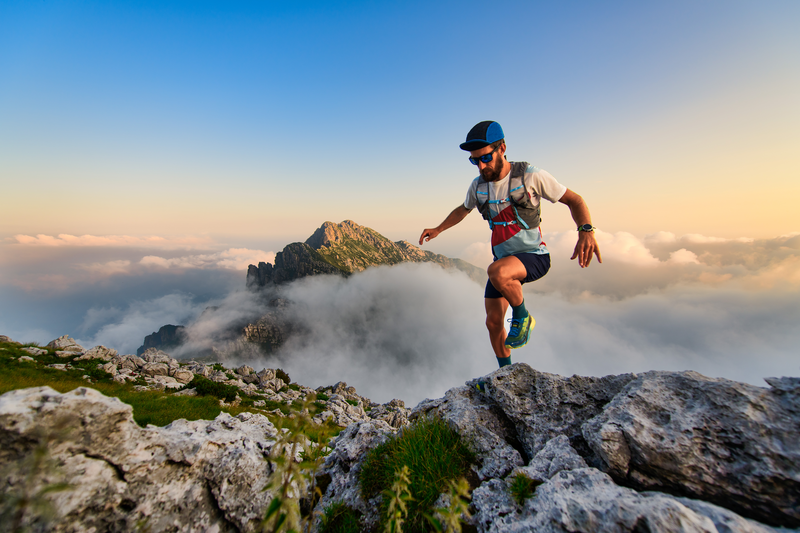
94% of researchers rate our articles as excellent or good
Learn more about the work of our research integrity team to safeguard the quality of each article we publish.
Find out more
ORIGINAL RESEARCH article
Front. Plant Sci. , 04 January 2024
Sec. Plant Bioinformatics
Volume 14 - 2023 | https://doi.org/10.3389/fpls.2023.1318383
This article is part of the Research Topic Advances on Genomics and Genetics of Horticultural Crops and their Contribution to Breeding Efforts, volume II View all 12 articles
Natural resistance-associated macrophage proteins (NRAMPs) are a class of metal transporters found in plants that exhibit diverse functions across different species. Transporter proteins facilitate the absorption, distribution, and sequestration of metallic elements within various plant tissues. Despite the extensive identification of NRAMP family genes in various species, a full analysis of these genes in tree species is still necessary. Genome-wide identification and bioinformatics analysis were performed to understand the roles of NRAMP genes in copper (CuCl2) stress in Kandelia obovata (Ko). In Arachis hypogaea L., Populus trichocarpa, Vitis vinifera, Phaseolus vulgaris L., Camellia sinensis, Spirodela polyrhiza, Glycine max L. and Solanum lycopersicum, a genome-wide study of the NRAMP gene family was performed earlier. The domain and 3D structural variation, phylogenetic tree, chromosomal distributions, gene structure, motif analysis, subcellular localization, cis-regulatory elements, synteny and duplication analysis, and expression profiles in leaves and CuCl2 were all investigated in this research. In order to comprehend the notable functions of the NRAMP gene family in Kandelia obovata, a comprehensive investigation was conducted at the genomic level. This study successfully found five NRAMP genes, encompassing one gene pair resulting from whole-genome duplication and a gene that had undergone segmental duplication. The examination of chromosomal position revealed an unequal distribution of the KoNRAMP genes across chromosomes 1, 2, 5, 7, and 18. The KoNRAMPs can be classified into three subgroups (I, II, and SLC) based on phylogeny and synteny analyses, similar to Solanum lycopersicum. Examining cis-regulatory elements in the promoters revealed five hormone-correlated responsive elements and four stress-related responsive elements. The genomic architecture and properties of 10 highly conserved motifs are similar among members of the NRAMP gene family. The conducted investigations demonstrated that the expression levels of all five genes exhibited alterations in response to different levels of CuCl2 stress. The results of this study offer crucial insights into the roles of KoNRAMPs in the response of Kandelia obovata to CuCl2 stress.
Plants have developed adaptive mechanisms that facilitate the uptake of necessary nutrients and mitigate the adverse effects of heavy metal (HM) toxicity. One important process is the existence of membrane transport systems in plants, which are essential for numerous aspects of vital mineral and nutrient homeostasis as well as the accumulation, translocation, and detoxification of hazardous metals (Wu et al., 2021; Liu et al., 2022; Ma et al., 2023). Previous studies have demonstrated that NRAMPs play a crucial role as integral membrane transporters in various species, including plants. These proteins are responsible for metal uptake, transport, accumulation, and detoxification (Zhang et al., 2020; Tian et al., 2021). The NRAMP gene was initially identified in rats in 1993 (Vidal et al., 1993). The NRAMPs are a significantly conserved family of integral membrane proteins that play a crucial role in facilitating the transport of divalent metals across the cellular membrane. NRAMP genes have been detected in several organisms, including bacteria, fungi, plants, and mammals (Cellier et al., 1996; Segond et al., 2009). The NRAMP proteins have a high degree of conservation across various organisms, from bacteria to mammals. These proteins typically consist of 10-12 transmembrane domains (TMD) and possess a consensus transport signature (Cellier et al., 1995; Tan et al., 2023).
However, the majority of NRAMP proteins can transport several metal ions, including cobalt (Co), nickel (Ni), copper (Cu), iron (Fe), manganese (Mn), zinc (Zn), and cadmium (Cd)(Nevo and Nelson, 2006; Ishida et al., 2018). The roles of NRAMP proteins in plants have been investigated in Arabidopsis thaliana (Segond et al., 2009), Camellia sinensis (Li et al., 2021), Spirodela polyrhiza (Chen et al., 2021), Glycine Max L. (Qin et al., 2017), Solanum tuberosum (Tian et al., 2021), Populus trichocarpa (Ma et al., 2023), Phaseolus vulgaris L. (Ishida et al., 2018), Arachis hypogaea L. (Tan et al., 2023). Understanding the physiological role of these elements in plant development requires identifying a particular collection of transporters that supply the delicate balance of metal concentration across the cellular membrane (Ishida et al., 2018; Kravchenko et al., 2018). Nevertheless, the function of the NRAMP protein family and the Cu transport pathways in Kandelia obovata is still unclear.
Mangroves serve as a significant reservoir for HMs, with Cu, lead (Pb), and zinc being the predominant HM pollutants (Cheng et al., 2017) (Bodin et al., 2013). HMs represent a significant class of anthropogenic harmful substances within mangrove ecosystems (Huang and Wang, 2010). Among them, Cu, Zn, and Pb are often encountered pollutants (Qiu et al., 2011; Li et al., 2015). Shen et al. (2021) reported that HM enrichment on the root surface of Kandeliaobovata is higher than some other mangrove plants in South China, including Acanthus ilicifolius, Aeagiceras corniculatum, Bruguiera gymnorrhiza, and Heritiera littorlis. A viviparous mangrove species found in the intertidal zones of tropical and subtropical coasts, Kandelia obovata is a member of the Rhizophoraceae family in the Malpighiales (Sheue et al., 2003). The distribution of this species spreads from northern Vietnam to southeast China and south Japan in the East Asian region (Sheue et al., 2003; Yang et al., 2019). The native distribution of this species encompasses the Hainan, Guangdong (including Hong Kong and Macau), Guangxi, Fujian, and Taiwan Provinces in China (Li and Lee, 1997). Kandelia obovata, a mangrove species found in China, exhibits notable cold resistance and salt tolerance, enabling its survival in northern regions (Yang et al., 2019). Prior research has demonstrated that this species could collect HMs (Weng et al., 2012; Weng et al., 2014). Kandeliaobovata is a woody plant found in tidal salt wetlands in tropical and subtropical regions from East to Southeast Asia (Sheue et al., 2003; Hu et al., 2020). Kandelia obovata adapts to transitional ecosystems characterized by the interface between land and water by effectively coping with periodic and aperiodic tidal influences. These tidal impacts give rise to elevated salinity levels, substantial erosion, and anaerobic conditions (Giri et al., 2011; Hu et al., 2020).
