- Department of Agricultural Biotechnology, National Institute of Agricultural Sciences (NAS), Rural Development Administration, Jeonju, Republic of Korea
Over the decades, extensive research efforts have been undertaken to understand how secondary plant metabolites are affected by genetic, environmental, and agronomic factors. Understanding the genetic basis of stress-response metabolite biosynthesis is crucial for sustainable agriculture production amidst frequent occurrence of climatic anomalies. Although it is known that environmental factors influence phytochemical profiles and their content, studies of plant compounds in relation to stress mitigation are only emerging and largely hindered by phytochemical diversities and technical shortcomings in measurement techniques. Despite these challenges, considerable success has been achieved in profiling of secondary metabolites such as glucosinolates, flavonoids, carotenoids, phenolic acids and alkaloids. In this study, we aimed to understand the roles of glucosinolates, flavonoids, carotenoids, phenolic acids and alkaloids in relation to their abiotic stress response, with a focus on the developing of stress-resilient crops. The focal genus is the Brassica since it (i) possesses variety of specialized phytochemicals that are important for its plant defense against major abiotic stresses, and (ii) hosts many economically important crops that are sensitive to adverse growth conditions. We summarize that augmented levels of specialized metabolites in Brassica primarily function as stress mitigators against oxidative stress, which is a secondary stressor in many abiotic stresses. Furthermore, it is clear that functional characterization of stress-response metabolites or their genetic pathways describing biosynthesis is essential for developing stress-resilient Brassica crops.
1 Introduction
Climate change will intensify the adverse effects of both biotic and abiotic stress conditions, thus threatening agricultural sustainability and ultimately leading to economic losses (Lozano-Elena et al., 2022). Furthermore, it is eminent that future predictions and forecasts on climatic conditions indicate the possibility of an increase in the frequency and severity of major abiotic stress constraints, including drought, salinity, cold, and low/high temperatures. The plant stress response pathway is highly intricate and regulated at multiple levels through integrated and coordinated signals perceived by sensors to adjust or adapt to adverse conditions. Therefore, it is important to identify suitable screening indices and quantifiable traits to facilitate the production of stress-resilient crops. For this purpose, the top priorities will include gaining insights into the role of multiple players at physiological, biochemical, and molecular levels in plant stress response mechanisms. To date, there have been several comprehensive analyses of the molecular biology of plant stress adaptive mechanisms, and the outcomes have been useful in significantly improving key agronomic traits such as plant growth and yield performance in stressed crops. Nevertheless, it is essential to implement innovative and alternative approaches considering the bottlenecks in our understanding the plants stress responses, given their polygenic nature and the occurrence of more prevalent episodes of climate abnormalities. It is known that plants continuously evolve to mitigate the stressors by developing constitutive, active, inducible, and tightly regulated immune and defense systems. In terms of metabolic adjustments to stress signals, plant cells coordinate their metabolic pathways to produce anti-stress agents focused on maintaining homeostasis inside cells. A generalized metabolic pathways leading to secondary metabolite biosynthesis/production was described in Figure 1.
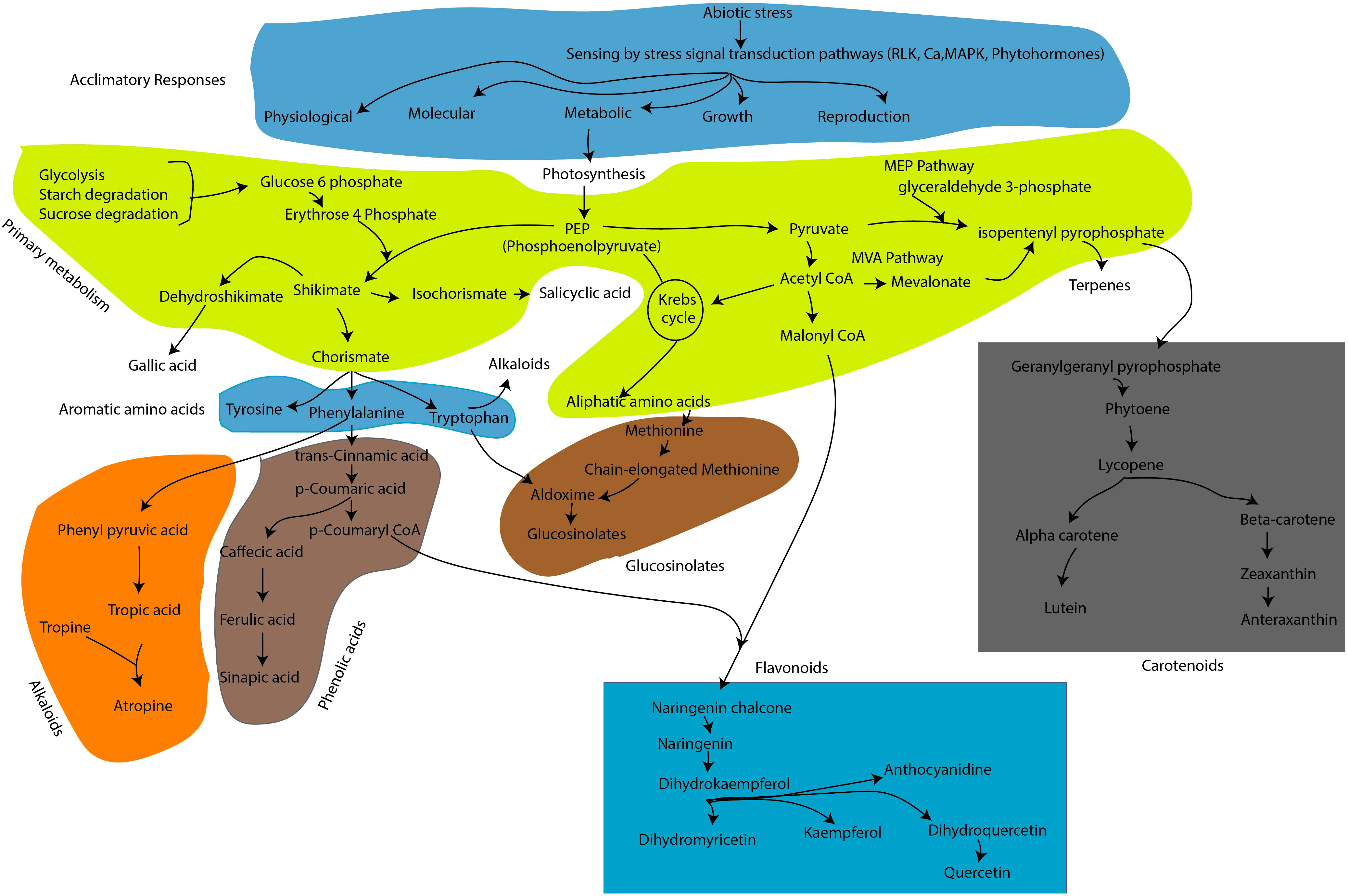
Figure 1 Schematic representation of metabolic pathways leading to secondary metabolite production in response to plant stress signals. For convenience of the readers, cascades of enzymatic reaction or other regulatory elements and intermediate biosynthesis were excluded in this figures. In figure, the biosynthesis pathways of flavonoid, carotenoid, phenolic acid, glucosinolates and alkaloids were illustrated among the several known secondary metabolites in plants.
Among the plant stress mitigation strategies, accumulation of secondary metabolites with selective stress-protective effects against growth limiting environmental constraints has been fairly studied and it continues to evolve with the assistance of technical advancements in specific and targeted metabolite profiling and quantification approaches. The literature shows that information on stress-response metabolite profile is rapidly expanding in recent times, indicating their potential role in plant stress metabolic adaptation (Chan et al., 2013). Synchronization with environmental growth constraints, factors such as diurnal or circadian oscillations also exert influence over the production of defense chemicals in plants (Doghri et al., 2022). This indicates that multiple factors modulate secondary metabolite production, which makes our tasks difficult in identification of precise metabolites for a particular stress conditions. This may be one of the reasons why the success story of producing climate-resilient crops is still elusive. Metabolomics approaches targeting qualitative and quantitative profiling of phytochemicals in plants involved in stress responses could provide valuable information about the metabolic adaptation of plants under adverse conditions (Shaw et al., 2022) since the metabolic profile has a direct relationship with the phenotype (Viana et al., 2022). Additionally, genetic pathways describing the metabolite biosynthesis and regulatory elements that control metabolite accumulation/reduction could offer the necessary information for genetic manipulation strategies aiming at developing stress-smart crops.
In general, plant metabolism can be influenced by several factors, including climatic factors (such as season, light, water, temperature, CO2 levels, air and soil pollutants), biotic factors (including plant species, genotype, pests, pathogens, and competitive habitats/weeds), and agronomic factors (such as soil type, fertilizer type, pesticides, cultural practices, and habitat manipulation) (Björkman et al., 2011). It is widely believed that over 50% of the estimated secondary metabolites (over 200,000) in plants are thought to be influenced by stress conditions, and some of these compounds could serve various defense roles in plants, including acting as antioxidants, antifungal agents, antibacterial agents, ant herbivore deterrents, and stress-inducible phytohormones, among others (Kessler and Kalske, 2018). The plant defense compounds are diverse in nature and comprises of several known metabolites such as terpenoids, alkaloids, phenolics, steroids, flavonoids, tannins, and possibly many other unidentified metabolites (Pant et al., 2021). In most cases, stress response production of secondary metabolites such as glucosinolates, carotenoids, flavonoids, phenolic acids, and alkaloids enhance plant antioxidant efficiencies through mitigating the oxidative stress, which is a secondary stressor of major abiotic stresses in plants, such as drought, salinity, cold and high temperature, among others. These plant defense associated secondary metabolites generally show increased levels after encountering both biotic and abiotic stresses. It is worth noting that these phytochemicals and their degradation products, possesses a wide range of biological properties, including antioxidant, anticancer, antimicrobial, anti-inflammatory, antidiabetic, and neuroprotective activities in the human diet (Ayadi et al., 2022). Consequently, abiotic stress factors that influence the quantity and quality of metabolite content may impact the nutritional benefits for both animal and human health. Therefore, controlling the optimal growth factors in crop plants is essential to harness the specific metabolite accumulation and/or maintaining desired metabolite profiles in edible parts of the plants and more importantly to maintain the intrinsic quality, including color, aroma, taste, and beneficial health properties of host plants. For instance, excess accumulation of certain glucosinolates (GSLs) acts as anti-nutritional factors in Brassica plants (Miao et al., 2021). Nonetheless, these stress-induced specialized compounds are valuable in mitigating adverse growth conditions through multiple mechanisms (Björkman et al., 2011). Also, previous studies analyzing the stress-responsive metabolic adaptation potential of glucosinolates, sterols, terpenes, and flavonoids proved their biological significance in plant abiotic stress responses (Nephali et al., 2020). Considering the biological significance of several known and a larger fraction of unknown defense chemicals, their diversities and multifactor-driven production mechanisms it is clear that our current understanding of the stress responsive metabolic adaptation of pants is far from complete. However, it is encouraging that modern technical advances in farming practices and analytical chemistry has enabled the identification of metabolites that accumulate under particular physiological conditions (Ljubej et al., 2021c), thereby enhancing our knowledge of their biological significance.
Our area of interest is the Brassica genus, commonly grown in the Mediterranean area, and their production is greatly affected by unfavorable environmental conditions. Brassica crops are widely cultivated due to its nutritional and economic significance within the Brassicaceae family, commonly referred to as crucifers. Considering this significance and the frequent occurrence of environmental growth constraints, it is crucial for the research community to develop strategies for dissecting the metabolic adaptation mechanism under stress conditions in Brassica vegetables and oils (Wu et al., 2021). The principal vegetable species belonging to the Brassica genus include Brassica oleracea (e.g., broccoli, cabbage, cauliflower, kale, Brussels sprouts, etc.), Brassica rapa (e.g., turnip, Chinese cabbage, and pak choi), Brassica napus (e.g., rapeseed and leaf rape), Brassica juncea, and Brassica carinata (mustards). It includes 39 species, many of which are important agricultural and horticultural crops with different edible organs such as inflorescences (e.g., broccoli and cauliflower), leaves (e.g., kale and pak choi), heads (e.g., white and red cabbage), as well as roots and bulbs (e.g., radish and turnip). They serve as vegetables, oilseed crops, and forage species (Neugart et al., 2018; Poveda et al., 2020; Ayadi et al., 2022). The Brassica genus consists of three diploid species (Brassica rapa L. (e.g., turnip, Chinese cabbage, and pak choi), Brassica oleracea L. (e.g., broccoli, cabbage, cauliflower, kale, Brussels sprouts, and kohlrabi), and Brassica nigra L. and three amphidiploids such as Brassica napus L (e.g., rapeseed or canola and leaf rape), Brassica carinata (mustards), and Brassica juncea (L.) (mustards) (He et al., 2021). Rapeseed has remained an important crop in the production of edible and non-edible oils for thousands of years (Wang et al., 2022).
