- 1Faculty of Life Science and Technology, Kunming University of Science and Technology, Kunming, Yunnan, China
- 2Engineering Research Center for Replacement Technology of Feed Antibiotics of Yunnan College, Kunming, Yunnan, China
- 3Yunnan Key Laboratory of Gastrodia and Fungal Symbiotic Biology, Zhaotong University, Zhaotong, Yunnan, China
- 4Yunnan Senhao Fungi Industry Co., Ltd, Zhaotong, Yunnan, China
Gastrodia elata Blume, a fully mycoheterotrophic perennial plant of the family Orchidaceae, is a traditional Chinese herb with medicinal and edible value. Interestingly, G. elata requires symbiotic relationships with Mycena and Armillaria strains for seed germination and plant growth, respectively. However, there is no comprehensive summary of the symbiotic mechanism between fungi and G. elata. Here, the colonization and digestion of hyphae, the bidirectional exchange of nutrients, the adaptation of fungi and G. elata to symbiosis, and the role of microorganisms and secondary metabolites in the symbiotic relationship between fungi and G. elata are summarized. We comprehensively and deeply analyzed the mechanism of symbiosis between G. elata and fungi from three perspectives: morphology, nutrition, and molecules. The aim of this review was to enrich the understanding of the mutualistic symbiosis mechanisms between plants and fungi and lay a theoretical foundation for the ecological cultivation of G. elata.
1 Introduction
Orchidaceae is one of the largest plant families, comprising 750 genera and approximately 27 000 species (Dressler, 1981; Dressler, 1993; Chase et al., 2015). Orchids can be divided into three categories according to their different physiological characteristics. Fully photoautotrophic orchids obtain all necessary carbon from photosynthesis (Cameron et al., 2006). Partially mycoheterotrophic orchids require mycorrhizal fungi to stimulate seed germination and seedling growth and then develop green leaves and photosynthesize (Arditti, 1967). They obtain carbon from both fungi and photosynthesis (Rasmussen and Rasmussen, 2009). Fully mycoheterotrophic orchids are achlorophyllous and obtain their entire carbon supply from their associated mycorrhizal fungi (Leake, 1994). Over 99% of orchids live in nature as mycoheterotrophs (Merckx, 2013), of which more than 200 achlorophyllous orchid species from at least 25 lineages are full mycoheterotrophs (Leake, 1994; Merckx, 2013), including Gastrodia elata Blume. G. elata, known as “Tianma” in China, is a precious herbal medicine with high medicinal and nutritional value (Zheng et al., 2022) and is regarded as one of the most important medicinal herbs in Oriental countries (Sun et al., 2023).
G. elata, as a rootless, leafless, achlorophyllous, and fully mycoheterotrophic orchid, cannot produce nutrients by photosynthesis and can only survive symbiotically with fungi (Ho et al., 2021; Shan et al., 2021). It requires symbiotic interactions with Mycena and Armillaria strains to obtain nutrients for its complex life cycle. The seeds of G. elata are dust-like and lack nutritional reserves, which makes seed germination under natural conditions entirely dependent on Mycena (Park and Lee, 2013a). Mycena strains are responsible for the nutrient supply during seed germination, protocorm growth, and differentiation in the early stages of G. elata growth (Xu and Guo, 1989; Kim et al., 2006). Armillaria strains eventually replace Mycena strains as new symbionts of G. elata for tuber expansion, flowering, and fruit setting (Zhang and Li, 1980; Xu and Guo, 1989; Guo and Wang, 2001; Sekizaki et al., 2008; Park and Lee, 2013a). Different strains, as well as the growth rate and hyphal activity of symbiotic fungi, directly influence the quality and yield of G. elata (Guo and Wang, 2001; Wang et al., 2001a; Sun and Chen, 2003).
The coevolution of plants and fungi has been ongoing for over 400 million years, resulting in four main mycorrhizal types: ectomycorrhizas, arbuscular mycorrhizas, orchid mycorrhizas and ericoid mycorrhizas (Shi et al., 2023). The symbiosis between G. elata and fungi is one of the most unique mycorrhizas in orchids. Substantial knowledge on the interaction between G. elata and fungi, including structural and ultrastructural changes, nutrient transport, signal exchange, and genetic differences, has been gained through previous studies (Liu, 1981; Xu and Guo, 1991; Wang et al., 1997; Xu et al., 2001; Yuan et al., 2018; Cai et al., 2023). Different fungal strains affect the growth and quality of G. elata (Cha and Igarashi, 1995; Guo and Wang, 2001; Sekizaki et al., 2008; Guo et al., 2016), and fungal hyphae colonize G. elata by forming densely coiled structures called pelotons (Wang et al., 1997). The hyphae are digested to provide nutrients for G. elata and obtain nutrients from G. elata cells (Lan et al., 1994; Genre et al., 2020). Strigolactone was discovered to be an important signal that promotes the symbiotic relationship between G. elata and Armillaria (Yuan et al., 2018). The genomes of G. elata and Armillaria have recently been successively released, providing molecular evidence for their symbiosis (Xu et al., 2021; Cai et al., 2023).
In this review, we focus on the roles of Mycena and Armillaria in the life cycle of G. elata from the cellular scale to the ecosystem scale. Finally, the current understanding of the morphological, nutrient exchange, and molecular mechanisms underlying these symbiotic relationships is presented. This serves as both a theoretical guide for the planting and production of G. elata and a reference for the study of the symbiotic relationship between orchid mycorrhizae.
2 Overview of G. elata and its applications
2.1 Classification of G. elata
There are over 100 species in the genus Gastrodia (Orchidaceae), distributed in East Asia, Southeast Asia, and Oceania, with 36 species in China (Zhou et al., 2021b; Plant Plus of China, 2023; Plants of the World Online, 2023) (Table 1). Among them, G. elata is the most widely cultivated in China. There are six forms of G. elata: G. elata Bl. f. glauca, G. elata Bl. f. viridis, G. elata Bl. f. flavida, G. elata Bl. f. elata, G. elata Bl. f. pilifera, and G. elata Bl. f. alba (Zhou and Chen, 1983). Among them, G. elata Bl. f. glauca, G. elata Bl. f. viridis, G. elata Bl. f. flavida, G. elata Bl. f. elata are the four major forms that have been domesticated and cultivated. They have different inflorescence colors and tuber shapes. For example, the inflorescence colors of G. elata Bl. f. glauca, G. elata Bl. f. viridis, G. elata Bl. f. flavida, and G. elata Bl. f. elata are dark, green, yellow, and red, respectively. In these subspecies, the mature tubers of G. elata Bl. f. glauca are the largest and have the highest contents of gastrodin (Wang et al., 2019).
However, wild G. elata is considered to possess higher medicinal value and is more popular and expensive than cultivated G. elata. As a result, wild G. elata is being overexploited and has been listed as a vulnerable species by the International Union for Conservation of Nature (IUCN) (Tsai et al., 2014; IUCN Red List, 2023). In China, G. elata has also been included in the second-grade protected plants according to the “List of National Key Protected Wild Plants (the second batch)” (List of National Key Protected Wild Plants, 2023).
2.2 The application value of G. elata
G. elata has many pharmacological effects (Wang et al., 2021b; Zhou et al., 2021a) and contains over 200 bioactive components and plant secondary metabolites, such as gastrodin (4-hydroxy methyl phenyl-β-D-glucopyranoside), gastrodigenin (p-hydroxybenzyl alcohol), p-hydroxybenzaldehyde, vanillin (4-hydroxy-3-methoxybenzaldehyde), parishin, G. elata polysaccharides, amino acids, and other compounds, which have been demonstrated to be the main components associated with the pharmacological activity of G. elata (Yu et al., 2005; Kim et al., 2007; Chen et al., 2011b). Among these, gastrodin and gastrodigenin are regarded as phytochemical indicators of G. elata in the Chinese pharmacopeia and are used in G. elata quality control (Sun et al., 2023).
G. elata has historically been used to treat headaches, vertigo, epilepsy, dizziness, paralysis, rheumatism, etc. (Kim et al., 2007). These effects were documented in Shennong’s Classic of Materia Medica (Shennong Bencaojing), which dates back to approximately 2000 years ago. Modern pharmacology research has shown that the tuber of G. elata has neuroprotective (Lu et al., 2022), anti-inflammatory, antidiabetic (Yang et al., 2018), antioxidative, antiepileptic, anticonvulsive, antipsychotic, anxiolytic, antidepressant (Gao et al., 2023; Huang et al., 2023), circulatory system modulating, memory-enhancing (Chen et al., 2011a; Chen et al., 2011c), cardiovascular disease ameliorating (Chen and Sheen, 2011), and other effects. Liu et al. (2018) summarized the mechanism by which G. elata acts on neurological diseases and psychiatric disorders, including modulating neurotransmitters, antioxidation, anti-inflammation, anti-apoptosis, suppressing microglial activation, regulating mitochondrial cascades, and upregulating neurotrophins. The efficacy of G. elata in treating cardiovascular diseases is mediated by its multitarget pharmacological properties, including reducing inflammation, inhibiting apoptosis, regulating autophagy, improving metabolism, inhibiting oxidative stress, and modulating the gut microbiota (Sun et al., 2023).
2.3 The life cycle of G. elata
Because of the complexity of the G. elata life cycle, vegetative growth and reproduction have long been a subject of biological speculation. Scientists did not know how vital symbiotic fungi were for G. elata growth until they fully comprehended the entire embryonic cycle (Zhou, 1981). The whole life cycle of G. elata, from seed germination to flowering plants, can take nearly three years and includes five stages: seed germination, protocorm growth and development, the first asexual reproduction to the formation of an immature tuber, the second asexual reproduction to the formation of a mature tuber, and the bolting, flowering and seed setting of the mature tuber (Figure 1). Among them, the first four stages are called the nutritional growth period of G. elata, and the last stage is the reproductive growth period. Generally, G. elata seeds are mixed with the Mycena strain and sown from June to August of the first year (Zhou, 1981; Xu et al., 1989). The seed coat ruptures as the embryo continues to grow during germination, releasing an oval-shaped tissue known as the protocorm. Protocorms progressively begin to form after approximately 20 days (Xu et al., 1989). The protocorm goes through cell division and tissue development, and at the top, a slender bud (some also produce branches) emerges. The meristem at the apex of the slender buds and branches continually expands, forming many bulbs with a diameter of approximately 2 mm. This form of G. elata is called the vegetative propagation corm (Zhou, 1981). The first asexual reproduction of vegetative propagation corms is initiated by exploiting Armillaria. When the bulb becomes a long strip with a diameter greater than 1 cm, it is considered an immature tuber. Immature tubers are tiny tubers of G. elata that cannot grow scapes and can be used for asexual reproduction (Xu et al., 1989); furthermore, G. elata spends the winter of that year as immature tubers. In the spring of the next year (approximately April), the immature tuber ends its dormancy for the second asexual reproduction. At this point, the immature tuber is similar to a mother tuber, developing a new tuber at its front end, which will form a mature tuber in autumn (Figure 2B). Immature tubers are sacrificed because their nutrients are exhausted by mature tubers. The flesh of mature tubers is thick and spherical, with an 8-20 cm body length. Notably, a protocorm can form multiple immature tubers, while an immature tuber can only produce one mature tuber. In the spring of the third year, the mature tubers end dormancy. Ultimately, a scape emerges from the mature tuber, and in summer, a flower develops from the scape to produce seeds. The nutrients for reproductive growth come entirely from mature tubers. The stored nutrients complete the whole process from bolting to seed maturation (Hsieh et al., 2022). More than 80% of the whole life cycle of G. elata is spent underground as a tuber (Yuan et al., 2018). Only the scape is exposed above the ground, and sunlight helps bees to carry out pollination (Sugiura, 2017). This is why wild G. elata is hard to find.