Copper is a protein cofactor that participates in electron transfer activities, hence playing a crucial role in these biochemical processes. Additionally, Cu is an essential component for plants. Cu fulfills several functions in biological processes, such as photosynthesis, respiration, ethylene sensing, reactive oxygen metabolism, and cell wall remodeling (Burkhead et al., 2009). Cu is a vital plant element, but excessive quantities can lead to phytotoxicity. Soils can get contaminated by Cu and other HMs due to human activities, such as industrial, mining, and agricultural practices. These activities include sewage sludges, organic wastes, fertilizers, and fungicides. Therefore, it is crucial to investigate the correlation between HMs and plants, given their impact on crop yield and plant development (Mourato and Martins, 1900; Fageria, 2001).
As far as current information is concerned, the presence of the NRAMP gene family in Kandelia obovata has not been documented. Therefore, this study is the first instance in which a genome-wide analysis was conducted to identify NRAMP genes inside the genome of Kandelia obovata. This study aimed to identify and characterize five NRAMP genes and then investigated their expression levels in response to five CuCl2 treatments. In order to gain a deeper understanding of the evolution of NRMAP genes in Kandelia obovata, various aspects were examined using bioinformatics methodologies. These included the analysis of gene structures, physicochemical properties, chromosomal distribution, synteny and duplication structures, conserved motifs, cis-elements, phylogenetic relationships, subcellular localization, and the expression profiles of NRAMP homologs. The present study investigates the characterization and expression analysis of the NRAMP family in Kandelia obovata. This research seeks to establish a theoretical foundation for future investigations on the response of the NRAMP family to CuCl2 treatment in Kandelia obovata plants.
For Kandelia obovata, the genome sequences were obtained from the NCBI database (https://www.ncbi.nlm.nih.gov/; BioProject/GWH, Accession codes: PRJCA002330/GWHACBH00000000) and the Kandelia obovata protein database (https://www.omicsclass.com/article/310) (Hu et al., 2020). Two databases confirm hypothetical proteins: Pfam (http://pfam.xfam.org/) and NCBI CDD (https://www.omicsclass.com/article/310, E-value 1.2 × 10−28). The protein sequence analysis of NRAMP linked with the domain profile was conducted utilizing the Pfam database (http://pfam.xfam.org). The Kandelia obovata genome database (https://www.omicsclass.com/article/310) and the National Center for Biotechnology Information (NCBI) database (https://www.ncbi.nlm.nih.gov/) were employed to identify and validate five NRAMP family genes, namely KoNRAMP1, KoNRAMP2, KoNRAMP3, KoNRAMP4, and KoNRAMP5. Protparam (http://web.expasy.org/protparam/) was utilized to obtain physicochemical properties (Si et al., 2023).
Using the NCBI database and https://www.omicsclass.com/article/310, the genomic locations and protein sequences of every NRAMP gene in Kandelia obovata were identified. The distribution positions of NRAMP genes on chromosomes were also evaluated. The MapGene2Chromosome (MG2C) tool was utilized to determine the chromosomal locations of NRAMP genes in Kandelia obovata. The MG2C tool, version 2.0, was used at the URL: http://mg2c.iask.in/mg2c (Hussain et al., 2022a).
Protein sequences of NRAMP genes from the following species were used in the phylogenetic analysis: Vitis vinifera (Vv), Aegiceras corniculatum (Ac), Solanum tuberosum (St), Populus trichocarpa (Pt), Kandelia obovata (Ko), and Arabidopsis thaliana (At). The MEGA11 (V 6.06) software, available at www.megasoftware.net, was commonly employed to align protein sequences (Hashemipetroudi et al., 2023). The phylogenetic tree was constructed using the neighbor-joining (NJ) method, using 1000 bootstrap replicates. Using Fig Tree V1.4.4, the phylogenetic tree was examined and modified (Hussain et al., 2023).
The genome of Kandelia obovata has been revealed to include five genes belonging to the NRAMP family. The exon/intron arrangements of the five NRAMP genes were exhibited along with the structural analyses of the genes via web software (http://gsds.cbi.pku.edu.cn) (Shang et al., 2023). More conserved strings or sections were found in the protein sequences of the five NRAMP proteins, according to the online tool MEME v5.4.1, which can be accessed at https://meme-suite.org/meme/tools/glam2scan (Shang et al., 2023). The application employed the following settings: sequencing of alphabet DNA, RNA, or protein; site distribution limited to zero or one occurrence per sequence (zoops); motif finding mode set to classic mode; and 10 motifs. Using the TBtools program, the MEME findings were displayed following downloading the relevant mast file (Hussain et al., 2022b).
The Minspan (Available online) generated synteny associations of NRAMP genes from the following species: Vitis vinifera (Vv), Aegiceras corniculatum (Ac), Solanum tuberosum (St), Populus trichocarpa (Pt), and Kandelia obovata (Ko). With the KaKs Calculator 2.0 (https://sourceforge.net/projects/kakscalculator2/), the synonymous (Ks), non-synonymous (Ka), and Ka/Ks ratios were computed in order to examine the evolutionary constraints of each pair of NRAMP genes (Hussain et al., 2023).
The NRAMP family members’ 2,500 bp upstream sequences were gathered using the Kandelia obovata genome assembly database. The PlantCARE tool (http://bioinformatics.psb.ugent.be/webtools/plantcare/html/) was employed to identify CREs from the obtained sequences. TBtools was used to generate the most prevalentCREs identified for the NRAMP genes, following a comprehensive analysis of the frequency of each CRE motif (Yang et al., 2022; Si et al., 2023; Yaghobi and Heidari, 2023).
The three-dimensional (3D) structure can be estimated using SWISS-MODEL (https://swissmodel.expasy.org/interactive) (Shang et al., 2023). The subcellular localization of the NRAMP family genes was predicted using two online tools.
ProtComp 9.0, available at http://linux1.softberry.com/berry.phtml?topic=protcomppl&group=programs&subgroup=proloc, is a software tool used (Si et al., 2023).
The CELLO server, which can be visited at cello.life.nctu.edu.tw (Hussain et al., 2023; Sajjad et al., 2023).
In the experiments, one-year-old Kandelia obovata seedlings were planted in the mangrove conservation site (109.22° N, 21.42° E) of Golden Bay Mangrove Reserve in Beihai, Guangxi Province. The soil was irrigated with CuCl2 irrigation every six months and was watered with the nearby seawater every morning and evening as part of semi-natural cultivation techniques. Five different concentrations of CuCl2—0, 50, 100, 200, and 400 mg/L—were utilized for the Cu0, Cu50, Cu100, Cu200, and Cu400 treatments, respectively, throughout two years. Cu0 mg/L was used as the starting concentration for the control treatment, which only used local seawater. The soil sample used for this experiment had a background Cu concentration of < 1.0%, which was classified as non-polluted (Liao et al., 2023). After two years of treatment, plant samples were gathered to evaluate the parameters (Hussain et al., 2023).
Total RNA extraction from the leaves was performed using TRIzol (Invitrogen, http://www.invitrogen.com). The ABI PRISM 7500 Real-time PCR Systems, manufactured by Applied Biosystems, were utilized in this study to perform quantitative real-time PCR (qRT-PCR) assays. The 2−∆∆CT approach, as previously outlined (Hussain et al., 2022c; Sun et al., 2022), was employed for data analysis. The actin gene of Kandelia obovata (KoActin) was used as a reference gene using the sequence given by Sun et al., 2022 (forward primer: CAATGCAGCAGTTGAAGGAA, reverse primer: CTGCTGGAAGGAACCAAGAG). The KoNRAMPs gene primers utilized in real-time PCR are listed in Table 1 and were created with the PRIMER 5.0 software (http://www.premierbiosoft.com) (Hussain et al., 2023).