With this background information, in this study, we conducted comprehensive analyses of abiotic stress-induced secondary metabolite production in Brassica, namely, glucosinolates, carotenoids, flavonoids, phenolic acids, and alkaloids. This study could facilitate an understanding of their stress-specific and non-specific roles in metabolic adaptation to adverse growth conditions. Altogether, this study presents the starting point for an understanding of the stress response metabolic adaptation in Brassica, which expected to provide a potential metabolite or its biosynthesis candidate gene for improved stress management in Brassica crops. It also offers convenient and alternative approaches for managing short-term abiotic stress conditions through exogenous application of potential stress-mitigating metabolites in crop plants as shown previously (Hasanuzzaman et al., 2014; Linić et al., 2021; Hong et al., 2023). Additionally, it can be useful in harnessing the desired metabolic composition for the benefit of consumers through effective and controlled stress management strategies.
2 Glucosinolates
Glucosinolates (GSLs) are a class of sulfur- and nitrogen-containing secondary metabolites derived from amino acids, mainly found in Brassica crops, such as broccoli, cabbage, and oilseed rape (Tang et al., 2023b). The bioactive GSL metabolites confer benefits to plant defense, human health, and the unique flavor of some Brassica crops (Miao et al., 2021). They play multifunctional roles in plants, including stress response, growth, and development. Being specialized metabolites of Brassica, the diverse functions of GSLs and their degradation products under different physiological conditions have been fairly studied (Shaw et al., 2022). Studies have pointed out that GSL content and profiles can differently impact plant fitness under stressful conditions (Klein and Papenbrock, 2008; Muthusamy et al., 2021). On the basis of structure, GSLs can be classified into aliphatic, indolic and aromatic compounds, constituting a total of 200 types of GSLs with various substituents (Martínez-Ballesta et al., 2013). The role of GSLs in different biotic stress responses and their importance in offering stress tolerance have been well-documented until recently(Martínez-Ballesta et al., 2013; Liu et al., 2021; Abuyusuf et al., 2023). However, the responses of GSL metabolism to abiotic stress are relatively understudied.
In an attempt to identify the molecular basis of the low-temperature stress tolerance in Brassica oleracea var. acephala, it was revealed that chilling increase the total amount of aliphatic glucosinolates, while freezing increase the total amount of indolic glucosinolates, including glucobrassicin (Ljubej et al., 2021b). In another study, BrPP5 overexpression-mediated thermotolerance in Brassica rapa L. ssp. pekinensis demonstrated that stress tolerance in transgenic lines positively correlated with enhanced accumulation of total GSL content compared to that of wild-type lines (Muthusamy et al., 2021), possibly indicating that GSL content is crucial in determining high-temperature stress tolerance. Individual profiling showed that the concentration of glucobrassicin, 4-methoxyglucobrassicin, neoglucobrassicin and gluconasturtiin were elevated in thermotolerant lines. In case of short-term high-temperature stress (40°C for 8 h) on pakchoi seedlings increased both aliphatic and indolic glucosinolates, including 4-methoxy-glucobrassicin (Rao et al., 2021). In another study investigating the specialized volatiles responding to chronic and short-term heat stress, it was demonstrated that glucosinolate volatiles constitute a major part of emission blend in heat-stressed B. nigra plants, especially under chronic stress (Kask et al., 2016). These results suggest that GSL volatiles could constitute specialized volatile defense pathways against heat stress in Brassica crops. Similarly, drought stress could also increase the total GSL content in Brassica rapa L. ssp. pekinensis. Detailed analysis revealed that indolic GSLs were higher than those in controls (Shawon et al., 2020). Studies also revealed that drought-induced accumulation of glucosinolates in Brassica rapa L. ssp. pekinensis leaves directly or indirectly control stomatal closure to prevent water loss under drought stress conditions (Eom et al., 2018). This study showed that glucobrassicanapin and 4-methoxyglucobrassicin were abundantly found in drought-stressed plants compared to controls. In particular, there was approximately a six-fold increase in levels of gluconapin and 4-hydroxyglucobrassicin under drought conditions. In contrast, drought exposure had no impact on GSL production, with the exception of glucoraphanin in Pak choi (Brassica rapa subsp. chinensis) (Park et al., 2021). Furthermore, it was shown that prolonged drought exposure had a positive correlation with glucoraphanin accumulation in Pak choi plants. GSLs and ITCs (GSL derivatives) under drought stress promote stomatal closure by stimulating the production of reactive oxygen species (ROS) (Salehin et al., 2019). Drought stress in curly kale (Brassica oleracea L. convar. Acephala (DC) var. sabellica) increased the expression of genes associated with glucosinolates, especially aliphatic alkenyl glucosinolates and genes associated with glucosinolate breakdown (Podda et al., 2019). It was also shown that drought stress in Brassica oleracea L. crops influences both qualitative and quantitative changes in GSL content and suggests a possible link between water stress resistance and the accumulation of NGBS (Ben Ammar et al., 2023). They also revealed that the highest increase in the glucosinolate amount was detected in broccoli roots, followed by cauliflower leaves under water stress conditions. As of now, the impact of GSL accumulation on drought stress responses of those cultivars is not clear. The stress-induced secondary metabolite accumulation also depends on the developmental stages of plants. For instance, reduced UVB and UV exposure in Pak choi sprouts elevated the concentrations of glucosinolates and their hydrolysis products, while similar growth conditions in mature 15- and 30-leaf plants improved the concentrations of flavonoids, hydroxycinnamic acids, carotenoids, and chlorophylls (Heinze et al., 2018). Apart from that Pak choi plants under long-day growth conditions were shown to enhance GSL production, especially gluconapin and neoglucobrassicin indicating GSLs also could respond according to light quantities.
In the case of salinity stress (40-100 mM NaCl) in broccoli (B. oleracea var. italica), there was a reduction in the amount of individual and total aliphatic GSLs, as well as indolic GSLs, compared to controls (Sarikamiş and Çakir, 2017), possibly indicating GSLs break down upon exposure to stress. It is worth noting that GSL biosynthesis-related genes in B. rapa such as BrMYBs (BrMYB28.1, BrMYB28.2, BrMYB34.3, BrMYB51.2, BrMYB28.1 and BrMYB29.1), were found to be differentially expressed based on the duration of stress exposure, as well as to different treatments like cold, drought, salt and ABA (Seo et al., 2017). This indicates the existence of complex regulatory system for GSL production in stress responses. The time dependent expression trend for GSL biosynthesis-related genes was also observed in the cold-stressed leaves of B. juncea (Tang et al., 2023a). While the contribution of time-dependent GSL production in cold stress warrants further study, it is clear that exogenous application of GSLs during cold stress confers cold-stress tolerance in B. juncea (Tang et al., 2023a). Interestingly, GSLs and ITCs (GSL derivatives) are also attributable to tastes such as umami, sourness, acidic bitterness, irritant and saltiness, bitterness, astringency, and richness. In some cases, stress-induced metabolic changes can negatively impact the crop nutritional attributes of crops. For instance, a high glucosinolate (GSL) concentration in edible oil seeds, such as rapeseed, can result in severe diseases of the liver, thyroid, and kidney if consumed (Wang et al., 2022). However, studies show that control over GSL accumulation is possible through efficient N management and variety selection to the meet the consumer demands (Wang et al., 2022). Chemical structures of some of the flavonoids with potential roles in abiotic stress responses in Brassica crops has been shown in Figure 2. Additionally, the GSL profiles and their potential stress response in Brassica crops published elsewhere listed in Supplementary Table S1.
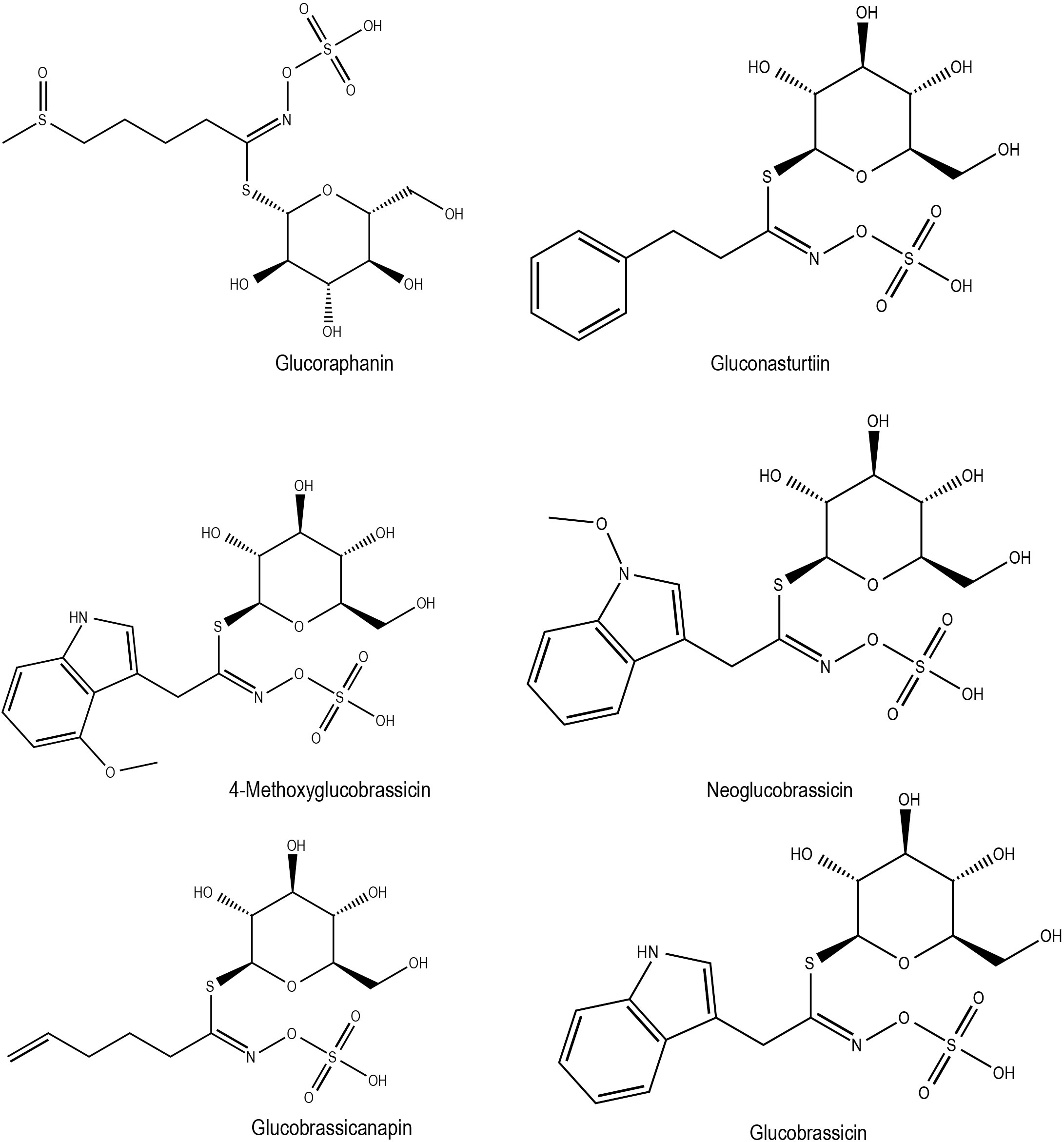
Figure 2 Chemical structures of glucosinolates with potential role in Brassica abiotic stress responses.
3 Phenolic compounds
Phenolic compounds are ubiquitously present in plants and are found in variety of biological processes including plant stress responses. By evolution, plants had accumulated an array of phenolic compounds to adapt in changing environments (Kumar et al., 2023) (Figure 1). Phenolic compounds can be classified into two major groups flavonoids (e.g. anthocyanidins, flavanones, isoflavones and others) and non-flavonoids (e.g. Phenolic acids, lignins/lignans, coumarins, quinones, tannins, stilbenes, and others) based on the number and arrangement of the carbon atoms in their chemical structures (Cartea et al., 2011). Among phenolic compounds, flavonoids, phenolic acids, lignins/lignans, coumarins, quinones, tannins, stilbenes, cutin, suberin were either directly or indirectly known to participate in plant stress responses(Šamec et al., 2021a). Flavonoids and phenolic acids are two phenolic compounds were widely studied for their role in stress mitigation and also for their beneficial role in promoting human health.
