- 1College of Life Science, Northwest Normal University, Lanzhou, China
- 2State Key Laboratory of Herbage Improvement and Grassland Agro-ecosystems, College of Ecology, Lanzhou University, Lanzhou, China
Plant species loss, driven by global changes and human activities, can have cascading effects on other trophic levels, such as arthropods, and alter the multitrophic structure of ecosystems. While the relationship between plant diversity and arthropod communities has been well-documented, few studies have explored the effects of species composition variation or plant functional groups. In this study, we conducted a long-term plant removal experiment to investigate the impact of plant functional group loss (specifically targeting tall grasses and sedges, as well as tall or short forbs) on arthropod diversity and their functional groups. Our findings revealed that the removal of plant functional groups resulted in increased arthropod richness, abundance and the exponential of Shannon entropy, contrary to the commonly observed positive correlation between plant diversity and consumer diversity. Furthermore, the removal of different plant groups had varying impacts on arthropod trophic levels. The removal of forbs had a more pronounced impact on herbivores compared to graminoids, but this impact did not consistently cascade to higher-trophic arthropods. Notably, the removal of short forbs had a more significant impact on predators, as evidenced by the increased richness, abundance, the exponential of Shannon entropy, inverse Simpson index and inverse Berger-Parker index of carnivores and abundance of omnivores, likely attributable to distinct underlying mechanisms. Our results highlight the importance of plant species identity in shaping arthropod communities in alpine grasslands. This study emphasizes the crucial role of high plant species diversity in controlling arthropods in natural grasslands, particularly in the context of plant diversity loss caused by global changes and human activities.
1 Introduction
Global changes (Butchart et al., 2010; Griggs et al., 2013; Maes et al., 2016) and human activities have resulted in a decline in biodiversity (Dirzo et al., 2014; Vogel, 2017; Leather, 2018), which in turn affects ecosystem functioning and services (Loreau and Hector, 2001; Cardinale et al., 2012; Isbell et al., 2017). For instance, experimental warming and livestock grazing have been shown to significantly alter plant species composition and net primary production in alpine grasslands on the Tibetan Plateau, particularly the relative abundance of grasses, sedges, and herbs (Klein et al., 2007; Wang et al., 2012; Liu et al., 2018). However, the impact of plant species loss on other trophic levels, such as consumer diversity and multitrophic structure, has rarely been investigated in natural grasslands (Hooper et al., 2005; Duffy et al., 2007; Haddad et al., 2009; Scherber et al., 2010).
Arthropods play a vital role in grassland ecosystems and their abundance and community structure are greatly influenced by primary producers (Wilson, 1987; Siemann et al., 1998; Uchida & Ushimaru, 2014). A diverse plant community provides a wider range of resources and higher productivity, thereby supporting a greater variety of consumers (Strong, 1984; Srivastava & Lawton, 1998). For example, numerous studies have shown a positive relationship between plant diversity and herbivore diversity and abundance (Haddad et al., 2009; Ebeling et al., 2018). This relationship is attributed to the increased availability of ecological niches and dietary diversity for herbivores in diverse plant communities. As a result, the richness of carnivores is also promoted due to the higher diversity and abundance of herbivores (Siemann et al., 1998; Haddad et al., 2001; Gamfeldt et al., 2005; Dassou & Tixier, 2016; Jacquot et al., 2019). This is consistent with the resource heterogeneity hypothesis (Hutchinson, 1959; Lewinsohn & Roslin, 2008; Moreira et al., 2016), as a greater diversity of herbivores provides more specialized prey for certain carnivores, leading to an increase in their richness. Additionally, diverse plant communities can provide more structurally diverse habitats (Strong, 1984), which can support higher abundances of predators and limit herbivore populations (the Enemies Hypothesis, Root, 1973). While many manipulating biodiversity experiments have found a positive correlation between plant diversity and consumer diversity (herbivores and carnivores) (Koricheva et al., 2000; Haddad et al., 2001; Wimp et al., 2004; Crutsinger et al., 2006; Johnson et al., 2006; Haddad et al., 2009; Scherber et al., 2010; Borer et al., 2012; Ebeling et al., 2018), there have been a few observational and experimental studies that reported no impact of plant diversity on consumer diversity (Currie, 1991; Wright & Samways, 1998; Hawkins & Porter, 2003; Jetz et al., 2009).
While the relationships between plant diversity and arthropod communities have been well-documented, few studies have specifically investigated the impact of variation in plant species composition or functional groups on these communities (Schaffers et al., 2008). It is important to consider the influence of plant composition and functional groups as they form the basis of arthropod food webs by providing resources such as foliage, nectar, and pollen (Bronstein et al., 2006; Gish et al., 2015). Many phytophagous insects rely on specific plant species or genera for their survival (Bernays & Graham, 1988; Forister et al., 2015). Therefore, changes in plant species composition or functional groups can have a significant impact on herbivores and other higher trophic level arthropods (Symstad et al., 2000; Schaffers et al., 2008; Tobisch et al., 2023).
To address this knowledge gap, this study utilizes a long-term experiment in an alpine meadow on the Tibetan Plateau, where different plant functional groups have been systematically removed since 2012 (Zhou, 2019). Specifically, plant species from different height categories, including tall grass and sedge species, tall forb species, and short forb species, were targeted for removal. The experiment focuses on the impacts of plant diversity loss on arthropod diversity and their functional groups. This approach reflects realistic scenarios observed in ecosystems affected by overgrazing (Mu et al., 2016; Wang and Wesche, 2016; Niu et al., 2016a; Abdalla et al., 2018), human activities (such as grazing exclusion and fertilizer addition), and climate change (such as experimental warming) (Klein et al., 2007; Chen et al., 2013; Niu et al., 2014; Zhang et al., 2015), which can lead to changes in plant species abundance and composition.
The study aims to address the following questions: 1) Does the removal of plant species result in a decrease in arthropod abundance and diversity? 2) How does the removal of different plant functional groups affect arthropod communities across various trophic levels, such as herbivores, carnivores, and omnivores? Based on the well-established positive relationship between plant diversity and arthropod diversity, we predict that the overall diversity and abundance of arthropods will decrease in response to the removal of any of the three targeted plant groups. Furthermore, we expect that different plant groups will have varying impacts on arthropod trophic levels, which can be attributed to different mechanisms.
2 Materials and methods
2.1 Study site
The experiment was conducted in the Gansu Gannan Grassland Ecosystem National Observation and Research Station (35°58’N, 101°53’E) located in Maqu County of Gansu Province. The site experiences more than 2580 hours of annual sunshine, with no absolute frost-free period throughout the year and an annual average frost period of no less than 270 days. The average annual temperature is 1.20°C, with the highest temperature during the growing season ranging from 23.6-28.9°C. The average annual precipitation is 620-780mm, mainly occurring from June to September.
The vegetation at the site is typical alpine meadow on the eastern Tibetan Plateau, with Kobresia setschwanensis as the dominant species and other abundant grass and forb species such as Elymus nutans, Koeleria cristata, Anemone obtusiloba, and Anemone rivularis (Supplementary Figure S1). These species account for more than 80% of the total aboveground biomass of the quadrat plant community (Zhou, 2019).
2.2 Species removal experiment
The plant species removal experiment was established in a fenced alpine meadow in 2012, with no grazing from April to November (Zhou, 2019; Zhou et al., 2019). The experiment included five replicates for each treatment, with a total of 20 1.5m×1.5m plots (Supplementary Figure S2). This design was chosen to balance longer periods of field work with a large labor force, due to the relatively low β diversity of the plant community and soil heterogeneity (Niu et al., 2016b). Following a typical random blocks experimental design, we established 5 blocks as repetitions for plant removal. The removal was carried out once a year, in the beginning of the growing season in May since 2012. The target plant species (Supplementary Table S1) are removed with hand scissors, at the soil surface leaving the rest of the vegetation undisturbed. It is important to note that when removing the target plant species, we made efforts to remove the roots without damaging neighboring non-target individuals. Considering the actual loss of plant species diversity due to land use and climate change, we implemented four treatments: Control without removal (CK), removal of tall forb species (B: Re_tall_Forbs), removal of short forb species (C: Re_short_Forbs), and removal of tall grass and sedge species (D: Re_tall_Grasses_Sedges).
Numerous studies have demonstrated that height is a crucial trait in determining species fitness (Westoby et al., 2002; Díaz et al., 2016; Thomson et al., 2018; Du et al., 2022). Fertilization and removal experiments conducted on the Qinghai-Tibet Plateau have also shown that height differences play a dominant role in species fitness differences, species coexistence, and productivity in alpine grassland plant communities (Liu et al., 2016). Given the large number of forb species, we categorized them into tall (above 30cm) and short (below 15cm) categories to balance the number of species and biomass in the experimental site (Supplementary Table S1). The number of species removed varied by among treatments (Re_tall_Forbs: 19 species; Re_short_Forbs: 19 species; Re_tall_Grasses_Sedges: 12 species). Importantly, this approach ensures that the loss of species diversity and biomass is comparable between treatments.
