- 1College of Agriculture, Yangtze University, Jingzhou, Hubei, China
- 2State Key Laboratory for Zoonotic Diseases, College of Veterinary Medicine, Jilin University, Changchun, Jilin, China
- 3College of Animal Science, Yangtze University, Jingzhou, Hubei, China
Endophytic fungi are important microbial resources for developing novel antibacterial and antifungal drugs to prevent and control crop diseases. Panax notoginseng has been used as a Chinese medicinal herb for a long time, as it has various bioactivities. However, information on endophytic fungi isolated from Panax notoginseng is rare. In this study, an endophytic fungus known as SQGX-6, which was later identified as the golden hair fungus Arcopilus aureus, was isolated from Panax notoginseng. SQGX-6 was extracted using ethyl acetate, and the active components of the fungus were identified using ultra-performance liquid chromatography-mass spectrometry (UHPLC-MS). The antifungal and antioxidant activities of the extract were determined and evaluated in vitro and in vivo. SQGX-6 and its extract inhibited the growth of Corn stalk rot (Fusarium graminearum), Corn southern leaf blight (Helminthosporium maydis), and Tomato gray mold (Botrytis cinerea) in vitro. The free radical scavenging rates for 2,2-Diphenyl-1-pyridinyl hydrazide (DPPH) radical scavenging activity, 3-Ethylbenzothiazoline-6-Sulfonic Acid Radical scavenging (ABTS) activity were also downregulated by the SQGX-6 extract. In vivo, the SQGX-6 extract inhibited the mycelial growth rates of the three aforementioned fungi and downregulated malondialdehyde (MDA) content and upregulated peroxidase (POD) and phenylalanine ammonia-lyase (PAL) content in fruits, leading to significant reduction in damage to cherry tomatoes caused by Botrytis cinerea. UHPLC-MS was performed to identify various active substances, including Alkaloids, Azoles, Benzofurans, Coumarins, Flavonoids, Organic acids, Phenols, and plant growth regulators contained in the extract. These results suggested that the endophytic fungus SQGX-6 of Panax notoginseng and its extract have excellent antifungal and antioxidant activities, and thus, it is an important microbial resource for the developing novel drugs against plant fungal infections.
1 Introduction
Crop diseases caused by bacterial and fungal plant pathogens result in huge economic losses in agriculture every year, which is a major problem that needs to be solved (Rahman et al., 2023). Antibiotics are chemical substances that can prevent or treat diseases caused by bacteria and fungi. However, the overuse of antibiotics has led microbial pathogens to evolve and exhibit resistance to antibiotics, leading to the emergence of drug-resistant “Superbugs” and “Super fungi” around the world. Antibiotic resistance seriously threatens global public health and economic stability (Manganyi and Ateba, 2020). Traditional agricultural chemical bactericides not only contaminate the soil and the environment but also facilitate the selection of antibiotic-resistant bacteria (Nagarajan et al., 2022).
Natural products of microbial origin are an important resource for the development of novel antimicrobial compounds as they have different types of chemical structures and bioactivities (Keller, 2019). Researchers have discovered over 1,500 compounds isolated from fungi between 1993 and 2001, half of which were found to have antibacterial, antifungal, or antitumor activities (Mao et al., 2021). Many researchers in the field of agriculture have screened, identified, and described antimicrobial substances with unique structures and exceptional biological activities from natural products in recent years (Omomowo and Babalola, 2019).
Plant endophytic fungi are microorganisms that live in plant tissues at a certain stage of the life cycle of the plant without causing disease symptoms (Sutton, 1982). Studies have shown that many endophytic fungi stimulate the growth of the host plant, including promoting the growth of medicinal plants, enhancing tolerance to stress, facilitating the accumulation of bioactive metabolites, and substituting for active components of medicinal plants (Aly et al., 2010). Endophytic fungi cultivated from medicinal plants can produce antifungal substances, such as Terpenoids (Liu et al., 2019), Saponins (Yan et al., 2019), Steroids (Hu et al., 2017), Alkaloids (Cui et al., 2017), Peptides (Latz et al., 2018), and Aromatics (Tanney et al., 2016). Therefore, studying endophytic fungi in medicinal plants might help researchers discover new antifungal drugs. Panax notoginseng, also known as “Tian Sanqi” or “Shen Sanqi”, is a valuable traditional Chinese medicinal herb. It was first reported in the “Compendium of Materia Medica” and its roots have hemostatic effects (Jiang et al., 2022). They can also promote blood circulation, reduce swelling, and provide pain relief (Sun et al., 2010). Panax notoginseng also has antioxidant, anti-diabetic, and antitumor activities (Xie et al., 2017). However, reports on endophytic fungi isolated from Panax notoginseng are rare.
Medicinal plants and their associated microflora are important resources for the development of antioxidant products, and antifungal and anticancer drugs (Luo et al., 2023; Yang et al., 2023). However, information on endophytic fungi associated with the medicinal plant Panax notoginseng is limited. In this study, we used Panax notoginseng cultivated in Yunnan, China, as a material to isolate and identify endophytic fungi with antimicrobial properties in plants. An endophytic fungus, Arcopilus aureus SQGX-6, from Panax notoginseng was extracted using ethyl acetate, and its antifungal and antioxidant activities against pathogenic fungi were evaluated in vitro and in vivo. These results can not only enrich the microbial strain resource bank but also provide insights into the use of green micro-ecology to prevent and control plant diseases and contribute to the development of new natural antifungal products.
2 Materials and methods
2.1 Isolation of endophytes
Endophytes were isolated following the method described in another study (Doin de Moura et al., 2021). Panax notoginseng is a perennial herbaceous plant that belongs to the family Panaxaceae. We collected five fresh three-year-old Panax notoginseng from Yanshan County, Wenshan Zhuang and Miao Autonomous Prefecture, Yunnan Province, China (23.60° N and 104.33° E) and stored them in fresh-keeping bags for 48 h. Then, they were sent to the laboratory for treatment. More information on Panax notoginseng is provided in Supplementary Figure S1. The roots were rinsed with 75% ethanol for 30 s, and then, rinsed thrice with sterile water. After another rinsing with a 3% sodium hypochlorite solution for 2 min, the roots were washed with sterile water five times. The roots were placed on sterile absorbent filter paper and inoculated with sterile forceps onto a potato dextrose agar (PDA) medium until single fungal colonies were obtained.
2.2 Identification of the endophyte SQGX-6
The morphological characteristics of the isolated fungal colonies were analyzed using a light microscope and a scanning electron microscope after the colonies were grown at 28°C for seven days on a PDA medium (Wang et al., 2016). For identification of SQGX-6, specific primers ITS1 (5′-TCCG TAGGTGAACCTGCGG-3′) and ITS4 (5′- TCCTCCGCTTATTGATATGC -3′) were designed, based on the conserved sequences of some known fungi, for PCR (Matar et al., 2022; Nartey et al., 2022). The PCR products were sequenced (Sangon, Shanghai), and the sequencing results were BLAST searched to identify homologs in the NCBI database.The phylogenetic tree was constructed using the N-J method in MEGA-X (Vidal et al., 2020). The sequence was submitted to GenBank (accession number OR053652.1). The fungal strain SQGX-6 was deposited in the China Center for Type Culture Collection (CCTCC) on May 11, 2021, at Wuhan University, Wuhan, Hubei Province, China, with the deposit number of CCTCCM 2022601.
2.3 Plate confrontation method
The antifungal activity of SQGX-6 was assessed using the plate confrontation method (Vidal et al., 2020). The source of the pathogenic fungi F. graminearum, H. maydis, and B. cinerea used in this study was donated by Zhang Xiaolei, an associated professor of the College of Agriculture, Yangtze University. An agar plug from a five-day-old fungal pathogen colony was placed on one side of a Petri dish (90 mm diameter), with an agar plug of each SQGX-6 placed on the opposite side, 5 cm from the pathogen. PDA plates inoculated with the pathogen alone were used as the control. The opposing plates were placed in an incubator at 28°C and constant humidity for seven days in the dark. The colony diameter of the pathogen was measured after seven days and compared to the control. The inhibition percentage was calculated according to the following formula:
Here, Ri indicates the distance between the radial mycelial growth of the pathogenic fungus and the endophytic fungus and Rc indicates the distance between the radial mycelial growth of the pathogenic fungus on the control plate (Chen et al., 2018; Kim et al., 2023). The experiment was repeated three times.
2.4 Preparation of endophytic fungal culture medium and ethyl acetate extract
The endophytic fungal culture medium and ethyl acetate extract were prepared based on previous reports. Five pieces of SQGX-6 of a similar size were collected using a drill (6 mm), placed in a PDB liquid medium (50 mL/250 mL) as seed, and cultivated at 28°C for seven days. After cultivation, the seed was inoculated in a 10% ratio in a PDB liquid medium (500 mL/1000 mL) at 28°C for another 7–10 days. The culture medium and mycelium were filtered using a Brinell funnel and extracted thrice with ethyl acetate (3 × 500 mL). The upper phase was collected, decompressed and concentrated using a rotary evaporator. After dissolving with trace methanol, the concentrated solution was vacuum-frozen and dried in a 10 mL ampoule bottle for 72 h, and then, stored in a refrigerator at 4°C (Aqueveque et al., 2017; Zeng et al., 2019).
2.5 Determination of the antioxidant activity of the SQGX-6 extract
The SQGX-6 extract was adjusted to five different concentrations (60, 80, 100, 200, and 400 μg/mL) for the antioxidant activity assays: 2,2-Diphenyl-1-pyridinyl hydrazide (DPPH) radical scavenging activity, 3-Ethylbenzothiazoline-6-Sulfonic Acid Radical scavenging (ABTS) activity, and superoxide anion radical (O2−) scavenging activity; ascorbic acid (VC) was used as a positive control (Li et al., 2022a; Wu et al., 2022). The equations used to evaluate the different scavenging activities are as follows:
Here, A0 indicates the absorbance of the control group, and A1 indicates the absorbance of the extraction solution/standard.
