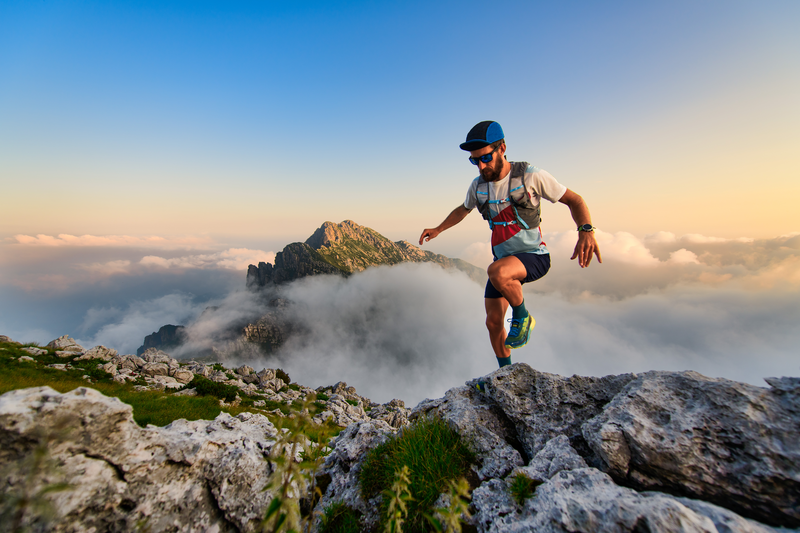
95% of researchers rate our articles as excellent or good
Learn more about the work of our research integrity team to safeguard the quality of each article we publish.
Find out more
EDITORIAL article
Front. Plant Sci. , 16 January 2024
Sec. Plant Physiology
Volume 14 - 2023 | https://doi.org/10.3389/fpls.2023.1334467
This article is part of the Research Topic Abiotic Stress Signaling in Plants: Functional Genomic Intervention, Volume II View all 18 articles
Editorial on the Research Topic
Abiotic stress signaling in plants: functional genomic intervention, volume II
Abiotic stresses such as high temperature, low temperature, drought, and salinity limit crop productivity worldwide. Understanding plant responses to these stresses is essential for the engineering of stress tolerance in plants. In Arabidopsis, the signal transduction pathways have been elucidated for perceiving and responding to different abiotic stress conditions (Zhu, 2016). A significant portion of plant genomes codes for proteins involved in signaling such as receptors, sensors, kinases, phosphatases, transcription factors, and transporters/channels (Pandey, 2020; Chen et al., 2021). Despite decades of physiological and molecular efforts, knowledge about how plants sense temperature fluctuations, drought, water submergence, and salinity signals is still limited. Further, the stress combinations pose another layer of complexity to this problem (Zandalinas et al., 2023). One of the major constraints hampering our understanding of these signal transduction processes has been the slow pace of gene-function studies in plants (Shinozaki and Yamaguchi-Shinozaki, 2022). In this post-genomic era, investigation and understanding of multiple genes and gene families regulating a particular physiological and developmental aspect of plant’s life cycle is of utmost importance (Pandey et al., 2016; Baekelandt et al., 2023; Tyczewska et al., 2023). With a holistic understanding of the signaling pathways involving not only one gene but multiple genes from a signaling cascade, plant biologists can lay a foundation for designing and generating future crops that can withstand environmental stresses without jeopardizing the crop yield.
In the present Research Topic, various novel genes, mainly transcription factors, have been identified and characterized, that can serve as promising candidates for enhancing abiotic stress tolerance in crops. Han et al. have functionally characterized GmWRKY21 that encodes a nuclear-localized WRKY transcription factor. GmWRKY21 is expressed across different tissues including roots, leaves, and flowers of soybean and is substantially upregulated in response to aluminum stress. The overexpression of GmWRKY21 in Arabidopsis increased the root growth of seedlings in transgenic lines under aluminum stress, which coincided with higher proline and lower malondialdehyde (MDA) accumulation and upregulated expression of aluminum stress-specific as well as general stress marker genes. Similarly, Wang et al. identified GLYCINE MAX ABA REPRESSOR1 (GmABR1) encoding an Ethylene Response Factor (ERF) transcription factor as a positive regulator of aluminum stress tolerance in Arabidopsis thaliana. The Basic Leucine Zipper (bZIP) family represents another large family of transcription factors that play an important role in mediating plant responses to abiotic stresses. Samtani et al. identified two homeologs of wheat OCS-ELEMENT BINDING FACTOR1 (TaOBF1-5B and TaOBF1-5D) as heat-responsive TabZIP members. The heterologous overexpression of TaOBF1-5B resulted in enhanced thermotolerance in transgenic Arabidopsis thaliana and Oryza sativa. TaOBF1-5B has been shown to interact with HEAT SHOCK PROTEIN70 (TaHSP90) in the nucleus and TaSTI (a co-chaperone) in the nucleolus. MYBs (myeloblastosis) are another class of transcription factors that play a pivotal role in regulating anthocyanin synthesis. Liu et al. identified and characterized PqMYB113 from the leaves of Paeonia qiui, a wild species of tree peony native to China. The overexpression of PqMYB113 resulted in increased anthocyanin accumulation in Arabidopsis thaliana and Nicotiana tabacum.