2.3 Measurement of plant community
Three surveys were conducted to measure the plant community in each removal plot from late July to late August 2021. Within a 50 cm×50 cm quadrat, we measured and counted the plant species, as well as the height and abundance of each species in the plot. During the third plant quadrat survey, we collected plant material by cutting all plants in each plot at the soil surface, and then oven-dried the samples at 75 °C for 48 hours and measured their dry biomass (g m-2).
2.4 Arthropod sampling
For arthropod sampling, we used a trap method to collect surface arthropods three times in early July, early August, and late August 2021. It needs to note that no pre-treatment data of arthropods are available. Prior to sampling, we checked the local weather forecast for the next 48 hours and chose clear weather. To minimize damage to the plant community, we laid 20 trap bottles for each sampling site according to the plant growth of each sample site. Each trap bottle had a top diameter of 5.7 cm, a bottom diameter of 5.7 cm, a height of 7.1 cm, and a volume of approximately 200 ml. We poured 50-70 ml of 20% alcohol solution and 10-20 ml of white vinegar into each trap bottle, buried it in the ground and kept the mouth level with the surface. After 48 hours, we retrieved the trap bottles and separated the collected surface arthropods, which were then stored in 75% alcohol liquid. In the laboratory, we observed and identified the arthropods at least to the family level, or where possible, to the genus and species level (Ward et al., 2001). We recorded the species abundance for each quadrat and classified all arthropods into different functional groups based on taxonomy, life history characteristics, and feeding relationships (Supplementary Table S2).
2.5 Statistical analysis
Due to the random block design and repeated measurements, the experimental data are not completely independent. Therefore, to examine the overall impacts of plant species loss on arthropods, we conducted statistical analyses using linear mixed-effects models (LMMs). We calculated the effect size and its corresponding 95% confidence interval (CI) to quantify the treatment effect of plant species loss on arthropods. The regression coefficient in the LMMs represents the direction and magnitude of the treatment effect (Wu et al., 2022). Before analysis, we performed a natural logarithmic transformation on the response variable data to improve normality.
To visualize the differences in arthropod species composition under different treatments, we used non-metric multidimensional scaling (NMDS) and performed Permutational multivariate analysis of variance (PERMANOVA). The “metaMDS” function in the “vegan” package was used for NMDS, and a distance matrix was created based on arthropod abundance data using Bray-Curtis coefficients. Bray-Curtis distances were then used for NMDS ordering. All analyses were permuted 999 times (Minchin, 1987; Legendre and Legendre, 1998).
Furthermore, to investigate the effects of species loss on arthropod species diversity, we estimated five measures: (a) arthropod abundance, which refers to the number of individual arthropods; (b) arthropod richness, which refers to the number of arthropod species; (c) the exponential of Shannon entropy (Chao et al., 2014), which is often sensitive to the presence of rare species (Nagendra, 2002); (d) the inverse Simpson index, which emphasizes the dominant members (Nagendra, 2002), can be interpreted as the effective number of dominant species in the assemblage; (e) inverse Berger-Parker index, which is a preponderance measure that simply represents the proportional abundance of the most abundant species (Magurran, 2004). The four diversity indices can give an emphasis respectively on total species (species richness), rare species (Shannon diversity), dominant species (Simpson diversity) and the top dominant species (Berger-Parker) (Supplementary Table S6).
Based on correlations between our observations and data formats, as well as parameters for more accurate estimates of complex data structures. We used LMMs to examine the impacts of plant species diversity on arthropod and functional groups diversity (i.e. abundance, richness, the exponential of Shannon entropy, inverse Simpson index and inverse Berger-Parker index). Overall, arthropod diversity as a response variable and plant species diversity as a predictor explained the relationship between arthropods and herbivores and plant diversity. At the same time, we also analyzed the correlation between the diversity of different functional groups of arthropods, and set the corresponding prediction and response variables according to their significant difference changes under different treatments and the cascade relationship between different trophic levels. The all LMMs included the block as an independent random intercept effect.
All data analysis was conducted in R version 4.3.1 (http://www.r-project.org.version4.3.1). The “vegan” package was used for NMDS, and the “lme4” package was used for LMMs (Bates et al., 2015). We calculated the marginal and conditional R2-values of the models using the “glmm.hp” package (Lai et al., 2022). We use the “iNEXT” package to calculate R/E curves and sample completeness curves based on sample size. The results showed that the sampling effort was adequate, respectively covering (98.0%, 98.2%, 98.9%, 98.7%) of species richness in the communities (Supplementary Figure S3).
3 Results
3.1 Effects of plant functional groups removal on arthropod community
In the removing experiments, 2659 arthropods were totally sampled. Herbivores, carnivores, and omnivores accounted for 36.5%, 11.25%, and 52.25% of all arthropods in the control, 25.36%, 2.37%, and 72.27% in the treatment of removing tall grasses and sedges, 28.55%, 15.82%, and 55.63% in the treatment of removing tall forbs, and 29.94%, 11.75%, and 58.31% in the treatment of removing short forbs.
The forbs removal (including tall and short) significantly increased arthropod species richness, while removing tall grasses and sedges did not (Figure 1A). The abundance of arthropods increased significantly in the treatments of removing tall grasses and sedges and short forbs, but not in the treatment of removing tall forbs. Specifically, the former showed a 101% increase, and the latter showed a 150% increase (Figure 1B). For arthropods in the remove tall forbs treatment, the exponential of Shannon entropy was increased significantly, but did not change in the treatments of removing short forbs and tall grasses and sedges (Figure 2A). In the treatments involving the removal of tall grasses and sedges and forbs, there was no change observed in the inverse Simpson index and inverse Berger-Parker index of arthropods (Figures 2B, C).
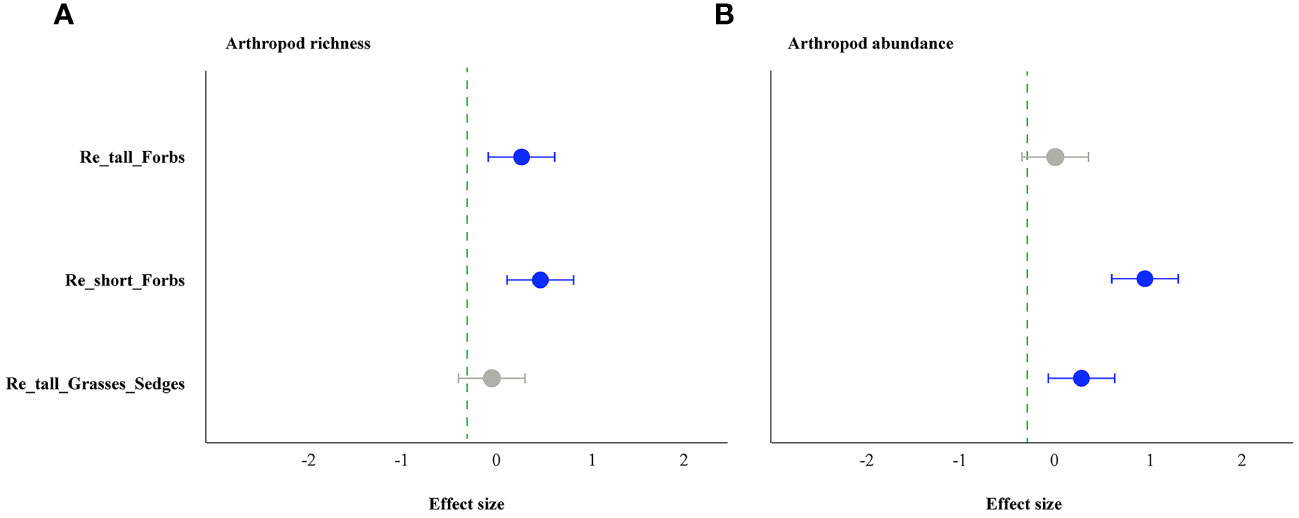
Figure 1 The effects of removing plant functional groups on arthropod richness (A) and abundance (B) by linear mixed-effects models (LMMs). The estimated values of the LMMs represent the positive (blue) or negative (red) effects of different species removals. The uncertainty associated with each treatment is depicted by the median values (above the bars) and a 95% credibility interval. A significant effect is indicated when the 95% confidence interval error line does not overlap with 0. The treatments include the removal of tall forb species (Re_tall_Forbs), short forb species (Re_short_Forbs), and tall grasses and sedges (Re_tall_Grasses_Sedges).