3.1 Flavonoids
The flavonoid biosynthetic pathway is one of the most intensively investigated pathways for production of agronomically robust, stress-tolerant plants and natural dietary antioxidants (Ahmed et al., 2014). With more than 8000 flavonoids are found in plants, mostly with C6-C3-C6 structural framework, including chalcones, flavans, flavones, flavonols, anthocyanins, proanthocyanidins, with stilbenes being the only exception with C6-C2-C6 framework, synthesized through the flavonoid biosynthetic pathway (reviewed) (Chen et al., 2023b). Like other plants, flavonoids of Brassica mostly act as antioxidants thus helping the plants to overcome the adverse effects of major abiotic stresses such as drought, salinity, low and high temperature etc. A study detailing the zinc supplementation based salt tolerance mechanism in mustard [Brassica juncea (L.) Czern & Coss] has identified increase in flavonoid content contribute to stress tolerance (Ahmad et al., 2017) (Supplementary Table S1). Similarly, a mild drought stress in canola cultivars increased the flavonol and total flavonoid content (Rezayian et al., 2018). However, anthocyanin content showed a significant decrease in both cultivars under similar conditions. A study comparing yellow-coated and black-coated rape seeds of turnip rape (Brassica rapa L.) showed that a higher flavonoid content in black-seeded rapes was positively correlated with tolerance to NaCl-induced salinity stress (Xuan et al., 2015). High salinity induced the expression of Arabidopsis dihydroflavonol-4-reductase (DFR) in B. napus, increases the antioxidant efficiencies, possibly through anthocyanin accumulation (Kim et al., 2017). A study to investigate the exogenous application of liquiritoside on Chinese flowering cabbage (Brassica rapa subsp. parachinensis) for its impact on oxidative stress showed that enhanced accumulation of flavonoids, along with other phytochemicals, is helpful in mitigating the adverse effects (Akram et al., 2021). Apart from the major abiotic stress constraints, a recent study dealing with specific sound waves in phytochemical content of broccoli (Brassica oleracea) showed that a specific sound waves can significantly improve the flavonoid content (Kim et al., 2020). Flavonoids and their derivatives (flavonols and anthocyanins), as well as hydroxycinnamic acids, are the most common chelators of metal ions in Brassica plants, with the help of functional hydroxyl groups, in plants exposed to biotic and abiotic stresses, in addition to their radical scavenging activities (Salami et al., 2023). Low temperatures (8°C) increase the phytochemical (phenolic acids 3%; flavonoids 5%; carotenoids 15%; glucosinolates 21%) content in Kale (Brassica oleracea var. acephala) (Ljubej et al., 2021a). In contrast, short freezing (at −8°C, for 1 h after previous acclimation at 8°C, for 23 h) significantly reduced flavonoid content by 21% in the same crop. In another study, it was revealed that the total concentration of flavonoids remained unchanged despite declining temperature and global radiation in most of the kale cultivars under study. However, quercetin concentration increased while kaempferol decreased (Schmidt et al., 2010). Interestingly, the quercetin-to-kaempferol ratio was shown to increase in all of the investigated cultivars in response to decreasing temperature. Low temperature in Brassica oleracea var. sabellica enhance specific flavonol glycosides and hydroxycinnamic acid derivatives (Neugart et al., 2013; Rezayian and Zarinkamar, 2023). Similarly, exposure to cold and freezing temperatures in Brassica napus L. var. oleifera L. cv. Jantar significantly increases anthocyanin content (Solecka et al., 1999).
In rapeseed, drought stress treatments with different moisture regimes increased phenolic compounds and flavonoids (Salami et al., 2023). Among other environmental factors, light and cold stress significantly enhanced anthocyanin accumulation (Loreti et al., 2008). Supplementation of zinc and calcium in salt stressed Brassica juncea was shown to mitigate salt stress adverse effects by enhancing antioxidant properties through several routes, including flavonoid accumulation (an increase of 86.19% over the control) (Ahmad et al., 2018). Selenium (Se) in plants has been shown to counteract major abiotic stresses such as cold, drought, water, salinity, and heavy metals by modulating the antioxidant system and various other unidentified pathways (Feng et al., 2013). Later, it was found that selenium induces flavonoid content to improve antioxidant capacities in turnip (Brassica rapa ssp. rapa) (Thiruvengadam and Chung, 2015). In general, abiotic stress conditions can indirectly regulate genes associated with flavonoid biosynthesis by modulating ABA homeostasis (Gai et al., 2020). It has been demonstrated that elevated ABA levels induce the production of flavones, anthocyanins, flavonols, isoflavones (e.g. kaempferitrin, sakuranetin, kaempferol) under drought conditions, which are beneficial in mitigating stress-induced damages (Loreti et al., 2008). It has been observed that an improved flavonoid content is beneficial in waterlogging stress conditions, as recently demonstrated in rape seed plants (Hong et al., 2023). This study also highlights the significant induction of flavonoids such as naringenin and epiafzelechin. Ahmed et al. (Ahmed et al., 2014) identified the association of dihydroflavonol 4-reductase (DFR)genes with anthocyanins and suggested that these genes could play a role in cold and freezing stress tolerance of Brassica rapa. Chemical structures of some of the flavonoids with potential roles in abiotic stress responses in Brassica crops has been shown in Figure 3. Studies have also shown that stress factor, Ultraviolet-B (UV-B) light, can induce the accumulation of flavonoids in Pakchoi (Brassica rapa L.) under specific light quantities (Hao et al., 2022). Almost all of the individual flavonoids identified in response to UV-B were relatively enhanced in seedlings treated with 4 µmol·m−2·s−1 UV-B for 4 h (Hao et al., 2022). Two flavonoids, kaempferol and quercetin appeared during anther development in Brassica napus has been shown to protect the haploidic pollen from UV light damage and water losses (Hsieh and Huang, 2007). The flavonoids found in Brassica crops can be useful in preventing cardiovascular diseases and some types of cancer, mainly by inhibiting LDL and DNA oxidation through scavenging peroxyl and hydroxyl radicals (Cao et al., 1997). The most important flavonoids in the human diet are quercetin and kaempferol, which are present as complex glycosides in Brassica species (Neugart and Bumke-Vogt, 2021).
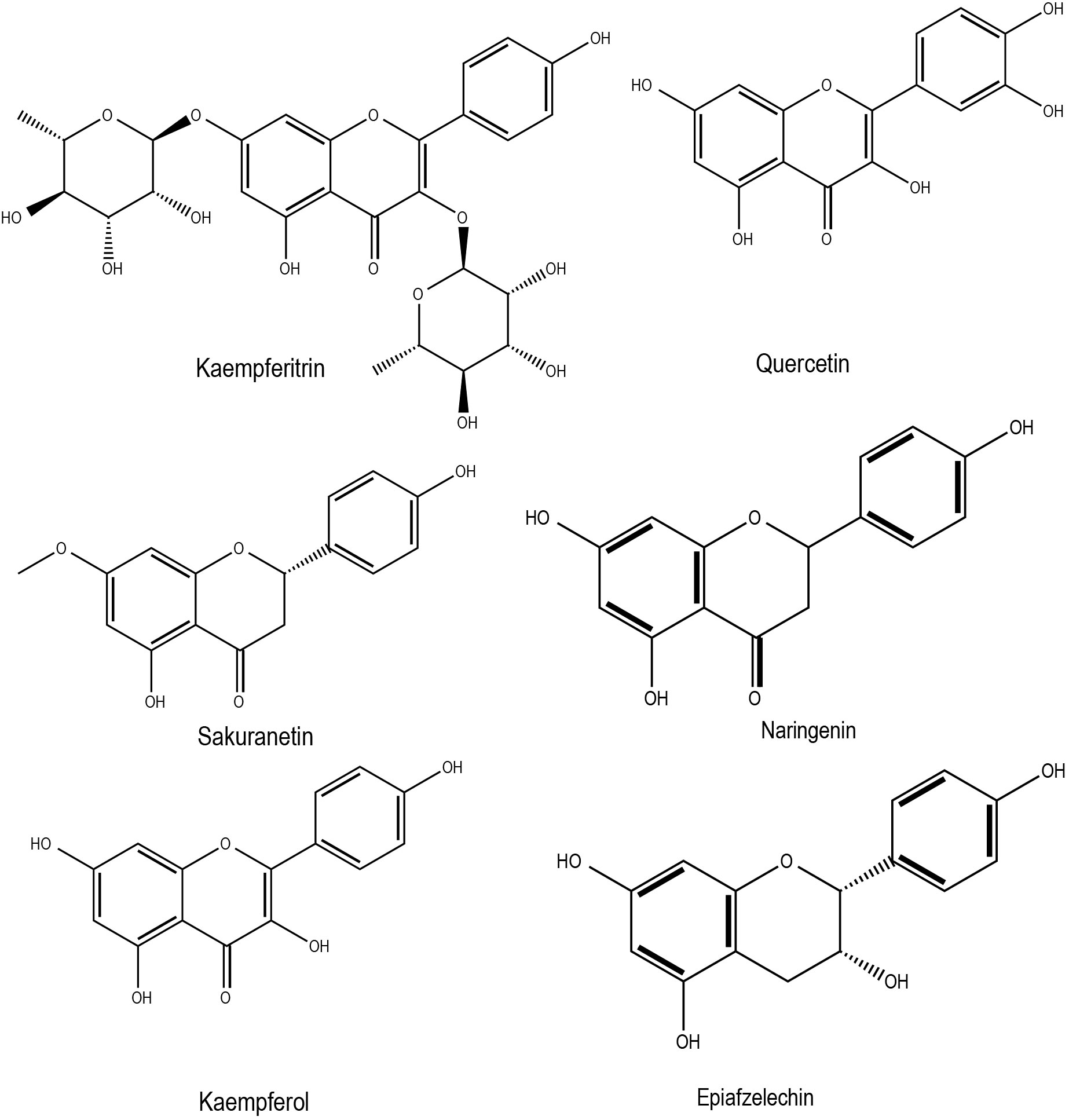
Figure 3 Chemical structures of flavonoids with potential role in Brassica abiotic stress responses.
3.2 Phenolic acids
Among the phenolic acids in Brassica vegetables, caffeic acid, p-coumaric acid, and ferulic acid have been reported in cabbages, while sinapic acid derivative were mainly found in Chinese cabbage and broccoli apart from cabbage (Park et al., 2014). The phenolic acid profiles of salt-tolerant Brassicas, such as kale and white cabbage, contained significantly higher level of total phenolic acids, including total hydroxycinnamic acids, when compared to salt-sensitive Chinese cabbage (Linić et al., 2019) (Supplementary Table S1). In contrast, hydroxybenzoic acids tended to decrease under the applied salinity conditions. Additionally, cell wall-bound phenolic acids, particularly sinapic acid (SiA), were found to be relatively higher in tolerant cultivars. In the case of salt-sensitive cultivars, a notable decrease was reported for protocatechuic acid (PA), 4-coumaric acid (pCoA), salicylic acid (SA), and caffeic acid (CaA) under salinity stress conditions. In fact, the offspring derived from salt-treated parental lines showed significantly higher phenolic acid contents and increased antioxidant capacities (Benincasa et al., 2021). In an attempt to use natural metabolites exogenously to ameliorate the adverse effects of high salinity stress in Chinese cabbage, foliar application of salicylic acid (SA) and ferulic acid (FA) (10–100 μM) had attenuating effects on salt-stressed plants (Linić et al., 2021). Further, it elevated the levels of phenolic acids, including ferulic acid and sinapic acid, endogenously along with flavonoids (KAE, and QUE). However, the endogenous SA levels were reduced in phenolic acid-treated plants growing under salt stress conditions. Another study demonstrated that the application of SA could ameliorate mild salinity stress effects in Indian mustard (Brassica juncea (L.) Czern. et Coss.) plants (Yusuf et al., 2008). In B. napus, SA application conferred salt stress tolerance by enhancing the activities of antioxidant defense and glyoxalase enzymes (Hasanuzzaman et al., 2014). The supplementation of SA (0.5 mM) in conjunction with 2.0 mM SO42− significantly improved maximum chlorophyll content, PS II efficiency, and gas exchange parameters compared to individual applications of 0.5 mM SA or 2.0 mM SO42− in salt- stressed mustard (Brassica juncea L.) (Rasheed et al., 2022). The study also explained the potential for SA to inhibit stress ethylene, while in combination with S, it may promote S-assimilation, thereby enhancing optimal ethylene formation and ethylene sensitivity to ultimately reverse salt stress. Moreover, seed priming with SA was shown to enhance the germination and growth responses of B. napus under drought and salinity stresses (Raees et al., 2022). Similarly, SA application detoxified the stress effects of NiCl2 and NaCl in B. juncea, improving growth, leaf water potential, pigment levels, and photosynthetic attributes (Yusuf et al., 2012). A recent study aiming to investigate the role of phenolic acids in salt tolerance of Chinese cabbage (Brassica rapa ssp. pekinensis), white cabbage (Brassica oleracea var. capitata), and kale (Brassica oleracea var. acephala) found that higher levels of hydroxycinnamic acids were positively correlated with salt tolerance in kale and white cabbage (Linić et al., 2019). In the case of salt-sensitive Chinese cabbage, the levels of caffeic, salicylic, and 4-coumaric acids were reduced.