The data was analyzed using Statistix 8.1, an analytical software developed in Tallahassee, FL, USA. A one-way ANOVA was employed to evaluate the data, and the results were presented as the mean and standard deviation (SD) of the three replicates. Five distinct Cu stress plants (Cu0, Cu50, Cu100, Cu200, and Cu400 mg/L) were compared for differences in leaf mean values using an LSD (least significant difference) test at p < 0.05 (Khan et al., 2022). The statistical tool GraphPad Prism version 9.0.0 for Windows, developed by GraphPad Software, located in San Diego, California, USA (https://www.graphpad.com), was used to construct the graphs (Asim et al., 2022).
The total number of NRAMP genes found in the genome of Kandelia obovata is five, which is significantly more than the number of NRAMPs found in other plant species such as Arabidopsis thaliana, peanut (Arachis hypogaea L.), common bean (Phaseolus vulgaris), potato (Solanum tuberosum), soybean (Glycine Max L.), Spirodela polyrhiza, and tea plant (Camellia sinensis). The molecular weight of the NRAMP family exhibited ranging from 49.4 to 139, with an average value of 84.12 kilodaltons (kDa). The protein KoNRAMP5 exhibited the highest isoelectric point (pI) of 7.61, while KoNRAMP displayed the lowest pI of 5.01. The isoelectric point (pI) of the NRAMP family exhibited an average range of 5.01 to 7.61. The hydrophobic nature of five NRAMPs was demonstrated by the range of their grand average hydropathy index (GRAVY) values, which varied from -0.082 to 0.608. Identifying the subcellular localization of NRAMP proteins can enhance the accessibility of comprehending their molecular activity. Five NRAMPs were probably located in the plasma membrane, based on the subcellular localization prediction of NRAMP proteins (Table 2). The NRAMP family exhibited an average amino acid length of 452 to 1272, an aliphatic index of 89.87 to 120.7, and an instability range of 30.07 to 48.94. The MapChart web tool was utilized to map the genomic chromosomal distribution of the discovered NRAMP genes in Kandelia obovata by utilizing their respective chromosomal positions and assigning them to the corresponding chromosomes. The chromosomal locations of the five NRAMP genes, namely KoNRAMP1, KoNRAMP2, KoNRAMP3, KoNRAMP4, and KoNRAMP5, were identified as chromosome 01 (Chr01), chromosome 02 (Chr02), chromosome 05 (Chr05), chromosome 07 (Chr07), and chromosome 18 (Chr18) (Figure 1; Table 2).
Figure 1 The schematic representation displays the distribution of the NRAMP gene on the five chromosomes of Kandelia obovata. The gene’s name is indicated in blue on the right side. The chromosomal regions where the NRAMP genes are located are denoted by black letters. The chromosomal numbers can be observed at the uppermost region of each chromosome. The chromosomal numbers can be found at the uppermost region of each chromosome (Chr).
Aligning all KoNRAMPs, BLAST in NCBI revealed that their amino acid sequences are 55–75% similar (Figure 2). NRAMPs were discovered in three transmembrane domains (TMD) of Kandelia obovata, according to domain analysis (PF01566) (Figure 2). The protein structures of KoNRAMPs were confirmed using the SWISS-MODEL workspace and SOSUI tool, as depicted in Figure 3. The significant conservation seen in all investigated species suggests that the crystal structure of a divalent metal transporter. The NRAMP proteins of Kandelia obovata exhibit secondary structures that are analogous to NRAMP proteins seen in other species, as depicted in Figure 3. These proteins also demonstrate a sequence identity and similarity ranging from 55% to 75%.
Figure 2 Multiple alignments of the amino acid sequence were performed utilizing information from each KoNRAMP transporter. The predicted transmembrane regions are displayed as black boxes. The symbols “*” that appear above the sequence indicate every 10 residues of amino acids.
Figure 3 The 3D and transmembrane structures of KoNRAMP. The SWISS-MODEL software is utilized for the prediction of three-dimensional structural homology models. The SOSUI program validated the transmembrane structures.
An unrooted NJ tree was constructed by aligning five KoNRAMP, six AtNRAMP, five StNRAMP, twelve PtNRAMP, eight VvNRAMP, and eight AcNRAMP from Kandelia obovata (Ko), Arabidopsis thaliana (At), Solanum tuberosum (St), Populus trichocarpa (Pt), Vitis vinifera (Vv), and Aegiceras corniculatum (Ac). This allowed for the phylogenetic relationships among the NRAMP proteins from these five species. Three subgroups (I, II, and SLC) of NRAMP proteins could be identified based on the phylogenetic tree: Subgroup I consisted of 18 NRAMP proteins, which were classified into several species-specific groups. Specifically, this subgroup comprised two KoNRAMP proteins (KoNRAMP2/4), four AtNRAMP proteins (AtNRAMP2/4/5/7), three StNRAMP proteins (StNRAMP1/2/3), three PtNRAMP proteins (PtNRAMP4/9/10), two VvNRAMP proteins (VvNRAMP1/7), and four AcNRAMP proteins (AcNRAMP10/13/16/18). Subgroup II comprised of a total of 26 NRAMP proteins, including one KoNRAMP protein (KoNRAMP5), three AtNRAMP proteins (AtNRAMP1/3/6), one StNRAMP protein (StNRAMP5), four PtNRAMP proteins (PtNRAMP1/2/3/6), five VvNRAMP proteins (VvNRAMP3/4/5/6/8), and 12 AcNRAMP proteins (AcNRAMP1/2/3/4/5/6/7/8/9/12/14/17). The subgroup SLC comprised a total of eleven NRAMP proteins, including two KoNRAMP proteins (KoNRAMP1/3), one StNRAMP protein (StNRAMP4), five PtNRAMP proteins (PtNRAMP5/7/8/11/12), one VvNRAMP protein (VvNRAMP2), and two AcNRAMP proteins (AcNRAMP11/15). Hence, it can be observed from Figure 4 that Subgroup I and SLC had a higher number of NRAMP members compared to Subgroup II.
Figure 4 The most significant likelihood technique was employed to conduct a phylogenetic analysis of NRAMP proteins derived from Kandelia obovata (Ko), Arabidopsis thaliana (At), Solanum tuberosum (St), Populus trichocarpa (Pt), Vitis vinifera (Vv), and Aegiceras corniculatum (Ac). Subgroup I, Subgroup II, and SLC are the three groups of NRAMP proteins; a distinct color denotes each. The red asterisk symbol (*) was used to represent the Kandelia obovata NRAMP proteins.
A phylogenetic tree was constructed utilizing the individual sequences of the NRAMP protein. The NRAMP proteins were categorized into three categories: I, II, and SLC. This classification scheme aligns with the subsequent evolutionary groups that are elaborated upon. The homologous gene pairs exhibiting significant sequence similarities demonstrated strong evolutionary connections and similar exon-intron organizations, as illustrated in Figure 5. In order to further our understanding of the evolutionary dynamics of the NRAMP gene family, an investigation was conducted on the exon-intron arrangements of the NRAMP genes. Subgroup I consists of two constituents from the KoNRAMPs group, accounting for two out of four members. The presence of four exons characterizes each constituent within this subgroup. It is worth noting that the KoNRAMP constituent comprises four introns, while the KoNRAMP2 constituent contains three introns.
Figure 5 Kandelia obovata’s NRAMP family genes were examined for their gene structure and motif makeup. The NRAMP genes of both genomes were classified into two distinct categories according to their evolutionary ties—the gene structure of the NRAMPs. The color green visually represents the UTR regions, while the CDS or exons are depicted in yellow. A black horizontal line indicates the presence of introns. Additionally, the conserved motif structures identified in the NRAMPs are denoted by the letter (Ko). Various colored boxes display distinct motifs. The red asterisk symbol (*) was used to represent the Kandelia obovata NRAMP proteins.