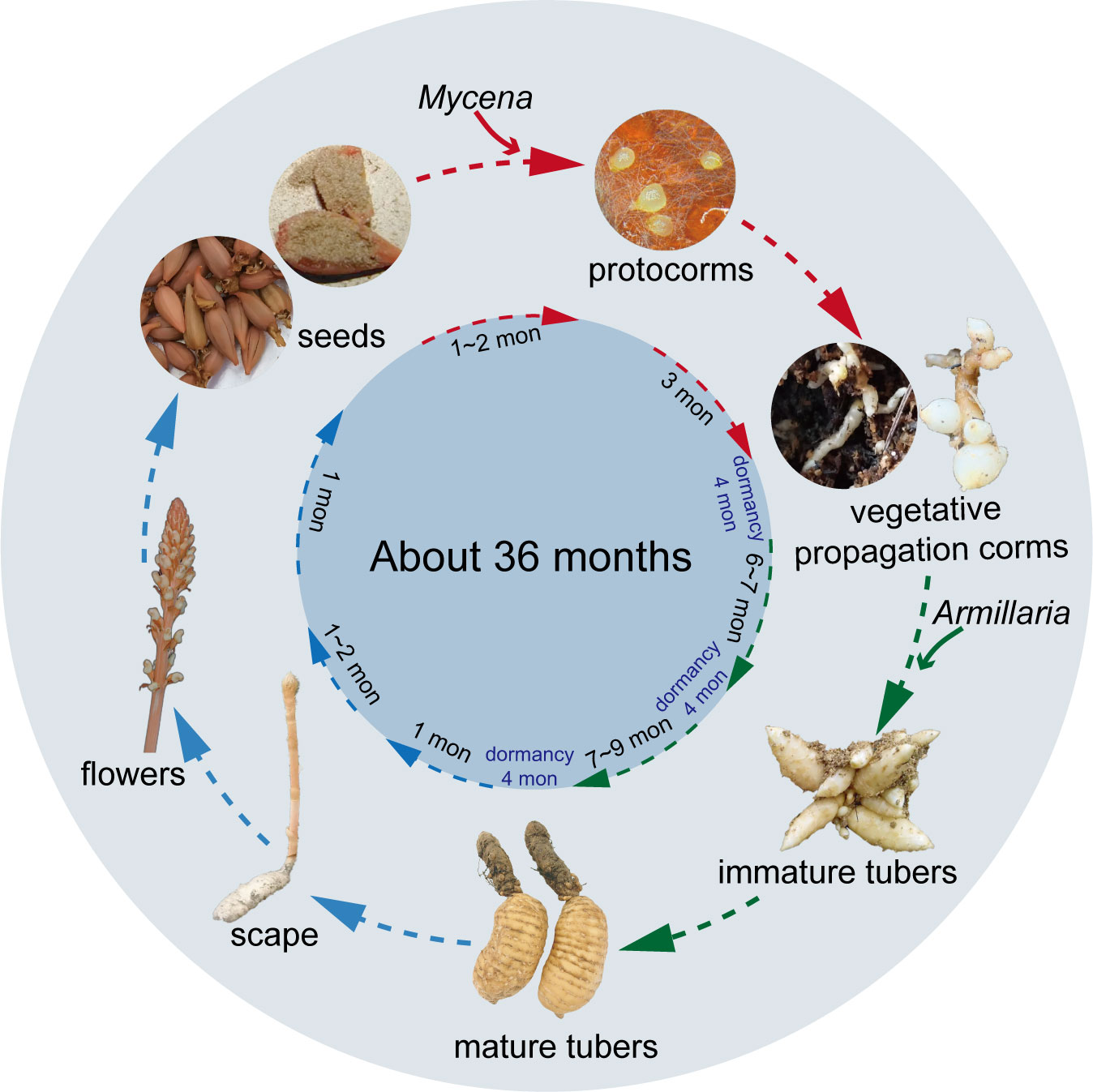
Figure 1 The life cycle of Gastrodia elata. Mycena is essential for the stage denoted by the red arrowhead, Armillaria is necessary for the stage depicted by the green shear head, and no fungi are required for the stage indicated by the blue shear head.
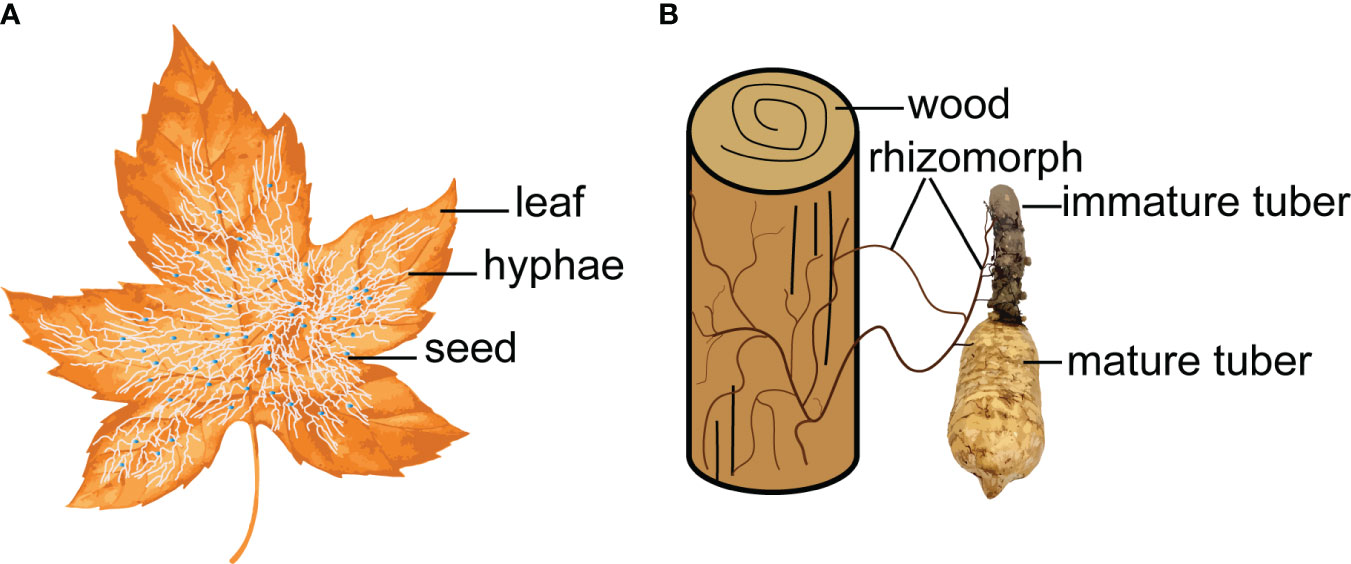
Figure 2 Symbiotic fungi act as a bridge to connect the nutrient exchange between leaf/wood and G. elata. (A), Mycena. (B), Armillaria.
Currently, successful artificial cultivation and industrialization of G. elata can be achieved, primarily by mimicking wild cultivation beneath the forest floor. This lays the industrial foundation for the application of traditional Chinese medicine. However, there are many uncertain factors in the long life cycle, such as climate and wild animals, which increase the difficulty of cultivating G. elata. Recently, some scientists have tried to make the process less time-consuming by a tissue culture approach (Hsieh et al., 2022). During the life cycle, G. elata should be dormant for at least one winter; otherwise, the size and yield of the mature tubers will decrease. However, the mechanism is still unknown. We may be able to reduce the planting time of G. elata in the future by shortening its dormancy time.
3 Classification and function of symbiotic fungi
3.1 Mycena
It is estimated that a single plant of G. elata produces more than 2 million seeds; naturally, the seed germination rate is extremely low, and the yield is unstable (Zhou et al., 2005; Hsieh et al., 2022) because the seeds of G. elata are minute, with most containing an undifferentiated embryo that lacks a well-defined endosperm (Xu et al., 1990). Because of the lack of nutritional reserves, seed germination in nature entirely depends on Mycena strains, which provide nutrients needed for seed germination and protocorm development (Arditti, 1967; Xu et al., 1990; Xu and Guo, 1991; Kim et al., 2006; Dearnaley, 2007). Therefore, these fungi that can promote the germination of G. elata seeds are also known as germinating fungi.
Germinating fungi of the genus Mycena belong to the family Mycenaceae of Basidiomycota and feed on dead trees and fallen leaves (Li et al., 2021). Xu et al. (1980) successfully discovered the sexual reproduction process of G. elata using the leaf fungal bed method, proving that under natural conditions, the sexual reproduction stage of G. elata can only germinate when nourished by germinating fungi. In 1989, protocorms were collected, and conventional tissue separation and monomer separation methods were used to isolate 12 strains that can effectively promote the germination of G. elata seeds (Xu and Guo, 1989). They also successfully induced growth of the fruiting body of the germinating fungus and identified it as Mycena osmundicola by morphological and microscopic observation and enzyme ester isozyme analysis. To study the existence of germinating fungi in the distribution areas of wild G. elata, (Xu et al., 2001) collected deciduous humus soil from the original site to sow G. elata seeds and isolated 11 strains from germinated protocorms. After mixing and sowing G. elata seeds, they obtained three fungi that had a promoting effect on germination. To study the diversity of fungal strains for seed germination of G. elata, scientists isolated 132 strains from the roots of 45 orchid plants and found that Mycena dendrobii (Guo et al., 1999), Mycena anoectochila (Xu et al., 2001), and Mycena orchidicola (Fan et al., 1996) could promote seed germination of G. elata. Guo et al. (1999) isolated M. dendrobii from wild Dendrobium densiflorum and conducted symbiotic germination experiments with seeds of 12 orchid species, and the results showed that the fungus could promote the growth of G. elata and D. densiflorum. From this, we speculate that germinating fungi can not only promote the germination of G. elata seeds but also coexist with other orchid plants, indicating that germinating fungi have a wide range of applications. In existing studies, most mycorrhizal Mycena were isolated from various members of the Orchidaceae or protocorms of G. elata. Species of Mycena with tiny basidiomata are abundant, which complicates identification without basidiomata solely based on the few reliable DNA sequences in GenBank (Figure 3A). Currently, only four species (M. osmundicola, M. orchidicola, M. dendrobii, and M. anoectochila) are known to be able to form basidiomata in cultivation and have thus been successfully identified (Xu and Guo, 1989; Fan et al., 1996; Guo et al., 1997; Guo et al., 1999). Therefore, these four species have become commonly used fungi for seed germination of G. elata in China (Guo and Wang, 2001; Park and Lee, 2013a; Pan et al., 2015; Kitahara et al., 2022).
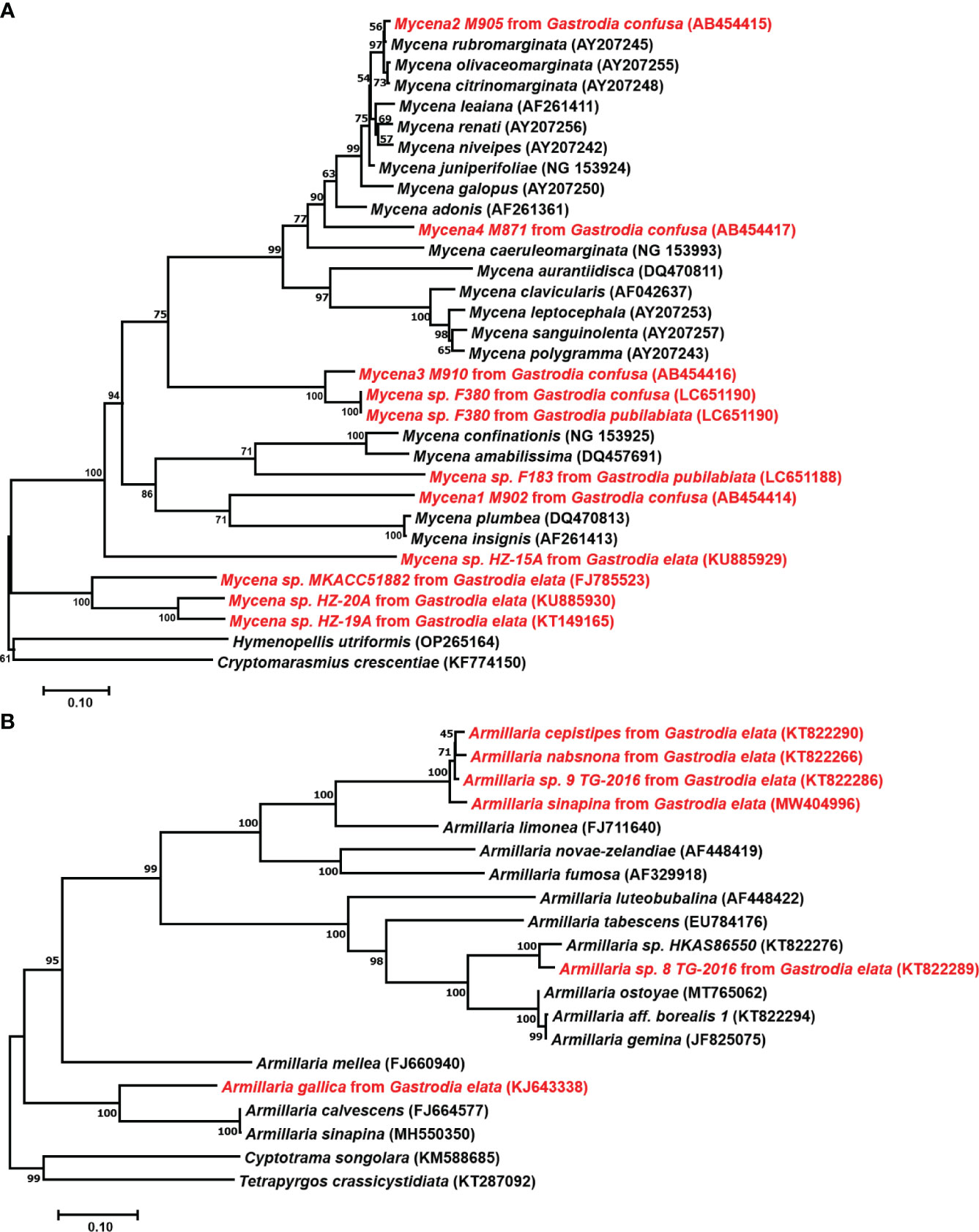
Figure 3 Phylogenetic positions of Mycena and Armillaria. (A), Position of Mycena based on 28S LSU ribosomal RNA sequences. Mycena found in Gastrodia confusa by Ogura-Tsujita et al., 2009, Gastrodia pubilabiata by Kitahara et al., 2022, and Gastrodia elata from GenBank. (B), Position of Armillaria based on ITS sequences. The GenBank ID are shown in parentheses, and the strains used for Gastrodia are highlighted in red. The tree was constructed using neighbor-joining with 1000 bootstrap replicates.