Here, A indicates the absorbance of ABTS and B indicates the absorbance of the combination of ABTS and the test sample.
Here, A0 indicates the absorbance of pyrogallol, and A1 indicates the absorbance of the sample after 5 min of reaction.
2.6 Growth rate inhibition analysis
The antifungal effects of the three pathogenic fungi were evaluated by the growth rate method (Xu et al., 2021; Qi et al., 2022; Zhang et al., 2022). The pathogenic fungal agar plugs (6 mm in diameter) were placed at center of each PDA plate and different concentrations of SQGX-6 extract (8 µg/mL, 16 µg/mL, 32 µg/mL, 64 µg/mL, 128 µg/mL, 256 µg/mL, and 512 µg/mL) were added. The plates were incubated at 28°C in a constant temperature and humidity chamber for seven days, using sterile distilled water as the negative control. The diameter of each colony was measured when the negative control dish was filled with fungi. The inhibition rate of the extract on mycelial growth was calculated using the following equation:
2.7 Application of SQGX-6 in plant disease control
The bacteriostatic effect of the SQGX-6 extract was examined in vivo as described in another study (Mariana Medina-Romero et al., 2017). Cherry tomatoes (n = 20; diameter: 2–3.5 cm; weight: 7–9 g) with no diseases or wounds in the local area were selected. They exhibited no visible signs of diseases or wounds on their surfaces. The tomato surface were disinfected with 75% ethanol for 2 min, rinsed thrice with sterile distilled water for 60 s each, and then, air-dried on a horizontal flow clean bench. A punch was used to create a hole (4 mm in depth and 6 mm in diameter) in the center of each tomato fruit. Subsequently, 20 µL of SQGX-6 extract at different concentrations (16 µg/mL, 64 µg/mL, and 256 µg/mL) were injected into each wound, with sterile distilled water used as the negative control. After the samples were placed for 1 h in the horizontal flow clean, each wound was inoculated with 20 μL (1 × 105 spores/mL) of gray mycosis spore suspension, forming the control group. Five tomatoes were selected in each treatment group. After air drying at room temperature, the tomatoes were placed in a light incubator, where they were exposed to 12 h of fluorescence and 12 h of dark light, and stored at 25°C for eight days. The diameter of each colony of the gray mold fungal pathogen on tomatoes was measured vertically using a Vernier caliper every two days. Cherry tomato samples were collected after lesions and seeds were removed, and they were flash-frozen using liquid nitrogen. The inhibition rate was calculated using the following equation:
, where A and B represent the diameter of tomato lesions in the positive control samples and different treatment groups, respectively.
2.8 Disease resistance induction assay
After inoculation with 16 µg/mL, 64 µg/mL, and 256 µg/mL of SQGX-6 extract, cherry tomatoes were collected for defense-associated enzyme activity assays. Three enzymes, including malondialdehyde (MDA), peroxidase (POD) and phenylalanine ammonia-lyase (PAL) were tested (Li et al., 2019). Images were taken every two days. About 0.1 g of cherry tomato tissue was taken and 1 mL of the extract was added and homogenized in an ice bath. After grinding, the homogenate was centrifuged at 8,000 g at 4°C for 10 min and the supernatant was collected and placed on ice for analysis. The activities of MDA, POD and PAL were measured using MDA, POD, and PAL activity kits (Solebro, Beijing), respectively, following the instructions of the manufacturers. The contents of MDA were expressed in nmol/g mass, and the activities of POD and PAL were expressed in U/g FW.
2.9 Identification of the components in antifungal compounds
Ultra-performance liquid chromatography-mass spectrometry (UHPLC-MS) (Sciex Exion LC AD and QTrap 6500+) was performed to analyze the chemical composition of the endophytic fungal SQGX-6 extract. In total, 20 mg of the sample was extracted in a centrifuge tube using 500 μL of a pre-cooled methanol solution (methanol: water = 3:1, v/v, including an internal standard) and incubated at 4°C overnight. After the samples were centrifuged for 15 min at 4°C and 12,000 rpm, the supernatant was collected and filtered through a 0.22 µm filter (Millipore, USA). The flow-through was diluted in 10-fold with a methanol solution and stored at –80°C until further analysis. The liquid chromatography mobile phase conditions are shown in Supplementary Table S1. The samples were separated by UHPLC with an EXIONLC System (Sciex). Mobile phase A consisted of 0.1% formic acid in water, and mobile phase B consisted of acetonitrile. The column temperature was maintained at 40°C. The autosampler temperature was maintained at 4°C, and the injection volume was 2 μL. All buffers or solutions used were MS grade.
2.10 Statistical analysis
All experiments were conducted in triplicate, and the results were expressed as the mean ± standard deviations (SD). The differences between different groups of data were determined by conducting Duncan’s multiple comparison test using SPSS 17.0 (SPSS Inc., Chicago, USA). All differences between groups were considered to be statistically significant at P< 0.05.
3 Results
3.1 Isolation and screening of antagonistic endophytic fungi
After washing the roots and root tips of Panax notoginseng, the water used to rinse the parts for the last time was collected and cultivated on the PDA plates for seven days. No microbial growth was occurred on the surface of the PDA plates, which indicated that the surface of the Panax notoginseng roots and root tips were sterilized effectively (data not shown). Subsequently, the roots were placed on a PDA medium and cultivated until fungal colonies were obtained. In total, 24 endophytic fungi were isolated from Yanshan County, Wenshan Zhuang and Miao Autonomous Prefecture, Yunnan Province, China. To screen for the endophytic fungi with antifungal properties, the 24 fungi isolated were co-cultured with pathogenic fungi. We found that one of the fungi showed considerably higher antifungal activity than the others and designated it as SQGX-6 (Supplementary Figure S2).
We found that the isolate SQGX-6 had typical morphological characteristics of endophytic fungi. After 15 days of culture, the colony showed a cotton-like morphology, ranging in color from orange-yellow to red, with inconspicuous alternating orange-yellow and red concentric rings, which appeared as a wheel (Figures 1A, B). Secreted brown-red pigments and mature teardrop-shaped conidia were also observed (Figure 1C). Under the light microscope, dense mycelium and spores of SQGX-6 were observed (Figure 1D). Scanning electron microscopy (SEM) images of SQGX-6 showed the three-dimensional structure of the mycelial clusters, and the fungal hyphae were prominent. The hyphae had a uniform thickness, were separated regularly like bamboo joints, and were arranged in a rope-like manner (Figures 1E, F). We also found straight or curly branches that gradually tapered to the tip.
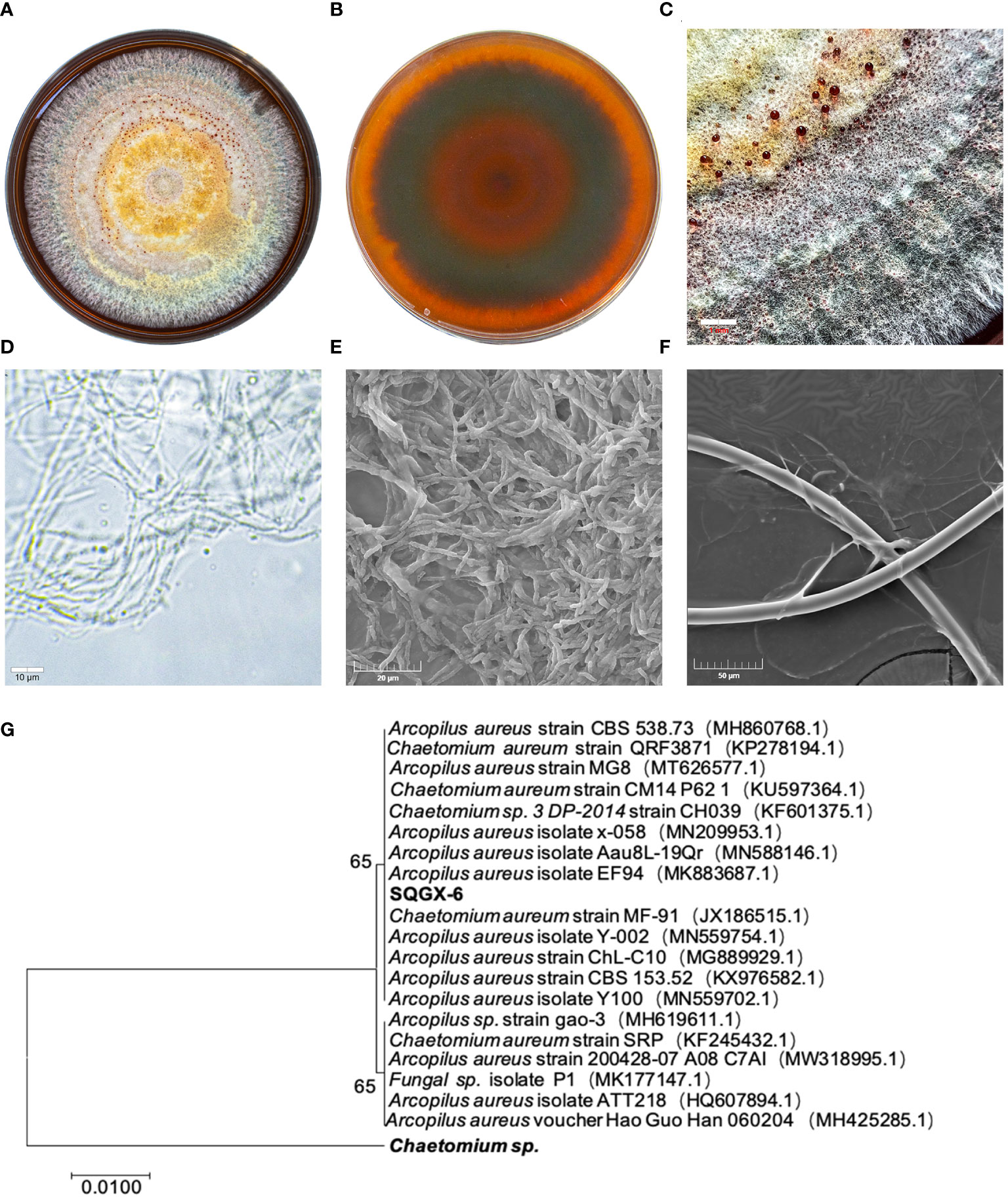
Figure 1 Morphological characteristics of the SQGX-6 strain were assessed using PDA medium and evaluation of growth via scanning electron microscopy, molecular biological identification and phylogenetic tree construction. (A) Front side of the SQGX-6 endophytic fungus after 15 days. (B) Back side view of the SQGX-6 endophytic fungus at the same age. (C) Partial enlarged view of the SQGX-6 Endophytic Fungus (bar = 1 mm). (D) Features observation of SQGX-6 mycelia under an optical microscope (bar = 10 μm). (E, F) SEM images of SQGX-6, with scale bars of 20 μm in (E) and 50 μm in (F). (G) Phylogenetic evolution of Panax notoginseng endophytic fungus based on the ITS sequence.
