PGA, poly-gamma-glutamic acid; dPGA, denatured poly-gamma-glutamic acid; Fe, iron pulsing; WS, water soaking.
It is well-known that overproduction of ROS can be observed during water stress while stress tolerance is associated with the ability to reduce ROS by increased activity of the antioxidant defense mechanism (Wang et al., 2019). In seeds, accumulation of H2O2 is also necessary to activate germination since ROS can modulate metabolic and hormone signaling pathways that induce germination (Farooq et al., 2021).
In the case of DAB staining, similar levels of H2O2 are observed in the presence and absence of stress (Figure 6). This may indicate that the proposed priming treatments were effective in balancing ROS production during seedling growth both under optimal and stress conditions. In terms of varieties tested, Apollo showed the lowest observed values in the absence of stress (Figure 6A). Considering the priming treatments, dPGA and dPGA-Fe resulted in the highest levels of H2O2 accumulation in the seedlings of Cerere and Lomello varieties. In the presence of PEG stress (Figure 6B), accumulation of H2O2 was induced by Fe pulsing, specifically for Carnaroli and Lomello varieties. In the case of Apollo, PGA and dPGA also resulted in enhanced H2O2 accumulation compared to non-stress conditions.
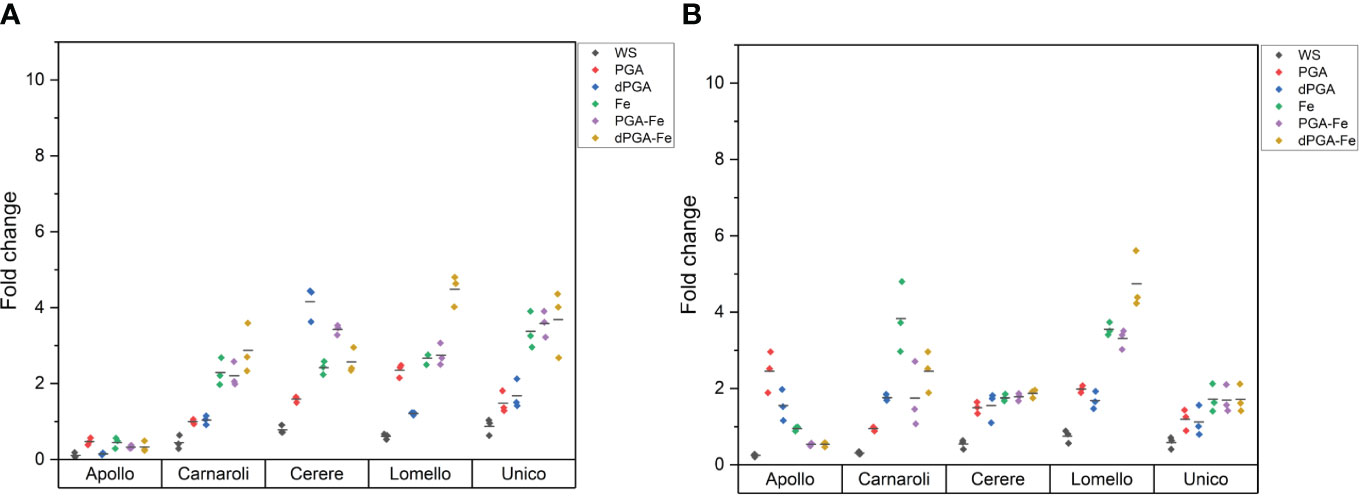
Figure 6 Semiquantitative measurement of H2O2 staining through DAB staining in 10 DAS rice seedlings in the (A) absence and (B) presence of 25% PEG-induced stress. Horizontal bars indicate the average fold change with respect to the control from the three replicates. PGA, poly-gamma-glutamic acid; dPGA, denatured poly-gamma-glutamic acid; Fe, iron pulsing; WS, water soaking.
In conclusion, the semiquantitative measurement of Fe and H2O2 levels in seedlings supported the genotype-specific responses to water deprivation stress and priming treatments.
3.4 Changes in gene expression profiles in response to priming and water deprivation stress during the transition from seed to seedlings
To further investigate the observed genotype-specific responses to priming and water stress, qRT-PCR analyses were conducted on genes selected on the basis of the available literature and a preliminary data mining approach (Supplementary Figure 3). These genes belong to specific processes like DNA damage response (DDR), antioxidant defense mechanism (ADM), and Fe and Zn homeostasis (Fe/Zn). Additionally, few genes related to drought stress response and amino acid transport (SAAT) were included. DDR genes were selected because DNA damage can accumulate during the early pre-germinative phase, when rapid water uptake generates enhanced ROS production (Rajjou et al., 2012; Pagano et al., 2023). This damage must be repaired prior to cell division to minimize induction of mutations and inhibition of seedling growth and development (Waterworth et al., 2015; Waterworth et al., 2022), therefore DDR genes are envisioned as hallmarks of the seed repair response (Pagano et al., 2019; Forti et al., 2020a; Forti et al., 2020b). Rice genes associated with the antioxidant defense were evaluated as further indicators of stress tolerance (Wang et al., 2019; Panda et al., 2021). Fe/Zn related genes were selected to evaluate metal homeostasis during germination as these are important minerals for metabolic processes during germination. This is in agreement with the fact that the supply of ferrous ions as a priming treatment lead to positive effect on germination, as evidenced by the phenotypic and biochemical analyses.
To address the transition phase from seed to seedlings, the expression of the selected genes was evaluated in Carnaroli and Cerere varieties at different timepoints during germination; namely, in dry seeds (CTRL, 0 h), during seed imbibition (16 h) and at radicle protrusion (3 DAS), both in presence and absence of stress. The two varieties were selected since they exhibited sensitivity to water deprivation stress and responded differently to the imposed priming methods. Specifically, Carnaroli showed efficient responses to all the priming methods while Cerere’s response was mostly related to the presence of iron (Fe, PGA-Fe, dPGA-Fe).
During seed imbibition (16 h), only WS, PGA, and dPGA priming treatments were considered in the absence of stress because this was the timepoint selected for stopping the imposed soaking treatments before proceeding with Fe pulsing and subsequent sowing on water or PEG-containing plates. Control dry, non-primed seeds (CLTR, 0 h) are also included in this comparison. The results are presented as a heatmap (Figure 7) where the blue color indicates low expression while red color indicates high gene expression. In the Carnaroli variety, the PARP1, APX6, SOD-Fe, NAS1, NRAMP1 and MIR genes were highly expressed in dry seeds (Figure 7B, CTRL). Changes in the expression patterns of the investigated genes were observed in response to the different priming treatments. The WS treatment resulted in a significant upregulation mainly of DDR-related genes (NBS1, RAD51, TDP1α, LigIV), and SAAT-related genes (C3H50, AAP1). Differently, some of the ADM (SOD-Fe) and Fe/Zn-related genes (MIR) were downregulated. The PGA priming resulted in significant upregulation of NBS1, AOX1b, SOD-Cu/Zn, and C3H50 genes and downregulation of PARP1, SOD-Fe, NAS1, NRAMP1 and MIR genes. Further downregulation was observed following dPGA priming where mostly the Fe/Zn homeostasis was involved (NAS1, NRAMP1, MIR) along with few DDR (PARP1) and ADM (APX6, SOD-Cu/Zn) genes. In the Cerere variety, all the tested genes were expressed at low levels in dry seeds (Figure 7B, CTRL). In this case, the priming treatments that mostly induced the expression of the genes were dPGA and WS while PGA resulted in a slight upregulation of only few DDR-related genes (NBS1, PARP1, FPG). In the case of dPGA, all tested genes were significantly upregulated compared to CTRL, whereas the WS treatment induced the expression of most ADM-, Fe/Zn- and SAAT-related genes.