3.2 Armillaria
The genus Armillaria belongs to the family Physalacriaceae of Basidiomycota (Sipos et al., 2018). Armillaria has a strong ability to degrade cellulose and lignin, making it the causative agent of forest root rot (Sipos et al., 2017). However, Armillaria is essential for the growth of G. elata. Mycorrhizal symbiosis between Armillaria and G. elata was first described by Kusano, 1911. In 1965, Xu successfully cultivated G. elata for the first time by using wood with Armillaria (Xu, 2013), and he summarized a set of asexual propagation and cultivation techniques suitable for the large-scale production of G. elata.
The classification and identification of Armillaria is relatively complex. The sexual reproduction of Armillaria makes species identification based on the morphological characteristics of fruiting bodies reasonable. However, the macroscopic and microscopic characteristics of fruiting bodies overlap widely among related species (Antonín et al., 2009; Park et al., 2018). After the discovery of the tetrapolar heterothallic coordination mechanism of Armillaria and the difference in colony morphology between haploid and diploid fungi, this method was widely used to identify fungal species. However, single-spore (haploid) isolates must be available for mating assays. This restricts their value in identifying samples collected as rhizomorphs, which are frequently connected to G. elata tubers (Guo et al., 2016). Recent years have seen a rise in the use of molecular data, notably DNA sequence data, to identify fungal species (Cai et al., 2011). Coetzee et al. (2000) clarified the phylogenetic relationships among biological species of Armillaria from China based on the sequences from tef1-alpha and IGS-1 genes and resolved four main phylogenetic groups, namely, the “Armillaria mellea”, “Armillaria ostoyae”, “Armillaria tabescens”, and “Armillaria gallica” clusters. Guo et al. (2016) phylogenetically analyzed Chinese Armillaria samples using the sequences of the internal transcribed spacer region, translation elongation factor-1 alpha gene and beta-tubulin gene and revealed at least 15 phylogenetic lineages of Armillaria from China, in which 7 phylogenetic lineages of Armillaria were used for the cultivation of G. elata. They also found that G. elata f. glauca and G. elata f. elata form symbiotic relationships with various phylogenetic lineages of Armillaria.
In the G. elata growth stage, Armillaria is the only nutrient source, and the growth rate, activity, and other characteristics of the strain directly affect the quality and yield of G. elata. Hyphae are the nutrient organ of Armillaria. The rhizomorph is an adaptive metamorphosis of hyphae that occurs under adverse environmental conditions or in the later stages of growth. It mainly plays a role in transporting nutrients, water, and oxygen while constantly proliferating, extending, and searching for new nutritional sources (Wong et al., 2020). However, few Armillaria species can benefit the growth of G. elata (Figure 3B). A. mellea, A. gallica, Armillaria sinapina, Armillaria singula, Armillaria nabsnona, etc., are widely used in the cultivation of G. elata (Zhang and Li, 1980; Xu and Guo, 1989; Guo and Wang, 2001; Sekizaki et al., 2008). Additionally, because Armillaria species degeneration occurs during multigenerational reproduction because of the unstable compatibility of foreign strains with G. elata in the primary production area, a focus of study has been on isolating and identifying more and better Armillaria strains to increase strain resources.
4 Mechanism of interaction between symbiotic fungi and G. elata
4.1 The process of fungal colonization and digestion
Previous studies on the symbiotic germination of G. elata seeds and Mycena offered extensive information on the changes in structure and ultrastructure (Peterson and Currah, 1990; Xu, 1990; Uetake et al., 1997; Fan, 1998; Fan et al., 1999a; Fan et al., 1999b; Fan et al., 2002; Chen et al., 2014; Li et al., 2020b). Mycena invades G. elata seeds, first penetrating the seed coat layer and then moving through the suspensor remnant, stipe cell, peloton cells, and digestive cells (Figure 4). Mature seeds of G. elata have an oval-shaped proembryo surrounded by a thin seed coat layer (Xu and Guo, 1989). The proembryo consists of stipe cells, proembryo cells, and meristematic cells. A layer of suspensor remnant rich in nutrients such as polysaccharides is attached to the periphery of the stipe cell, resembling a gelatinous cell structure, and is a vestige of stipe cells that degenerate during embryonic development (Xu and Fan, 2001). Hyphae of Mycena can invade from any cell in the seed coat, accumulate in the suspensor remnant, and then enter the stipe cell (Fan et al., 1999b). The stipe cell is the only pathway for hyphae to invade the proembryo (Li et al., 2020b). When hyphae invade the proembryo from the stipe cell, the proembryo will differentiate into peloton cells and digestive cells. Proembryo cells with a peloton are called peloton cells (Fan et al., 1999a), while digestive cells are large proembryo cells that have the capacity to break down hyphae (Fan et al., 1999a). At the initial stage when proembryo cells are invaded by hyphae, organelles such as mitochondria, endoplasmic reticulum and vacuoles may play a role in the digestion of hyphae (Xu and Fan, 2001). Gradually dominant hyphae can utilize nutrients from embryonic cells for reproduction. The cytoplasm and organelles of the proembryo cells will no longer exist, and the hyphae will be full of cells and form a peloton (Xu and Fan, 2001). In the peloton cells, hyphae are complete in structure, rich in contents, vigorous, and sometimes vacuolated. Digestive cells are the key sites of hyphal digestion, in which hyphae expand rapidly, integrity is destroyed, cytoplasm and organelles are all released, and hyphae decay. After leaving the peloton cells, the hyphae extend inward into larger cells, where they no longer form hyphae and are gradually digested. Digested hyphae reach apical meristematic cells through intercellular transmission (Lan et al., 2002). The meristematic cells obtain nutrients and undergo vigorous division, and the embryo expands, breaking through the seed coat and germinating, forming protocorms. The protocorm differentiates into vegetative propagation corm and vascular tissue. The hyphae invading the embryo cells continue to infect the top of the protocorm along cortical cells, but when they are near the top of the protocorm and below the meristematic cells, the hyphae no longer continue to infect upward (Li et al., 2020b). Regardless of whether the protocorm has a nutritional relationship with Armillaria, it can undergo asexual reproduction to form a vegetative propagation corm. The vegetative propagation corm needs nutrients from Armillaria as soon as possible; otherwise, it will die due to nutrient depletion. Li et al. (2020b) found that there was a tubular endocytic network attached to the lysed hyphae in cortical cells, so they concluded that the hyphae were digested by endocytosis. These discoveries provide crucial clues for the symbiotic seed germination of G. elata with Mycena.
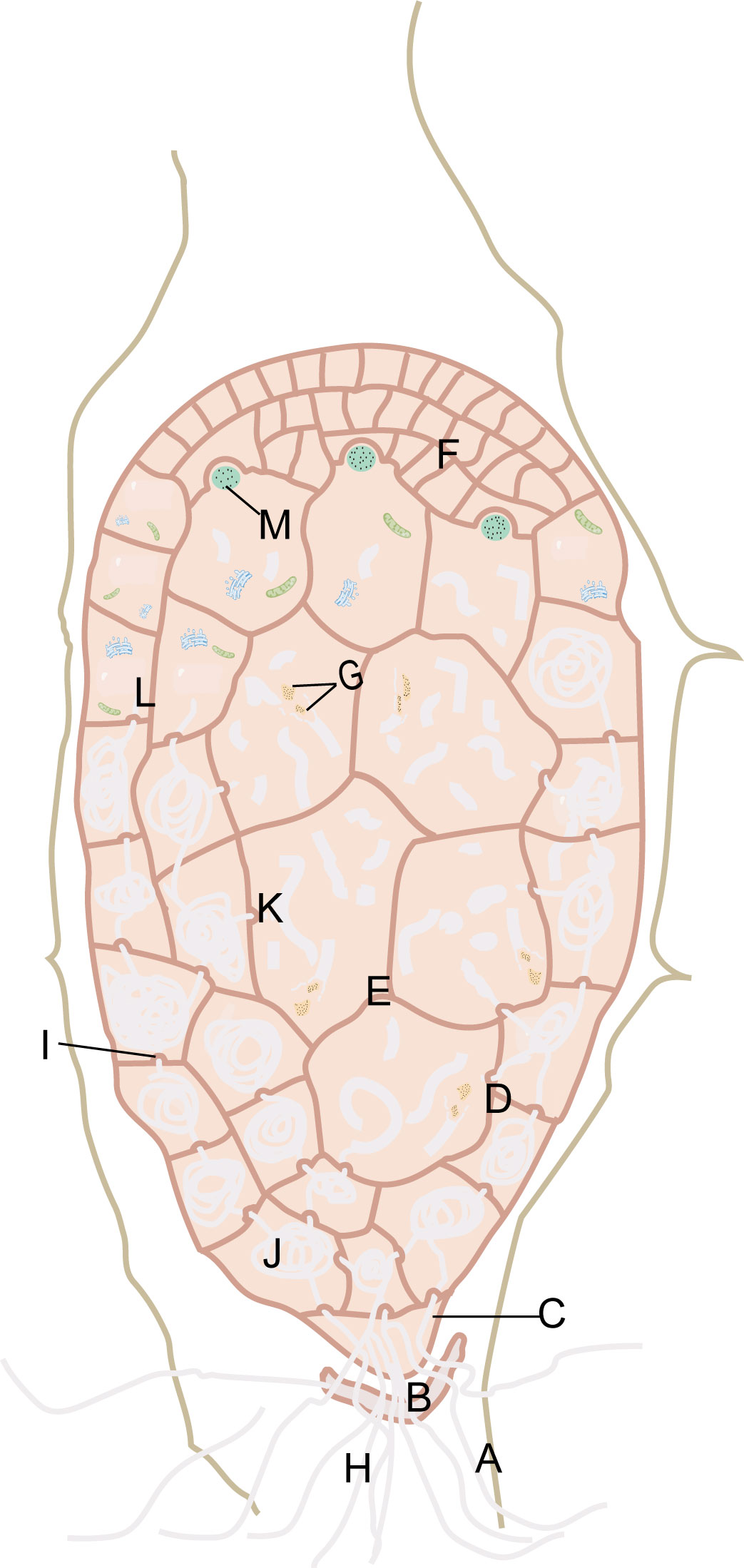
Figure 4 Mycena invade the seeds of G. elata. Seed-coat layer (A). Suspensor remnant (B). Stipe cell (C). Peloton cells (D). Digestive cells (E). Meristematic cells (F). Released cytoplasm and organelles of the hyphae (G). Hyphae (H). Papillary protrusion (I). Peloton (J). Hyphal fragments (K). The proembryo cells in the initial stage of hyphal invasion (L). Digested hyphae (M).
Armillaria invades the vegetative propagation corm of G. elata in turn through the susceptible fungal cells, the hyphal channel or the hyphal flow, the peloton cells, the hyphal flow, and the digestive cells (Figure 5). The cell layer of the vegetative propagation corm is, from outside to inside, the epidermis, peloton cells in the cortex, the hyphal channel or hyphal flow of susceptible fungal cells, and digestive cells in the endodermis (Xu and Guo, 2000). Armillaria must first develop rhizomorphs attached to the epidermis of G. elata before invading it. The hyphae in the rhizomorph penetrate the epidermal cells of G. elata with mechanical force and directly enter a layer of cortical cells outside the endodermis (Xu, 2001). This layer of cells is known as susceptible fungal cells by certain scientists (Wang et al., 1997; Xu, 2001; Xu and Fan, 2001). During the colonization process, the outer sheath and cortex of the rhizomorph are gradually dissolved, leaving only a layer of membrane surrounding the hyphae but still maintaining the morphology of the rhizomorph, which is called the hyphal channel (Wang et al., 1997; Xu, 2001; Xu and Fan, 2001). The hyphae of Armillaria break through the membrane; that is, it loses the form of the rhizomorph and spreads into the cells of the new susceptible fungal cells in the form of hyphae. The bundle-shaped hyphae are called hyphal flow (Wang et al., 1997; Xu, 2001; Xu and Fan, 2001). At this point, the hyphae can nourish themselves with the protoplasm of susceptible fungal cells and colonize the surrounding areas. The hyphae invade the digestive cells inward and the peloton cells outward, with the hyphae flow as the center. The cell walls of the colonized cells exhibit papillary protrusions (Wang et al., 1997; Xu, 2001; Xu and Fan, 2001). After penetrating the papillary protrusions, the hyphae reach digestive cells, where Armillaria is digested (Xu and Mu, 1990). Within cortical cells, hyphae are enveloped by vesicles generated by the protoplasm of the cells. These vesicles twist and coil the hyphae into pelotons, facilitating their gradual division into fragments for subsequent digestion and absorption (Wang et al., 1997; Xu, 2001; Xu and Fan, 2001). The hyphal fragments are released into digestive cells via hyphal flow. Additionally, pelotons have the capacity to breach cell walls and enter adjacent cortical cells (Xu and Mu, 1990). In digestive cells, Armillaria is ultimately digested and absorbed, and viable hyphae cease to exist.