The investigation of phenolic acids in drought-stressed Chinese cabbage (Brassica rapa) revealed that concentration of individual phenolic acids changes depending on the duration of the stress. After one week of drought stress, ferulic acid content was reduced compared to that of controls, while the amount of total phenolic acids increased over the controls (Shawon et al., 2020). The significance of salicylic acid (SA), also known as 2-hydroxybenzoic acid, as a signal molecule in inducing the plant defense responses, including local and systemic acquired resistance (SAR), is well-established in model plants (Simoh et al., 2010). In B. napus, pretreatment with salicylic acid alleviated drought-induced stress symptoms (La et al., 2019a). Furthermore, SA-mediated modulation coincided with the antagonistic downregulation of ABA-signaling genes. Additionally, SA pretreatment proved valuable in radical scavenging and enhanced proline synthesis, which likely helps plant maintains redox homeostasis. Similarly, Chinese cabbage pretreated with SA exhibited superior growth parameters and increased tolerance to drought stress conditions compared to the control group (La et al., 2019b). The exogenous application of nitric oxide (NO) in salt-stressed canola (Brassica napus) plants enhanced phenolic acids such as gallic acid and coumaric acid, along with other non-enzymatic antioxidants like catechin, diadzein, and kaempferol, in conjunction with antioxidant enzymes, thus promoting salt stress tolerance (Rezayian and Zarinkamar, 2023).
The levels of soluble p-coumaric, sinapic and ferulic acids increased approximately 3-, 4- and 5-fold, respectively, in winter oilseed rape leaves (Brassica napus L. var. oleifera L. cv. Jantar) exposed to cold and then to freezing treatments (Solecka et al., 1999). However, caffeic acid did not change during cold treatment. Cold-stressed heading-type kimchi cabbage (B. rapa L. ssp. pekinensis) accumulated phenolic acids, including p-coumaric, ferulic, and sinapic acids, which subsequently improved free-radical scavenging activity and antioxidant activity (Eom et al., 2022). Additionally, there were reports of phenolic acids improving the disease resistance in Brassica crops. For instance, pretreatment of Brassica napus plants with p-coumaric acid conferred resistance to black rot disease by inducing jasmonic acid-mediated phenolic accumulation (Islam et al., 2019). Exposure to cold and freezing temperatures in Brassica napus L. var. oleifera L. cv. Jantar significantly increased the soluble phenolic acid (e.g. p-coumaric, sinapic and ferulic acids) contents (Solecka et al., 1999). Conversely, high temperatures in winter crop Brassica oleracea var. sabellica favored the production of hydroxycinnamic acid derivatives such as disinapoyl-gentiobiose, while lower temperatures promoted the biosynthesis of sinapic acid-acylated flavonol tetraglycosides, such as kaempferol-3-O-sinapoyl-sophoroside-7-O-diglucoside (Neugart et al., 2016). Taken together, it is clear that phenolic acids contribute to abiotic stress tolerance in Brassica plant by enhancing the antioxidant efficiencies. Chemical structures of some of the phenolic acids with potential roles in abiotic stress responses in Brassica crops has been shown in Figure 4.
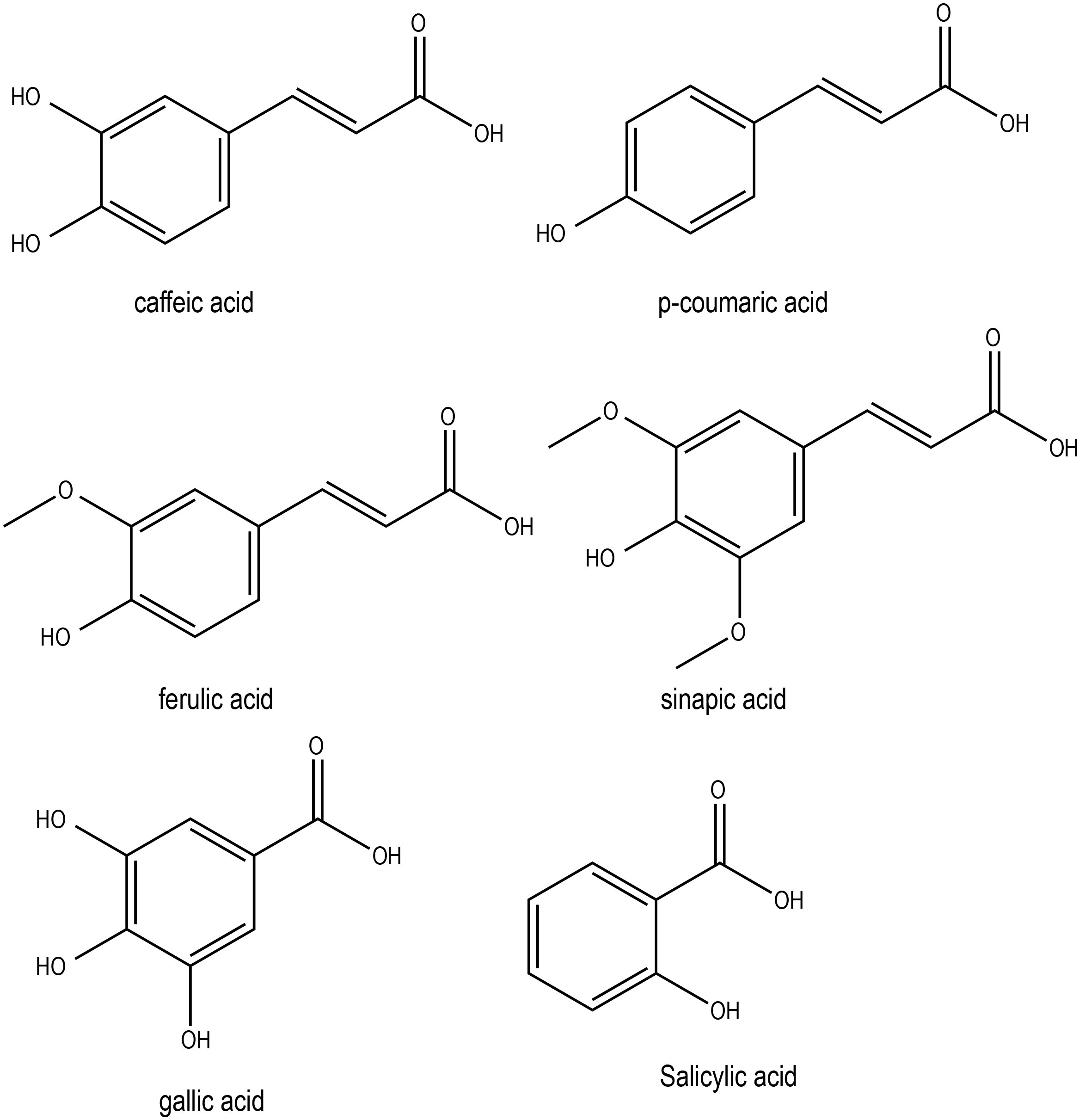
Figure 4 Chemical structures of phenolic acids with potential role in Brassica abiotic stress responses.
3.3 Other phenolic compounds
Among the various phenolic compounds found in Brassica crops, the lignin content in relation to stress responses has been fairly studied. In fact, a decade ago, it was proven that water-deficit stress in B. napus accumulates lignin content in leaves (Lee et al., 2014). In 2013, a study stated the possible molecular association between the enhanced expression of dirigent family genes and elevated soluble lignin content, contributing to tolerance against water stress in Brassica rapa crops (Thamil Arasan et al., 2013). A recent study detailing triacontanol-mediated drought tolerance in Brassica juncea L. found that elevated lignin levels or lignification processes helps plants reduce the negative consequences of drought stress (Ahmad et al., 2021). Other studies have demonstrated a positive correlation between enhanced lignin and disease resistance in B. napus (Wang et al., 2023). On the contrary, moderate salinity stress in B. napus has been shown to reduce the expression of genes associated with lignin biosynthesis pathways. Although, it is not clear whether this expression pattern is truly reflected in lignin content, it was hypothesized that it is an attempt by plants to preferentially allocate the energy for primary metabolism, eventually enhanced biomass production (Chen et al., 2023a). It is important to note that salinity stress (i.e. 150 mmol L−1 sodium chloride) in hydroponically grown canola, Brassica napus L., plants resulted in elevated levels of lignin (Hashemi et al., 2010). This study also has shown that silicon supplementation reduced lignin content under stress, improving the stress tolerance, possibly indicating the negative role of lignin accumulation in salinity tolerance. Lignin also plays a role in cadmium (Cd) detoxification processes in Brassica chinensis L (Wang et al., 2020b). The study concluded that Cd compartmentalization in the secondary cell wall by coupling with lignin side chain region, is responsible for Cd detoxification related to cultivar-dependent Cd accumulation. While it is known that lignin deposition could increase the mechanical strength of plant cells under stress, the mode of action in plant abiotic stresses mitigation mechanisms is not clear. The biological significance of stilbenes, tanins, cutins and other phenolic compounds were relatively understudied or unavailable in Brassica crops. However, preliminary studies are emerging to dissect their role in abiotic stress responses of Brassica crops. For instance, a recent study has shown that severe drought stress in Brassica napus L. significantly accumulates cutins, which might help the plants to avoid transpirational water loss during stress conditions (Fang et al., 2022).
4 Carotenoids
Carotenoids are a group of isoprenoid metabolites that play critical roles as light harvesting pigments and structural components of photosystems in plants. In general carotenoids can be divided into two classes: unsaturated C40 hydrocarbons as carotenes and their oxygenated derivatives known as xanthophylls (Ortega-Hernández et al., 2021). Carotenoids and its derivatives are crucial for the biosynthesis of phytohormones such as abscisic acid (ABA) and strigolactones (SLs), thus acting as signaling molecules in response to environmental and developmental cues (Sun et al., 2022). Carotenoid oxygenase is a key enzyme involved in the metabolism of carotenoids, showing differential expression pattern in response to abiotic stress conditions like salt, drought, and cold in both B. rapa and B. oleracea plants (Kim et al., 2016). Carotenoids are important, as their oxidized derivatives produced by specific carotenoid cleavage dioxygenases serve as photosynthetic accessory pigments, colorants, apocarotenoids, and are crucial for plant growth and development (Kim et al., 2016). A study by Kumar et al. (Kumar et al., 2013) identified carotenoid content as one of the simple indices for screening and identifying temperature stress-tolerant genotypes of Brassica juncea. Another study dealing with Kale (B. oleracea) under cold treatments found that individual carotenoid content varied at 15°C/10°C (Hwang et al., 2017). They found that the lutein, α-carotene, and β-carotene contents were very low, while the zeaxanthin contents was very high, and the ratio of individual to the total carotenoid content of kale was recorded as 4553% for carotene and 210% for zeaxanthin. Very recently, a study in Chinese cabbage (Brassica rapa subsp. Pekinensis) has revealed that a potential heat stress-tolerant candidate gene identified as BraA09g011460.3C (HD-zip I subfamily) has a significantly lower expression level in high carotenoid cultivars, possibly indicating a negative correlation with carotenoid content regulation in Chinese cabbage plants (Yin et al., 2023). At low temperature (8°C) for the short term, carotenoid content was induced by 15%, while other phytochemicals like phenolic acids, flavonoids, and glucosinolates contents were higher by 3%, 5%, and 21%, respectively, in kale (Brassica oleracea var. acephala) exposed to low temperature over the control plants (Ljubej et al., 2021a) (Supplementary Table S1). However, carotenoid content along with chlorophylls was reduced in most cases (Ben Ammar et al., 2022), which is aligned with previous findings. In fact, carotenoids and chlorophylls are functionally related and share common components in their biosynthesis.
