- 1Research, The Natural History Museum, London, United Kingdom
- 2Department of Ecosystem Stewardship, Royal Botanic Gardens Kew, London, United Kingdom
Introduction: Though used as the model liverwort in culture for several decades, the biology of Marchantia polymorpha subsp. ruderalis in nature has never been documented in detail in a single account.
Methods: Here we synthesize routine field observations documented with hundreds of images of M. ruderalis colonies (or groups) showing sex differentiation over 3 years on two populations of M. ruderalis after major heathland fires in 2020.
Results: Initial post-fire establishment is from airborne spores rather than a spore bank but thereafter spread is via gemmae which have less exacting germination requirements. Young sporelings are highly gemmiferous but gemmae production becomes less frequent after sex organ formation. Over the course of a year there are up to three waves of carpocephalum production with the overwhelming majority of antheridiophores appearing 2-3 months ahead of the archegoniophores though no differences in growth rates were apparent between male and female thalli. Spermatozoids are produced almost continuously throughout the year, whilst sporophyte maturation is restricted to the summer months.
Discussion: Because of the asynchrony between antheridiophore and archegoniophore production a 1:1 sex ratio is only apparent over this period. The spring months see an excess of males with more females in the summer. An almost 100% fertilization rate, with fertilization distances of up to 19 m far exceeding those in all other bryophytes, is attributed to vast spermatozoid production for most of the year, dispersal on surface oil films between thalli and highly effective intra-thallus spermatozoid transport via the pegged-rhizoid water-conducting system. Archegoniophores do develop on female-only populations but have shorter stalks than those where fertilization has occurred. Eventual disappearance post fires is attributed to a fall in topsoil nutrient levels preventing new sporeling establishment and competition from Ceratodon purpureus and Polytrichum spp. A major drought in the summer of 2022 almost wiped out the heathland Marchantia populations but all the other bryophytes survived.
Introduction
Marchantia polymorpha subsp. ruderalis Bischl. & Boissel. Dub. is a Circumboreal-temperate cosmopolitan liverwort found throughout Europe and in all continents except Antarctica (Blockeel et al., 2014). Other than describing it as a species of disturbed habitats, particularly after fires and man-made, where it can become invasive e.g., between paving stones in urban streets and in greenhouses, floras and field guides provide almost no information on its actual biology and longevity. Paton (1999) reports that sporophytes are uncommon but makes no mention of the time of year. Quoting from Duckett and Pressel (2009); Blockeel et al. (2014) state that carpocephala (gametangiophores) may be stimulated by long days and fairly high temperatures and that gemmae are always present. M. polymorpha subsp. polymorpha L. and subsp. montevagans Bischl. & Boissel. Dub. occur in more permanent habitats and appear to be less fertile than subsp. ruderalis (Paton, 1999).
For more than four centuries Marchantia polymorpha has figured as the archetypal liverwort in textbook descriptions (Figure 1A) (Gerarde, 1597). With wild material readily available, ease of cultivation and amenability to biochemical and molecular analyses it is unsurprising therefore that today Marchantia is the model liverwort (Shimamura, 2016; Bowman et al., 2022), alongside the hornwort Anthoceros agrestis (Szövényi et al., 2015; Frangedakis et al., 2021) and the mosses Ceratodon purpureus (Shaw and Gaughan, 1993; Kollar et al., 2021), Funaria hygrometrica and Physcomitrium patens (Wood et al., 2000). Wild collections are the usual starting point for advanced research programmes on these model species except for Physcomitrim patens where the Gransden strain, isolated from a single spore in 1959, continues to be widely used despite its reduced fertility (Rensing et al., 2020).
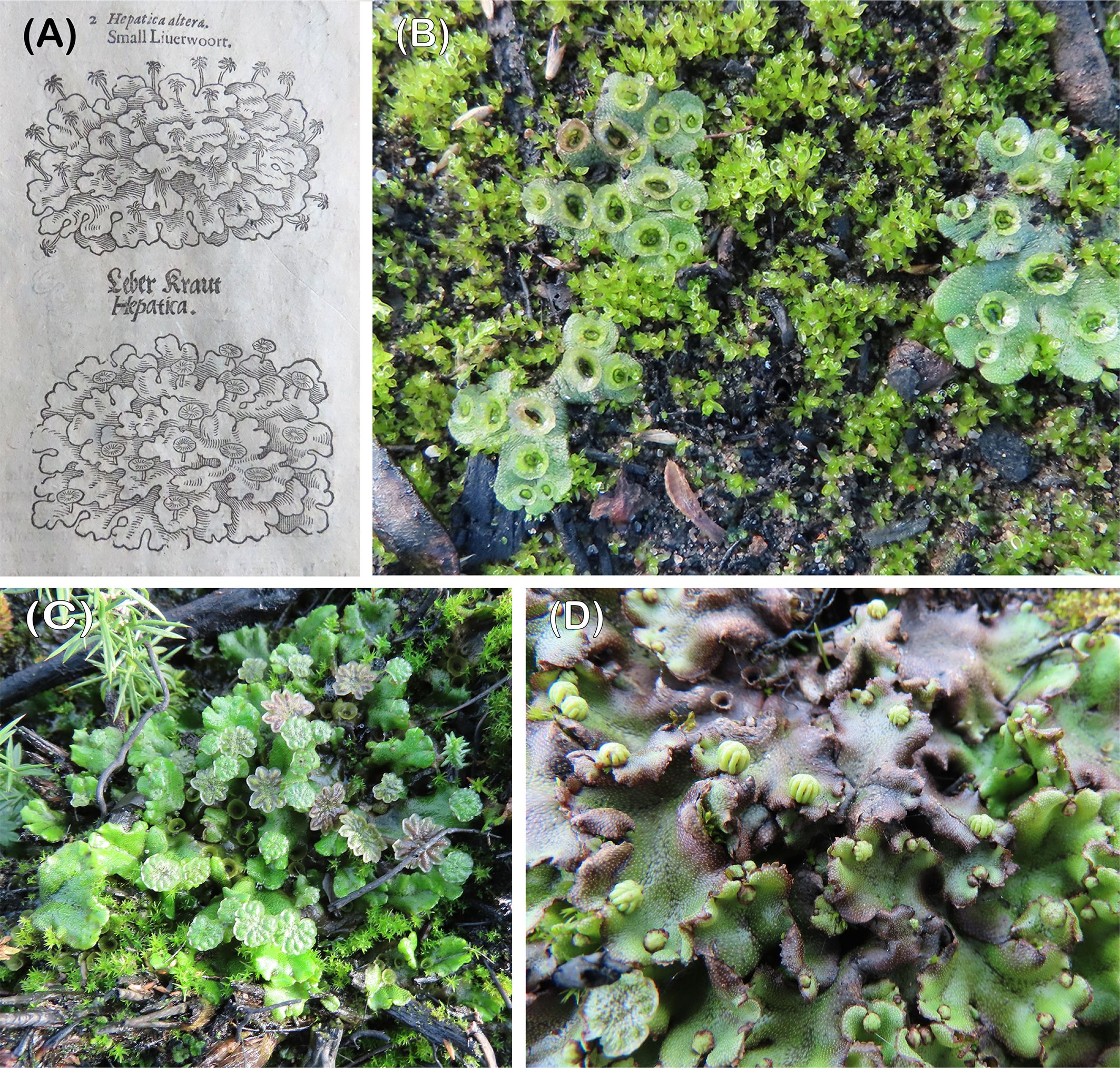
Figure 1 (A) One of the earliest illustrations of male and female Marchantia: Gerarde’s Herbal, 1597, (B) highly gemmiferous sporelings growing with young Funaria. Thursley, October 2020, (C) male colony with three generations of antheridiophores; old, mature and young. Thursley, December 2020, (D) young archegoniophores. Thursley, April 2021.