Similarly, just one KoNRAMP5 gene with 13–14 exons and 12–13 introns is present in subgroup II. Within the subgroup SLC, it has been observed that most NRAMP genes had an elongated coding sequence (CDS), accompanied by five comparatively shorter CDSs within the SLC subtribe. Furthermore, these genes have a remarkably conserved structure. Most genes in each of the three categories contained a surprising number of CDSs and introns, suggesting their activities might be very similar.
The protein sequences of NRAMPs were analyzed, identifying 10 conserved motifs (Figure 5). The conserved motifs seen in all NRAMP genes exhibited a range of two to eight; similarly, the KoNRAMP genes displayed motifs ranging from two to eight. The KoNRAMP3 protein has two distinct motifs at positions 6 and 9, whereas KoNRAMP1 and KoNRAMP4 proteins possess five motifs. The protein KoNRAMP5 exhibited a total of six distinct motifs, whereas KoNRAMP2 displayed a larger repertoire with a total of eight motifs. Most KoNRAMP proteins are anticipated to possess motifs one and three, except for KoNRAMP3.
Conversely, motif 4 has been detected in KoNRAMP1/2/5 proteins. Motifs 1 and 3 were identified in all genes except for KoNRAMP3 and StNramp4, while motif 2 was identified in all genes except KoNRAMP1/3 and StNRAMP4. Similarly, motif 4 was observed in all genes except for KoNRAMP3/4.
After looking at the cis-element research, which may provide insights into regulatory gene expression pathways, we looked at the 2500-bp upstream promoter sequences of NRAMP genes. Concerning the NRAMP genes, it is noteworthy that the light-responsiveness gene exhibits the greatest abundance of cis-elements, with a total of 91. Furthermore, the promoter sequences of NRAMP genes were found to contain 53 cis-elements associated with phytohormones, including Abscisic acid (18), Gibberellin (8), Auxin (8), Salicylic acid (3), and MeJA (16). Additionally, three cis-elements related to defense and stress responses, namely low temperature (4), drought (7), and Endosperm expression (2), as well as two cis-elements associated with Zein metabolism (2), one cis-element linked to Cell cycle (1), and nine cis-elements involved in Anaerobic responses were also identified (Figure 6). The observed variability in the response components provides evidence for the regulatory roles of NRAMP genes in a wide range of physiological and biological processes.
Figure 6 The promoters of the KoNRAMP gene have been found to include regulatory elements referred to as CREs. The positional distribution of the predicted CREs on the KoNRAMP promoters is shown by vertical bars. The promoter sequences (2500 bp) of five KoNRAMP genes were analyzed using PlantCARE. In the context of this legend, distinct cis-elements were symbolically represented by various colors.
A collinearity analysis revealed significant orthologs of the NRAMP genes in Kandelia obovata and the other six inherited plant species: Arabidopsis thaliana, Solanum tuberosum, Populus trichocarpa, Vitis vinifera, and Aegiceras corniculatum (Figure 7). In chromosome 01, a gene from Kandelia obovata had syntenic associations with two genes from Populus trichocarpa, as well as with one gene each from Arabidopsis thaliana, Solanum tuberosum, Vitis vinifera, and Aegiceras corniculatum. In contrast, it is seen that within chromosome 02, a specific gene of Kandelia obovata exhibits syntenic relationships with two genes of Arabidopsis thaliana and Vitis vinifera. This gene demonstrates a syntenic link with one gene each from Solanum tuberosum, Populus trichocarpa, and Aegiceras corniculatum.
Figure 7 Synteny analysis of NRAMP genes in the chromosomes of Aegiceras corniculatum, Vitis vinifera, Populus trichocarpa, Solanum tuberosum, Arabidopsis thaliana, and Kandelia obovata was examined. The grey lines in the background show the syntenic NRAMP gene pairs, and the collinear blocks in the genomes of Kandelia obovata and the other five plant species are highlighted by the red lines. The box’s distinct colors represented plant species. The chromosomal number of each species is indicated at the upper and lower ends of each chromosome.
Similar syntenic relationships were seen in chromosome 18, where one gene from Kandelia obovata showed up with two genes from Arabidopsis thaliana, one gene from Populus trichocarpa, and one gene from Aegiceras corniculatum (Figure 7). It is worth mentioning that multiple homologs of Kandelia obovata (referred to as KoNRAMPs) have demonstrated their ability to persistently coexist with Arabidopsis thaliana, Solanum tuberosum, Populus trichocarpa, Vitis vinifera, and Aegiceras corniculatum through a syntenic association. This finding suggests that both segmental repetition and whole-genome duplication played significant roles in the evolutionary development of the KoNRAMPs gene family.
Segmental and tandem duplication promotes the emergence of new gene families and plant genomes. In order to gain a deeper comprehension of the duplication events of the Kandelia obovata NRAMP gene, an examination was conducted on both segmental and tandem duplications within the KoNRAMP gene family. The chromosomal dispersals of five KoNRAMP genes were evaluated. The findings of this study indicate that a single segmental duplication event involving the KoNRAMP2 and KoNRAMP4 gene pairs of chromosomes A02 and A07 was observed, as depicted in Figure 8. It is worth noting that the KoNRAMP gene was not present in the remaining chromosomes. Tandem repeats paralogous genes were not identified in regions A01, A05, and A18, each with a solitary gene irrespective of the chromosome. The results of this study have illustrated the importance of duplication events in the expansion of the KoNRAMP family genes.
Figure 8 Circles represent the distribution of the KoNRAMP gene chromosomes and the interactions between chromosomes. The red and blue lines represent the syntenic NRAMP gene pair, whereas the syntenic blocks in the Kandelia obovata genome are depicted by the grey lines in the background.
In order to further the understanding of the evolutionary constraints on the KoNRAMP gene family, an analysis was conducted to determine the values of Ka (non-synonymous substitution rate), Ks (synonymous substitution rate), and the Ka/Ks ratio for Kandelia obovata. The duplicated KoNRAMP gene pairs displayed a Ka/Ks ratio of 0.86, indicating that the NRAMP family genes in Kandelia obovata may have experienced selection pressure or a discriminatory load during their evolutionary development (Table 3).
The expression profiling of five KoNRAMP genes (KoNRAMP1, KoNRAMP2, KoNRAMP3, KoNRAMP4, and KoNRAMP5) was conducted using qRT-PCR. This analysis was carried out in the presence of five distinct CuCl2 treatments, namely Cu0, Cu50, Cu100, Cu200, and Cu400 mg/L, as depicted in Figure 9. In the present investigation, the expression levels of KoNRAMP1, KoNRAMP4, and KoNRAMP5 were significantly reduced across all five CuCl2 treatments compared to the control group (Cu0). The expression levels of all five genes were shown to be significantly down-regulated in the Cu400 condition compared to the Cu0 condition. This observation can be attributed to the increased KoNRAMPs expression levels in the leaf when Cu availability was limited. However, when Cu was abundant (Cu400), the transcript levels of these genes decreased in comparison to both the Cu0 condition and other levels of Cu. The expression levels of KoNRAMP2 and KoNRAMP3 genes did not show statistical significance when comparing Cu50 with the control group. However, these genes exhibited statistically significant up-regulation in the Cu100 and Cu200 groups compared to the Cu0 group.
Figure 9 Expression of KoNRAMPs in leaves of seedling-stage Kandelia obovata plants under different Cu stress conditions (Cu0, Cu50, Cu100, Cu200, and Cu400 mg/L) as determined by qRT-PCR analysis. There is a statistically significant difference (p < 0.05) seen between the control group and all experimental conditions, as determined by the Least Significant Difference (LSD) test. *p < 0.05, *** means p < 0.001. The vertical axis shows the relative gene expression, and the horizontal axis shows NRAMP1–5 genes.