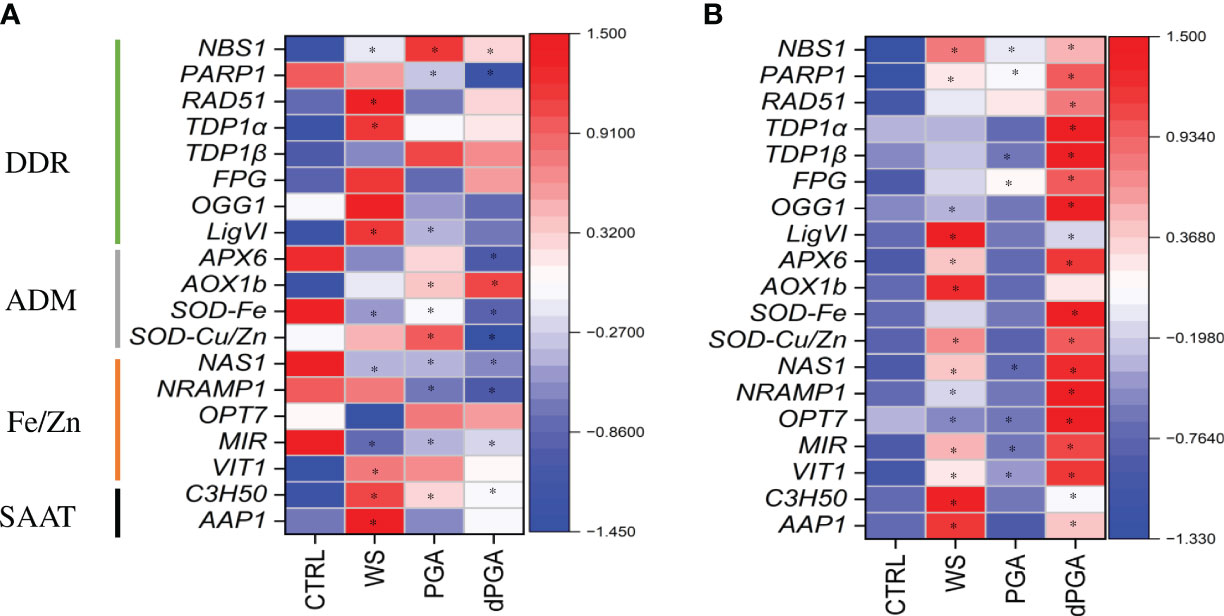
Figure 7 Heatmaps representing the expression patterns of the selected genes during seed imbibition (16 h) in (A) Carnaroli and (B) Cerere seeds subjected to priming treatments. *p ≤ 0.05 as indicated by the Student t-test. CTRL, dry seeds, unprimed control; WS, water soaking; PGA, poly-gamma-glutamic acid; dPGA, denatured poly-gamma-glutamic acid; LigVI, DNA LIGASE VI; FPG, FORMAMIDOPYRIMIDINE-DNA GLYCOSYLASE; NBS1, NIJMEGEN BREAKAGE SYNDROME 1 MUTATED GENE; OGG1, 8-OXOGUANINE DNA GLYCOSYLASE; OPT7, OLIGOPEPTIDE TRANSPORTER 7; PARP1, POLY [ADP-RIBOSE] POLYMERASE 1; RAD51, RADIATION SENSITIVE PROTEIN 51; TDP1α, TYROSYL-DNA PHOSPHODIESTERASE 1α; TDP1ß, TYROSYL-DNA PHOSPHODIESTERASE 1β; AoX1b, ALTERNATIVE OXIDASE 1B; APX6, ASCORBATE PEROXIDASE 6; Cu/ZnSOD, CU/ZN SUPEROXID DISMUTASE; SODFe, FE SUPEROXID DISMUTASE; MIR, MITOCHONDRIAL-IRON REGULATED; NAS1, NICOTIANAMINE SYNTHASE 1; NRAMP1, NATURAL RESISTANCE-ASSOCIATED PROTEIN 1; VIT1, VACUOLAR IRON TRANSPORTER; AAP1, ASPARTATE AMINOTRANSFERASE 1; C3H50, CYSTEINE 2 HISTIDINE.
At 3 DAS, the gene espression pattern was investigated in response to all priming methods in the presence and absence of PEG-induced stress (Figure 8). In this case, the CTRL samples represent seedlings obtained from unprimed seeds. The genotype-specific expression pattern is again quite evident by observing the data obtained under optimal conditions (Figures 8A, C) while PEG induced specific changes mosty when considering the different priming treatments (Figures 8B, C). In Carnaroli at 3 DAS, all the genes were already highly expressed in the CTRL samples (Figure 8A). The priming treatment resulting in a further upregulation of most genes (except for AOX1b wich is downregulated) in the dPGA-Fe combination. The Fe and PGA-Fe treatments also resulted in upregulation of Fe/Zn-related genes. Differently, under PEG stress, all the genes were poorly expressed in the CTRL samples for this variety (Figure 8B). Significant gene upregulation was mainly observed following PGA-Fe priming while DDR-related genes were induced also by WS. As for Cerere, the genes were expressed at low levels in CTRL samples both in the presence or absence of stress (Figures 8C, D). Under optimal conditions, WS mostly induced a significant upregulation of Fe/Zn-related genes while the PGA-Fe priming lead to the upregulation of DDR and ADM genes (Figure 8C). Differently, upon PEG stress the priming treatments that generally induce gene upregulation were PGA and dPGA (Figure 8C). Gene upregulation was also observed following Fe pulsing (alone or in combination) but at a lower extent.
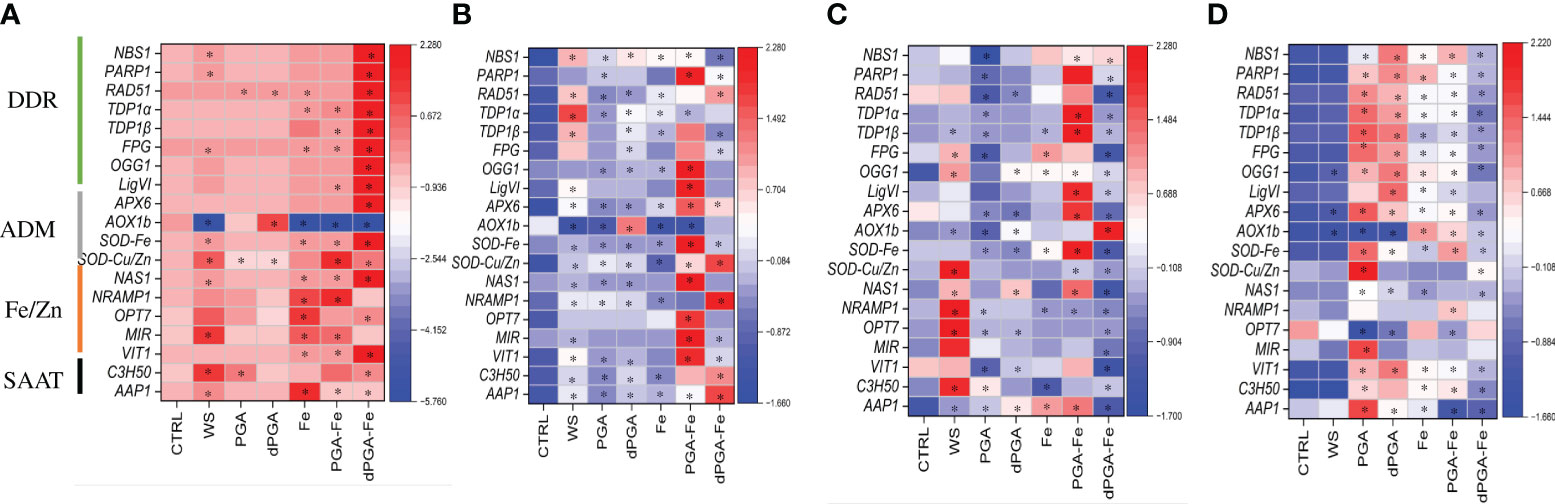
Figure 8 Heatmaps representing the expression patterns of selected genes at 3 days after sowing in presence/absence of priming and PEG stress. (A) Carnaroli seedlings under optimal conditions. (B) Carnaroli seedlings under PEG-induced stress. (C) Cerere seedlings under optimal conditions. (D) Cerere seedlings under PEG-induced stress. *p ≤ 0.05 as indicated by the Student t-test. CTRL, dry seeds, unprimed control; WS, water soaking; PGA, poly-gamma-glutamic acid; dPGA, denatured poly-gamma-glutamic acid; Fe, iron pulsing; LigVI, DNA LIGASE VI; FPG, FORMAMIDOPYRIMIDINE-DNA GLYCOSYLASE; NBS1, NIJMEGEN BREAKAGE SYNDROME 1 MUTATED GENE; OGG1, 8-OXOGUANINE DNA GLYCOSYLASE; OPT7, OLIGOPEPTIDE TRANSPORTER 7; PARP1, POLY [ADP-RIBOSE] POLYMERASE 1; RAD51, RADIATION SENSITIVE PROTEIN 51; TDP1α, TYROSYL-DNA PHOSPHODIESTERASE 1α; TDP1ß, TYROSYL-DNA PHOSPHODIESTERASE 1β; AoX1b, ALTERNATIVE OXIDASE 1B; APX6, ASCORBATE PEROXIDASE 6; Cu/ZnSOD, CU/ZN SUPEROXID DISMUTASE; SODFe, FE SUPEROXID DISMUTASE; MIR, MITOCHONDRIAL-IRON REGULATED; NAS1, NICOTIANAMINE SYNTHASE 1; NRAMP1, NATURAL RESISTANCE-ASSOCIATED PROTEIN 1; VIT1, VACUOLAR IRON TRANSPORTER; AAP1, ASPARTATE AMINOTRANSFERASE 1; C3H50, CYSTEINE 2 HISTIDINE.