In striking contrast to the vast body of research on cultured plants, the behaviour of these bryophytes in nature remains very poorly documented except perhaps for the reproductive cycles of Funaria (Duckett and Pressel, 2022) and to a lesser extent of Ceratodon (Kollar et al., 2021; Duckett et al., 2023). The wild reproductive biology of Marchantia from initial establishment to its disappearance has never been set out systematically in a single account though snapshots of particular phases have been reported in popular articles e.g., remarkable fertilization distances (Pressel and Duckett, 2019), mammoth sporophyte production (Duckett and Pressel, 2009), asynchrony between antheridiophore and archegoniophore formation (Pressel et al., 2021; Duckett et al., 2023), a dramatic decline in gemmae production with thallus age (Duckett et al., 2023) and extinction following drought (Duckett et al., 2023). These reveal that the biology of Marchantia in nature is far more complicated than might appear at first sight.
Reported here is a synthesis of over three years of field data collected at approximately monthly intervals on Marchantia ruderalis following fires in the summer of 2020 at two lowland heaths in Southern England plus other incidental information on other populations across southern England. We hope that this will serve as a foundation reference work for future molecular studies.
Sites and methods
Thursley Common, National Nature Reserve and Chobham Common (both Sites of Special Scientific Interest) are major lowland heath nature reserves in southern England with extensive areas of open dry and wet heathland, peat bogs, pine and deciduous woodlands. They are renowned for dragonfly and bird diversity. Periodically they are subjected to major fires, the last prior those in 2020 being at Thursley in 2006 (Duckett et al., 2008). Post the fires the areas recolonized by Marchantia were previously dominated by Ericaceae; Calluna vulgaris and Erica cinerea on dry heath, Erica tetralix in wetter areas. Full species lists and details of post fire recolonization are given in Pressel et al. (2021) and Duckett et al. (2023), see also Figure 2.
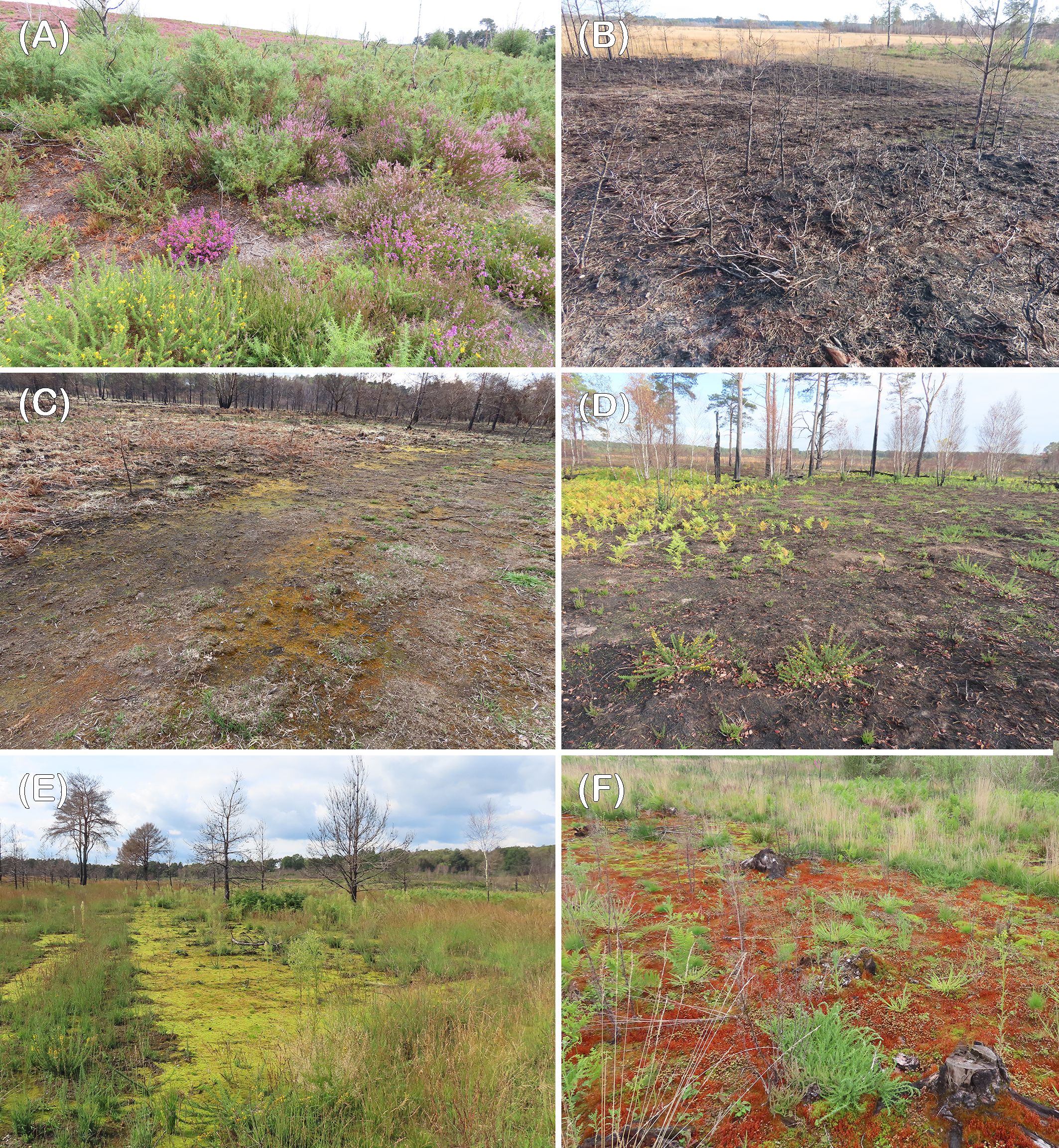
Figure 2 (A, B, D) Thursley, (C, E, F) Chobham. (A) Unburnt area with Erica, Calluna and Ulex sp., (B) Burnt area prior to recolonisation, December 2020, (C, D) later recolonisation stages showing different degrees of bryophyte coverage, late spring to autumn 2021, (E, F) almost continuous carpets of Funaria and Ceratodon, 2022.
Both sites have the same mild Atlantic climate. Monthly mean temperatures range from 18 degrees in the summer months down to 7 degrees in the winter. Monthly rainfall is between 65 and 85 mm throughout the year. There are light frosts (-1 to -5 degrees) between 30 and 45 days but almost no days when temperatures persist below zero for 24h. Apart from the short frosts and periods of up to 4 weeks without rain, in spring and summer, Marchantia grew continuously throughout the year at both study sites.
The sites were visited at approximately monthly intervals since the fires. On each visit over 100 images were taken of groups of Marchantia colonies. These were subsequently analysed for the numbers of male, females, and vegetative individuals. The presence of sporophytes on mature archegoniophores on at least 20 individuals was noted in the field.
More incidental observations were made on ten other Marchantia populations across southern England.
Observations and Discussion
Initial colonization
Marchantia first appeared at Thursley some 100 days after the 2020 fire as very numerous small thalli scattered over an area of several hectares. Only a small fraction of seemingly suitable burnt areas were colonized. It was very frequent in damper areas previously containing Erica tetralix and Molinia caerulea with an understory of Polytrichum species, Sphagnum compactum, Campylopus paradoxus and C. brevipilus but was absent in drier areas normally dominated by Calluna vulgaris and with Campyoplus introflexus and Cephaloziella divaricata.
Some of the 2020 burnt areas had also been affected by the earlier fire of 2006 (Duckett et al., 2008). However, after the 2006 fire Marchantia had a much wider and more even distribution than in 2020, enabling the measurement of fertilization distances (Pressel and Duckett, 2019). The most likely explanation for the more restricted distribution in 2020 was a dry period from April to June.