Under drought conditions, the neoxanthin and antheraxanthin contents were significantly decreased in kale (Brassica oleracea L. var. acephala) (Barickman et al., 2020). Similarly, a significant reduction in total carotenoid content was reported for drought-stressed kale (Brassica oleracea var. acephala) leaves (Lee et al., 2017). The ratio of individual carotenoids to the total carotenoid content of kale leaves was reported as 51.4% for lutein, 4.44% for zeaxanthin, 2.76% for α-carotene, and 41.4% for β-carotene. UVB-treated (5 and 10 kJ m−2 d−1) canola (Brassica napus L.) seedlings were found to accumulate carotenoids and flavonoids (Sangtarash et al., 2009) compared to that of controls (0 kJ m−2 d−1). Whereas water stress reduced carotenoid content. Interestingly, water stress combined with enhanced UVB exposure also reduced carotenoid levels. Similarly, short-term waterlogging stress in kale (Brassica oleracea L. var. acephala) plants exhibited a decrease in carotenoid (CAR) concentrations of β-carotene, lutein, zeaxanthin, neoxanthin, and chlorophyll A (ChlA) and chlorophyll B (ChlB) concentrations (Brazel et al., 2021). In contrast, total glucosinolates content was found to be elevated. Nevertheless, the application of plant growth- promoting rhizobacteria (Pseudomonas sp. and Staphylococcus sp) and Biochar (Morus alba L. wood) in Brassica napus L. under drought conditions has positive growth impacts, found to be increased with carotenoid content along with other photosynthetic pigments (Lalay et al., 2022). In another study, SA pretreated Chinese cabbage plants retained carotenoid content during drought stress, which is similar to that of controls, while the non-treated drought-stressed plants showed a significant reduction (21.8%) in carotenoid content (La et al., 2019b). Application of 5-aminolevulinic acid (ALA) in rapeseed (Brassica napus L.) leaves under optimal growth conditions significantly increased carotenoid content (Liu et al., 2016). However, under drought stress conditions, similar treatment did not avert the drought impacts or failed to maintain carotenoid levels. This is despite the fact that carotenoids are involved in the stabilization and protection of the lipid phase of the thylakoid membrane and are also useful in scavenging radical species. Brassica rapa ssp. rapa seedlings exposed to environmentally hazardous copper oxide nanoparticles (CuO NPs) reduced the carotenoid, chlorophyll, and sugar content while increasing anthocyanins, glucosinolates and phenolic compounds. Reactive oxygen species (ROS), malondialdehyde (MDA), and hydrogen peroxide (H2O2) production were also enhanced in CuO NPs-treated seedlings (Chung et al., 2019). In another study, the Chinese Kale (Brassica oleracea var. alboglabra) sprouts under salinity stress conditions (160 mM Nacl solution) enhanced carotenoid content by 53% over the controls (Wang et al., 2020a). However, the carotenoid content does not positively correlate with the antioxidant capacities of the plants under study. On the other hand, salinity stress (50–200 mM NaCl) in Brassica leafy vegetables like Chinese cabbage, white cabbage, and kale did not significantly influence the carotenoid content (Šamec et al., 2021b). Among other factors, sulphur supplementation in kale sprouts does not cause any significant changes in carotenoid content (Kopsell et al., 2007). Similarly, the exogenous application of MeJA on kale plants does not exert any impact on carotenoid content (Sun et al., 2012). It is not clear whether enhanced carotenoid content can contribute stress tolerance. From the literature it is evident that abiotic stress conditions such as drought, waterlogging, temperature, and heavy metal stresses were reduced the total carotenoid content in Brassica crops while UVB exposure found to enhance the carotenoid content. To understand the biological significance further study detailing the functions of individual carotenoids in relation to stress responses are essential. Chemical structures of some of the carotenoids with potential roles in abiotic stress responses in Brassica crops has been shown in Figure 5.
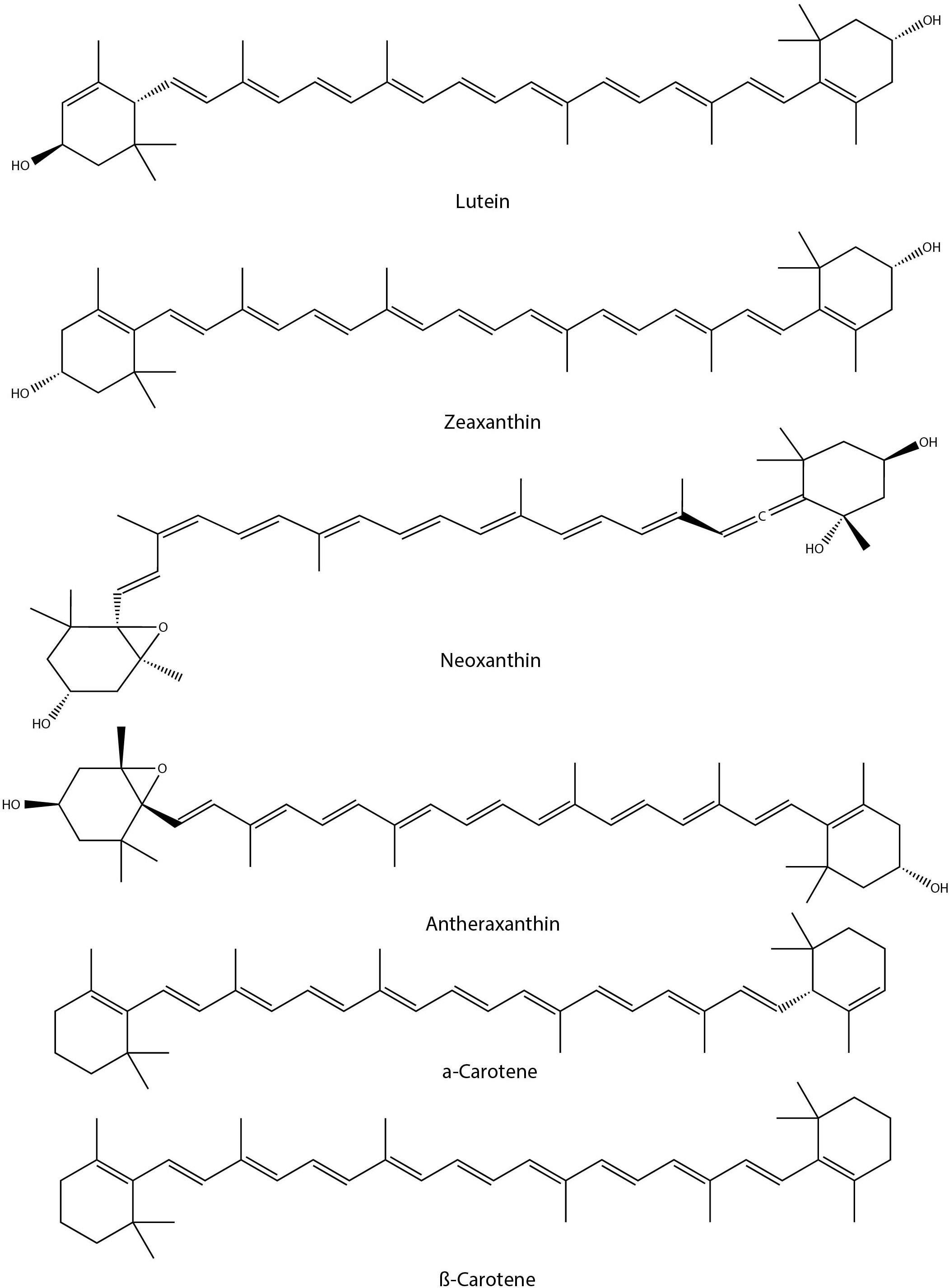
Figure 5 Chemical structures of carotenoids with potential role in Brassica abiotic stress responses.
5 Alkaloids
Alkaloids are among the most abundant plant secondary metabolites, constituting approximately 20% of all plant-based secondary metabolites. They are predominantly solid compounds and possess antioxidant potential, which can be induced in response to both abiotic and biotic stress conditions (Huang et al., 2020; Tan et al., 2021). Alkaloids can be categorized into subgroups based on the basic heterocyclic nucleus in their structure which includes pyridine, tropane, isoquinoline, phenanthrene, phenylethylamine, indole, purine, imidazole and terpenoids (Pereira et al., 2023). Despite their biological significance in abiotic stress responses in other plants, alkaloids were relatively understudied in Brassica crops possibly due to their low availability in brassica vegetables. A study identified Brassica napus gene identified as Cab026133.1, encoding a tropinone reductase (BnTR1) was found to positively affect alkaloid metabolism, primarily producing atropine, ultimately enhancing low-temperature tolerance (LT) in BnTR1 overexpressing Arabidopsis plants (Huang et al., 2020) (Supplementary Table S1). Ectopically expressing BnTR1 in Arabidopsis plants significantly increased alkaloid content. In fact, the addition of 10 nmol of atropine per plant significantly rescued the susceptibility of control plants, thus confirming the role of alkaloids in LT tolerance. B. juncea, known for its excellent cadmium (Cd) accumulation and high phytoremediation potential, as well as its high biomass production in Cd-contaminated soil, has been shown to significantly accumulate alkaloids to counter the stress effects (Tan et al., 2021). An integrated transcriptomic and metabolomic analysis aimed at characterizing alkali stress responses in canola (Brassica napus L.) revealed that alkali stress in canola roots for 72 h differentially regulate isoquinoline alkaloid biosynthesis, among many other metabolic pathways (Wang et al., 2021). Similarly, an attempt to dissect the molecular basis of Cd stress response mechanisms in the hairy roots of Brassica campestris L. demonstrated that genes related isoquinoline alkaloid biosynthesis-related pathway were highly enriched under stress (Sun et al., 2023). Chemical structures of some of the alkaloids with potential roles in abiotic stress responses in Brassica crops has been shown in Figure 6.
6 Conclusions and future directions
Major abiotic stresses differentially regulate the content of secondary metabolites, such as glucosinolates, carotenoids, flavonoids, phenolic acids, and alkaloids in Brassica crops. In general, changes in the content of these metabolites have implications for the plant’s stress responses. In some cases, they act as stress mitigators by functioning as non-enzymatic antioxidants. Therefore, their elevated levels are expected to help the plants avert the negative consequences of adverse stress conditions (Figure 7). Nevertheless, specific compounds within the classes have shown differential changes in content under similar environmental conditions. This necessitates further study of individual compounds to decipher their biological significance in plant metabolic adaptation under adverse growth conditions. While stress-induced metabolites serve multiple functions in plants, they are mostly known for their role in countering oxidative stress resulting from the excessive accumulation of reactive oxygen species during stress. It is important to note that plants possess vast arrays of defense chemicals and are responsive to environmental conditions. Any variation in the content of these metabolites under stress may reveal stress-responsive traits. However, in most model and non-model plants, the study of plant stress metabolic adaptation has been limited to few classes of secondary metabolites, such as glucosinolates, flavonoids, carotenoids, alkaloids and phenolic acids. This indicates that a larger fraction of secondary metabolites and their biological significance in plant stress response is yet to be identified. Furthermore, several known and unknown factors found to be synchronized with most of the abiotic stress conditions, altering the secondary metabolite production. This adds to the challenges in precisely deciphering the particular metabolite production pathway in stress adaptation. Detailed study of the genetic basis of secondary metabolite production pathway is needed to generate the resources that can facilitate metabolic engineering to achieve stress-resilient crop production. However, most of the key metabolite production pathways are still emerging or limited in their harnessing of nutraceutical importance for human and animal diets, rather than addressing stress mitigation mechanisms. The application of next-generation metabolomics using high-throughput MS techniques, coupled with robust bioinformatics data analysis platform, is expected to generate precise and accurate metabolite datasets for specific stress conditions. It is interesting to note that identification of effective stress-mitigating metabolites or their synthetic decoys can accelerate crop stress management programs through exogenous applications. This study clearly shows that the identification of stress-response metabolites will enhance our understanding of complex networks and interplay of associated systems in plants during abiotic stresses, which is more useful for comprehending plant stress adaptability.