To confirm the phylogenetic relationship, a phylogenetic tree was constructed using the MEGA-X software, and SQGX-6 was compared to its closely related strains. The homology with Arcopilus aureus was 100% (Figure 1G). Thus, this endophytic fungus belonged to the phylum Ascomycetes, class Sordariomycetes, order Sordariales, family Chaetomiaceae, and genus Arcopilus.
3.2 SQGX-6 inhibited the growth of pathogenic fungi
To determine whether the endophytic fungus SQGX-6 has the antifungal effects, three common crop pathogenic fungi, including Corn stalk rot (Fusarium graminearum, F. graminearum), corn southern leaf blight (Helminthosporium maydis, H. maydis), and Tomato gray mold (Botrytis cinerea, B. cinerea), were co-cultured with SQGX-6 using the plate confrontation method. We found that SQGX-6 inhibited the growth of F. graminearum, H. maydis and B. cinerea, resulting in the atrophy of the pathogenic fungal mycelium after seven days of cultivation on PDA plates (Figure 2A). Compared to the inhibition rate in the control group, the inhibition rate of SQGX-6 against the growth of the three pathogenic fungi exceeded 50%, the inhibition rate against B. cinerea was the highest (74.07%), and the inhibition rates against the other two pathogenic fungi were 66.61% and 61.30%, respectively (Figure 2B). These results suggested that SQGX-6 can strongly inhibit fungi and is a promising antifungal agent.
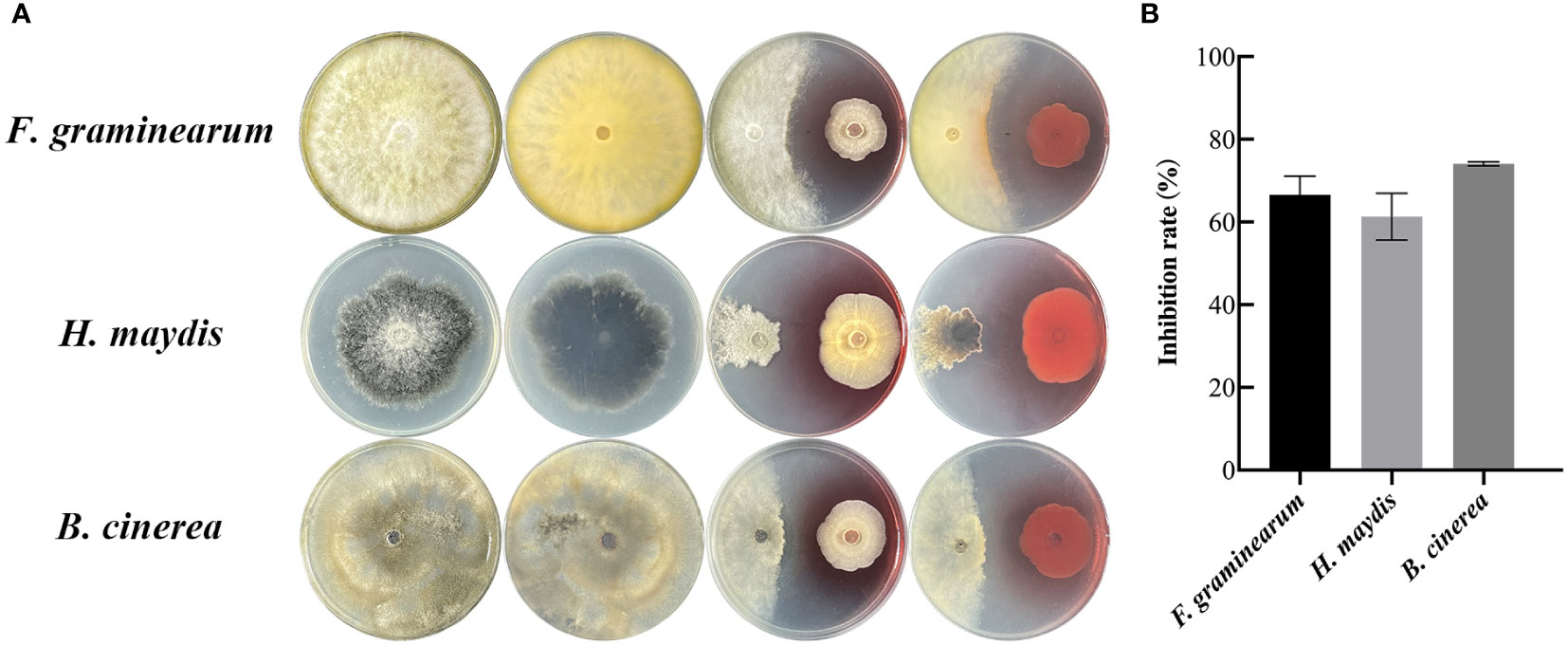
Figure 2 Confrontation experiment between the endophytic fungus SQGX-6 and three other pathogenic fungi. (A) Corn stalk rot (Fusarium graminearum), corn southern leaf blight (Helminthosporium maydis), and tomato gray mold (Botrytis cinerea). (B) Antifungal activity of SQGX-6 against these three pathogenic fungi.
3.3 SQGX-6 extract showed antioxidant activity
To evaluate whether the SQGX-6 extract has antioxidant activity, the DPPH radical scavenging test, ABTS free radical scavenging test, and superoxide anion radical scavenging test were performed. DPPH is commonly used to evaluate the free radical scavenging effects of various antioxidants. The results showed that the SQGX-6 extract exhibited strong antioxidant activity in a dose-dependent manner compared to the control, where the highest DPPH radical scavenging rate was 72.23% at 400 μg/mL (Figure 3A). The SQGX-6 extract also showed a strong ABTS scavenging activity. The ABTS scavenging activity was above 80% when the concentration of the SQGX-6 extract was 60–400 μg/mL. This activity was the same as that recorded in the VC control group (Figure 3B). The scavenging activity on the superoxide anion radicals increased along with the concentration of the SQGX-6 extract. The highest scavenging rate of superoxide anion radicals was 35.90% when the concentration of the SQGX-6 extract was 400 μg/mL (Figure 3C). These results showed that the SQGX-6 extract had a high antioxidant activity.