Long term spore survival in bryophytes is best documented in Sphagnum where viable spores can persist in situ for several decades (Clymo and Duckett, 1986; Sundberg and Rydin, 2000). There are numerous accounts of viability being retained for many years in herbarium specimens and the long term survival of moss spores, particularly of ephemeral and annual taxa (Furness and Hall, 1981; Jonsson, 1993). On the basis of extensive experimental data, During (1997) considers bryophyte spore banks to closely parallel those in seeds. We are not however aware of any studies specific to Marchantia and very few authors even mention liverworts. Van Zanten and Gradstein (1988), in their wide ranging appraisal of dispersal geography, follow Inoue (1961) by stating that Marchantia spores are likely to remain viable in situ for about a year or more whereas taxa that experience seasonal droughts (e.g., Fossombronia, Riccia) are likely to be much longer lived. Thus, we conclude that the post 2020 fire Marchantia at Thursley almost certainly came from airborne spores rather than those lying dormant in the soil since the previous fire in 2006. We now require measurements of the bryophyte spore rain at our two sites like those in Pettersson (1940).
Subsequent to the initial establishment event, new thalli grew clustered around existing ones with numbers declining radially with distance, in line with recorded distances of gemmae splash dispersal not exceeding 1.2 m (Stieha et al., 2014). This strongly suggests that secondary colonization, following initial establishment, occurred from gemmae rather than spores. A spore rain, either blown in or locally produced, should be excluded, as it would have produced a more uniform distribution. Establishment from spores appears to require much more exacting nutrient requirements e.g., high nitrogen as available immediately after fire, than those suitable for gemmae and is thus the most critical phase in the ecology of Marchantia. Needed now is precise information, particularly on soil surface N, P and K, post fires and comparisons with nutrient levels in culture media.
All the thalli on the two heaths were initially highly gemmiferous and vegetative (Figure 1B). Once the first gametangiophores began to appear 186 days from the fire, the thalli had become progressively less gemmiferous (Pressel et al., 2021). Our observations that urban pavement populations are invariably highly gemmiferous, point to similar proliferation via gemmae with sporeling establishment a much rarer event. In addition, the facts that these populations are almost always vegetative, or have one sex only, are further hallmarks of initial establishment from a single spore. On the other hand, single sex clusters of between 20 to over 200 individual plants after the 2006 (Pressel et al., 2021) and 2020 fires point to secondary origins from gemmae.
Sexual cycles
The first gametangiophores to develop in the winter months of 2020/21 were exclusively male (Figure 1C). Archegoniophores appeared nearly 2-3 months later (Figure 1D) with a further 2-3 months until spore dispersal in the summer (Figures 3A, C). 2021 saw two further cycles of sex organ production (Figures 3B, D) and this pattern was repeated in subsequent years at both sites. At all times except in midsummer (Figure 3C) antheridiophore and archegoniophore production are always out of step (Figures 3A, E). The summer cycles from archegoniophore initiation until spore dispersal were as low as 60 days to over double this time during the other seasons. These seasonal changes are summarised in Figures 4A, B.
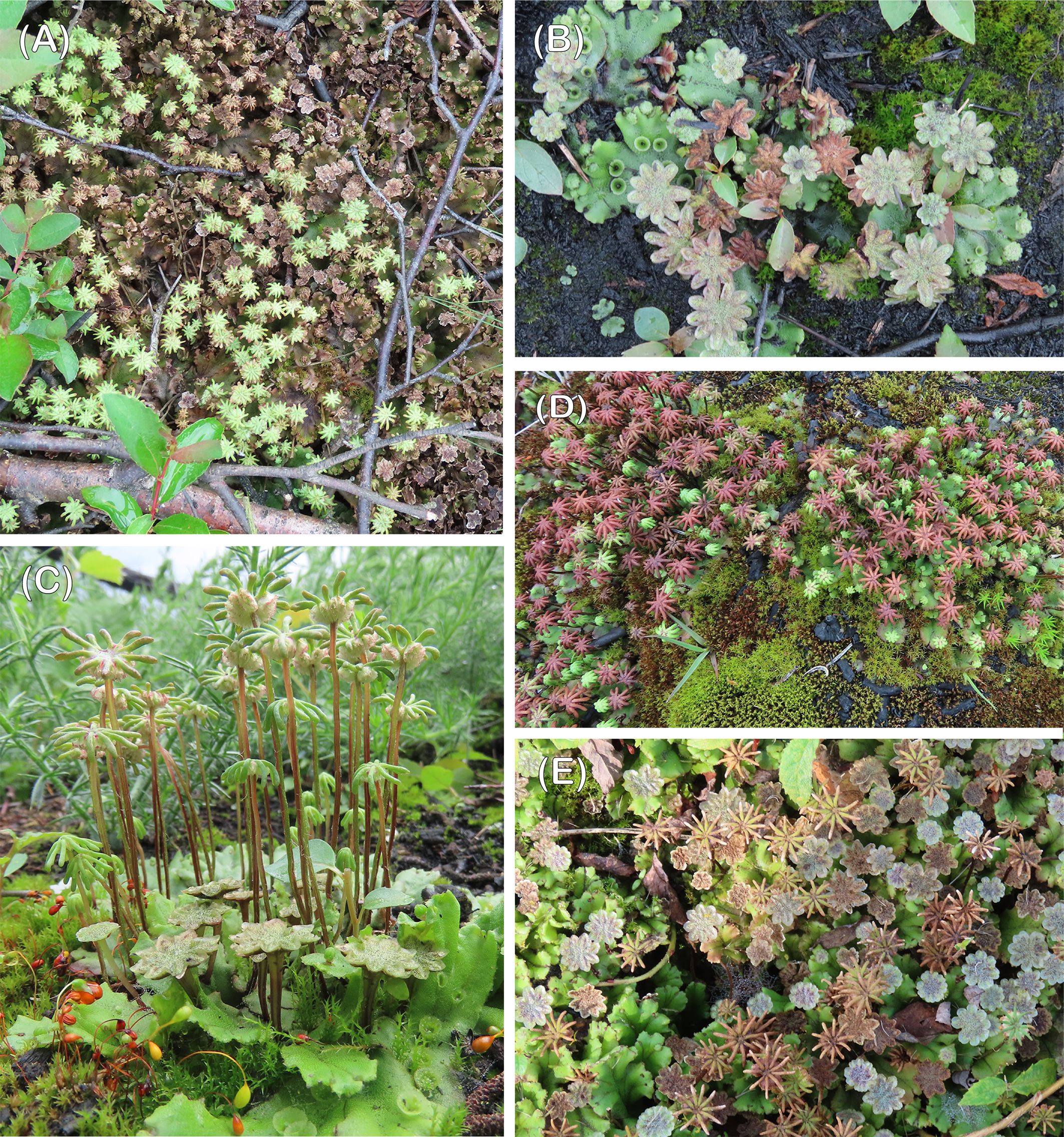
Figure 3 (A) Mature archegoniophores and old antheridiophores. Chobham, June 2021, (B) male colony with three generations of antheridiophores; old, mature and young. Chobham, June 2020, (C) mature male and female alongside Funaria with dehisced capsules. Chobham, July 2021, (D) female colonies with old and young archegoniophores. Thursley, August 2021, (E) old females and young males. Chobham, September 2021.
