- Faculty of Biology, University of Bialystok, Białystok, Poland
Introduction: Inbreeding depression (ID) in food-deceptive plants have been reported previously, however, it has not been often proven that selfed seeds germinate better than outbred ones or that selfing affects ID. To resolved these issues, food-deceptive related Dactylorhiza majalis, D. incarnata var. incarnata and D. fuchsii orchids were investigated.
Methods: Hand pollination treatments and control pollination were conducted. Fruit set, number of seeds per fruit, seed length, number of well-developed seeds per fruit, and proportion of in vitro asymbiotic germination seeds, were analyzed in relation to inflorescence levels and used as fitness indicators for these orchids. The ID and pollen limitation were measured.
Results: The lowest ID (δ = −1.000) was in D. majalis, and present in combination with a high pollen limitation in its populations. D. fuchsii showed higher ID (δ = 0.366), and D. incarnata var. incarnata weak ID (δ = 0.065), although ID varied between its fitness components. The seed number per fruit differed significantly between the treatments and the inflorescence levels in treatments.
Discussion: This study emphasizes that the breeding system rather than the flower position on the inflorescence shaped the quality and quantity of reproductive output. The ID and its effect on germination of food-deceptive orchid seeds undoubtedly played an important role.
1 Introduction
Inbreeding depression (ID) is a mechanism that is defined as the reduction in the fitness of offspring resulting from an interbreeding between related individuals (Barrett & Harder, 2017). The effects of ID have usually been studied in relation to the mating systems, population size, area of geographical distribution, and their consequences on different developmental plant life stages (i.e., seeds, juveniles, and adult stages) (Glaettli & Goudet, 2006; Angeloni et al., 2011; Valtueña et al., 2014; Blambert et al., 2016). Angeloni et al. (2011), using meta-analysis of 116 studies and 107 plants, found that ID was significant for all life history stages and reproductive traits. In their study, ID increased with population size and varied depending on the method of measuring plant fitness. They also concluded, that self-compatible plant species are much more prone to inbreeding than self-incompatible species, and often to display ID. This can contribute to a faster removal of deleterious alleles in different life stages. They also explained that significant ID was found for all the life stages, but the lowest ID in the early life stage (i. g. germination) than in the late life stages for self-compatible species can occur.
From another point of view, the study by Baskin & Baskin (2015) on 743 germination cases has showed that outbred seeds can germinate better, equal (in 50.1% of cases), or less well than inbred seeds. The authors recommended improving the germination procedure in the natural environment, but there is no doubt that this procedures requires further investigation. Mechanisms associated with ID, such as a lower germination rate and low juvenile survival, as well as a decline in the growth fitness of progeny derived from selfing, promote undoubtedly the evolution of outcrossing (Smithson, 2006).
The mating system has frequently been emphasized as the best predictor of ID (Johnson & Nilsson, 1999; Juillet et al., 2006). In Orchidaceae, in which approximately one-third of all known orchids implement deception, outcrossing is exhibited as the main mating system (Ackerman, 1986; Dafni, 1987; Nilsson, 1992; Jersáková et al., 2006). Husband & Schemske (1996) reported that genetic load is observed in outcrossing plants to favor the evolution if inbreeding avoidance. They showed empirical evidences that outcrossing species have significantly greater ID at seed viability than selfing species. Therefore, ID may be considered as a mechanism favoring the evolution and maintenance of food deception strategy in orchids. Then, Smithson (2006) compiled ID from the literature based on the various life stages within nectarless orchids (δ = -0.064 – 0.713) and these wild spectrum of values did not fully support the predictions and experimental data of Husband and Schemske (1996). However, the earlier scarce surveys of food-deceptive, and self-compatible orchids evaluated that the fruit set was the effect of different mating system i.e. outcrossing, selfing, or mix-mating. In this way, the mating systems can contribute to the different effects of reproductive output and ID (Johnson & Nilsson, 1999; Smithson, 2006; Tremblay et al., 2005; Kropf & Renner, 2008; Ostrowiecka et al., 2019).
Our study focused on Dactylorhiza taxa, which are food-deceptive orchids with no rewards for their pollinators (Claessens and Kleynen, 2011). More published studies of ID have analyzed an early life stage in this group of plants, but there are still often contradictory results. In Dactylorhiza genus, only two taxa, D. sambucina and D. praetermissa, have been examined for ID (Ferdy et al., 2001; Juillet et al., 2006). Depending on different developmental life stages of these plants (e. g. seeds, germination, or juvenile and adult) and type of crosses (outcrossing or selfing) both strong and weak ID were recognized. The negative correlation between fitness indicators and the position of the fruit on D. praetermissa inflorescence suggested that resource might be limited, and selective abortion might be important. Further, ID may vary through ontogeny, and the total amount of ID suffered by an inbred individual is a product of reduced fitness at all life stages. In this context, in ID studies of food-deceptive Dactylorhiza taxa it is important to consider their current mating system, and to compare fitness component traits at different life stages in natural pollinated, inbred, and outbred individuals (Carr & Duasch, 1996; Husband & Schemske, 1996). Therefore, we focused on three Dactylorhiza taxa i.e. D. majalis, D. incarnata var. incarnata, and D. fuchsii. They are terrestrial, long-lived, self-compatible, and tuberous perennial orchids that reproduce either by seeds or (rarely) vegetatively (Vakhrameeva et al., 2008; Claessens and Kleynen, 2011; Ostrowiecka et al., 2019). They produce a single multi-flowered inflorescence and flowers with short spurs (Naczk et al., 2018). The flower number on inflorescence differs between taxa, and they open from bottom to top sequentially but very quickly or all flowers on an inflorescence can be open simultaneously (Ostrowiecka et al., 2019). Pollination occurs by different taxonomical groups of insects (Hymenoptera, Diptera, and/or Coleoptera), which can promote cross-pollination and/or geitonogamy (Hedrén & Nordström, 2009; Ostrowiecka et al., 2019; Wróblewska et al., 2019). Dactylorhiza majalis blooms first from the beginning of May to the beginning of June. The flowering period of D. incarnata and D. fuchsii occurs between June and July (Ostrowiecka et al., 2019), and the fruits occur from the end of July. The variable fruit set ranges from 7.4% to 77.5% in three Dactylorhiza taxa (Vallius et al., 2004; Kindlmann & Jersáková, 2006; Claessens and Kleynen, 2011). Pollen limitation has also been observed in these plants and has been recognized as an important phenomenon that shapes the quality and quantity of the fruit set and seeds (Kropf and Renner, 2008). Molecular markers such as cpDNA (trnL, trnF and psbC–trnK), internal transcribed spacer (ITS) sequences, and flow cytometry data confirmed the taxonomic status of the studied three orchids (Wróblewska et al., 2019).