To investigate if the gene expression trends persisted, 7 DAS seedlings grown under PEG-induced stress were evaluated (Supplementary Figure 4). At this timepoint, results were similar in the two varieties. Namely, the CTRL samples were charaterized by high levels of gene expression wherease, in most cases, the priming treatments resulted in downregulation. In Carnaroli, upregulated genes included NRAMP1 and OPT7 in response to PGA, and SOD-Cu/Zn and AAP1 in response to Fe and dPGA-Fe. In Cerere, the only upregulated gene was AOX1b following dPGA priming.
To summarize, the different germinative behaviour was supported by genotype-specific gene expression patterns following the transition from seeds to seedlings. Specific genes were induced by specific priming treatments both in presence and absence of PEG stress. At 16 h, WS-induced gene upregulation was present in the Carnaroli variety while dPGA mostly affected gene expression in Cerere. PEG treatments added another layer of complexity to the investigated system. In Carnaroli, most genes were downregulated by PEG at 3 DAS in CTRL samples, in agreement with the seeds not being able to germinate under stress in the absence of priming, in contrast with the high expression recorded under optimal conditions. In Cerere, WS stimulates gene expression at 3 DAS under optimal conditions while PGA and dPGA induce the expression of genes under PEG stress. Although it seems difficult to correlate the germination behaviour with the gene expression patters, it can be stated that all genes tested were differentially expressed in a genotype-, priming-, stress- and stage-dependent manner.
4 Discussion
In the current study, different seed priming treatments were developed to improve rice germination under water deprivation stress. Given the empirical nature of priming protocols and the influence that the genetic base can have on the efficacy of these treatments, five varieties of high economic impact in the Mediterranean area were tested. In a context where erratic climate changes highly affect crop productivity, screening for tolerant varieties along with the possible applications of easy-to-use, sustainable, and economic treatments to enhance germination and yield is of utmost importance.
The priming protocols developed in this study utilize novel seed priming agents like poly-gamma-glutamic acid (PGA), denatured γ-PGA (dPGA), applied alone or in combination with iron pulsing (Fe). PGA is a biodegradable, non-immunogenic and non-toxic anionic homopolymer made-up of D- and L-glutamic acid units linked by γ-amide bonds (Kimura et al., 2004; Ogunleye et al., 2015). It is mainly produced and secreted by Bacillus species (Osera et al., 2009). When released in the environment, γ-PGA contributes to biofilm formation and can help other organisms survive under adverse conditions (Yu et al., 2016). Additionally, the release of glutamic acid subunits from the long polymeric chains can represent a source of carbon and nitrogen (Ogunleye et al., 2015). Since it is biodegradable and non-toxic, γ-PGA has been utilized in a wide array of applications in agriculture, cosmetics, and medical industries. In agriculture, its application generates a positive impact on plant growth, soil microbial community, nitrogen pools, and water/fertilizer use efficiency (Xu et al., 2013; Zhang et al., 2017). Recent reports associated its use to drought tolerance in several plant species, including rapeseed (Xu et al., 2020) and corn (Yin et al., 2018). Currently, there is no report proposing the use of γ-PGA as a seed priming treatment nor has this been tested on rice. Nevertheless, agricultural byproducts, including rice bran, have been used to enhance bacterial PGA production through fermentation (Tang et al., 2015; Song et al., 2019). Moreover, the denatured PGA (formed by single or few glutamic acid residues) can be envisioned as nutripriming, as an exogenous source of glutamic acid. This compound is used by plant cells for the production of GABA (gamma-aminobutyric acid), a key player in the plant response to drought as well as nitrogen mobilizer in germinating seeds (Seifikalhor et al., 2019). Iron pulsing was previously applied to rice seeds by using a ferrous salt (FeSO4), and this treatment proved to be favorable during germination (Dey et al., 2021). It also promoted seedling growth by facilitating photosynthesis and nitrogen assimilation, leading to increased chlorophyll content, more efficient activity of the antioxidant system, and ultimately improved yield.
To test whether these priming methods could aid rice seeds germinate better under water deprivation stress, polyethylene glycol (PEG) was used; this agent is widely used to regulate the osmotic potential due to the high hydrophilicity that allows it to sequester water molecules. Previous reports indicated PEG as an ideal system for germination screening due to its high molecular weight that prevents its entry through the plant cell walls while simulating water retention (Islam et al., 2018; Sobahan et al., 2022). According to a 100-rice variety screening study (Islam et al., 2018), as well as other more recent reports (Sobahan et al., 2022), 25% PEG is considered the highest concentration that can be utilized for water stress simulation. Data collected in the present work on multiple parameters related to germination efficiency (G%, GI, MGT, Z), seedling growth (root, shoot, VII) and stress resilience (GDTIMax, SLWTI, RLWTI), evidenced a genotype-specific germination behavior. Carnaroli was responsive to all the priming methods, whereas Cerere and Apollo showed positive changes in the germinative behavior under the treatment with Fe, PGA-Fe, and dPGA-Fe. While the strong genotype-dependent effect of seed priming is an acknowledged feature of these treatments (Harris et al., 2001; Pradhan et al., 2015; Bhardwaj et al., 2021), it also represents a major drawback for designing efficient and universal protocols as it delays the search for common hallmarks of seed quality (Pagano et al., 2023). This was reported also in rice where a recent study investigated the genetic diversity of hydropriming applied under different soil moisture conditions (Nakao et al., 2020). The authors considered 27 genotypes, including O. sativa and O. glaberrima, representative of either wetland or dryland conditions, and reported wide genotypic differences in priming efficiency with the upland rice showing better responsiveness (Nakao et al., 2020). In our study, the application of integrative PCA further reinforced the genotype-dependent effect while also allowing to attribute each variety to the specific genetic clades that take into consideration the main breeding trends (Mongiano et al., 2018); To this purpose the G2 clade includes Carnaroli and Lomello, G4 includes Apollo, and G5 includes Cerere and Unico. Importantly, under PEG-induced stress, it was possible to also distinguish tolerant (e.g., Lomello) and sensitive (Carnaroli, Cerere, Apollo) or highly sensitive (Unico) varieties. When semiquantitative measurement of Fe and H2O2 levels were carried out in seedlings, the genotype-specific responses to water stress and priming treatments were further reinforced. The fact that H2O2 has not accumulated in stressed seedlings when priming was applied further supports the protective effect of the proposed treatments. This is in agreement with other studies carried out in rice showing that different priming techniques (hydropriming, application of selenium or salicylic acid) were able to lower ROS production under heavy metal pollution and nutrient starvation, leading to improved germination (Khan et al., 2020).
A schematic representation of the response to PEG-induces stress and the effect of seed priming treatments developed in this study is provided in Figure 9, taking into consideration the phenotyping and molecular analyses. The data pinpoints the clade distinction between the five popular rice Italian varieties along with their sensitivity/tolerance to water deprivation stress, and their responsiveness to priming. Differences observed among the varieties may be related to their seed type and breeding programs. Carnaroli, a variety obtained through hybridization and part of the G2 clade, is characterized by long grains. Cerere, a variety obtained through mutagenesis and part of the G5 clade, is characterized by the presence of short oval grains (Mongiano et al., 2018). Our study shows that both varieties are sensitive to PEG-induced stress while enhanced tolerance is gained after priming, possibly because it differentially modulates the expression of genes involved in the seed stress response.