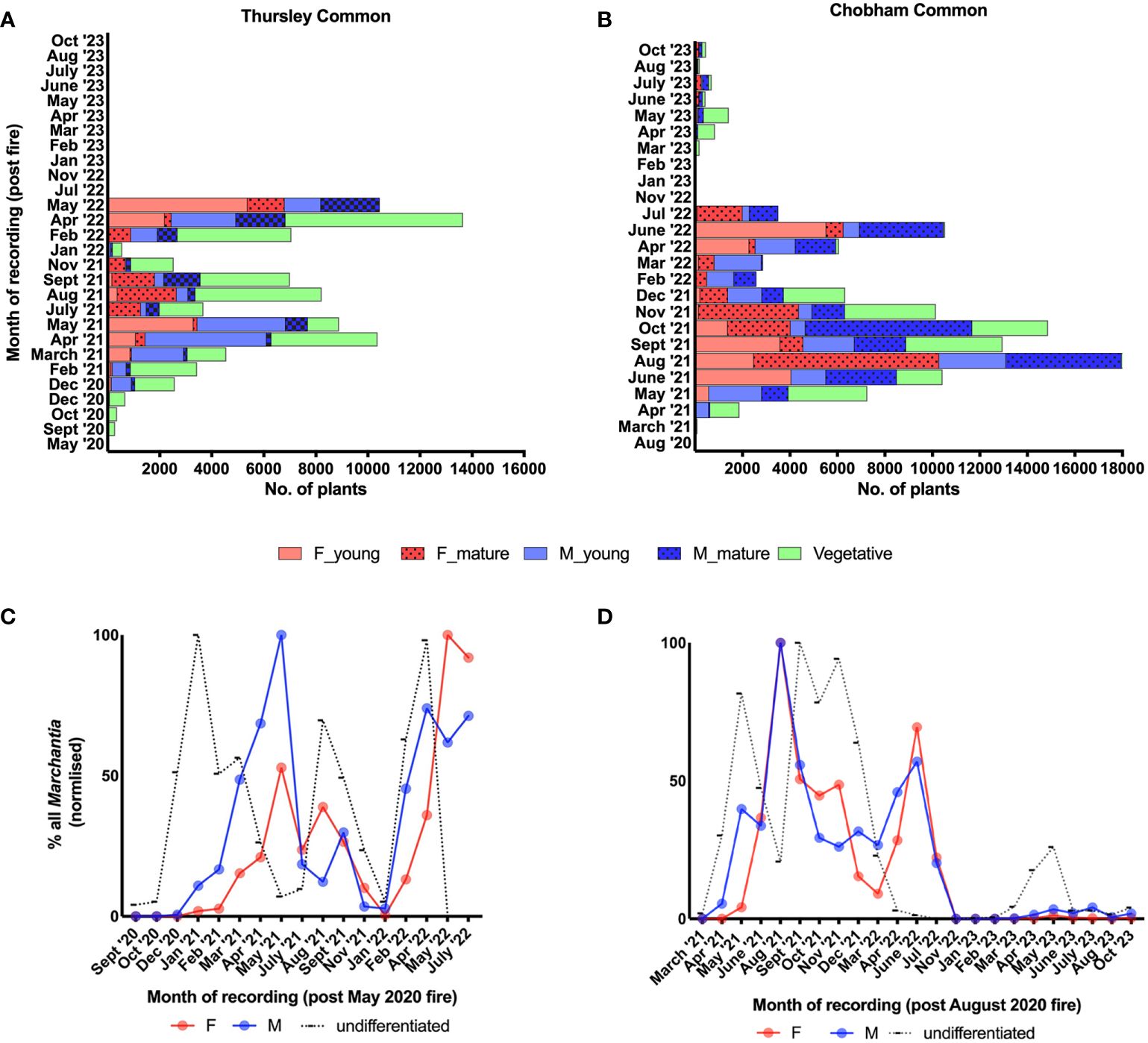
Figure 4 Seasonal changes in reproduction (A, B) and sex ratios - male (M), female (F) and undifferentiated (C, D), in Marchantia polymorpha subsp. ruderalis populations after wildfires (in May and August 2020) at two different heathlands in Southern England, Thursley National Nature Reserve and Chobham Common, respectively. Note these follow similar patterns at both sites (Thursley – A, C; Chobham – B, D) and across 2 1/2 years until the complete disappearance at Thursley and scarce re-emergence at Chobham associated with drought in July 2022.
Sperm discharge occurs continuously throughout the year except over late summer and early winter (August to December), whereas mature archegonia are absent from late autumn until spring (September until March). We have noted spore discharge from late May until October but peaking in July and August. There is never a time of year when maturing archegonia lack a supply of spermatozoids. Although midsummer counting of male and female thalli produces, at first sight, a roughly 1:1 sex ratio, because of the temporal differences between male and female maturation times set out above, this is a gross oversimplification (Figures 4C, D). After initial establishment most of the vegetative thalli are those where gametangiophores have decayed and disappeared.
Given the multiple cycles of gametangiophore production per annum (Figures 1C, 3B, D), prevailing temperature conditions, rather than day length, are the most likely trigger, assuming sufficient rain to keep the thalli hydrated. We found no evidence for any significant dormant period, cf. Lunularia cruciata (Duckett and Pressel, 2023) just faster and slower periods of thallus growth. Funaria also has several life cycles per year in striking contrast to the production of spores once a year in Campylopus introflexus and Ceratodon (Duckett et al., 2023) or 16 months in Polytrichum (Duckett and Pressel, 2023).
The constancy of this reproductive cycle over three years and at two different sites indicates this to be typical of post fire Marchantia ruderalis populations. Other wild populations in other habitats behave similarly with only antheridiophores present in the winter months. The most extreme example of temporal disparity between the sexes we have so far encountered is a huge population of tens of thousands of thalli on clinker between old railway lines at Didcot Railway Centre, southern England (Figure 5A). In August 2023 all the hundreds of sexual thalli we observed were female and we did not see a single antheridiophore, young, mature or moribund. However, the fact that all the females were also producing numerous sporophytes like those at Thursley and Chobham indicates that many of the vegetative thalli also present were males that had since lost their antheridiophores (Figure 5B). As expected, by October 2023, males with young antheridiophores (Figure 5C) were growing alongside females with long dead archegoniophores (Figure 5D). The only archegoniophores lacking sporophytes that we have seen have been on small single sex urban street populations (Figure 5E). The only difference between these and fertilised ones is shorter carpocephala stalks. Thus, archegoniophore maturation is not dependent on fertilization (Bisher, 1998) as considered by some earlier authors e.g., Goebel (1905).