Here, the second key issue in investigation ID patterns of three Dactylorhiza taxa is phylogenetic relationship between them. Dactylorhiza majalis is allotetrapolyploid, and has survived in central European glacial refugia during the last ice age (Balao et al., 2016), or formed probably at different times in the recent half of the Quaternary (Brandrud et al., 2020; Hawranek, 2021). Clo (2022) investigated the effect of polyploidy on the evolution of ID using meta-analysis, and hypothesized that ID should be lower in allopolyploids compared to diploids progenitors. This meta-analysis showed theoretical expectations that due to an initial bottleneck, the masking of deleterious mutations, and/or a slower increase in homozygosity during selfing events, (new)polyploid lineages benefit from a strong decrease in ID (se also e. g. Otto & Whitton, 2000; Clo, 2022). However, empirical studies on the relationship between allopolyploidy and ID have still produced conflicting results (Johnston and Schoen, 1996; Rosquist, 2001; Pannell et al., 2004; Barringer & Geber, 2008; Vandepitte et al., 2011). In resolving these above-mentioned issues and to bridge the knowledge gap between food-deception strategy and mating systems in closely related diploid and polyploid Dactylorhiza orchids, we analyzed the various fitness traits (i.e., fruit set, quantity and quality of seeds, and germination) over the life stage categories following control pollination, self and outcross hand pollinations to determine (1) how mating system shapes the magnitude of ID at these fitness components, (2) how the flower position along the inflorescence affects reproductive success and ID, and (3) what are the fitness traits in which ID is the more pronounced.
2 Material and methods
2.1 Study sites
This two-year study was conducted from May to July across three populations each of D. majalis (KA, 2014-2015; SKI, 2015-2016; and SKII, 2016-2017), D. incarnata var. incarnata (ZB, 2014-2015; RO and MR, 2015-2016), and D. fuchsii (BR, CM, and GR, 2015-2016), located in northeastern Poland (Supplementary Table S1; Figure 1). Dactylorhiza majalis grows in wet meadows, cohabitating with abundant entomophilous and rewarding plants, comprising approximately 20% of the herb layer. The abundance of D. majalis varied, with around 120–200 (to ca. 1,000) flowering individuals (Supplementary Table S1). The three D. incarnata var. incarnata populations varied in sizes ranging from 35 to 200 flowering plants (Supplementary Table S1). These populations inhabited sedge communities in the Biebrza Valley and Rospuda Valley, with a rewarding plant species cover of about 10%. Dactylorhiza fuchsii was found in open hornbeam forests in the Białowieża Primeval Forest and nearby areas and one population in the Biebrza Valley (84–193 flowering plants), all with a low density of rewarding plants.
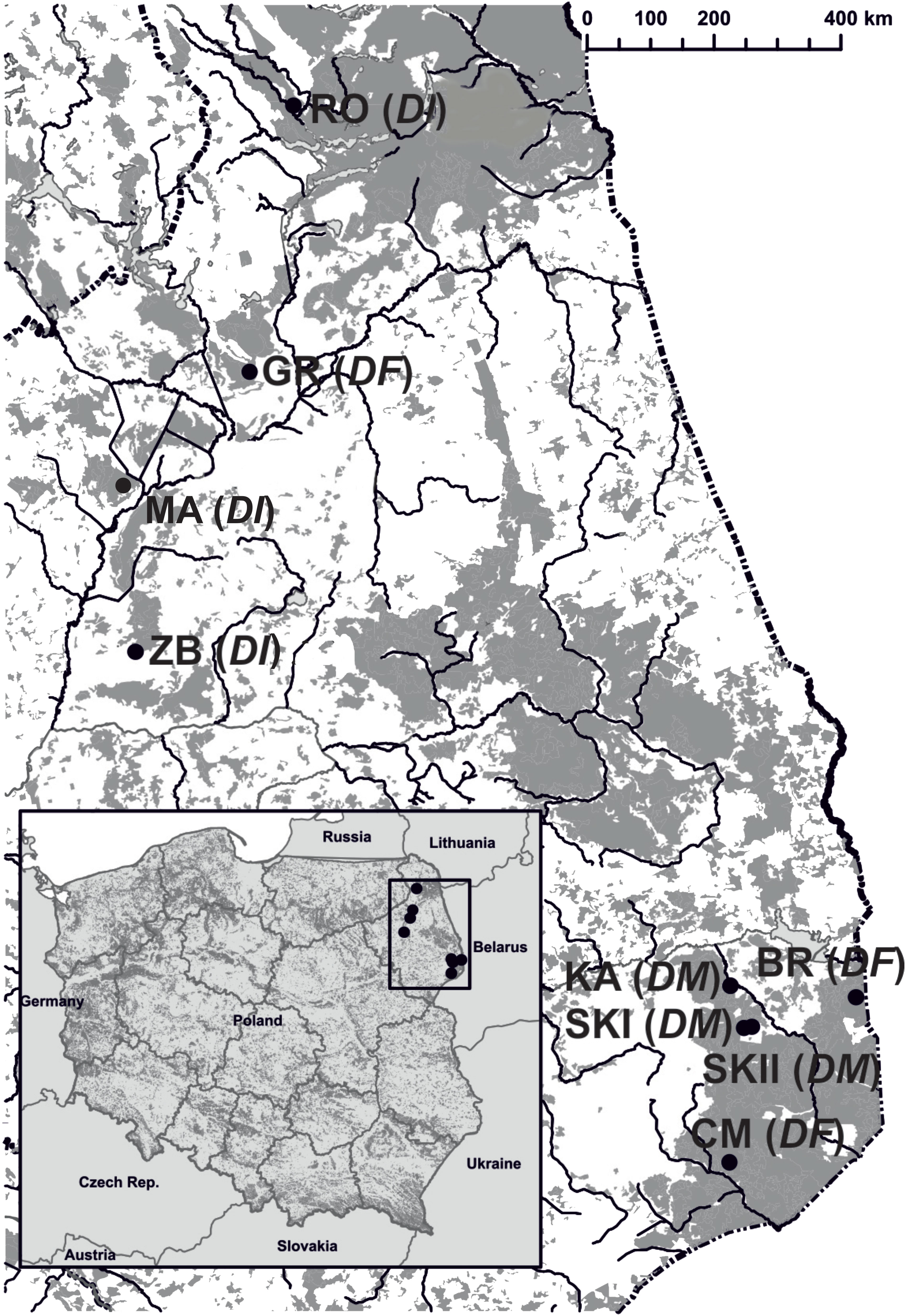
Figure 1 Localities of nine Dactylorhiza populations in north-eastern Poland. D. majalis (DM), KA, SKI, and SKII, D. incarnata var. incarnata (DI), ZB, MR, and RO, D. fuchsii (DF) CM, BR, and GR.