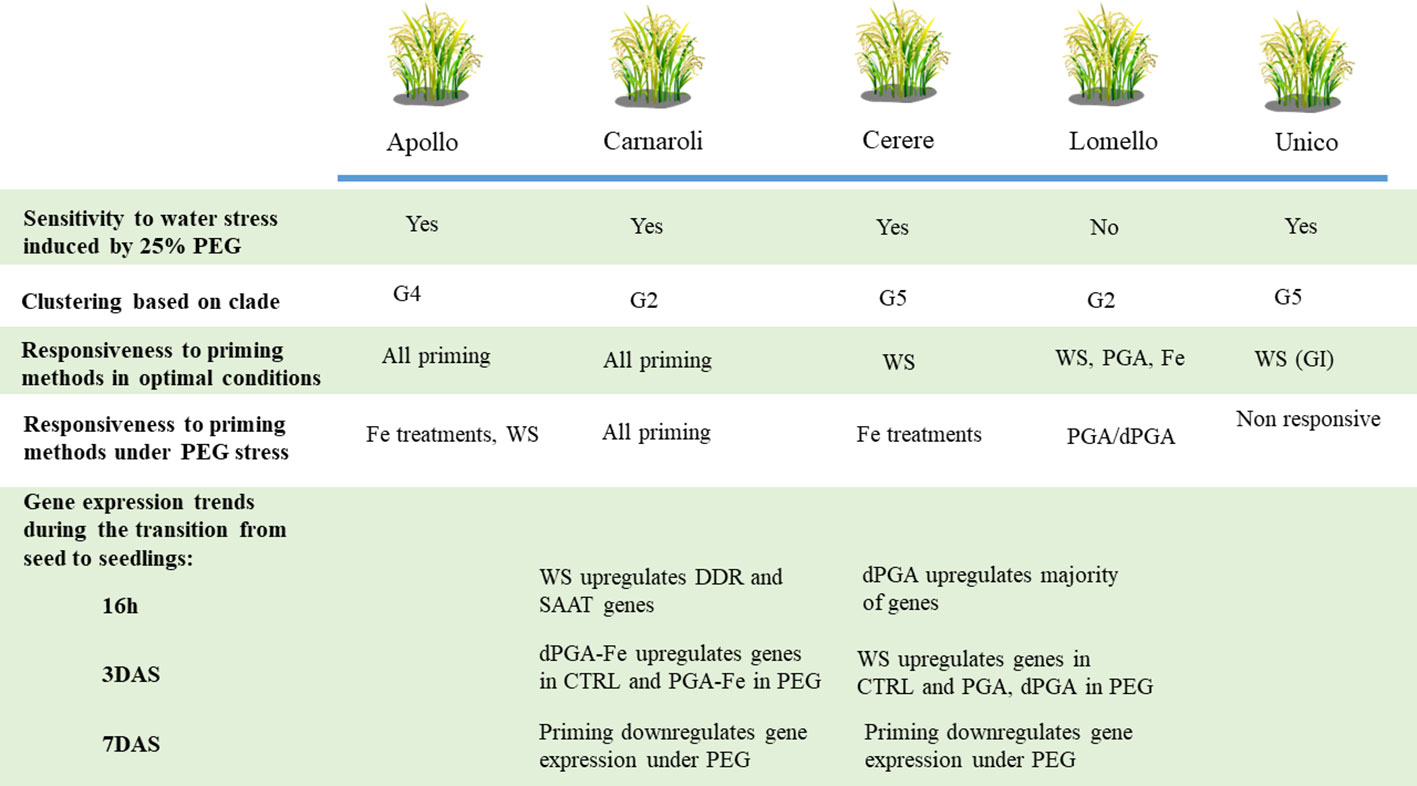
Figure 9 Overview of the main results obtained in the current study. The sensitivity/tolerance to water deprivation stress and responsiveness to priming treatments is considered for each investigated variety. Varietal clustering based on genetic clades is provided. Gene expression trends during the transition from seed to seedling are provided for the Carnaroli and Cerere varieties.
To further explore the genotype-dependent response to priming, a targeted gene expression profile study was carried out on Carnaroli and Cerere varieties particularly focusing on the transition from seed to seedlings. A panel of genes was investigated to cover processes related to DDR, antioxidant defense, Fe/Zn homeostasis and stress response. Among the genes associated with DDR, NBS1 (Nijmegen breakage syndrome 1 mutated gene), is a component of the MRN (MRE11-RAD50-NBS1) double-strand breaks sensor complex, while RAD51 is directly involved in HR (homologous recombination) (Banerjee and Roy, 2021). Additional genes involved in BER (Base Excision Repair) were also selected as this type of repair pathway is often associated with damage induced by accumulation of ROS (Roldán-Arjona and Ariza, 2009). The FPG (formamidopyrimidine-DNA glycosylase) gene, encoding for a DNA repair enzyme that excises oxidized purines from damaged DNA, and the OGG1 (8-oxoguanine glycosylase) gene, involved in the removal of 8-oxoG products, were proven to be highly induced during seed imbibition in multiple species (Macovei et al., 2011; Forti et al., 2021; Kowalik and Groszyk, 2023). The two TDP1 (tyrosyl-DNA phosphodiesterase 1) genes, TDP1α and TDP1β, encoding enzymes involved in hydrolyzing phosphodiester bond formed between topoisomerase I and the 3’-DNA, were proved to be highly active during seed imbibition as well as seedling growth under stress (Macovei et al., 2010; Gualtieri et al., 2021). PARP1 (poly-(ADP-ribose) polymerase 1) gene plays an important role in mediating the response to DNA damage during gametophyte development (Banerjee and Roy, 2021). The LigVI (DNA ligase VI) gene is a plant-specific ligase essential for DNA ligase-mediated rejoining of single- and double-strand breaks, previously identified as a major determinant of seed quality in A. thaliana (Waterworth et al., 2010). Antioxidant-related genes, such as APX6, encoding for an ascorbate peroxidase isoform found in the mitochondria which participates in H2O2 detoxification, was shown to have a more stable expression compared with other isoforms (Rossatto et al., 2017). The AOX1b gene encodes an alternative oxidase known to be induced by several stresses, including oxidative stress caused by H2O2 exposure (Feng et al., 2009). The superoxide dismutases SOD-Fe and SOD-Cu/Zn were proven to be highly expressed in rice during salt and/or osmotic stress (Rosatto et al., 2017). For Fe/Zn genes, NAS1 (nicotianamine synthase 1) is the gene related to Fe and Zn uptake and transport and it is highly expressed during the early stage of germination in rice (Nozoye et al., 2007). The NRAMP1 (natural resistance-associated macrophage protein 1) gene is also associated with metal transport, being part of an important protein transporter family, highly expressed during germination (Nozoye et al., 2007). The OPT7 (iron deficiency-regulated oligopeptide transporter 7) gene is associated with transport of ferrous ions bound to nicotianamine (Bashir et al., 2015). Another metal transporter coding gene is VIT1 (vacuolar iron transporter), associated with Fe accumulation and reallocation during seed development and germination (Eroglu et al., 2017). Among other stress-related genes, C3H50 (Cystenin3-Histidine50) encodes a zinc finger protein, highly induced by drought in rice via post-transcription regulation of messenger RNAs (Seong et al., 2020). This gene showed the highest expression among various C3H orthologs, especially in roots during drought stress, maybe because it has the highest number of drought-responsive elements (DRE). The AAP1 (amino acid transporter protein 1) gene, encoding for a member of the AAT protein family, was shown to absorb and translocate a wide variety of amino acids (Ji et al., 2020). Therefore, the genes selected for this investigation cover broad aspects of the seed response to stress both during imbibition and seedling establishment. The data collected in this study indicate that these genes are differentially expressed in Carnaroli and Cerere both from the point of view of the temporal transition as well as considering the priming methods and stress treatment (Figure 9). When considering seed imbibition, the WS treatment mostly resulted in gene upregulation in Carnaroli, while in Cerere dPGA had the same effect. At the radicle protrusion stage (3 DAS), further differences were evidenced among the two varieties, as the investigated genes are highly expressed in Carnaroli while low expression values were obtained for Cerere under control conditions. Under PEG-induced stress, PGA-Fe and dPGA-Fe treatments had a greater influence on the gene expression in Carnaroli, while in Cerere mostly PGA and dPGA priming affected the expression of some genes. However, these differences subsided at later seedling development (7 DAS), when the majority of genes were downregulated by priming. This may indicate that, at a molecular level, the priming effect is fundamental during the early phases of gemination to boost seed germination and stress response.