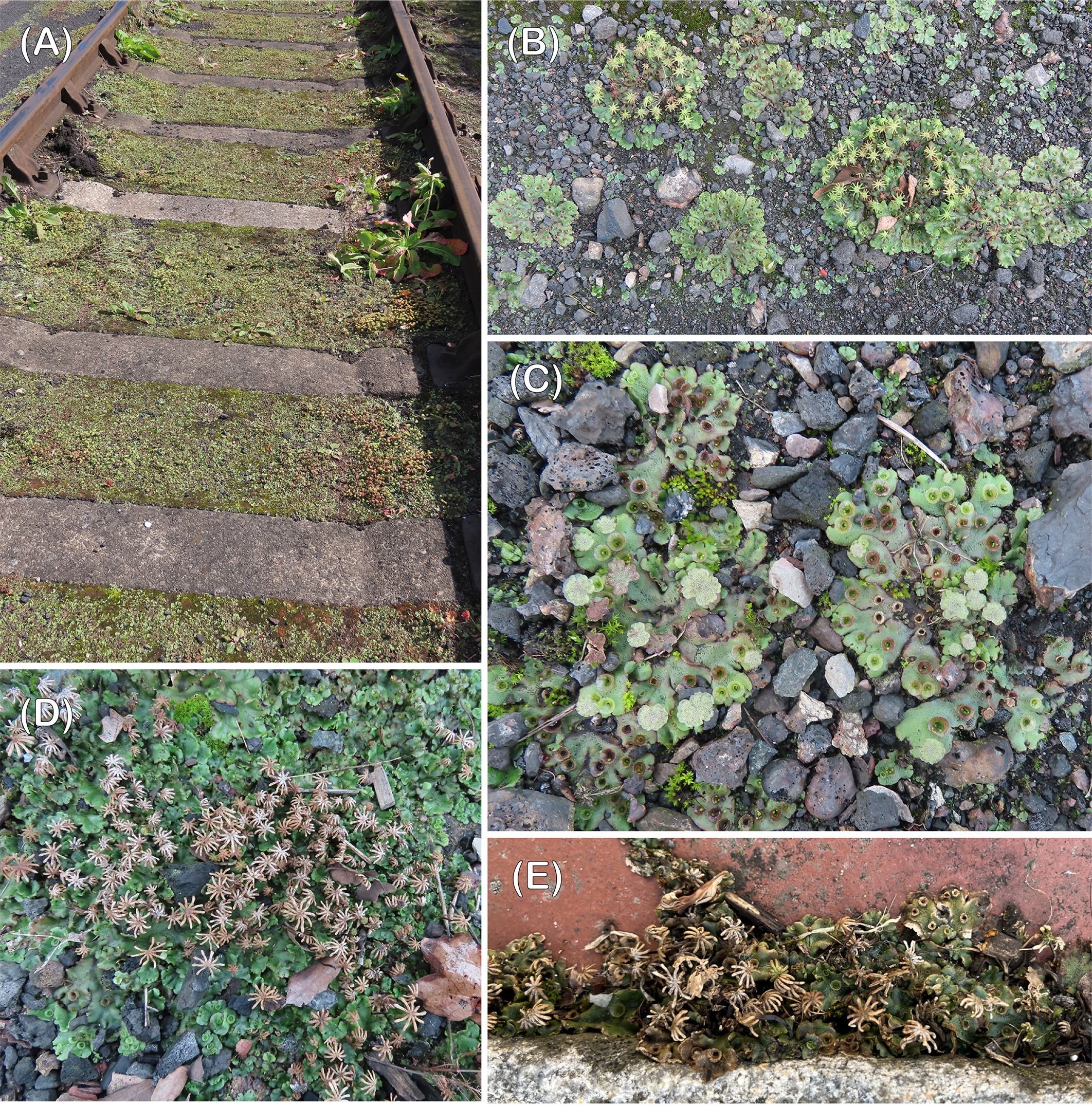
Figure 5 (A) Huge population of Marchantia polymorpha subsp. ruderalis on clinker along an old railway line. Didcot Oxfordshire, England, August 2023, (B) railway clinker colonies August 2023; sporophyte production by all the females indicates that the vegetative colonies are males that have lost their antheridiophores, (C, D) the railway clinker colonies, October 2023 – young males (C) and females with dead archegoniophores (D), (E) female only pavement population; note the shorter archegoniophore stalks compared with those that have produced sporophytes (Figure 1C).
Analysis of 100’s of digital field images revealed no differences between the diameters of male and female colonies, but female thalli tended to be more branched while males produced fewer gemmae and fewer antheridiophores. Ten cm diameter male colonies typically bore around 10 antheridiophores compared with 15-20 archegoniophores produced by same size female colonies. A parallel study of Marchantia inflexa populations in the Southern USA and the Caribbean (Stieha et al., 2014) also details earlier and more profuse gemmae production by males.
Following spermatozoid discharge, old antheridiophores can persist for 2-3 months while, after spore dispersal, old dead archegoniophores can still be found more than 6 months later. Vegetative thalli with few gemmae are almost certainly ones where gametangiophores had decayed previously rather than juvenile individuals.
Our documentation of sexual dynamics in Marchantia has major implications for working out sex ratios in bryophytes generally. These are typically female biased with explanations ranging from environmental factors to sex-specific traits (Longton and Schuster, 1983; McLetchie, 1992; Shaw and Gaughan, 1993; Bowker et al., 2000; McLetchie and Puterbaugh, 2000; Crowley et al., 2005; Bisang et al., 2006; Cronberg et al., 2006, 2015; Rydgren et al., 2006; Hedenäs et al., 2010; Horsley et al., 2011, 2010; Stark et al., 2010; Baughman et al., 2017; Bisang and Glime, 2017). In Marchantia this female bias has been reported in diverse locations, from open habitats in the Falkland Islands (M. berteroana, Engels, 1980; Duckett et al., 2012) to tropical forests in Puerto Rico (M. chenopoda, Moyá, 1992) and Trinidad (M. inflexa). In M. inflexa this is attributed to a faster growth rate and lower gemma production by females (Stieha et al., 2014). This bias was not apparent at Thursley nor at Chobham, where female favouritism is highly restricted to a few months in the summer. The female bias in the Falklands may simply be due to the fact that all the collections were in the summer months. In a study of sex ratios in Costa Rican Marchantia sp. populations, Garrison (2007) recorded an increasingly strong female metapopulation bias during the rainy season and suggested this might be due to higher male nutrient requirements.
Manipulating day length in green house grown plants Wann (1925) found that long days favour gametangiophore production in Marchantia. His discovery that antheridiophores appear after 4-6 weeks and archegoniophores only after 6-8 weeks fits closely with our field observations. Voth and Hamner (1940) also reported a greater number of gametangiophores under a long photoperiod than comparable plants on short photoperiod but did not record a temporal disparity between antheridiophore and archegoniophore formation.
Pointing out a general ignorance of the role of a circadian clock in daylength measurement in basal land plants Kanesaka et al. (2023) found a wildtype accession of Marchantia polymorpha (Takaragaike-1) to be an obligate long-day plant with a critical daylength of about 10 hours and requiring multiple long days for gametangiophore production. They then explored the timing of gametangiophore formation under various non-24-h light/dark cycles to examine the effect of phase alteration in circadian rhythms. They found that daylength measurement in Marchantia is based on the relative amounts of light and darkness rather than the intrinsic rhythms generated by a circadian clock. Thus, Marchantia may have a daylength measurement system different from that of angiosperms that centres on a circadian clock function.
Unfortunately, Kanesaka et al. (2023) only used male plants. Our field data strongly point to a long day requirement for archegoniophore formation from spring until early autumn but that this is highly unlikely for the antheridiophores as these are produced throughout the winter months with daylength down to 7h50min at the solstice. Kanesaka et al.’s work clearly requires repeating with females. It should be highlighted however, that the earlier production of antheridiophores ensures an abundant supply of spermatozoids to the archegoniophores and explains the abundant production of sporophytes in Marchantia (Duckett and Pressel, 2009). Whether this is the rule across dioicous bryophytes invites investigation. Antheridium production certainly precedes the formation of female shoots in monoicous Funaria hygrometrica (Duckett et al., 2023).
Needed now are in vitro studies comparing male and female growth rates and an analysis of the energetic costs of producing antheridiophores versus archegoniophores. This is of particular interest as stalked antheridiophores are restricted to just Marchantia and Neohodgsonia (Bischler-Causse et al., 1995). Sex-specific PCR assays (Iwasaki et al., 2021) are also needed to provide definitive proof of our assumption of a one:one sex ratio in vegetative thalli of Marchantia ruderalis.