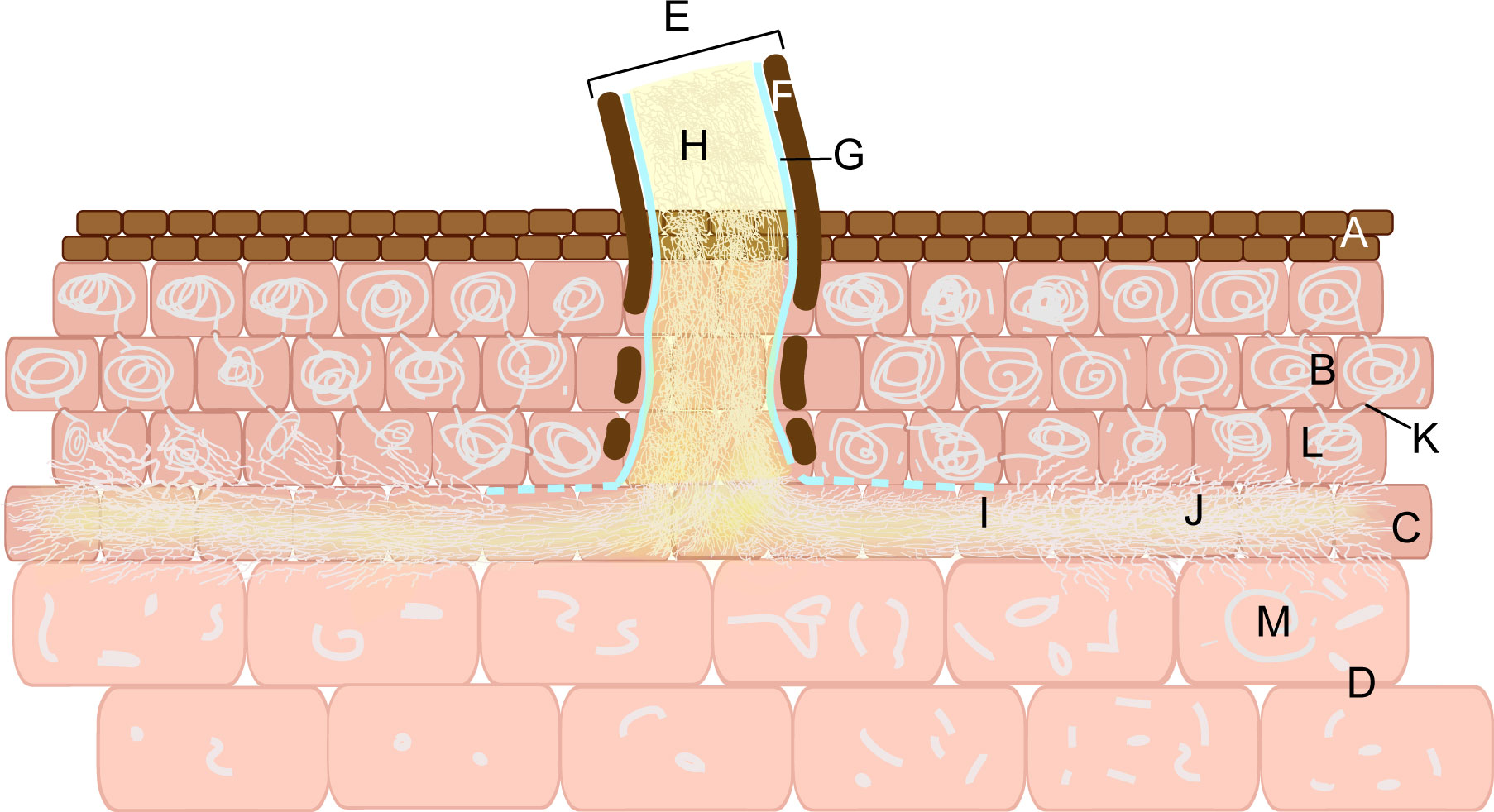
Figure 5 Armillaria invades the vegetative propagation corm of G. elata. Epidermal cells (A). Peloton cells (B). Susceptible fungal cell (C). Digestive cells (D). Rhizomorph (E). Outer sheath (F). Membrane (G). Hyphae (H). The hyphal channel (I). Hyphal flow (J). Papillary protrusion (K). Peloton (L). Hyphal fragments (M).
4.2 Nutrient acquisition in symbiotic relationships
In nature, some mycoheterotrophic orchids are associated with ectomycorrhizal fungi and form tripartite symbioses between trees, mycobionts and orchids (McKendrick et al., 2000). The G. elata growth process is a ternary germination and cropping system (Yuan et al., 2020), which depends on a symbiotic relationship with Mycena and Armillaria. Symbiotic fungi decay leaves or wood to obtain nutrients for their own growth and provide nutrients for G. elata due to colonization and digestion (Suetsugu et al., 2020). Symbiotic fungi act as a bridge to connect the nutrient exchange between leaf/wood and G. elata (Figure 2). Scientists have investigated the effects of different wood as a substrate on the size and ergothioneine concentrations in G. elata (Rong and Cai, 2010). Park and Lee (2013b) investigated 14 tree species and suggested that the use of Ulmus davidiana might increase the production of G. elata tubers.
Symbiotic fungi not only provide nutrients for G. elata but also obtain nutrients from it (Xu and Guo, 1989; Lan et al., 1994; Xu and Guo, 2000; Lan et al., 2002). From the time the proembryo cells are initially able to digest hyphae to the point when they are colonized by hyphae is the stage of mutual benefit in the symbiotic relationship between Mycena and G. elata. Lan et al. (2002) labeled M. osmundicola with 3H-glucose, and the seeds of G. elata were sown on the saprophytic leaves of labeled M. osmundicola. They discovered that many developing silver grains were also found in the newly formed vascular tissue of vegetative propagation corms, indicating that M. osmundicola not only provided nutrients during seed germination and protocorm formation but also needed nutrients from germinating fungi during vegetative propagative corm differentiation and growth. In the symbiotic nutrient chain between G. elata and Armillaria, during hyphal flow, Armillaria can utilize the protoplasm of susceptible fungal cells, which is a favorable stage for Armillaria. Lan et al. (1994) demonstrated this by using 3H-glucose to label G. elata with the pouring method. In cortical cells, Armillaria can utilize the nutrients of cortical cells for division and growth and can invade new cortical cells via a peloton cell. Moreover, some hyphae are also digested by G. elata cortical cells, which is beneficial for the growth of both G. elata and Armillaria. Cortical cells are the site of symbiosis between G. elata and Armillaria. In digestive cells, the complete digestion and absorption of hyphae is a favorable stage for G. elata.
The carbon source has been revealed to be critical to establish optimal symbiosis (Kiers et al., 2011; Hennion et al., 2019). The nutrient exchange between plants and mycorrhizal fungi is that plants provide photosynthetically fixed C to symbiotic fungi, and they benefit from fungi by absorbing mineral nutrients, such as N and P (Bücking and Kafle, 2015; Jacquemyn et al., 2015; Wang et al., 2017). Cameron et al. (2006) demonstrated for the first time mutualism in orchid mycorrhizae, bidirectional transfer of C between a green orchid and its fungal symbiont, and a fungus-dependent pathway for organic N acquisition by an orchid. However, (Fochi et al., 2017)investigated the expression of fungal and plant nitrogen (N) transport and assimilation genes in mycorrhizas formed between the fungus Tulasnella calospora and the achlorophyllous protocorms of the photosynthetic orchid Serapias vomeracea. Their research suggested, for the first time, that nutrients flow back to the fungal partner from the nonphotosynthetic orchid host (Dearnaley and Cameron, 2017). Yeh et al. (2019) proposed that cells of nonphotosynthetic orchids export ammonium (NH4+) to their fungal partners and receive N, P and C for germination and growth. In addition, decayed pelotons can also release N, P and C to nonphotosynthetic orchids when pelotons are digested (Bougoure et al., 2014). This result is largely consistent with the nutrient exchange between G. elata and symbiotic fungi. The nonphotosynthetic orchid G. elata completely replaces its photosynthetic capacity by taking up C from symbiotic fungi (Suetsugu et al., 2020). The symbiotic fungi Mycena and Armillaria obtain C through parasitism of fallen leaves or wood, providing all C sources to maintain the germination and growth of G. elata (Kikuchi et al., 2008a; Kikuchi et al., 2008b). Because the cell walls of fungi are mainly composed of glucan and chitin (Ruiz-Herrera and Ortiz-Castellanos, 2019; Chen et al., 2020), the digestion of hyphae may provide a large amount of organic C and N for G. elata. Moreover, high levels of sucrose accumulate in G. elata tubers at all stages, indicating that sucrose may be the main form of carbohydrates transported to G. elata at the symbiotic interface (Ho et al., 2021). Symbiotic cells are the main sites for extracellular sucrose exchange at the heterotrophic interface of fungi. Sugar transporters have been identified that are located on contiguous plant and fungal cells, and these transporters may regulate sugar exchange, ensuring benefits for both partners in this symbiotic relationship (Hennion et al., 2019). The sucrose transporter gene SUT4 in G. elata was shown to mediate sucrose import at the symbiotic interface for carbon allocation of Armillaria-colonized juvenile tubers (Ho et al., 2021). Based on the amplification of the gene encoding trehalase in the genome of Gastrodia menghaiensis, a species closely related to G. elata, (Jiang et al., 2022) proposed that it may have evolved the ability to use trehalose as its organic carbon source. The absence of nitrate transporters and the increase in the number of urease genes indicate that the absorption of nitrogen by G. menghaiensis mainly occurs in the form of ammonium. While most raw nutrients primarily originate from fungi, the highly expressed genes for fatty acid and ammonium root transporters indicate that fungi obtain nutrients from G. menghaiensis. G. elata may share some features with G. menghaiensis, however, further research is needed. Arginases can hydrolyze arginine acid in hyphae to urea, which is further hydrolyzed to ammonium and carbonic acid by ureases (Witte et al., 2005). The number of genes encoding ureases in G. elata is sharply increased, indicating that urea metabolism may be an important source of N for G. elata (Yuan et al., 2018).
Through experimental studies, the penetration of Armillaria into G. elata is divided into two forms (Xu and Guo, 2000; Morrison, 2004). One is normal physiological colonization. G. elata induces Armillaria colonization by secreting specific chemicals, such as strigolactone (Hua et al., 2024), and then secretes enzymes to digest Armillaria for energy. The more Armillaria colonizes, the more energy G. elata acquires and the more quickly it grows. The other is pathological infection; Armillaria will penetrate the digestive layer of the mother tuber (immature tuber), infiltrate the stele layer, and subsequently invade the new tuber along the vascular bundle, resulting in the decay of the new tuber. However, this rarely happens. When Armillaria’s nutritional supply is insufficient and G. elata’s development or resistance declines, Armillaria will use the nutrients in G. elata to grow (Xu and Guo, 2000). Therefore, we speculate that if G. elata has strong inducibility to the corresponding Armillaria and the invasion of Armillaria is weak, G. elata grows normally. In contrast, if inducibility is weak and invasiveness is strong, G. elata will be consumed. Therefore, only the combination of G. elata with strong inducibility and Armillaria with weak invasion ability can allow G. elata to obtain nutrients through corresponding strategies for maintenance.