2.2 Open and hand pollination experiments
Over a two-year period (2014 to 2017), open and hand pollination experiments were conducted on three Dactylorhiza taxa during peak flowering, when most flowers were open. Open pollination treatments (n = 5 inflorescences per population/year, 90–340 flowers per population/year, Supplementary Table S1) and four hand pollination experiments were carried out on bagged inflorescences, during the optimum of flowering (almost all freshly flowers on the inflorescences were opened). For the hand pollination treatments, we employed three treatments on nylon mesh bag-enclosed inflorescences: (1) spontaneous self-pollination to assess autogamy (n = 2–5 inflorescences per population/year, 20–154 flowers per population/year, Supplementary Table S1), (2) induced self-pollination to determine self-compatibility (using pollen from the same flower) (n = 2–5 inflorescences per population/year, 37–203 flowers per population/year, Supplementary Table S1), and (3) geitonogamy (using pollen from another flower on the same plant) (n = 1–5 inflorescences per population/year, 37–203 flowers per population/year, Supplementary Table S1). Additionally, (4) induced xenogamy (cross-pollination with pollen from different plants within the same population, approximately 3 m away) was performed (n = 5 inflorescences per population/year, 76–177 flowers per population/year, Supplementary Table S1). All flowers for each treated plant were hand pollinated. After each treatment, the inflorescences were quickly rebagged. In total, 518 inflorescences and 9994 flowers were analyzed over two years across all treatments and taxa (Supplementary Table S1).
Pollination treatments were conducted in two stages: initially without division of the inflorescence, followed by targeted treatments on lower, middle, and upper sections of the inflorescence in each population to investigate the effect of flower position on fitness traits (fruit set, seed quantity and quality, germination). Flower allocation to each level was based on near-equal numbers. Statistical analyses of seed variability and the likelihood of in vitro asymbiotic germination to protocorm stage were performed for all hand pollination treatments, both divided and non-divided of inflorescence.
2.3 Fruit set, seed quantity and quality
Upon maturity, fruit set, total seed number per fruit, mean viable seed length (mm), seed categories based on embryo shape, and viable seeds germinating into protocorms on various asymbiotic media were measured. Seeds were immediately sown post-collection and evenly spread in Petri dishes marked with a grid (6 × 6 for D. fuchsii and D. majalis, 10 × 11 for D. incarnata var. incarnata). A random selection of 11 or 20 squares (confidence level of 0.02) was used for analysis, with a pseudorandom number generator implemented in the R environment (R 3.6/4.0 software, R Core Team, 2021). Categories included seeds with deformed embryos and well-developed globular embryos (Lemus et al., 2021). Sixty well-formed seeds per capsule were randomly chosen and measured using an OLYMPUS SZX2-ILLT stereomicroscope and MultiScan v.14.02 software.
For in vitro germination, seeds were stratified at 4°C for 12 weeks, surface sterilized in 5% NaOCl with Tween 80, and air-dried before sowing on Malmgren (1996) (D. fuchsii) and FAST (Lindén, 1980) (D. majalis and D. incarnata var. incarnata) media. Germination and protocorm development were assessed under a microscope after 40 days of incubation in the dark at 19–20°C. Total seed germination and protocorm development were assessed under a light microscope.
3 Data analysis
3.1 Fruit set and seed variability
Given the non-normal distribution of the data, traditional transformations (arcsin, log + 1, square root) were ineffective for ANOVA requirements. Therefore, Kruskal–Wallis tests and Dunn’s post-hoc tests with Bonferroni correction were employed to identify differences in fruit production, seed number per fruit, seed length, well-developed seed frequency per fruit, and in vitro germination rates between control and hand pollination treatments, and among flower positions on the inflorescence for each taxon. Variables included treatment types (control, selfing, cross-pollination), inflorescence levels (lower, middle, upper), and dependent factors (fruit set, seed number, seed length, well-developed seed proportion, in vitro germination rates). R 3.6/4.0 software was used for analysis, and standard errors were provided with mean values (R Core Team, 2021).
3.2 ID coefficient and pollen limitation
The ID coefficient δ was calculated from five reproductive traits (fruit set, seed number, seed length, well-developed seed proportion, in vitro germination), both for entire inflorescences and division of inflorescence on three levels, using formulas by Ågren and Schemske (1993) and Lande and Schemske (1985). The formula δ = 1 − (Ws/Wo) was used when Ws < Wo, and δ = (Wo/Ws) − 1 for Ws > Wo, where Ws represents the average fitness of selfed progeny and Wo represents that of outcrossed progeny. The estimation was carried out using data where Ws denotes the average fitness of selfed progeny from autogamy and geitonogamy treatments and Wo denotes the average fitness of manually outcrossed progeny (Lande and Schemske, 1985; Charlesworth & Charlesworth, 1987). Cumulative δ values were calculated incorporating the correlation among data sets, using the approach by Husband & Schemske (1996): δ = 1 − (WsF/WoF x WsNS/WoNS x WsSL/WoSL x WsDS/WoDS x WsIV/WoIV), where F is fruit set; NS is seed number per fruit; SL is seed length, DS is frequency of properly developed seeds per fruit, and IV is proportion in vitro asymbiotic germinated seeds to protocorm. The values of δ range from −1 to 1, where 0 signifies no ID, positive values indicate that the outcrossed offspring outperformed the inbred offspring, and negative values indicate the opposite (Charlesworth & Charlesworth, 1987).
Pollen limitation (PL) was calculated using the Larson and Barrett (2000) formula: PL = 1 – (control fruit set/cross-pollination fruit set), where PL values range from 0 (no pollen limitation) to 1 (maximum pollen limitation), and negative values indicate greater pollen receipt in naturally pollinated flowers (Lázaro and Traveset, 2006, González-Varo et al., 2009).
4 Results
4.1 Reproductive success and seed viability
There were significant differences in fruit set between control pollination and hand pollination treatments in the three Dactylorhiza taxa (Figure 2; Supplementary Table S2). In D. majalis, D. incarnata var. incarnata, and D. fuchsii, fruit set was 3 to 4 times lower in control pollination (35.7–44.0%) compared to hand pollination (85.0–90.2% for cross-pollination and 88.9–95.6% for self-pollination, Figure 2; Supplementary Table S2). No significant decrease in fruit set was observed from the lower to the upper position on the inflorescence for each Dactylorhiza species in both control and hand pollination treatments (Figure 2; Supplementary Table S1). Spontaneous autogamy was recorded in less than 1% of all studied flowers across the three Dactylorhiza taxa; hence, it was excluded from further analysis.