Remarkable fertilization distances and prolific sporophyte production
Our recording of fertilization distances of up to 19 m at Thursley in 2007 far exceeds those for all other bryophytes (see Pressel and Duckett, 2019 for full listings). Moreover, whatever the distance to the nearest male, every perianth on every archegoniophore produced a sporophyte which then went on to produce spores. This extremely high fertilization rate we attribute to: 1. The continuous production of huge numbers of spermatozoids. Each antheridium of Marchantia contains over 250,000 spermatozoids, far more than in any other land plant (Pressel and Duckett, 2019). 2. Long distance sperm dispersal between thalli on surface water films via oil droplets liberated from the antheridia (Muggoch and Walton, 1942). 3. Highly effective intra-thallus sperm transport both from the antheridiophores and into the archegoniophores via the peg rhizoid system (Duckett et al., 2013). 4. Resistance of the thalli to dehydration. Whereas on at least half our field visits in the summer months the mosses on the heaths were clearly dried up and desiccated, we only saw dehydrated Marchantia thalli following an extreme drought in the summer of 2022, although these, differently from the mosses, never recovered. The sight of fully hydrated Marchantia gametangiophores alongside dehydrated mosses, including Polytrichum spp. is most striking (see Duckett et al., 2013 for images).
Post fire disappearance
The question of the ultimate disappearance of Marchantia from burnt site merits careful consideration. The reproductive biology of the liverwort followed much the same course after both the 2006 (Duckett and Pressel, 2009) and 2020 fires (this paper). After the 2006 Thursley fire it lasted until the winter of 2009; at both sites after the 2020 fires, it disappeared in the summer of 2022 following a severe drought. In general terms it is attributed to a fall in nutrient levels and competition at fire sites whereas persistence in urban sites is most likely due to additional nutrients from pollution, particularly NOx (Duckett and Pressel, 2016). Absence of mycorrhizal-like associations may also limit survival when nutrient levels fall (Rimington et al., 2020; Bowman et al., 2022); there is no interaction between Marchantia and the ericoid and Cephaloziella fungal endophytes, and Cephaloziella is long persistent after fires (Pressel et al., 2021; personal observations). It is also possible that shifts in soil bacterial and fungal communities (other than mycorrhizal) and/or in their interactions with Marchantia, may impact the establishment and ultimate demise of Marchantia post-fire. Recent research has highlighted the presence in Marchantia polymorpha subsp. ruderalis of several bacterial and fungal endophytes, with functions spanning the symbiotic spectrum, from beneficial to pathogenic (e.g. Alcaraz et al., 2018; Nelson et al., 2018; Poveda, 2024). These include bacterial genera capable of plant growth promotion, exudate degradation, nitrogen fixation, methylotrophs and disease-suppression (Alcaraz et al., 2018). In vitro experiments have demonstrated both beneficial and detrimental effects to Marchantia elicited by diverse fungal endophytes and that these effects could be shaped by nutrients available and the presence of other fungi (Nelson et al., 2018). As such, future research on the establishment and persistence of Marchantia post-fire should also consider this important aspect of its biology. Marchantia rapidly outcompetes Funaria with its short life cycle (Figure 1B) but may coexist for at least two years with Ceratodon (Figures 6A-C). Indeed, it can still reproduce sexually when overrun by this moss (Figures 6B, D). Ultimately Polytrichum spp. (Figure 6E) and Campylopus introflexus take over from these three species. These findings are one of the best examples to date of the detailed timing of a bryophyte succession.
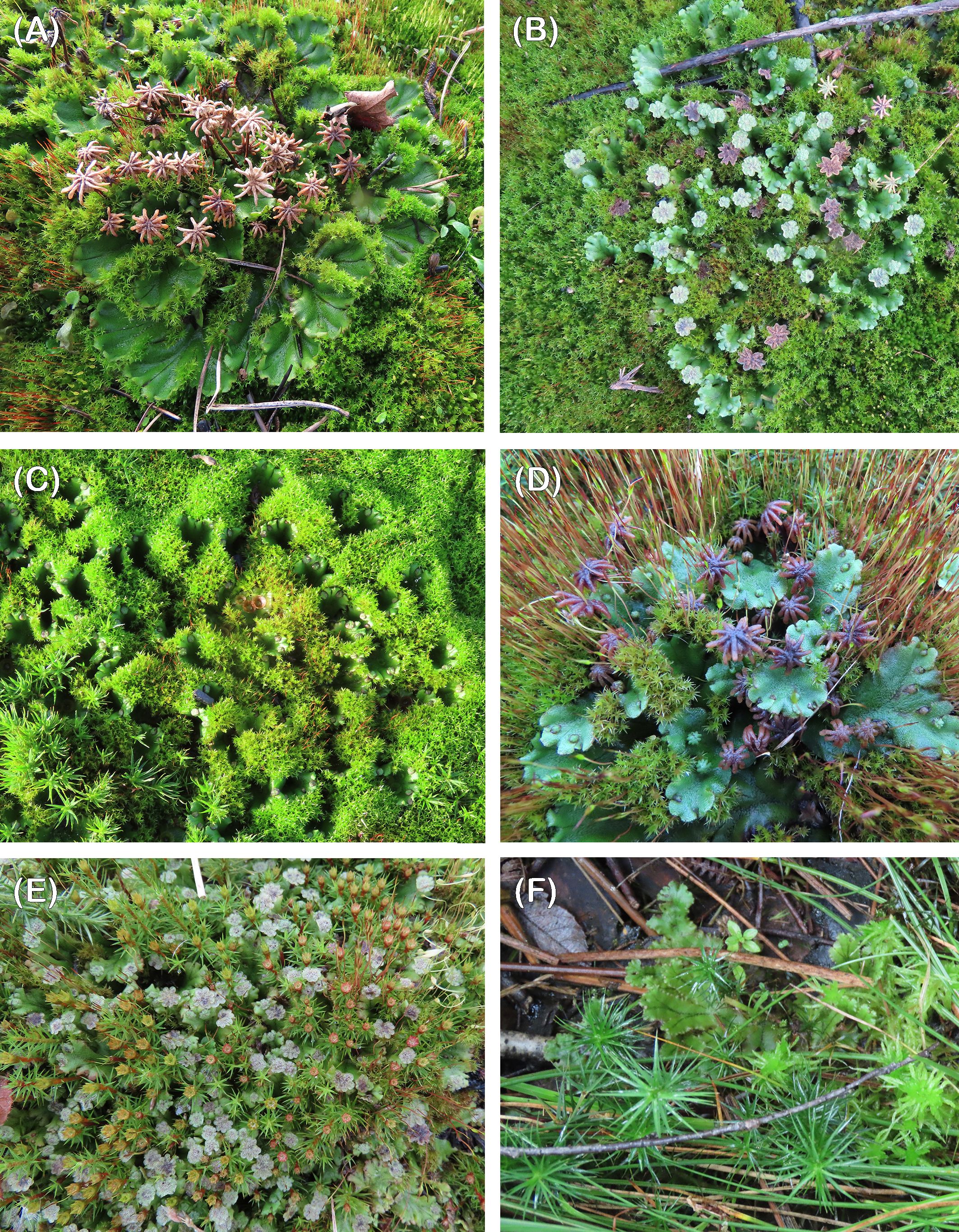
Figure 6 (A) Old females being overgrown by Ceratodon. Chobham, December 2021, (B) young and old males being overgrown by Ceratodon. Chobham, December 2021, (C) thalli almost completely overgrown by Ceratodon. Chobham, January 2022, (D) females with old and young archegoniophores surrounded by Ceratodon with spear stage sporophytes. Chobham, April 2022, (E) old and mature males being overgrown by male Polytrichum juniperinum, Chobham, April 2022, (F) vegetative Marchantia growing in a damp hollow with Polytrichum commune and Sphagnum fimbriatum. Chobham, September 2023.
Perhaps the most dramatic and surprising finding from our observations is the extinction of Marchantia caused by the severe drought in the summer of 2022 (Duckett et al., 2023). It has not reappeared at Thursley but recovered in a very damp site at Chobham associated with Polytrichum commune and Sphagnum spp. (Figure 6F). A dissection of the genes associated with desiccation tolerance across model bryophytes now beckons.