Given the results obtained in this study, the proposed seed priming treatments can be envisioned as sustainable and versatile practices to help addressing the impact of water deprivation stress in rice. Considering the observed genotype-specific differences, further varieties should be screened and the priming treatments can be tested also in relation to other types of stress. Ultimately, filed trials should be envisioned to ascertain the effect of these priming methods on final yield under drought conditions.
Data availability statement
The original contributions presented in the study are included in the article/Supplementary Material. Further inquiries can be directed to the corresponding author.
Author contributions
CD: Data curation, Formal analysis, Investigation, Methodology, Validation, Writing – review & editing. AP: Data curation, Formal analysis, Validation, Writing – review & editing. CC: Formal analysis, Supervision, Visualization, Writing – review & editing. DS: Formal analysis, Methodology, Writing – review & editing. IS-L: Funding acquisition, Investigation, Resources, Visualization, Writing – review & editing. AB: Resources, Validation, Writing – review & editing. AM: Conceptualization, Data curation, Investigation, Supervision, Writing – original draft.
Funding
The author(s) declare financial support was received for the research, authorship, and/or publication of this article. IS-L, CD, and AM acknowledged funding received for Rice Research provided through the International Rice Research Institute (IRRI), Philippines. AM acknowledged the FRG (Fondo Ricerca Giovani) funds received through the University of Pavia from the Italian Ministry of Education and Research (MUR). CD was supported by a PhD scholarship received through the Dipartimenti di Eccellenza Program (2018-2022) - Department of Biology and Biotechnology ‘L. Spallanzani’, University of Pavia, provided by MUR.
Acknowledgments
The authors would like to acknowledge Dr. Carlo Minoia and his team at SA.PI.SE (Sardo Piemontese Sementi) Coop. Agricola (https://sapise.it/), as well as Prof. Graziano Rossi and Prof. Andrea Mondoni from the University of Pavia, Botanical Garden Plant Germplasm Bank (https://terraeambiente.dip.unipv.it/it/dipartimento/risorse/banca-del-germoplasma-vegetale), for kindly providing the seed materials used in this study.
Conflict of interest
The authors declare that the research was conducted in the absence of any commercial or financial relationships that could be construed as a potential conflict of interest.
The author(s) declared that they were an editorial board member of Frontiers, at the time of submission. This had no impact on the peer review process and the final decision.
Publisher’s note
All claims expressed in this article are solely those of the authors and do not necessarily represent those of their affiliated organizations, or those of the publisher, the editors and the reviewers. Any product that may be evaluated in this article, or claim that may be made by its manufacturer, is not guaranteed or endorsed by the publisher.
Supplementary material
The Supplementary Material for this article can be found online at: https://www.frontiersin.org/articles/10.3389/fpls.2024.1344383/full#supplementary-material
References
Ali, L. G., Nulit, R., Ibrahim, M. H., Yien, C. Y. S. (2021). Efficacy of KNO3, SiO2 and SA priming for improving emergence, seedling growth and antioxidant enzymes of rice (Oryza sativa), under drought. Sci. Rep. 11 (1), 3864. doi: 10.1038/s41598-021-83434-3
Bailly, C., El-Maarouf-Bouteau, H., Corbineau, F. (2008). From intracellular signaling networks to cell death: the dual role of reactive oxygen species in seed physiology. C.R. Biol. 331 (10), 806–814. doi: 10.1016/j.crvi.2008.07.022
Balestrazzi, A., Confalonieri, M., Macovei, A., Carbonera, D. (2011). Seed imbibition in Medicago truncatula Gaertn.: Expression profiles of DNA repair genes in relation to PEG-mediated stress. J. Plant Physiol. 168 (7), 706–713. doi: 10.1016/j.jplph.2010.10.008
Banerjee, S., Roy, S. (2021). An insight into understanding the coupling between homologous recombination mediated DNA repair and chromatin remodeling mechanisms in plant genome: an update. Cell Cycle 20 (18), 1760–1784. doi: 10.1080/15384101.2021.1966584
Bashir, K., Ishimaru, Y., Itai, R. N., Senoura, T., Takahashi, M., An, G., et al. (2015). Iron deficiency regulated OsOPT7 is essential for iron homeostasis in rice. Plant Mol. Biol. 88 (1-2), 165–176. doi: 10.1007/s11103-015-0315-0
Batlang, U., Baisakh, N., Ambavaram, M. M., Pereira, A. (2013). Phenotypic and physiological evaluation for drought and salinity stress responses in rice. Methods Mol. Biol. 956, 209–225. doi: 10.1007/978-1-62703-194-3_15
Bhandari, U., Gajurel, A., Khadka, B., Thapa, I., Chand, I., Bhatta, D., et al. (2023). Morpho-physiological and biochemical response of rice (Oryza sativa L.) to drought stress: A review. Heliyon 9 (3), e13744. doi: 10.1016/j.heliyon.2023.e13744
Bhardwaj, A., Sita, K., Sehgal, A., Bhandari, K., Kumar, S., Prasad, P. V. V., et al. (2021). Heat priming of lentil (Lens culinaris Medik.) seeds and foliar treatment with γ-aminobutyric acid (GABA), confers protection to reproductive function and yield traits under high-temperature stress environments. Int. J. Mol. Sci. 22, 5825. doi: 10.2135/cropsci2000.402436x
Counce, P. A., Keisling, T. C., Mitchell, A. J. (2000). A uniform, objective, and adaptive system for expressing rice development. Crop Sci. 40 (2), 436–443. doi: 10.2135/cropsci2000.402436x
Devika, O. S., Singh, S., Sarkar, D., Barnwal, P., Suman, J., Rakshit, A. (2021). Seed priming: a potential supplement in integrated resource management under fragile intensive ecosystems. Front. Sustain. Food Syst. 5. doi: 10.3389/fsufs.2021.654001
Dey, S., Paul, S., Nag, A., Banerjee, R., Gopal, G., Mukherjee, A., et al. (2021). Iron-pulsing, a novel seed invigoration technique to enhance crop yield in rice: A journey from lab to field aiming towards sustainable agriculture. Sci. Total Environ. 769, 144671. doi: 10.1016/j.scitotenv.2020
Ermoli, F., Bontà, V., Vitali, G., Calvio, C. (2021). SwrA as global modulator of the two-component system DegSU in Bacillus subtilis. Res. Microbiol 172 (6), 103877. doi: 10.1016/j.resmic.2021.103877
Eroglu, S., Giehl, R. F., Meier, B., Takahashi, M., Terada, Y., Ignatyev, K., et al. (2017). Metal tolerance protein 8 mediates manganese homeostasis and iron reallocation during seed development and germination. Plant Physiol. 174 (3), 1633–1647. doi: 10.1104/pp.16.01646
Farooq, M., Barsa, S. M., Wahid, A. (2006). Priming of field-sown rice seed enhances germination, seedling establishment, allometry and yield. Plant Growth Regul. 49, 285–294. doi: 10.1007/s10725-006-9138-y
Farooq, M. A., Zhang, X., Zafar, M. M., Ma, W., Zhao, J. (2021). Roles of reactive oxygen species and mitochondria in seed germination. Front. Plant Sci. 12. doi: 10.3389/fpls.2021.781734