4.3 Adaptation of fungi to symbiosis
The increased secretion of some enzymes by fungi is beneficial for establishing symbiotic relationships. The seed coat of G. elata is composed of only lignin (Li et al., 2016), and the lignin-degrading ability of the germination-promoting fungus Mycena is the potentially key to their symbiosis. Manganese peroxidase is a fungal lignin-modifying enzyme, and when Mycena breaks through the seed coats of G. elata, manganese peroxidase and laccase are responsible for the degradation of lignin (Manavalan et al., 2015). Ren et al. (2021) conducted transcriptome analysis and found that the upregulation of manganese-dependent peroxidase short genes was conducive to the invasion of G. elata seeds by hyphae. In addition, the expression of laccase genes was significantly upregulated to produce more laccase for degrading the lignin seed coat. Compared with nonsymbiotic Armillaria, Armillaria that were symbiotic with G. elata had more glycoside hydrolases, carbohydrate-binding modules, and glycosyl transferases (Zhan et al., 2020; Cai et al., 2023). These enzymes contribute to the degradation of cell walls, fungal colonization and secondary metabolic synthesis, (Deng et al., 2021; Liu et al., 2021) which may contribute to the successful establishment of symbiosis between Armillaria and G. elata. Virulence attenuation is related to the enhanced adaptability of fungi to G. elata. Once symbiosis is established, G. elata begins to grow with increased biological activity, while the fungi are restricted (Fan et al., 1999a). Ren et al. (2021) showed this through comparative transcriptome analysis of seed symbiotic Mycena hyphae and pure cultured hyphae, and furthermore, they sequenced and analyzed the genome of Mycena and found that 5024 genes were annotated in the pathogen-host interactions database, among which more than half were linked to reduced virulence and loss of pathogenicity. Zhan et al. (2020) assembled a draft genomic sequence of A. gallica 012m and found that the gene families related to the pathogenicity/saprophytic phase, including hydrophobins, carbohydrate active enzyme AA3, and cytochrome P450 monooxygenases, had significantly contracted in A. gallica 012m, which might be beneficial for G. elata to reduce injury. They also found, through genome-guided analysis, that rhizomorphs exhibit higher infectivity compared to vegetative mycelia. This characteristic aids G. elata in nutrient acquisition, as rhizomorphs continually colonize G. elata’s nutritional stems and generate hyphae that G. elata can subsequently digest. Guo et al. (2016) revealed at least 15 phylogenetic lineages in China through the phylogenetic analysis of Armillaria, of which 7 species that are less virulent and aggressive or preferentially saprotrophic are related to cultivated G. elata. Virulence experiments demonstrated that A. mellea has a greater or equal virulence than A. ostoyae, A. ostoyae has a greater virulence than A. gallica and A. cepistipes, and A. tabescens has the weakest virulence among those five species (Gregory, 1985; Morrison, 2004; Caballero et al., 2022). The reason is that the saprophytic colonization scores of Armillaria with monopodially branched rhizomorphs are significantly higher than those of dichotomously branched species, while the dichotomously branched species are more aggressive than monopodially branched species (Morrison, 2004) (Table 2). Therefore, we speculate that Armillaria with monopodially branched rhizomorphs is more suitable for cultivating G. elata.
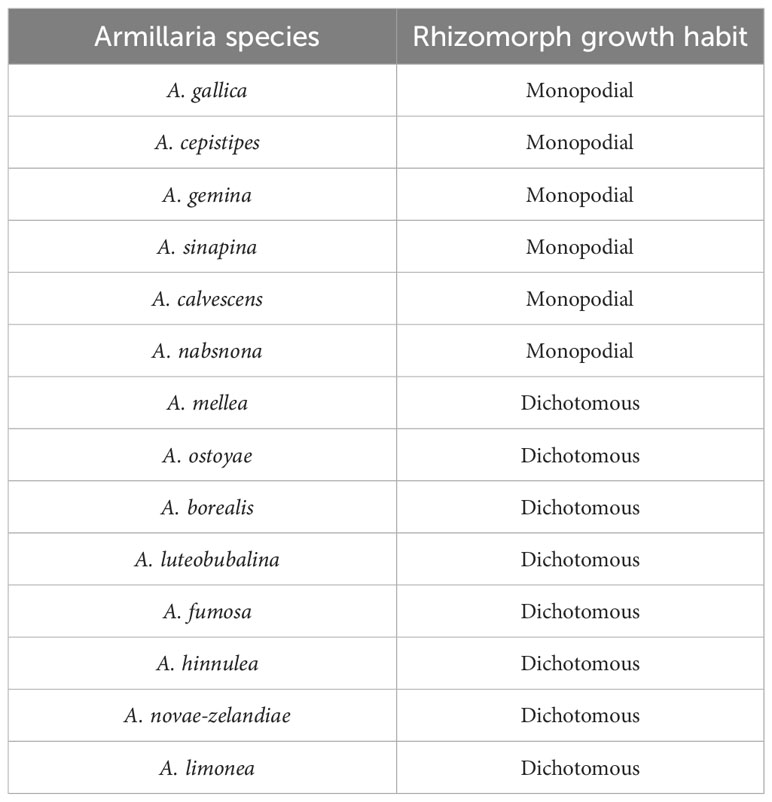
Table 2 Rhizomorph growth habit of some Armillaria species (Morrison, 2004).
4.4 Response of G. elata to fungi
The evolutionary adaptation of G. elata to the mycoheterotrophic lifestyle was critically dependent on gene loss. The genome of G. elata has been continuously reported in recent years, offering insights into how G. elata adapted to heterotrophy in Mycena and Armillaria. In G. elata, genes important in the control of flowering time, the circadian clock, nutrient absorption, immunity, growth of the roots and leaves, and photosynthesis were all severely lost (Yuan et al., 2018; Park et al., 2020; Xu et al., 2021; Bae et al., 2022).
The expansion of many gene families in G. elata is additional evidence for adaptation to the mycoheterotrophic lifestyle of fungi. Phylogenetic analysis showed that the number of genes of G. elata concerned with mycorrhizal association was significantly expanded (Bae et al., 2022; Jiang et al., 2022). These phenomena represent evolutionary events and may be the result of G. elata adapting to a heterotrophic lifestyle in the presence of Armillaria (Yuan et al., 2018; Bae et al., 2022). Yuan et al. (2018) found that the increase in the number of genes encoding carotenoid cleavage dioxygenases and ABC transporters indicated that G. elata has a strengthened ability to interact with Armillaria to improve the efficiency of establishing symbiotic relationships. Genes involved in the Ca2+ spiking process have been shown to regulate the colonization of plants by fungi and are found in large quantities in G. elata. Some glycoside hydrolases from gene families were highly expressed in the cortex layer of G. elata, which supports the view that the hyphae of Armillaria are digested in the digestive cells of G. elata. Strigolactone is a plant hormone that has been proven to have branch-inducing effects in Armillaria (Yuan et al., 2018; Favre-Godal et al., 2020). The number of key genes for the biosynthesis and secretion of strigolactone has grown in G. elata (Yuan et al., 2018). Jiang et al. (2022) found that 36 beta-glucosidase genes and 4 glycoside hydrolase family 18 chitinases in G. menghaiensis may be involved in the degradation of the fungal cell wall to provide nutrients for G. menghaiensis.
Gene contraction in G. elata is a characteristic facilitating adaptation to the mycoheterotrophic lifestyle. G. elata seed germination is hampered not only by insufficient nutritional reserves and an impermeable seed coat but also by the presence of seed germination inhibitors such as phenolics and abscisic acid (Van Waes and Debergh, 1986; Ren et al., 2021). The downregulation of 9-cis-epoxycarotenoid dioxygenase (NCED-2) expression in G. elata reduces abscisic acid production, thereby alleviating abscisic acid’s suppression of seed germination. Additionally, a significantly downregulated receptor protein (PYL12-like) can hinder abscisic acid signaling and thereby break seed dormancy (Ren et al., 2021).
G. elata must fend against pathogen assaults despite being fully dependent on symbiotic fungi for survival. Significant defensive reactions are induced by fungal colonization in G. elata, which possesses genetic, pharmacological, and physical defenses against fungi. Armillaria can only colonize vegetative propagation corms and immature tubers (mother tubers). Newborn tubers can resist colonization by Armillaria. From the perspective of G. elata, this is because there is an isolation area without nutrient reserves at the contact between the vegetative propagation corm and the new tuber, which limits the spread of Armillaria along the cortical cells to invade the new tubers. The cell wall of the mother tuber near the new tuber thickens and becomes corky. Then, a fracture layer will form at the bottom of the new tuber. The corked and thickened cell wall is a physical defense structure of G. elata against Armillaria infection (Fan et al., 1999a).
The epidermal cells of vegetative propagation corms are initially digested by the matching enzymes released by Armillaria when they penetrate. G. elata causes cortical cells to create numerous hydrolases as a form of defense after learning that epidermal cells have penetrated. Hydrolase breaks hyphae down into tiny molecules that G. elata may consume, supplying it with ongoing nutrients for growth. (Wang and Xu, 1993; Fan et al., 1999a) Additionally, Armillaria has the ability to induce G. elata to produce a set of defense proteins (Yuan et al., 2020). The monocot mannose binding lectin antifungal protein family has been proven to inhibit fungal growth in G. elata (Xu et al., 1998; Wang et al., 2001b; Nagel et al., 2008). More than 80% of the gastrodia antifungal protein genes in G. elata are highly expressed during the growth stage before establishing a stable symbiotic relationship with Armillaria (Yuan et al., 2018). In addition, G. elata can transport S-(p-HA)-glutathione phytoalexin to Armillaria and prevent its excessive growth. Defense proteins and phytoalexins are chemical defenses of G. elata against Armillaria infection (Yuan et al., 2018).
G. elata also retains some defense-related genes. Zeng et al. (2017) used transcriptome approaches to identify 1750 differentially expressed genes between G. elata seeds and protocorms. Most of these differentially expressed genes were presumably involved in plant defense, molecular signaling, secondary metabolism, and energy metabolism. Zeng et al. (2018) compared the proteomes of the early and late stages of protocorms. Among them, defense genes (e.g., pathogenesis-/wound-related proteins, peroxidases, and serine/threonine-protein kinase) were highly expressed in late-stage protocorms, suggesting that fungal colonization triggered significant defense responses in G. elata. The G. menghaiensis genome contains 28 terpene synthase genes, which defend against pathogens (Zeng et al., 2017). The G. menghaiensis genome contains 65 R genes (resistance), which are important components of the plant defense system (Ren et al., 2021).
4.5 Microbes can affect the symbiosis of G. elata and Armillaria
Microbes and their secondary metabolites are also believed to affect the symbiosis of fungi and G. elata. The fungal community of tubers in different growth phases and the soils surrounding G. elata were characterized by high-throughput sequencing (Chen et al., 2019; Yuan et al., 2020). Mycorrhizosphere bacteria, Rahnella sp. HPDA25 has been proven to secrete indole-3-acetic acid to promote the growth of A. gallica and its parasitic host G. elata (Liu et al., 2022). The coculture of HPDA25 and A. gallica also decreased the expression levels of glycolysis-related genes, which may advance rhizomorph growth by inhibiting glycolysis in A. gallica. Irpex lacteus tended to promote the growth of Armillaria in coculture by producing 2,3-dihydroxydodacane-4,7-dione to selectively inhibit the phytopathogen and endophyte in the host G. elata, which is conducive to the symbiosis of G. elata and Armillaria symbiosis (Wang et al., 2021a). Many beneficial compounds were isolated from the coculture of Armillaria sp. and the endophytic fungus associated with G. elata (Li et al., 2019; Li et al., 2020a). In contrast, Armillaria can also affect the structure of the microbial community associated with G. elata, as evidenced by the increased diversity of bacteria and fungi from the immature tuber to mature tuber periods (Yuan et al., 2018). In addition, early studies have shown that Armillaria, as a medicinal fungus, can secrete various antibacterial and antifungal compounds, such as armillaric acid and sesquiterpene aryl esters, which show strong inhibition against gram-positive bacteria, Streptococcus spp., yeast, Rifai aggr., Mucor spp., Gliocladium viren, Fusarium spp., Rhizopus stoloniferp, and Trichoderma spp. (Yuan et al., 2020). Mycena is a source of plant hormones and nutrients for G. elata according to a study of the secondary metabolites of fungi (Liang et al., 2018).
5 Conclusion and future perspectives
The roles that Mycena and Armillaria play in the life cycle of G. elata are crucial. Various developmental phases result in various histological and ultrastructural properties during the colonization and digestion of hyphae in G. elata. The material basis of symbiotic partnerships is the bilateral flow of nutrients between G. elata and fungi. Moreover, to adapt to symbiosis, gene expression and enzyme release are altered in both fungi and G. elata. Additionally, some microbes and their byproducts are advantageous for symbiosis between fungi and G. elata. We have thoroughly explained the three components of the symbiotic mechanism: morphology, feeding, and chemicals.
However, there are still many issues with the symbiotic mechanism that need to be further clarified. We believe that the following topics should be the main focus of future research. i) What are the advantages of G. elata and fungi developing a symbiotic connection in order for both to adapt to the natural environment? ii) What evolutionary trends and features do fungi and G. elata have in their genomes that enable them to develop and sustain symbiotic relationships? iii) How are the immune systems, signaling pathways, and metabolic processes of fungi and G. elata regulated and controlled to adapt to symbiosis?