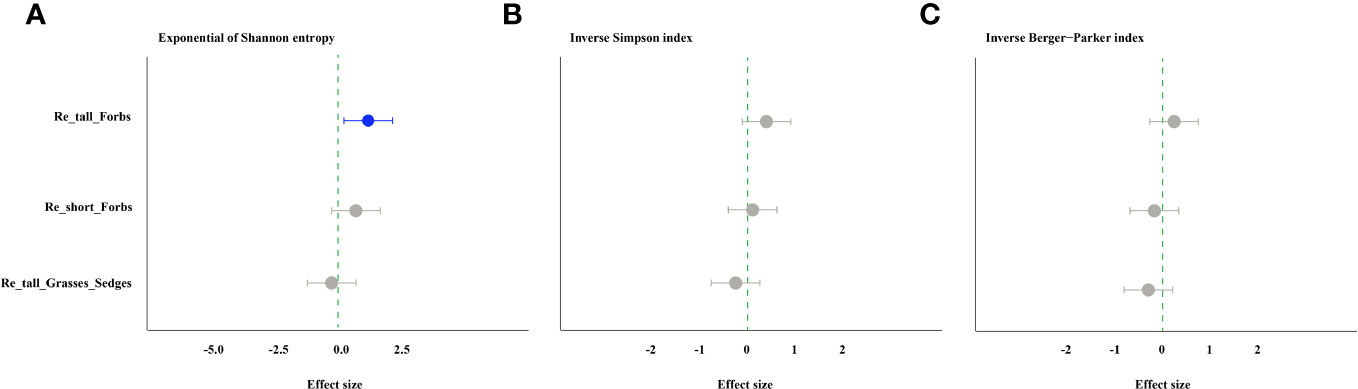
Figure 2 The effects of removing plant functional groups on the exponential of Shannon entropy (A), inverse Simpson index (B) and inverse Berger-Parker index of arthropod (C) by linear mixed-effects models (LMMs). The estimated values of the LMMs represent the positive (blue) or negative (red) effects of different species removals. The comments in the figure correspond to those shown in Figure 1.
Overall, there was no significant correlation between arthropod richness, the exponential of Shannon entropy, inverse Simpson index and inverse Berger-Parker index and plant diversity (Supplementary Figure S8), while higher plant diversity significantly reduced arthropod abundance (Supplementary Figure S7). Furthermore, compared to the control, both removing short forbs and tall grasses and sedges significantly altered the species composition of the arthropod community (Figure 3; Supplementary Table S3).
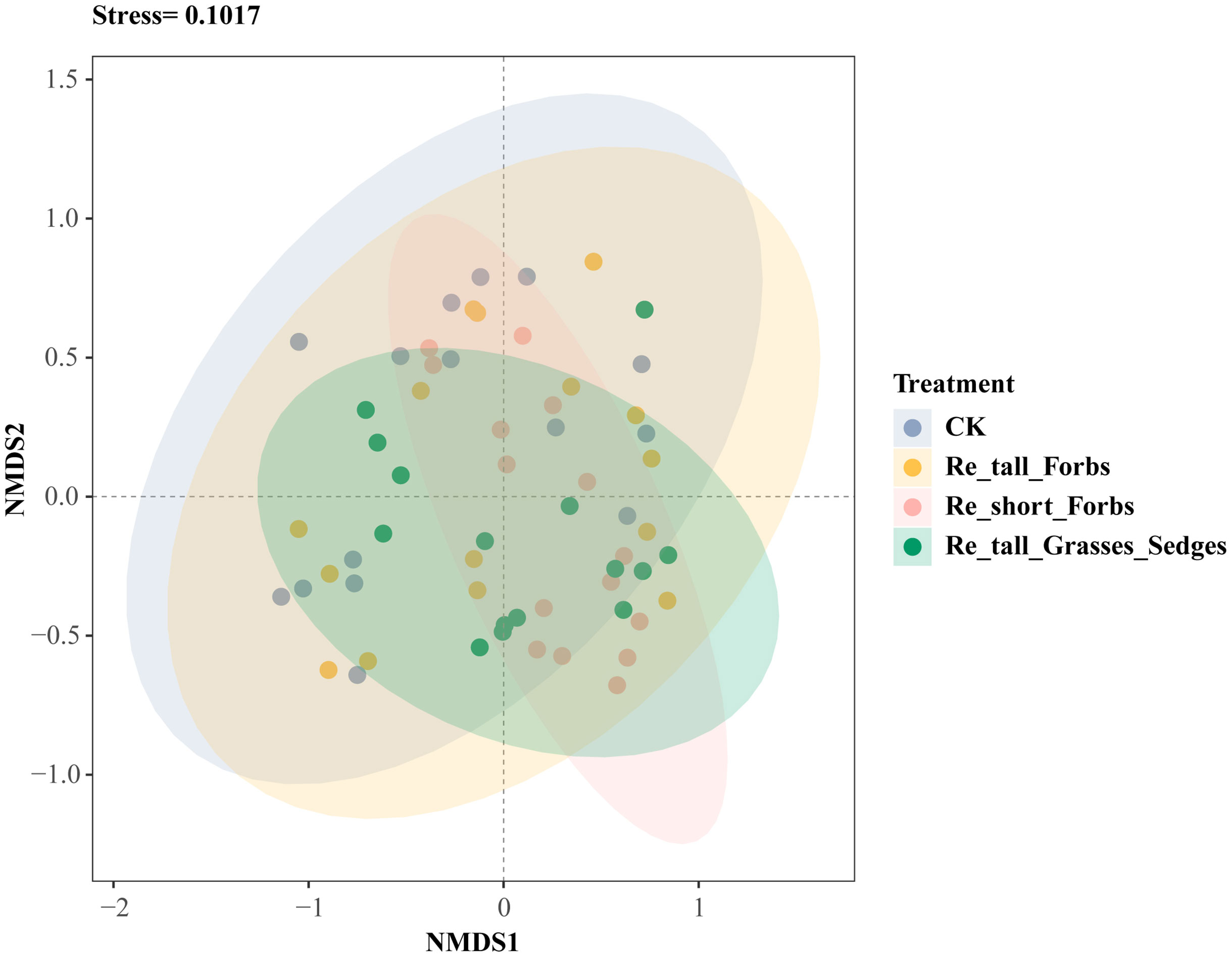
Figure 3 The differences in arthropod species composition between the treatments and the control shown by nonmetric multidimensional scaling analysis (NMDS).
3.2 Effects of plant functional groups removal on the trophic groups of arthropods
As comparing the treatments to the control, the removal of tall and short forbs had a greater effect on the various trophic arthropods compared to the removal of tall grasses and sedges. Specifically, the removal of tall forbs significantly increased herbivore richness and carnivore abundance (Figures 4A, 5B). However, herbivore abundance did not change in the treatment of removing forbs and tall grasses and sedges (Figure 5A). The increase in herbivore richness was a direct effect of the treatment and was not influenced by plant diversity (Figure 6A). Similarly, the variation in carnivore abundance was a direct result of the treatment, as there was no significant relationship between carnivore abundance and herbivore richness or abundance (Figures 6B, C).
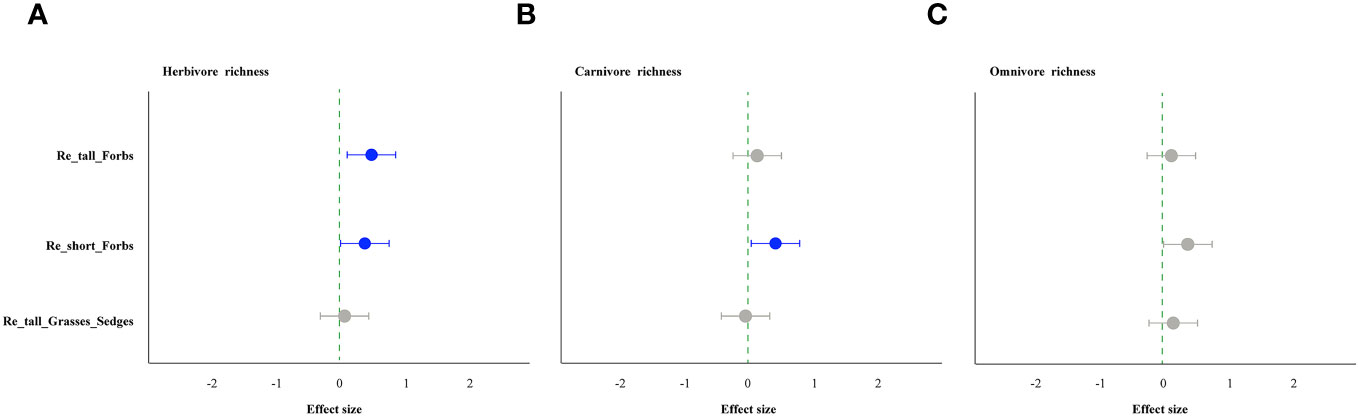
Figure 4 The effect of plant species removal on the richness of arthropod functional groups, as determined by linear mixed-effects models (LMMs). Herbivores (A), carnivores (B), and omnivores (C). The comments in the figure correspond to those shown in Figure 1.