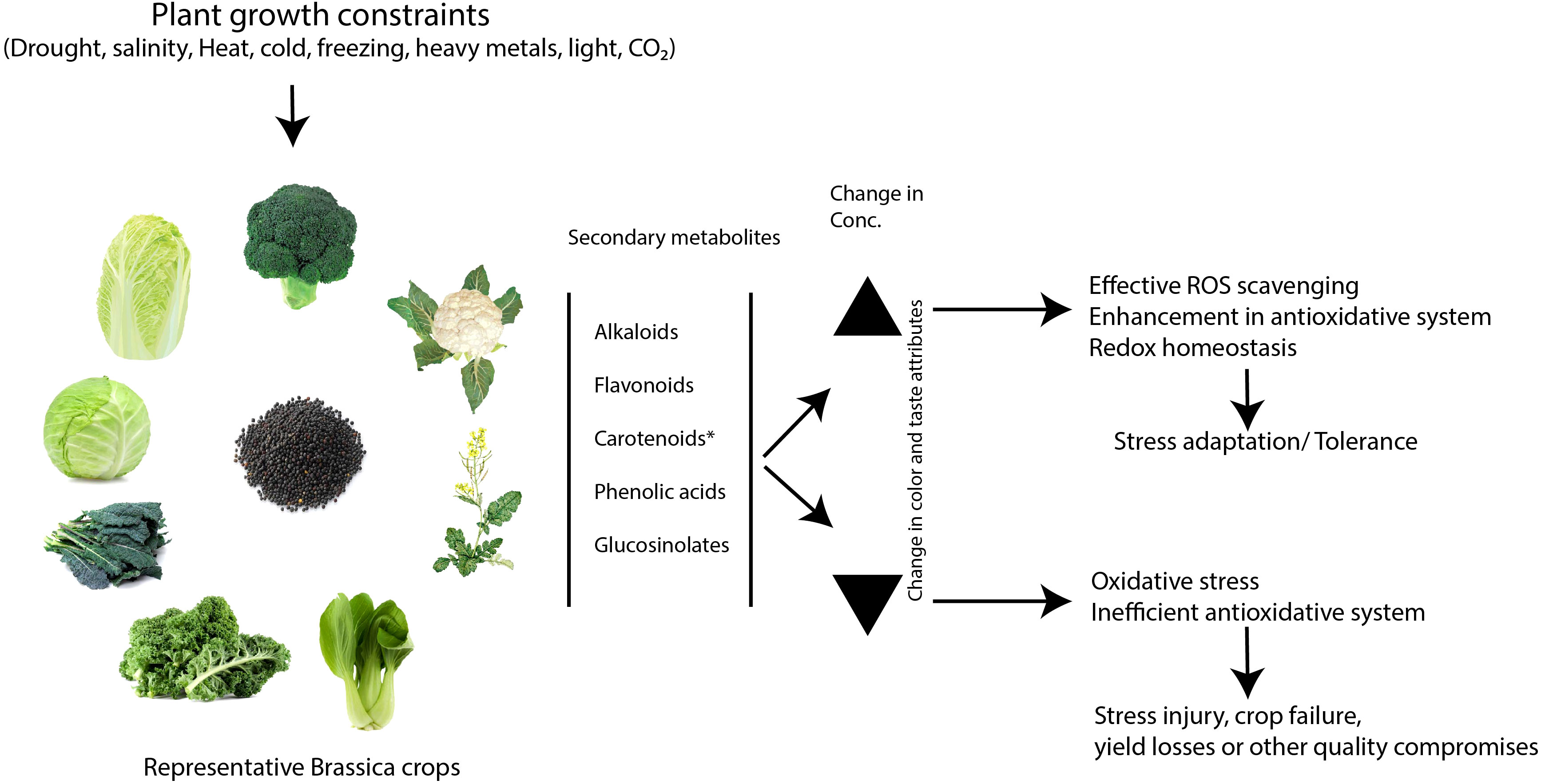
Figure 7 Metabolic adjustment of secondary metabolite biosynthesis and its implications in plant stress responses. The figure represents the hypothetical model illustrating the potential roles of secondary metabolites, such as glucosinolates, flavonoids, carotenoids, phenolic acids, and alkaloids, during stress-adaptive metabolic changes in Brassica crops, based on the literature. In figure, * represents the lack of evidence supporting the positive correlation between carotenoid content and stress tolerance in Brassica against major abiotic stresses.
Author contributions
MM: Conceptualization, Data curation, Formal analysis, Writing – original draft, Writing – review & editing. SL: Conceptualization, Data curation, Formal analysis, Funding acquisition, Project administration, Resources, Writing – review & editing.
Funding
The author(s) declare financial support was received for the research, authorship, and/or publication of this article. This work was supported by the Rural Development Administration (Korea) through the Rural Program for Agricultural Science and Technology Development (grant number PJ01570603).
Conflict of interest
The authors declare that the research was conducted in the absence of any commercial or financial relationships that could be construed as a potential conflict of interest.
Publisher’s note
All claims expressed in this article are solely those of the authors and do not necessarily represent those of their affiliated organizations, or those of the publisher, the editors and the reviewers. Any product that may be evaluated in this article, or claim that may be made by its manufacturer, is not guaranteed or endorsed by the publisher.
Supplementary material
The Supplementary Material for this article can be found online at: https://www.frontiersin.org/articles/10.3389/fpls.2023.1323085/full#supplementary-material
References
Abuyusuf, M., Rubel, M. H., Kim, H. T., Jung, H. J., Nou, I. S., Park, J. I. (2023). Glucosinolates and biotic stress tolerance in Brassicaceae with emphasis on cabbage: A review. Biochem. Genet. 61, 451–470. doi: 10.1007/s10528-022-10269-6
Ahmad, P., Ahanger, M. A., AlYemeni, M. N., Wijaya, L., Egamberdieva, D., Bhardwaj, R., et al. (2017). Zinc application mitigates the adverse effects of nacl stress on mustard [brassica juncea (L.) czern & coss] through modulating compatible organic solutes, antioxidant enzymes, and flavonoid content. J. Plant Interact. 12, 429–437. doi: 10.1080/17429145.2017.1385867
Ahmad, J., Ali, A. A., Al-Huqail, A. A., Qureshi, M. I. (2021). Triacontanol attenuates drought-induced oxidative stress in Brassica juncea L. by regulating lignification genes, calcium metabolism and the antioxidant system. Plant Physiol. Biochem. 166, 985–998. doi: 10.1016/j.plaphy.2021.07.009
Ahmad, P., AlYemeni, M. N., Ahanger, M. A., Wijaya, L., Alam, P., Kumar, A., et al. (2018). Upregulation of antioxidant and glyoxalase systems mitigates nacl stress in brassica juncea by supplementation of zinc and calcium. J. Plant Interact. 13, 151–162. doi: 10.1080/17429145.2018.1441452
Ahmed, N. U., Park, J. I., Jung, H. J., Yang, T. J., Hur, Y., Nou, I. S. (2014). Characterization of dihydroflavonol 4-reductase (DFR) genes and their association with cold and freezing stress in Brassica rapa. Gene 550, 46–55. doi: 10.1016/j.gene.2014.08.013
Akram, W., Khan, W. U., Shah, A. A., Yasin, N. A., Li, G. (2021). Liquiritoside Alleviated Pb Induced Stress in Brassica rapa subsp. Parachinensis: Modulations in Glucosinolate Content and Some Physiochemical Attributes. Front. Plant Sci. 12. doi: 10.3389/fpls.2021.722498
Ayadi, J., Debouba, M., Rahmani, R., Bouajila, J. (2022). Brassica genus seeds: A review on phytochemical screening and pharmacological properties. Molecules 27, 6008. doi: 10.3390/molecules27186008
Barickman, T. C., Ku, K. M., Sams, C. E. (2020). Differing precision irrigation thresholds for kale (Brassica oleracea L. var. acephala) induces changes in physiological performance, metabolites, and yield. Environ. Exp. Bot. 180, 104253. doi: 10.1016/j.envexpbot.2020.104253
Ben Ammar, H., Arena, D., Treccarichi, S., Di Bella, M. C., Marghali, S., Ficcadenti, N., et al. (2023). The effect of water stress on the glucosinolate content and profile: A comparative study on roots and leaves of Brassica oleracea L. Crops. Agronomy 13, 579. doi: 10.3390/agronomy13020579
Ben Ammar, H., Picchi, V., Arena, D., Treccarichi, S., Bianchi, G., Lo Scalzo, R., et al. (2022). Variation of bio-morphometric traits and antioxidant compounds of Brassica oleracea L. Accessions in relation to drought stress. Agronomy 12, 2016. doi: 10.3390/agronomy12092016
Benincasa, P., Bravi, E., Marconi, O., Lutts, S., Tosti, G., Falcinelli, B. (2021). Transgenerational effects of salt stress imposed to rapeseed (Brassica napus var. oleifera del.) plants involve greater phenolic content and antioxidant activity in the edible sprouts obtained from offspring seeds. Plants 10, 932. doi: 10.3390/plants10050932
Björkman, M., Klingen, I., Birch, A. N. E., Bones, A. M., Bruce, T. J. A., Johansen, T. J., et al. (2011). Phytochemicals of Brassicaceae in plant protection and human health - Influences of climate, environment and agronomic practice. Phytochemistry 72, 538–556. doi: 10.1016/j.phytochem.2011.01.014
Brazel, S. R., Barickman, T. C., Sams, C. E. (2021). Short-term waterlogging of kale ( Brassica oleracea L. var. acephala ) plants causes a decrease in carotenoids and chlorophylls while increasing nutritionally important glucosinolates. Acta Hortic. 1329, 175–180. doi: 10.17660/ActaHortic.2021.1329.21
Cao, G., Sofic, E., Prior, R. L. (1997). Antioxidant and prooxidant behavior of flavonoids: Structure-activity relationships. Free Radic. Biol. Med. 22, 749–760. doi: 10.1016/S0891-5849(96)00351-6
Cartea, M. E., Francisco, M., Soengas, P., Velasco, P. (2011). Phenolic compounds in Brassica vegetables. Molecules 16, 251–280. doi: 10.3390/molecules16010251
Chan, K. X., Wirtz, M., Phua, S. Y., Estavillo, G. M., Pogson, B. J. (2013). Balancing metabolites in drought: The sulfur assimilation conundrum. Trends Plant Sci. 18, 18–29. doi: 10.1016/j.tplants.2012.07.005
Chen, B., Bian, X., Tu, M., Yu, T., Jiang, L., Lu, Y., et al. (2023a). Moderate salinity stress increases the seedling biomass in oilseed rape (Brassica napus L.). Plants 12, 1–18. doi: 10.3390/plants12081650
Chen, Y. Y., Lu, H. Q., Jiang, K. X., Wang, Y. R., Wang, Y. P., Jiang, J. J. (2023b). The flavonoid biosynthesis and regulation in Brassica napus: A review. Int. J. Mol. Sci. 24, 357. doi: 10.3390/ijms24010357
Chung, I. M., Rekha, K., Venkidasamy, B., Thiruvengadam, M. (2019). Effect of Copper Oxide Nanoparticles on the Physiology, Bioactive Molecules, and Transcriptional Changes in Brassica rapa ssp. rapa Seedlings. Water. Air. Soil Pollut. 230, 1–14. doi: 10.1007/s11270-019-4084-2
Doghri, M., Rodríguez, V. M., Kliebenstein, D. J., Francisco, M. (2022). Plant responses underlying timely specialized metabolites induction of Brassica crops. Front. Plant Sci. 12. doi: 10.3389/fpls.2021.807710
Eom, S. H., Ahn, M. A., Kim, E., Lee, H. J., Lee, J. H., Wi, S. H., et al. (2022). Plant Response to Cold Stress: Cold Stress Changes Antioxidant Metabolism in Heading Type Kimchi Cabbage (Brassica rapa L. ssp. Pekinensis). Antioxidants 11, 700. doi: 10.3390/antiox11040700
Eom, S. H., Baek, S. A., Kim, J. K., Hyun, T. K. (2018). Transcriptome analysis in chinese cabbage (brassica rapa ssp. Pekinensis) provides the role of glucosinolate metabolism in response to drought stress. Molecules 23, 1186. doi: 10.3390/molecules23051186
Fang, S., Zhao, P., Tan, Z., Peng, Y., Xu, L., Jin, Y., et al. (2022). Combining physio-biochemical characterization and transcriptome analysis reveal the responses to varying degrees of drought stress in Brassica napus L. Int. J. Mol. Sci. 23, 8555. doi: 10.3390/ijms23158555
Feng, R., Wei, C., Tu, S. (2013). The roles of selenium in protecting plants against abiotic stresses. Environ. Exp. Bot. 87, 58–68. doi: 10.1016/j.envexpbot.2012.09.002
Gai, Z., Wang, Y., Ding, Y., Qian, W., Qiu, C., Xie, H., et al. (2020). Exogenous abscisic acid induces the lipid and flavonoid metabolism of tea plants under drought stress. Sci. Rep. 10, 1–13. doi: 10.1038/s41598-020-69080-1
Hao, J., Lou, P., Han, Y., Zheng, L., Lu, J., Chen, Z., et al. (2022). Ultraviolet-B irradiation increases antioxidant capacity of pakchoi (Brassica rapa L.) by inducing flavonoid biosynthesis. Plants 11, 766. doi: 10.3390/plants11060766
Hasanuzzaman, M., Alam, M. M., Nahar, K., Ahamed, K. U., Fujita, M. (2014). Exogenous salicylic acid alleviates salt stress-induced oxidative damage in Brassica napus by enhancing the antioxidant defense and glyoxalase systems. Aust. J. Crop Sci. 8, 631–639. doi: 10.3316/informit.292398401589540
Hashemi, A., Abdolzadeh, A., Sadeghipour, H. R. (2010). Beneficial effects of silicon nutrition in alleviating salinity stress in hydroponically grown canola, Brassica napus L., plants. Soil Sci. Plant Nutr. 56, 244–253. doi: 10.1111/j.1747-0765.2009.00443.x
He, Z., Ji, R., Havlickova, L., Wang, L., Li, Y., Lee, H. T., et al. (2021). Genome structural evolution in Brassica crops. Nat. Plants 7, 757–765. doi: 10.1038/s41477-021-00928-8
Heinze, M., Hanschen, F. S., Wiesner-Reinhold, M., Baldermann, S., Gräfe, J., Schreiner, M., et al. (2018). Effects of Developmental Stages and Reduced UVB and Low UV Conditions on Plant Secondary Metabolite Profiles in Pak Choi (Brassica rapa subsp. chinensis). J. Agric. Food Chem. 66, 1678–1692. doi: 10.1021/acs.jafc.7b03996
Hong, B., Zhou, B., Peng, Z., Yao, M., Wu, J., Wu, X., et al. (2023). Tissue-specific transcriptome and metabolome analysis reveals the response mechanism of Brassica napus to waterlogging stress. Int. J. Mol. Sci. 24, 6015. doi: 10.3390/ijms24076015
Hsieh, K., Huang, A. H. C. (2007). Tapetosomes in Brassica tapetum accumulate endoplasmic reticulum-derived flavonoids and alkanes for delivery to the pollen surface. Plant Cell 19, 582–596. doi: 10.1105/tpc.106.049049