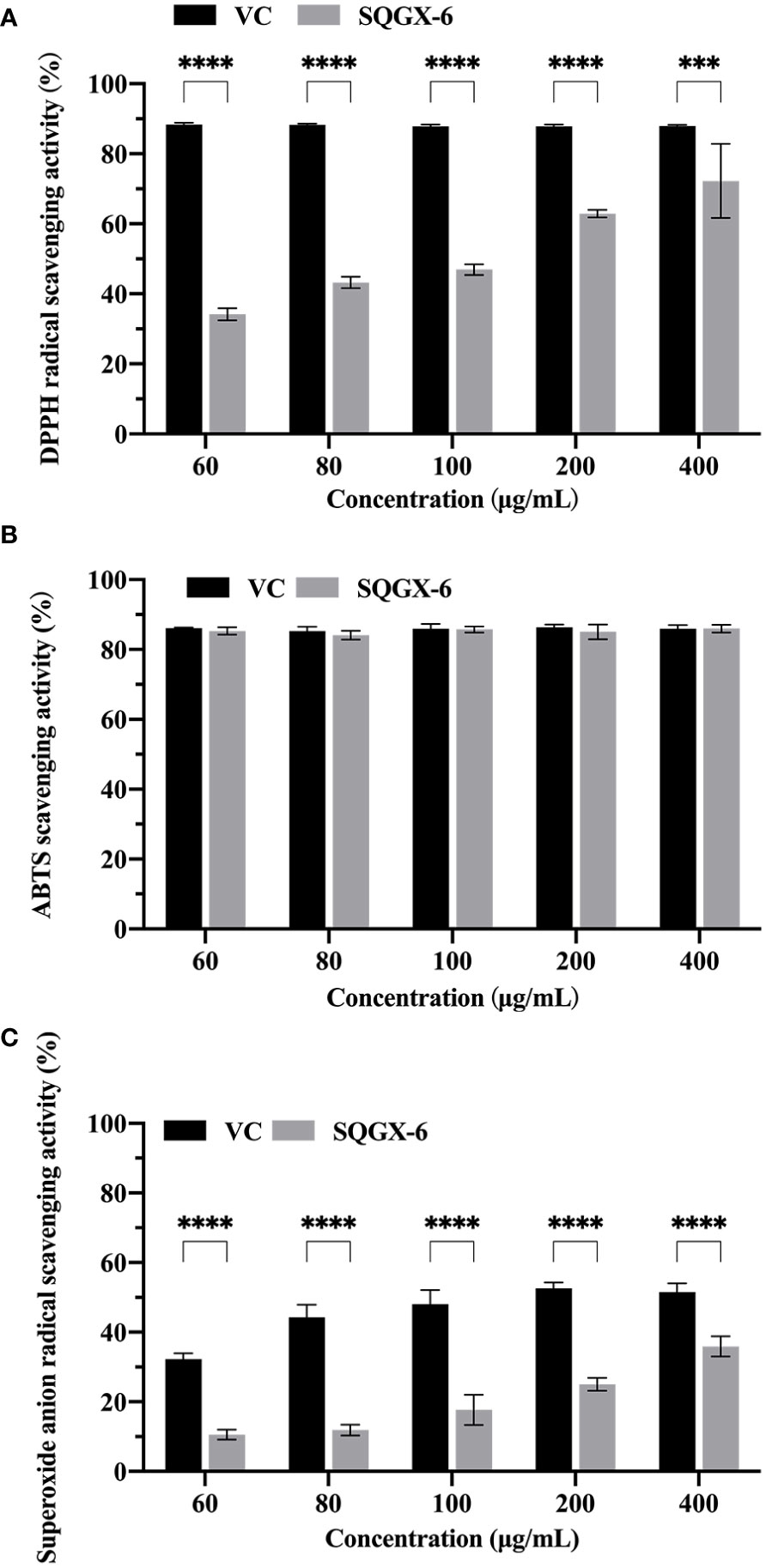
Figure 3 Antioxidant activity of the SQGX-6 extract. (A) DPPH radical scavenging activity. (B) ABTS radical scavenging activity. (C) Superoxide anion radical scavenging activity. Notably, a significant difference was observed between the groups (P< 0.001); ***: P<0.001; ****: P<0.0001.
3.4 SQGX-6 extract had antifungal effects on pathogenic fungi
To analyze the effect of the SQGX-6 extract on the growth of crop pathogenic fungi, including F. graminearum, H. maydis and B. cinerea, the pathogenic fungi were cultured on PDA plates with different concentrations of the SQGX-6 extract, and the growth rate was determined by measuring the colony diameters. The SQGX-6 extract inhibited the growth of the three pathogenic fungi (Figure 4), which matched the results of the SQGX-6 confrontation experiment. As the concentration and action time of the extract increased, its inhibitory effect on the growth of F. graminearum increased. At a concentration of 256 μg/mL, the extract completely inhibited the growth of F. graminearum. At 24 h, the inhibition rate of the extract was 66.3% (Figures 4A, B), which increased to 90.13% at 168 h (Figure 4C). The diameter and growth inhibition rate of H. maydis were positively correlated with the concentration and action time of the SQGX-6 extract. At 24 h, the diameter of on H. maydis after treatment with 512 μg/mL of the extract was 6.47 mm, and the inhibition rate was 23.62%. At 144 h and 168h, 512 μg/mL of the extract had similar inhibition rates of 57.19% and 58.67%, respectively for H. maydis (Figures 4D, F). Similar results were observed for the fungus B. cinerea. At 72 h, the inhibition rate of the 512 μg/mL of the extract against B. cinerea was 63%. At 120 h, the inhibition rate was the highest at 83.30%. However, the inhibition rate decreased slightly after 120 h, with rates of 82.02%, 81.49%, and 79.08% recorded at 144 h, 168 h, and 192 h, respectively (Figures 4G–I). These results indicated that the SQGX-6 extract can inhibit the growth of pathogenic fungi.
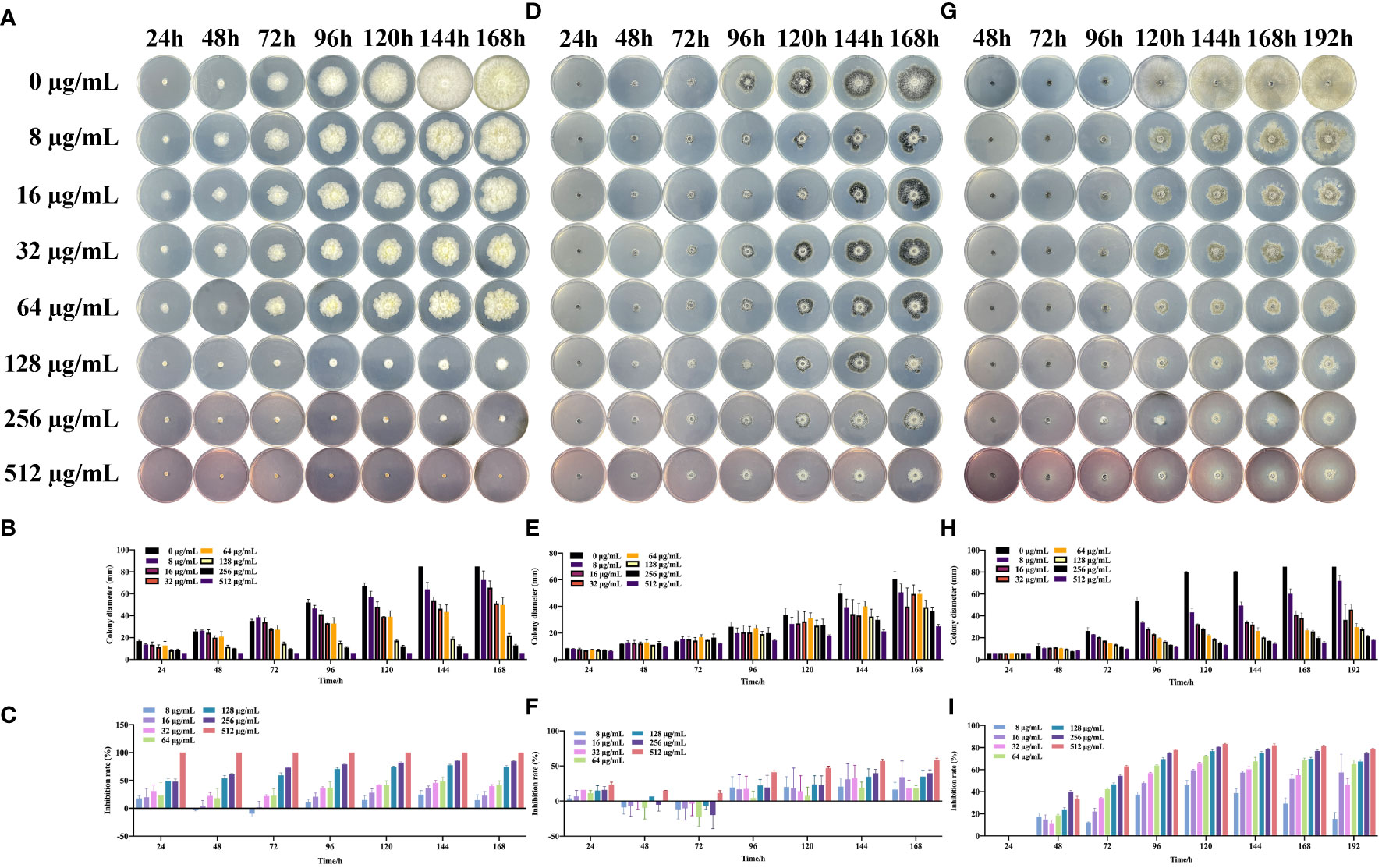
Figure 4 Effects of the SQGX-6 extract on the mycelial growth of three pathogenic fungi. Colony diameters were measured daily over a 7-day period, and the graph’s indicated concentration is the final concentration after the extract was added to the PDA plate. Displayed data represent images from triplicate replicates. (A–C) represent F. graminearum; (D–F) to H. maydis; (G–I) to B. cinerea.
3.5 SQGX-6 extract inhibited the rotting of artificially damaged cherry tomatoes
After cultivation for eight days at 25°C, no fungi were observed in cherry tomatoes (0 μg/mL) without B. cinerea, whereas many fungi were observed in cherry tomatoes inoculated with B. cinerea alone (Figure 5A). Although large areas of fungi were found in the B. cinerea infection group, the growth of B. cinerea was substantially inhibited by the SQGX-6 extract in a dose dependent manner. Among which, the most prominent inhibitory effect was observed at 64 μg/mL, the inhibition rate was 67.42%, and the diameter of the fungus was only 4.42 mm (Figure 5B). At 48 h, the lesion area of tomatoes in the B. cinerea infection group was 13.89 mm in diameter. A higher concentration of the extract significantly alleviated the degree of rot caused by B. cinerea compared to the effect of a lower concentration of the extract on the degree of rot (Figure 5C). These results showed that treatment with the SQGX-6 extract can inhibit postharvest B. cinerea infection in cherry tomatoes.