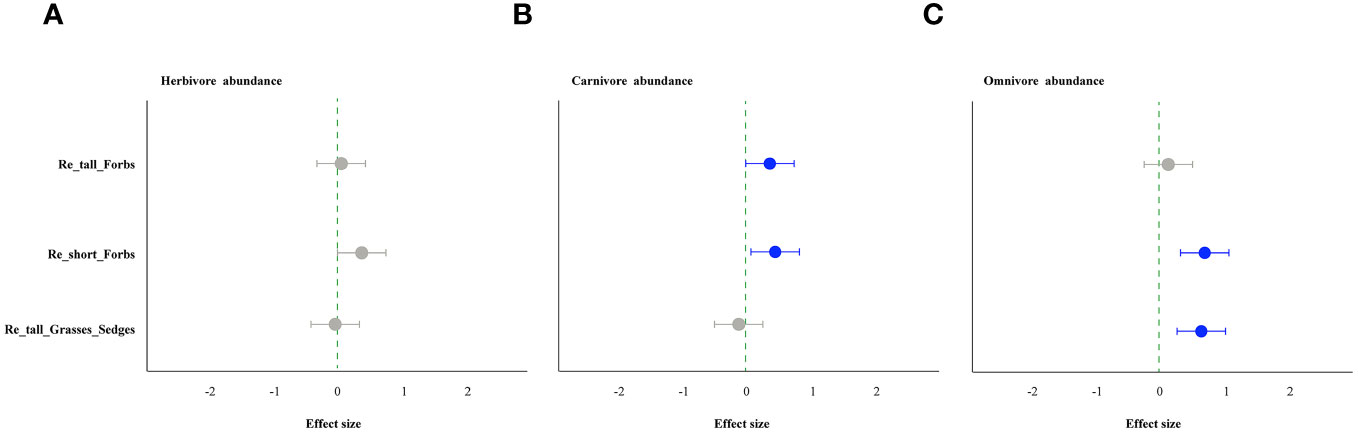
Figure 5 The effect of plant species removal on the abundance of arthropod functional groups by linear mixed-effects models (LMMs). Herbivore (A), carnivore (B), and omnivore (C). The comments in the figure correspond to those shown in Figure 1.
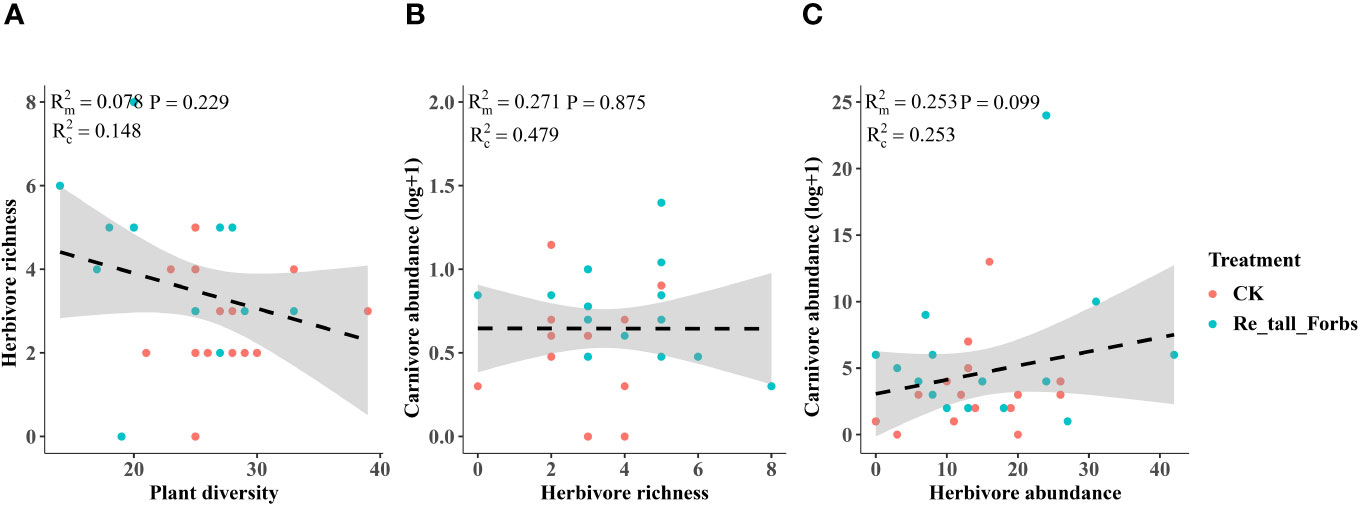
Figure 6 The relationships between plant diversity and the diversity of arthropod functional groups in the removal of tall forbs and the control. Plant diversity and herbivore richness (A), herbivore richness and carnivore abundance (B), the relationship between herbivore abundance and carnivore abundance (C). The figure includes the R2 value and P value of the regression model, along with a 95% confidence interval.
The removal of short forbs significantly increased the richness (Figure 4), the exponential of Shannon entropy (Supplementary Figure S4), inverse Simpson index (Supplementary Figure S5) and inverse Berger-Parker index (Supplementary Figure S6) of herbivores and carnivores, as well as the abundance of carnivores (Figure 5B) and omnivores (Figure 5C). The higher herbivore richness observed was a direct consequence of the treatment, as there was no correlation between herbivore richness and plant diversity (Figure 7A). The increased richness and abundance of carnivores were also direct outcomes of the treatment, as they were not related to herbivore richness and abundance (Figures 7B, C, E). However, the higher abundance of omnivores in the treatment was driven by the increased herbivore richness and abundance, as indicated by their significant correlations (Figures 7D, F). For carnivores in the treatment, the higher the exponential of Shannon entropy was driven by the increased herbivore richness, due to their significant correlation (Supplementary Figure S9). However, the exponential of Shannon entropy of herbivore, inverse Simpson index and inverse Berger-Parker index of herbivores and carnivores were direct outcomes of the treatment, as they were not related to plant diversity and herbivore richness (Supplementary Figure S9).
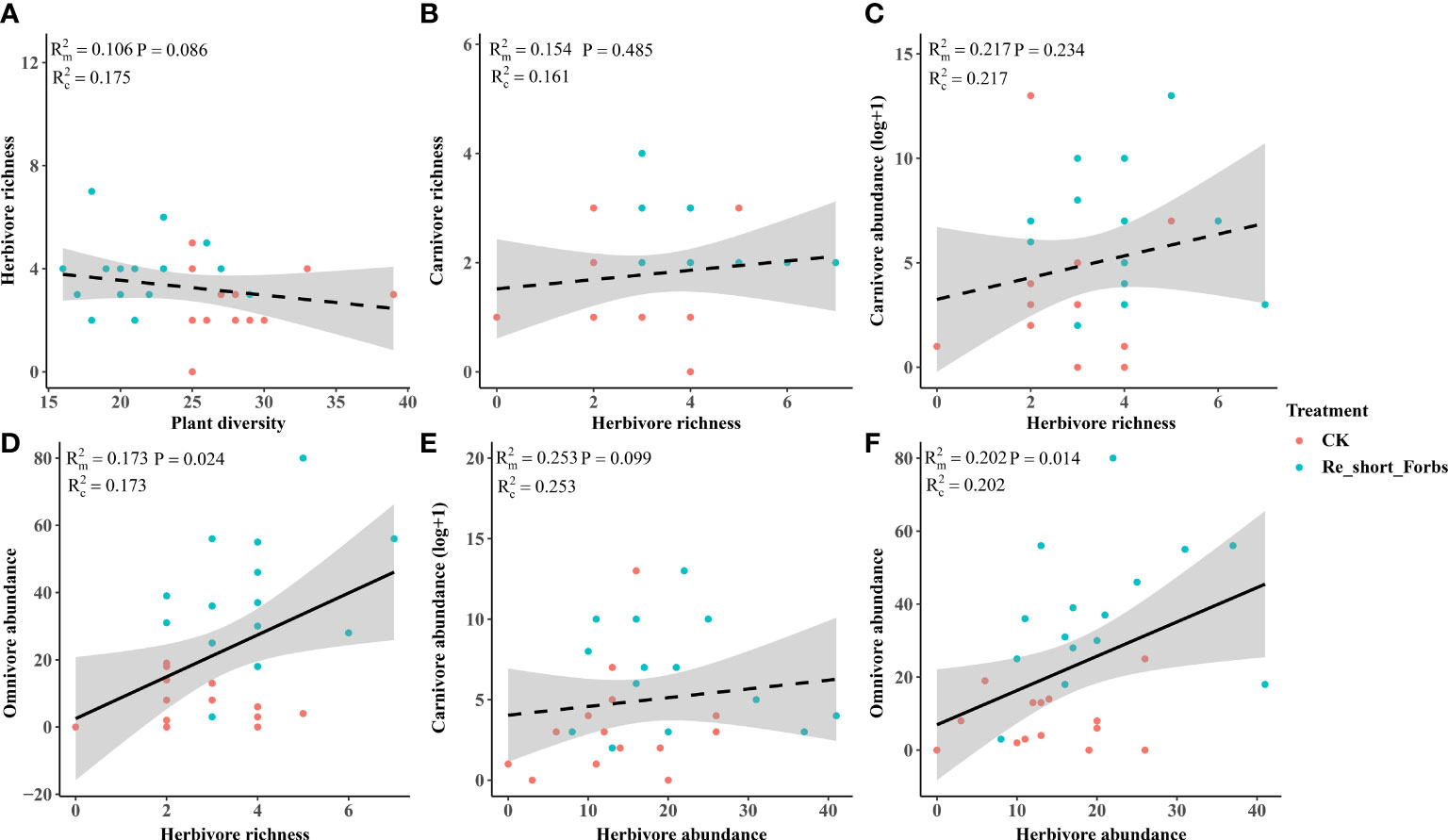
Figure 7 The relationships between plant diversity and arthropod trophic groups in the removal of short forbs and the control. Plant diversity and herbivore richness (A), herbivore richness and carnivore richness (B), herbivore richness and carnivore abundance (C), herbivore richness and omnivore abundance (D), herbivore abundance and carnivore abundance (E), herbivore abundance and omnivore abundance (F). The figure includes the R2 value and P value of the regression model, along with a 95% confidence interval.