Numerous NRAMP genes have been found and functionally described as a result of their significant role in the absorption and transport of metal ions (Thomine et al., 2003; Cailliatte et al., 2010; Sasaki et al., 2012; Peris-Peris et al., 2017; Chang et al., 2020). However, Kandelia obovata does not have comprehensive information about this family. The genome of Kandelia obovata was used in this investigation to identify five NRAMP genes that are relevant to reported plant species, including Arabidopsis thaliana (six) (Segond et al., 2009), Camellia sinensis (11) (Li et al., 2021), Spirodela polyrhiza (three) (Chen et al., 2021), Glycine Max L. (13) (Qin et al., 2017), Solanum tuberosum (five) (Tian et al., 2021), Populus trichocarpa (11) (Ma et al., 2023), Phaseolus vulgaris L. (seven) (Ishida et al., 2018), Arachis hypogaea L. (15) (Tan et al., 2023). Based on the findings of previously published investigations, it has been observed that numerous plant species possess a range of three to fifteen NRAMP genes. Our research outcome aligns with a prior investigation in this regard.
Fifteen (15) NRAMP family genes were found in the genome of Arachis hypogaea and subsequently designated as AhNRAMP1-AhNRAMP15. The upregulation of the majority of AhNRAMPs is triggered by iron deficiency in the roots of Arachis hypogaea, and there is a positive correlation between their expression and the accumulation of cadmium. This suggests that AhNRAMPs play a significant role in the interactions between iron and cadmium in Arachis hypogaea (Tan et al., 2023). Thirteen (13) NRAMP family genes have been discovered from Glycine Max L. Among these genes, GmNRAMP1-13 exhibit distinct regulatory patterns in response to shortages in nitrogen, phosphorus, potassium, Fe, and sulfur, as well as toxicities caused by excessive amounts of Fe, Cu, Cd, and Mn. According to the results of the expression study, it has been indicated that GmNRAMP genes exhibit functionality across many tissues and developmental stages (Qin et al., 2017).
A comprehensive set of 11 NRAMP family genes was discovered in Populus trichocarpa and Camellia sinensis genomes. These genes were subsequently designated as PtNRAMP1-PtNRAMP11 and CsNRAMP1-CsNRAMP11. According to the findings of gene expression research, it was observed that the PtNRAMP genes exhibited varying responses to metal stress conditions, such as deficiencies in Fe and Mn, as well as toxicities caused by Fe, Mn, Zn, and Cd (Ma et al., 2023). To evaluate the effects of Pb treatment on roots and leaves, the expression levels of CsNRAMPs were found (Li et al., 2021). A comprehensive analysis has shown the identification of NRAMP family genes in Phaseolus vulgaris L., Arabidopsis thaliana, Solanum tuberosum, and Spirodela polyrhiza. Specifically, 7, 6, 5, and 3 NRAMP family genes have been identified from these respective species. Notably, the products PvNRAMP1-7 have been found to possess transporters involved in metal homeostasis in Phaseolus vulgaris L. (Ishida et al., 2018). The induction of StNRAMPs expression appears to be unique to HMs, suggesting a potential involvement in the transportation and uptake of HMs such as (Cu, Cd, Zn, nickel (Ni), and Pb (Tian et al., 2021). The SpNRAMP gene’s expression level was affected by Cd stress, particularly in the context of Fe or Mn shortage (Chen et al., 2021).
The NRAMP proteins were grouped into three subgroups by phylogenetic analysis, which aligns with recent research that made a similar division among seven P. vulgaris NRAMP proteins (Ishida et al., 2018). A comprehensie set of eleven NRAMP members (PtNRAMP1–11) were discovered in Populus trichocarpa, a widely used woody model plant. These members were further categorized into three groups through phylogenetic analysis (Ma et al., 2023). Additional investigation of the PtNRAMP gene structure revealed that members of closely related subgroups typically exhibited comparable exon-intron arrangements, suggesting a shared evolutionary lineage. Additionally, it was shown that the PtNRAMP genes in the various subgroups had distinct exon-intron architectures. These modest variations might significantly impact the evolutionary process of these genes. Hence, there is speculation on the involvement of distinct gene architectures in disparate tasks (Ma et al., 2023). The structure of genes has undergone changes during evolutionary processes, leading to an increase in the number of exons/introns. Generally, genes with fewer introns go through the editing process and leave the nucleus sooner (Hashemipetroudi et al., 2023; Yaghobi and Heidari, 2023). Based on this, the different gene structures may affect the expression pattern of NRAMP genes.
There was an unequal distribution of the 11 PtNRAMP genes over the six chromosomes. Only one PtNRAMP gene was found on chromosomes 1, 6, and 16, two on chromosome 7, and three on chromosomes 2 and 5. PtNRAMP5, PtNRAMP6, and PtNRAMP7 may have been tandemly duplicated during evolution, as shown by their clustering on chromosome 5 (Ma et al., 2023). The distribution of the five StNRAMP genes is observed throughout five chromosomes, specifically Chr00, Chr02, Chr04, Chr09, and Chr1(Tian et al., 2021). Cis-acting elements distinctly regulate gene transcription and expression in plants (Chen et al., 2021). The promoter regions of NRAMPs were shown to possess several stress response and hormone response components. Since numerous stressors can affect a gene’s expression, NRAMP genes may be involved (Chen et al., 2021). Examining cis-acting elements has indicated that StNRAMP2 possesses more elements responsive to MYB transcription factors. The sensitivity of StNRAMP1 to the five HMs in the stem tissue was negligible, resulting in no statistically significant variation in relative expression. Furthermore, it has been noted that the gene’s 2000 bp upstream region exhibits supplementary elements that are receptive to phytohormones, including methyl jasmonate, gibberellin, salicylic acid, and auxin. Consequently, it is plausible that this gene may have played a role in plant growth and development throughout evolution (Tian et al., 2021). According to studies, many phytohormones, including JA, ABA, and SA, have been linked to the plant’s response to various metal stresses (Ma et al., 2023). Additionally, our investigation revealed the presence of several cis-elements linked with HMs in the promoters of the KoNRAMP gene. These cis-elements might play a role in the transportation and uptake of metallic substances. The findings of this study align with prior research, demonstrating the presence of multiple cis-acting elements associated with hormone responsiveness within the putative promoter regions of Spirodela polyrhiza (Chen et al., 2021), Solanum tuberosum (Tian et al., 2021), Populus trichocarpa (Ma et al., 2023), Phaseolus vulgaris L. (Ishida et al., 2018), and Arachis hypogaea L. NRAMP genes (Tan et al., 2023).
As several earlier experiments have demonstrated, the entire genome, segmental, tandem, and gene duplication events are necessary for the growth and evolution of gene families (Vision et al., 2000; Grassi et al., 2008; Chen et al., 2021). According to the study conducted by Force et al., 1999 it was found that the likelihood of tandem duplicate events was greater when the complementarity level was higher than segmental duplicates. Furthermore, the duplication events involving tandem and segmental duplications were identified as the primary types of duplication (Kong et al., 2007). According to earlier findings, Theobroma cacao exhibited the existence of a single tandem duplicated pair and one pair of segmental duplications (Ullah et al., 2018). Glycine max L. showed six duplicated blocks (Qin et al., 2017), while Oryza sativa L. demonstrated one syntenic block with the paralogous pair (Wang et al., 2019). Additionally, A. thaliana showcased two pairs of segmental duplications (Segond et al., 2009). Nevertheless, the results indicated the absence of tandem duplication in Kandelia obovata. Previous investigations have shown similar findings; no tandem duplication was found in Arachis hypogaea L. (Tan et al., 2023), and S. polyrhiza (Chen et al., 2021). Thus, the number of KoNRAMP genes in Kandelia obovata may be related to whole-genome duplication during allopolyploid speciation.