Favreau, B., Gaal, C., Pereira de Lima, I., Droc, G., Roques, S., Sotillo, A., et al. (2023). A multi-level approach reveals key physiological and molecular traits in the response of two rice genotypes subjected to water deficit at the reproductive stage. Plant Environ. Interact. 4 (5), 229–257. doi: 10.1002/pei3.10121
Feng, H. Q., Li, H. Y., Sun, K. (2009). Enhanced expression of alternative oxidase genes is involved in the tolerance of rice (Oryza sativa L.) seedlings to drought stress. Z Naturforsch. CJ Biosci. 64 (9-10), 704–710. doi: 10.1515/znc-2009-9-1016
Forti, C., Ottobrino, V., Bassolino, L., Toppino, L., Rotino, G. L., Pagano, A., et al. (2020a). Molecular dynamics of pre-germinative metabolism in primed eggplant (Solanum melongena L.) seeds. Hortic. Res. 7 (1), 87. doi: 10.1038/s41438-020-0310-8
Forti, C., Shankar, A., Singh, A., Balestrazzi, A., Prasad, V., Macovei, A. (2020b). Hydropriming and biopriming improve Medicago truncatula seed germination and upregulate DNA repair and antioxidant genes. Genes (Basel) 11 (3), 242. doi: 10.3390/genes11030242
Forti, C., Ottobrino, V., Doria, E., Bassolino, L., Toppino, L., Rotino, G. L., et al. (2021). Hydropriming applied on fast germinating Solanum villosum Miller seeds: Impact on pre-germinative metabolism. Front Plant Sci. 12, 639336. doi: 10.3389/fpls.2021.639336
Gerna, D., Roach, T., Arc, E., Stöggl, W., Limonta, M., Vaccino, P., et al. (2018). Redox poise and metabolite changes in bread wheat seeds are advanced by priming with hot steam. Biochem. J. 475 (23), 3725–3743. doi: 10.1042/BCJ20180632
Gualtieri, C., Gianella, M., Pagano, A., Cadeddu, T., Araújo, S., Balestrazzi, A., et al. (2021). Exploring microRNA signatures of DNA damage response using an innovative system of genotoxic stress in Medicago truncatula seedlings. Front. Plant Sci. 12. doi: 10.3389/fpls.2021.645323
Habib-Ur-Rahman, M., Ahmad, A., Raza, A., Hasnain, M. U., Alharby, H. F., Alzahrani, Y. M., et al. (2022). Impact of climate change on agricultural production; Issues, challenges, and opportunities in Asia. Front. Plant Sci. 13. doi: 10.3389/fpls.2022.925548
Han, C., Yang, P. (2015). Studies on the molecular mechanisms of seed germination. Proteomics 15 (10), 1671–1679. doi: 10.1002/pmic.201400375
Harris, D., Pathan, A. K., Gothkar, P., Joshi, A., Chivasa, W., Nyamudeza, P. (2001). On-farm seed priming: using participatory methods to revive and refine a key technology. Agric. Syst. 69, 151–164. doi: 10.1016/S0308-521X(01)00023-3
He, H., Serraj, R. (2012). Involvement of peduncle elongation, anther dehiscence and spikelet sterility in upland rice response to reproductive-stage drought stress. Environm. Exp. Bot. 75, 120–127. doi: 10.1016/j.envexpbot.2011.09.004
Holdsworth, M. J., Finch-Savage, W. E., Grappin, P., Job, D. (2008). Post-genomics dissection of seed dormancy and germination. Trends Plant Sci. 13 (1), 7–13. doi: 10.1016/j.tplants.2007.11.002
Howell, K. A., Narsai, R., Carroll, A., Ivanova, A., Lohse, M., Usadel, B., et al. (2009). Mapping metabolic and transcript temporal switches during germination in rice highlights specific transcription factors and the role of RNA instability in the germination process. Plant Physiol. 149, 961–980. doi: 10.1104/pp.108.129874
Ishibashi, Y., Yuasa, T., Iwaya-Inoue, M. (2018). Mechanisms of maturation and germination in crop seeds exposed to environmental stresses with a focus on nutrients, water status, and reactive oxygen species. Adv. Exp. Med. Biol. 1081, 233–257. doi: 10.1007/978-981-13-1244-1_13
Islam, M. M., Kayesh, E., Zaman, E., Urmi, T. A., Haque, M. M. (2018). Evaluation of rice (Oryza sativa L.) genotypes for drought tolerance at germination and early seedling stage. Agriculturists 16 (1), 44–54. doi: 10.3329/agric.v16i1.37533
Jain, M., Nijhawan, A., Tyagi, A. K., Khurana, J. P. (2006). Validation of housekeeping genes as internal control for studying gene expression in rice by quantitative real-time PCR. Biochem. Biophys. Res. Commun. 345 (2), 646–651. doi: 10.1016/j.bbrc.2006.04.140
Ji, Y., Huang, W., Wu, B., Fang, Z., Wang, X. (2020). The amino acid transporter AAP1 mediates growth and grain yield by regulating neutral amino acid uptake and reallocation in Oryza sativa. J. Exp. Bot. 71 (16), 4763–4777. doi: 10.1093/jxb/eraa256
Kader, M. A. (2005). A comparison of seed germination calculation formulae and the associated interpretation of resulting data. J. Proc. - R. Soc New SOouth Wales 138, 65–75. doi: 10.5962/p.361564
Kawaguchi, R., Suriyasak, C., Matsumoto, R., Sawada, Y., Sakai, Y., Hamaoka, N., et al. (2023). Regulation of reactive oxygen species and phytohormones in osmotic stress tolerance during seed germination in indica rice. Front. Plant Sci. 14. doi: 10.3389/fpls.2023.1186960
Khan, F., Hussain, S., Khan, S., Geng, M. (2020). Seed priming improved antioxidant defense system and alleviated Ni-induced adversities in rice seedlings under N, P, or K deprivation. Front. Plant Sci. 11. doi: 10.3389/fpls.2020.565647
Khan, S., Ibrar, D., Bashir, S., Rashid, N., Hasnain, Z., Nawaz, M., et al. (2022). Application of Moringa leaf extract as a seed priming agent enhances growth and physiological attributes of rice seedlings cultivated under water deficit regime. Plants (Basel). 11 (3), 261. doi: 10.3390/plants11030261
Kimura, K., Tran, L. S. P., Uchida, I., Itoh, Y. (2004). Characterization of Bacillus subtilis γ-glutamyltransferase and its involvement in the degradation of capsule poly-γ-glutamate. Microbiol 150 (12), 4115–4123. doi: 10.1099/mic.0.27467-0
Kota, S., Vispo, N. A., Quintana, M. R., Cabral, C. L. U., Centeno, C. A., Egdane, J., et al. (2023). Development of a phenotyping protocol for combined drought and salinity stress at seedling stage in rice. Front. Plant Sci. 14. doi: 10.3389/fpls.2023.1173012
Kowalik, S., Groszyk, J. (2023). Profiling of barley, wheat, and rye FPG and OGG1 genes during grain germination. Int. J. Mol. Sci. 24 (15), 12354. doi: 10.3390/ijms241512354
Kranner, I., Minibayeva, F. V., Beckett, R. P., Seal, C. E. (2010). What is stress? Concepts, definitions and applications in seed science. New Phytol. 188 (3), 655–673. doi: 10.1111/j.1469-8137.2010.03461.x
Kruthika, N., Jithesh, M. N. (2023). Morpho-physiological profiling of rice (Oryza sativa) genotypes at germination stage with contrasting tolerance to salinity stress. J. Plant Res. 136 (6), 907–930. doi: 10.1007/s10265-023-01491-3
Lou, D., Lu, S., Chen, Z., Lin, Y., Yu, D., Yang, X. (2023). Molecular characterization reveals that OsSAPK3 improves drought tolerance and grain yield in rice. BMC Plant Biol. 23 (1), 53. doi: 10.1186/s12870-023-04071-8
Macovei, A., Balestrazzi, A., Confalonieri, M., Carbonera, D. (2010). The tyrosyl-DNA phosphodiesterase gene family in Medicago truncatula Gaertn: bioinformatic investigation and expression profiles in response to copper- and PEG-mediated stress. Planta 232 (2), 393–407. doi: 10.1007/s00425-010-1179-9