Data availability statement
The original contributions presented in the study are included in the article/supplementary material. Further inquiries can be directed to the corresponding author.
Author contributions
JD: Writing – original draft, Methodology, Investigation. SP: Writing – review & editing, Methodology, Investigation. JK: Writing – review & editing, Methodology, Investigation.
Funding
The author(s) declare that no financial support was received for the research, authorship, and/or publication of this article.
Acknowledgments
We thank James Giles, the warden at Thursley for facilitating access to the post fire sites.
Conflict of interest
The authors declare that the research was conducted in the absence of any commercial or financial relationships that could be construed as a potential conflict of interest.
The author(s) declared that they were an editorial board member of Frontiers, at the time of submission. This had no impact on the peer review process and the final decision.
Publisher’s note
All claims expressed in this article are solely those of the authors and do not necessarily represent those of their affiliated organizations, or those of the publisher, the editors and the reviewers. Any product that may be evaluated in this article, or claim that may be made by its manufacturer, is not guaranteed or endorsed by the publisher.
References
Alcaraz, L. D., Peimbert, M., Barajas, H. R., Dorantes-Acosta, A. E., Bowman, J. L., Arteaga-Vázquez, M. A. (2018). Marchantia liverworts as a proxy to plants’ basal microbiomes. Sci. Rep. 8, 12712. doi: 10.1038/s41598-018-31168-0
Baughman, J. T., Payton, A. C., Paasch, A. E., Fisher, K. M., McDaniel, S. F. (2017). Multiple factors influence population sex ratios in the Mojave Desert moss Syntrichia caninervis. Am. J. Bot. 104, 733–742. doi: 10.3732/ajb.1700045
Bisang, I., Ehrlén, J., Hedenäs, L. (2006). Reproductive effort and costs of reproduction do not explain female-biased sex ratios in the moss Drepanocladus trifarius (Amblystegiaceae). Am. J. Bot. 93, 1313–1319. doi: 10.3732/ajb.93.9.1313
Bisang, I., Ehrlén, J., Korpelainen, H., Hedenas, L. (2015). No evidence of sexual niche partitioning in a dioecious moss with rare sexual reproduction. Ann. Bot. 116, 771–779. doi: 10.1093/aob/mcv133
Bisang, I., Glime, J. M. (2017). “Chapter 3-2 Sexuality: sex ratio and sex expression,” in Bryophyte Ecology, vol. 1 . Ed. Glime, J. M. (Mitchigan Technology University, Mitchigan USA: Ebook).
Bischler-Causse, H., Glenny, D., Boisselier-Dubayle, M. C. (1995). On neohodgsonia H. Perss. (Marchantiales, hepaticae). Cryptogam. Bryol. Lichenol. 16, 235–245.
Bisher, H. (1998). Systematics and evolution of the genera of the marchantiales. Bryophytorum Bibliotheca. 51, 1–201.
Blockeel, T. L., Bosanquet, S. D. S., Hill, M. O., Preston, C. D. (2014). Atlas of British and Irish Bryophytes Vol. 2 (Newbury: Pisces Publications).
Bowker, M. A., Stark, L. R., McLetchie, D. N., Mishler, B. D. (2000). Sex expression, skewed sex ratios, and microhabitat distribution in the dioecious desert moss Syntrichia caninervis (Pottiaceae). Am. J. Bot. 87, 517–526. doi: 10.2307/2656595
Bowman, J. L., Arteaga-Vazquez, M., Berger, F., Briginshaw, L. N., Carella, P., Aguilar-Cruz, A., et al. (2022). The renaissance and enlightenment of Marchantia as a model system. Plant Cell 34, 3512–3542. doi: 10.1093/plcell/koac219
Clymo, R. S., Duckett, J. G. (1986). Regeneration of sphagnum. New Phytol. 102, 589–614. doi: 10.1111/j.1469-8137.1986.tb00834.x
Cronberg, N., Rydgren, K., Økland, R. H. (2006). Clonal structure and genet-level sex ratios suggest different roles of vegetative and sexual reproduction in the clonal moss Hylocomnium splendens. Ecography 29, 95–103. doi: 10.1111/j.2006.0906-7590.04361.x
Crowley, P. H., Stieha, C. R., McLetchie, D. N. (2005). Overgrowth competition, fragmentation and sex-ratio dynamics: a spatially explicit, sub-individual-based model. J. Theor. Biol. 233, 25–42. doi: 10.1016/j.jtbi.2004.09.017
Duckett, J. G., Andrew, E., Kowal, J., Pressel, S. (2023). Fires, drought, extinction and regeneration. Field Bryol. 129, 19–34.
Duckett, J. G., Ligrone, R., Renzaglia, K. S., Pressel, S. (2013). Pegged and smooth rhizoids in complex thalloid liverworts (Marchantiophta): structure, function and evolution. Bot. J. Linn. 174, 68–92. doi: 10.1111/boj.12121
Duckett, J. G., Matcham, H. W., Pressel, S. (2008). Thursley Common NNR: bryophyte recolonization one year after the great fire of July 2006. Field Bryol. 94, 3–11.
Duckett, J. G., Pressel, S. (2009). Extraordinary features of the reproductive biology of Marchantia at Thursley Common. Field Bryol. 97, 2–11.
Duckett, J. G., Pressel, S. (2016). Not necessarily NOXious: blossoming bryophytes on London’s roadside trees. Field Bryol. 115, 100.
Duckett, J. G., Pressel, S. (2022). Do moss sporophytes maintain water balance? New insights from sporophyte water relations and the natural maturation cycle in Funaria hygrometrica Hedw. J. Bryol. 44, 187–198. doi: 10.1080/03736687.2022.2154736
Duckett, J. G., Pressel, S. (2023). The 16-month reproductive cycles of Polytrichum formosum and P. juniperinum. Field Bryol. 130, 64.
Duckett, J. G., Russell, S., Upson, R., Tangney, R. (2012). Lower plants inventory and conservation in the Falkland Islands; bryophyte reconnaissance and collecting expedition. Field Bryol. 106, 32–42.
Engels, J. J. (1980). Falkland Islands (Islas Malvinos) Hepaticae and Anthocerotophyta: a taxonomic and phytogeographic study. Fieldiana 25, 1–209.
Frangedakis, E., Shimamura, M., Villarreal, J. C., Li, F. W., Tomaselli, M., Waller, M., et al. (2021). The hornworts: morphology, evolution and development. New Phytol. 229, 735–754. doi: 10.1111/nph.16874
Furness, S. B., Hall, R. H. (1981). An explanation of the intermittent occurrence of Physcomitrium sphaericum (Hedw.) Brid. J. Bryol. 11, 733–744. doi: 10.1179/jbr.1981.11.4.733
Garrison, L. (2007). Population abundance, sexual expression, and gender ratios of Marchantia sp. along an elevational gradient in Monteverde, Costa Rica. Trop. Ecol. Conserv. 545, 1–10.
Hedenäs, L., Bisang, I., Korpelainen, H., Cronholm, B. (2010). The true sex ratio in European Pseudocalliergon trifarium (Bryophyta: Amblystegiaceae) revealed by a novel molecular approach. Biol. J. Linn. Soc 100, 132–140. doi: 10.1111/j.1095-8312.2010.01408.x
Horsley, K., Stark, L. R., McLetchie, D. N. (2011). Does the silver moss Bryum argenteum exhibit sex-specific patterns in vegetative growth rate, asexual fitness or prezygotic reproductive investment? Ann. Bot. 107, 897–907. doi: 10.1093/aob/mcr027
Inoue, H. (1961). Studies in spore germination and the early stages in gametophyte germination in the Marchantiales. J. Hattori Bot. Lab. 23, 148–191.