In order to get an additional understanding of the transcript levels, we conducted a quantitative real-time polymerase chain reaction (qRT-PCR) to assess the expression of KoNRAMP genes in response to various Cu stress conditions. Our findings show that the expression levels of KoNRAMPs varied significantly in response to five distinct CuCl2 treatments, exhibiting upregulation and downregulation. In leaves, the transcript abundance of KoNRAMP1, KoNRAMP4, and KoNRAMP5 decreased in response to CuCl2 treatment. The experimental findings revealed that the expression of all SpNRAMP gene families was downregulated in response to 50 µM Cd treatments (Chen et al., 2021). The expression patterns of StNRAMP exhibited significant variations across different tissues in response to various HM treatments. The expression pattern of StNRAMP2 is comparable under stress induced by Pb and Cu, as evidenced by the peak in relative expression seen at 24 hours (Tian et al., 2021). As Tian et al. (2021) reported, these findings supported the notion that NRAMPs were responsible for the intake and transport of HMs. The results of our study indicate that the expression levels of the KoNRAMP2 and KoNRAMP3 genes were significantly increased in response to Cu concentrations of 100 and 200 mg/L. The results of this study were in agreement with prior research. Specifically, the expression levels of CsNRAMP1, CsNRAMP2, CsNRAMP9, and CsNRAMP10 were shown to be up-regulated in leaves. Additionally, CsNRAMP2 exhibited a highly responsive reaction to Pb treatment, indicating its heightened sensitivity (Li et al., 2021). Under the stress conditions of Cd and Fe, most PtNRAMPs genes exhibited up-regulation in both leaves and roots, except for PtNRAMP3 and PtNRAMP8. In addition, it was shown that PtNRAMP1, PtNRAMP3, PtNRAMP6, PtNRAMP7, and PtNRAMP10 exhibited up-regulation in both leaves and roots in response to Zn and Mn stress, as reported in the study (Ma et al., 2023). The findings of this study align with other research, suggesting that NRAMPs play a crucial role in the absorption and transportation of HMs (Tian et al., 2021). In order to enhance the validation of the functionality of the KoNRAMP genes, it is important to conduct a series of experiments involving gene overexpression and knockdown.
This work identified five KoNRAMP genes by doing a thorough genome-wide analysis of the NRAMP gene family in Kandelia obovata. On the five chromosomes of Kandelia obovata, these KoNRAMP genes were located. The expression patterns of five KoNRAMP genes were validated using qRT-PCR. Comparing the Cu400 condition to the Cu0 condition showed that the expression levels of all five genes were considerably down-regulated. Based on phylogenetic analysis, the KoNRAMP genes were divided into three subgroups (subgroup I, II, SLC). To better understand the evolution of the NRAMP gene family in the Kandelia obovata genome, various analyses were conducted, including domain and 3D structural variation, gene structure, phylogenetic and synteny, chromosomal distributions, motif analysis, subcellular localization, cis-regulatory elements, and expression profiling against CuCl2 stress treatments (Figure 10). Multiple promoter investigations have revealed the presence of many stress and hormone response elements, which play a crucial role in the response to Cu and other metal-induced stress. In general, this research offers significant insights for future functional investigations about the biological functions of NRAMP genes in Kandelia obovata.
Figure 10 An overview of the thorough investigation of the NRAMP gene family in Kandelia obovata. The figure was created using the BioRender (https://www.biorender.com/) tool, which was modified from Sajjad et al. (2023). Different colors were used to illustrate the sketches from the various analyses. The arrow symbol represents the several analyses conducted to comprehend the characterization of the KoNRMAP genes.
The original contributions presented in the study are included in the article/supplementary material. Further inquiries can be directed to the corresponding author.
QH: Conceptualization, Writing – original draft, Writing – review & editing. TY: Formal analysis, Software, Methodology, Writing – review & editing. CS: Funding acquisition, Supervision, Writing – review & editing. SL: Formal analysis, Methodology, Writing – review & editing. AK: Writing – review & editing. JN: Writing – review & editing. A-ZM: Writing – review & editing. ME: Writing – review & editing.
The author(s) declare financial support was received for the research, authorship, and/or publication of this article. This research was funded by the National Key R&D Program of China (2018YFA0902500), the National Natural Science Foundation of China (42376219, 41706137), the CAS Key Laboratory of Science and Technology on Operational Oceanography (No. OOST2021- 07), the Graduate Education Innovation Project (Guangdong Province, 2020JGXM094).
We thank the Instrument Analysis Center of Shenzhen University. We are also grateful for the support from the public service platform of instruments and equipment at the College of Life Sciences and Oceanography at Shenzhen University. The authors extend their appreciation to the Researchers Supporting Project number (RSPD2023R941), King Saud University, Riyadh, Saudi Arabia.
The authors declare that the research was conducted in the absence of any commercial or financial relationships that could be construed as a potential conflict of interest.
All claims expressed in this article are solely those of the authors and do not necessarily represent those of their affiliated organizations, or those of the publisher, the editors and the reviewers. Any product that may be evaluated in this article, or claim that may be made by its manufacturer, is not guaranteed or endorsed by the publisher.
Asim, M., Hussain, Q., Wang, X., Sun, Y., Liu, H., Khan, R., et al. (2022). Mathematical modeling reveals that sucrose regulates leaf senescence via dynamic sugar signaling pathways. Int. J. Mol. Sci. 23, 6498. doi: 10.3390/ijms23126498
Bodin, N., N’Gom-Kâ, R., Kâ, S., Thiaw, O. T., Tito de Morais, L., Le Loc’h, F., et al. (2013). Assessment of trace metal contamination in mangrove ecosystems from Senegal, West Africa. Chemosphere 90, 150–157. doi: 10.1016/j.chemosphere.2012.06.019
Burkhead, J. L., Gogolin Reynolds, K. A., Abdel-Ghany, S. E., Cohu, C. M., Pilon, M. (2009). Copper homeostasis. New Phytol. 182, 799–816. doi: 10.1111/j.1469-8137.2009.02846.x
Cailliatte, R., Schikora, A., Briat, J. F., Mari, S., Curie, C. (2010). High-affinity manganese uptake by the metal transporter nramp1 is essential for Arabidopsis growth in low manganese conditions. Plant Cell 22, 904–917. doi: 10.1105/tpc.109.073023
Cellier, K., Belouchi, A., Gros, P. (1996). Resistance to intracellular infections: Comparative genomic analysis of Nramp. Trends Genet. 12, 201–204. doi: 10.1016/0168-9525(96)30042-5