Author contributions
J-JL: Writing – original draft. X-QY: Visualization, Writing – original draft. Z-YL: Investigation, Visualization, Writing – original draft. J-YM: Investigation, Resources, Writing – original draft. S-BL: Investigation, Visualization, Writing – original draft. W-PZ: Writing – review & editing. Y-CL: Funding acquisition, Writing – review & editing. L-BL: Funding acquisition, Project administration, Supervision, Writing – review & editing.
Funding
The author(s) declare financial support was received for the research, authorship, and/or publication of this article. This work is funded by the Major Science and Technology Plan Special Project in Yunnan Province, China (202202AG050008), the Open Fund of Yunnan Key Laboratory of Gastrodia and Fungal Symbiotic Biology, Zhaotong University.
Conflict of interest
Authors J-YM and S-BL are employed by the company Senhao Fungi Industry Co., Ltd.
The remaining authors declare that the research was conducted in the absence of any commercial or financial relationships that could be construed as a potential conflict of interest.
Publisher’s note
All claims expressed in this article are solely those of the authors and do not necessarily represent those of their affiliated organizations, or those of the publisher, the editors and the reviewers. Any product that may be evaluated in this article, or claim that may be made by its manufacturer, is not guaranteed or endorsed by the publisher.
References
Antonín, V., Tomšovský, M., Sedlák, P., Májek, T., Jankovský, L. (2009). Morphological and molecular characterization of the Armillaria cepistipes – A. gallica complex in the Czech Republic and Slovakia. Mycol Prog. 8, 259–271. doi: 10.1007/s11557-009-0597-1
Arditti, J. (1967). Factors affecting the germination of orchid seeds. Bot. Rev. 33, 1–97. doi: 10.1007/BF02858656
Bae, E. K., An, C., Kang, M. J., Lee, S., Lee, S. J., Kim, K. T., et al. (2022). Chromosome-level genome assembly of the fully mycoheterotrophic orchid Gastrodia elata. G3 Genes|Genomes|Genetics 12, jkab433. doi: 10.1093/g3journal/jkab433
Bougoure, J., Ludwig, M., Brundrett, M., Cliff, J., Clode, P., Kilburn, M., et al. (2014). High-resolution secondary ion mass spectrometry analysis of carbon dynamics in mycorrhizas formed by an obligately myco-heterotrophic orchid. Plant Cell Environ. 37, 1223–1230. doi: 10.1111/pce.12230
Bücking, H., Kafle, A. (2015). Role of arbuscular mycorrhizal fungi in the nitrogen uptake of plants: current knowledge and research gaps. Agronomy 5, 587–612. doi: 10.3390/agronomy5040587
Caballero, J. R. I., Lalande, B. M., Hanna, J. W., Klopfenstein, N. B., Kim, M.-S., Stewart, J. E. (2022). Genomic comparisons of two Armillaria species with different ecological behaviors and their associated soil microbial communities. Microb. Ecol. 85, 708–729. doi: 10.1007/s00248-022-01989-8
Cai, L., Giraud, T., Zhang, N., Begerow, D., Cai, G., Shivas, R. G. (2011). The evolution of species concepts and species recognition criteria in plant pathogenic fungi. Fungal Diversity 50, 121–133. doi: 10.1007/s13225-011-0127-8
Cai, J., Muhammad, I., Chen, B., Xu, P., Li, Y., Xu, H., et al. (2023). Whole genome sequencing and analysis of Armillaria gallica Jzi34 symbiotic with Gastrodia elata. BMC Genomics 24, 275. doi: 10.1186/s12864-023-09384-4
Cameron, D. D., Leake, J. R., Read, D. J. (2006). Mutualistic mycorrhiza in orchids: evidence from plant–fungus carbon and nitrogen transfers in the green-leaved terrestrial orchid Goodyera repens. New Phytol. 171, 405–416. doi: 10.1111/j.1469-8137.2006.01767.x
Cha, J. Y., Igarashi, T. (1995). Armillaria species associated with Gastrodia elata in Japan. Eur. J. For. Pathol. 25, 319–326. doi: 10.1111/j.1439-0329.1995.tb01347.x
Chase, M. W., Cameron, K. M., Freudenstein, J. V., Pridgeon, A. M., Salazar, G., van den Berg, C., et al. (2015). An updated classification of Orchidaceae. Botanical J. Linn. Soc. 177, 151–174. doi: 10.1111/boj.12234
Chen, Y. Y., Bao, Z. X., Li, Z. Z. (2011c). High allozymic diversity in natural populations of mycoheterotrophic Orchid Gastrodia elata, an endangered medicinal plant in China. Biochem. Systematics Ecol. 39, 526–535. doi: 10.1016/j.bse.2011.07.013
Chen, X., Cao, D. X., Zhou, L., Jin, H. Y., Dong, Q., Yao, J., et al. (2011b). Structure of a polysaccharide from Gastrodia elata Bl., and oligosaccharides prepared thereof with anti-pancreatic cancer cell growth activities. Carbohydr. Polymers 86, 1300–1305. doi: 10.1016/j.carbpol.2011.06.029
Chen, W., Jiang, X., Yang, Q. (2020). Glycoside hydrolase family 18 chitinases: The known and the unknown. Biotechnol. Adv. 43, 107553. doi: 10.1016/j.bioteChadv.2020.107553
Chen, P. J., Liang, K. C., Lin, H. C., Hsieh, C. L., Su, K. P., Hung, M. C., et al. (2011a). Gastrodia elata Bl. attenuated learning deficits induced by forced-swimming stress in the inhibitory avoidance task and morris water maze. J. Medicinal Food 14, 610–617. doi: 10.1089/jmf.2010.1209
Chen, P. J., Sheen, L. Y. (2011). Gastrodiae Rhizoma (tiān má): a review of biological activity and antidepressant mechanisms. J. Traditional Complementary Med. 1, 31–40. doi: 10.1016/S2225-4110(16)30054-2
Chen, J., Wang, H., Liu, S. S., Li, Y. Y., Guo, S. X., Chen, J., et al. (2014). Ultrastructure of symbiotic germination of the orchid Dendrobium officinale with its mycobiont, Sebacina sp. Aust. J. Bot. 62, 229–234. doi: 10.1071/BT14017
Chen, L., Wang, Y. C., Qin, L. Y., He, H. Y., Yu, X. L., Yang, M. Z., et al. (2019). Dynamics of fungal communities during Gastrodia elata growth. BMC Microbiol. 19, 158. doi: 10.1186/s12866-019-1501-z
Coetzee, M. P. A., Wingfield, B. D., Harrington, T. C., Dalevi, D., Coutinho, T. A., Wingfield, M. J. (2000). Geographical diversity of Armillaria mellea s. s. based on phylogenetic analysis. Mycologia 92, 105–113. doi: 10.1080/00275514.2000.12061134
Dearnaley, J. D. W. (2007). Further advances in orchid mycorrhizal research. Mycorrhiza 17, 475–486. doi: 10.1007/s00572-007-0138-1
Dearnaley, J. D. W., Cameron, D. D. (2017). Nitrogen transport in the orchid mycorrhizal symbiosis - further evidence for a mutualistic association. New Phytol. 213, 10–12. doi: 10.1111/nph.14357
Deng, Q., Wu, H., Gu, Q., Tang, G., Liu, W. (2021). Glycosyltransferase FvCpsA regulates fumonisin biosynthesis and virulence in fusarium verticillioides. Toxins 13, 718. doi: 10.3390/toxins13100718
Dressler, R. L. (1981). The orchids: natural history and classification (Cambridge, Massachusetts: Harvard University Press).
Dressler, R. L. (1993). Phylogeny and classification of the orchid family (Cambridgeshire: Cambridge University Press).
Fan, L. (1998). Study on the change of ultrastructure between protocorms of Gastrodia elata and Mycena anoectochila in symbiotic germination. J. Jilin Argricultural Univ. 226. doi: 10.13327/j.jjlau.1998.s1.198
Fan, L., Guo, S. X., Cao, W. Q., Xiao, P. G., Xu, J. T. (1996). Isolation, culture, identification and biological activity of Mycena orchidicola sp. nov. in Cymbidium sinense (Orchidaceae). Acta Mycolagica Sin. 15, 251–255. doi: 10.13346/j.mycosystema.1996.04.003
Fan, L., Guo, S. X., Xu, J. T. (1999a). Interaction between protocorms of Gastrodia elata (Orchidaceae) and Mycena Dendrobii insymbiotic germination. Mycosystema 18, 219–225+229-232. doi: 10.13346/j.mycosystema.1999.02.021
Fan, L., Guo, S. X., Xu, J. T. (1999b). Ultrastructral changes during the symbiotic development of Gastrodia elata associated with Mycena orchidicola. Mycosystema 18, 431–435. doi: 10.13346/j.mycosystema.1999.04.017
Fan, L., Guo, S. X., Xu, J. T. (2002). Ultrastructural changes during the symbiotic development of Gastrodia elata (Orchidaceae) protocorms associated with Mycena osmundicola. J. Capital Normal Univ. (Natural Sci. Edition) 23, 52–56. doi: 10.19789/j.1004-9398.2002.03.014
Favre-Godal, Q., Gourguillon, L., Lordel-Madeleine, S., Gindro, K., Choisy, P. (2020). Orchids and their mycorrhizal fungi: an insufficiently explored relationship. Mycorrhiza 30, 5–22. doi: 10.1007/s00572-020-00934-2
Fochi, V., Chitarra, W., Kohler, A., Voyron, S., Singan, V. R., Lindquist, E. A., et al. (2017). Fungal and plant gene expression in the Tulasnella calospora - Serapias vomeracea symbiosis provides clues about nitrogen pathways in orchid mycorrhizas. New Phytol. 213, 365–379. doi: 10.1111/nph.14279
Gao, M., Wu, Y., Yang, L., Chen, F., Li, L., Li, Q., et al. (2023). Anti-depressant-like effect of fermented Gastrodia elata Bl. by regulating monoamine levels and BDNF/NMDAR pathways in mice. J. Ethnopharmacology 301, 115832. doi: 10.1016/j.jep.2022.115832
Genre, A., Lanfranco, L., Perotto, S., Bonfante, P. (2020). Unique and common traits in mycorrhizal symbioses. Nat. Rev. Microbiol. 18, 649–660. doi: 10.1038/s41579-020-0402-3
Gregory, S. C. (1985). The use of potato tubers in pathogenicity studies of Armillaria isolates. Plant Pathol. 34, 41–48. doi: 10.1111/j.1365-3059.1985.tb02758.x
Guo, S. X., Fan, L., Cao, W. Q., Chen, X. M. (1999). Mycena dendrobii, a new mycorrhizal fungus. Mycosystema 18, 141–144. doi: 10.13346/j.mycosystema.1999.02.007
Guo, S. X., Fan, L., Cao, W. Q., Xu, J. T., Xiao, P. G. (1997). Mycena anoectochila sp. nov. isolated from mycorrhizal roots of Anoectochilus roxburghii from Xishuangbanna, China. Mycologia 89, 952–954. doi: 10.1080/00275514.1997.12026866
Guo, S. X., Wang, Q. Y. (2001). Character and action of good strain on stimulating seed germination of Gastrodia elata. Mycosystema 20, 408–412. doi: 10.3969/j.issn.1672-6472.2001.03.023
Guo, T., Wang, H. C., Xue, W. Q., Zhao, J., Yang, Z. L. (2016). Phylogenetic analyses of Armillaria reveal at least 15 phylogenetic lineages in China, seven of which are associated with cultivated Gastrodia elata. PloS One 11, e0154794. doi: 10.1371/journal.pone.0154794
Hennion, N., Durand, M., Vriet, C., Doidy, J., Maurousset, L., Lemoine, R., et al. (2019). Sugars en route to the roots. Transport, metabolism and storage within plant roots and towards microorganisms of the rhizosphere. Physiologia Plantarum 165, 44–57. doi: 10.1111/ppl.12751
Ho, L. H., Lee, Y. I., Hsieh, S. Y., Lin, I. S., Wu, Y. C., Ko, H. Y., et al. (2021). GeSUT4 mediates sucrose import at the symbiotic interface for carbon allocation of heterotrophic Gastrodia elata (Orchidaceae). Plant Cell Environ. 44, 20–33. doi: 10.1111/pce.13833
Hsieh, C. H., Liang, Z. C., Shieh, W. J., Chang, S. L., Ho, W.-J. (2022). Effects of nutrients and growth regulators on seed germination and development of juvenile rhizome proliferation of Gastrodia elata in vitro. Agriculture 12, 1210. doi: 10.3390/agriculture12081210
Hua, Z. Y., Teng, X. Y., Huang, J. W., Zhou, J. H., Zhao, Y. Y., Huang, L. Q., et al. (2024). The Armillaria response to Gastrodia elata is partially mediated by strigolactone-induced changes in reactive oxygen species. Microbiological Res. 278, 127536. doi: 10.1016/j.micres.2023.127536
Huang, H. S., Lin, Y. E., Panyod, S., Chen, R. A., Lin, Y. C., Chai, L. M. X., et al. (2023). Anti-depressive-like and cognitive impairment alleviation effects of Gastrodia elata Blume water extract is related to gut microbiome remodeling in ApoE–/– mice exposed to unpredictable chronic mild stress. J. Ethnopharmacology 302, 115872. doi: 10.1016/j.jep.2022.115872
IUCN Red List. (2023). Available at: https://www.iucnredlist.org/species/46671/11069069 (Accessed November 17, 2023).