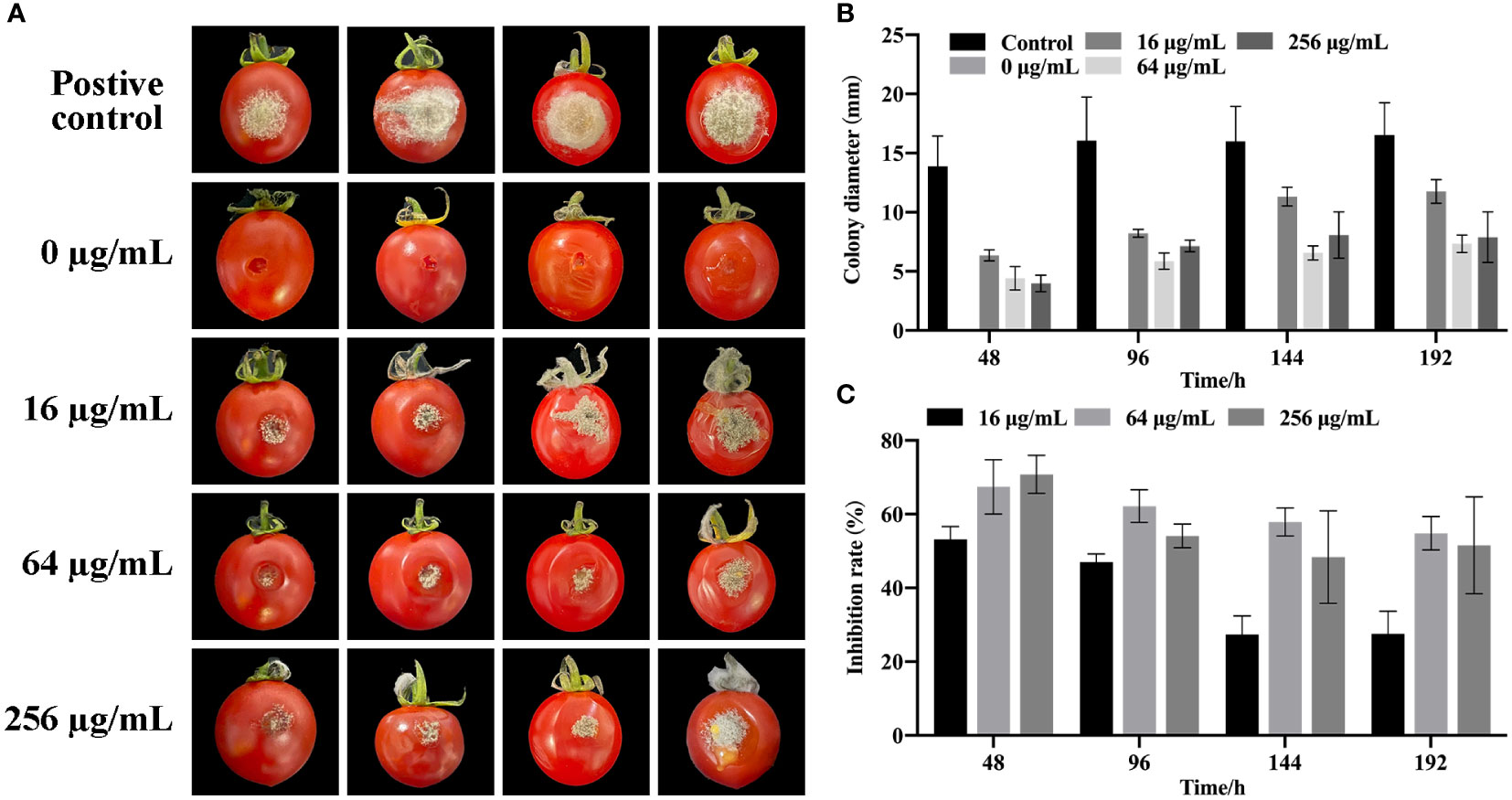
Figure 5 Impact of SQGX-6 treatment on B. cinerea infection in postharvest cherry tomatoes. (A) Inoculation of cherry tomato fruits with a 20 μL B. cinerea spore suspension at a concentration of 1×105 spores/mL, followed by SQGX-6 treatment at various concentrations (0 μg/mL, 16 μg/mL, 64 μg/mL, or 256 μg/mL) and storage at 25°C. Lesion diameters and disease incidence were recorded on the 8th day post-inoculation. The vertical bar signifies the standard deviation of the mean (n = 5). (B) Diameter of SQGX-6 extracts’ inhibitory effect on postharvest B. cinerea growth in cherry tomatoes. (C) Inhibition rate of SQGX-6 extract treatment on postharvest B. cinerea infection of cherry tomatoes.
3.6 SQGX-6 extract increased the resistance of cherry tomatoes infected with B. cinerea
To elucidate the mechanism underlying the antifungal effects of the SQGX-6 extract, the effects of the SQGX-6 extract on the contents of MDA, POD and PAL in cherry tomatoes were further investigated. The results showed that the MDA content of infected cherry tomatoes changed significantly after treatment with 16 μg/mL, 64 μg/mL and 256 μg/mL of the extract, compared to the MDA content in the control group. The MDA content in the control group continued to increase after tomato inoculation, but it did not increase in the groups that received different concentrations of the SQGX-6 extract. Thus, the accumulation of MDA in the SQGX-6 extract treatment group was significantly slower than that in the control group (Figure 6A). At 192 h post-infection, the MDA content in the group treated with 256 μg/mL of the extract was approximately 16-fold lower than that in the control group. These results showed that treatment with the SQGX-6 extract significantly decreased the MDA content in cherry tomatoes after harvest.
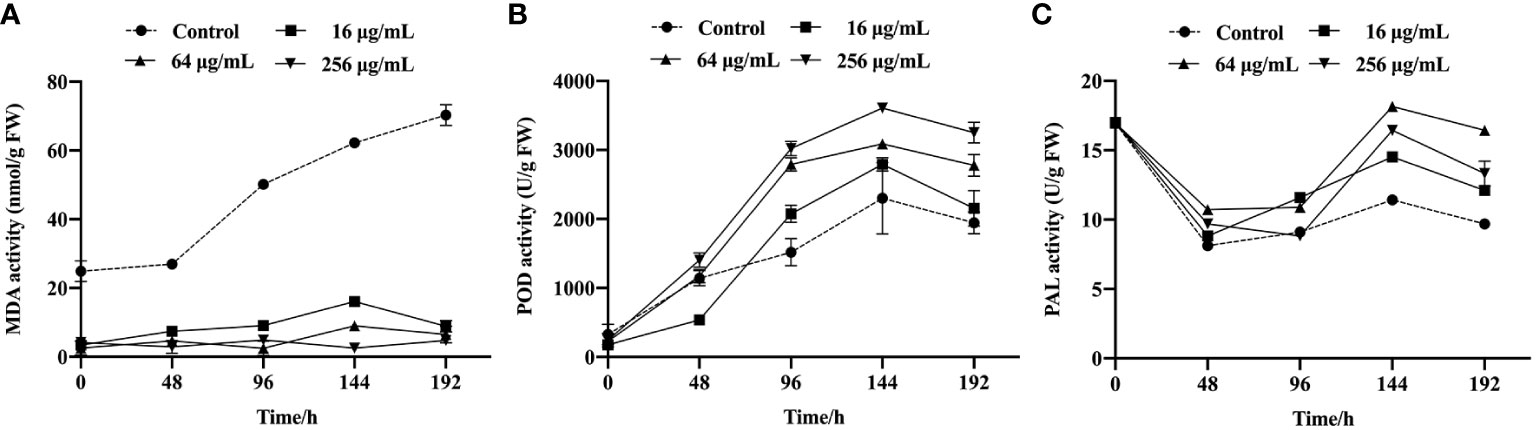
Figure 6 Cherry tomatoes were infected with B. cinerea and incubated at 25 °C to study the activities of MDA (A), POD (B), and PAL (C) in the fruits, the experiment was conducted for 192 h.
The POD activity in the control group and the SQGX-6 extract treatment groups increased throughout the infection period (Figure 6B). After 96 h of infection, the POD content in the groups treated with 16 μg/mL and 64 μg/mL of the extract was approximately two times higher than that in the control group. From 144 h to the 192 h, the POD activity of each treatment group was significantly higher than that of the control group. These results indicated that the SQGX-6 extract increased the POD activity in cherry tomatoes infected with B. cinerea.
Similarly, the PAL content in cherry tomatoes infected with B. cinerea increased after treatment with the SQGX-6 extract (Figure 6C). At 144 h, when the PAL activity in the group treated with 256 μg/mL of the extract was approximately 1.6 times higher than that in the control group. Over the next two days, the PAL activity decreased in all groups. At 192 h, the PAL activities in the groups treated with 16 μg/mL, 64 μg/mL and 256 μg/mL of the extract were 1.25 times, 1.7 times, and 1.4 times higher than the PAL activity of the control group, respectively (Figure 6C). These results suggested that the SQGX-6 extract can enhance the activity of disease-resistant enzymes to induce disease resistance in cherry tomatoes.
3.7 UPLC-MS detection of the components in the SQGX-6 extract
To identify the components of the SQGX-6 extract, it was analyzed using the ultra-performance liquid chromatography-mass spectrometry (UPLC/MS) technique. In total, 618 compounds were identified from the extract (Supplementary Table S1). The top 20 compounds, ranked by classification, were Alkaloids, Quinolines and their derivatives, Amino acids and their derivatives, Carboxylic acids and derivatives, Azoles, Benzene and substituted derivatives, Benzofurans, Carboxylic acids and their derivatives, Coumarins, Fatty acids, Acyls, Flavonoids, Nucleotides and their derivatives, Organic acids, Phenols, Phytohormone, Purine nucleosides, and Steroids and their steroid derivatives (Figure 7A). According to the ranking based on composition, the top 20 compounds were 2-Chloro-DL-Phenylalanine, Vidarabine, Adenosine, Gentisic acid, 4-Hydroxyphenylacetylglutamic acid, 2-Picolinic acid, 2-Phenylacetamide, Adenine, Benzofuran, N6-isopentenyladenosine, Oleic acid, Vaccenic acid, Petroselinic acid, 7-Ethyl-10-Hydroxycamptothecin, 4-Methyl-5-thiazoleethanol, Afzelin, Kaempferol 3-Orhamnoside (Kaempferin), Isoquinoline, Quinoline, Oxypeucedanin, L-pipecolic acid, Pyrrolidonecarboxylic acid, L-Pipecolic acid, Pipecolic acid, (2E)-Decenovl-ACP, and Nandrolone (Figure 7B). The names of the identified chemical components in the extract, along with their KEGG IDs, CAS numbers, molecular weights, and molecular formulae are presented in Supplementary Table S2. To summarize, 2-Chloro-DL-Phenylalanine, Vidarabine, and Adenosine were the compounds with the highest concentrations in the extract of the endophytic fungus. This might be closely related to the bioactivity of secondary metabolites produced by the endophytic fungi.