Cellier, M., Privé, G., Belouchi, A., Kwan, T., Rodrigues, V., Chia, W., et al. (1995). Nramp defines a family of membrane proteins. Proc. Natl. Acad. Sci. U. S. A. 92, 10089–10093. doi: 10.1073/pnas.92.22.10089
Chang, J. D., Huang, S., Yamaji, N., Zhang, W., Ma, J. F., Zhao, F. J. (2020). OsNRAMP1 transporter contributes to cadmium and manganese uptake in rice. Plant Cell Environ. 43, 2476–2491. doi: 10.1111/pce.13843
Chen, Y., Zhao, X., Li, G., Kumar, S., Sun, Z., Li, Y., et al. (2021). Genome-wide identification of the Nramp gene family in spirodela polyrhiza and expression analysis under cadmium stress. Int. J. Mol. Sci. 22, 6414. doi: 10.3390/ijms22126414
Cheng, S., Tam, N. F. Y., Li, R., Shen, X., Niu, Z., Chai, M., et al. (2017). Temporal variations in physiological responses of Kandelia obovata seedlings exposed to multiple heavy metals. Mar. pollut. Bull. 124, 1089–1095. doi: 10.1016/j.marpolbul.2017.03.060
Fageria, N. K. (2001). Adequate and toxic levels of copper and manganese in upland rice, common bean, corn, soybean, and wheat grown on an oxisol. Commun. Soil Sci. Plant Anal. 32, 1659–1676. doi: 10.1081/CSS-100104220
Force, A., Lynch, M., Pickett, F. B., Amores, A., Yan, Y., Postlethwait, J. (1999). Preservation of duplicate genes by complementary, degenerative mutations. Genetics 151, 1531–1545. doi: 10.1093/genetics/151.4.1531
Giri, C., Ochieng, E., Tieszen, L. L., Zhu, Z., Singh, A., Loveland, T., et al. (2011). Status and distribution of mangrove forests of the world using earth observation satellite data. Glob. Ecol. Biogeogr. 20, 154–159. doi: 10.1111/j.1466-8238.2010.00584.x
Grassi, A., De Lanave, C., Saccone, C. (2008). Genome duplication and gene-family evolution : The case of three OXPHOS gene families. Gene 421, 1–6. doi: 10.1016/j.gene.2008.05.011
Hashemipetroudi, S. H., Arab, M., Heidari, P., Kuhlmann, M. (2023). Genome-wide analysis of the laccase (LAC) gene family in Aeluropus littoralis : A focus on identification, evolution and expression patterns in response to abiotic stresses and ABA treatment. Front. Plant Sci. 14, 1112354. doi: 10.3389/fpls.2023.1112354
Hu, M. J., Sun, W. H., Tsai, W. C., Xiang, S., Lai, X. K., Chen, D. Q., et al. (2020). Chromosome-scale assembly of the Kandelia obovata genome. Hortic. Res. 7, 75. doi: 10.1038/s41438-020-0300-x
Huang, G. Y., Wang, Y. S. (2010). Physiological and biochemical responses in the leaves of two mangrove plant seedlings (Kandelia candel and Bruguiera gymnorrhiza) exposed to multiple heavy metals. J. Hazard. Mater. 182, 848–854. doi: 10.1016/j.jhazmat.2010.06.121
Hussain, Q., Ye, T., Nkoh, N., Zhou, Q., Shang, C. (2023). Genome-wide identification and expression analysis of the copper transporter (COPT/ctr) gene family in kandelia obovata, a typical mangrove plant. Int. J. Mol. Sci. 24, 15579. doi: 10.3390/cimb44110381
Hussain, Q., Zhan, J., Liang, H., Wang, X., Liu, G., Shi, J., et al. (2022c). Key genes and mechanisms underlying natural variation of silique length in oilseed rape (Brassica napus L .) germplasm. Crop J. 10, 617–626. doi: 10.1016/j.cj.2021.08.010
Hussain, Q., Zheng, M., Chang, W., Ashraf, M. F., Khan, R., Asim, M., et al. (2022a). Genome-wide identification and expression analysis of snRK2 gene family in dormant vegetative buds of liriodendron chinense in response to abscisic acid, chilling, and photoperiod. Genes (Basel). 13, 1–19. doi: 10.3390/genes13081305
Hussain, Q., Zheng, M., Furqan, M., Khan, R., Yasir, M., Farooq, S., et al. (2022b). Scientia Horticulturae Genome-wide identification , characterization and expression analysis of the ABA receptor PYL gene family in response to ABA , photoperiod , and chilling in vegetative buds of Liriodendron chinense. Sci. Hortic. (Amsterdam). 303, 111200. doi: 10.1016/j.scienta.2022.111200
Ishida, J. K., Caldas, D. G. G., Oliveira, L. R., Frederici, G. C., Leite, L. M. P. (2018). and mui, T Genome-wide characterization of the NRAMP gene family in Phaseolus vulgaris provides insights into functional implications during common bean development. S. Genet. Mol. Biol. 41, 820–833. doi: 10.1590/1678-4685-gmb-2017-0272
Khan, R., Ma, X., Hussain, Q., Asim, M., Iqbal, A., Ren, X., et al. (2022). Application of 2,4-epibrassinolide improves drought tolerance in tobacco through physiological and biochemical mechanisms. Biology. 11, 1192. doi: 10.3390/biology11081192
Kong, H., Landherr, L. L., Frohlich, M. W., Leebens-Mack, J., Ma, H., DePamphilis, C. W. (2007). Patterns of gene duplication in the plant SKP1 gene family in angiosperms: Evidence for multiple mechanisms of rapid gene birth. Plant J. 50, 873–885. doi: 10.1111/j.1365-313X.2007.03097.x
Kravchenko, Y., Kursitys, I., Kravchenko, D. (2018). Architecture and method of integrating information and knowledge on the basis of the ontological structure. Adv. Intell. Syst. Comput. 658, 93–103. doi: 10.1007/978-3-319-67349-3_8
Li, J., Duan, Y., Han, Z., Shang, X., Zhang, K., Zou, Z., et al. (2021). Genome-wide identification and expression analysis of the NRAMP family genes in tea plant (Camellia sinensis). Plants 10, 1055. doi: 10.3390/plants10061055
Li, M. S., Lee, S. Y. (1997). Mangroves of China: A brief review. For. Ecol. Manage. 96, 241–259. doi: 10.1016/S0378-1127(97)00054-6
Li, R., Li, R., Chai, M., Shen, X., Xu, H., Qiu, G. (2015). Heavy metal contamination and ecological risk in Futian mangrove forest sediment in Shenzhen Bay, South China. Mar. pollut. Bull. 101, 448–456. doi: 10.1016/j.marpolbul.2015.09.048
Liao, J., Xu, Y., Zhang, Z., Zeng, L., Qiao, Y., Guo, Z., et al. (2023). Effect of Cu addition on sedimentary bacterial community structure and heavy metal resistance gene abundance in mangrove wetlands. Front. Mar. Sci. 10. doi: 10.3389/fmars.2023.1157905
Liu, W., Huo, C., He, L., Ji, X., Yu, T., Yuan, J., et al. (2022). The NtNRAMP1 transporter is involved in cadmium and iron transport in tobacco (Nicotiana tabacum). Plant Physiol. Biochem. 173, 59–67. doi: 10.1016/j.plaphy.2022.01.024
Ma, X., Yang, H., Bu, Y., Zhang, Y., Sun, N., Wu, X., et al. (2023). Genome-wide identification of the NRAMP gene family in Populus trichocarpa and their function as heavy metal transporters. Ecotoxicol. Environ. Saf. 261, 115110. doi: 10.1016/j.ecoenv.2023.115110
Mourato, M. P., Martins, L. L., Cuypers, A. (2009). Effect of copper on antioxidant enzyme activities and mineral nutrition of white lupin plants grown in nutrient solution. J. Plant Nutr. 32, 1882–1900. doi: 10.1080/01904160903242375
Nevo, Y., Nelson, N. (2006). The NRAMP family of metal-ion transporters. Biochim. Biophys. Acta - Mol. Cell Res. 1763, 609–620. doi: 10.1016/j.bbamcr.2006.05.007
Peris-Peris, C., Serra-Cardona, A., Śanchez-Sanuy, F., Campo, S., Ariño, J., Segundo, B. S. (2017). Two NRAMP6 isoforms function as iron and manganese transporters and contribute to disease resistance in rice. Mol. Plant-Microbe Interact. 30, 385–398. doi: 10.1094/MPMI-01-17-0005-R
Qin, L., Han, P., Chen, L., Walk, T. C., Li, Y., Hu, X., et al. (2017). Genome-wide identification and expression analysis of NRAMP family genes in soybean (Glycine max L.). Front. Plant Sci. 8. doi: 10.3389/fpls.2017.01436