In contrast, the removal of tall grasses and sedges only significantly increased the abundance of omnivores (Figure 5C). Furthermore, there was a negative correlation between omnivore abundance and herbivore abundance (Figure 8B).
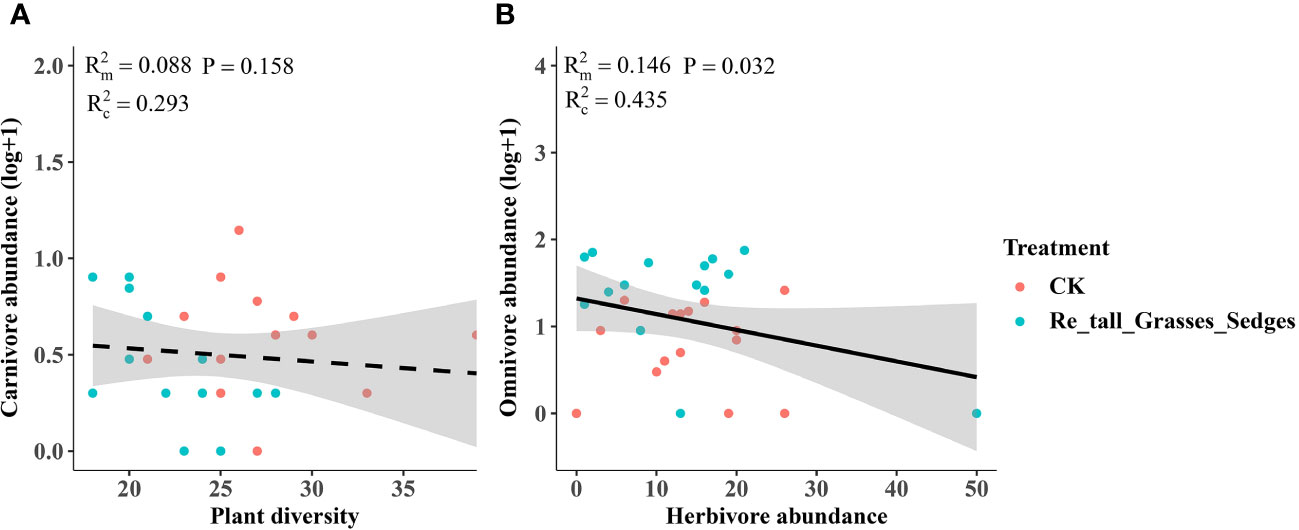
Figure 8 The relationship between plant diversity and the abundance of arthropod functional groups in the removal of tall grasses and sedges and the control. Plant diversity and carnivore abundance (A), herbivore abundance and omnivore abundance (B). The figure includes the R2 value and P value of the regression model, along with a 95% confidence interval.
4 Discussion
A diverse plant community can provide a wide range of resources and higher productivity, which in turn supports a greater diversity of consumers (Strong, 1984; Srivastava & Lawton, 1998). Numerous studies have reported a positive correlation between plant diversity and consumer diversity, including herbivores and carnivores, in manipulating biodiversity experiments (Koricheva et al., 2000; Haddad et al., 2001; Wimp et al., 2004; Crutsinger et al., 2006; Johnson et al., 2006; Haddad et al., 2009; Scherber et al., 2010; Borer et al., 2012; Ebeling et al., 2018). However, our long-term removal experiment yielded unexpected results, as we found that the loss of plant species increased both the richness and abundance of arthropods, especially the number of rare species (exponential of Shannon entropy) of arthropods, contrary to our initial prediction. Additionally, we observed different impacts of plant functional groups on arthropods. Specifically, the removal of forbs (including tall and short) significantly increased arthropod species richness, even higher the exponential of Shannon entropy after removing tall forbs, while the loss of tall grasses and sedges, or short forbs, significantly increased arthropod abundance. These removal treatments also led to changes in the species composition of arthropod communities, despite the absence of an overall correlation between plant diversity and arthropod richness. These findings indicate that high plant species diversity in natural grasslands on the Tibetan Plateau plays a crucial role in controlling the diversity and abundance of arthropods, as well as maintaining interactions within food webs (Barnes and Scherber, 2020).
Furthermore, our study revealed that the removal of different plant groups had varying impacts on arthropods across trophic levels, potentially due to different underlying mechanisms. Diverse plant functional groups can provide distinct food resources for arthropods, thus influencing their composition and diversity. For instance, legumes (e.g. Oxytropis kansuensis, Oxytropis ochrocephala, Tibetia himalaica), which contain high levels of nitrogen, can serve as a high-quality resource for certain herbivores (Kareiva, 1984). In contrast, gramineous plants are known for their low ammonia and hardness levels (Symstad et al., 2000), resulting in lower quality food resources for arthropods (Pinder and Kroh, 1987). Our study found that the removal of forbs had a greater impact on arthropods across different trophic levels compared to the removal of tall grasses and sedges, with differences also observed between tall and short forbs. The loss of both tall and short forbs, rather than tall graminoids, significantly increased herbivore richness (Figure 4A). Specifically, the loss of short forbs significantly increased the diversity of rare and dominant herbivores (Supplementary Figures S4–S6). This contrasts with a previous study that showed some orders of herbivores positively responded to the presence of forbs (Symstad et al., 2000). The observed impact of treatment may not be a result of indirect plant diversity loss, as herbivore richness was not related to plant diversity (Figures 6A, 7A). Instead, this may correspond to the resource concentration hypothesis (Root, 1973), which suggests that specialist herbivores are attracted to their host plants when other forbs are removed, leading to an increase in herbivore richness. This hypothesis is based on the idea that high plant diversity will increase nutrient heterogeneity within the plant community, and high-quality resources will be diluted, leading to a reduction in arthropod diversity (Otway et al., 2005). Additionally, nitrogen levels within plant species were found to be reduced in plant communities with high plant species richness (van Ruijven and Berendse, 2005; Borer et al., 2015), suggesting that plant species nutrition may be lower in communities with high plant diversity. Surprisingly, the removal of tall grasses and sedges did not have a significant impact on herbivores, which is inconsistent with several studies that have shown the presence of grasses significantly decreases herbivory rates or some orders of herbivores (Symstad et al., 2000; Loranger et al., 2014).