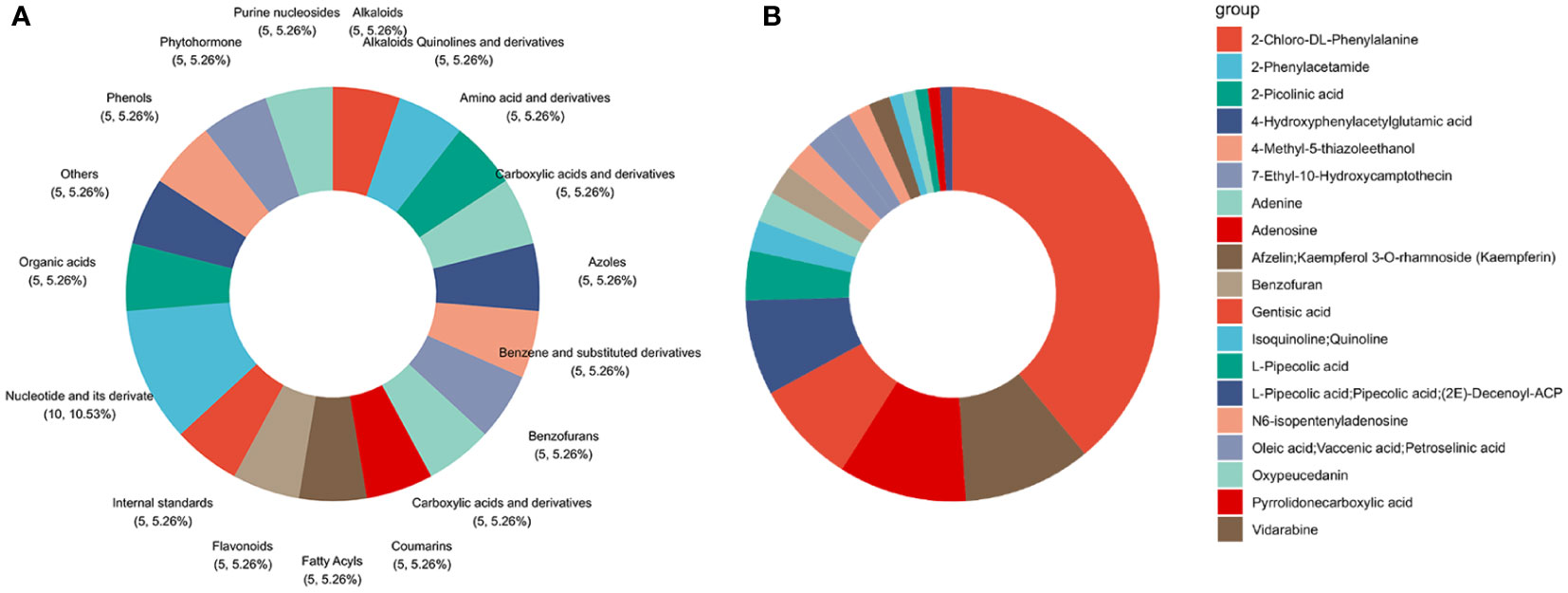
Figure 7 Determination of chemical species and components in the endophytic fungus extract via UHPLC−MS analysis. (A) Classification chart of the top twenty extracts. (B) These extracts ranked in terms of ingredient contents.
4 Discussion
Synthetic pesticides are commonly used after cash crops are cultivated and harvested for treating diseases caused by fungi. However, when using agricultural chemicals, several factors, including the duration and frequency of treatment, concentration, and active ingredients, are often ignored, leading to high production costs (Lydia Macias-Rubalcava et al., 2018). Additionally, developing antifungal crops requires extensive research, application, and production processes, which are time-consuming and expensive (Lydia Macias-Rubalcava et al., 2018). Compared to synthetic pesticides, endophytic fungi are a novel and important microbial resource for producing biologically active compounds. They possess several secondary metabolites and have the advantages of renewable resources and reliable productivity. However, only a few endophytic fungi have been identified. In a study (Jiao et al., 2020), the morbidity of cherry tomatoes decreased to 52.9% after treatment with the endophytic fungus ϵ-PL at a concentration of 3,200 mg/L, compared to the morbidity of cherry tomatoes in the control group. The endophytic fungus H4 has showed high antifungal activity against Clostridium fragilis and pretreatment with the H4 extract significantly decreased the severity and morbidity of Anthrax (Li et al., 2021). In this study, we identified the novel endophytic fungus SQGX-6. Treatment with SQGX-6 and its extract considerably decreased the time and degree of decay of tomatoes after infection with B. cinerea.
SQGX-6 and its ethyl acetate extract inhibited the cell growth of all three pathogenic fungi. The antifungal effect of the extract was better than that of the endophytic fungi, which might be due to the production of a large number of active substances after fermentation (Fatima et al., 2022). By performing UHLC-MS, we found that the extract mainly contained Alkaloids, Azoles, Benzofuran, Coumarin, Flavonoids, Organic acids, Phenols and phytoauxin, which possess various biological activities, including antibacterial, antifungal, immunosuppressive, antiviral, antioxidant, anti-inflammatory and anticancer (Toghueo, 2020). For example, the organic acids isolated from the endophytic mucor of the medicinal plant Pulsatilla chinensis showed a weak antifungal effect on Aspergillus terreus, with MIC values of 127.8 µM and 111.2 µM, respectively (Abdou et al., 2020). Rosemary extract contains phenolic and flavonoid substances, the extract of which has antifungal effects on Candida albicans and Candida donovani (Meccatti et al., 2021). This study found that the secondary metabolites of endophytic fungus SQGX-6 extract are very abundant, many of these metabolites which are more effective and safer than traditional antifungal agents (Manganyi and Ateba, 2020).
Different free radicals produce different degrees of oxidative damage, and since a single antioxidant system cannot reflect the biological significance, different complementary systems are needed to determine the real effect of the sample on antioxidants. In this study, the antioxidant activity of the samples was evaluated using three antioxidant indices, including DPPH, ABTS, and superoxide anion radical scavenging capacity. Our results showed that the SQGX-6 extract exhibited high antioxidant activity at specific concentrations, which was comparable to VC. However, further studies are needed to determine the antioxidant potentials of the SQGX-6 extract.
Besides enhancing antifungal effects, inducing resistance is an effective way to control postharvest rot in tomato fruits (Zhu and Zhang, 2016; Debode et al., 2018). Some researchers reported that resistance and defense to pathogenic fungi might be associated with enzyme activities (Prasad et al., 2022). We found that the SQGX-6 extract can induce the resistance of cherry tomatoes to gray mold. The MDA content of cherry tomatoes inoculated with the Botrytis tomato fungus after induction by the SQGX-6 extract was significantly lower than that in the control group, indicating that the SQGX-6 extract could inhibited MDA production. A reduction in MDA levels decreased peroxidation damage to the cell membrane, which in turn increased the stress resistance of plants to pathogenic fungi (Song et al., 2014). The contents of POD and PAL in tomato fruits increased after treatment with the SQGX-6 extract. Changes in the PAL activity were strongly correlated with plant resistance and the total phenol content (Bill et al., 2017). Compared to the activity of PAL in the infection group, the activity of PAL was upregulated in the SQGX-6 extract-treated group, which indicated that the SQGX-6 extract stimulated the active defense response of cherry tomatoes and increased the defense of cherry tomatoes against tomato fungi during storage. Therefore, a higher level of defense enzymes might be a key factor in reducing the susceptibility of cherry tomatoes to B. cinerea (Li et al., 2022b). A study showed that benzothiadiazole (BTH) functions as an analog of salicylic acid (SA) and can be used to postpone the postharvest decay of various fruits. A study showed that BTH treatment increased the activities of CAT, SOD, PPO, and PAL in lychee fruits, thus increasing their resistance against frost disease after harvest (Xu et al., 2021a).
Microecological control of plant diseases is an eco-friendly approach (Samaras et al., 2021). Due to its broad ecological adaptability, fungi are highly favorable broad-spectrum agents for the control of plant diseases. These products are mainly used in farmland to increase crop yield and protect crops from diseases. In this study, the endophytic fungus SQGX-6 and its extract effectively inhibited three pathogenic fungi that affect crops and also decreased the rotting condition of tomato fruits caused by the tomato gray mold pathogenic fungus. Treatment with different concentrations of the extract can not only improve the POD activity of tomato fruits to a certain extent, thus improving the antioxidant capacity of tomatoes, but it can also delay the rotting process of tomatoes by inhibiting the activities of MDA and PAL activity.