Macovei, A., Balestrazzi, A., Confalonieri, M., Faé, M., Carbonera, D. (2011). New insights on the barrel medic MtOGG1 and MtFPG functions in relation to oxidative stress response in planta and during seed imbibition. Plant Physiol. Biochem. 49 (9), 1040–1050. doi: 10.1016/j.plaphy.2011.05.007
Macovei, A., Pagano, A., Leonetti, P., Carbonera, D., Balestrazzi, A., Araújo, S. S. (2017). Systems biology and genome-wide approaches to unveil the molecular players involved in the pre-germinative metabolism: implications on seed technology traits. Plant Cell Rep. 36 (5), 669–688. doi: 10.1007/s00299-016-2060-5
Mahmood, T., Iqbal, M. S., Li, H., Nazir, M. F., Khalid, S., Sarfraz, Z., et al. (2022). Differential seedling growth and tolerance indices reflect drought tolerance in cotton. BMC Plant Biol. 22 (1), 331. doi: 10.1186/s12870-022-03724-4
Marthandan, V., Geetha, R., Kumutha, K., Renganathan, V. G., Karthikeyan, A., Ramalingam, J. (2020). Seed priming: a feasible strategy to enhance drought tolerance in crop plants. Int. J. Mol. Sci. 21 (21), 8258. doi: 10.3390/ijms21218258
Massaiu, I., Pasotti, L., Sonnenschein, N., Rama, E., Cavaletti, M., Magni, P., et al. (2019). Integration of enzymatic data in Bacillus subtilis genome-scale metabolic model improves phenotype predictions and enables in silico design of poly-γ-glutamic acid production strains. Microb. Cell Fact. 18 (1), 1–20. doi: 10.1186/s12934-018-1052-2
Mongiano, G., Titone, P., Tamborini, L., Pilu, R., Bregaglio, S. (2018). Evolutionary trends and phylogenetic association of key morphological traits in the Italian rice varietal landscape. Sci. Rep. 8 (1), 13612. doi: 10.1038/s41598-018-31909-1
Nakao, Y., Sone, C., Sakagami, J. I. (2020). Genetic diversity of hydro priming effects on rice seed emergence and subsequent growth under different moisture conditions. Genes (Basel) 11 (9), 994. doi: 10.3390/genes11090994
Nozoye, T., Inoue, H., Takahashi, M., Ishimaru, Y., Nakanishi, H., Mori, S., et al. (2007). The expression of iron homeostasis-related genes during rice germination. Plant Mol. Biol. 64, 35–47. doi: 10.1007/s11103-007-9132-4
Ogunleye, A., Bhat, A., Irorere, V. U., Hill, D., Williams, C., Radecka, I. (2015). Poly-γ-glutamic acid: production, properties and applications. Microbiol 161 (Pt 1), 1–17. doi: 10.1099/mic.0.081448-0
Oladosu, Y., Rafii, M. Y., Samuel, C., Fatai, A., Magaji, U., Kareem, I., et al. (2019). Drought resistance in rice from conventional to molecular breeding: a review. Int. J. Mol. Sci. 20 (14), 3519. doi: 10.3390/ijms20143519
Osakabe, Y., Osakabe, K., Shinozaki, K., Tran, L. S. (2014). Response of plants to water stress. Front. Plant Sci. 5. doi: 10.3389/fpls.2014.00086
Osera, C., Amati, G., Calvio, C., Galizzi, A. (2009). SwrAA activates poly-γ-glutamate synthesis in addition to swarming in Bacillus subtilis. Microbiol 155 (7), 2282–2287. doi: 10.1099/mic.0.026435-0
Pagano, A., Araújo, S. S., Macovei, A., Leonetti, P., Balestrazzi, A. (2017). The seed repair response during germination: Disclosing correlations between DNA repair, antioxidant response, and chromatin remodeling in Medicago truncatula. Front. Plant Sci. 8. doi: 10.3389/fpls.2017.01972
Pagano, A., de Sousa Araújo, S., Macovei, A., Dondi, D., Lazzaroni, S., Balestrazzi, A. (2019). Metabolic and gene expression hallmarks of seed germination uncovered by sodium butyrate in Medicago truncatula. Plant Cell Environ. 42 (1), 259–269. doi: 10.1111/pce.13342
Pagano, A., Folini, G., Pagano, P., Sincinelli, F., Rossetto, A., Macovei, A., et al. (2022). ROS accumulation as a hallmark of dehydration stress in primed and overprimed Medicago truncatula seeds. Agronomy 12, 268. doi: 10.3390/agronomy12020268
Pagano, A., Macovei, A., Balestrazzi, A. (2023). Molecular dynamics of seed priming at the crossroads between basic and applied research. Plant Cell Rep. 42 (4), 657–688. doi: 10.1007/s00299-023-02988-w
Panda, D., Mishra, S. S., Behera, P. K. (2021). Drought tolerance in rice: focus on recent mechanisms and approaches. Rice Sci. 28 (2), 119–132. doi: 10.1016/j.rsci.2021.01.002
Paparella, S., Araújo, S. S., Rossi, G., Wijayasinghe, M., Carbonera, D., Balestrazzi, A. (2015). Seed priming: state of the art and new perspectives. Plant Cell Rep. 34, 1281–1293. doi: 10.1007/s00299-015-1784-y
Pérez-Fernández, M. A., Calvo-Magro, E., Ferrer-Castán, D. (2006). Simulation of germination of pioneer species along an experimental drought gradient. J. Environm. Biol. 27 (4), 679–685.
Pradhan, N., Prakash, P., Manimurugan, C., Tiwari, S. K., Sharma, R., Singh., P. (2015). Screening of tomato genotypes using osmopriming with PEG 6000 under salinity stress. Res. Environ. Life Sci. 8, 245–250.
Rajjou, L., Duval, M., Gallardo, K., Catusse, J., Bally, J., Job, C., et al. (2012). Seed germination and vigor. Annu. Rev. Plant Biol. 63, 507–533. doi: 10.1146/annurev-arplant-042811-105550
Ranal, M. A., Santana, D. G. D. (2006). How and why to measure the germination process? Braz. J. Bot. 29, 1–11. doi: 10.1590/S0100-84042006000100002
Rang, Z. W., Jagadish, S. V. K., Zhou, Q. M., Craufurd, P. Q., Heuer, S. (2011). Effect of high temperature and water stress on pollen germination and spikelet fertility in rice. Environm. Exp. Bot. 70 (1), 58–65. doi: 10.1016/j.envexpbot.2010.08.009
Reed, R. C., Bradford, K. J., Khanday, I. (2022). Seed germination and vigor: ensuring crop sustainability in a changing climate. Heredity (Edinb) 128 (6), 450–459. doi: 10.1038/s41437-022-00497-2
Roldán-Arjona, T., Ariza, R. R. (2009). Repair and tolerance of oxidative DNA damage in plants. Mutat. Res. 681 (2-3), 169–179. doi: 10.1016/j.mrrev.2008.07.003
Rossatto, T., do Amaral, M. N., Benitez, L. C., Vighi, I. L., Braga, E. J. B., de Magalhães Júnior, A. M., et al. (2017). Gene expression and activity of antioxidant enzymes in rice plants, cv. BRS AG, under saline stress. Physiol. Mol. Biol. Plants. 23, 865–875. doi: 10.1007/s12298-017-0467-2
Rout, G. R., Sahoo, S. (2015). Role of iron in plant growth and metabolism. Rev. Agric. Sci. 3, 1–24. doi: 10.7831/ras.3.1
Samota, M. K., Sasi, M., Awana, M., Yadav, O. P., Amitha Mithra, S. V., Tyagi, A., et al. (2017). Elicitor-induced biochemical and molecular manifestations to improve drought tolerance in rice (Oryza sativa L.) through seed-priming. Front. Plant Sci. 8. doi: 10.3389/fpls.2017.00934
Sano, N., Lounifi, I., Cueff, G., Collet, B., Clément, G., Balzergue, S., et al. (2022). Multi-omics approaches unravel specific features of embryo and endosperm in rice seed germination. Front. Plant Sci. 13. doi: 10.3389/fpls.2022.867263
Sano, N., Permana, H., Kumada, R., Shinozaki, Y., Tanabata, T., Yamada, T., et al. (2012). Proteomic analysis of embryonic proteins synthesized from long-lived mRNAs during germination of rice seeds. Plant Cell Physiol. 53, 687–698. doi: 10.1093/pcp/pcs024
Seifikalhor, M., Aliniaeifard, S., Hassani, B., Niknam, V., Lastochkina, O. (2019). Diverse role of γ-aminobutyric acid in dynamic plant cell responses. Plant Cell Rep. 38 (8), 847–867. doi: 10.1007/s00299-019-02396-z