Similarly, Jin et al. reported on a homolog of the RelA/SpoT protein (HpRSH) from Haematococcus pluvialis, which catalyzes Mg2+-dependent synthesis and hydrolysis of guanosine tetraphosphate (ppGpp). The transcription of HpRSH was significantly upregulated by environmental stresses, such as darkness, high light, nitrogen limitation, and salinity stress. Apart from these positive regulators, Ge et al. identified STRESS-INDUCED DUF1644 (OsSIDP301), a member of the DUF1644 (Domain of unknown function 1644) family, as a negative regulator of salt stress and grain size in rice. OsSIDP301 determines salt stress tolerance by modulating genes involved in the salt-response and ABA signaling pathways. Moreover, OsSIDP301 interacts with OsBUL1 COMPLEX1 (OsBC1), which positively regulates grain size in rice.
Another approach to identify the stress-responsive gene(s) and associated physiological processes is to compare the contrasting accessions/varieties. Gao et al. performed the phenotypic and metabolic level comparison of two oat cultivars, Baiyan7 (BY, tolerant cultivar) and Yizhangyan4 (YZY, sensitive cultivar) under saline-alkali stress, which is a major detrimental abiotic stress factor for reducing agricultural productivity. Saline-alkali induced metabolites were mainly associated with enhancements in energy metabolism and accumulations of organic acids. The differential abundances of these metabolites revealed that BY showed better adaptability to saline-alkali stress compared with YZY cultivars. Similarly, Dutta et al. performed a genome-wide analysis and identified 10 cytosine-5 DNA methyltransferases (C5-MTases) and 8 demethylases (DeMets) in potatoes. Based on comparative expression profiling in heat-sensitive (HS) and -tolerant (HT) genotypes, several positive [SELF PRUNING 6A (StSP6A) and BEL1-LIKE HOMEODOMAIN (StBEL5)] and negative [SELF PRUNING 5G (StSP5G), SUCROSE TRANSPORTER4 (StSUT4), and RELATED TO AP2 1 (StRAP1)] regulators were identified that regulate potato tuberization under high-temperature stress.
Genome-wide analyses of gene families followed by ranking analysis have also been reported in the Research Topic to identify the key genes. For instance, CALCIUM-DEPENDENT PROTEIN KINASES (CDPKs) are a major group of calcium (Ca2+) sensors in plants. CDPKs play a dual function of “Ca2+ sensor and responder.” Deepika et al. identified commonly induced CaCDPKs in response to drought, salt, and abscisic acid in chickpea. Rajasheker et al. reported the stress-responsive behavior of proline-rich proteins and hybrid proline-rich proteins super family genes in Sorghum bicolor. Further, “omics” based approaches have also been used for the identification of genes and regulatory networks associated with nitrogen (N) addition in Solidago canadensis (Wu et al.), hydrogen peroxide stress in pepper (Tang et al.) and coleoptile senescence in rice (Sasi et al.).
Apart from genetic engineering, priming with microbes and chemicals are also used as alternatives to enhance plant performance under stress conditions. Li et al. demonstrated the protective role of Piriformospora indica (a root endophytic fungus) in wheat against Rhizoctonia cerealis and Fusarium graminearum infections. RNA-seq suggested that transcriptome changes caused by F. graminearum were more severe than those caused by R. cerealis. Further, the supplementation of P. indica reduced the number of differentially expressed genes (DEGs) by 18% and 58% in wheat injected with F. graminearum and R. cerealis, respectively, indicating the activation of specific mechanisms. Further, the DEGs related to disease resistance, such as WRKYs and MAPKs were upregulated in wheat by P. indica colonization.
In addition to the research articles, the Research Topic also featured fundamental reviews. For instance, Lim et al. reviewed the core signaling and function of ABA, which is one of the major stress hormones in plants. In addition, the role of non-hormone signal mediators was also highlighted. Li et al. provided a comprehensive insight into sulfur dioxide, which has recently emerged as a signaling mediator in plants. Finally, Khan et al. reviewed the recent advances in the application of thermal techniques for inducing stress-priming during the seedling stage, which is another non-genetic approach.