Qiu, Y. W., Yu, K. F., Zhang, G., wang, W. X. (2011). Accumulation and partitioning of seven trace metals in mangroves and sediment cores from three estuarine wetlands of Hainan Island, China. J. Hazard. Mater. 190, 631–638. doi: 10.1016/j.jhazmat.2011.03.091
Sajjad, M., Ahmad, A., Riaz, M. W., Hussain, Q., Yasir, M., Lu, M. (2023). Recent genome resequencing paraded COBRA-Like gene family roles in abiotic stress and wood formation in Poplar. Front. Plant Sci. 14. doi: 10.3389/fpls.2023.1242836
Sasaki, A., Yamaji, N., Yokosho, K., Ma, J. F. (2012). Nramp5 is a major transporter responsible for manganese and cadmium uptake in rice. Plant Cell 24, 2155–2167. doi: 10.1105/tpc.112.096925
Segond, D., Dellagi, A., Lanquar, V., Rigault, M., Patrit, O., Thomine, S., et al. (2009). NRAMP genes function in Arabidopsis thaliana resistance to Erwinia chrysanthemi infection. Plant J. 58, 195–207. doi: 10.1111/j.1365-313X.2008.03775.x
Shang, C., Ye, T., Zhou, Q., Chen, P., Li, X., Li, W., et al. (2023). Genome-wide identification and bioinformatics analyses of host defense peptides snakin/GASA in mangrove plants. Genes. 14, 923. doi: 10.3390/genes14040923
Shen, X., Li, R., Chai, M., Cheng, S., Tam, N. F. Y., Han, J. (2021). Does combined heavy metal stress enhance iron plaque formation and heavy metal bioaccumulation in Kandelia obovata? Environ. Exp. Bot. 186, 104463. doi: 10.1016/j.envexpbot.2021.104463
Sheue, C. R., Liu, H. Y., Yong, J. W. H. (2003). Kandelia obovata (Rhizophoraceae), a new mangrove species from Eastern Asia. Taxon 52, 287–294. doi: 10.2307/3647398
Si, X., Lyu, S., Hussain, Q., Ye, H., Huang, C., Li, Y., et al. (2023). Analysis of Delta (9) fatty acid desaturase gene family and their role in oleic acid accumulation in Carya cathayensis kernel. Front. Plant Sci. 14. doi: 10.3389/fpls.2023.1193063
Sun, M. M., Liu, X., Huang, X. J., Yang, J. J., Qin, P. T., Zhou, H., et al. (2022). Genome-wide identification and expression analysis of the NAC gene family in kandelia obovata, a typical mangrove plant. Curr. Issues Mol. Biol. 44, 5622–5637. doi: 10.3390/cimb44110381
Tan, Z., Li, J., Guan, J., Wang, C., Zhang, Z., Shi, G. (2023). Genome-wide identification and expression analysis reveals roles of the NRAMP gene family in iron/cadmium interactions in peanut. Int. J. Mol. Sci. 24, 1713. doi: 10.3390/ijms24021713
Thomine, S., Lelièvre, F., Debarbieux, E., Schroeder, J. I., Barbier-Brygoo, H. (2003). AtNRAMP3, a multispecific vacuolar metal transporter involved in plant responses to iron deficiency. Plant J. 34, 685–695. doi: 10.1046/j.1365-313X.2003.01760.x
Tian, W., He, G., Qin, L., Li, D., Meng, L., Huang, Y., et al. (2021). Genome-wide analysis of the NRAMP gene family in potato (Solanum tuberosum): Identification, expression analysis and response to five heavy metals stress. Ecotoxicol. Environ. Saf. 208, 111661. doi: 10.1016/j.ecoenv.2020.111661
Ullah, I., Wang, Y., Eide, D. J., Dunwell, J. M. (2018). Evolution, and functional analysis of Natural Resistance-Associated Macrophage Proteins (NRAMPs) from Theobroma cacao and their role in cadmium accumulation. Sci. Rep. 8, 14412. doi: 10.1038/s41598-018-32819-y
Vidal, S. M., Malo, D., Vogan, K., Skamene, E., Gros, P. (1993). Natural resistance to infection with intracellular parasites: Isolation of a candidate for Bcg. Cell 73, 469–485. doi: 10.1016/0092-8674(93)90135-D
Vision, T. J., Brown, D. G., Tanksley, S. D. (2000). The origins of genomic duplications in arabidopsis. Science 80. 290, 2114–2117. doi: 10.1126/science.290.5499.2114
Wang, J., Li, Y., Fu, Y., Xie, H., Song, S., Qiu, M., et al. (2019). Mutation at Different Sites of Metal Transporter Gene OsNramp5 Affects Cd Accumulation and Related Agronomic Traits in Rice (Oryza sativa L.). Front. Plant Sci. 10. doi: 10.3389/fpls.2019.01081
Weng, B., Huang, Y., Liu, J., Lu, H., Yan, C. (2014). Alleviated toxicity of cadmium by the rhizosphere of kandelia obovata (S., L.) Yong. Bull. Environ. Contam. Toxicol. 93, 603–610. doi: 10.1007/s00128-014-1372-9
Weng, B., Xie, X., Weiss, D. J., Liu, J., Lu, H., Yan, C. (2012). Kandelia obovata (S., L.) Yong tolerance mechanisms to Cadmium: Subcellular distribution, chemical forms and thiol pools. Mar. pollut. Bull. 64, 2453–2460. doi: 10.1016/j.marpolbul.2012.07.047
Wu, D., He, G., Tian, W., Saleem, M., Li, D., Huang, Y., et al. (2021). OPT gene family analysis of potato (Solanum tuberosum) responding to heavy metal stress: Comparative omics and co-expression networks revealed the underlying core templates and specific response patterns. Int. J. Biol. Macromol. 188, 892–903. doi: 10.1016/j.ijbiomac.2021.07.183
Yaghobi, M., Heidari, P. (2023). Genome-wide analysis of aquaporin gene family in triticum turgidum and its expression profile in response to salt stress. Genes (Basel). 14, 202. doi: 10.3390/genes14010202
Yang, J., Ling, C., Liu, Y., Zhang, H., Hussain, Q., Lyu, S., et al. (2022). Genome-wide expression profiling analysis of kiwifruit GolS and RFS genes and identification of AcRFS4 function in raffinose accumulation. Int. J. Mol. Sci. 23, 8836. doi: 10.3390/ijms23168836
Yang, Y., Zhang, Y., Chen, Y., Gul, J., Zhang, J., Liu, Q., et al. (2019). Complete chloroplast genome sequence of the mangrove species Kandelia obovata and comparative analyses with related species. PeerJ 7, e7713. doi: 10.7717/peerj.7713
Keywords: Kandelia obovata, NRAMP gene family, copper stress, phylogenetic analysis, gene expression profiling
Citation: Hussain Q, Ye T, Shang C, Li S, Khan A, Nkoh JN, Mustafa AE-ZMA and Elshikh MS (2024) NRAMP gene family in Kandelia obovata: genome-wide identification, expression analysis, and response to five different copper stress conditions. Front. Plant Sci. 14:1318383. doi: 10.3389/fpls.2023.1318383
Received: 11 October 2023; Accepted: 14 December 2023;
Published: 04 January 2024.
Edited by:
Eleni Tani, Agricultural University of Athens, GreeceReviewed by:
Panagiotis Madesis, University of Thessaly, GreeceCopyright © 2024 Hussain, Ye, Shang, Li, Khan, Nkoh, Mustafa and Elshikh. This is an open-access article distributed under the terms of the Creative Commons Attribution License (CC BY). The use, distribution or reproduction in other forums is permitted, provided the original author(s) and the copyright owner(s) are credited and that the original publication in this journal is cited, in accordance with accepted academic practice. No use, distribution or reproduction is permitted which does not comply with these terms.
*Correspondence: Chenjing Shang, Y2pzaGFuZ0BzenUuZWR1LmNu
†ORCID: Quaid Hussain, orcid.org/0000-0001-6340-8482
Disclaimer: All claims expressed in this article are solely those of the authors and do not necessarily represent those of their affiliated organizations, or those of the publisher, the editors and the reviewers. Any product that may be evaluated in this article or claim that may be made by its manufacturer is not guaranteed or endorsed by the publisher.
Research integrity at Frontiers
Learn more about the work of our research integrity team to safeguard the quality of each article we publish.