Although removing forbs increased herbivore, we did not observe consistent cascading impacts on higher-trophic predators. In the treatment where tall forbs were removed, the increased abundance of carnivores may be a direct result of the treatment, as there was no significant relationship between carnivore abundance and herbivore richness and abundance (Figures 6B, C). On the other hand, the removal of short forbs had a significant impact on high-trophic arthropods, with the richness, abundance, diversity of rare and dominant carnivores and abundance of omnivores being significantly increased by different mechanisms. The increased carnivore abundance may also be a direct result of the treatment, whereas the higher omnivore abundance observed in the treatment was likely a trophic cascading impact caused by the increased herbivore richness and abundance (Figures 7D, F). For carnivores (i.e., rare species) in the treatment, the higher the exponential of Shannon entropy observed was also likely caused by trophic cascading effect as its significant correlation with herbivore richness (Supplementary Figure S9). Similar results have been observed in other studies, where predator abundance and species richness decreased with increasing tree species richness (Schuldt and Assmann, 2011). In our study, the direct impact of removing forbs on carnivores abundance and dominate species diversity of carnivores may be independent of trophic mechanisms mediated by the herbivore community. Instead, changes in abiotic conditions produced by removing forbs in the plant community could be more relevant to predator communities (van Schalkwyk et al., 2019; Tobisch et al., 2023). The removal of forbs, especially short forbs, could decrease structural complexity and increase activity space under the vegetation due to their high cover. This variation in vegetation structure or local habitat conditions may be more important for predators (Brose, 2003; Schaffers et al., 2008; Tobisch et al., 2023), such as enhancing activity and thus predation rate.
In contrast, the removal of tall grasses and sedges significantly increased omnivore abundance rather than carnivore abundance (Figures 5C, 8A). The higher omnivore density observed after removing grasses and sedges may also be a result of changes in microhabitats, in contrast to the treatment of removing short forbs where higher omnivore density was likely caused by the trophic impact of increased herbivores. The increased omnivore abundance in the treatment of removing tall grasses and sedges may be responsible for the control of herbivores, as there was a negative correlation between omnivore abundance and herbivore abundance. This top-down impact may be an important reason why herbivore abundance did not change after removing graminoids. The differential impacts of the three plants groups’ removal suggest that plant species composition, specifically the identity of plant species in the community, is a more important determinant of arthropods in grasslands than plant species richness per se or the number of plant functional groups (Koricheva et al., 2000; Symstad et al., 2000; Loranger et al., 2014).
5 Conclusion
This study demonstrated that the loss of plant functional groups increased arthropod richness and abundance and the diversity of rare arthropod species, which contrasts with the well-reported positive correlation between plant diversity and consumer diversity in manipulating biodiversity experiments, but consistent with agricultural experiments using multi-species mixtures (e.g., reviewed in Andow, 1991). There were differences in the impacts of plant functional group loss, leading to changes in the species composition of arthropod communities. Furthermore, the removal of different plant groups had differential impacts on arthropod trophic levels. More evident impacts of removing forbs rather than graminoids on herbivores did not show consistent cascading impacts on higher-trophic carnivores, possibly due to different underlying mechanisms. Our results highlight the importance of plant species identity as a determinant of arthropods in alpine grasslands, surpassing the significance of plant species richness or functional groups. This study emphasizes the role of high plant species diversity in controlling arthropods in natural grasslands, particularly in the face of plant diversity loss caused by global changes and human activities.
Data availability statement
The raw data supporting the conclusions of this article will be made available by the authors, without undue reservation.
Ethics statement
Ethical approval was not required for the studies involving humans because this research was done jointly by the research team and members of other universities, all of whom are postgraduate students or above. The studies were conducted in accordance with the local legislation and institutional requirements. The participants provided their written informed consent to participate in this study. Ethical approval was not required for the study involving animals in accordance with the local legislation and institutional requirements because this study illustrates the relationship between plants and arthropods, and does not involve large animals and pets.
Author contributions
NL: Conceptualization, Funding acquisition, Project administration, Resources, Supervision, Writing – review & editing. HY: Data curation, Formal analysis, Investigation, Methodology, Project administration, Software, Supervision, Visualization, Writing – original draft. XZ: Project administration, Supervision, Writing – review & editing. YT: Methodology, Supervision, Validation, Writing – original draft. WC: Investigation, Validation, Writing – original draft. QJ: Investigation, Validation, Writing – original draft. YL: Validation, Visualization, Writing – original draft. YC: Data curation, Validation, Writing – original draft. HH: Software, Validation, Writing – original draft. SW: Formal analysis, Investigation, Validation, Writing – original draft.
Funding
The author(s) declare financial support was received for the research, authorship, and/or publication of this article. This work was supported by the National Natural Science Foundation of China (Grant/Award Numbers: 32262270) and the Natural Science Foundation of Gansu Province Team Project (Grant/Award Number: 22JR5RA133).
Acknowledgments
We thank Yanan Li, Wenzhao Hu, Huanghuang Yu and Lize Wang for invaluable help in fieldwork and data collection. We also appreciate Hongjian Wang for help with insect species identification. We are especially grateful to Yuxian Wang, Hui Ma, Ting Yang and Xiaoli Wang for their help during data analysis. We would like to express our gratitude to the editors and reviewers for their constructive comments, which greatly improved the manuscript.
Conflict of interest
The authors declare that the research was conducted in the absence of any commercial or financial relationships that could be construed as a potential conflict of interest.
Publisher’s note
All claims expressed in this article are solely those of the authors and do not necessarily represent those of their affiliated organizations, or those of the publisher, the editors and the reviewers. Any product that may be evaluated in this article, or claim that may be made by its manufacturer, is not guaranteed or endorsed by the publisher.
Supplementary material
The Supplementary Material for this article can be found online at: https://www.frontiersin.org/articles/10.3389/fpls.2024.1305768/full#supplementary-material
References
Abdalla, M., Hastings, A., Chadwick, D. R., Jones, D. L., Evans, C. D., Jones, M. B., et al. (2018). Critical review of the impacts of grazing intensity on soil organic carbon storage and other soil quality indicators in extensively managed grasslands. Agric. Ecosyst. Environ. 253, 62–81. doi: 10.1016/j.agee.2017.10.023