Taken together, the Research Topic highlights the novel research findings in the field of abiotic stress signaling in plants, that may pave the path for developing “stress-tolerant” crops in the near future.
AS: Writing – original draft, Writing – review & editing. AP: Writing – review & editing. MB: Writing – review & editing. GP: Conceptualization, Funding acquisition, Writing – original draft, Writing – review & editing.
The author(s) declare that financial support was received for the research, authorship, and/or publication of this article. GP is thankful to the Department of Biotechnology (DBT), Govt. of India; Science and Engineering Research Board (SERB), Govt. of India; Council for Scientific and Industrial Research (CSIR), Govt. of India; Delhi University (IoE/FRP grant), India for research funding.
The authors declare that the research was conducted in the absence of any commercial or financial relationships that could be construed as a potential conflict of interest.
All claims expressed in this article are solely those of the authors and do not necessarily represent those of their affiliated organizations, or those of the publisher, the editors and the reviewers. Any product that may be evaluated in this article, or claim that may be made by its manufacturer, is not guaranteed or endorsed by the publisher.
Baekelandt, A., Saltenis, V. L., Nacry, P., Malyska, A., Cornelissen, M., Nanda, A. K., et al. (2023). Paving the way towards future-proofing our crops. Food Energy Secur. 12 (3), e441. doi: 10.1002/fes3.441
Chen, X., Ding, Y., Yang, Y., Song, C., Wang, B., Yang, S., et al. (2021). Protein kinases in plant responses to drought, salt, and cold stress. J. Integr. Plant Biol. 63 (1), 53–78. doi: 10.1111/jipb.13061
Pandey, G. K. (2020). Protein phosphatases and stress management in plants: Functional genomics perspective (Switzerland: Springer International Publishing).
Pandey, G. K., Prasad, M., Pandey, A., Böhmer, M. (Eds.) (2016). Abiotic Stress Signaling in Plants: Functional Genomic Intervention (Lausanne: Frontiers Media). doi: 10.3389/978-2-88919-891-7
Shinozaki, K., Yamaguchi-Shinozaki, K. (2022). Functional genomics in plant abiotic stress responses and tolerance: From gene discovery to complex regulatory networks and their application in breeding. Proc. Japan Academy Ser. B 98 (8), 470–492. doi: 10.2183/pjab.98.024
Tyczewska, A., Twardowski, T., Woźniak-Gientka, E. (2023). Agricultural biotechnology for sustainable food security. Trends Biotechnol. 41, 331–341. doi: 10.1016/j.tibtech.2022.12.013
Zandalinas, S. I., Peláez-Vico, M. Á., Sinha, R., Pascual, L. S., Mittler, R. (2023). The impact of multifactorial stress combination on plants, crops, and ecosystems: how should we prepare for what comes next? Plant J. doi: 10.1111/tpj.16557
Keywords: abiotic stress, stress signaling, stress tolerance, functional genomics, crop productivity
Citation: Srivastava AK, Pandey A, Böhmer M and Pandey GK (2024) Editorial: Abiotic stress signaling in plants: functional genomic intervention, volume II. Front. Plant Sci. 14:1334467. doi: 10.3389/fpls.2023.1334467
Received: 07 November 2023; Accepted: 28 December 2023;
Published: 16 January 2024.
Edited and Reviewed by:
Anna N. Stepanova, North Carolina State University, United StatesCopyright © 2024 Srivastava, Pandey, Böhmer and Pandey. This is an open-access article distributed under the terms of the Creative Commons Attribution License (CC BY). The use, distribution or reproduction in other forums is permitted, provided the original author(s) and the copyright owner(s) are credited and that the original publication in this journal is cited, in accordance with accepted academic practice. No use, distribution or reproduction is permitted which does not comply with these terms.
*Correspondence: Ashish Kumar Srivastava, YXNoaXNoQGJhcmMuZ292Lmlu; Girdhar Kumar Pandey, Z2twYW5kZXlAc291dGguZHUuYWMuaW4=
Disclaimer: All claims expressed in this article are solely those of the authors and do not necessarily represent those of their affiliated organizations, or those of the publisher, the editors and the reviewers. Any product that may be evaluated in this article or claim that may be made by its manufacturer is not guaranteed or endorsed by the publisher.
Research integrity at Frontiers
Learn more about the work of our research integrity team to safeguard the quality of each article we publish.