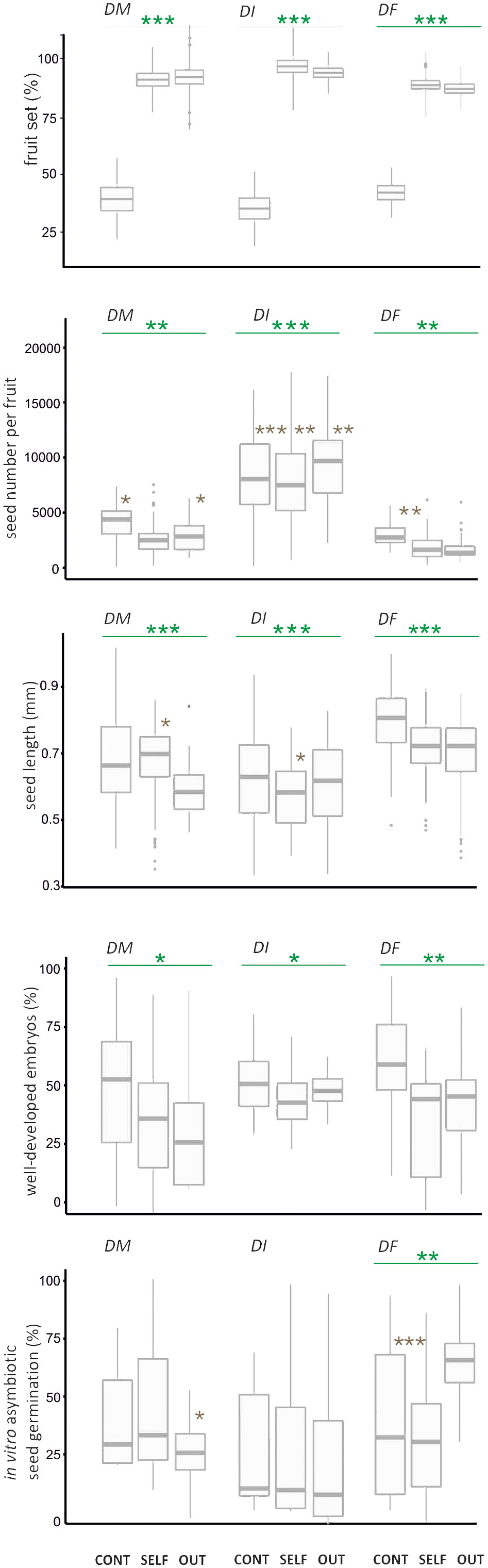
Figure 2 Fitness traits for fruit set quantity and quality, and germination of Dactylorhiza majalis (DM), D. incarnata var. incarnata (DI), and D. fuchsii (DF) seeds that were the effect of control pollination (natural pollination, CONT), selfing (SELF), and outcrossing (OUT). Dark green line, comparison between treatments; Brown asterisk, comparison between levels of inflorescence; *p < 0.05; **p < 0.001; ***p < 0.001. The box-and-whisker plot includes the mean (denoted by a horizontal bar in the box), standard error (denoted by a square), and standard deviation (denoted by the box).
The seed number per fruit varied significantly between control and hand pollination treatments, and often between inflorescence levels within each taxon (Figure 2). In D. majalis and D. fuchsii, seed numbers per fruit were higher in control pollination than in both selfing and crossing experiments. In contrast, D. incarnata var. incarnata exhibited a higher number of seeds in outcrossing compared to selfing and control pollination (Figure 2, Supplementary Table S2). The lower part of the inflorescence produced a higher seed count per fruit than the upper part in control pollination across the three taxa (Supplementary Table S2).
Significant variations in seed length and the number of seeds with well-developed embryos were observed only between the different orchid treatments (Table S2). Seeds from control pollination showed a higher frequency of well-developed embryos than those from selfing and outcrossing in all Dactylorhiza taxa (Figure 2).
No significant differences were observed in in vitro asymbiotic seed germination between control and hand pollination treatments in D. majalis and D. incarnata var. incarnata (Figure 2; Supplementary Table S2). However, a significant difference was noted in the proportion of in vitro germinated seeds among all treatments in D. fuchsii (Figure 2). Notably, in vitro asymbiotic seed germination varied significantly between inflorescence levels in control pollination for D. fuchsii and in cross-pollination for D. majalis (Supplementary Table S2). The frequency of in vitro seed germination from the control fruit set was comparable to that of in vitro germinated seeds from selfing in D. fuchsii (Figure 2).
4.2 ID and pollen limitation
The three Dactylorhiza taxa exhibited varying levels of ID. The cumulative ID at the taxa level was negative and lowest in D. majalis (δ = −1.000), nearly close to zero (δ = 0.065) in D. incarnata var. incarnata, and moderately positive in D. fuchsii (δ = 0.366). Differences in ID were observed between species for all fitness parameters and across inflorescence levels (Figure 3). Dactylorhiza majalis showed negative ID values in three out of four reproductive features studied (seed length, frequency of well-developed embryos in seeds, and proportion of in vitro seed germination), both with and without division of inflorescence into three levels (Figure 3). The lowest ID values were consistently noted at the upper level of the inflorescence in D. majalis.
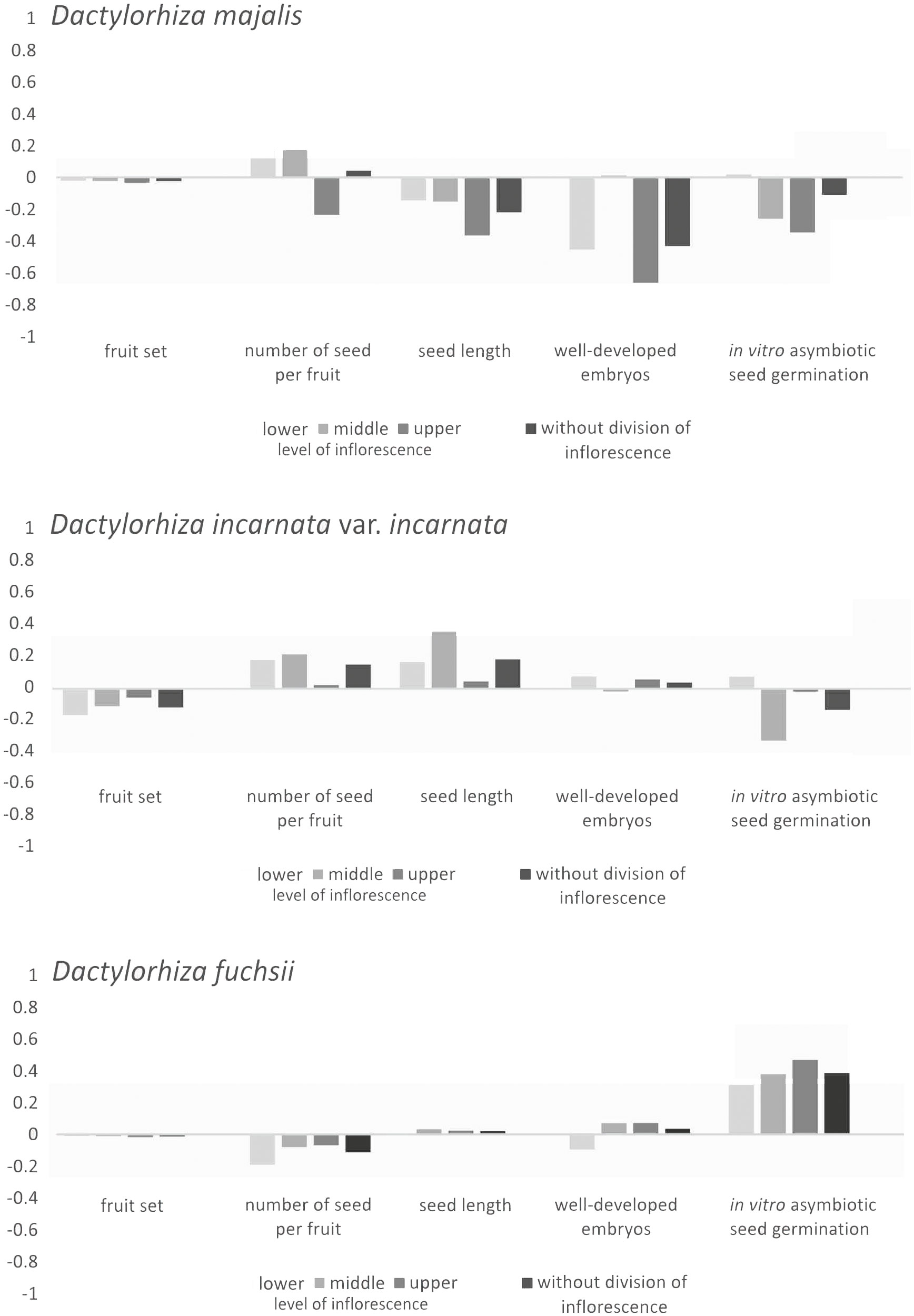
Figure 3 Distribution of inbreeding depression (δ) in fruit set, seed number per fruit, seed length, frequency of well-developed embryos in seeds, and proportion of in vitro asymbiotic seed germination of Dactylorhiza majalis, D. incarnata var. incarnata, and D. fuchsii, with division on three levels and without division of inflorescence (see text, Material and Methods).