5 Conclusion
In this study, an endophytic fungus with antifungal activity was isolated from the Chinese medicinal herb Panax notoginseng and was identified as Arcopilus aureus. Arcopilus aureus and its ethyl acetate extract showed different degrees of bioactivity, including antioxidant activity and inhibitory effects on the growth of F. graminearum, H. maydis, and B. cinerea, which can effectively decrease the postharvest decay of cherry tomatoes caused by pathogenic fungi in vitro and in vivo. Therefore, organic compounds produced by endophytic fungi might play an important role in the biological control of crop pathogens and might be used as eco-friendly antifungal agents for controlling plant diseases. However, further investigation is needed to develop these antifungal agents.
Data availability statement
The datasets presented in this study can be found in online repositories. The names of the repository/repositories and accession number(s) can be found below: https://www.ncbi.nlm.nih.gov/genbank/, OR053652.
Author contributions
DS: Data curation, Formal analysis, Investigation, Methodology, Writing – original draft. FL: Project administration, Supervision, Writing – review & editing. LW: Methodology, Writing – original draft. RC: Investigation, Writing – original draft. FL: Supervision, Writing – original draft. LG: Supervision, Writing – original draft. NL: Supervision, Writing – original draft. FZ: Supervision, Visualization, Writing – review & editing. LL: Conceptualization, Funding acquisition, Resources, Writing – review & editing.
Funding
The author(s) declare financial support was received for the research, authorship, and/or publication of this article. This research was supported by the National Key Research and Development Program of China (2021YFD1800405) and the National Natural Science Foundation of China (32072823).
Conflict of interest
The authors declare that the research was conducted in the absence of any commercial or financial relationships that could be construed as a potential conflict of interest.
Publisher’s note
All claims expressed in this article are solely those of the authors and do not necessarily represent those of their affiliated organizations, or those of the publisher, the editors and the reviewers. Any product that may be evaluated in this article, or claim that may be made by its manufacturer, is not guaranteed or endorsed by the publisher.
Supplementary material
The Supplementary Material for this article can be found online at: https://www.frontiersin.org/articles/10.3389/fpls.2024.1305376/full#supplementary-material
Supplementary Figure 1 | Three-year-old Panax notoginseng from Yanshan County, Wenshan Zhuang and Miao Autonomous Prefecture, Yunnan Province, China (23.60° N and 104.33° E). S1A. Five fresh three-year-old Panax notoginseng, the average height is 68-75 cm. S1B. Single Panax notoginseng, the number of leaves per plant is about 20-25 at the time of collection. S1C. Close up of the root of Panax notoginseng, with a diameter of approximately 15 cm.
Supplementary Figure 2 | PCR amplification and agarose gel electrophoresis of endophytic fungus SQGX-6 from Panax notoginseng.
Supplementary Table 1 | Table of mobile phase conditions for liquid chromatography.
Supplementary Table 2 | Identified chemical constituents of bioactive fungal extract.
References
Abdou, R., Shabana, S., Rateb, M. E., Terezine, E. (2020). bioactive prenylated tryptophan analogue from an endophyte of Centaurea stoebe. Nat. Prod. Res. 34 (4), 503–510. doi: 10.1080/14786419.2018.1489393
Aly, A. H., Debbab, A., Kjer, J., Proksch, P. (2010). Fungal endophytes from higher plants: a prolific source of phytochemicals and other bioactive natural products. Fungal Diversity 41 (1), 1–16. doi: 10.1007/s13225-010-0034-4
Aqueveque, P., Cespedes, C. L., Becerra, J., Aranda, M., Sterner, O. (2017). Antifungal activities of secondary metabolites isolated from liquid fermentations of Stereum hirsutum (Sh134-11) against Botrytis cinerea (grey mould agent). Food Chem. Toxicol. 109, 1048–1054. doi: 10.1016/j.fct.2017.05.036
Bill, M., Korsten, L., Remize, F., Glowacz, M., Sivakumar, D. (2017). Effect of thyme oil vapours exposure on phenylalanine ammonia-lyase (PAL) and lipoxygenase (LOX) genes expression, and control of anthracnose in 'Hass' and 'Ryan' avocado fruit. Sci. Hortic-Amsterdam 224, 232–237. doi: 10.1016/j.scienta.2017.06.026
Chen, P., Chen, R., Chou, J. (2018). Screening and evaluation of yeast antagonists for biological control of botrytis cinerea on strawberry fruits. Mycobiology 46 (1), 33–46. doi: 10.1080/12298093.2018.1454013
Cui, H., Lin, Y., Luo, M., Lu, Y., Huang, X., She, Z. (2017). Diaporisoindoles A-C: three isoprenylisoindole alkaloid derivatives from the mangrove endophytic fungus diaporthe sp. SYSU-HQ3. Org Lett. 19 (20), 5621–5624. doi: 10.1021/acs.orglett.7b02748
Debode, J., De Tender, C., Cremelie, P., Lee, A. S., Kyndt, T., Muylle, H., et al. (2018). Trichoderma-inoculated miscanthus straw can replace peat in strawberry cultivation, with beneficial effects on disease control. Front. Plant Sci. 9. doi: 10.3389/fpls.2018.00213
Doin de Moura, G. G. D., de Barros, A. V., MaChado, F., Martins, A. D., da Silva, C. M., Durango, L. G. C., et al. (2021). Endophytic bacteria from strawberry plants control gray mold in fruits via production of antifungal compounds against Botrytis cinerea L. Microbiol. Res. 251 (4), 126793. doi: 10.1016/j.micres.2021.126793
Fatima, I., Hakim, S., Imran, A., Ahmad, N., Imtiaz, M., Ali, H., et al. (2022). Exploring biocontrol and growth-promoting potential of multifaceted PGPR isolated from natural suppressive soil against the causal agent of chickpea wilt. Microbiol. Res. 260, 127015. doi: 10.1016/j.micres.2022.127015
Hu, Z., Wu, Y., Xie, S., Sun, W., Guo, Y., Li, X., et al. (2017). Phomopsterones A and B, two functionalized ergostane-type steroids from the endophytic fungus phomopsis sp. TJ507A. Org Lett. 19 (1), 258–261. doi: 10.1021/acs.orglett.6b03557
Jiang, Y., Li, H., Huang, P., Li, S., Li, B., Huo, L., et al. (2022). Panax notoginseng saponins protect PC12 cells against Aβ induced injury via promoting parkin-mediated mitophagy. J. Ethnopharmacol 285, 114859. doi: 10.1016/j.jep.2021.114859
Jiao, W., Liu, X., Chen, Q., Du, Y., Li, Y., Yue, F., et al. (2020). Epsilon-poly-L-lysine (ϵ-PL) exhibits antifungal activity in vivo and in vitro against Botrytis cinerea and mechanism involved. Postharvest Biol. Tec. 168, 111270. doi: 10.1016/j.postharvbio.2020.111270
Keller, N. P. (2019). Fungal secondary metabolism: regulation, function and drug discovery. Nat. Rev. Microbiol. 17 (3), 167–180. doi: 10.1038/s41579-018-0121-1
Kim, S. H., Lee, Y., Balaraju, K., Jeon, Y. (2023). Evaluation of Trichoderma atroviride and Trichoderma longibrachiatum as biocontrol agents in controlling red pepper anthracnose in Korea. Front. Plant Sci. 14. doi: 10.3389/fpls.2023.1201875
Latz, M. A. C., Jensen, B., Collinge, D. B., Jørgensen, H. J. L. (2018). Endophytic fungi as biocontrol agents: elucidating mechanisms in disease suppression. Plant Ecol. Divers. 11 (5-6), 555–567. doi: 10.1080/17550874.2018.1534146
Li, B., Ding, Y., Tang, X., Wang, G., Wu, S., Li, X., et al. (2019). Effect of L-arginine on maintaining storage quality of the white button mushroom (Agaricus bisporus). Food Bioproc Tech. 12 (4), 563–574. doi: 10.1007/s11947-018-2232-0
Li, P., Feng, B., Yao, Z., Wei, B., Zhao, Y., Shi, S. (2022b). Antifungal activity of endophytic bacillus K1 against botrytis cinerea. Front. Microbiol. 13. doi: 10.3389/fmicb.2022.935675
Li, X., Jing, T., Zhou, D., Zhang, M., Qi, D., Zang, X., et al. (2021). Biocontrol efficacy and possible mechanism of Streptomyces sp. H4 against postharvest anthracnose caused by Colletotrichum fragariae on strawberry fruit. Postharvest Biol. Tec. 175, 111401. doi: 10.1016/j.postharvbio.2020.111401
Li, N., Xu, D., Huang, R., Zheng, J., Liu, Y., Hu, B., et al. (2022a). A New Source of Diterpene Lactones From Andrographis paniculata (Burm. f.) Nees-Two Endophytic Fungi of Colletotrichum sp. With Antibacterial and Antioxidant Activities. Front. Microbiol. 13, 819770. doi: 10.3389/fmicb.2022.819770
Liu, H., Chen, Y., Li, H., Li, S., Tan, H., Liu, Z., et al. (2019). Four new metabolites from the endophytic fungus Diaporthe lithocarpus A740. Fitoterapia 137, 104260. doi: 10.1016/j.fitote.2019.104260