Andow, D. A. (1991). Yield loss to arthropods in vegetationally diverse agroecosystems. Environ. Entomol. 20, 1228–1235. doi: 10.1093/ee/20.5.1228
Barnes, A. D., Scherber, C. (2020). Biodiversity enhances the multitrophic control of arthropod herbivory. Sci. Adv. 6, eabb6603. doi: 10.1126/sciadv.abb6603
Bates, D., Mächler, M., Bolker, B., Walker, S. (2015). Fitting linear mixed-effects models using lme4. J. Stat. Software 67, 1–48. doi: 10.18637/jss.v067.i01
Bernays, E. A., Graham, M. (1988). On the evolution of host specificity in phytophagous arthropods. Ecology 69, 886–892. doi: 10.2307/1941237
Borer, E. T., Lind, E. M., Ogdahl, E. J., Seabloom, E. W., Tilman, D., Montgomery, R. A., et al. (2015). Food-web composition and plant diversity control foliar nutrient content and stoichiometry. J. Ecol. 103, 1432–1441. doi: 10.1111/1365-2745.12461
Borer, E. T., Seabloom, E. W., Tilman, D. (2012). Plant diversity controls arthropod biomass and temporal stability. Ecol. Lett. 15, 1457–1464. doi: 10.1111/ele.12006
Bronstein, J. L., Alarco´n, R., Geber, M. (2006). The evolution of plant–insect mutualisms. New Phytol. 172, 412–428. doi: 10.1111/j.1469-8137.2006.01864.x
Brose, U. (2003). Bottom-up control of carabid beetle communities in early successional wetlands: mediated by vegetation structure or plant diversity? Oecologia 135, 407–413. doi: 10.1007/s00442-003-1222-7
Butchart, S. H. M., Walpole, M., Collen, B., Strien, A., Scharlemann, J., Almond, R., et al. (2010). Global biodiversity: indicators of recent declines. Science 328, 1164–1168. doi: 10.1126/science.1187512
Cardinale, B. J., Duffy, J. E., Gonzalez, A., Hooper, D. U., Perrings, C., Venail, P. (2012). Biodiversity loss and its impact on humanity. Nature 486, 59–67. doi: 10.1038/nature11148
Chao, A., Gotelli, N. J., Hsieh, T. C., Sander, E. L., Ma, K. H., Colwell, R. K., et al. (2014). Rarefaction and extrapolation with Hill numbers: a framework for sampling and estimation in species diversity studies. Ecol. Monographs. 84, 45–67. doi: 10.1890/13-0133.1
Chen, L., Zhao, N., Zhang, L., Gao, Y. (2013). Responses of two dominant plant species to drought stress and defoliation in the Inner Mongolia Steppe of China. Plant Ecol. 214, 221–229. doi: 10.1007/s11258-012-0161-y
Crutsinger, G. M., Collins, M. D., Fordyce, J. A., Gompert, Z., Nice, C. C., Sanders, N. J. (2006). Plant genotypic diversity predicts community structure and governs an ecosystem process. Science 313, 966–968. doi: 10.1126/science.1128326
Currie, D. J. (1991). Energy and large-scale patterns of animal- and plant-species richness. Am. Nat. 137, 27–49. doi: 10.1086/285144
Dassou, A. G., Tixier, P. (2016). Response of pest control by generalist carnivores to local-scale plant diversity: a meta-analysis. Ecol. Evol. 6, 1143–1153. doi: 10.1002/ece3.1917
Díaz, S., Kattge, J., Cornelissen, J. H. C., Wright, I. J., Lavorel, S., Dray, S., et al. (2016). The global spectrum of plant form and function. Nature 529, 167–171. doi: 10.1038/nature16489
Dirzo, R., Young, H. S., Galetti, M., Ceballos, G., Isaac, N. J., Collen, B. (2014). Defaunation in the anthropocene. Science 345, 401–406. doi: 10.1126/science.1251817
Du, Y., Zhao, Y., Dong, S., Chen, G., Wang, X., Ma, K. (2022). The diversity distribution and climatic niche of samara species in China. Front. Plant Sci. 13. doi: 10.3389/fpls.2022.895720
Duffy, J. E., Cardinale, B. J., France, K. E., McIntyre, P. B., Thébault, E., Loreau, M., et al. (2007). The functional role of biodiversity in ecosystems: incorporating trophic complexity. Ecol. Lett. 10, 522–538. doi: 10.1111/j.1461-0248.2007.01037.x
Ebeling, A., Hines, J., Hertzog, L. R., Lange, M., Meyer, S. T., Simons, N. K., et al. (2018). Plant diversity effects on arthropods and arthropod-dependent ecosystem functions in a biodiversity experiment. Basic Appl. Ecol. 26, 50–63. doi: 10.1016/j.baae.2017.09.014
Forister, M. L., Novotny, V., Panorska, A. K., Baje, L., Basset, Y., Butterill., P. T., et al. (2015). The global distribution of diet breadth in insect herbivores. Proc. Natl. Acad. Sci. U.S.A. 112, 442–447. doi: 10.1073/pnas.1423042112
Gamfeldt, L., Hillebrand, H., Jonsson, P. (2005). Species richness changes across two trophic levels simultaneously affect prey and consumer biomass. Ecol. Lett. 8, 696–703. doi: 10.1111/j.1461-0248.2005.00765.x
Gish, M., Mescher, M. C., De Moraes, C. M. (2015). Targeted predation of extrafloral nectaries by insects despite localized chemical defences. Proc. R. Soc. B: Biol. Sci. 282, 20151835. doi: 10.1098/rspb.2015.1835
Griggs, D., Stafford-Smith, M., Gaffney, O., Rockström, J., Öhman, M. C., Shyamsundar, P., et al. (2013). Sustainable development goals for people and planet. Nature 495, 305–307. doi: 10.1038/495305a
Haddad, N. M., Crutsinger, G. M., Gross, K., Haarstad, J., Knops, J. M. H., Tilman, D. (2009). Plant species loss decreases arthropod diversity and shifts trophic structure. Ecol. Lett. 12, 1029–1039. doi: 10.1111/j.1461-0248.2009.01356.x
Haddad, N. M., Tilman, D., Haarstad, J., Ritchie, M., Knops, J. M. H. (2001). Contrasting effects of plant richness and composition on insect communities: a field experiment. Am. Nat. 158, 17–35. doi: 10.1086/320866
Hawkins, B. A., Porter, E. E. (2003). Does herbivore diversity depend on plant diversity? The case of California butterflies. Am. Nat. 161, 40–49. doi: 10.1086/345479
Hooper, D. U., Chapin, F. S., Ewel, J. J., Hector, A., Inchausti, P., Lavorel, S., et al. (2005). Effects of biodiversity on ecosystem functioning: a consensus of current knowledge. Ecol. Monographs. 75, 3–35. doi: 10.1890/04-0922
Hutchinson, G. E. (1959). Homage to Santa Rosalia or why are there so many kinds of animals? Am. Nat. 93, 145–159. doi: 10.1086/282070
Isbell, F., Gonzalez, A., Loreau, M., Cowles, J., Diaz, S., Hector, A., et al. (2017). Linking the influence and dependence of people on biodiversity across scales. Nature 546, 65–72. doi: 10.1038/nature22899
Jacquot, M., Massol, F., Muru, D., Derepas, B., Tixier, P., Deguine, J. (2019). Arthropod diversity is governed by bottom-up and top-down forces in a tropical agroecosystem. Agric. Ecosyst. Environ. 285, 106623. doi: 10.1016/j.agee.2019.106623
Jetz, W., Kreft, H., Ceballos, G., Mutke, J. (2009). Global associations between terrestrial producer and vertebrate consumer diversity. Proc. Biol. Sci. 276, 269–278. doi: 10.1098/rspb.2008.1005
Johnson, M. T., Lajeunesse, M. J., Agrawal, A. A. (2006). Additive and interactive effects of plant genotypic diversity on arthropod communities and plant fitness. Ecol. Lett. 9, 24–34. doi: 10.1111/j.1461-0248.2005.00833.x
Kareiva, P. (1984). Community ecology: insects on plants. Community patterns and mechanisms D. R. Strong, J. H. Lawton, and richard southwood. Harvard university press, cambridge, mass . 1984 vi, 313 pp., illus. $35; paper, $18.95. Science 226, 532–532. doi: 10.1126/science.226.4674.532.a
Klein, J. A., Harte, J., Zhao, X. (2007). Experimental warming, not grazing, decreases rangeland quality on the Tibetan Plateau. Ecol. Appl. 17, 541–557. doi: 10.1890/05-0685
Koricheva, J., Mulder, C., Schmid, B., Joshi, J., Huss-Danell, K. (2000). Numerical responses of different trophic groups of invertebrates to manipulations of plant diversity in grasslands. Oecologia 125, 271–282. doi: 10.1007/s004420000450
Lai, J., Zou, Y., Zhang, S., Zhang, X., Mao, L. (2022). glmm.hp: an R package for computing individual effect of predictors in generalized linear mixed models. J. Plant Ecol. 15, 1302–1307. doi: 10.1093/jpe/rtac096
Leather, S. R. (2018). Ecological Armageddon–more evidence for the drastic decline in insect numbers. Ann. Appl. Biol. 172, 1–3. doi: 10.1111/aab.12410
Legendre, P., Legendre, L. (1998). Numerical ecology. 2nd ed. (Amsterdam, The Netherlands: Elsevier).