Conversely, in D. fuchsii, the ID estimate increased up to 0.400 in in vitro asymbiotic seed germination without division of inflorescence and across the three inflorescence levels (Figure 3). Other ID values in D. fuchsii were near zero (Figure 3).
Moderate ID was recorded for seed length, seed number per fruit, and the proportion of seeds with well-developed embryos in D. incarnata var. incarnata (Figure 3). PL was high, reaching 0.481 in D. fuchsii, 0.643 in D. incarnata var. incarnata, and the highest at 0.663 in D. majalis.
5 Discussion
5.1 Potential of inbreeding depression
The link between mating systems and inbreeding depression (ID) has been well-established in many studies. Smithson (2006) discovered that nectarless orchids tend to exhibit high levels of ID, which may be indicative of reduced self-fertilization (autogamy and/or geitonogamy). In orchids employing food-deceptive strategies, particularly those that are self-compatible, high ID is often observed. This is likely due to the fact that deceived pollinators typically visit only a few flowers among plants within populations, thereby promoting cross-pollination and reducing the likelihood of inbreeding (Ackerman, 1986; Dafni, 1987; Gill, 1989; Nilsson, 1992; Neiland & Wilcock, 1998; Jersáková et al., 2006; Juillet et al., 2006; Kropf & Renner, 2008; Jacquemyn & Brys, 2020). However, it appears that the impact of ID varies across different fitness traits. Smithson (2006) and Sletvold et al. (2012) noted significant ID in food-deceptive Orchidaceae, particularly in the early stages of life history, ranging from fruit set to protocorm development. Our study of Dactylorhiza orchids revealed that ID varied considerably among reproductive traits. In early life stages, such as seed viability, distinct patterns of ID were observed, suggesting that deceptive strategies in these orchids cannot be attributed to a single mechanism for reproductive success. In D. fuchsii, significant ID was evident, with outcrossed progeny demonstrating superior fitness compared to selfed progeny. This shows a strong outcrossing mating system, as described by Husband & Schemske (1996). However, the frequency of in vitro germinated seeds from selfed and control-pollinated flowers was similar, yet lower than that from outcross pollination. It provided only partial support for the hypothesis that inbred progeny harboring deleterious alleles are eliminated during over-fertilization or early seed development.
The observed cumulative δ value in D. fuchsii (0.366) aligns roughly with previous estimates for other species, such as D. sambucina (δ = 0.42, Nilsson, 1980), but is lower than reported by Jersáková et al. (2006), (δ = 0.63) and Juillet et al. (2006), (δ = 0.47). This value was similar to Smithson (2006) findings for nectarless orchids (δ = 0.32 ± 0.05) and lower than that reported by Husband & Schemske (1996) for allogamous angiosperms (δ = 0.53). The remarkably low ID in D. majalis and the absence of ID in D. incarnata var. incarnata raise intriguing questions. In D. majalis, strong outbreeding depression, evidenced by differences in seed length, well-developed embryos, and in vitro seed germination between outcross and self-progeny, was observed. However, no significant differences were noted across three inflorescence levels. Dactylorhiza incarnata var. incarnata exhibited outbreeding depression in seed germination, while hand-selfed flowers produced seeds with similar viability to naturally pollinated flowers. These results suggest that in D. majalis and D. incarnata var. incarnata, pollinator limitation is likely, and ID is negligible, with biparental inbreeding being more common. The negligible ID in D. incarnata var. incarnata (δ = 0.065) may reflect a historical prevalence of selfing, leading to a reduced genetic load and decreased inbreeding depression (Husband & Schemske, 1996). Hedrén & Nordström (2009) proposed that populations of D. incarnata var. incarnata might consist of several inbred lines. This hypothesis is supported by studies from Naczk et al. (2018) and Vallius et al. (2004). The extremely low ID in the allopolyploid D. majalis, potentially exacerbated by within-population relative pollination, suggests reduced fitness due to detrimental alleles at a local geographic scale (Husband et al., 2008). There is evidence suggesting a lack of selection against selfed progeny in this polyploid. It is plausible that inbred progeny harboring deleterious alleles are not eliminated during over-fertilization or early germination phases (Ortiz-Barney & Ackerman, 1999). Dactylorhiza majalis, an allotetrapolyploid relative to its diploid progenitors D. incarnata and D. fuchsii (Pillon et al., 2007), represents a complex taxonomic group that evolved through multiple and independent hybridization events. The genus’s taxonomic complexity is likely attributable to its migration history during glaciations and interglacial periods, as well as episodes of polyploidization. Otto & Whitton (2000) noted that taxa belonging to polyploidy, which are at or near mutation-selection equilibrium, are expected to harbor greater genetic loads than comparable diploids, potentially leading to higher levels of ID in the progenitors (Ronfort, 1999; Pannell et al., 2004).