Huang, Y., Hussain, M. A., Luo, D., Xu, H., Zeng, C., Havlickova, L., et al. (2020). A Brassica napus reductase gene dissected by associative transcriptomics enhances plant adaption to freezing stress. Front. Plant Sci. 11. doi: 10.3389/fpls.2020.00971
Hwang, S.-J., Chun, J.-H., Kim, S.-J. (2017). Effect of cold stress on carotenoids in kale leaves (Brassica oleracea). Korean J. Environ. Agric. 36, 106–112. doi: 10.5338/KJEA.2017.36.2.19
Islam, M. T., Lee, B. R., La, V. H., Lee, H., Jung, W. J., Bae, D. W., et al. (2019). p-Coumaric acid induces jasmonic acid-mediated phenolic accumulation and resistance to black rot disease in Brassica napus. Physiol. Mol. Plant Pathol. 106, 270–275. doi: 10.1016/j.pmpp.2019.04.001
Kask, K., Kännaste, A., Talts, E., Copolovici, L., Niinemets, Ü. (2016). How specialized volatiles respond to chronic and short-term physiological and shock heat stress in Brassica nigra. Plant Cell Environ. 39, 2027–2042. doi: 10.1111/pce.12775
Kessler, A., Kalske, A. (2018). Plant secondary metabolite diversity and species interactions. Annu. Rev. Ecol. Evol. Syst. 49, 115–138. doi: 10.1146/annurev-ecolsys-110617-062406
Kim, Y., Hwang, I., Jung, H. J., Park, J. I., Kang, J. G., Nou, I. S. (2016). Genome-Wide Classification and Abiotic Stress-Responsive Expression Profiling of Carotenoid Oxygenase Genes in Brassica rapa and Brassica oleracea. J. Plant Growth Regul. 35, 202–214. doi: 10.1007/s00344-015-9520-y
Kim, J. Y., Kang, Y. E., Lee, S. I., Kim, J. A., Muthusamy, M., Jeong, M. J. (2020). Sound waves affect the total flavonoid contents in Medicago sativa, Brassica oleracea and Raphanus sativus sprouts. J. Sci. Food Agric. 100, 431–440. doi: 10.1002/jsfa.10077
Kim, J., Lee, W. J., Vu, T. T., Jeong, C. Y., Hong, S. W., Lee, H. (2017). High accumulation of anthocyanins via the ectopic expression of AtDFR confers significant salt stress tolerance in Brassica napus L. Plant Cell Rep. 36, 1215–1224. doi: 10.1007/s00299-017-2147-7
Klein, M., Papenbrock, J. (2008). Sulfotransferases and their role in glucosinolate biosynthesis. Sulfur Assim. Abiotic Stress Plants, 149–166. doi: 10.1007/978-3-540-76326-0_7
Kopsell, D. A., Sams, C. E., Charron, C. S., Kopsell, D. E., Randle, W. M. (2007). Kale carotenoids remain stable while glucosinolates and flavor compounds respond to changes in selenium and sulfur fertility. Acta Hortic. 744, 303–310. doi: 10.17660/ActaHortic.2007.744.31
Kumar, K., Debnath, P., Singh, S., Kumar, N. (2023). An overview of plant phenolics and their involvement in abiotic stress tolerance. Stresses 3, 570–585. doi: 10.3390/stresses3030040
Kumar, S., Sairam, R. K., Prabhu, K. V. (2013). Physiological traits for high temperature stress tolerance in Brassica juncea. Indian J. Plant Physiol. 18, 89–93. doi: 10.1007/s40502-013-0015-1
La, V. H., Lee, B. R., Islam, M. T., Park, S. H., Jung, H., Bae, D. W., et al. (2019a). Characterization of salicylic acid-mediated modulation of the drought stress responses: Reactive oxygen species, proline, and redox state in Brassica napus. Environ. Exp. Bot. 157, 1–10. doi: 10.1016/j.envexpbot.2018.09.013
La, V. H., Lee, B. R., Zhang, Q., Park, S. H., Islam, M. T., Kim, T. H. (2019b). Salicylic acid improves drought-stress tolerance by regulating the redox status and proline metabolism in Brassica rapa. Hortic. Environ. Biotechnol. 60, 31–40. doi: 10.1007/s13580-018-0099-7
Lalay, G., Ullah, S., Ahmed, I. (2022). Physiological and biochemical responses of Brassica napus L. @ to drought-induced stress by the application of biochar and Plant Growth Promoting Rhizobacteria. Microsc. Res. Tech. 85, 1267–1281. doi: 10.1002/jemt.23993
Lee, H.-J., Chun, J.-H., Kim, S.-J. (2017). Effects of Water Stress on Carotenoid and Proline Contents in Kale (Brassica oleracea var. acephala) leaves. Korean J. Environ. Agric. 36, 97–105. doi: 10.5338/KJEA.2017.36.2.16
Lee, B.-R., Zhang, Q., Kim, T.-H. (2014). Lignification in relation to the influence of water-deficit stress in Brassica napus. J. Korean Soc Grassl. Forage Sci. 34, 15–20. doi: 10.5333/KGFS.2014.34.1.15
Linić, I., Mlinarić, S., Brkljačić, L., Pavlović, I., Smolko, A., Salopek-Sondi, B. (2021). Ferulic acid and salicylic acid foliar treatments reduce short-term salt stress in chinese cabbage by increasing phenolic compounds accumulation and photosynthetic performance. Plants (Basel Switzerland) 10, 2346. doi: 10.3390/plants10112346
Linić, I., Šamec, D., Grúz, J., Vujčić Bok, V., Strnad, M., Salopek-Sondi, B. (2019). Involvement of phenolic acids in short-term adaptation to salinity stress is species-specific among Brassicaceae. Plants (Basel Switzerland) 8, 155. doi: 10.3390/plants8060155
Liu, D., Hu, L. Y., Ali, B., Yang, A. G., Wan, G. L., Xu, L., et al. (2016). Influence of 5-aminolevulinic acid on photosynthetically related parameters and gene expression in Brassica napus L. under drought stress. Soil Sci. Plant Nutr. 62, 254–262. doi: 10.1080/00380768.2016.1198216
Liu, Z., Wang, H., Xie, J., Lv, J., Zhang, G., Hu, L., et al. (2021). The roles of cruciferae glucosinolates in disease and pest resistance. Plants 10, 1–15. doi: 10.3390/plants10061097
Ljubej, V., Karalija, E., Salopek-sondi, B., Šamec, D. (2021a). Effects of short-term exposure to low temperatures on proline, pigments, and phytochemicals level in kale (Brassica oleracea var. acephala). Horticulturae 7, 0–9. doi: 10.3390/horticulturae7100341
Ljubej, V., Radojčić Redovniković, I., Salopek-Sondi, B., Smolko, A., Roje, S., Šamec, D. (2021b). Chilling and freezing temperature stress differently influence glucosinolates content in brassica oleracea var. Acephala. Plants 10, 1–11. doi: 10.3390/plants10071305
Ljubej, V., Radojčić Redovniković, I., Salopek-Sondi, B., Smolko, A., Roje, S., Šamec, D. (2021c). Chilling and Freezing Temperature Stress Differently Influence Glucosinolates Content in Brassica oleracea var. acephala. Plants 10, 1305. doi: 10.3390/plants10071305
Loreti, E., Povero, G., Novi, G., Solfanelli, C., Alpi, A., Perata, P. (2008). Gibberellins, jasmonate and abscisic acid modulate the sucrose-induced expression of anthocyanin biosynthetic genes in Arabidopsis. New Phytol. 179, 1004–1016. doi: 10.1111/j.1469-8137.2008.02511.x
Lozano-Elena, F., Fàbregas, N., Coleto-Alcudia, V., Caño-Delgado, A. I. (2022). Analysis of metabolic dynamics during drought stress in Arabidopsis plants. Sci. Data 9, 1–12. doi: 10.1038/s41597-022-01161-4
Martínez-Ballesta, M., del, C., Moreno, D. A., Carvajal, M. (2013). The physiological importance of glucosinolates on plant response to abiotic stress in Brassica. Int. J. Mol. Sci. 14, 11607–11625. doi: 10.3390/ijms140611607
Miao, H., Zeng, W., Wang, J., Zhang, F., Sun, B., Wang, Q. (2021). Improvement of glucosinolates by metabolic engineering in Brassica crops. aBIOTECH 2, 314–329. doi: 10.1007/s42994-021-00057-y
Muthusamy, M., Kim, J., Kim, S., Park, S., Lee, S. (2021). Brpp5.2 overexpression confers heat shock tolerance in transgenic brassica rapa through inherent chaperone activity, induced glucosinolate biosynthesis, and differential regulation of abiotic stress response genes. Int. J. Mol. Sci. 22, 6437. doi: 10.3390/ijms22126437
Nephali, L., Piater, L. A., Dubery, I. A., Patterson, V., Huyser, J., Burgess, K., et al. (2020). Biostimulants for plant growth and mitigation of abiotic stresses: A metabolomics perspective. Metabolites 10, 1–26. doi: 10.3390/metabo10120505
Neugart, S., Baldermann, S., Hanschen, F. S., Klopsch, R., Wiesner-Reinhold, M., Schreiner, M. (2018). The intrinsic quality of brassicaceous vegetables: How secondary plant metabolites are affected by genetic, environmental, and agronomic factors. Sci. Hortic. (Amsterdam). 233, 460–478. doi: 10.1016/j.scienta.2017.12.038
Neugart, S., Bumke-Vogt, C. (2021). Flavonoid glycosides in brassica species respond to uv-b depending on exposure time and adaptation time. Molecules 26, 1–15. doi: 10.3390/molecules26020494
Neugart, S., Fiol, M., Schreiner, M., Rohn, S., Zrenner, R., Kroh, L. W., et al. (2013). Low and moderate photosynthetically active radiation affects the flavonol glycosides and hydroxycinnamic acid derivatives in kale (Brassica oleracea var. sabellica) dependent on two low temperatures. Plant Physiol. Biochem. 72, 161–168. doi: 10.1016/j.plaphy.2013.04.002
Neugart, S., Krumbein, A., Zrenner, R. (2016). Influence of light and temperature on gene expression leading to accumulation of specific flavonol glycosides and hydroxycinnamic acid derivatives in kale (Brassica oleracea var. sabellica). Front. Plant Sci. 7. doi: 10.3389/fpls.2016.00326
Ortega-Hernández, E., Antunes-Ricardo, M., Jacobo-Velázquez, D. A. (2021). Improving the Health-Benefits of Kales (Brassica oleracea L. var. acephala DC) through the Application of Controlled Abiotic Stresses: A Review. Plants 10, 2629. doi: 10.3390/plants10122629
Pant, P., Pandey, S., Dall’Acqua, S. (2021). The influence of environmental conditions on secondary metabolites in medicinal plants: A literature review. Chem. Biodivers. 18, e2100345. doi: 10.1002/cbdv.202100345
Park, S., Arasu, M. V., Jiang, N., Choi, S. H., Lim, Y. P., Park, J. T., et al. (2014). Metabolite profiling of phenolics, anthocyanins and flavonols in cabbage (Brassica oleracea var. capitata). Ind. Crops Prod. 60, 8–14. doi: 10.1016/j.indcrop.2014.05.037
Park, J. E., Kim, J., Purevdorj, E., Son, Y. J., Nho, C. W., Yoo, G. (2021). Effects of long light exposure and drought stress on plant growth and glucosinolate production in pak choi (Brassica rapa subsp. chinensis). Food Chem. 340, 128167. doi: 10.1016/j.foodchem.2020.128167
Pereira, A. G., Cassani, L., Garcia-Oliveira, P., Otero, P., Mansoor, S., Echave, J., et al. (2023). “Plant alkaloids: production, extraction, and potential therapeutic properties,” in Natural Secondary Metabolites (Cham: Springer International Publishing), 157–200. doi: 10.1007/978-3-031-18587-8_6
Podda, A., Pollastri, S., Bartolini, P., Pisuttu, C., Pellegrini, E., Nali, C., et al. (2019). Drought stress modulates secondary metabolites in Brassica oleracea L. convar. acephala (DC) Alef, var. sabellica L. J. Sci. Food Agric. 99, 5533–5540. doi: 10.1002/jsfa.9816