Jacquemyn, H., Brys, R., Waud, M., Busschaert, P., Lievens, B. (2015). Mycorrhizal networks and coexistence in species-rich orchid communities. New Phytol. 206, 1127–1134. doi: 10.1111/nph.13281
Jiang, Y., Hu, X., Yuan, Y., Guo, X., Chase, M. W., Ge, S., et al. (2022). The Gastrodia menghaiensis (Orchidaceae) genome provides new insights of orchid mycorrhizal interactions. BMC Plant Biol. 22, 179. doi: 10.1186/s12870-022-03573-1
Kiers, E. T., Duhamel, M., Beesetty, Y., Mensah, J. A., Franken, O., Verbruggen, E., et al. (2011). Reciprocal rewards stabilize cooperation in the mycorrhizal symbiosis. Science 333, 880–882. doi: 10.1126/science.1208473
Kikuchi, G., Higuchi, M., Morota, T., Nagasawa, E., Suzuki, A. (2008a). Fungal symbiont and cultivation test of Gastrodia elata blume (Orchidaceae). J. Japanese Bot. 83, 88–95. doi: 10.51033/jjapbot.83_2_10034
Kikuchi, G., Higuchi, M., Yoshimura, H., Morota, T., Suzuki, A. (2008b). In vitro symbiosis between Gastrodia elata blume (Orchidaceae) and Armillaria kummer (Tricholomataceae) species isolated from the orchid tuber. J. Japanese Bot. 83, 77–87. doi: 10.51033/jjapbot.83_2_10033
Kim, Y. I., Chang, K. J., Ka, K. H., Hur, H., Hong, I. P., Shim, J. O., et al. (2006). Seed germination of Gastrodia elata using symbiotic fungi, Mycena osmundicola. Mycobiology 34, 79–82. doi: 10.4489/MYCO.2006.34.2.079
Kim, H. J., Hwang, I. K., Won, M. H. (2007). Vanillin, 4-hydroxybenzyl aldehyde and 4-hydroxybenzyl alcohol prevent hippocampal CA1 cell death following global ischemia. Brain Res. 1181, 130–141. doi: 10.1016/j.brainres.2007.08.066
Kitahara, M., Nagamune, K., Kinoshita, A., Yugeta, C., Ohara, N., Shimazaki, A., et al. (2022). In-vitro symbiotic germination of seeds of five mycoheterotrophic Gastrodia orchids with Mycena and Marasmiaceae fungi. Mycoscience 63, 88–95. doi: 10.47371/mycosci.2022.04.001
Kusano, S. (1911). Gastrodia elata and its symbiotic association with Armillaria mellea. J.agri.col.tokyo Imp.univ 4, 1.
Lan, J., Xu, J. T., Li, J. S. (1994). Study on symbiotic relation between Gastrodia elata and Armillariella mellea by autoradiogarphy. Acta Mycolagica Sin. 13, 219–222+247-248. doi: 10.13346/j.mycosystema.1994.03.012
Lan, J., Zhu, Y. Y., He, X. X., Tang, Y. J., Xu, J. T. (2002). The transport pathway of labelled Mycena osmundicola and assimilated labelled materials in the embryonic cells of Gastrodia elata. Acta Agriculturae Nucleatae Sin. 16, 49–52. doi: 10.3969/j.issn.1000-8551.2002.01.010
Leake, J. R. (1994). The biology of myco-heterotrophic (‘saprophytic’) plants. New Phytol. 127, 171–216. doi: 10.1111/j.1469-8137.1994.tb04272.x
Li, Y. Y., Boeraeve, M., Cho, Y. H., Jacquemyn, H., Lee, Y. I. (2021). Mycorrhizal switching and the role of fungal abundance in seed germination in a fully mycoheterotrophic orchid, Gastrodia confusoides. Front. Plant Sci. 12. doi: 10.3389/fpls.2021.775290
Li, Y. Y., Chen, X. M., Guo, S. X., Lee, Y, I. (2016). Embryology of two mycoheterotrophic orchid species, Gastrodia elata and Gastrodia nantoensis: ovule and embryo development. Botanical Stud. 57, 18. doi: 10.1186/s40529-016-0137-7
Li, Y. Y., Guo, S. X., Lee, Y. I. (2020b). Ultrastructural changes during the symbiotic seed germination of Gastrodia elata with fungi, with emphasis on the fungal colonization region. Bot. Stud. 61, 1–8. doi: 10.1186/s40529-019-0280-z
Li, H. T., Tang, L., Liu, T., Yang, R., Yang, Y., Zhou, H., et al. (2019). Polyoxygenated meroterpenoids and a bioactive illudalane derivative from a co-culture of Armillaria sp. and Epicoccum sp. Org. Chem. Front. 6, 3847–3853. doi: 10.1039/C9QO01087D
Li, H. T., Tang, L. H., Liu, T., Yang, R. N., Yang, Y. B., Zhou, H., et al. (2020a). Protoilludane-type sesquiterpenoids from Armillaria sp. by co-culture with the endophytic fungus Epicoccum sp. associated with Gastrodia elata. Bioorganic Chem. 95, 103503. doi: 10.1016/j.bioorg.2019.103503
Liang, X. X., Pan, Y., Fan, Q. J. (2018). Secondary metabolites of Mycena dendrobii, a fungus stimulating the germination of Gastrodia elata. Chem. Nat. Compd 54, 390–392. doi: 10.1007/s10600-018-2358-3
List of National Key Protected Wild Plants. (2023). List of National Key Protected Wild Plants - Documents of the State Council Department - Chinese government website. Available at: https://www.gov.cn/zhengce/zhengceku/2021-09/09/content_5636409.htm (Accessed November 17, 2023).
Liu, C. Y. (1981). Studies on the morphological changes of intracellular structures of Gastrodia elata Bl. in the period of hyphae digested. J. Integr. Plant Biol. 23, 92–96.
Liu, Y., Gao, J., Peng, M., Meng, H., Ma, H., Cai, P., et al. (2018). A review on central nervous system effects of gastrodin. Front. Pharmacol. 9. doi: 10.3389/fphar.2018.00024
Liu, T., Hua, Z., Han, P., Zhao, Y., Zhou, J., Jin, Y., et al. (2022). Mycorrhizosphere bacteria, Rahnella sp. HPDA25, promotes the growth of Armillaria gallica and its parasitic host Gastrodia elata. Front. Microbiol. 13. doi: 10.3389/fmicb.2022.842893
Liu, C., Talbot, N. J., Chen, X.-L. (2021). Protein glycosylation during infection by plant pathogenic fungi. New Phytol. 230, 1329–1335. doi: 10.1111/nph.17207
Lu, C., Qu, S., Zhong, Z., Luo, H., Lei, S. S., Zhong, H. J., et al. (2022). The effects of bioactive components from the rhizome of Gastrodia elata blume (Tianma) on the characteristics of Parkinson’s disease. Front. Pharmacol. 13. doi: 10.3389/fphar.2022.963327
Manavalan, T., Manavalan, A., Heese, K. (2015). Characterization of lignocellulolytic enzymes from white-rot fungi. Curr. Microbiol. 70, 485–498. doi: 10.1007/s00284-014-0743-0
McKendrick, S. L., Leake, J. R., Taylor, D. L., Read, D. J. (2000). Symbiotic germination and development of myco-heterotrophic plants in nature: ontogeny of Corallorhiza trifida and characterization of its mycorrhizal fungi. New Phytol. 145, 523–537. doi: 10.1046/j.1469-8137.2000.00603.x
Morrison, D. J. (2004). Rhizomorph growth habit, saprophytic ability and virulence of 15 Armillaria species. For. Pathol. 34, 15–26. doi: 10.1046/j.1439-0329.2003.00345.x
Nagel, A. K., Schnabel, G., Petri, C., Scorza, R. (2008). Generation and characterization of transgenic plum linesexpressing the Gastrodia antifungal protein. HortScience 43, 1514–1521. doi: 10.21273/HORTSCI.43.5.1514
Ogura-Tsujita, Y., Gebauer, G., Hashimoto, T., Umata, H., Yukawa, T. (2009). Evidence for novel and specialized mycorrhizal parasitism: the orchid Gastrodia confusa gains carbon from saprotrophic Mycena. Proc. R. Soc B. 276, 761–767. doi: 10.1098/rspb.2008.1225
Pan, Y., Liang, X., Fan, Q. (2015). Study on the primary metabolites of Mycena dendrobii, a fungus stimulating the germination of Gastrodia elata. Sydowia -Horn- 67, 127–132. doi: 10.12905/0380.sydowia67-2015-0127
Park, E. J., Lee, W. Y. (2013a). In vitro symbiotic germination of myco-heterotrophic Gastrodia elata by Mycena species. Plant Biotechnol. Rep. 7, 185–191. doi: 10.1007/s11816-012-0248-x
Park, E. J., Lee, W. Y. (2013b). Quantitative effects of various tree species on tuber growth and pharmacological compositions of Gastrodia elata. Hortic. Environ. Biotechnol. 54, 357–363. doi: 10.1007/s13580-013-0030-1
Park, K. H., Oh, S. Y., Park, M. S., Kim, M.-S., Klopfenstein, N. B., Kim, N. K., et al. (2018). Re-evaluation of Armillaria and Desarmillaria in South Korea based on ITS/tef1 sequences and morphological characteristics. For. Pathol. 48, e12447. doi: 10.1111/efp.12447
Park, J., Suh, Y., Kim, S. (2020). A complete chloroplast genome sequence of Gastrodia elata (Orchidaceae) represents high sequence variation in the species. Mitochondrial DNA Part B 5, 517–519. doi: 10.1080/23802359.2019.1710588
Peterson, R. L., Currah, R. S. (1990). Synthesis of mycorrhizae between protocorms of Goodyera repens (Orchidaceae) and Ceratobasidium cereale. Can. J. Bot. 68, 1117–1125. doi: 10.1139/b90-141
Plant Plus of China. (2023). iPlant- plant species information system. Available at: http://www.iplant.cn/ (Accessed November 17, 2023).
Plants of the World Online. (2023). Plants of the world online. Available at: https://powo.science.kew.org (Accessed November 17, 2023).
Rasmussen, H. N., Rasmussen, F. N. (2009). Orchid mycorrhiza: implications of a mycophagous life style. Oikos 118, 334–345. doi: 10.1111/j.1600-0706.2008.17116.x
Ren, L. Y., Zhao, H., Liu, X. L., Zong, T. K., Qiao, M., Liu, S. Y., et al. (2021). Transcriptome reveals roles of lignin-modifying enzymes and abscisic acid in the symbiosis of Mycena and Gastrodia elata. IJMS 22, 6557. doi: 10.3390/ijms22126557
Rong, L., Cai, C. (2010). Effects of different woods on the yield of Gastrodia elata. J. Wuhan Botanical Res. 28, 761–766. doi: 10.3724/SP.J.1142.2010.40521
Ruiz-Herrera, J., Ortiz-Castellanos, L. (2019). Cell wall glucans of fungi. A review. Cell Surface 5, 100022. doi: 10.1016/j.tcsw.2019.100022
Sekizaki, H., Kuninaga, S., Yamamoto, M., Asazu, S. N., Sawa, S., Kojoma, M., et al. (2008). Identification of Armillaria nabsnona in Gastrodia tubers. Biol. Pharm. Bull. 31, 1410–1414. doi: 10.1248/bpb.31.1410
Shan, T., Yin, M., Wu, J., Yu, H., Liu, M., Xu, R., et al. (2021). Comparative transcriptome analysis of tubers, stems, and flowers of Gastrodia elata Blume reveals potential genes involved in the biosynthesis of phenolics. Fitoterapia 153, 104988. doi: 10.1016/j.fitote.2021.104988
Shi, J., Wang, X., Wang, E. (2023). Mycorrhizal symbiosis in plant growth and stress adaptation: from genes to ecosystems. Annu. Rev. Plant Biol. 74, 569–607. doi: 10.1146/annurev-arplant-061722-090342
Sipos, G., Anderson, J. B., Nagy, L. G. (2018). Armillaria. Curr. Biol. 28, R297–R298. doi: 10.1016/j.cub.2018.01.026
Sipos, G., Prasanna, A. N., Walter, M. C., O’Connor, E., Bálint, B., Krizsán, K., et al. (2017). Genome expansion and lineage-specific genetic innovations in the forest pathogenic fungi Armillaria. Nat. Ecol. Evol. 1, 1931–1941. doi: 10.1038/s41559-017-0347-8
Suetsugu, K., Matsubayashi, J., Tayasu, I. (2020). Some mycoheterotrophic orchids depend on carbon from dead wood: novel evidence from a radiocarbon approach. New Phytol. 227, 1519–1529. doi: 10.1111/nph.16409
Sugiura, N. (2017). Floral morphology and pollination in Gastrodia elata, a mycoheterotrophic orchid. Plant Species Biol. 32, 173–178. doi: 10.1111/1442-1984.12137
Sun, S. Q., Chen, G. H. (2003). The influences of different Armillaria on the yields of Gastrodia and the content of gastrodin. Shandong Sci. 16, 7–10.