5.2 Mating system and pollen limitation
A mixed mating system was observed in all studied Dactylorhiza taxa (Hedrén & Nordström, 2009; Ostrowiecka et al., 2019). Ostrowiecka et al. (2019) found that pollinator behavior in D. majalis likely promotes geitonogamy, explaining the development of selfed seeds in fruits at different levels of the inflorescence with germination potential similar to that of outcrosses within populations. Our results, particularly concerning the level of fruit set, corroborate previous studies on Dactylorhiza (Neiland & Wilcock, 1998; Vallius, 2000; Claessens and Kleynen, 2011). We did not confirm spontaneous autogamy in the three Dactylorhiza taxa, and our findings affirm that these taxa are highly dependent on pollinators, with a notable presence of pollen limitation, especially in D. majalis. This observation aligns with the common occurrence of pollen limitation in deceptive orchids (Tremblay, 2006; Henneresse et al., 2017). The foraging behavior of deceived pollinators likely results in the transfer of low amounts of pollen per visit, increasing the risk of pollen limitation and reducing the likelihood of receiving pollen from different individuals. Ostrowiecka et al. (2019) reported that mean visitation frequency by Apis mellifera was extremely low and time of visits of A. mellifera on inflorescences lasted from 11-40 s in studied D. majalis. Additionally, our data suggest that pollinator foraging behavior remained consistent across the inflorescence during the flowering season, indicating that flower position on the inflorescence did not significantly impact fitness components such as seed length, well-developed seeds, and in vitro asymbiotic seed germination. However, the observed decrease in seed number per fruit from the lower to the upper level of the inflorescence is typically attributed to the resource hypothesis, suggesting increased competition for resources among pollinated flowers (Devlin, 1989; Berry & Calvo, 1991; Obeso, 1993; Diggle, 1995). This decrease in seed set across the inflorescence has also been noted in Dactylorhiza maculata and Epipactis helleborine, potentially due to limited resources and morphological differences in the upper flowers (Light & MacConaill, 1998; Vallius, 2000). In summary, our study as the first revealed that fitness traits in early life stage in three Dactylorhiza taxa depend significantly on type of mating system, but little influence on flower position on inflorescence. These hypotheses warrant further investigation in the context of the three taxa studied.
6 Conclusions
Our experimental findings revealed that high ID was maintained only in the diploid, food-deceptive D. fuchsii, particularly at the asymbiotic in vitro germination stage. In contrast, ID manifested at low to moderate levels in the allotetraploid D. majalis and, to some extent, in its diploid ancestor D. incarnata var. incarnata, with little evidence of selection against selfed offspring. Additionally, our study showed that the mating system, rather than the position of flowers on the inflorescence, primarily influenced the quality and quantity of reproductive output. The impact of ID on the germination life stage played a significant role in the reproductive ecology of these taxa.
Data availability statement
The original contributions presented in the study are included in the article/Supplementary Material. Further inquiries can be directed to the corresponding author.
Author contributions
AW: Conceptualization, Analysis, Investigation, Methodology, Project administration, Writing – original draft, editing. BO: Investigation, Writing – original draft. EB: Investigation, Writing – original draft. EJ: Investigation, Writing – original draft. IT: Investigation, Writing – original draft. PM: Analysis with R package, Writing – original draft. All authors contributed to the article and approved the submitted version.
Funding
The author(s) declare financial support was received for the research, authorship, and/or publication of this article. This study was financially supported by a grant from the National Science Center in Poland (no. 2013/09/B/NZ8/03350).
Acknowledgments
We thank two reviewers for their many insightful comments and suggestions, helped to improve our manuscript.
Conflict of interest
The authors declare that the research was conducted in the absence of any commercial or financial relationships that could be construed as a potential conflict of interest.
Publisher’s note
All claims expressed in this article are solely those of the authors and do not necessarily represent those of their affiliated organizations, or those of the publisher, the editors and the reviewers. Any product that may be evaluated in this article, or claim that may be made by its manufacturer, is not guaranteed or endorsed by the publisher.
Supplementary material
The Supplementary Material for this article can be found online at: https://www.frontiersin.org/articles/10.3389/fpls.2024.1244393/full#supplementary-material
References
Ackerman, J. (1986). Mechanisms and evolution of food-deceptive pollination systems in orchids. Lindleyana 1, 108–113. doi: 10.1017/S1464793105006986
Ågren, J., Schemske, D. W. (1993). Outcrossing rate and inbreeding depression in two annual monoecious herbs, Begonia hirsuta and B. semiovata. Evolution 47, 125–135. doi: 10.2307/2410123
Angeloni, F., Ouborg, N. J., Leimu, R. (2011). Meta-analysis on the association of population size and life history with inbreeding depression in plants. Biol. Conserv. 144, 35–43. doi: 10.1016/j.biocon.2010.08.016
Balao, F., Tannhäuser, M., Lorenzo, M. T., Hedren, M., Paun, O. (2016). Genetic differentiation and admixture between sibling allopolyploids in the Dactylorhiza majalis complex. Heredity 116, 351–361. doi: 10.1038/hdy.2015.98
Barrett, S. C., Harder, L. D. (2017). The ecology of mating and its evolutionary consequences in seed plants. Ann. Rev. Ecol. Evol. Syst. 48, 135–157. doi: 10.1146/annurev-ecolsys-110316-023021
Barringer, B. C., Geber, M. A. (2008). Mating system and ploidy influence levels of inbreeding depression in Clarkia (Onagraceae). Evol. Int. J. Org. Evol. 62, 1040–1051. doi: 10.1111/j.1558-5646.2008.00361.x
Baskin, C. C., Baskin, J. M. (2015). Inbreeding depression and the cost of inbreeding on seed germination. Seed Sci. Res. 25, 355–385. doi: 10.1017/S096025851500032X
Berry, P. E., Calvo, R. N. (1991). Pollinator limitation and position dependent fruit set in the high Andean orchid Myrosmodes cochleare (Orchidaceae). Plant Syst. Evol. 174, 93–101. doi: 10.1007/BF00937697
Blambert, L., Mallet, B., Humeau, L., Pailler, T. (2016). Reproductive patterns, genetic diversity and inbreeding depression in two closely related Jumellea species with contrasting patterns of commonness and distribution. Ann. Bot. 118, 93–103. doi: 10.1093/aob/mcw014
Brandrud, M. K., Baar, J., Lorenzo, M. T., Athanasiadis, A., Bateman, R. M., Chase, M. W., et al. (2020). Phylogenomic relationships of diploids and the origins of allotetraploids in Dactylorhiza (Orchidaceae). Syst. Biol. 69, 91–109. doi: 10.1093/sysbio/syz035
Carr, D. E., Duasch, M. R. (1996). Inbreeding depression in two species of Mimulus (Scrophulariaceae) with contrasting mating systems. Am. J. Bot. 83, 586–593. doi: 10.1002/j.1537-2197.1996.tb12743.x
Charlesworth, D., Charlesworth, B. (1987). Inbreeding depression and its evolutionary consequences. Annu. Rev. Ecol. Syst. 18, 237–268. doi: 10.1146/annurev.es.18.110187.001321
Claessens, J., Kleynen, J. (2011). The flower of the European orchid, form and function (Voerendaal: Jean Claessens and Jacques Kleynen).