Lewinsohn, T. M., Roslin, T. (2008). Four ways towards tropical herbivore megadiversity. Ecol. Lett. 11, 398–416. doi: 10.1111/j.1461-0248.2008.01155.x
Liu, H., Mi, Z., Lin, L., Wang, Y., Zhang, Z., Zhang, F., et al. (2018). Shifting plant species composition in response to climate change stabilizes grassland primary production. Proc. Natl. Acad. Sci. U.S.A. 115, 4051–4056. doi: 10.1073/pnas.1700299114
Liu, J., Zhang, X., Song, F., Zhou, S., Cadotte, M. W., Bradshaw, C. J. (2016). Explaining maximum variation in productivity requires phylogenetic diversity and single functional traits. Ecology 96, 176–183. doi: 10.1890/14-1034.1
Loranger, H., Weisser, W. W., Ebeling, A., Eggers, T., De Luca, E., Loranger, J., et al. (2014). Invertebrate herbivory increases along an experimental gradient of grassland plant diversity. Oecologia 174, 183–193. doi: 10.1007/s00442-013-2741-5
Loreau, M., Hector, A. (2001). Partitioning selection and complementarity in biodiversity experiments. Nature 412, 72–76. doi: 10.1038/35083573
Maes, J., Liquete, C., Teller, A., Erhard, M., Maria Luisa, P., Barredo, J. I., et al. (2016). An indicator framework for assessing ecosystem services in support of the EU Biodiversity Strategy to 2020. Ecosyst. Serv. 17, 14–23. doi: 10.1016/j.ecoser.2015.10.023
Magurran, A. E. (2004). Measuring biological diversity. Curr. Biol. 31, R1174–R1177. doi: 10.1016/j.cub.2021.07.049
Minchin, P. R. (1987). An evaluation of the relative robustness of techniques for ecological ordination. Vegetatio 69, 89–107. doi: 10.1007/BF00038690
Moreira, X., Abdala-Roberts, L., Rasmann, S., Castagneyrol, B., Mooney, K. A. (2016). Plant diversity effects on insect herbivores and their natural enemies: current thinking, recent findings, and future directions. Curr. Opin. Insect Sci. 14, 1–7. doi: 10.1016/j.cois.2015.10.003
Mu, J., Zeng, Y., Wu, Q., Niklas, K. J., Niu, K. (2016). Traditional grazing regimes promote biodiversity and increase nectar production in Tibetan alpine meadows. Agric. Ecosyst. Environ. 233, 336–342. doi: 10.1016/j.agee.2016.09.030
Nagendra, H. (2002). Opposite trends in response for the Shannon and Simpson indices of landscape diversity. Appl. Geogr. 22, 175–186. doi: 10.1016/S0143-6228(02)00002-4
Niu, K., Choler, P., de Bello, F., Mirotchnick, N., Du, G., Sun, S. (2014). Fertilization decreases species diversity but increases functionaldiversity: A three-year experiment in a Tibetan alpine meadow. Agric. Ecosyst. Environ. 182, 106–112. doi: 10.1016/j.agee.2013.07.015
Niu, K., He, J., Lechowicz., M. J. (2016b). Grazing-induced shifts in community functional composition and soil nutrient availability in Tibetan alpine meadows. J. Appl. Ecol. 53, 1554–1564. doi: 10.1111/1365-2664.12727
Niu, K., He, J., Zhang, S., Lechowicz, M. J. (2016a). Grazing increases functional richness but not functional divergence in Tibetan alpine meadow plant communities. Biodiversity Conserv. 25, 2441–2452. doi: 10.1007/s10531-015-0960-2
Otway, S. J., Hector, A., Lawton, J. H. (2005). Resource dilution effects on specialist insect herbivores in a grassland biodiversity experiment. J. Anim. Ecol. 74, 234–240. doi: 10.1111/j.1365-2656.2005.00913.x
Pinder, J. E., III, Kroh, G. C. (1987). Insect herbivory and photosynthetic pathways in old-field ecosystems. Ecology 68, 254–259. doi: 10.2307/1939255
Root, R. B. (1973). Organization of a plant-arthropod association in simple and diverse habitats: the fauna of collards (Brassica oleracea). Ecol. Monographs. 43, 95–124. doi: 10.2307/1942161
Schaffers, A. P., Raemakers, I. P., Sýkora, K. V., Ter Braak, C. J. (2008). Arthropod assemblages are best predicted by plant species composition. Ecology 89, 782–794. doi: 10.1890/07-0361.1
Scherber, C., Eisenhauer, N., Weisser, W. W., Schmid, B., Voigt, W., Fischer, M., et al. (2010). Bottom-up effects of plant diversity on multitrophic interactions in a biodiversity experiment. Nature 468, 553–556. doi: 10.1038/nature09492
Schuldt, A., Assmann, T. (2011). Belowground carabid beetle diversity in the western Palaearctic - effects of history and climate on range-restricted taxa (Coleoptera, Carabidae). Zookeys 100, 461–474. doi: 10.3897/zookeys.100.1540
Siemann, E., Tilman, D., Haarstad, J., Ritchie, M. (1998). Experimental tests of the dependence of arthropod diversity on plant diversity. Am. Nat. 152, 738–750. doi: 10.1086/286204
Srivastava, D. S., Lawton, J. H. (1998). Why more productive sites have more species: an experimental test of theory using tree-hole communities. Am. Nat. 152, 510–529. doi: 10.1086/286187
Strong, M. F. (1984). The third world conservation lecture: Conservation strategies for a changing world. Environmentalist. 4, 29–36. doi: 10.1007/BF02337114
Symstad, A. J., Siemann, E., Haarstad, J. (2000). An experimental test of the effect of plant functional groupdiversity on arthropod diversity. Oikos 89, 243–253. doi: 10.1034/j.1600-0706.2000.890204.x
Thomson, F. J., Letten, A. D., Tamme, R., Edwards, W., Moles, A. T. (2018). Can dispersal investment explain why tall plant species achieve longer dispersal distances than short plant species? New Phytol. 217, 407–415. doi: 10.1111/nph.14735
Tobisch, C., Rojas-Botero, S., Uhler, J., Müller, J., Kollmann, J., Moning, C., et al. (2023). Plant species composition and local habitat conditions as primary determinants of terrestrial arthropod assemblages. Oecologia 201, 813–825. doi: 10.1007/s00442-023-05345-6
Uchida, K., Ushimaru, A. (2014). Biodiversity declines due to abandonment and intensification of agricultural lands: patterns and mechanisms. Ecol. Monographs. 84, 637–658. doi: 10.1890/13-2170.1
van Ruijven, J., Berendse, F. (2005). Diversity–productivity relationships: Initial effects, long-term patterns, and underlying mechanisms. Proc. Natl. Acad. Sci. U.S.A. 102, 695–700. doi: 10.1073/pnas.0407524102
van Schalkwyk, J., Pryke, J. S., Samways, M. J., Gaigher, R. (2019). Congruence between arthropod and plant diversity in a biodiversity hotspot largely driven by underlying abiotic factors. Ecol. applications: Publ. Ecol. Soc. America. 29, e01883. doi: 10.1002/eap.1883
Vogel, G. (2017). Where have all the insects gone? Science 356, 576–579. doi: 10.1126/science.356.6338.576
Wang, S., Duan, J., Xu, G., Wang, Y., Zhang, Z., Rui, Y., et al. (2012). Effects of warming and grazing on soil N availability, species composition, and ANPP in an alpine meadow. Ecology 93, 2365–2376. doi: 10.1890/11-1408.1
Wang, Y., Wesche, K. (2016). Vegetation and soil responses to livestock grazing in Central Asian grasslands: a review of Chinese literature. Biodivers Conserv. 25, 2401–2420. doi: 10.1007/s10531-015-1034-1
Ward, D. F., New, T. R., Yen, A. L. (2001). Effects of pitfall Trap spacing on the abundance, richness and composition of invertebrate catches. J. Insect Conserv. 5, 47–53. doi: 10.1023/a:1011317423622
Westoby, M., Falster, D. S., Moles, A. T., Vesk, P. A., Wright, I. J. (2002). Plant ecological strategies: Some leading dimensions of variation between species. Annu. Rev. Ecol. Evol. Syst. 33, 125–159. doi: 10.1146/annurev.ecolsys.33.010802.150452
Wilson, E. O. (1987). The little things that run the world (The Importance and Conservation of Invertebrates). Conserv. Biol. 1, 344–346. doi: 10.1111/j.1523-1739.1987.tb00055.x
Wimp, G. M., Young, W. P., Woolbright, S. A., Martinsen, G. D., Keim, P., Whitham, T. G. (2004). Conserving plant geneticdiversity for dependent animal communities. Ecol. Lett. 7, 776–780. doi: 10.1111/j.1461-0248.2004.00635.x
Wright, M., Samways, M. (1998). Insect species richness tracking plant species richness in a diverse flora: gall-insects in the Cape Floristic Region, South Africa. Oecologia 115, 427–433. doi: 10.1007/s004420050537
Wu, L., Zhang, Y., Guo, X., Ning, D., Zhou, X., Feng, J., et al. (2022). Reduction of microbial diversity in grassland soil is driven by long-term climate warming. Nat. Microbiol. 7, 1054–1062. doi: 10.1038/s41564-022-01147-3
Zhang, L., Meng, L., Zhang, X., Zheng, S. (2015). “Analysis the influence of grassland degradation in Du Meng County of Heilongjiang Province,” in Proceedings of the 3rd International Conference on Advances in Energy and Environmental Science, Advances in Engineering Research. (Paris: Atlantis Press), 2352–5401. doi: 10.2991/icaees-15.2015.222
Zhou, J. (2019). Response of plant diversity and ecosystem function to plant species removal in an alpine meadow. [dissertation/PhD dissertation]. (Lan zhou (Gansu): Lanzhou University).
Keywords: arthropods, species diversity, arthropod diversity, alpine meadow, functional groups
Citation: Lu N, Yang H, Zhou X, Tan Y, Cai W, Jiang Q, Lu Y, Chen Y, He H and Wang S (2024) The loss of plant functional groups increased arthropod diversity in an alpine meadow on the Tibetan Plateau. Front. Plant Sci. 15:1305768. doi: 10.3389/fpls.2024.1305768
Received: 02 October 2023; Accepted: 02 February 2024;
Published: 16 February 2024.
Edited by:
Jinliang Liu, Wenzhou University, ChinaReviewed by:
Paulo A. V. Borges, University of the Azores, PortugalCibele De Cássia Silva, Universidade Federal de Goiás, Brazil
Copyright © 2024 Lu, Yang, Zhou, Tan, Cai, Jiang, Lu, Chen, He and Wang. This is an open-access article distributed under the terms of the Creative Commons Attribution License (CC BY). The use, distribution or reproduction in other forums is permitted, provided the original author(s) and the copyright owner(s) are credited and that the original publication in this journal is cited, in accordance with accepted academic practice. No use, distribution or reproduction is permitted which does not comply with these terms.
*Correspondence: Ningna Lu, 004321@nwnu.edu.cn
†ORCID: Ningna Lu, orcid.org/0009-0000-1995-6510