Sun, X., Jia, B., Sun, J., Lin, J., Lu, B., Duan, J., et al. (2023). Gastrodia elata Blume: A review of its mechanisms and functions on cardiovascular systems. Fitoterapia 167, 105511. doi: 10.1016/j.fitote.2023.105511
Tsai, C. C., Wu, P. Y., Kuo, C. C., Huang, M. C., Yu, S. K., Hsu, T. W., et al. (2014). Analysis of microsatellites in the vulnerable orchid Gastrodia flavilabella: the development of microsatellite markers, and cross-species amplification in Gastrodia. Botanical Stud. 55, 72. doi: 10.1186/s40529-014-0072-4
Uetake, Y., Farquhar, M. L., Peterson, R. L. (1997). Changes in microtubule arrays in symbiotic orchid protocorms during fungal colonization and senescence. New Phytol. 135, 701–709. doi: 10.1046/j.1469-8137.1997.00686.x
Van Waes, J. M., Debergh, P. C. (1986). In vitro germination of some Western European orchids. Physiologia Plantarum 67, 253–261. doi: 10.1111/j.1399-3054.1986.tb02452.x
Wang, X. C., Bauw, G., Van Damme, E. J. M., Peumans, W. J., Chen, Z.-L., Van Montagu, M., et al. (2001b). Gastrodianin-like mannose-binding proteins: a novel class of plant proteins with antifungal properties. Plant J. 25, 651–661. doi: 10.1046/j.1365-313x.2001.00999.x
Wang, Q. Y., Guo, S. X., Guan, F. B. (2001a). Studies on influences of Armillaria mellea strains from different sources on yield of Gastrodia elata. Chin. Traditional Herbal Drugs 32, 839–841. doi: 10.1037/xan0000099
Wang, Y., Shahid, M. Q., Ghouri, F., Ercişli, S., Baloch, F. S. (2019). Development of EST-based SSR and SNP markers in Gastrodia elata (herbal medicine) by sequencing, de novo assembly and annotation of the transcriptome. 3 Biotech. 9, 292. doi: 10.1007/s13205-019-1823-4
Wang, W., Shi, J., Xie, Q., Jiang, Y., Yu, N., Wang, E. (2017). Nutrient exchange and regulation in arbuscular mycorrhizal symbiosis. Mol. Plant 10, 1147–1158. doi: 10.1016/j.molp.2017.07.012
Wang, H., Wang, Z. Y., Zhang, F. S., Liu, J., He, X. X. (1997). A cytological study on the nutrient-uptake mechanism of a saprophytic orchid Gastrodia elata. Acta Botanica Sin. 39, 500–504+589-590. doi: CNKI:SUN:ZWXB.0.1997-06-002
Wang, H., Xu, J. T. (1993). A cytochemical study of acid phosphatase in the cortical cells of Gastrodia elata infected by Armillaria mellea. Acta Mycolagica Sin. 12, 152–157+177-178. doi: 10.13346/j.mycosystema.1993.02.012
Wang, D. L., Yang, X. Q., Shi, W. Z., Cen, R. H., Yang, Y. B., Ding, Z. T. (2021a). The selective anti-fungal metabolites from Irpex lacteus and applications in the chemical interaction of Gastrodia elata, Armillaria sp., and endophytes. Fitoterapia 155, 105035. doi: 10.1016/j.fitote.2021.105035
Wang, Y., Zhao, L., Li, A. Y. (2021b). Gastrodin - A potential drug used for the treatment of Tourette Syndrome. J. Pharmacol. Sci. 145, 289–295. doi: 10.1016/j.jphs.2021.01.005
Witte, C. P., Rosso, M. G., Romeis, T. (2005). Identification of three urease accessory proteins that are required for urease activation in arabidopsis. Plant Physiol. 139, 1155–1162. doi: 10.1104/pp.105.070292
Wong, J. W. H., Plett, K. L., Natera, S. H. A., Roessner, U., Anderson, I. C., Plett, J. M. (2020). Comparative metabolomics implicates threitol as a fungal signal supporting colonization of Armillaria luteobubalina on eucalypt roots. Plant Cell Environ. 43, 374–386. doi: 10.1111/pce.13672
Xu, J. T. (1990). Cytological observation on hyphae invading Mycena osmundicola in the process of seed germination of Gastrodia elata Bl. Acta Academiae Medicinae Sinicae 12, 313–317.
Xu, J. T. (2001). The changes of cell structure in the courses of Armillaria mellea penetrating the nutritional stems of Gastrodia elata. Acta Academiae Medicinae Sinicae 23, 150–153+205-206.
Xu, J. T. (2013). A review of the 50 years research history of Gastrodia elata cultivation in China. Edible medicinal mushrooms 21, 58–63.
Xu, J. T., Fan, L. (2001). Cytodifferentiation of the seeds (protocorms) and vegetative propagation corms colonized by mycorrhizal fungi. Acta Botanica Sin. 433, 1003–1010.
Xu, J. T., Guo, S. X. (1989). Fungus associated with nutrition of seed germination of Gastrodia elata: Mycena osmundicola Lange. Acta Mycolagica Sin. 8, 221–226+245. doi: 10.13346/j.mycosystema.1989.03.011
Xu, J. T., Guo, S. X. (1991). Nutritional relationship between Gastrodia elata and fungi and its application in cultivation. Bull. Med. Res. 20, 31–32.
Xu, J. T., Guo, S. X. (2000). Retrospect on the research of the cultivation of Gastrodia elata Bl, a rare traditional Chinese medicine. Chin. Med. J. 113, 686–692.
Xu, J. T., Guo, S. X., Fan, L., Na, R. (2001). Symbiotic germination between Gastrodia elata and fungal species of Mycena. Mycosystema 20, 137–141. doi: 10.13346/j.mycosystema.2001.01.027
Xu, Y., Lei, Y., Su, Z., Zhao, M., Zhang, J., Shen, G., et al. (2021). A chromosome-scale Gastrodia elata genome and large-scale comparative genomic analysis indicate convergent evolution by gene loss in mycoheterotrophic and parasitic plants. Plant J. 108, 1609–1623. doi: 10.1111/tpj.15528
Xu, Q., Liu, Y., Wang, X. C., Gu, H. Y., Chen, Z. L. (1998). Purification and characterization of a novel anti-fungal protein from Gastrodia elata. Plant Physiol. Biochem. 36, 899–905. doi: 10.1016/S0981-9428(99)80008-4
Xu, J. T., Mu, C. (1990). The relation between growth of Gastrodia elata protocorms and fungi. J. Integr. Plant Biol. 32, 26–31+93-94.
Xu, J. T., Ran, Y., Guo, S. X. (1989). Studies on the life cycle of Gastrodia elata. Acta Academiae Medicinae Sinicae 11, 237–241.
Xu, J. T., Ran, Y., Guo, S. X. (1990). Studies on nutrition source of seeds germination of Gastrodia elata Bl. Acta Academiae Med Sinicae 12, 431–434+469-470.
Xu, J. T., Ran, Y., Wang, X. W., Lu, J. Z., Wang, M. (1980). Study on the method of sexal reproduction of Gastrodia elata Bl. Acta Pharm. Sin. 15, 100–104+129. doi: 10.16438/j.0513-4870.1980.02.007
Yang, M., Han, N., Li, H., Meng, L. (2018). Transcriptome analysis and microsatellite markers development of a traditional Chinese medicinal herb Halenia elliptica D. Don (Gentianaceae). Evol. Bioinform. Online 14, 1176934318790263. doi: 10.1177/1176934318790263
Yeh, C. M., Chung, K., Liang, C. K., Tsai, W. C. (2019). New insights into the symbiotic relationship between orchids and fungi. Appl. Sci. 9, 585. doi: 10.3390/app9030585
Yu, S. J., Kim, J. R., Lee, C. K., Han, J. E., Lee, J. H., Kim, H.-S., et al. (2005). Gastrodia elata Blume and an active component, p-hydroxybenzyl alcohol reduce focal ischemic brain injury through antioxidant related gene expressions. Biol. Pharm. Bull. 28, 1016–1020. doi: 10.1248/bpb.28.1016
Yuan, Y., Jin, X. H., Liu, J., Zhao, X., Zhou, J. H., Wang, X., et al. (2018). The Gastrodia elata genome provides insights into plant adaptation to heterotrophy. Nat. Commun. 9, 1615. doi: 10.1038/s41467-018-03423-5
Yuan, Q. S., Xu, J., Jiang, W., Ou, X., Wang, H., Guo, L. P., et al. (2020). Insight to shape of soil microbiome during the ternary cropping system of Gastradia elata. BMC Microbiol. 20, 108. doi: 10.1186/s12866-020-01790-y
Zeng, X., Li, Y. Y., Ling, H., Chen, J., Guo, S. X. (2018). Revealing proteins associated with symbiotic germination of Gastrodia elata by proteomic analysis. Bot. Stud. 59, 8. doi: 10.1186/s40529-018-0224-z
Zeng, X., Li, Y., Ling, H., Liu, S., Liu, M., Chen, J., et al. (2017). Transcriptomic analyses reveal clathrin-mediated endocytosis involved in symbiotic seed germination of Gastrodia elata. Botanical Stud. 58, 31. doi: 10.1186/s40529-017-0185-7
Zhan, M., Tian, M., Wang, W., Li, G., Lu, X., Cai, G., et al. (2020). Draft genomic sequence of Armillaria gallica 012m: insights into its symbiotic relationship with Gastrodia elata. Braz. J. Microbiol. 51, 1539–1552. doi: 10.1007/s42770-020-00317-x
Zhang, W. J., Li, B. F. (1980). The biological relationship of Gastrodia elata and Armillaria mellea. Acta Botanica Sin. 22, 57–62.
Zheng, T., Gao, Y. G., Zhang, Z. L., Li, X. Y., Zang, P., Zhao, Y., et al. (2022). A study on the anti-skin tumor and anti-UVB damage effects of Gastrodia elata Bl. Products transformed by Armillaria mellea. Food Agric. Immunol. 33, 736–751. doi: 10.1080/09540105.2022.2120853
Zhou, X., Chen, X. Q. (1983). Overview on Gastradia species in China. Acta Botanica Yunnanica, 361–368.
Zhou, Y. L., Li, M. Y., Lv, T. Y., Huang, M. X., Cheng, B. L., Zhang, Y. Y., et al. (2021a). Gastrodin inhibits virus infection by promoting the production of type I interferon. Front. Pharmacol. 11. doi: 10.3389/fphar.2020.608707
Zhou, Y., Liang, Z. S., Zhang, Y. J., Mu, X. Q. (2005). Study of reproductive biology and artificial pollination of G.elata Bl. J. Northwest Sci-Tech Univ. Agric. Forestry (Natural Sci. Edition) 33, 33–37.
Keywords: Orchidaceae, Gastrodia elata, Mycena, Armillaria, fungi, mutualistic symbiosis
Citation: Liu J-J, Yang X-Q, Li Z-Y, Miao J-Y, Li S-B, Zhang W-P, Lin Y-C and Lin L-B (2024) The role of symbiotic fungi in the life cycle of Gastrodia elata Blume (Orchidaceae): a comprehensive review. Front. Plant Sci. 14:1309038. doi: 10.3389/fpls.2023.1309038
Received: 07 October 2023; Accepted: 13 December 2023;
Published: 08 January 2024.
Edited by:
Stefano Ghignone, National Research Council (CNR), ItalyReviewed by:
Cristiana Sbrana, National Research Council (CNR), ItalyXiaohua Jin, Chinese Academy of Sciences (CAS), China
Yunsheng Wang, Kaili University, China
Copyright © 2024 Liu, Yang, Li, Miao, Li, Zhang, Lin and Lin. This is an open-access article distributed under the terms of the Creative Commons Attribution License (CC BY). The use, distribution or reproduction in other forums is permitted, provided the original author(s) and the copyright owner(s) are credited and that the original publication in this journal is cited, in accordance with accepted academic practice. No use, distribution or reproduction is permitted which does not comply with these terms.
*Correspondence: Wen-Ping Zhang, d3B6aGFuZ0BrdXN0LmVkdS5jbg==; Yi-Cen Lin, eWljZW5saW5Aa3VzdC5lZHUuY24=; Lian-Bing Lin, bGlubGJAa3VzdC5lZHUuY24=