Clo, J. (2022). The evolution of the additive variance of a trait under stabilizing selection after autopolyploidization. J. Evol. Biol. 35, 891–897. doi: 10.1111/jeb.14010
Dafni, A. (1987). “Floral mimicry - mutualism and unidirectional exploitation of insects by plants,” in Insects and the plant surface. Eds. Southwood, T. R. F., Juniper, B. E. (Arnold, London), 6.
Devlin, B. (1989). Components of seed and pollen yield of Lobelia cardinalis, variation and correlations. Am. J. Bot. 76, 204–214. doi: 10.1002/j.1537-2197.1989.tb11303.x
Diggle, P. K. (1995). Architectural effects and the interpretation of fruit and seed development. Annu. Rev. Ecol. Syst. 26, 531–552. doi: 10.1146/annurev.es.26.110195.002531
Ferdy, J. B., Loriot, S., Sandmeier, M., Lefranc, M., Raquin, C. (2001). Inbreeding depression in a rare deceptive orchid. Can. J. Bot. 79, 1181–1188. doi: 10.1139/cjb-79-10-1181
Gill, D. E. (1989). “Fruiting failure, pollinator inefficiency, and speciation in orchids,” in Speciation and its consequences. Eds. Otte, D., Endler, J. A. (Sinauer Associates, Sunderland), 433–457.
Glaettli, M., Goudet, J. (2006). Inbreeding effects on progeny sex ratio and gender variation in the gynodioecious Silene vulgaris (Caryophyllaceae). New Phytol. 172, 763–777. doi: 10.1111/j.1469-8137.2006.01866.x
González-Varo, J. P., Arroyo, J., Aparicio, A. (2009). Effects of fragmentation on pollinator assemblage, pollen limitation and seed production of Mediterranean myrtle (Myrtus communis). Biol. Conserv. 142, 1058–1065. doi: 10.1016/j.biocon.2009.01.017
Hawranek, A.-S. (2021). The impact of recurrent origins and gene flow on the genetic structure of allopolyploid marsh orchids (Dactylorhiza, Orchidaceae). the Master’s Thesis. Viena University. submitted by BSc.
Hedrén, M., Nordström, S. (2009). Polymorphic populations of Dactylorhiza incarnata s.l. (Orchidaceae) on the Baltic island of Gotland, Morphology, habitat preference and genetic differentiation. Ann. Bot. 104, 527–542. doi: 10.1093/aob/mcp102
Henneresse, T., Wesselingh, R. A., Tyteca, D. (2017). Effects of floral display, conspecific density and rewarding species on fruit set in the deceptive orchid Orchis militaris (Orchidaceae). Plant Ecol. Evol. 150, 279–292. doi: 10.5091/plecevo.2017.1313
Husband, B. C., Ozimec, B. S., Pollock, M. L. (2008). Mating consequences of polyploid evolution in flowering plants, current trends and insights from synthetic polyploids. Int. J. Plant Sci. 169, 195–206. doi: 10.1086/523367
Husband, B. C., Schemske, D. W. (1996). Evolution of the magnitude and timing of inbreeding depression in plants. Evolution 50, 54–70. doi: 10.2307/2410780
Jacquemyn, H., Brys, R. (2020). Lack of strong selection pressures maintains wide variation in floral traits in a food-deceptive orchid. Ann. Bot. 126, 445–453. doi: 10.1093/aob/mcaa080
Jersáková, J., Johnson, S. D., Kindlmann, P. (2006). Mechanisms and evolution of deceptive pollination in orchids. Biol. Rev. 81, 219–235. doi: 10.1017/S1464793105006986
Johnston, M. O., Schoen, D. J. (1996). Correlated evolution of self-fertilization and inbreeding depression: an experimental study of nine populations of Amsinckia (Boraginaceae). Evol. 50, 1478–1491. doi: 10.1111/j.1558-5646.1996.tb03921.x
Johnson, S. D., Nilsson, L. A. (1999). Pollen carryover, geitonogamy, and the evolution of deceptive pollination system in orchids. Ecology 80, 2607–2619. doi: 10.1890/0012-9658(1999)080[2607:PCGATE]2.0.CO;2
Juillet, N., Dunand-Martin, S., Gigord, L. D. B. (2006). Evidence for inbreeding depression in the food-deceptive colour-dimorphic orchid Dactylorhiza sambucina (L.) soò. Plant Biol. 9, 147–151. doi: 10.1055/s-2006-924310
Kindlmann, P., Jersáková, J. (2006). Effect of floral display on reproductive success in terrestrial orchids. Folia Geobotanica 41, 47–60. doi: 10.1007/BF02805261
Kropf, M., Renner, S. S. (2008). Pollinator-mediated selfing in two deceptive orchids and a review of pollinium tracking studies addressing geitonogamy. Oecologia 155, 497–508. doi: 10.1007/s00442-007-0919-4
Lande, R., Schemske, D. W. (1985). The evolution of self-fertilization and inbreeding depression in plants. I. Genetic models. Evolution 39, 24–40. doi: 10.2307/2408514
Larson, B. M. H., Barrett, S. C. H. (2000). A comparison of pollen limitation in flowering plants. Biol. J. Linn. Soc. 69, 503–520. doi: 10.1111/j.1095-8312.2000.tb01221.x
Lázaro, A., Traveset, A. (2006). Reproductive success of the endangered shrub Buxus balearica Lam. (Buxaceae): pollen limitation, and inbreeding and outbreeding depression. Plant Syst. Evol. 261, 117–128. doi: 10.1007/s00606-005-0404-7
Lemus, R. E. M., Ávila-Díaz, I., Diego, Y. H. (2021). Mating system and female reproductive success of the endemic and endangered epiphyte Rhynchostele cervantesii (Orchidaceae) in a cloud forest in Michoacan, Mexico. Plant Ecol. Evol. 154, 56–62. doi: 10.5091/plecevo.154.1
Light, M. H. S., MacConaill, M. (1998). Factors affecting germinable seed yield in Cypripedium calceolus var. pubescens (Willd.) Correll and Epipactis helleborine (L.) Cranz (Orchidaceae). Botanical J. Linn. Soc. 126, 3–26. doi: 10.1111/j.1095-8339.1998.tb02512.x
Lindén, B. (1980). Aseptic germination of seeds of northern terrestrial orchids. Annales Botanici Fennici 17, 174–182.
Malmgren, S. (1996). “Orchid propagation, theory and practice,” in North American native terrestrial orchids: propagation and production, 1996 Mar 16–17; Germantown, Maryland, United States. Ed. Allen, C. (Germantown, MD: North American Native Terrestrial Orchid Conference), 63–71.