Seong, S. Y., Shim, J. S., Bang, S. W., Kim, J. K. (2020). Overexpression of OsC3H10, a CCCH-zinc finger, improves drought tolerance in rice by regulating stress-related genes. Plants 9 (10), 1298. doi: 10.3390/plants9101298
Shahzad, A., Ullah, S., Dar, A. A., Sardar, M. F., Mehmood, T., Tufail, M. A., et al. (2021). Nexus on climate change: agriculture and possible solution to cope future climate change stresses. Environ. Sci. Pollut. Res. Int. 28 (12), 14211–14232. doi: 10.1007/s11356-021-12649-8
Shobhana, V. G., Senthil, N., Kalpana, K., Abirami, B., Sangeetha, J., Saranya, B., et al. (2013). Comparative studies on the iron and zinc contents estimation using atomic absorption spectrophotometer and grain staining techniques (PRussian Blue and DTZ) in maize germplasms. J. Plant Nutr. 36 (2), 329–342. doi: 10.1080/01904167.2012.744419
Sobahan, M. A., Akter, N., Rana, M. M. (2022). Polyethylene glycol mediated drought stress impacts on germination, growth and accumulation of proline in rice (Oryza sativa L.). SAARC Journal of Agriculture 20 (1), 107–119. doi: 10.3329/sja.v20i1.60544
Song, D. Y., Reddy, L. V., Charalampopoulos, D., Wee, Y. J. (2019). Poly-(γ-glutamic acid) production and optimization from agro-industrial bioresources as renewable substrates by Bacillus sp. FBL-2 through response surface methodology. Biomolecules. 9 (12), 754. doi: 10.3390/biom9120754
Sperotto, R. A., Ricachenevsky, F. K., de Abreu Waldow, V., Fett, J. P. (2012). Iron biofortification in rice: it’s a long way to the top. Plant Sci. 190, 24–39. doi: 10.1016/j.plantsci.2012.03.004
Stanley, C. B., Strey, H. H. (2003). Measuring osmotic pressure of poly (ethylene glycol) solutions by sedimentation equilibrium ultracentrifugation. Macromolecules 36 (18), 6888–6893. doi: 10.1021/ma034079e
Székely, Á., Szalóki, T., Lantos, C., Pauk, J., Jancsó, M. (2023). Data of germination ability of tetraploid rice lines under multiple stress factors. Data Brief. 48, 109235. doi: 10.1016/j.dib.2023.109235
Tang, B., Lei, P., Xu, Z., Jiang, Y., Xu, Z., Liang, J., et al. (2015). Highly efficient rice straw utilization for poly-(γ-glutamic acid) production by Bacillus subtilis NX-2. Bioresour. Technol. 193, 370–376. doi: 10.1016/j.biortech.2015.05.110
Thomsen, R., Sølvsten, C. A. E., Linnet, T. E., Blechingberg, J., Nielsen, A. L. (2010). Analysis of qPCR data by converting exponentially related Ct values into linearly related X0 values. J. Bioinform. Comput. Biol. 8 (5), 885–900. doi: 10.1142/s0219720010004963
Wang, X., Liu, H., Yu, F., Hu, B., Jia, Y., Sha, H., et al. (2019). Differential activity of the antioxidant defense system and alterations in the accumulation of osmolyte and reactive oxygen species under drought stress and recovery in rice (Oryza sativa L.) tillering. Sci. Rep. 9 (1), 8543. doi: 10.1038/s41598-019-44958-x
Wang, W., Zhang, C., Zheng, W., Lv, H., Li, J., Liang, B., et al. (2022). Seed priming with protein hydrolysate promotes seed germination via reserve mobilization, osmolyte accumulation and antioxidant systems under PEG-induced drought stress. Plant Cell Rep. 41 (11), 2173–2186. doi: 10.1007/s00299-022-02914-6
Waqas Mazhar, M., Ishtiaq, M., Hussain, I., Parveen, A., Hayat Bhatti, K., Azeem, M., et al. (2022). Seed nano-priming with Zinc Oxide nanoparticles in rice mitigates drought and enhances agronomic profile. PloS One 17 (3), e0264967. doi: 10.1371/journal.pone.0264967
Waterworth, W. M., Bray, C. M., West, C. E. (2015). The importance of safeguarding genome integrity in germination and seed longevity. J. Exp. Bot. 66 (12), 3549–3558. doi: 10.1093/jxb/erv080
Waterworth, W. M., Bray, C. M., West, C. E. (2019). Seeds and the art of genome maintenance. Front. Plant Sci. 10. doi: 10.3389/fpls.2019.00706
Waterworth, W. M., Masnavi, G., Bhardwaj, R. M., Jiang, Q., Bray, C. M., West, C. E. (2010). A plant DNA ligase is an important determinant of seed longevity. Plant J. 63 (5), 848–860. doi: 10.1111/j.1365-313X.2010.04285.x
Waterworth, W. M., Latham, R., Wang, D., Alsharif, M., West, C. E. (2022). Seed DNA damage responses promote germination and growth in Arabidopsis thaliana. Proc. Nat. Acad. Sci. 119 (30), e2202172119. doi: 10.1073/pnas.2202172119
Xu, Z., Ma, J., Lei, P., Wang, Q., Feng, X., Xu, H. (2020). Poly-γ-glutamic acid induces system tolerance to drought stress by promoting abscisic acid accumulation in Brassica napus L. Sci. Rep. 10 (1), 252. doi: 10.1038/s41598-019-57190-4
Xu, Z., Wan, C., Xu, X., Feng, X., Xu, H. (2013). Effect of poly (γ-glutamic acid) on wheat productivity, nitrogen use efficiency and soil microbes. Acta Agric. Scand. B Soil Plant Sci. 13 (3), 744–755. doi: 10.1080/09064710.2013.849752
Yang, P., Li, X., Wang, X., Chen, H., Chen, F., Shen, S. (2007). Proteomic analysis of rice (Oryza sativa) seeds during germination. Proteomics 7, 3358–3368. doi: 10.1002/pmic.200700207
Yin, A., Jia, Y., Qiu, T., Gao, M., Cheng, S., Wang, X., et al. (2018). Poly-γ-glutamic acid improves the drought resistance of maize seedlings by adjusting the soil moisture and microbial community structure. Appl. Soil Ecol. 129, 128–135. doi: 10.1016/j.apsoil.2018.05.008
Yu, F., Li, M., He, D., Yang, P. (2021). Advances on post-translational modifications involved in seed germination. Front. Plant Sci. 12. doi: 10.3389/fpls.2021.642979
Yu, Y., Yan, F., Chen, Y., Jin, C., Guo, J. H., Chai, Y. (2016). Poly-γ-glutamic acids contribute to biofilm formation and plant root colonization in selected environmental isolates of Bacillus subtilis. Front. Microbiol. 7. doi: 10.3389/fmicb.2016.01811
Keywords: poly-gamma glutamic acid (γ-PGA), polyethylene glycol (PEG), iron pulsing, seed germination, gene expression, antioxidant response, DNA damage response
Citation: Dueñas C Jr, Pagano A, Calvio C, Srikanthan DS, Slamet-Loedin I, Balestrazzi A and Macovei A (2024) Genotype-specific germination behavior induced by sustainable priming techniques in response to water deprivation stress in rice. Front. Plant Sci. 15:1344383. doi: 10.3389/fpls.2024.1344383
Received: 25 November 2023; Accepted: 22 January 2024;
Published: 08 February 2024.
Edited by:
Zhou Li, Sichuan Agricultural University, ChinaReviewed by:
Lei Wang, Chinese Academy of Sciences (CAS), ChinaSzilvia Veres, University of Debrecen, Hungary
Copyright © 2024 Dueñas, Pagano, Calvio, Srikanthan, Slamet-Loedin, Balestrazzi and Macovei. This is an open-access article distributed under the terms of the Creative Commons Attribution License (CC BY). The use, distribution or reproduction in other forums is permitted, provided the original author(s) and the copyright owner(s) are credited and that the original publication in this journal is cited, in accordance with accepted academic practice. No use, distribution or reproduction is permitted which does not comply with these terms.
*Correspondence: Anca Macovei, YW5jYS5tYWNvdmVpQHVuaXB2Lml0