Iwasaki, M., Kajiwara, T., Yasui., Y., Yoshitake, Y., Miyazaki, M., Kawamura, S., et al. (2021). Identification of the sex-determining factor in the liverwort Marchantia polymorpha reveals unique evolution of sex chromosomes in a haploid system. Curr. Biol. 31, 5522–5532. doi: 10.1016/j.cub.2021.10.023
Jonsson, B. G. (1993). The bryophyte diaspore bank and its role after small-scale disturbance in a boreal forest. J. Veg. Sci. 4, 819–826. doi: 10.2307/3235620
Kanesaka, Y., Inoue, K., Tomita, Y., Yamaoka, S., Araki, T. (2023). Circadian clock does not play an essential role in daylength measurement for growth-phase transition in Marchantia polymorpha. Front. Plant. Sci. 14, 1275503. doi: 10.3389/fpls.2023.1275503
Kollar, L. M., Kiel, S., James, A. J., Carnley, C. T., Scolla, D. N., Clarke, T. N., et al. (2021). The genetic architecture of sexual dimorphism in the moss Ceratodon purpureus. Proc. R. Soc B. 288, 20202908. doi: 10.1098/rspb.2020.2908
Longton, R. E., Schuster, R. M. (1983). “Reproductive Biology,” in New Manual of Bryology. Ed. Schuster, R. M. (Hattori Botanical Laboratory, NiChinan), 386–462.
McLetchie, D. N. (1992). Sex ratio from germination through maturity and its reproductive consequences in the liverwort Sphaerocarpos texanus. Oecologia 92, 273–278. doi: 10.1007/BF00317375
McLetchie, D. N., Puterbaugh, M. N. (2000). Population sex ratios, sex-specific clonal traits and tradeoffs among these traits in the liverwort Marchantia inflexa. Oikos 90, 227–237. doi: 10.1034/j.1600-0706.2000.900203.x
Moyá, M. T. (1992). Phenological observations and sex ratios in Marchantia chenopoda L. (Hepaticae: Marchantiaceae). Trop. Bryol. 6, 161–168. doi: 10.11646/bde.6.1.18
Muggoch, H., Walton, J. (1942). On the dehiscence of the antheridium and the part played by surface tension in the dispersal of spermatocytes in Bryophyta. Proc. R. Soc Lond. B 130, 448–461. doi: 10.1098/rspb.1942.0012
Nelson, J. M., Hauser, D. A., Hinson, R., Shaw, A. J. (2018). A novel experimental system using the liverwort Marchantia polymorpha and its fungal endophytes reveals diverse and context-dependent effects. New Phytol. 218, 1217–1232. doi: 10.1111/nph.15012
Paton, J. A. (1999). The Liverwort Flora of the British Isles (Colchester: Harley Books). doi: 10.1163/9789004285385
Pettersson, B. (1940). Experimentelle Untersuchungen über die euanemochore Ver- breitung der Sporenpflanzen. Acta Bot. Fenn. 25, 1–102.
Poveda, J. (2024). Analysis of Marchantia polymorpha-microorganism interactions: basis for understanding plant-microbe and plant-pathogen interactions. Front. Plant Sci. 15, 1301816. doi: 10.3389/fpls.2024.1301816
Pressel, S., Duckett, J. G. (2019). Do motile spermatozoids limit the effectiveness of sexual reproduction in bryophytes? Not in the liverwort Marchantia polymorpha. J. Systematics Evol. 57, 371–381. doi: 10.1111/jse.12528
Pressel, S., Kowal, J., Duckett, J. G. (2021). A tale of two fires: heathland bryophyte successions at Thursley NNR, Surrey, long and short-term. Field Bryol. 125, 34–49.
Rensing, S. A., Goffinet, B., Meyberg, R., Wu, S.-Z., Bezanilla, M. (2020). The moss Physcomitrium (Physcomitrella) patens: A model organism for non-seed plants. Plant Cell 32, 1361–1376. doi: 10.1105/tpc.19.00828
Rimington, W. R., Duckett, J. G., Field, K. F., Bidartondo, M. I., Pressel, S. (2020). The distribution and evolution of fungal symbioses in ancient lineages of land plants. Mycorrhiza 30, 23–49. doi: 10.1007/s00572-020-00938-y
Rydgren, K., Cronberg, N. H., Økland, R. H. (2006). Factors influencing reproductive success in the clonal moss Hylocomium splendens. Oecologia 147, 445–454. doi: 10.1007/s00442-005-0290-2
Rydgren, K., Halvorsen, R., Cronberg, N. (2010). Infrequent sporophyte production maintains a female biased sex ratio in the unisexual clonal moss Hylocomium splendens. J. Ecol. 98, 1224–1231. doi: 10.1111/j.1365-2745.2010.01639.x
Shaw, A. J., Gaughan, J. F. (1993). Control of sex ratios in haploid populations of the moss Ceratodon purpureus. Am. J. Bot. 80, 584–591. doi: 10.1002/j.1537-2197.1993.tb13844.x
Shimamura, M. (2016). Marchantia polymorpha: taxonomy, phylogeny and morphology of a model system. Plant Cell Physiol. 57, 130–256. doi: 10.1093/pcp/pcv192
Stark, L. R., McLetchie, D. N., Eppley, S. M. (2010). Sex ratios and the shy male hypothesis in the moss Bryum argenteum (Bryaceae). Bryologist 113, 788–797. doi: 10.1639/0007-2745-113.4.788
Stieha, C. R., Middleton, A. R., Stieha, J. K., Trott, S. H., McLetchie, D. N. (2014). The dispersal process of asexual propagules and the contribution to population persistence in Marchantia (Marchantiaceae). Am. J. Bot. 101, 348–356. doi: 10.3732/ajb.1300339
Sundberg, S., Rydin, H. (2000). Experimental evidence for a persistent spore bank in Sphagnum. New Phytol. 148, 105–116. doi: 10.1046/j.1469-8137.2000.00746.x
Szövényi, P., Frangedakis, E., Ricca, M., Quandt, D., Wicke, S., Langdale, J. A. (2015). Establishment of Anthoceros agrestis as a model species for studying the biology of hornworts. BMC Plant Biol. 15, 98. doi: 10.1186/s12870-015-0481-x
Van Zanten, B. O., Gradstein, S. R. (1988). Experimental dispersal geography of neotropical liverworts. Beiheft zu Nova Hedwigia 90, 41–94.
Voth, P. D., Hamner, K. C. (1940). Responses of Marchantia polymorpha to nutrient supply and photoperiod. Bot. Gaz. 102, 169–205. doi: 10.1086/334943
Wann, F. B. (1925). Some of the factors involved in sexual reproduction of Marchantia polymorpha. Am. J. Bot. 12, 307–318. doi: 10.1002/j.1537-2197.1925.tb05836.x
Keywords: carpocephala, female bias, fertilization distances, post-fire establishment, sex ratio, spore viability
Citation: Duckett JG, Pressel S and Kowal J (2024) The biology of Marchantia polymorpha subsp. ruderalis Bischl. & Boissel. Dub in nature. Front. Plant Sci. 15:1339832. doi: 10.3389/fpls.2024.1339832
Received: 16 November 2023; Accepted: 06 May 2024;
Published: 30 May 2024.
Edited by:
Jorge Poveda, University of Valladolid, SpainCopyright © 2024 Duckett, Pressel and Kowal. This is an open-access article distributed under the terms of the Creative Commons Attribution License (CC BY). The use, distribution or reproduction in other forums is permitted, provided the original author(s) and the copyright owner(s) are credited and that the original publication in this journal is cited, in accordance with accepted academic practice. No use, distribution or reproduction is permitted which does not comply with these terms.
*Correspondence: Silvia Pressel, cy5wcmVzc2VsQG5obS5hYy51aw==