Naczk, A. M., Kowalkowska, A. K., Wiśniewska, N., Haliński, Ł.P., Kapusta, M., Czerwicka, M. (2018). Floral anatomy, ultrastructure and chemical analysis in Dactylorhiza incarnata/maculata complex (Orchidaceae). Botanical J. Linn. Soc. 187, 512–536. doi: 10.1093/botlinnean/boy027/5037731
Neiland, M. R. M., Wilcock, C. C. (1998). Fruit set, nectar reward, and rarity in the Orchidaceae. Am. J. Bot. 85, 1657–1671. doi: 10.2307/2446499
Nilsson, L. A. (1980). The pollination ecology of Dactylorhiza sambucina (Orchidaceae). Botaniska Not. 133, 367–385.
Nilsson, L. A. (1992). Animal pollinators adjust plant gender in relation to floral display, Evidence from Orchis morio (Orchidaceae). Evolutionary Trends Plants 6, 33–40.
Obeso, J. R. (1993). Seed mass variation in the perennial herb Asphodelus albus, sources of variation and position effect. Oecologia 93, 571–575. doi: 10.1007/BF00328967
Ortiz-Barney, E., Ackerman, J. D. (1999). The cost of selfing in Encyclia cochleata (Orchidaceae). Plant Syst. Evol. 219, 55–64. Available at: https://jstor.org/stable/23643728.
Ostrowiecka, B., Tałałaj, I., Brzosko, E., Jermakowicz, E., Mirski, P., Kostro-Ambroziak, A., et al. (2019). Pollinators and visitors of the generalized food-deceptive orchid Dactylorhiza majalis in North-Eastern Poland. Biologia 74, 1247–1257. doi: 10.2478/s11756-019-00285-0
Otto, S. P., Whitton, J. (2000). Polyploid incidence and evolution. Annu. Rev. Genet. 34, 401–437. doi: 10.1146/annurev.genet.34.1.401
Pannell, J. R., Obbard, D. J., Buggs, R. J. A. (2004). Polyploidy and the sexual system, what can we learn from Mercurialis annua? Evolution 82, 547–560. doi: 10.1111/J.1095-8312.2004.00340.X
Pillon, Y., Hedrén, M. F., Bateman, M., Devey, R. M., Shipunov, D. S., van der Bank, A. B., et al. (2007). Evolution and temporal diversification of western European polyploid species complexes in Dactylorhiza (Orchidaceae). Taxon. 56, 1185–1208. doi: 10.2307/25065911
R Core Team (2021). R, A language and environment for statistical computing Vol. 79 (Vienna, Austria: R Foundation for Statistical Computing), 226–230. Available at: https://www.R-project.org/. doi: 10.1111/j.1399-3054.1990.tb06734.x
Ronfort, J. (1999). The mutation load under tetrasomic inheritance and its consequences for the evolution of the selfing rate in autotetraploid species. Genetical Res. 74, 31–42. doi: 10.1017/S0016672399003845
Rosquist, G. (2001). Reproductive biology in diploid Anthericum ramosum and tetraploid A. liliago (Anthericaceae). Oikos 92, 143–152. doi: 10.1034/j.1600-0706.2001.920117.x
Sletvold, N., Grindeland, J. M., Zu, P., Ågren, J. (2012). Strong inbreeding depression and local outbreeding depression in the rewarding orchid Gymnadenia conopsea. Conserv. Genet. 13, 1305–1315. doi: 10.1007/s10592-012-0373-7
Smithson, A. (2006). Pollinator limitation and inbreeding depression in orchid species with and without nectar rewards. New Phytol. 169, 419–430. doi: 10.1111/j.1469-8137.2005.01592.x
Tremblay, R. L. (2006). The effect of flower position on male and female reproductive success in a deceptively pollinated tropical orchid. Botanical J. Linn. Soc. 151, 405–410. doi: 10.1111/j.1095-8339.2006.00504.x
Tremblay, R. L., Ackerman, J. D., Zimmerman, J. K., Calvo, R. N. (2005). Variation in sexual reproduction in orchids and its evolutionary consequences, a spasmodic journey to diversification. Biol. J. Linn. Soc. 84, 1–54. doi: 10.1111/j.1095-8312.2004.00400.x
Vakhrameeva, M. G., Tatarenko, I. V., Varlygina, T. I., Torosyan, G. K., Zagulskiy, M. N. (2008). Orchids of Russia and adjacent countries (Ruggell: A. R. G. Gantner Verlag).
Vallius, E. (2000). Position-dependent reproductive success of flowers in Dactylorhiza maculata (Orchidaceae). Funct. Ecol. 14, 573–579. doi: 10.1046/j.1365-2435.2000.t01-1-00450.x
Vallius, E., Salonen, V., Kull, T. (2004). Factors of divergence in co-occurring varieties of Dactylorhiza incarnata (Orchidaceae). Plant Syst. Evol. 248, 177–189. doi: 10.1007/s00606-004-0192-5
Valtueña, F. J., López, J., Ortega-Olivencia, A., Rodriguez-Riañ, O. T., González, M. (2014). Contrasting inbreeding depression in early and late stages of the life cycle of a Mediterranean shrub, Anagyris foetida (Leguminosae). Turkish J. Bot. 38, 334–346. doi: 10.3906/bot-1303-28
Vandepitte, K., Jacquemyn, H., Roldán-Ruiz, I., Honnay, O. (2011). The consequences of mating over a range of parental genetic similarity in a selfing allopolyploid plant species. J. Evol. Biol. 24, 2750–2758. doi: 10.1111/jeb.2011.24.issue-12
Keywords: Dactylorhiza fuchsii, Dactylorhiza incarnata var. incarnata, Dactylorhiza majalis, fruit set, inbreeding depression, in vitro asymbiotic seed germination
Citation: Wróblewska A, Ostrowiecka B, Brzosko E, Jermakowicz E, Tałałaj I and Mirski P (2024) The patterns of inbreeding depression in food-deceptive Dactylorhiza orchids. Front. Plant Sci. 15:1244393. doi: 10.3389/fpls.2024.1244393
Received: 22 June 2023; Accepted: 04 March 2024;
Published: 25 March 2024.
Edited by:
John Kelly, University of Kansas, United StatesReviewed by:
Rong Wang, East China Normal University, ChinaShu-Mei Chang, University of Georgia, United States
Copyright © 2024 Wróblewska, Ostrowiecka, Brzosko, Jermakowicz, Tałałaj and Mirski. This is an open-access article distributed under the terms of the Creative Commons Attribution License (CC BY). The use, distribution or reproduction in other forums is permitted, provided the original author(s) and the copyright owner(s) are credited and that the original publication in this journal is cited, in accordance with accepted academic practice. No use, distribution or reproduction is permitted which does not comply with these terms.
*Correspondence: Ada Wróblewska, YWRhYm90QHV3Yi5lZHUucGw=