Poveda, J., Francisco, M., Cartea, M. E., Velasco, P. (2020). Development of transgenic brassica crops against biotic stresses caused |by pathogens and arthropod pests. Plants 9, 1–23. doi: 10.3390/plants9121664
Raees, N., Ullah, S., Nafees, M. (2022). Interactive effect of tocopherol, salicylic acid and ascorbic acid on agronomic characters of two genotypes of Brassica napus L. Under induced drought and salinity stresses. Gesunde Pflanz 75 (5), 1905-1923. doi: 10.1007/s10343-022-00808-x
Rao, S. Q., Chen, X. Q., Wang, K. H., Zhu, Z. J., Yang, J., Zhu, B. (2021). Effect of short-term high temperature on the accumulation of glucosinolates in Brassica rapa. Plant Physiol. Biochem. 161, 222–233. doi: 10.1016/j.plaphy.2021.02.013
Rasheed, F., Sehar, Z., Fatma, M., Iqbal, N., Masood, A., Anjum, N. A., et al. (2022). Involvement of ethylene in reversal of salt stress by salicylic acid in the presence of sulfur in mustard (Brassica juncea L.). J. Plant Growth Regul. 41, 3449–3466. doi: 10.1007/s00344-021-10526-9
Rezayian, M., Niknam, V., Ebrahimzadeh, H. (2018). Differential responses of phenolic compounds of Brassica napus under drought stress. Iran. J. Plant Physiol. 8, 2417–2425. doi: 10.22034/ijpp.2018.540887
Rezayian, M., Zarinkamar, F. (2023). Nitric oxide, calmodulin and calcium protein kinase interactions in the response of Brassica napus to salinity stress. Plant Biol. 25, 411–419. doi: 10.1111/plb.13511
Salami, M., Heidari, B., Tan, H. (2023). Comparative profiling of polyphenols and antioxidants and analysis of antiglycation activities in rapeseed (Brassica napus L.) under different moisture regimes. Food Chem. 399, 133946. doi: 10.1016/j.foodchem.2022.133946
Salehin, M., Li, B., Tang, M., Katz, E., Song, L., Ecker, J. R., et al. (2019). Auxin-sensitive Aux/IAA proteins mediate drought tolerance in Arabidopsis by regulating glucosinolate levels. Nat. Commun. 10, 4021. doi: 10.1038/s41467-019-12002-1
Šamec, D., Karalija, E., Šola, I., Vujčić Bok, V., Salopek-Sondi, B. (2021a). The role of polyphenols in abiotic stress response: The influence of molecular structure. Plants 10, 1–24. doi: 10.3390/plants10010118
Šamec, D., Linić, I., Salopek-Sondi, B. (2021b). Salinity stress as an elicitor for phytochemicals and minerals accumulation in selected leafy vegetables of Brassicaceae. Agronomy 11, 361. doi: 10.3390/agronomy11020361
Sangtarash, M. H., Qaderi, M. M., Chinnappa, C. C., Reid, D. M. (2009). Differential sensitivity of canola (Brassica napus) seedlings to ultraviolet-B radiation, water stress and abscisic acid. Environ. Exp. Bot. 66, 212–219. doi: 10.1016/j.envexpbot.2009.03.004
Sarikamiş, G., Çakir, A. (2017). Influence of salinity on aliphatic and indole glucosinolates in broccoli (Brassica oleracea var. Italica). Appl. Ecol. Environ. Res. 15, 1781–1788. doi: 10.15666/aeer/1503_17811788
Schmidt, S., Zietz, M., Schreiner, M., Rohn, S., Kroh, L. W., Krumbein, A. (2010). Genotypic and climatic influences on the concentration and composition of flavonoids in kale (Brassica oleracea var. sabellica). Food Chem. 119, 1293–1299. doi: 10.1016/j.foodchem.2009.09.004
Seo, M.-S., Jin, M., Sohn, S.-H., Kim, J. S. (2017). Expression profiles of BrMYB transcription factors related to glucosinolate biosynthesis and stress response in eight subspecies of Brassica rapa. FEBS Open Bio 7, 1646–1659. doi: 10.1002/2211-5463.12231
Shaw, R. K., Shen, Y., Yu, H., Sheng, X., Wang, J., Gu, H. (2022). Multi-omics approaches to improve clubroot resistance in brassica with a special focus on Brassica oleracea L. Int. J. Mol. Sci. 23, 9280. doi: 10.3390/ijms23169280
Shawon, R. A., Kang, B. S., Lee, S. G., Kim, S. K., Ju Lee, H., Katrich, E., et al. (2020). Influence of drought stress on bioactive compounds, antioxidant enzymes and glucosinolate contents of Chinese cabbage (Brassica rapa). Food Chem. 308, 125657. doi: 10.1016/j.foodchem.2019.125657
Simoh, S., Linthorst, H. J. M., Lefeber, A. W. M., Erkelens, C., Kim, H. K., Choi, Y. H., et al. (2010). Metabolic changes of Brassica rapa transformed with a bacterial isochorismate synthase gene. J. Plant Physiol. 167, 1525–1532. doi: 10.1016/j.jplph.2010.06.022
Solecka, D., Boudet, A. M., Kacperska, A. (1999). Phenylpropanoid and anthocyanin changes in low-temperature treated winter oilseed rape leaves. Plant Physiol. Biochem. 37, 491–496. doi: 10.1016/S0981-9428(99)80054-0
Sun, Y., Liu, X., Li, W., Wang, X., Zhong, X., Gao, Y., et al. (2023). The regulatory metabolic networks of the Brassica campestris L. hairy roots in response to cadmium stress revealed from proteome studies combined with a transcriptome analysis. Ecotoxicol. Environ. Saf. 263, 115214. doi: 10.1016/j.ecoenv.2023.115214
Sun, T., Rao, S., Zhou, X., Li, L. (2022). Plant carotenoids: recent advances and future perspectives. Mol. Hortic. 2, 1–21. doi: 10.1186/s43897-022-00023-2
Sun, B., Yan, H., Zhang, F., Wang, Q. (2012). Effects of plant hormones on main health-promoting compounds and antioxidant capacity of Chinese kale. Food Res. Int. 48, 359–366. doi: 10.1016/j.foodres.2012.04.021
Tan, P., Zeng, C., Wan, C., Liu, Z., Dong, X., Peng, J., et al. (2021). Metabolic profiles of Brassica juncea roots in response to cadmium stress. Metabolites 11, 383. doi: 10.3390/metabo11060383
Tang, Y., Zhang, G., Jiang, X., Shen, S., Guan, M., Tang, Y., et al. (2023b). Genome-wide association study of glucosinolate metabolites (mGWAS) in Brassica napus L. Plants 12, 639. doi: 10.3390/plants12030639
Tang, B., Zhang, B. H., Mo, C. Y., Fu, W. Y., Yang, W., Wang, Q. Q., et al. (2023a). Comparative transcriptomics reveal the mechanisms underlying the glucosinolate metabolic response in leaf Brassica juncea L. under cold stress. Agronomy 13, 1922. doi: 10.3390/agronomy13071922
Thamil Arasan, S. K., Park, J. I., Ahmed, N. U., Jung, H. J., Hur, Y., Kang, K. K., et al. (2013). Characterization and expression analysis of dirigent family genes related to stresses in Brassica. Plant Physiol. Biochem. 67, 144–153. doi: 10.1016/j.plaphy.2013.02.030
Thiruvengadam, M., Chung, I. M. (2015). Selenium, putrescine, and cadmium influence health-promoting phytochemicals and molecular-level effects on turnip (Brassica rapa ssp. rapa). Food Chem. 173, 185–193. doi: 10.1016/j.foodchem.2014.10.012
Viana, V. E., Aranha, B. C., Busanello, C., Maltzahn, L. E., Panozzo, L. E., de Oliveira, A. C., et al. (2022). Metabolic profile of canola (Brassica napus L.) seedlings under hydric, osmotic and temperature stresses. Plant Stress 3, 100059. doi: 10.1016/j.stress.2022.100059
Wang, M., Cai, C., Lin, J., Tao, H., Zeng, W., Zhang, F., et al. (2020a). Combined treatment of epi-brassinolide and NaCl enhances the main phytochemicals in Chinese kale sprouts. Food Chem. 315, 126275. doi: 10.1016/j.foodchem.2020.126275
Wang, X.s., Fu, H.l., Gong, F.y., Zhang, Y., He, C.t., Yang, Z. (2020b). Lignin side chain region participates in Cd detoxification related to the cultivar-dependent Cd accumulation in Brassica chinensis L. J. Hazard. Mater. 392, 122264. doi: 10.1016/j.jhazmat.2020.122264
Wang, C., Li, Z., Zhang, L., Gao, Y., Cai, X., Wu, W. (2022). Identifying key metabolites associated with glucosinolate biosynthesis in response to nitrogen management strategies in two rapeseed (Brassica napus) varieties. J. Agric. Food Chem. 70, 634–645. doi: 10.1021/acs.jafc.1c06472
Wang, D., Lu, Q., Jin, S., Fan, X., Ling, H. (2023). Pectin, lignin and disease resistance in Brassica napus L.: an update. Horticulturae 9, 1–12. doi: 10.3390/horticulturae9010112
Wang, W., Pang, J., Zhang, F., Sun, L., Yang, L., Zhao, Y., et al. (2021). Integrated transcriptomics and metabolomics analysis to characterize alkali stress responses in canola (Brassica napus L.). Plant Physiol. Biochem. PPB 166, 605–620. doi: 10.1016/j.plaphy.2021.06.021
Wu, X., Huang, H., Childs, H., Wu, Y., Yu, L., Pehrsson, P. R. (2021). Glucosinolates in brassica vegetables: characterization and factors that influence distribution, content, and intake. Annu. Rev. Food Sci. Technol. 12, 485–511. doi: 10.1146/annurev-food-070620-025744
Xuan, L., Hussain, N., Wang, Z., Jiang, Y., Chen, M., Jiang, L. (2015). Comparison of vitality between seedlings germinated from black-coated and yellow-coated seeds of a turnip rape (Brassica rapa L.) subjected to NaCl and CdCl2 stresses. Plant Growth Regul. 76, 61–70. doi: 10.1007/s10725-014-0019-5
Yin, L., Sun, Y., Chen, X., Liu, J., Feng, K., Luo, D., et al. (2023). Genome-Wide Analysis of the HD-Zip Gene Family in Chinese Cabbage (Brassica rapa subsp. pekinensis) and the Expression Pattern at High Temperatures and in Carotenoids Regulation. Agronomy 13, 1324. doi: 10.3390/agronomy13051324
Yusuf, M., Fariduddin, Q., Varshney, P., Ahmad, A. (2012). Salicylic acid minimizes nickel and/or salinity-induced toxicity in Indian mustard (Brassica juncea) through an improved antioxidant system. Environ. Sci. pollut. Res. 19, 8–18. doi: 10.1007/s11356-011-0531-3
Keywords: Brassica, abiotic stress, glucosinolates, flavonoids, carotenoids, phenolic acids, alkaloids
Citation: Muthusamy M and Lee SI (2024) Abiotic stress-induced secondary metabolite production in Brassica: opportunities and challenges. Front. Plant Sci. 14:1323085. doi: 10.3389/fpls.2023.1323085
Received: 17 October 2023; Accepted: 13 December 2023;
Published: 04 January 2024.
Edited by:
Adriano Sofo, University of Basilicata, ItalyReviewed by:
Branka Salopek Sondi, Rudjer Boskovic Institute, CroatiaSayed Mohammad Mohsin, Sher-e-Bangla Agricultural University, Bangladesh
Copyright © 2024 Muthusamy and Lee. This is an open-access article distributed under the terms of the Creative Commons Attribution License (CC BY). The use, distribution or reproduction in other forums is permitted, provided the original author(s) and the copyright owner(s) are credited and that the original publication in this journal is cited, in accordance with accepted academic practice. No use, distribution or reproduction is permitted which does not comply with these terms.
*Correspondence: Soo In Lee, c2lsZWVAa29yZWEua3I=