Luo, Z., Liu, L., Nie, Q., Huang, M., Luo, C., Sun, Y., et al. (2023). HPLC-based metabolomics of Dendrobium officinale revealing its antioxidant ability. Front. Plant Sci. 14. doi: 10.3389/fpls.2023.1060242
Lydia Macias-Rubalcava, M., Elvira Sanchez-Fernandez, R., Roque-Flores, G., Lappe-Oliveras, P., Mariana Medina-Romero, Y. (2018). Volatile organic compounds from Hypoxylon anthochroum endophytic strains as postharvest mycofumigation alternative for cherry tomatoes. Food. Microbiol. 76, 363–373. doi: 10.1016/j.fm.2018.06.014
Manganyi, M. C., Ateba, C. N. (2020). Untapped potentials of endophytic fungi: A review of novel bioactive compounds with biological applications. Microorganisms 8 (12), 1934. doi: 10.3390/microorganisms8121934
Mao, Z., Zhang, W., Wu, C., Feng, H., Peng, Y., Shahid, H., et al. (2021). Diversity and antibacterial activity of fungal endophytes from Eucalyptus exserta. BMC Microbiol. 21 (1), 155. doi: 10.1186/s12866-021-02229-8
Mariana Medina-Romero, Y., Roque-Flores, G., Lydia Macias-Rubalcava, M. (2017). Volatile organic compounds from endophytic fungi as innovative postharvest control of Fusarium oxysporum in cherry tomato fruits. Appl. Microbiol. Biotechnol. 101 (22), 8209–8222. doi: 10.1007/s00253-017-8542-8
Matar, N., Macadre, C., Ammar, G. A. G., Peres, A., Collet, B., Boustany, N. E., et al. (2022). Identification of beneficial Lebanese Trichoderma spp. wheat endophytes. Front. Plant Sci. 13. doi: 10.3389/fpls.2022.1017890
Meccatti, V. M., De Oliveira, J. R., Figueira, L. W., Netto, A. A. L., Zamarioli, L. S., Marcucci, M. C., et al. (2021). Rosmarinus officinalis L. (rosemary) extract has antibiofilm effect similar to the antifungal nystatin on Candida samples. Anais Da Academia Bras. Cienc. 93 (2), e20190366. doi: 10.1590/0001-3765202120190366
Nagarajan, K., Ibrahim, B., Ahmad Bawadikji, A., Lim, J., Tong, W., Leong, C., et al. (2022). Recent developments in metabolomics studies of endophytic fungi. J. Fungi. 8 (1), 28. doi: 10.3390/jof8010028
Nartey, L. K., Pu, Q., Zhu, W., Zhang, S., Li, J., Yao, Y., et al. (2022). Antagonistic and plant growth promotion effects of Mucor moelleri, a potential biocontrol agent. Microbiol. Res. 255, 126922. doi: 10.1016/j.micres.2021.126922
Omomowo, O. I., Babalola, O. O. (2019). Bacterial and fungal endophytes: tiny giants with immense beneficial potential for plant growth and sustainable agricultural productivity. Microorganisms 7 (11), 481. doi: 10.3390/microorganisms7110481
Prasad, A., Patel, P., Niranjan, A., Mishra, A., Saxena, G., Singh, S. S., et al. (2022). Biotic elicitor-induced changes in growth, antioxidative defense, and metabolites in an improved prickleless Solanum viarum. Appl. Microbiol. Biotechnol. 106 (19-20), 6455–6469. doi: 10.1007/s00253-022-12159-8
Qi, D., Zou, L., Zhou, D., Zhang, M., Wei, Y., Li, K., et al. (2022). Biocontrol potential and antifungal mechanism of a novel Streptomyces sichuanensis against Fusarium oxysporum f. sp. cubense tropical race 4 in vitro and in vivo. Appl. Microbiol. Biotechnol. 106 (4), 1633–1649. doi: 10.1007/s00253-022-11788-3
Rahman, M., Borah, S. M., Borah, P. K., Bora, P., Sarmah, B. K., Lal, M. K., et al. (2023). Deciphering the antimicrobial activity of multifaceted rhizospheric biocontrol agents of solanaceous crops viz., Trichoderma harzianum MC2, and Trichoderma harzianum NBG. Front. Plant Sci. 14. doi: 10.3389/fpls.2023.1141506
Samaras, A., Karaoglanidis, G. S., Tzelepis, G. (2021). Insights into the multitrophic interactions between the biocontrol agent Bacillus subtilis MBI 600, the pathogen Botrytis cinerea and their plant host. Microbiol. Res. 248, 126752. doi: 10.1016/j.micres.2021.126752
Song, M., Fan, S., Pang, C., Wei, H., Yu, S. (2014). Genetic analysis of the antioxidant enzymes, methane dicarboxylic aldehyde (MDA) and chlorophyll content in leaves of the short season cotton (Gossypium hirsutum L.). Euphytica 198 (1), 153–162. doi: 10.1007/s10681-014-1100-x
Sun, S., Wang, C., Tong, R., Li, X., Fishbein, A., Wang, Q., et al. (2010). Effects of steaming the root of Panax notoginseng on chemical composition and anticancer activities. Food Chem. 118 (2), 307–314. doi: 10.1016/j.foodchem.2009.04.122
Sutton, J. C. (1982). Epidemiology of wheat head blight and maize ear rot caused by Fusarium graminearum. J. Plant Pathol. 4 (2), 195–209. doi: 10.1080/07060668209501326
Tanney, J. B., McMullin, D. R., Green, B. D., Miller, J. D., Seifert, K. A. (2016). Production of antifungal and antiinsectan metabolites by the Picea endophyte Diaporthe maritima sp. nov. Fungal Biol. 120 (11), 1448–1457. doi: 10.1016/j.funbio.2016.05.007
Toghueo, R. M. K. (2020). Bioprospecting endophytic fungi from Fusarium genus as sources of bioactive metabolites. Mycology 11 (1), 1–21. doi: 10.1080/21501203.2019.1645053
Vidal, A., Parada, R., Mendoza, L., Cotoras, M. (2020). Endophytic Fungi Isolated from Plants Growing in Central Andean Precordillera of Chile with Antifungal Activity against Botrytis cinerea. J. Fungi. 6 (3), 149. doi: 10.3390/jof6030149
Wang, X. W., Houbraken, J., Groenewald, J. Z., Meijer, M., Andersen, B., Nielsen, K. F., et al. (2016). Diversity and taxonomy of Chaetomium and chaetomium-like fungi from indoor environments. Stud. Mycol 84, 145–224. doi: 10.1016/j.simyco.2016.11.005
Wu, J., Chen, R., Tan, L., Bai, H., Tian, L., Lu, J., et al. (2022). Ultrasonic disruption effects on the extraction efficiency, characterization, and bioactivities of polysaccharides from Panax notoginseng flower. Carbohydr.Polym. 291, 119535. doi: 10.1016/j.carbpol.2022.119535
Xie, J., Wu, Y., Zhang, T., Zhang, M., Zhu, W., Gullen, E. A., et al. (2017). New and bioactive natural products from an endophyte of Panax notoginseng. Rsc Adv. 7 (60), 38100–38109. doi: 10.1039/c7ra07060h
Xu, Y., Wang, L., Liang, W., Liu, M. (2021). Biocontrol potential of endophytic Bacillus velezensis strain QSE-21 against postharvest grey mould of fruit. Biol. Control. 161, 104711. doi: 10.1016/j.biocontrol.2021.104711
Xu, D., Xi, P., Lin, Z., Huang, J., Lu, S., Jiang, Z., et al. (2021a). Efficacy and potential mechanisms of benzothiadiazole inhibition on postharvest litchi downy blight. Postharvest Biol. Tec. 181, 111660. doi: 10.1016/j.postharvbio.2021.111660
Yan, H., Jin, H., Fu, Y., Yin, Z., Yin, C. (2019). Production of rare ginsenosides rg3 and rh2 by endophytic bacteria from panax ginseng. J. Agric. Food Chem. 67 (31), 8493–8499. doi: 10.1021/acs.jafc.9b03159
Yang, C., Wang, Z., Wan, J., Qi, T., Zou, L. (2023). Burkholderia gladioli strain KJ-34 exhibits broad-spectrum antifungal activity. Front. Plant Sci. 14. doi: 10.3389/fpls.2023.1097044
Zeng, Y., Yang, H., Wang, H., Zong, M., Lou, W. (2019). Immune enhancement activity of a novel polysaccharide produced by Dendrobium officinale endophytic fungus Fusarium solani DO7. J. Funct. Foods 53, 266–275. doi: 10.1016/j.jff.2018.12.038
Zhang, H., Kong, N., Liu, B., Yang, Y., Li, C., Qi, J., et al. (2022). Biocontrol potential of Trichoderma harzianum CGMCC20739 (Tha739) against postharvest bitter rot of apples. Microbiol. Res. 265, 127182. doi: 10.1016/j.micres.2022.127182
Keywords: endophytic fungus of Panax notoginseng, extraction, antifungal, antioxidant, biological control
Citation: Sun D, Li F, Wang L, Chen R, Liu F, Guo L, Li N, Zhang F and Lei L (2024) Identification and application of an endophytic fungus Arcopilus aureus from Panax notoginseng against crop fungal disease. Front. Plant Sci. 15:1305376. doi: 10.3389/fpls.2024.1305376
Received: 01 October 2023; Accepted: 16 January 2024;
Published: 07 February 2024.
Edited by:
Ahmed Abdelkhalek, City of Scientific Research and Technological Applications, EgyptReviewed by:
Amr H. Hashem, Al-Azhar University, EgyptBamisope Steve Bamisile, South China Agricultural University, China
Copyright © 2024 Sun, Li, Wang, Chen, Liu, Guo, Li, Zhang and Lei. This is an open-access article distributed under the terms of the Creative Commons Attribution License (CC BY). The use, distribution or reproduction in other forums is permitted, provided the original author(s) and the copyright owner(s) are credited and that the original publication in this journal is cited, in accordance with accepted academic practice. No use, distribution or reproduction is permitted which does not comply with these terms.
*Correspondence: Fuxian Zhang, emhhbmdmdXhpYW45OUAxNjMuY29t; Liancheng Lei, bGVpbGlhbmNoZW5nQDE2My5jb20=
†These authors have contributed equally to this work